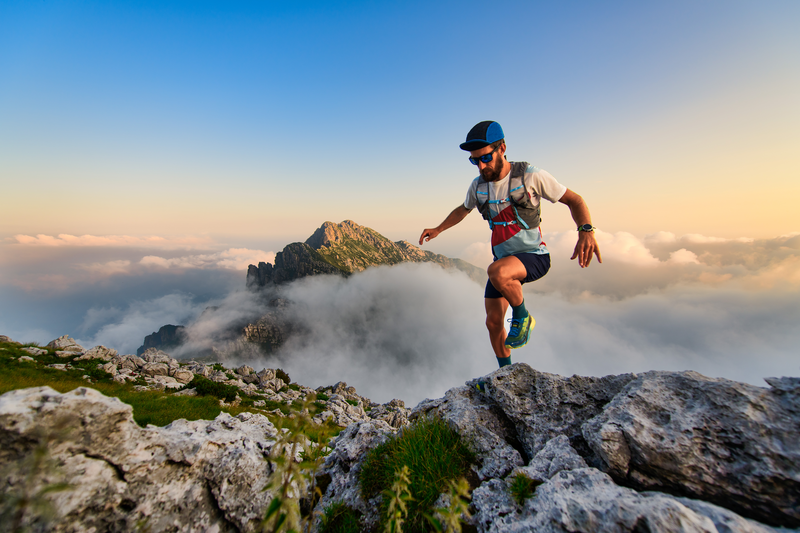
95% of researchers rate our articles as excellent or good
Learn more about the work of our research integrity team to safeguard the quality of each article we publish.
Find out more
ORIGINAL RESEARCH article
Front. Urol. , 22 March 2023
Sec. Neurourology, Behavioural Urology, and Urodynamics
Volume 3 - 2023 | https://doi.org/10.3389/fruro.2023.1079790
This article is part of the Research Topic Novel Therapeutic Targets for Lower Urinary Tract Symptoms View all 7 articles
Symptom exacerbation due to stress is prevalent in many disease states, including functional disorders of the urinary bladder (e.g., overactive bladder (OAB), interstitial cystitis/bladder pain syndrome (IC/BPS)); however, the mechanisms underlying the effects of stress on micturition reflex function are unclear. In this study we designed and evaluated a stress-induced symptom exacerbation (SISE) mouse model that demonstrates increased urinary frequency and somatic (pelvic and hindpaw) sensitivity. Cyclophosphamide (CYP) (35 mg/kg; i.p., every 48 hours for a total of 4 doses) or 7 days of repeated variate stress (RVS) did not alter urinary bladder function or somatic sensitivity; however, both CYP alone and RVS alone significantly (p ≤ 0.01) decreased weight gain and increased serum corticosterone. CYP treatment when combined with RVS for 7 days (CYP+RVS) significantly (p ≤ 0.01) increased serum corticosterone, urinary frequency and somatic sensitivity and decreased weight gain. CYP+RVS exposure in mice significantly (p ≤ 0.01) increased (2.6-fold) voiding frequency as we determined using conscious, open-outlet cystometry. CYP+RVS significantly (p ≤ 0.05) increased baseline, threshold, and peak micturition pressures. We also evaluated the expression of NGF, BDNF, CXC chemokines and IL-6 in urinary bladder in CYP alone, RVS alone and CYP+RVS mouse cohorts. Although all treatments or exposures increased urinary bladder NGF, BDNF, CXC and IL-6 content, CYP+RVS produced the largest increase in all inflammatory mediators examined. These results demonstrated that CYP alone or RVS alone creates a change in the inflammatory environment of the urinary bladder but does not result in a change in bladder function or somatic sensitivity until CYP is combined with RVS (CYP+RVS). The SISE model of CYP+RVS will be useful to develop testable hypotheses addressing underlying mechanisms where psychological stress exacerbates symptoms in functional bladder disorders leading to identification of targets and potential treatments.
Animal models are used to mirror interstitial cystitis/bladder pain syndrome (IC/BPS) pathophysiology to increase our understanding of the underlying mechanisms and identify potential targets for treatment interventions. Patients with lower urinary tract (LUT) disorders report worse symptoms during stress (1, 2). Stress may contribute to the exacerbation and likely the development of LUT disorders including IC/BPS and overactive bladder (OAB) (3–6). A majority of IC/BPS patients report exacerbation of symptoms by clinical stress (6–8), and experimental stress increases bladder pain and urgency (9). Individuals with urologic chronic pelvic pain syndrome (UCPPS) exhibit greater lifetime stress and widespread pain symptoms and less resiliency and coping mechanisms (10).
Animal models of stress demonstrate symptoms of bladder dysfunction (e.g., increased micturition frequency, pain) as well as anxiety-like behavior (3) that may be due, in part, to dysregulation of the hypothalamic-pituitary-adrenal (HPA) axis. Individuals with bladder dysfunction disorders may have abnormalities in the HPA axis, and stress may contribute to the increase in bladder symptoms (1, 2). Although stress may exacerbate LUT symptoms, the pathophysiology underlying the development and progression of stress on urinary frequency and pelvic pain remains unknown.
Symptom exacerbation, often referred to as flares, among patients with IC/BPS is well recognized and attributed to multiple triggers, including infection, diet, physical activities, sexual activities, allergies, and stress (6, 11–13). The proportion (15-95%) of IC/BPS patients with flares varies widely (6, 11–13). Although flares vary in terms of duration, frequency, intensity, and type of symptoms, flares are bothersome, disruptive, and associated with increased pelvic pain and urologic symptoms (6, 11–13). Few studies have addressed symptom flare in IC/BPS patients and basic research investigations of flares are hampered by the lack of animal models that mimic symptom flare upon which one can then develop testable hypotheses (6, 11–13).
In this study, we describe the initial characterization a mouse model where administration of cyclophosphamide (CYP) (35 mg/kg; i.p., every 48 hours for a total of 4 doses) when combined with 7 days of stressor presentation results in increased voiding frequency and increased somatic sensitivity. Neither the administration of CYP or the 7 days of repeated variate stress (RVS) (14–17) alone increased voiding frequency or somatic sensitivity in C57Bl/6 mice. We refer to this mouse model as a stress-induced symptom exacerbation (SISE) mouse model. In this paper, we describe our characterization of the SISE model including, bladder function with conscious, open outlet continuous cystometry and somatic sensitivity with von Frey filament testing. We have also examined corticosterone expression, and expression of inflammatory mediators including neurotrophic factor (NGF, BDNF) (18–20), chemokine (CXC) and interleukin-6 (IL-6) (18, 21–34) expression in the urinary bladder. These initial results demonstrated that CYP alone, or RVS alone creates a change in the inflammatory environment of the urinary bladder and a systemic increase in corticosterone expression but does not result in a change in bladder function or somatic sensitivity until CYP is combined with RVS (CYP+RVS).
Female C57BL/6 wildtype (WT) mice used in this study were bred locally at the Larner College of Medicine at the University of Vermont. To maintain diversity in this colony, mice (C57BL/6J) were also purchased from The Jackson Laboratory and incorporated into breeding. The litters were average in size (6-8 pups), weight, and activity (feeding, drinking, behaviors) with consistency in the overall proportion of male and female mice. The UVM Institutional Animal Care and Use Committee approved all experimental protocols involving animal usage (IACUC #X9-020). Animal Care was under the supervision of the UVM Office of Animal Care Management in accordance with the Association for Assessment and Accreditation of Laboratory Animal Care (AAALAC) and National Institutes of Health (NIH) guidelines. We used female mice because of the female predominance of IC/BPS (24, 35, 36). Estrous cycle status was not determined for mice in these studies. All efforts were made to minimize the potential for animal pain, stress, or distress. Separate groups of littermate WT were used in the following experiments. Mice were randomly assigned to control, CYP, RVS or CYP+RVS cohorts (N = 8) after weaning and weighed daily (Figure 1). Cohorts consisted of mice from 3-5 litters.
Figure 1 Experimental cohorts and experimental outcomes. Overview of studies using control (C57Bl/6) mice (handled and returned to home cage), mice treated with cyclophosphamide (CYP) alone, mice exposed to repeated variate stress (RVS) alone, or mice exposed to both CYP + RVS. Primary outcome measures measured in this study: urinary bladder function and referred somatic sensitivity of the pelvic region and hindpaw. Tissues were also harvested for current and future studies. The RVS protocol (7 day) consisted of 5 different stressors with a novel stressor delivered each day (see methods for additional details including duration of stressors) (14, 37–39). Swim and foot shock stressors are repeated on the last two days of RVS. sec, seconds; DRG, dorsal root ganglia.
Mice (N = 8, per treatment group) received cyclophosphamide (CYP) (35 mg/kg every 48 hours for a total of 4 injections) intraperitoneally (i.p.) to create a mouse model that did not exhibit changes in bladder or somatic function in the absence of RVS (Figure 1) (Table 1). CYP is metabolized to acrolein, an irritant expelled in the urine (40–42). Injections were performed in mice anesthetized with isoflurane (3%). The control group received no CYP treatment. CYP alone and CYP+RVS groups received the same dose and frequency of administration described here.
