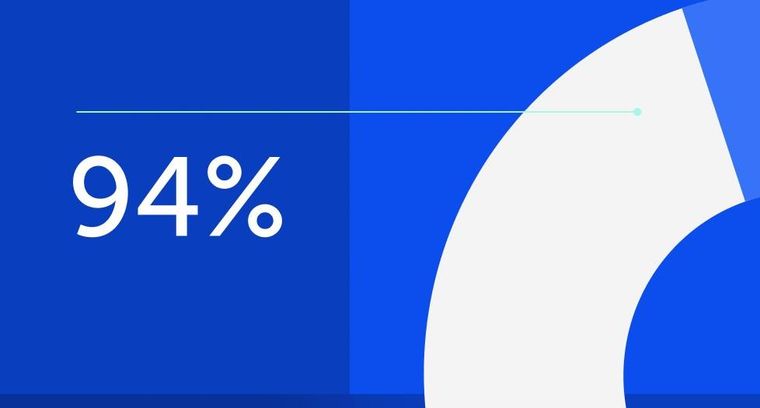
94% of researchers rate our articles as excellent or good
Learn more about the work of our research integrity team to safeguard the quality of each article we publish.
Find out more
ORIGINAL RESEARCH article
Front. Urol., 25 January 2023
Sec. Neurourology, Behavioural Urology, and Urodynamics
Volume 2 - 2022 | https://doi.org/10.3389/fruro.2022.1086179
This article is part of the Research TopicNovel Therapeutic Targets for Lower Urinary Tract SymptomsView all 7 articles
Psychological stress is associated with urinary bladder dysfunction (e.g., increased voiding frequency, urgency and pelvic pain); however, the mechanisms underlying the effects of stress on urinary bladder function are unknown. Transient receptor potential (TRP) channels (vanilloid family) may be potential targets for intervention due to their distribution in the LUT and role in pain. Here, we examine a model of repeated variate stress (RVS) of 2 week (wk) or 4 wk duration in female mice and its effects on bladder function, anxiety-like behavior, and TRPV transcript expression in urinary bladder and lumbosacral spinal cord and associated dorsal root ganglia (DRG). Using continuous infusion, open-outlet cystometry in conscious mice, RVS significantly (p ≤ 0.05) decreased infused volume and intermicturition interval. Bladder pressures (threshold, average, minimum, and maximum pressures) were unchanged with RVS. Quantitative PCR demonstrated significant (p ≤ 0.05) changes in TrpV1 and TrpV4 mRNA expression between control and RVS cohorts in the urothelium, lumbosacral spinal cord, and DRG. Future directions will examine the contribution of TRP channels on bladder function, somatic sensation and anxiety-like behavior following RVS.
Psychological stress is associated with lower urinary tract (LUT) symptom development including increased voiding frequency, urgency and pelvic pain (1–4). Animal models of stress exhibit similar symptoms of bladder dysfunction and anxiety-like behaviors (1) that may be due, in part, to disruption of the hypothalamic-pituitary-adrenal (HPA) axis. Cortisol, by feeding back on the HPA axis, normally attenuates inflammation; however, abnormalities in the feedback loop may cause dysregulation of the inflammatory response. Thus, patients with bladder function disorders may have dysregulation of the HPA axis (5, 6). Although stress is associated with LUT symptom onset and aggravation (2–4, 7–10), the underlying mechanisms that connect stress with voiding dysfunction are unknown.
Researchers use psychological and physical stressor/naturally occurring disease models to study underlying mechanisms and identify potential treatment targets for individuals with stress-induced bladder dysfunction (9). Rodent stress models have been shown to induce pain behaviors along with increased voiding frequency (11–15) and to increase inflammatory cell infiltration, urothelial breakdown, peripheral and central sensitization, and blood flow to brain regions involved with voiding urgency (9, 15). The repeated variate stress (RVS) model has been used to examine stress-induced changes in bladder function and pelvic sensation in rats (13, 14, 16, 17). The RVS paradigm (13, 14, 18–21) has multiple advantages including a lack of habituation with novel stressor exposure and reproducible and robust changes in urinary bladder function. In addition, RVS in rats also produced changes in the inflammatory milieu of the urinary bladder (13, 14).
Multiple transient receptor potential (TRP) channels (TRPA1, TRPV1, TRPV4) have specific tissue distributions in the LUT, are implicated in bladder disorders including overactive bladder (OAB) and IC/BPS and may be sensors of stretch and/or chemical irritation in the LUT (14, 22–27). The potential involvement of TRPV1 and TRPV4 in urinary bladder dysfunction and somatic sensitivity in stress models has been considered in a limited manner in a specific model of social stress in mice (28) or in rats following RVS (13, 14). It is important to understand the tissue expression as well as the mechanisms by which these channels act to identify potential therapeutic targets for stress-induced urinary bladder dysfunction and somatic sensitivity.
The goal of these studies was to evaluate bladder function (i.e., voiding frequency, functional bladder capacity and micturition pressures) in female mice following RVS of 2 week (wk) or 4 wk duration. Although shorter durations (1 wk) of RVS have been used in rats (13, 14) or in a specific transgenic mouse model (16), 1 wk RVS in wildtype (C57BL/6) mice is insufficient to reliably produce robust and reproducible changes in urinary bladder function and somatic sensation (29) or anxiety-like behaviors (S.E. Hammack, personal communication). The current studies compared bladder function using continuous infusion, open-outlet cystometry in conscious female mice after 2 wk or 4 wk RVS. In addition, we examined TrpV1 and TrpV4 mRNA expression using Real-Time Quantitative Reverse Transcription-Polymerase Chain Reaction (Q-PCR) after 2 wk or 4 wk RVS in the following LUT tissues: lumbosacral spinal cord (L1, L2, L5-S1) and associated lumbosacral dorsal root ganglia (DRG) and in the urothelium and detrusor smooth muscle of the urinary bladder. These studies establish the foundation of future studies that will evaluate the role(s) of TRPV1 and TRPV4 in LUT function and somatic sensation following RVS.
