- 1Department of Chemistry, Institute of Biochemistry, NanoGlycobiology Research Group, Universität für Bodenkultur Wien, Vienna, Austria
- 2Competence Center for Periodontal Research, University Clinic of Dentistry, Medical University of Vienna, Vienna, Austria
Tannerella forsythia, a member of the “red complex” bacteria implicated in severe periodontitis, employs various survival strategies and virulence factors to interact with the host. It thrives as a late colonizer in the oral biofilm, relying on its unique adaptation mechanisms for persistence. Essential to its survival are the type 9 protein secretion system and O-glycosylation of proteins, crucial for host interaction and immune evasion. Virulence factors of T. forsythia, including sialidase and proteases, facilitate its pathogenicity by degrading host glycoproteins and proteins, respectively. Moreover, cell surface glycoproteins like the S-layer and BspA modulate host responses and bacterial adherence, influencing colonization and tissue invasion. Outer membrane vesicles and lipopolysaccharides further induce inflammatory responses, contributing to periodontal tissue destruction. Interactions with specific host cell types, including epithelial cells, polymorphonuclear leukocytes macrophages, and mesenchymal stromal cells, highlight the multifaceted nature of T. forsythia's pathogenicity. Notably, it can invade epithelial cells and impair PMN function, promoting dysregulated inflammation and bacterial survival. Comparative studies with periodontitis-associated Porphyromonas gingivalis reveal differences in protease activity and immune modulation, suggesting distinct roles in disease progression. T. forsythia's potential to influence oral antimicrobial defense through protease-mediated degradation and interactions with other bacteria underscores its significance in periodontal disease pathogenesis. However, understanding T. forsythia's precise role in host-microbiome interactions and its classification as a keystone pathogen requires further investigation. Challenges in translating research data stem from the complexity of the oral microbiome and biofilm dynamics, necessitating comprehensive studies to elucidate its clinical relevance and therapeutic implications in periodontitis management.
1 Introduction
Periodontitis is a highly prevalent inflammatory disease affecting the tooth-supporting tissues, impacting more than half of the adult population worldwide. Despite decades of intensive research, the precise etiological factors driving the initiation and progression of this disease remain elusive (1, 2). There is a consensus that periodontitis is triggered by a polymicrobial oral biofilm, with tissue destruction resulting from the host immune response to this biofilm. At the close of the last century, seminal work by Socransky and colleagues identified the “red complex,” comprising Porphyromonas gingivalis, Treponema denticola, and Tannerella forsythia, as associated with severe forms of periodontitis (2). Initially, these three Gram-negative anaerobes were considered as causative agents, but with the advent of sequencing technologies in oral microbiome research, it is now recognized that the etiology of periodontitis is far more complex (3). The progression of periodontitis is characterized by a shift from a symbiotic to a dysbiotic host-microbiome interaction in the oral cavity, driven by various risk factors and marked by an increase in bacterial biomass and alterations in the abundance of specific species (4–6). Notably, the relative abundance of red-complex bacteria significantly rises in subgingival plaque during periodontitis (7). While periodontitis is considered a polymicrobial infection with no sole pathogen, P. gingivalis is believed to play a distinctive role due to its ability to manipulate the host response, thereby tipping the balance from health to disease (5, 8). P. gingivalis has been extensively studied among periodontitis-associated bacteria, but research on other potential pathogens, particularly T. forsythia (Figure 1), remains limited. In this perspective paper, we describe important virulence factors of T. forsythia and explore the bacterium's potential to modulate the host response.
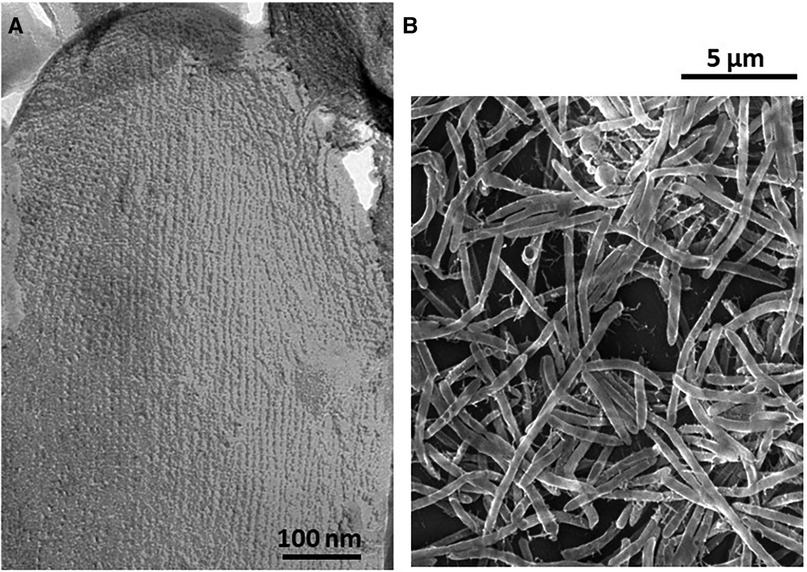
Figure 1. (A) Transmission electron microscopy of a freeze-etched and platinum carbon-shadowed T. forsythia cell showing the square S-layer lattice covering the entire cell surface. (B) Scanning electron microscopy image of rod-shaped T. forsythia cells grown on a hydroxyapatite disc.