Mice assigned to the RVS group (hereafter RVS mice) or CYP+RVS (hereafter CYP+RVS mice) group were exposed to 7 days of stressors with a single stressor being presented on each day between 9 am-12 pm as described previously (14, 15, 37, 38) (Figure 1). Control mice (no stress or CYP) were handled daily and remained in home cages in the animal facility following weighing. Oscillation stress: Mice were placed inside a plastic chamber 25 × 16 ×13 cm (L ×W × H), that was secured to a clinical rotator (Fisher Scientific, Morris Plains, NJ), and oscillated at low to medium speed for 30 minutes (min). Forced swim: Mice were placed in a cylindrical container 29 × 37 cm (D × H) that was filled with room temperature water to a depth that prevented the tail from touching the bottom of the container. After 5 min of monitored swimming, mice were placed in a holding chamber for 30 min prior to being returned to their home cage. Electrical foot shock: Mice were placed inside a Plexiglass conditioning chamber (Med Associates, St. Albans, VT) 30 × 25 × 35 cm (L × W × H). After a 5 min acclimation period, two 0.2 mA, 5 second (s) scrambled foot shocks were delivered through the grid floor with a 1 min inter-trial interval. Restraint: Mice were placed in a cylindrical restraining device 30 × 115 mm (D × L) for 60 min. Pedestal: Mice were placed on an elevated (60 cm) platform 20 × 20 cm (L × W) for 30 min. Forced swim and electrical foot shock were the 2 stressors repeated on days (D) 6 and D7 (Table 1; Figure 1).
Mice (N = 8, per treatment group) received cyclophosphamide (CYP) (35 mg/kg every 48 hours for a total of 4 injections) (Day(D) 1, D3, D5 and D7) (Figure 1, Table 1) intraperitoneally (i.p.) to create a mouse model that did not exhibit changes in bladder or somatic function in the absence of RVS. The CYP dosing schedule (Table 1) was combined with daily (D1-D7) exposure to various stressors (Figure 1) described above. In pilot studies, we evaluated different doses of CYP (15, 25, 30, 35 mg/kg) at the same dosing frequency that when combined with RVS would result in increased voiding frequency. Neither the frequency of CYP dosing nor the duration of the RVS protocol was altered. Intraperitoneal injection of CYP (35 mg/kg; every 48 hours for a total of 4 doses) combined with 7-day RVS in female mice resulted in the most consistent increases in voiding frequency.
On D7, mice in all treatment groups (N = 8 for each: control, CYP, RVS, CYP+RVS) were anesthetized with isoflurane (3-4%) in 100% O2, a lower midline abdominal incision was made, and polyethylene tubing (PE-10, Clay Adams, Parsippany, New Jersey) was inserted into the bladder dome and secured with a nylon purse-string suture (6-zero) (14, 43–45). The end of the PE tubing was heat flared, but the catheter did not extend into the bladder body or neck, and it was not associated with inflammation or altered cystometric function (14, 43–45). The distal end of the tubing was sealed, tunneled subcutaneously, and externalized at the back of the neck (14, 43–45). Abdominal and neck incisions were closed with nylon sutures (6–0). Mice received postoperative analgesics (subcutaneous carprofen, 5.0 mg/kg, once a day for two days) and recovered from survival surgery for 48 hr before performing cystometry. Mice were returned to home cages and no stressor or CYP administration occurred for the remaining 2 days of the protocol (Table 1).
For cystometry, a conscious, unrestrained mouse was placed in a Plexiglas cage with a wire bottom. Prior to the start of recording, the bladder was emptied, and the catheter was connected via a T-tube to a pressure transducer (Grass Model PT300, West Warwick, RI) and microinjection pump (Harvard Apparatus 22, South Natick, MA). A Small Animal Cystometry Lab Station (MED Associates, Fairfax, VT) was used for urodynamic measurements (14, 43–45). Saline solution was infused at room temperature into the bladder at a rate of 25 µl/min to elicit repetitive bladder contractions. At least six reproducible micturition cycles were recorded after the initial stabilization period of 25–30 min (14, 43–45). The following cystometric parameters were recorded in each animal: baseline pressure (pressure at the beginning of the bladder filling), threshold pressure (bladder pressure immediately prior to micturition), peak micturition pressure, intercontraction interval (ICI; time between micturition events), infused volume (IV), void volume (VV) (14, 43–45). Mice in these studies had residual volume of less than 10 μl. At the conclusion of the experiment, the mouse was euthanized (5% isoflurane plus thoracotomy). Experiments were conducted at similar times of the day (9 am -12 pm) to avoid the possibility that circadian variations were responsible for changes in bladder capacity measurements (14, 43–45). An individual blinded to treatment or group analyzed the cystometric data; groups were decoded after data analysis.
Mice were removed from the study when adverse events occurred that included a significant postoperative event, lethargy, pain, or distress not relieved by our IACUC-approved regimen of postoperative analgesics (14, 43–45). In addition, behavioral movements such as grooming, standing, walking, and defecation rendered bladder pressure recordings during these events unusable. Approximately 10% of the total number of mice dedicated to these studies were removed from the study.
Referred (secondary) hyperalgesia was measured by testing the frequency of withdrawal responses to the application of calibrated von Frey monofilaments to the abdominal (14, 43–45) region overlying the urinary bladder + suprapubic region and the hindpaw. Mice were returned to home cages, weighed daily, but no stressor or CYP administration occurred for the remaining 2 days of the protocol (Table 1) before somatic sensitivity testing. Separate groups (N = 10 each) of mice were evaluated: control, CYP, RVS, CYP+RVS. Mechanical sensitivity assessment was performed using von Frey monofilaments (Stoelting, Wood Dale, IL) with forces of 0.1–4 g applied to the pelvic region (14, 43–45). Mechanical sensitivity was then evaluated in the hindpaw in an identical manner as previously described (14, 43–45). Testing of the plantar region of the hindpaw and lower abdominal area was performed by perpendicular application of von Frey filaments until the hair bent slightly. All mice were first habituated in a clear acrylic testing chamber for 20 min/day for 4 days prior to the initiation of the CYP, RVS or CYP+RVS treatment or handling (control) with a fan to generate ambient noise. On day of testing (D9) (Table 1), the mice were placed in the acrylic testing chamber on top of a metal mesh floor (IITC Life Science Inc., Woodland Hills, CA) and habituated again for 10 min before the application of von Frey filaments in an up-down method for 1–3 s with a minimum interstimulus interval of 2 min (14, 43–45). The following behaviors were considered positive responses to pelvic region stimulation: sharp retraction of the abdomen, jumping, or immediate licking or scratching of the pelvic area (14, 43–45). A positive response to hindpaw stimulation was sharp withdrawal of the paw or licking of the tested hindpaw (14, 43–46). All somatic testing was performed in a blinded manner. The groups were decoded after data analysis. Separate cohorts of mice were used for cystometry and somatic sensitivity testing to avoid any potential confounding events of an abdominal incision.
Female mice were deeply anesthetized with isoflurane (5%) in 100% O2, blood from the abdominal aorta was collected (see below), the urinary bladder dissected and then mice were euthanized via thoracotomy. The urinary bladder was quickly snap-frozen on dry ice prior to processing (47). In addition, urinary bladders from some mice were used to determine if the lower concentration (35 mg/kg) of CYP produced inflammatory changes in the urinary bladder. Urinary bladders were harvested, cut along the midline, opened and pinned to Sylgard-coated plates and photographed. The pinned bladders were then prepared for tissue sectioning (15 µm), H+E staining and brightfield microscopy (Olympus BX40) as previously described (20, 41).
We determined serum corticosterone from control, CYP, RVS and CYP+RVS mice (N = 8 each) using ELISAs (ADI‐900‐097, Enzo Life Sciences, Farmingdale, NY) (14). At time of euthanasia on D9 (Table 1), blood from the abdominal aorta was collected in serum tubes between 9 am-12 pm and allowed to coagulate at room temperature for 1 hr. Samples were centrifuged in an Eppendorf 5415R centrifuge at 10,000 rpm for 10 min. Serum was aliquoted and frozen at −20 °C until analysis. We determined NGF and BDNF, IL-6 and CXC (CXCL9, CXCL10) protein content in the urinary bladder of control, CYP, RVS and CYP+RVS treatment groups (N=8) using ELISAs (Promega, Madison, WI and R&D Systems, Minneapolis, MN) as previously described (18, 19, 31, 32, 48). According to the manufacturer, the NGF E-max or BDNF E-max immunoassay systems (Promega, Madison, WI) demonstrate very low cross-reactivity with structurally related growth factors. We have recently (20) determined urinary bladder NGF expression under various experimental conditions and compared results obtained with Promega and Biosensis (BioSensis, Thebarton SA, Australia) kits and found no statistical differences between results. According to the manufacturer, no significant cross-reactivity or interference is observed with other cytokines or chemokines with any Quantikine M immunoassay kit (R&D Systems, Minneapolis, MN) used in the present study for IL-6 or CXC detection. The standards provided with these systems generated linear standard curves (R2 = 0.996–0.998, p ≤ 0.001). Absorbance values of standards and samples were corrected by subtraction of the background value (absorbance due to nonspecific binding). No samples were diluted, and all samples had absorbance values on the linear portion of the standard curve. Curve fitting of standards and evaluation of NGF, BDNF, CXC, IL-6 or corticosterone content of samples were performed using a least-squares fit.