Female C57BL/6J wild type (WT) mice used in this study were bred locally at the Larner College of Medicine at the University of Vermont. To maintain diversity in this colony, mice (C57BL/6J) were also purchased from The Jackson Laboratory and incorporated into breeding. The litters were average in size (6-8 pups), weight, and activity (feeding, drinking, behaviors). The UVM Institutional Animal Care and Use Committee approved all experimental protocols involving animal usage (IACUC #X9-020). Animal Care was under the supervision of the UVM Office of Animal Care Management in accordance with the Association for Assessment and Accreditation of Laboratory Animal Care (AAALAC) and National Institutes of Health (NIH) guidelines. We used female mice because of the female predominance of IC/BPS (24, 67, 74). Estrous cycle status was not determined in these studies. All efforts were made to minimize the potential for animal pain, stress, or distress. Separate groups of littermate WT were used in the following experiments. Mice were randomly assigned to control, 2 wk or 4 wk RVS cohorts. Figure 1 is a timeline of experimental procedures and outcome measures described in sections below.
Figure 1 Experimental timeline emphasizing the RVS paradigm with stressors, order of stressor presentation, duration of RVS exposure and experimental outcomes described in methodology section.
Mice assigned to the 2 wk or 4 wk RVS group were exposed daily to stressors with a single stressor being presented on each day between 10 am - 1 pm as described previously (13, 14, 16, 18) (Figure 1). Control mice (no stress) were handled daily and remained in home cages in the animal facility. Oscillation stress: Mice were placed inside a plastic chamber 25 × 16 ×13 cm (L ×W × H) that was secured to a clinical rotator (Fisher Scientific, Morris Plains, NJ), and oscillated at low to medium speed for 30 minutes (min). Forced swim: Mice were placed in a cylindrical container 29 × 37 cm (D × H) that was filled with room temperature water to a depth that prevented the tail from touching the bottom of the container. After 5 min of monitored swimming, mice were placed in a holding chamber for 30 min prior to being returned to their home cage. Electrical foot shock: Mice were placed inside a Plexiglas conditioning chamber (Med Associates, St. Albans, VT) 30 × 25 × 35 cm (L × W × H). After a 5 min acclimation period, two 0.2 mA, 5 second (s) scrambled foot shocks were delivered through the grid floor with a 1 min inter-trial interval. Restraint: Mice were placed in a cylindrical restraining device 30 × 115 mm (D × L) for 60 min. Pedestal: Mice were placed on an elevated (60 cm) platform 20 × 20 cm (L × W) for 30 min. Forced swim and electrical foot shock were the 2 stressors repeated on days (D) 6 and D7. This 7-day RVS protocol was repeated weekly for 2 wk or 4 wk RVS cohorts (Figure 1).
On day 15 or day 29 of the 2 wk or 4 wk RVS protocol (Figure 1), we used open field testing (control, 2 wk and 4 wk RVS mice (N = 4-11) to determine locomotor activity, anxiety-related behavior and exploratory behavior in these cohorts (Figure 1). An individual mouse was placed in a clear Plexiglas, unfamiliar box (open top) with walls 46 cm × 46 cm × 46 cm (L × W × H) to prevent escape and with a center square outlined. The arena was placed on the floor of a quiet laboratory for testing. We quantified the total time an individual mouse spent exploring the center of the square arena (all four paws in the center, ‘time spent in open area’) as opposed to the time spent hugging the walls of the arena over a 20 min period. The arena was sanitized with Peroxigard, rinsed and dried before an individual mouse was evaluated.
On day 15 or day 29 of the 2 wk or 4 wk RVS protocol (Figure 1), respectively, mice in each group (N = 3-5 for each: control, 2 wk, 4 wk) were anesthetized with isoflurane (3-4%) in 100% O2, a lower midline abdominal incision was made, and polyethylene tubing (PE-10, Clay Adams, Parsippany, New Jersey) was inserted into the bladder dome and secured with a nylon purse-string suture (6-zero) (16, 23, 30, 31). The end of the PE tubing was heat flared, but the catheter did not extend into the bladder body or neck, and it was not associated with inflammation or altered cystometric function (16, 23, 30, 31). The distal end of the tubing was sealed, tunneled subcutaneously, and externalized at the back of the neck (16, 23, 30, 31). Abdominal and neck incisions were closed with nylon sutures (6–0). Mice received postoperative analgesics (subcutaneous carprofen, 5.0 mg/kg) at the start of the surgical procedure and were evaluated with conscious cystometry 1-2 hours after tube implant.
For cystometry in conscious mice, an unrestrained animal was placed in a Plexiglas cage with a wire bottom. Before the start of the recording, the bladder was emptied and the catheter was connected via a T-tube to a pressure transducer (Grass Model PT300, West Warwick, RI) and microinjection pump (Harvard Apparatus 22, South Natick, MA). A Small Animal Cystometry Lab Station (MED Associates, Fairfax, VT) was used for urodynamic measurements (16, 23, 30, 31). Saline solution was infused at room temperature into the bladder at a rate of 25 µl/min to elicit repetitive bladder contractions. At least six reproducible micturition cycles were recorded after the initial stabilization period of 25–30 min (16, 23, 30, 31). The following cystometric parameters were recorded in each animal: baseline pressure (pressure at the beginning of the bladder filling), threshold pressure (bladder pressure immediately prior to micturition), peak micturition pressure, intermicturition interval (IMI; time between micturition events), infused volume (IV), and void volume (VV) (16, 23, 30, 31). The IV is the volume required to elicit a micturition event and is also referred to as functional bladder capacity. Mice in these studies had residual volume of less than 10 μl. At the conclusion of the experiment, the mouse was euthanized (5% isoflurane plus thoracotomy). Experiments were conducted at similar times of the day (10 am - 1 pm) to avoid the possibility that circadian variations were responsible for changes in bladder capacity measurements (16, 23, 30, 31). An individual blinded to treatment or group analyzed the cystometric data; groups were decoded after data analysis.