2 Survival strategies of Tannerella forsythia
T. forsythia and P. gingivalis are key members of the oral biofilm consortium, thriving as late colonizers (2). These bacteria are affiliated with the Bacteroidetes phylum and share several commonalities in cellular infrastructure, which are vital for their adaptation to the oral habitat and interaction with the host. This includes, e.g., the utilization of a type 9 protein secretion system (T9SS), a characteristic feature of Bacteroidetes members, for the export of specific virulence factors possessing a C-terminal structural targeting domain across the outer membrane (9–11). Additionally, they both have the capability to extensively O-glycosylate numerous proteins with a species-specific glycan (12–14). Deletion of the T9SS signal peptidase PorU in T. forsythia led to T9SS shutdown and reduced production of pro-inflammatory mediators by macrophages and gingival fibroblasts upon infection with the ΔporU deletion mutant compared to the T. forsythia parent strain (10).
O-glycosylation of cell surface proteins has been demonstrated to facilitate the persistence of T. forsythia in the host (15), likely through molecular mimicry of host sialic acid via the display of bacterial nonulosonic acids (16). However, complete immune evasion is not a viable strategy for either T. forsythia or P. gingivalis, as both rely on inflammation to obtain nutrients from tissue breakdown products (5).
In contrast to P. gingivalis, T. forsythia relies on other members of the biofilm consortium for survival due to its auxotrophy for the essential bacterial cell wall sugar N-acetylmuramic acid (MurNAc) (17). Consequently, the bacterium can only survive in the oral habitat by scavenging this compound from cell wall turnover products or decay of cohabiting bacteria (18), making it unique among oral bacteria. Notably, due to the absence of the MurAB enzymes involved in MurNAc biosynthesis, T. forsythia is resistant to the antibiotic fosfomycin (19).
3 Virulence factors of T. forsythia
3.1 Enzymes
3.1.1 Sialidase
The surfaces of vertebrate cells are decorated with a dense array of sialoglycoproteins (20). The red-complex bacteria express sialidases to cleave terminal sialic acid from host glycoproteins, such as mucins, which can be used as a carbon source and/or modify host cell surfaces to enhance attachment (21). T. forsythia harbours a dedicated sialic acid utilization and scavenging (nan) operon (22), encoding a sialidase-NanH-which has been shown to play a crucial role in bacterial colonization by exposing sialic acid-hidden epitopes on epithelial cells (23). Furthermore, attachment and survival of T. forsythia during epithelial KB cell infection were found to be diminished in a mutant lacking the sialic acid transporter NanT (24). Notably, a NanS sialic acid-esterase from T. forsythia collaborates with a P. gingivalis sialidase to optimize P. gingivalis' acquisition of sialic acid, even from diacetylated sialic acid-containing substrates (25). This underscores the interdependencies among the red-complex bacteria for their interaction capability with the host.
3.1.2 Proteases
Various proteases have been implicated in T. forsythia's pathogenicity, including, e.g., PrtH proteases which are associated with attachment loss (26), a trypsin-like cysteine protease exhibiting both arginine- and citrulline-specific activities (27), and secretory KLIKK proteases capable of targeting diverse protein substrates such as collagen, gelatine, elastin and casein (28). It is noteworthy that transcripts of KLIKK proteases were detected in almost all gingival crevicular fluid samples where T. forsythia was present. Specifically, miropin of T. forsythia deactivates a broad range of host proteases, including neutrophil elastases and cathepsin G (28), while karylisin inhibits complement activation at various stages (29). Additionally, mirolysin and karylisin have been shown to degrade and inactivate human cathelicidin (30, 31). These findings support the notion that these enzymes contribute to T. forsythia's pathogenicity through distinct mechanisms.