All values represent mean ± SEM. Normality of data was verified using the D’Agostino-Pearson normality test (GraphPad Prism). Comparisons among experimental groups were made using analysis of variance (ANOVA), repeated measures ANOVA, paired or unpaired t test where appropriate. When F-test statistic exceeded the critical value at α = 0.05, the Sidak’s multiple comparisons test was used to compare group means.
CYP, RVS or CYP+RVS significantly (p ≤ 0.01) decreased body weight in mice by day 4 of the CYP alone, RVS alone or combined CYP+RVS protocols (Figure 2A). Significant (p ≤ 0.01) weight reductions were maintained throughout the study (day 9) of the protocols (Figure 2A). CYP, RVS or CYP+RVS RVS significantly (p ≤ 0.01) increased serum corticosterone measured on day 9 compared to control mice (handling only) (Figure 2B); however, the magnitude of increase varied with treatment. Combined CYP+RVS treatment resulted in the greatest increase in serum corticosterone (4.5-fold) followed by increases resulting from RVS alone (3.7-fold) or CYP alone (2.1-fold) (Figure 2B).
Figure 2 Control, CYP, RVS or CYP+RVS effects on serum corticosterone, and body weight (A) Changes in body weight of mice over 9 days for mice in control, CYP, RVS or CYP+RVS cohorts. Beginning on day 4 and lasting for the full 9 day duration of study, mice in CYP, RVS, or CYP+RVS cohorts exhibited significant weight gain attenuation compared with control mice. Body weights were significantly (p ≤ 0.01) decreased in CYP, RVS or CYP+RVS mice groups from days 4–9. (B) Serum corticosterone significantly (p ≤ 0.01) increased in CYP, RVS or CYP+RVS mice groups exposure measured on day 9 of the study duration. Mice in the CYP+RVS group exhibited the greatest (4.5-fold) increase in serum corticosterone compared to CYP (2.1-fold) or RVS (3.7-fold) alone groups. B: Samples size are n = 8. Values are means ± SEM. *p ≤ 0.01.
We previously demonstrated inflammatory changes in the urinary bladder in rodents treated with intraperitoneal CYP when used at a concentration of 75 mg/kg with a frequency of treatment of every 48-72 hours for 8-10 days (41, 49). In the current study, we treated mice with intraperitoneal CYP at a reduced concentration (35 mg/kg) administered every 48 hours for 7 days. Urinary bladders harvested from mice treated with CYP showed evidence of petechial hemorrhages on the urothelial surface (Figures 3A-C) as well as areas of urothelial erosion and urothelial cell sloughing in tissue sections compared to control mice. Regions of urothelial erosion and sloughing were patchy with intact regions of urothelium also being observed (Figure 3E). In contrast, urinary bladder from control mice exhibited intact urothelium throughout the full extent of tissue sections (Figure 3D).
Figure 3 Urinary bladders from mice treated with low concentration CYP (35 mg/kg, i.p., every 48 hours for 7 days) exhibit urothelial petechial hemorrhages and erosion. (A, B) Gross images of urinary bladder from control (A) and CYP-treated mice (B). Bladders from CYP-treated mice (B) exhibit petechial hemorrhages on the urothelial surface compared to control mice (A). The rectangular region in B is enlarged in C to highlight regions of petechial hemorrhage (arrows). In control bladder (A), there is a vertically extending, large blood vessel (*). (D, E) H+E staining in urinary bladder sections (10 µm) from control (D) and CYP-treated mice (E). In CYP-treated mice, urinary bladder sections exhibit regions of urothelium erosion (E, bracket) and urothelial cell sloughing (E, arrows). In control mice, the urothelium was intact (D). Calibration bar in B represents: 1.5 mm in A-B, 0.6 mm in C and 100 µm in D, E.
CYP alone, RVS alone or combined CYP+RVS significantly (p ≤ 0.01) increased whole urinary bladder NGF in mice measured on day 7 of the RVS paradigm (Figure 4). Although urinary bladder NGF increased expression with CYP alone (2.8-fold) and RVS alone (3.1-fold) protocols, the greatest increase (8.1-fold) in urinary bladder NGF expression was demonstrated following the combined CYP+RVS protocol (Figure 4). A similar observation was made with urinary bladder BDNF expression where combined CYP+RVS produced the greatest increase (3.3-fold) in expression compared to CYP alone (1.8-fold) or RVS alone (2.3-fold) protocols (Figure 4). Baseline BDNF urinary bladder expression was greater than NGF expression, but urinary bladder NGF expression was significantly (p ≤ 0.01) greater than BDNF following CYP+RVS (Figure 4). No differences between urinary bladder NGF or BDNF expression were observed with CYP alone or RVS alone protocols (Figure 4).
Figure 4 Increased Expression of NGF and BDNF in Urinary Bladder with CYP, RVS or CYP+RVS. Basal expression of urinary bladder BDNF was significantly (p ≤ 0.01) greater than NGF expression in control mice. CYP, RVS and CYP+RVS significantly (p ≤ 0.01) increased both NGF and BDNF urinary bladder expression. CYP+RVS induced the greatest increase in NGF and BDNF urinary bladder expression compared to CYP or RVS alone. Urinary bladder NGF expression was significantly (p ≤ 0.01) greater than BDNF urinary bladder expression in mice exposed to CYP+RVS. Samples size are n = 8. Values are means ± SEM. * or #, p ≤ 0.01. Bracket and * indicates comparison between NGF and BDNF expression.
CYP alone, RVS alone or combined CYP+RVS significantly (p ≤ 0.01) increased whole urinary bladder CXCL9 and CXCL10 expression (Figure 5A). Although urinary bladder CXCL9 and CXCL10 increased expression with CYP alone (2.0-2.1-fold) and RVS alone (1.5-1.8-fold) protocols, the greatest increases (2.7-3.5-fold) in expression were demonstrated with the combined CYP+RVS protocol (Figure 5A). Baseline (control), CYP alone and RVS alone CXCL10 urinary bladder expression was significantly (p ≤ 0.01) greater than CXCL-9 expression; however, no differences were observed between CXCL9 and CXCL10 expression with combined CYP+RVS treatment (Figure 5A). Urinary bladder IL-6 expression was greatest with the combined CYP+RVS protocol compared to CYP alone (3.0-fold) or RVS alone (2.5-fold) treatment protocols (Figure 5B).
Figure 5 Increased Expression of CXC Chemokines and IL-6 in Urinary Bladder with CYP, RVS or CYP+RVS. (A) CYP alone, RVS alone or combined CYP+RVS significantly (p ≤ 0.01) increased whole urinary bladder CXCL9 (*, p ≤ 0.01)) and CXCL10 (#, p ≤ 0.01) expression in mice. Although urinary bladder CXCL9 and CXCL10 increased expression with CYP alone (2.0-2.1-fold) and RVS alone (1.5-1.8-fold) protocols, the greatest increases (2.7-3.5-fold) in expression were demonstrated with combined CYP+RVS (A). Baseline (control), CYP alone and RVS alone CXCL10 urinary bladder expression was significantly (line with *, p ≤ 0.01) greater than CXCL-9 expression; however, no differences were observed between CXCL9 and CXCL10 expression with combined CYP+RVS treatment (A). (B) Urinary bladder IL-6 expression was significantly (*, p ≤ 0.01) increased with CYP alone, RVS alone and CYP+RVS. The largest increase in IL-6 bladder expression was demonstrated with the combined CYP+RVS protocol compared to CYP alone (3.0-fold) or RVS alone (2.5-fold) treatment protocols (B). Samples size are n = 8. Values are means ± SEM. * or #, p ≤ 0.01. Line and * indicates comparison between CXCL9 and CXCL10 expression.
In this study, we selected a concentration of CYP (35 mg/kg, i.p.) and frequency of administration that when given alone, in the absence of RVS, did not affect voiding frequency (Figure 6B) compared to control (Figure 6A). In C57Bl/6 female mice exposed to 7-day RVS, no changes in voiding frequency were observed compared to control (handled) (Figure 6C). CYP when combined with 7-day RVS, significantly increased voiding frequency (Figure 6D). Summary data demonstrate that CYP+RVS significantly (p ≤ 0.01) decreased functional bladder capacity (2.4-fold vs. control) (infused volume that elicits a void event) (Figure 7A) and significantly (p ≤ 0.01) reduced the intermicturition interval (IMI) (2.4-fold vs. control) (increased voiding frequency) (Figure 7B). The magnitude of change in bladder capacity (2.2-2.5-fold) and IMI (2.1-2.6-fold) in the CYP+RVS cohort was similar when compared to each group (control, CYP, or RVS). No changes were observed in CYP alone or RVS alone cohorts when compared to control (Figures 7A, B).
Figure 6 Representative bladder function recordings showing volume infused and bladder pressure recorded during conscious, open outlet cystometry. Bladder function recordings from control (A), CYP (35 mg/kg, i.p., every 48 hours for 4 total doses), (B) RVS (7 day), (C) or CYP+RVS (7 day). (A) Control mice (handling and returned to home cage) infused intravesically with saline (0.9%) exhibited an infused volume (IV) necessary to elicit a micturition event (i.e., functional bladder capacity) that is like CYP (B) and RVS alone (C). (D). CYP+RVS mice exhibited a reduced infused volume (IV) required to elicit a micturition that results in increased voiding frequency compared to control, CYP or RVS mice. Timeline represents 250 s from the recorded cystometrogram.