Mice were removed from the study when adverse events occurred that included a significant postoperative event, lethargy, pain, or distress not relieved by our IACUC-approved regimen of postoperative analgesics (16, 23, 30, 31). In addition, behavioral movements such as grooming, standing, walking, and defecation rendered bladder pressure recordings during these events unusable. Approximately 15% of the total mice designated for study were removed from analysis.
On day 14 or 28 of the 2 wk and 4 wk RVS protocol, female (control, 2 wk and 4 wk RVS mice, N = 4-6 each) mice were deeply anesthetized with isoflurane (5%) and then euthanized via thoracotomy (Figure 1). The urinary bladder and lumbosacral (L1, L2, L5-S1) DRG and spinal cord were quickly dissected under RNase-free conditions as previously described (24, 32). The bladder was cut open along the midline and pinned to a Sylgard-coated dish and the urothelium was removed with the aid of fine forceps and a dissecting microscope. All tissues were snap-frozen on dry ice prior to processing as previously described (33). The urothelium + suburothelium was dissected (31, 34) from the detrusor and the specificity of the split bladder preparations was examined for the presence of α-smooth muscle actin (1:1000; Abcam, Cambridge, MA) and uroplakin II (1:25; American Research Products, Belmont, MA) by Western blotting or Q-PCR (24, 34, 35). We determined TrpV1 and TrpV4 transcript expression in the urinary bladder (urothelium + suburothelium, detrusor), lumbosacral (L1, L2, L5-S1) spinal cord and DRG of control, 2 wk and 4 wk RVS mice (N = 4-6 each) using Q-PCR as described (24, 32, 35) (Figure 1).
Extraction of total RNA from samples (N = 4-6) was performed using Stat-60 total RNA/mRNA isolation reagent (Tel-Test B Labs, Friendswood, TX). cDNA was synthesized from 2, 1, 0.5 µg of RNA from urinary bladder, spinal cord and DRG samples, respectively, using a mixture of random hexamers and oligo(dT) primers with M-MLV reverse transcriptase (Promega, Madison, WI). The primers sequences that we developed for TrpV1 and TrpV4 have been previously described (36).
We prepared the quantitative PCR standard for each transcript using the amplified cDNA products ligated directly into the pCR2.1 TOPO vector using the TOPO TA cloning kit (Invitrogen, Carlsbad, CA). To verify the nucleotide sequences of the inserts, we used automated fluorescent dideoxy dye terminator sequencing (Vermont Integrative Genomics Resource). All cDNA templates were diluted 10-fold (to limit the inhibitory effects of the reaction components) and assayed using Luna universal quantitative PCR master mix (New England Biolabs, Ipswich, MA). We performed real-time quantitative PCR (Q-PCR; 7500 Fast real-time PCR system, Applied Biosystems, Foster City, CA) under standard conditions as outlined: 1) serial heating at 94°C for 2 min and 2) amplification over 45 cycles at 94°C for 15 s and 55-60°C for 30 s. The amplified product was exposed to SYBR green I melting analysis by ramping the temperature of the reaction samples from 60°C to 95°C. We ensured the creation of a single DNA melting profile under the outlined dissociation assay conditions to confirm the amplification of a unique, single product that was free of primer dimers and other anomalous products.
A standard curve was constructed to analyze data by amplifying serially diluted plasmids containing the target sequence. Sequence detection software (version 1.3.1, Applied Biosystems, Norwalk, CT) was used at the termination of each assay for data analysis. Default baseline settings were selected in standard assays. The increase in SYBR green I fluorescence intensity (ΔRn) was plotted as a function of cycle number. The sequence detection software determined the threshold cycle as the amplification cycle at which ΔRn first intersects the established baseline. The relative quantity of the gene of interest is normalized to that of the housekeeping gene L32 or 18S for normalized data expression.
Values are presented as means ± S.E.M. P values less than or equal to 0.05 were considered statistically significant. Asterisks (*) indicate statistical differences at the p ≤ 0.05 level. Q-PCR data were compared using one-way analysis of variance (ANOVA) with GraphPad Prism statistical software. Cystometry data were analyzed offline using MED-CMG software (Med Associates). Values of functional bladder parameters were averaged for each mouse and averaged for each condition (control, 2 wk RVS, 4 wk RVS). Cystometry and open-field test results were analyzed using one-way ANOVA with Tukey’s honestly significant difference (HSD) post-hoc analysis with R software.
Using continuous flow, open-outlet cystometry in conscious female mice, 2 wk or 4 wk RVS significantly (p ≤ 0.05) reduced (1.7-fold) the IV (i.e., increased voiding frequency) (Figures 2, 3) and the IMI (1.7-fold) compared to control (handled daily but no RVS) (Figures 2, 3B). No differences were observed in IV or IMI between 2 wk and 4 wk RVS groups.
Figure 2 Representative cystometry traces from conscious mice in control, 2 wk RVS, and 4 wk RVS groups evaluated under continuous infusion and open-outlet conditions. RVS (2 wk or 4 wk) significantly (p ≤ 0.05) increased the voiding frequency (decreased the functional bladder capacity) and decreased the intermicturition interval (e.g., time between voiding events indicated by red line). In this figure, blue arrows represent voiding contractions whereas green asterisks represent non-voiding contractions (i.e., increases in bladder pressure without the release of urine).
Figure 3 RVS (2 wk and 4 wk) significantly (p ≤ 0.05) reduced the infused volume (IV) (A) (functional bladder capacity) and intermicturition interval (IMI) (B) compared to control (handled only) mice. No differences were observed in IV or IMI between 2 wk and 4 wk RVS groups. N = 3-5.
No changes were observed in minimum, maximum, average, or threshold pressures in mice following 2 wk or 4 wk RVS compared to control or between 2 wk and 4 wk mice exposed to RVS as measured with conscious cystometry (Table 1).
Table 1 Bladder pressures of control, 2 wk RVS, and 4 wk RVS mouse groups recorded during conscious cystometry.
Mice exposed to RVS (2 wk or 4 wk) spent significantly (p ≤ 0.05) less time in the open region of the testing arena compared to control (handled but no RVS) mice (Figure 4). No difference in time spent in open field was detected between 2 wk and 4 wk mice exposed to RVS.
Figure 4 Open-field testing demonstrated that RVS (2 wk or 4 wk) mice spent significantly (p ≤ 0.05) less time in the open area compared to control (handled only). No difference was detected in time spent in open field between mice exposed to 2 wk and 4 wk RVS. N = 4-11.
In bladder urothelium, TrpV1 mRNA expression was significantly (p ≤ 0.05) decreased after 4 wk but not 2 wk RVS (Figure 5A). TrpV4 was significantly (p ≤ 0.05) decreased in urothelium after both 2 wk and 4 wk (Figure 5B). No changes in TrpV1 or TrpV4 mRNA expression were observed in detrusor muscle after RVS (2 wk or 4 wk) (Figures 5A, B). In L6 and S1 spinal cord, 4 wk RVS significantly (p ≤ 0.05) increased (2.4-3.4-fold) TrpV1 mRNA expression (Figure 6). Conversely, 4 wk RVS significantly (p ≤ 0.05) increased (1.7-fold) TrpV4 transcript expression only in L6 spinal cord (Figure 7). RVS (4 wk) significantly (p ≤ 0.01) increased TrpV4 transcript in expression in lumbosacral (L6: 4-fold, S1: 1.9-fold) dorsal root ganglia (DRG) (Figure 8). RVS (2 or 4 wk) did not affect TrpV1 expression in lumbosacral (L1, L2, L5, L6, and S1) DRG examined (Figure 9). For Q-PCR data analyses, we have emphasized differences between control (no stress) mice and mice exposed to 2 wk or 4 wk RVS only for clarity of presentation.
Figure 5 TrpV1 (A) and TrpV4 (B) transcript expression in bladder urothelium (uro) and detrusor (det) from control, 2 wk RVS, and 4 wk RVS mouse groups as determined using Q-PCR. Data are expressed as fold change with statistical analyses being performed on the raw data. In bladder urothelium, TrpV1 mRNA expression was significantly (p ≤ 0.05) decreased after 4 wk but not 2 wk RVS (A). TrpV4 was significantly (p ≤ 0.05) decreased in urothelium after both 2 wk and 4 wk (B). No changes in TrpV1 or TrpV4 mRNA expression were observed in detrusor muscle after RVS (2 wk or 4 wk) compared to control. N = 4-6.
Figure 6 TrpV1 transcript expression in lumbosacral (L1, L2, L5-S1) spinal cord from control, 2 wk RVS, and 4 wk RVS mouse groups as determined using Q-PCR. In L6 (D) and S1 (E) spinal cord, 4 wk RVS significantly (p ≤ 0.05) increased (2.4-3.4-fold) TrpV1 mRNA expression. No changes in TrpV1 mRNA expression were observed in L1, L2, or L5 (A–C) spinal cord following 2 or 4 wk RVS compared to control. N = 4-6. Sc, spinal cord.
Figure 7 TrpV4 transcript expression in lumbosacral (L1, L2, L5-S1) spinal cord from control, 2 wk RVS, and 4 wk RVS mouse groups as determined using Q-PCR. In L6 (D) spinal cord, 4 wk RVS significantly (p ≤ 0.05) increased (1.7-fold) TrpV4 mRNA expression. No changes in TrpV4 mRNA expression were observed in L1, L2, L5 (A–C) or S1 (E) spinal cord following 2 or 4 wk RVS compared to control. N = 4-6. Sc, spinal cord.
Figure 8 TrpV4 transcript expression in lumbosacral (L1, L2, L5-S1) dorsal root ganglia (DRG) from control, 2 wk RVS, and 4 wk RVS mouse groups as determined using Q-PCR. RVS (4 wk) significantly (p ≤ 0.01) increased TrpV4 transcript expression in lumbosacral (L6 (D): 4-fold, S1 (E): 1.9-fold) DRG compared to control. No changes in TrpV4 mRNA expression were observed in L1, L2, or L5 (A-C) DRG following 2 or 4 wk RVS compared to control. N = 4-6.
Figure 9 TrpV1 transcript expression in lumbosacral (L1, L2, L5-S1) dorsal root ganglia (DRG) from control, 2 wk RVS, and 4 wk RVS mouse groups as determined using Q-PCR. No changes in TrpV1 mRNA expression were observed in L1, L2, L5 or S1 (A-E) DRG following 2 or 4 wk RVS compared to control. N = 4-6.