Figure 7 Summary histograms of functional bladder capacity (A) (mL) and intercontraction interval (B) (ICI) measured from bladder function testing in control, CYP, RVS and CYP+RVS mice. CYP+RVS mice exhibited significantly (*, p ≤ 0.01) reduced IV (A) and ICI (B) compared to control (handled), CYP alone and RVS alone mice. Values are means ± SEM. Samples size are n = 8; *, p ≤ 0.01 versus control. Seconds, s; milliliter, mL.
C57Bl/6 female mice exposed to CYP+RVS significantly (p ≤ 0.05) increased baseline, threshold, and peak micturition pressures (Figure 2) (Table 2). No changes in baseline, threshold or peak micturition pressures were observed in CYP alone or RVS alone cohorts compared to control (Figure 2, Table 2).
Table 2 Bladder pressures (baseline, threshold, peak micturition) recorded during conscious cystometry in control, CYP, RVS or CYP+RVS mice.
Female mice exposed to CYP+RVS exhibited significantly (p ≤ 0.01) decreased somatic sensitivity in the pelvic region (Figure 8A) with all monofilament forces evaluated (0.1 to 4 g). Female mice exposed to CYP+RVS exhibited significantly (p ≤ 0.01) decreased somatic sensitivity to hindpaw stimulation with monofilaments of greater force (0.4-4 g). Control, CYP alone or RVS alone mouse cohorts exhibited similar somatic sensitivity in the pelvic region (Figure 8A) and hindpaw (Figure 8B) with all monofilament forces evaluated (0.1 to 4 g).
Figure 8 Effects of CYP, RVS or CYP+RVS on pelvic and hindpaw region sensitivity as determined using calibrated von Frey filaments. (A) Pelvic stimulation was applied to the lower abdominal area, including the suprapubic region, overlying the urinary bladder. CYP+RVS mice (n = 10) exhibited significantly (*, p ≤ 0.01) increased pelvic response frequency with all von Frey filaments (0.1–4 g) tested compared to control mice, CYP or RVS alone (n = 10). (B) CYP+RVS mice (n = 10) exhibited significantly (*, p ≤ 0.01) increased hindpaw response frequency with all von Frey filaments (0.1–4 g) tested compared to control mice, CYP or RVS alone (n = 10). All somatic testing was performed blinded. Values are means ± SEM. Samples size are n = 10; *p ≤ 0.01.
Interstitial cystitis/bladder pain syndrome (IC/BPS) is an unpleasant sensation (pain, pressure, discomfort) perceived to be related to the urinary bladder, associated with LUT symptoms of more than six weeks duration, in the absence of infection or other identifiable causes (50). Symptom exacerbation (flare) is common with multiple, perceived triggers including stress. The impact of BPS/IC on quality of life is enormous (51) and flares are bothersome and disruptive (6, 11–13). Basic research investigations of flares are hampered by the lack of animal models (11–13). Effective treatments and a greater understanding of the contribution of stress to BPS/IC are needed.
A majority (78.4%) of participants enrolled in a Multidisciplinary Approach to the Study of Chronic Pelvic Pain (MAPP) study (6) that evaluated management and patient-reported flares in IC/BPS indicated that stress is a perceived trigger for their flares. Other contributing causes include specific foods, physical activities, sexual intercourse, and infections (6). Interestingly, female participants and those with somatic sensory hypersensitivity, are more likely to report various non-dietary factors as triggers than male participants and those without hypersensitivity (6). Female participants (32.1%) reported use of sleeping aids to manage IC/BSP flares (6). Although suggestive of a sleep disruption/disturbance, participants’ use of sleeping pills may be for the anxiolytic properties, consistent with participants reporting stress as a flare trigger (6).
Few studies have addressed flare triggers, underlying mechanisms, management, and treatment options (6, 11–13). The current study describes a novel mouse model where administration of CYP when combined with 7 days of stressor presentation (CYP+RVS) results in increased voiding frequency and increased somatic sensitivity. However, neither the CYP treatment alone or the 7 days of RVS alone increase voiding frequency or somatic sensitivity in C57Bl/6 mice despite increases in inflammatory mediators (e.g., NGF, BDNF, IL-6, CXC) and histological changes in the urinary bladder. We refer to this mouse model as a stress-induced symptom exacerbation (SISE) mouse model. Our longer-term goal is to use this mouse model to evaluate potential targets, management, and treatment interventions when stress is a contributing factor to flares. The SISE model in female mice demonstrate increased voiding frequency and increased pelvic and hindpaw sensitivity. Although increases in both pelvic and hindpaw sensitivity have been demonstrated previously in some animal urinary bladder inflammation models and may reflect overlapping dermatomes of the urinary bladder and hindpaw, not all models of urinary bladder inflammation (e.g., mice with chronic urothelial overexpression of NGF, NGF-OE) (44) exhibit both pelvic and hindpaw sensitivity. It would be of interest in future studies to evaluate upper limb sensitivity as well as other body regions with dermatomes distinct from the urinary bladder.
We also demonstrated increased expression of inflammatory mediators in the urinary bladder including neurotrophic factors (e.g., NGF, BDNF), chemokines (CXC) and IL-6 expression in the urinary bladder consistent with a large body of literature demonstrating roles for inflammatory mediators in preclinical animal models of and in IC/BPS patients (18, 21–34). Urinary bladder neurotrophic factor (i.e., NGF, BDNF), CXCL9, CXCL10 and IL-6 expression was increased in mice with CYP alone, RVS alone and CYP+RVS compared to control mice. In mice treated with CYP alone, the urinary bladders also exhibited physical signs of irritation/inflammation including urothelial erosion, urothelial cell sloughing and petechial hemorrhages. These studies demonstrate that despite no changes in urinary bladder function in mice treated with CYP alone or exposed to RVS (7-day) alone, changes in the urinary bladder inflammatory environment were present. This is consistent with the suggestion that low concentration (35 mg/kg) CYP treatment or RVS (7-day) exposure results in an underlying bladder inflammation that can be exacerbated when CYP and RVS are combined (CYP+RVS) producing changes in bladder function (i.e., increased voiding frequency) and enhanced somatic sensitivity. With the development of this SISE model, we have many follow-up questions that we wish to address including (1): How long do changes in bladder function and somatic sensitivity persist after cessation of CYP+RVS? (2) Can changes in bladder function and somatic sensation be reinstated with CYP or RVS alone? (3) Is 7-day RVS necessary when combined with CYP to elicit changes in bladder function and somatic sensation or can shorter duration RVS produce similar effects?
Multiple animal models of stress (e.g., including social stress (52–55), immobilization stress (56–59), water avoidance stress (60–62), footshock (63–65)) produce morphological, inflammatory and functional changes in the urinary bladder, and visceral sensitivity similar to signs and symptoms of IC/BPS. Among different types of stress, psychosocial stress may be the most relevant to everyday life. Psychosocial stress results from one’s cognitive appraisal of a perceived threat (either real or imagined) and our determination that we may not be able to overcome, adjust or adapt to that challenge (35, 66–71). Classically, the heightened HPA axis coordinates sympathetic nervous system activity and cortisol responses to maintain homeostasis (35, 66–71); however, repeated challenges and adversity or the inability to appropriately attenuate stress responses can result in mal-adaptations and cumulative and persistent damages (i.e., increased HPA and sympathetic reactivity; allostatic overload) that can manifest a variety of disorders including anxiety, depression, and gastrointestinal and urinary tract disorders (35, 36, 66–78).
A repeated variate stress (RVS) paradigm (7-day) in rats has been used to examine neurochemical expression in the bed nucleus of the stria terminalis (15, 17). This model of RVS was found to be anxiogenic, most likely mediated by neural circuits in the BNST (15, 79–82). Among other animal models with daily presentation of the same stressor, the RVS paradigm may be more relevant to human daily life stressors and lacks habituation because of novel stressor exposure. Plasma corticosterone, a steroid hormone involved in the HPA axis secreted by the adrenal gland in rodents during stressor exposure, is significantly increased (5.2-fold) in mice (14) and adrenal gland weights (37) in rats are increased following RVS. We have previously used the RVS protocol to characterize its effects on bladder function and somatic sensitivity in the rat (37, 38) and PACAP promoter-dependent EGFP BAC transgenic mouse strain (PACAP-EGFP) (14). RVS decreased (1) bladder capacity and void volume and increased voiding frequency in rats and PACAP-EGFP mice (14, 37) (2); increased somatic sensitivity of both the hindpaw or pelvic regions in rats and PACAP-EGFP mice (14, 37); and (3) produced changes in the inflammatory milieu (e.g., histamine, myeloperoxidase, NGF, chemokines) of the urinary bladder in rats (37, 38). It should be clarified that a 7-day RVS protocol in rats (15, 17, 82) and in specific transgenic mice (14) produced anxiety-like behaviors and changes in voiding function. However, other ongoing studies from our laboratory (83) and studies from other laboratories (S.E. Hammack, personal communication) indicate that using a 2-week RVS protocol in C57Bl/6 (wildtype) mice is needed to induce consistent anxiety-like behaviors and changes in voiding function.
NGF has been implicated in the peripheral sensitization of nociceptors (84–86), contributes to bladder sensory function and the development of referred hyperalgesia in response to urinary bladder inflammation (22, 44, 85–91). In addition, elevated NGF levels have been detected in the urine and urinary bladders of women with BPS/IC (23, 28). Intravenous administration of a humanized NGF neutralizing monoclonal antibody (tanezumab) in IC/BPS patients demonstrated proof of concept by improving the global response assessment and reducing the urgency episodes (92). BDNF is also upregulated in micturition reflex pathways in both humans and rodents with cystitis (93, 94) because of increased NGF synthesis (95–98). IC/BPS patients report symptom improvement following BDNF reduction (99). Pharmacological blockade of both NGF and BDNF in bladder inflammation models produce complementary improvements in bladder function (21, 100, 101). CYP+RVS mice exhibited significant increases in urinary bladder NGF and BDNF expression. Future studies are addressing NGF and/or BDNF blockade in the SISE model to determine effects on urinary bladder function and somatic sensitivity.
The underlying neural substrates, mechanisms and pathways linking psychosocial stress to altered behaviors and physiological disorders are still unclear. We previously used PACAP-EGFP transgenic mice to map specific neuronal PACAP populations in the CNS following RVS and/or RVS and bladder function testing (102). Bladder function testing in mice exposed to RVS increased numbers of PACAP-EGFP+ cells in lumbosacral spinal cord and dorsal root ganglia and supraspinal locations including the locus coeruleus, Barrington’s nucleus, rostral ventrolateral medulla, PAG, raphe, and amygdala (103). These supraspinal regions have reciprocal connections with the HPA axis which may represent some of the anatomical substrates connecting stress, bladder function and HPA function (104–106). Additional studies are needed to understand how stress or the failure to attenuate stress signaling can result in maladaptation and cumulative long-term damages that can manifest a variety of disorders including urinary bladder dysfunction (35, 66–69, 71, 105, 106). We have previously demonstrated improvements in bladder function following intravesical instillation of the PAC1 receptor antagonist, PACAP6-38, following RVS exposure in mice. Current studies are evaluating the contribution of PACAP and associated receptors in this SISE model.
IC/BPS patients exhibit HPA dysfunction and increased levels of stress (1, 107). Abnormal, or chronic HPA activation is associated with dysregulated responses to stress and inflammation and may result in aberrant sympathetic activation (1, 108, 109) that may contribute to changes in urinary bladder function and somatic sensation. For example, subcutaneous phenylephrine induced somatic sensitivity, increased c-Fos immediate early gene expression in spinal micturition pathways and bladder hyperactivity (110). These changes were reversed by a selective α1A-adrenoceptor antagonist (i.e., silodosin), and absent in TRPV1-/- mice. In future studies, we will evaluate the involvement of sympathetic activation in the current SISE model and voiding dysfunction by determining serum concentration of noradrenaline and the adrenal response.
In summary, these initial results demonstrated that CYP (35 mg/kg) alone or RVS alone creates a change in the inflammatory environment of the urinary bladder and a systemic increase in corticosterone expression but does not result in a change in bladder function or somatic sensitivity until CYP is combined with RVS (CYP+RVS). The SISE model of CYP+RVS will be useful to develop hypotheses that address the underlying mechanisms that contribute to stress exacerbation of signs and symptoms of functional bladder disorders leading to identification of novel targets, management, and potential treatments.
The original contributions presented in the study are included in the article/supplementary material, further inquiries can be directed to the corresponding author/s.
The animal study was reviewed and approved by University of Vermont IACUC.
MV conceived and designed research. SC, BG, and MV performed experiments. SC, BG, and MV analyzed data. BG and MV interpreted results of experiments. MV and BG prepared figures and drafted the manuscript. BG, SC, and MV edited and revised manuscript. All authors contributed to the article and approved the submitted version.
This work was funded by National Institute of Diabetes and Digestive and Kidney Diseases Grants 5R01DK120108 and 1R01DK124580 to MV.
The authors gratefully acknowledge the additional support provided by the Cell and Molecular Core in the Department of Neurological Sciences at The Larner College of Medicine and Vermont Integrative Genomics Resource. The authors thank Ms. Celia McClintock for their tremendous assistance with animal care, handling, and observation.
The authors declare that the research was conducted in the absence of any commercial or financial relationships that could be construed as a potential conflict of interest.
All claims expressed in this article are solely those of the authors and do not necessarily represent those of their affiliated organizations, or those of the publisher, the editors and the reviewers. Any product that may be evaluated in this article, or claim that may be made by its manufacturer, is not guaranteed or endorsed by the publisher.
1. Nazif O, Teichman JM, Gebhart GF. Neural upregulation in interstitial cystitis. Urology (2007) 69(4 Suppl):24–33. doi: 10.1016/j.urology.2006.08.1108
2. Westropp JL, Buffington CA. In vivo models of interstitial cystitis. J Urol (2002) 167(2 Pt 1):694–702. doi: 10.1016/S0022-5347(01)69129-8
3. Birder L, Andersson KE. Urothelial signaling. Physiol Rev (2013) 93(2):653–80. doi: 10.1152/physrev.00030.2012
4. Lai HH, Rawal A, Shen B, Vetter J. The relationship between anxiety and overactive bladder or urinary incontinence symptoms in the clinical population. Urology (2016) 98:50–7. doi: 10.1016/j.urology.2016.07.013
5. Lai HH, Shen B, Rawal A, Vetter J. The relationship between depression and overactive bladder/urinary incontinence symptoms in the clinical OAB population. BMC Urol (2016) 16(1):60. doi: 10.1186/s12894-016-0179-x
6. Lai HH, Vetter J, Song J, Andriole GL, Colditz GA, Sutcliffe S. Management of symptom flares and patient-reported flare triggers in interstitial Cystitis/Bladder pain syndrome (IC/BPS)-findings from one site of the MAPP research network. Urology (2019) 126:24–33. doi: 10.1016/j.urology.2019.01.012
7. Rothrock NE, Lutgendorf SK, Kreder KJ, Ratliff T, Zimmerman B. Stress and symptoms in patients with interstitial cystitis: A life stress model. Urology (2001) 57(3):422–7. doi: 10.1016/S0090-4295(00)00988-2
8. Rothrock NE, Lutgendorf SK, Kreder KJ, Ratliff TL, Zimmerman B. Daily stress and symptom exacerbation in interstitial cystitis patients. Urology (2001) 57(6 Suppl 1):122. doi: 10.1016/S0090-4295(01)01075-5
9. Lutgendorf SK, Kreder KJ, Rothrock NE, Ratliff TL, Zimmerman B. Stress and symptomatology in patients with interstitial cystitis: A laboratory stress model. J Urol (2000) 164(4):1265–9. doi: 10.1016/S0022-5347(05)67152-2
10. Naliboff BD, Stephens AJ, Afari N, Lai H, Krieger JN, Hong B, et al. Widespread psychosocial difficulties in men and women with urologic chronic pelvic pain syndromes: Case-control findings from the multidisciplinary approach to the study of chronic pelvic pain research network. Urology (2015) 85(6):1319–27. doi: 10.1016/j.urology.2015.02.047
11. Nickel JC, Stephens A, Landis JR, Mullins C, van Bokhoven A, Lucia MS, et al. Assessment of the lower urinary tract microbiota during symptom flare in women with urologic chronic pelvic pain syndrome: A MAPP network study. J Urol (2016) 195(2):356–62. doi: 10.1016/j.juro.2015.09.075
12. Sutcliffe S, Colditz GA, Goodman MS, Pakpahan R, Vetter J, Ness TJ, et al. Urological chronic pelvic pain syndrome symptom flares: Characterisation of the full range of flares at two sites in the multidisciplinary approach to the study of chronic pelvic pain (MAPP) research network. BJU Int (2014) 114(6):916–25. doi: 10.1111/bju.12778
13. Sutcliffe S, Jemielita T, Lai HH, Andriole GL, Bradley CS, Clemens JQ, et al. A case-crossover study of urological chronic pelvic pain syndrome flare triggers in the MAPP research network. J Urol (2018) 199(5):1245–51. doi: 10.1016/j.juro.2017.12.050
14. Girard BM, Campbell SE, Beca KI, Perkins M, Hsiang H, May V, et al. Intrabladder PAC1 receptor antagonist, PACAP(6-38), reduces urinary bladder frequency and pelvic sensitivity in mice exposed to repeated variate stress (RVS). J Mol Neurosci (2021) 71(8):1575–88. doi: 10.1007/s12031-020-01649-x
15. Hammack SE, Cheung J, Rhodes KM, Schutz KC, Falls WA, Braas KM, et al. Chronic stress increases pituitary adenylate cyclase-activating peptide (PACAP) and brain-derived neurotrophic factor (BDNF) mRNA expression in the bed nucleus of the stria terminalis (BNST): roles for PACAP in anxiety-like behavior. Psychoneuroendocrinology (2009) 34(6):833–43. doi: 10.1016/j.psyneuen.2008.12.013
16. Hammack SE, May V. Pituitary adenylate cyclase activating polypeptide in stress-related disorders: data convergence from animal and human studies. Biol Psychiatry (2015) 78(3):167–77. doi: 10.1016/j.biopsych.2014.12.003
17. King SB, Toufexis DJ, Hammack SE. Pituitary adenylate cyclase activating polypeptide (PACAP), stress, and sex hormones. Stress (2017) 20(5):1–11. doi: 10.1080/10253890.2017.1336535
18. Vizzard MA. Changes in urinary bladder neurotrophic factor mRNA and NGF protein following urinary bladder dysfunction. Exp Neurol (2000) 161(1):273–84. doi: 10.1006/exnr.1999.7254
19. Perkins M, Girard BM, Campbell SE, Hennig GW, Vizzard MA. Imatinib mesylate reduces neurotrophic factors and pERK and pAKT expression in urinary bladder of female mice with cyclophosphamide-induced cystitis. Front Syst Neurosci (2022) 16:884260. doi: 10.3389/fnsys.2022.884260
20. Hsiang HW, Girard BM, Vizzard MA. Changes in nerve growth factor signaling in female mice with cyclophosphamide-induced cystitis. Front Urol (2023) 2. doi: 10.3389/fruro.2022.1089220
21. Hu VY, Zvara P, Dattilio A, Redman TL, Allen SJ, Dawbarn D, et al. Decrease in bladder overactivity with REN1820 in rats with cyclophosphamide induced cystitis. J Urol (2005) 173(3):1016–21. doi: 10.1097/01.ju.0000155170.15023.e5
22. Zvara P, Vizzard MA. Exogenous overexpression of nerve growth factor in the urinary bladder produces bladder overactivity and altered micturition circuitry in the lumbosacral spinal cord. BMC Physiol (2007) 7:9. doi: 10.1186/1472-6793-7-9
23. Okragly AJ, Niles AL, Saban R, Schmidt D, Hoffman RL, Warner TF, et al. Elevated tryptase, nerve growth factor, neurotrophin-3 and glial cell line-derived neurotrophic factor levels in the urine of interstitial cystitis and bladder cancer patients. J Urol (1999) 161(2):438–41; discussion 41-2.