Psychological stress is associated with urinary bladder dysfunction; however, the mechanisms underlying these effects of stress are unknown (2–4, 10). We evaluated a RVS paradigm (16, 18, 21) in female mice (2 wk or 4 wk) to model chronic stress and its effects on urinary bladder function. Mice exposed to 2 wk or 4 wk RVS demonstrated a significantly decreased functional bladder capacity (i.e., infused volume) and intermicturition interval along with anxiety-like behavior (i.e., open field testing) (21) compared to control mice that were not exposed to RVS but were handled daily and returned to their home cage.
Although studies with rats (13, 14) and some transgenic mice (16) indicate that 7-day (1 wk) RVS is sufficient to affect urinary bladder function, 1 wk RVS in C57BL/6 (wildtype) mice does not consistently and robustly affect anxiety-like behavior or urinary bladder function (S.E. Hammack, personal communication) (29). In these studies, we demonstrated that 2 wk or 4 wk RVS consistently and robustly altered urinary bladder function. In addition, bladder parameters evaluated in the current study also demonstrated no difference in bladder function outcomes between 2 wk and 4 wk RVS suggesting a saturation effect on bladder function. Future studies will determine if somatic sensitivity (e.g., pelvic and hindpaw) (16) is affected by 2 wk and 4 wk RVS and if changes in somatic sensitivity are also saturated with 2 wk RVS. We will also correlate changes in serum corticosterone (16) in control, 2 wk and 4 wk RVS to to produce additional insight into the saturation effect observed. We have previously performed serum corticosterone determination (16) in control and stress cohorts at the conclusion of the study; however, we aim to use fecal corticosterone measurements to make daily measurements during the RVS paradigm.
Transient receptor potential (TRP) channels (TRPV1, TRPV4) may contribute to bladder disorders including overactive bladder (OAB) and interstitial cystitis/painful bladder syndrome (IC/BPS) as well as stress-induced bladder dysfunction (14, 22–27). Reports suggest that these receptors likely contribute to stretch-induced sensation in the control and pathological bladder (14, 27, 37, 38). The TRPV1 receptor plays a role in pain and TrpV1 mRNA expression increases (30-fold) when transitioning from acute to chronic (persistent) pain (39–41). Blockade of TRPV4 reduced bladder pain induced by cyclophosphamide as measured by mechanical hypersensitivity in the abdomen (22), and improved bladder function in rats following RVS (14). TRPV1 knockout mice exhibited reduced spinal cord signaling and abnormal cystometrogram activity despite unchanged LUT anatomy compared to wild type mice (42). Social stress in mice induces TRPV1-dependent afferent nerve activity that may lead to the development of overactive bladder symptoms (28, 43).
Our Q-PCR data demonstrated decreased TrpV1 mRNA expression in the urothelium and increased TrpV1 mRNA expression in L6-S1 spinal cord levels after 4 wk RVS. TrpV4 mRNA expression decreased in the urothelium after 2 wk and 4 wk RVS and increased in L6 spinal cord and L6-S1 DRG after 4 wk RVS. In contrast, neither TrpV1 nor TrpV4 mRNA were changed in detrusor smooth muscle following 2 or 4 wk RVS. Although there are changes in TrpV1 and TrpV4 mRNA expression in LUT tissues with 2 or 4 wk RVS, the direction of the change varies with LUT tissue examined. Lumbosacral spinal cord and DRG more consistently demonstrated increased TrpV1 and TrpV4 mRNA expression whereas urothelium demonstrated decreased TrpV1 and TrpV4 mRNA expression following RVS. Future studies will examine TRPV1 and TRPV4 protein expression in LUT tissues following RVS. TRPV1 and TRPV4 protein determination will provide insight into the correlation between protein and mRNA expression and whether there is differential regulation of TRPV1 and TRPV4 protein expression among LUT tissues following RVS.
There is a growing body of literature that demonstrates the involvement of TRPV4 in LUT, cell or tissue changes. TRPV4 agonists increased ATP release in cultured urothelial cells (44), whereas TrpV4 deletion or TRPV4 antagonists increased void volumes and IMI, and reduced ATP release (23, 45), suggesting a reduced afferent response (e.g., increased pressure threshold) to bladder filling. LUT TRPV4 expression increases and TRPV4 pharmacological blockade reduces voiding frequency in mice with NGF-overepression in urothelium, CYP-induced cystitis or following RVS in rats (13, 14, 23, 24). The role of TRPV4 in urinary bladder interstitial cells has also been assessed. TRPV4 agonists evoke slow propagating calcium waves in interstitial cells in the upper lamina propria (LP) of the urinary bladder (46). TRPV4 channel activation reduces detrusor contractions and hyperpolarizes PDGFRα+ detrusor interstitial cells via SK3 activation (47). These studies suggest that TRPV4 may mediate sensory signaling in the LP (46, 48) and contribute to regulation of smooth muscle cell excitability in the detrusor (47).
These studies are the foundation for ongoing and future studies addressing underlying mechanisms contributing to stress-induced bladder dysfunction. The goal of these studies was to evaluate bladder function (i.e., voiding frequency, functional bladder capacity and micturition pressures) in female mice following RVS of 2 wk or 4 wk duration. Mice exposed to RVS (2 wk or 4 wk) exhibited decreased infused volume (functional bladder capacity) and intermicturition interval with no changes observed between 2 wk and 4 wk RVS cohorts. In addition, no changes in micturition pressures (minimum, maximum, average, threshold) were observed in mice following either duration of RVS (2 wk and 4 wk). Mice exposed to RVS (2 wk or 4 wk) exhibited increased anxiety-like behavior compared to control mice using open-field testing and no changes were observed between 2 wk and 4 wk RVS. We demonstrated differential changes in TrpV1 and TrpV4 mRNA expression in LUT tissues (urothelium, detrusor, lumbosacral spinal cord and DRG) following 2 wk or 4 wk RVS. We will continue to use the RVS mouse model to determine mechanisms underlying the intersection of chronic stress, micturition and TRPV channels to provide additional insights into stress-induced or stress-exacerbated urinary bladder dysfunction.