24. Huang Y, Su M, Zhang C, Zhan H, Yang F, Gao Z, et al. Activation of translocator protein alleviates mechanical allodynia and bladder dysfunction in cyclophosphamide-induced cystitis through repression of BDNF-mediated neuroinflammation. Eur J Pain (2022) 26(6):1234–44. doi: 10.1002/ejp.1942
25. Chuang YC, Fraser MO, Yu Y, Chancellor MB, de Groat WC, Yoshimura N. The role of bladder afferent pathways in bladder hyperactivity induced by the intravesical administration of nerve growth factor. J Urol (2001) 165(3):975–9. doi: 10.1016/S0022-5347(05)66587-1
26. Clemow DB, Steers WD, McCarty R, Tuttle JB. Altered regulation of bladder nerve growth factor and neurally mediated hyperactive voiding. Am J Physiol (1998) 275(4 Pt 2):R1279–86. doi: 10.1152/ajpregu.1998.275.4.R1279
27. Dmitrieva N, McMahon SB. Sensitisation of visceral afferents by nerve growth factor in the adult rat. Pain (1996) 66(1):87–97. doi: 10.1016/0304-3959(96)02993-4
28. Lowe EM, Anand P, Terenghi G, Williams-Chestnut RE, Sinicropi DV, Osborne JL. Increased nerve growth factor levels in the urinary bladder of women with idiopathic sensory urgency and interstitial cystitis. Br J Urol (1997) 79(4):572–7. doi: 10.1046/j.1464-410X.1997.00097.x
29. Cruz CD. Neurotrophins in bladder function: what do we know and where do we go from here? Neurourol Urodyn (2014) 33(1):39–45. doi: 10.1002/nau.22438
30. Coelho A, Oliveira R, Antunes-Lopes T, Cruz CD. Partners in crime: NGF and BDNF in visceral dysfunction. Curr Neuropharmacol (2019) 17(11):1021–38. doi: 10.2174/1570159X17666190617095844
31. Guo M, Chang P, Hauke E, Girard BM, Tooke K, Ojala J, et al. Expression and function of chemokines CXCL9-11 in micturition pathways in cyclophosphamide (CYP)-induced cystitis and somatic sensitivity in mice. Front Syst Neurosci (2018) 12:9. doi: 10.3389/fnsys.2018.00009
32. Malley SE, Vizzard MA. Changes in urinary bladder cytokine mRNA and protein after cyclophosphamide-induced cystitis. Physiol Genomics (2002) 9(1):5–13. doi: 10.1152/physiolgenomics.00117.2001
33. Jiang YH, Jhang JF, Kuo HC. Can we use urinary Cytokine/Chemokine analysis in discriminating ulcer-type interstitial Cystitis/Bladder pain syndrome? Diagnostics (Basel) (2022) 12(5):1093. doi: 10.3390/diagnostics12051093
34. Jiang YH, Jhang JF, Hsu YH, Kuo HC. Usefulness of urinary biomarkers for assessing bladder condition and histopathology in patients with interstitial Cystitis/Bladder pain syndrome. Int J Mol Sci (2022) 23(19):12044. doi: 10.3390/ijms231912044
35. Mahon PB, Zandi PP, Potash JB, Nestadt G, Wand GS. Genetic association of FKBP5 and CRHR1 with cortisol response to acute psychosocial stress in healthy adults. Psychopharmacol (Berl) (2013) 227(2):231–41. doi: 10.1007/s00213-012-2956-x
36. Herman JP, McKlveen JM, Solomon MB, Carvalho-Netto E, Myers B. Neural regulation of the stress response: glucocorticoid feedback mechanisms. Braz J Med Biol Res (2012) 45(4):292–8. doi: 10.1590/S0100-879X2012007500041
37. Merrill L, Malley S, Vizzard MA. Repeated variate stress in male rats induces increased voiding frequency, somatic sensitivity, and urinary bladder nerve growth factor expression. Am J Physiol Regulatory Integr Comp Physiol (2013) 305(2):R147–56. doi: 10.1152/ajpregu.00089.2013
38. Merrill L, Vizzard MA. Intravesical TRPV4 blockade reduces repeated variate stress-induced bladder dysfunction by increasing bladder capacity and decreasing voiding frequency in male rats. Am J Physiol Regulatory Integr Comp Physiol (2014) 307(4):R471–80. doi: 10.1152/ajpregu.00008.2014
39. Longden TA, Dabertrand F, Hill-Eubanks DC, Hammack SE, Nelson MT. Stress-induced glucocorticoid signaling remodels neurovascular coupling through impairment of cerebrovascular inwardly rectifying k+ channel function. Proc Natl Acad Sci USA. (2014) 111(20):7462–7. doi: 10.1073/pnas.1401811111
40. Vizzard MA. Up-regulation of pituitary adenylate cyclase-activating polypeptide in urinary bladder pathways after chronic cystitis. J Comp Neurol (2000) 420(3):335–48. doi: 10.1002/(SICI)1096-9861(20000508)420:3<335::AID-CNE5>3.0.CO;2-#
41. Vizzard MA. Alterations in spinal cord fos protein expression induced by bladder stimulation following cystitis. Am J Physiol Regulatory Integr Comp Physiol (2000) 278(4):R1027–39. doi: 10.1152/ajpregu.2000.278.4.R1027
42. Vizzard MA. Alterations in neuropeptide expression in lumbosacral bladder pathways following chronic cystitis. J Chem Neuroanat (2001) 21(2):125–38. doi: 10.1016/S0891-0618(00)00115-0
43. Gonzalez EJ, Girard BM, Vizzard MA. Expression and function of transforming growth factor-beta isoforms and cognate receptors in the rat urinary bladder following cyclophosphamide-induced cystitis. Am J Physiol Renal Physiol (2013) 305(9):F1265–76. doi: 10.1152/ajprenal.00042.2013
44. Schnegelsberg B, Sun TT, Cain G, Bhattacharya A, Nunn PA, Ford AP, et al. Overexpression of NGF in mouse urothelium leads to neuronal hyperinnervation, pelvic sensitivity, and changes in urinary bladder function. Am J Physiol Regulatory Integr Comp Physiol (2010) 298(3):R534–47. doi: 10.1152/ajpregu.00367.2009
45. Girard BM, Campbell SE, Perkins M, Hsiang H, Tooke K, Drescher C, et al. TRPV4 blockade reduces voiding frequency, ATP release, and pelvic sensitivity in mice with chronic urothelial overexpression of NGF. Am J Physiol Renal Physiol (2019) 317(6):F1695–706. doi: 10.1152/ajprenal.00147.2019
46. Rudick CN, Chen MC, Mongiu AK, Klumpp DJ. Organ cross talk modulates pelvic pain. Am J Physiol Regul Integr Comp Physiol (2007) 293(3):R1191–8. doi: 10.1152/ajpregu.00411.2007
47. Arms L, Girard BM, Vizzard MA. Expression and function of CXCL12/CXCR4 in rat urinary bladder with cyclophosphamide-induced cystitis. Am J Physiol Renal Physiol (2010) 298(3):F589–600. doi: 10.1152/ajprenal.00628.2009
48. Girard BM, Malley SE, Vizzard MA. Neurotrophin/receptor expression in urinary bladder of mice with overexpression of NGF in urothelium. Am J Physiol Renal Physiol (2011) 300(2):F345–55. doi: 10.1152/ajprenal.00515.2010
49. Klinger MB, Dattilio A, Vizzard MA. Expression of cyclooxygenase-2 in urinary bladder in rats with cyclophosphamide-induced cystitis. Am J Physiol Regulatory Integr Comp Physiol (2007) 293(2):R677–85. doi: 10.1152/ajpregu.00305.2007
50. Clemens JQ, Erickson DR, Varela NP, Lai HH. Diagnosis and treatment of interstitial Cystitis/Bladder pain syndrome. J Urol (2022) 208(1):34–42. doi: 10.1097/JU.0000000000002756
51. Clemens JQ, Mullins C, Kusek JW, Kirkali Z, Mayer EA, Rodriguez LV, et al. The MAPP research network: a novel study of urologic chronic pelvic pain syndromes. BMC Urol (2014) 14:57. doi: 10.1186/1471-2490-14-57
52. Bhatnagar S, Vining C, Iyer V, Kinni V. Changes in hypothalamic-pituitary-adrenal function, body temperature, body weight and food intake with repeated social stress exposure in rats. J Neuroendocrinol (2006) 18(1):13–24. doi: 10.1111/j.1365-2826.2005.01375.x
53. Chang A, Butler S, Sliwoski J, Valentino R, Canning D, Zderic S. Social stress in mice induces voiding dysfunction and bladder wall remodeling. Am J Physiol Renal Physiol (2009) 297(4):F1101–8. doi: 10.1152/ajprenal.90749.2008
54. Miczek KA. Chronic delta9-tetrahydrocannabinol in rats: effect on social interactions, mouse killing, motor activity, consummatory behavior, and body temperature. Psychopharmacol (Berl) (1979) 60(2):137–46. doi: 10.1007/BF00432284
55. Wood SK, Baez MA, Bhatnagar S, Valentino RJ. Social stress-induced bladder dysfunction: potential role of corticotropin-releasing factor. Am J Physiol Regulatory Integr Comp Physiol (2009) 296(5):R1671–8. doi: 10.1152/ajpregu.91013.2008
56. Alexacos N, Pang X, Boucher W, Cochrane DE, Sant GR, Theoharides TC. Neurotensin mediates rat bladder mast cell degranulation triggered by acute psychological stress. Urology (1999) 53(5):1035–40. doi: 10.1016/S0090-4295(98)00627-X
57. Boucher W, Kempuraj D, Michaelian M, Theoharides TC. Corticotropin-releasing hormone-receptor 2 is required for acute stress-induced bladder vascular permeability and release of vascular endothelial growth factor. BJU Int (2010) 106(9):1394–9. doi: 10.1111/j.1464-410X.2010.09237.x
58. Boucher WS, Letourneau R, Huang M, Kempuraj D, Green M, Sant GR, et al. Intravesical sodium hyaluronate inhibits the rat urinary mast cell mediator increase triggered by acute immobilization stress. J Urol (2002) 167(1):380–4. doi: 10.1016/S0022-5347(05)65472-9
59. Spanos C, Pang X, Ligris K, Letourneau R, Alferes L, Alexacos N, et al. Stress-induced bladder mast cell activation: implications for interstitial cystitis. J Urol (1997) 157(2):669–72. doi: 10.1097/00005392-199702000-00085
60. Cetinel S, Ercan F, Cikler E, Contuk G, Sener G. Protective effect of melatonin on water avoidance stress induced degeneration of the bladder. J Urol (2005) 173(1):267–70. doi: 10.1097/01.ju.0000145891.35810.56
61. Robbins M, DeBerry J, Ness T. Chronic psychological stress enhances nociceptive processing in the urinary bladder in high-anxiety rats. Physiol Behav (2007) 91(5):544–50. doi: 10.1016/j.physbeh.2007.04.009
62. Smith AL, Leung J, Kun S, Zhang R, Karagiannides I, Raz S, et al. The effects of acute and chronic psychological stress on bladder function in a rodent model. Urology (2011) 78(4):967.e1–7. doi: 10.1016/j.urology.2011.06.041
63. Black LV, Ness TJ, Robbins MT. Effects of oxytocin and prolactin on stress-induced bladder hypersensitivity in female rats. J Pain (2009) 10(10):1065–72. doi: 10.1016/j.jpain.2009.04.007
64. Imaki T, Nahan JL, Rivier C, Sawchenko PE, Vale W. Differential regulation of corticotropin-releasing factor mRNA in rat brain regions by glucocorticoids and stress. J Neurosci (1991) 11(3):585–99. doi: 10.1523/JNEUROSCI.11-03-00585.1991
65. Robbins MT, Ness TJ. Footshock-induced urinary bladder hypersensitivity: role of spinal corticotropin-releasing factor receptors. J Pain (2008) 9(11):991–8. doi: 10.1016/j.jpain.2008.05.006
66. FitzGerald LZ, Kehoe P, Sinha K. Hypothalamic–pituitary– adrenal axis dysregulation in women with irritable bowel syndrome in response to acute physical stress. West J Nurs Res (2009) 31(7):818–36. doi: 10.1177/0193945909339320
67. Lovallo WR. Early life adversity reduces stress reactivity and enhances impulsive behavior: implications for health behaviors. Int J Psychophysiol (2013) 90(1):8–16. doi: 10.1016/j.ijpsycho.2012.10.006
68. Marshall RD, Garakani A. Psychobiology of the acute stress response and its relationship to the psychobiology of post-traumatic stress disorder. Psychiatr Clin North Am (2002) 25(2):385–95. doi: 10.1016/S0193-953X(01)00005-3
69. Mihaljevic M, Zeljic K, Soldatovic I, Andric S, Mirjanic T, Richards A, et al. The emerging role of the FKBP5 gene polymorphisms in vulnerability-stress model of schizophrenia: further evidence from a Serbian population. Eur Arch Psychiatry Clin Neurosci (2016) 267(6):527-39. doi: 10.1007/s00406-016-0720-7
70. Stephens MA, Mahon PB, McCaul ME, Wand GS. Hypothalamic-pituitary-adrenal axis response to acute psychosocial stress: Effects of biological sex and circulating sex hormones. Psychoneuroendocrinology (2016) 66:47–55. doi: 10.1016/j.psyneuen.2015.12.021
71. Turner-Cobb JM. Psychological and stress hormone correlates in early life: a key to HPA-axis dysregulation and normalisation. Stress (2005) 8(1):47–57. doi: 10.1080/10253890500095200
72. Herman JP, Figueiredo H, Mueller NK, Ulrich-Lai Y, Ostrander MM, Choi DC, et al. Central mechanisms of stress integration: hierarchical circuitry controlling hypothalamo-pituitary-adrenocortical responsiveness. Front Neuroendocrinol (2003) 24(3):151–80. doi: 10.1016/j.yfrne.2003.07.001
73. Herman JP, McKlveen JM, Ghosal S, Kopp B, Wulsin A, Makinson R, et al. Regulation of the hypothalamic-Pituitary-Adrenocortical stress response. Compr Physiol (2016) 6(2):603–21. doi: 10.1002/cphy.c150015
74. Herman JP, Ostrander MM, Mueller NK, Figueiredo H. Limbic system mechanisms of stress regulation: hypothalamo-pituitary-adrenocortical axis. Prog Neuropsychopharmacol Biol Psychiatry (2005) 29(8):1201–13. doi: 10.1016/j.pnpbp.2005.08.006
75. Herman JP, Prewitt CM, Cullinan WE. Neuronal circuit regulation of the hypothalamo-pituitary-adrenocortical stress axis. Crit Rev Neurobiol (1996) 10(3-4):371–94. doi: 10.1615/CritRevNeurobiol.v10.i3-4.50
76. Jankord R, Herman JP. Limbic regulation of hypothalamo-pituitary-adrenocortical function during acute and chronic stress. Ann N Y Acad Sci (2008) 1148:64–73. doi: 10.1196/annals.1410.012
77. Jeanneteau FD, Lambert WM, Ismaili N, Bath KG, Lee FS, Garabedian MJ, et al. BDNF and glucocorticoids regulate corticotrophin-releasing hormone (CRH) homeostasis in the hypothalamus. Proc Natl Acad Sci USA. (2012) 109(4):1305–10. doi: 10.1073/pnas.1114122109
78. Ressler KJ, Mayberg HS. Targeting abnormal neural circuits in mood and anxiety disorders: from the laboratory to the clinic. Nat Neurosci (2007) 10(9):1116–24. doi: 10.1038/nn1944
79. Lezak KR, Roelke E, Harris OM, Choi I, Edwards S, Gick N, et al. Pituitary adenylate cyclase-activating polypeptide (PACAP) in the bed nucleus of the stria terminalis (BNST) increases corticosterone in male and female rats. Psychoneuroendocrinology (2014) 45:11–20. doi: 10.1016/j.psyneuen.2014.03.007
80. Lezak KR, Roman CW, Braas KM, Schutz KC, Falls WA, Schulkin J, et al. Regulation of bed nucleus of the stria terminalis PACAP expression by stress and corticosterone. J Mol Neurosci (2014) 54(3):477–84. doi: 10.1007/s12031-014-0269-8
81. Missig G, Roman CW, Vizzard MA, Braas KM, Hammack SE, May V. Parabrachial nucleus (PBn) pituitary adenylate cyclase activating polypeptide (PACAP) signaling in the amygdala: implication for the sensory and behavioral effects of pain. Neuropharmacology (2014) 86:38–48. doi: 10.1016/j.neuropharm.2014.06.022
82. Roman CW, Lezak KR, Hartsock MJ, Falls WA, Braas KM, Howard AB, et al. PAC1 receptor antagonism in the bed nucleus of the stria terminalis (BNST) attenuates the endocrine and behavioral consequences of chronic stress. Psychoneuroendocrinology (2014) 47:151–65. doi: 10.1016/j.psyneuen.2014.05.014
83. Sidwell AB, McClintock C, Beca KI, Campbell SE, Girard BM, Vizzard MA. Repeated variate stress increased voiding frequency and altered TrpV1 and TrpV4 transcript expression in lower urinary tract (LUT) pathways in female mice. Front Urol (2023) 2. doi: 10.3389/fruro.2022.1086179
84. Dinarello CA. Role of pro- and anti-inflammatory cytokines during inflammation: experimental and clinical findings. J Biol Regul Homeost Agents (1997) 11(3):91–103.