The raw data supporting the conclusions of this article will be made available by the authors, without undue reservation.
The animal study was reviewed and approved by University of Vermont IACUC.
MV conceived and designed research. AS, KB, CM, SC, BG and MV performed experiments. AS, BG and MV analyzed data. AS, BG and MV interpreted results of experiments. AS and BG prepared figures. AS drafted the manuscript. BG, AS, and MV edited and revised manuscript. AS, CM, KB, BG, SC and MV approved final version of manuscript.
This work was funded by National Institute of Diabetes and Digestive and Kidney Diseases Grants 5R01DK120108 and 1R01DK124580 to MV.
The authors gratefully acknowledge the additional support provided by the Cell and Molecular Core in the Department of Neurological Sciences at The Larner College of Medicine and Vermont Integrative Genomics Resource. Gratitude is also expressed to Dr. Harrison W. Hsiang for statistical analyses support and helpful discussions. Figures 1, 2 created with BioRender.com under Agreement numbers GE24UVKWRO and PW24UVKK1L.
The authors declare that the research was conducted in the absence of any commercial or financial relationships that could be construed as a potential conflict of interest.
All claims expressed in this article are solely those of the authors and do not necessarily represent those of their affiliated organizations, or those of the publisher, the editors and the reviewers. Any product that may be evaluated in this article, or claim that may be made by its manufacturer, is not guaranteed or endorsed by the publisher.
The National Institutes of Health had no role in the experiments described, including the design, data collection, and analysis of studies performed in the Vizzard laboratory, decision to publish, or preparation of the manuscript. The contents are solely the responsibility of the authors and do not necessarily represent the official views of the National Institutes of Health.
1. Birder L, Andersson KE. Urothelial signaling. Physiol Rev (2013) 93(2):653–80. doi: 10.1152/physrev.00030.2012
2. Rothrock NE, Lutgendorf SK, Kreder KJ, Ratliff T, Zimmerman B. Stress and symptoms in patients with interstitial cystitis: A life stress model. Urology (2001) 57(3):422–7. doi: 10.1016/S0090-4295(00)00988-2
3. Rothrock NE, Lutgendorf SK, Kreder KJ, Ratliff TL, Zimmerman B. Daily stress and symptom exacerbation in interstitial cystitis patients. Urology (2001) 57(6 Suppl 1):122. doi: 10.1016/S0090-4295(01)01075-5
4. Lutgendorf SK, Kreder KJ, Rothrock NE, Ratliff TL, Zimmerman B. Stress and symptomatology in patients with interstitial cystitis: A laboratory stress model. J Urol. (2000) 164(4):1265–9. doi: 10.1016/S0022-5347(05)67152-2
5. Nazif O, Teichman JM, Gebhart GF. Neural upregulation in interstitial cystitis. Urology (2007) 69(4 Suppl):24–33. doi: 10.1016/j.urology.2006.08.1108
6. Westropp JL, Buffington CA. In vivo models of interstitial cystitis. J Urol (2002) 167(2 Pt 1):694–702. doi: 10.1097/00005392-200202000-00068
7. Anger JT, Dallas KB, Bresee C, De Hoedt AM, Barbour KE, Hoggatt KJ, et al. National prevalence of IC/BPS in women and men utilizing veterans health administration data. Front Pain Res (Lausanne). (2022) 3:925834. doi: 10.3389/fpain.2022.925834
8. Doggweiler R, Bschleipfer T. [Physical and psychological comorbidities of interstitial cystitis/bladder pain syndrome]. Aktuelle Urol. (2021) 52(6):569–74.
9. Birder L, Andersson KE. Animal modelling of interstitial Cystitis/Bladder pain syndrome. Int Neurourol J (2018) 22(Suppl 1):S3–9. doi: 10.5213/inj.1835062.531
10. Spanos C, Pang X, Ligris K, Letourneau R, Alferes L, Alexacos N, et al. Stress-induced bladder mast cell activation: Implications for interstitial cystitis. J urol (1997) 157(2):669–72. doi: 10.1097/00005392-199702000-00085
11. Smith AL, Leung J, Kun S, Zhang R, Karagiannides I, Raz S, et al. The effects of acute and chronic psychological stress on bladder function in a rodent model. Urology (2011) 78(4):967 e1–7. doi: 10.1016/j.urology.2011.06.041
12. Chang A, Butler S, Sliwoski J, Valentino R, Canning D, Zderic S. Social stress in mice induces voiding dysfunction and bladder wall remodeling. Am J Physiol Renal Physiol (2009) 297(4):F1101–8. doi: 10.1152/ajprenal.90749.2008
13. Merrill L, Malley S, Vizzard MA. Repeated variate stress in male rats induces increased voiding frequency, somatic sensitivity, and urinary bladder nerve growth factor expression. Am J Physiol Regul Integr Comp Physiol (2013) 305(2):R147–56. doi: 10.1152/ajpregu.00089.2013
14. Merrill L, Vizzard MA. Intravesical TRPV4 blockade reduces repeated variate stress-induced bladder dysfunction by increasing bladder capacity and decreasing voiding frequency in male rats. Am J Physiol Regul Integr Comp Physiol (2014) 307(4):R471–80. doi: 10.1152/ajpregu.00008.2014
15. Wang Z, Chang HH, Gao Y, Zhang R, Guo Y, Holschneider DP, et al. Effects of water avoidance stress on peripheral and central responses during bladder filling in the rat: A multidisciplinary approach to the study of urologic chronic pelvic pain syndrome (MAPP) research network study. PloS One (2017) 12(9):e0182976. doi: 10.1371/journal.pone.0182976
16. Girard BM, Campbell SE, Beca KI, Perkins M, Hsiang H, May V, et al. Intrabladder PAC1 receptor antagonist, PACAP(6-38), reduces urinary bladder frequency and pelvic sensitivity in mice exposed to repeated variate stress (RVS). J Mol Neurosci (2021) 71(8):1575–88. doi: 10.1007/s12031-020-01649-x
17. May V, Mathews ME, Torres NS, McQuesten J, Chang PL, Hauke E, et al. Role of PACAP/PAC1 signaling in micturition reflexes and somatic sensitivity following repeated variate stress (RVS) in PACAP promoter-dependent EGFP BAC transgenic mice. 12th Int Symposium VIP PACAP Related Peptdies (2017).
18. Hammack SE, Cheung J, Rhodes KM, Schutz KC, Falls WA, Braas KM, et al. Chronic stress increases pituitary adenylate cyclase-activating peptide (PACAP) and brain-derived neurotrophic factor (BDNF) mRNA expression in the bed nucleus of the stria terminalis (BNST): Roles for PACAP in anxiety-like behavior. Psychoneuroendocrinology (2009) 34(6):833–43. doi: 10.1016/j.psyneuen.2008.12.013
19. King SB, Toufexis DJ, Hammack SE. Pituitary adenylate cyclase activating polypeptide (PACAP), stress, and sex hormones. Stress (2017) 20(5):465–75. doi: 10.1080/10253890.2017.1336535
20. Lezak KR, Roman CW, Braas KM, Schutz KC, Falls WA, Schulkin J, et al. Regulation of bed nucleus of the stria terminalis PACAP expression by stress and corticosterone. J Mol Neurosci MN. (2014) 54(3):477–84. doi: 10.1007/s12031-014-0269-8
21. Roman CW, Lezak KR, Hartsock MJ, Falls WA, Braas KM, Howard AB, et al. PAC1 receptor antagonism in the bed nucleus of the stria terminalis (BNST) attenuates the endocrine and behavioral consequences of chronic stress. Psychoneuroendocrinology (2014) 47:151–65. doi: 10.1016/j.psyneuen.2014.05.014
22. Kawasaki S, Soga M, Sakurai Y, Nanchi I, Yamamoto M, Imai S, et al. Selective blockade of transient receptor potential vanilloid 4 reduces cyclophosphamide-induced bladder pain in mice. Eur J Pharmacol (2021) 899:174040. doi: 10.1016/j.ejphar.2021.174040
23. Girard BM, Campbell SE, Perkins M, Hsiang H, Tooke K, Drescher C, et al. TRPV4 blockade reduces voiding frequency, ATP release, and pelvic sensitivity in mice with chronic urothelial overexpression of NGF. Am J Physiol Renal Physiol (2019) 317(6):F1695–F706. doi: 10.1152/ajprenal.00147.2019
24. Girard BM, Merrill L, Malley S, Vizzard MA. Increased TRPV4 expression in urinary bladder and lumbosacral dorsal root ganglia in mice with chronic overexpression of NGF in urothelium. J Mol Neurosci (2013) 51(2):602–14. doi: 10.1007/s12031-013-0033-5
25. Gonzalez EJ, Merrill L, Vizzard MA. Bladder sensory physiology: Neuroactive compounds and receptors, sensory transducers, and target-derived growth factors as targets to improve function. Am J Physiol Regulat Integr Comp Physiol (2014) 306(12):R869–78. doi: 10.1152/ajpregu.00030.2014
26. Merrill L, Gonzalez EJ, Girard BM, Vizzard MA. Receptors, channels, and signalling in the urothelial sensory system in the bladder. Nat Rev Urology. (2016) 13(4):193–204. doi: 10.1038/nrurol.2016.13
27. Andersson KE, Gratzke C, Hedlund P. The role of the transient receptor potential (TRP) superfamily of cation-selective channels in the management of the overactive bladder. BJU Int (2010) 106(8):1114–27. doi: 10.1111/j.1464-410X.2010.09650.x
28. Mingin GC, Heppner TJ, Tykocki NR, Erickson CS, Vizzard MA, Nelson MT. Social stress in mice induces urinary bladder overactivity and increases TRPV1 channel-dependent afferent nerve activity. Am J Physiol Regulat Integr Comp Physiol (2015) 309(6):R629–38. doi: 10.1152/ajpregu.00013.2015
29. Girard BM, Campbell SE, Vizzard MA. Stress-induced symptom exacerbation: Stress increases voiding frequency, somatic sensitivity, and urinary bladder NGF and BDNF expression in mice with subthreshold cyclophosphamide (CYP). Front Urol (2022).