85. Dray A. Inflammatory mediators of pain. Br J Anaesth (1995) 75(2):125–31. doi: 10.1093/bja/75.2.125
86. Lindsay RM, Harmar AJ. Nerve growth factor regulates expression of neuropeptide genes in adult sensory neurons. Nature (1989) 337(6205):362–4. doi: 10.1038/337362a0
87. Guerios SD, Wang ZY, Bjorling DE. Nerve growth factor mediates peripheral mechanical hypersensitivity that accompanies experimental cystitis in mice. Neurosci Lett (2006) 392(3):193–7. doi: 10.1016/j.neulet.2005.09.026
88. Guerios SD, Wang ZY, Boldon K, Bushman W, Bjorling DE. Blockade of NGF and trk receptors inhibits increased peripheral mechanical sensitivity accompanying cystitis in rats. Am J Physiol Regulatory Integr Comp Physiol (2008) 295(1):R111–22. doi: 10.1152/ajpregu.00728.2007
89. Liu HT, Chen CY, Kuo HC. Urinary nerve growth factor levels in overactive bladder syndrome and lower urinary tract disorders. J Formos Med Assoc (2010) 109(12):862–78. doi: 10.1016/S0929-6646(10)60133-7
90. Liu HT, Chen CY, Kuo HC. Urinary nerve growth factor in women with overactive bladder syndrome. BJU Int (2011) 107(5):799–803. doi: 10.1111/j.1464-410X.2010.09585.x
91. Jaggar SI, Scott HC, Rice AS. Inflammation of the rat urinary bladder is associated with a referred thermal hyperalgesia which is nerve growth factor dependent. Br J Anaesth (1999) 83(3):442–8. doi: 10.1093/bja/83.3.442
92. Evans RJ, Moldwin RM, Cossons N, Darekar A, Mills IW, Scholfield D. Proof of concept trial of tanezumab for the treatment of symptoms associated with interstitial cystitis. J Urol (2011) 185(5):1716–21. doi: 10.1016/j.juro.2010.12.088
93. Pinto R, Frias B, Allen S, Dawbarn D, McMahon SB, Cruz F, et al. Sequestration of brain derived nerve factor by intravenous delivery of TrkB-Ig2 reduces bladder overactivity and noxious input in animals with chronic cystitis. Neuroscience (2010) 166(3):907–16. doi: 10.1016/j.neuroscience.2010.01.015
94. Qiao LY, Vizzard MA. Cystitis-induced upregulation of tyrosine kinase (TrkA, TrkB) receptor expression and phosphorylation in rat micturition pathways. J Comp Neurol (2002) 454(2):200–11. doi: 10.1002/cne.10447
95. Pezet S, Cunningham J, Patel J, Grist J, Gavazzi I, Lever IJ, et al. BDNF modulates sensory neuron synaptic activity by a facilitation of GABA transmission in the dorsal horn. Mol Cell Neurosci (2002) 21(1):51–62. doi: 10.1006/mcne.2002.1166
96. Pezet S, Malcangio M, McMahon SB. BDNF: a neuromodulator in nociceptive pathways? Brain Res Brain Res Rev (2002) 40(1-3):240–9. doi: 10.1016/S0165-0173(02)00206-0
97. Pezet S, McMahon SB. Neurotrophins: mediators and modulators of pain. Annu Rev Neurosci (2006) 29:507–38. doi: 10.1146/annurev.neuro.29.051605.112929
98. Michael GJ, Averill S, Nitkunan A, Rattray M, Bennett DL, Yan Q, et al. Nerve growth factor treatment increases brain-derived neurotrophic factor selectively in TrkA-expressing dorsal root ganglion cells and in their central terminations within the spinal cord. J Neurosci (1997) 17(21):8476–90. doi: 10.1523/JNEUROSCI.17-21-08476.1997
99. Almeida AG, Nunes ML, Palmini AL, Costa JC. Incidence of SUDEP in a cohort of patients with refractory epilepsy: The role of surgery and lesion localization. Arq Neuropsiquiatr (2010) 68(6):898–902. doi: 10.1590/S0004-282X2010000600013
100. Ryu JC, Tooke K, Malley SE, Soulas A, Weiss T, Ganesh N, et al. Role of proNGF/p75 signaling in bladder dysfunction after spinal cord injury. J Clin Invest (2018) 128(5):1772–86. doi: 10.1172/JCI97837
101. McMahon SB, Bennett DL, Priestley JV, Shelton DL. The biological effects of endogenous nerve growth factor on adult sensory neurons revealed by a trkA-IgG fusion molecule. Nat Med (1995) 1(8):774–80. doi: 10.1038/nm0895-774
102. Condro MC, Matynia A, Foster NN, Ago Y, Rajbhandari AK, Van C, et al. High-resolution characterization of a PACAP-EGFP transgenic mouse model for mapping PACAP-expressing neurons. J Comp Neurol (2016) 524(18):3827–48. doi: 10.1002/cne.24035
103. May V, Ojala J, Tooke K, Perkins M, Hsiang H, Campbell SE, et al. PACAP/Receptor mechanisms contribute to micturition dysfunction with repeated variate stress (RVS) in mice, in: Akira Arimura. Memorial VIP/PACAP and Related Peptides Symposium: 30 years after PACAP Discovery. (2019)
104. Fuentes IM, Christianson JA. The influence of early life experience on visceral pain. Front Syst Neurosci (2018) 12:2. doi: 10.3389/fnsys.2018.00002
105. Grundy L, Caldwell A, Brierley SM. Mechanisms underlying overactive bladder and interstitial Cystitis/Painful bladder syndrome. Front Neurosci (2018) 12:931. doi: 10.3389/fnins.2018.00931
106. Pierce AN, Christianson JA. Stress and chronic pelvic pain. Prog Mol Biol Transl Sci (2015) 131:509–35. doi: 10.1016/bs.pmbts.2014.11.009
107. Lai H, Gardner V, Vetter J, Andriole GL. Correlation between psychological stress levels and the severity of overactive bladder symptoms. BMC Urol (2015) 15:14. doi: 10.1186/s12894-015-0009-6
108. Sheng JA, Bales NJ, Myers SA, Bautista AI, Roueinfar M, Hale TM, et al. The hypothalamic-Pituitary-Adrenal axis: Development, programming actions of hormones, and maternal-fetal interactions. Front Behav Neurosci (2020) 14:601939. doi: 10.3389/fnbeh.2020.601939
109. Godoy LD, Rossignoli MT, Delfino-Pereira P, Garcia-Cairasco N, de Lima Umeoka EH. A comprehensive overview on stress neurobiology: Basic concepts and clinical implications. Front Behav Neurosci (2018) 12:127. doi: 10.3389/fnbeh.2018.00127
Keywords: flares, IC/BPS, psychological stress, bladder dysfunction, cystometry
Citation: Girard BM, Campbell SE and Vizzard MA (2023) Stress-induced symptom exacerbation: Stress increases voiding frequency, somatic sensitivity, and urinary bladder inflammation when combined with low concentration cyclophosphamide treatment in mice. Front. Urol. 3:1079790. doi: 10.3389/fruro.2023.1079790
Received: 25 October 2022; Accepted: 09 March 2023;
Published: 22 March 2023.
Edited by:
Naoki Yoshimura, University of Pittsburgh, United StatesReviewed by:
Ana Charrua, University of Porto, PortugalCopyright © 2023 Girard, Campbell and Vizzard. This is an open-access article distributed under the terms of the Creative Commons Attribution License (CC BY). The use, distribution or reproduction in other forums is permitted, provided the original author(s) and the copyright owner(s) are credited and that the original publication in this journal is cited, in accordance with accepted academic practice. No use, distribution or reproduction is permitted which does not comply with these terms.
*Correspondence: Margaret A. Vizzard, TWFyZ2FyZXQuVml6emFyZEB1dm0uZWR1
Disclaimer: All claims expressed in this article are solely those of the authors and do not necessarily represent those of their affiliated organizations, or those of the publisher, the editors and the reviewers. Any product that may be evaluated in this article or claim that may be made by its manufacturer is not guaranteed or endorsed by the publisher.
Research integrity at Frontiers
Learn more about the work of our research integrity team to safeguard the quality of each article we publish.