30. Gonzalez EJ, Girard BM, Vizzard MA. Expression and function of transforming growth factor-beta isoforms and cognate receptors in the rat urinary bladder following cyclophosphamide-induced cystitis. Am J Physiol Renal Physiol (2013) 305(9):F1265–76. doi: 10.1152/ajprenal.00042.2013
31. Schnegelsberg B, Sun TT, Cain G, Bhattacharya A, Nunn PA, Ford AP, et al. Overexpression of NGF in mouse urothelium leads to neuronal hyperinnervation, pelvic sensitivity, and changes in urinary bladder function. Am J Physiol Regulat Integr Comp Physiol (2010) 298(3):R534–47. doi: 10.1152/ajpregu.00367.2009
32. Girard BM, Malley SE, Braas KM, May V, Vizzard MA. PACAP/VIP and receptor characterization in micturition pathways in mice with overexpression of NGF in urothelium. J Mol Neurosci MN. (2010) 42(3):378–89. doi: 10.1007/s12031-010-9384-3
33. Arms L, Girard BM, Vizzard MA. Expression and function of CXCL12/CXCR4 in rat urinary bladder with cyclophosphamide-induced cystitis. Am J Physiol Renal Physiol (2010) 298(3):F589–600. doi: 10.1152/ajprenal.00628.2009
34. Corrow K, Girard BM, Vizzard MA. Expression and response of acid-sensing ion channels in urinary bladder to cyclophosphamide-induced cystitis. Am J Physiol Renal Physiol (2010) 298(5):F1130–9. doi: 10.1152/ajprenal.00618.2009
35. Girard BM, Malley SE, Vizzard MA. Neurotrophin/receptor expression in urinary bladder of mice with overexpression of NGF in urothelium. Am J Physiol Renal Physiol (2011) 300(2):F345–55. doi: 10.1152/ajprenal.00515.2010
36. Beca KIK, Girard BM, Heppner TJ, Hennig GW, Herrera GM, Nelson MT, et al. The role of PIEZO1 in urinary bladder function and dysfunction in a rodent model of cyclophosphamide-induced cystitis. Front Pain Res (Lausanne). (2021) 2:748385. doi: 10.3389/fpain.2021.748385
37. Andersson KE. TRP channels as lower urinary tract sensory targets. Med Sci (Basel) (2019) 7(5):67. doi: 10.3390/medsci7050067
38. Andersson KE, Birder L. Current pharmacologic approaches in painful bladder research: An update. Int neurourol J (2017) 21(4):235–42. doi: 10.5213/inj.1735022.511
39. Silva M, Martins D, Charrua A, Piscitelli F, Tavares I, Morgado C, et al. Endovanilloid control of pain modulation by the rostroventromedial medulla in an animal model of diabetic neuropathy. Neuropharmacology (2016) 107:49–57. doi: 10.1016/j.neuropharm.2016.03.007
40. Martins D, Tavares I, Morgado C. "Hotheaded": The role OF TRPV1 in brain functions. Neuropharmacology (2014) 85:151–7.
41. Martins D, Silva M, Tavares I. TRPV1 in pain control from the brain. Oncotarget (2017) 8(10):16101–2. doi: 10.18632/oncotarget.13316
42. Birder LA, Nakamura Y, Kiss S, Nealen ML, Barrick S, Kanai AJ, et al. Altered urinary bladder function in mice lacking the vanilloid receptor TRPV1. Nat Neurosci (2002) 5(9):856–60. doi: 10.1038/nn902
43. Mingin GC, Peterson A, Erickson CS, Nelson MT, Vizzard MA. Social stress induces changes in urinary bladder function, bladder NGF content, and generalized bladder inflammation in mice. Am J Physiol Regulat Integr Comp Physiol (2014) 307(7):R893–900. doi: 10.1152/ajpregu.00500.2013
44. Mochizuki T, Sokabe T, Araki I, Fujishita K, Shibasaki K, Uchida K, et al. The TRPV4 cation channel mediates stretch-evoked Ca2+ influx and ATP release in primary urothelial cell cultures. J Biol Chem (2009) 284(32):21257–64. doi: 10.1074/jbc.M109.020206
45. Gevaert T, Vriens J, Segal A, Everaerts W, Roskams T, Talavera K, et al. Deletion of the transient receptor potential cation channel TRPV4 impairs murine bladder voiding. J Clin Invest. (2007) 117(11):3453–62. doi: 10.1172/JCI31766
46. Heppner TJ, Hennig GW, Nelson MT, Vizzard MA. Rhythmic calcium events in the lamina propria network of the urinary bladder of rat pups. Front Syst Neurosci (2017) 11:87. doi: 10.3389/fnsys.2017.00087
47. Lee H, Koh BH, Peri LE, Corrigan RD, Lee HT, George NE, et al. Premature contractions of the bladder are suppressed by interactions between TRPV4 and SK3 channels in murine detrusor PDGFRalpha(+) cells. Sci Rep (2017) 7(1):12245.
Keywords: lumbosacral spinal cord, dorsal root ganglia, urinary bladder, urothelium, cystometry, TrpV1, TrpV4, repeated variate stress
Citation: Sidwell AB, McClintock C, Beča KI, Campbell SE, Girard BM and Vizzard MA (2023) Repeated variate stress increased voiding frequency and altered TrpV1 and TrpV4 transcript expression in lower urinary tract (LUT) pathways in female mice. Front. Urol. 2:1086179. doi: 10.3389/fruro.2022.1086179
Received: 01 November 2022; Accepted: 28 December 2022;
Published: 25 January 2023.
Edited by:
Naoki Yoshimura, University of Pittsburgh, United StatesReviewed by:
Shaoping Hou, Drexel University, United StatesCopyright © 2023 Sidwell, McClintock, Beča, Campbell, Girard and Vizzard. This is an open-access article distributed under the terms of the Creative Commons Attribution License (CC BY). The use, distribution or reproduction in other forums is permitted, provided the original author(s) and the copyright owner(s) are credited and that the original publication in this journal is cited, in accordance with accepted academic practice. No use, distribution or reproduction is permitted which does not comply with these terms.
*Correspondence: Margaret A. Vizzard, Margaret.Vizzard@med.uvm.edu
Disclaimer: All claims expressed in this article are solely those of the authors and do not necessarily represent those of their affiliated organizations, or those of the publisher, the editors and the reviewers. Any product that may be evaluated in this article or claim that may be made by its manufacturer is not guaranteed or endorsed by the publisher.
Research integrity at Frontiers
Learn more about the work of our research integrity team to safeguard the quality of each article we publish.