3.2 Cell surface proteins
3.2.1 2D-crystalline cell surface (S-) layer and glycosylation
T. forsythia cells are covered by a 2D-crystalline array formed by the self-assembly of the S-layer proteins TfsA and TfsB, which are multiply modified by a nonasaccharide within the phylum-wide, three-amino acid O-glycosylation motif D(S/T)(A/I/l/M/T/V/S/C/G/F/N/E/Q/D/P) (12, 32, 33). The S-layer plays a crucial role in host interaction, as demonstrated by various studies utilizing a T. forsythia S-layer ΔtfsAB deletion mutant. Infection with the mutant led to an earlier onset of pro-inflammatory mediator production in macrophages and human gingival fibroblasts compared to the parent strain (34) and induced the production of monocyte chemoattractant protein and granulocyte-macrophage colony-stimulating factor by human oral epithelial cells (35). Furthermore, the T. forsythia S-layer deficient mutant exhibited significantly reduced adherence to human gingival epithelial cells (36).
Moreover, O-glycosylation of the S-layer proteins was found to modulate the interaction between T. forsythia and the host. Specifically, only the native nonasaccharide ensured the bacterium's persistence in the host, whereas mutants with truncated S-layer glycosylation, lacking the nonulosonic acid (37) (e.g., in a ΔwecC deletion mutant lacking a trisaccharide branch), elicited a robust Th17 response and reduced periodontal bone loss in mice compared to the T. forsythia wild-type bacterium (15). Immune priming with the Th17-biasing strain triggered a productive neutrophil response, effectively mitigating P. gingivalis persistence and associated alveolar bone loss in mice (38). Notably, initial evidence suggests that the glycosylated S-layer of T. forsythia is recognized by macrophage-inducible C-type lectin receptors (39), primarily expressed in myeloid cells, such as macrophages and neutrophils.
Regarding T. forsythia's biofilm lifestyle, using a subgingival, multispecies biofilm model, a selective role for the glycosylated S-layer was identified in positioning this bacterium within the biofilm, its co-localization with P. gingivalis, and the prevalence of Campylobacter rectus (40).
Thus, not only the presence of an S-layer but also its specific glycosylation pattern influences the host response to T. forsythia.
3.2.2 Cell surface antigen BspA
T. forsythia's glycosylated cell surface antigen BspA triggers cytokine production in macrophages and dendritic cells via CD14 and TLR2-dependent mechanisms (41, 42); TLR2 activation is mediated through the sequence motif GC(S/T)GLXSIT (43). Animal studies have underscored the pivotal role of BspA in the cellular response to T. forsythia; mice infected by gavage with BspA-deficient T. forsythia displayed significantly reduced alveolar bone loss compared to those infected with the parent strain (43). Bone loss in infected mice was found to be linked to the activation of a Th2 response and notably decreased in animals lacking TLR2, highlighting a potential involvement of BspA in this process (42). Remarkably, the prevalence of the T. forsythia BspA genotype is markedly elevated in patients with chronic and aggressive periodontitis, suggesting a direct contribution of BspA to periodontal disease (44). Additionally, T. forsythia and BspA induce foam cell formation in THP-1 macrophages and promote the progression of atherosclerotic lesions in ApoE(−/−) mice (45), indicating a potential impact of this virulence factor on systemic health.
3.3 Outer membrane vesicles
Outer membrane vesicles (OMVs) from T. forsythia induce the production of TNF-α and IL-8 in U937 macrophages, and IL-6, IL-8, and MCP-1 in human periodontal ligament mesenchymal stromal cells (hPDL-MSCs) in a concentration-dependent manner (46). The response of host cells to T. forsythia OMVs is comparable to or even stronger than that elicited by the whole bacterium (46) and is mediated through TLR2 activation (47). Monocytes and differentiated macrophages may phagocytose T. forsythia's OMVs, leading to the activation of a pro-inflammatory response (48). Interestingly, several cargo proteins within OMVs are virulence factors which are predictably O-glycosylated (46), supporting a potential link between glycosylation and virulence of T. forsythia.
3.4 Rough-type lipopolysaccharide
T. forsythia produces a rough-type lipopolysaccharide (LPS), which induces an inflammatory response in macrophages in a concentration-dependent manner (49, 50). Additionally, another study revealed that T. forsythia LPS stimulates cytokine production in whole blood from periodontitis patients. This response was further increased upon co-stimulation with P. gingivalis LPS or T. denticola LPS, resulting in notably elevated levels of IL-1β and TNF-α (51); this may contribute to the immunodestructive host response characteristic of periodontitis.
4 Cell-specific responses to Tannerella forsythia
4.1 Epithelial cells
The oral epithelium serves a crucial role in the protecting underlying structures against bacterial invasion, functioning as both a mechanical and immune barrier (52). T. forsythia has the ability to invade oral epithelial cells, a process facilitated by, e.g., the activation of phosphatidylinositol 3-kinase by the BspA protein (53, 54). Surface-associated and secreted BspA protein induces the production of IL-8 by these cells through a TLR2 dependent mechanism (55). Moreover, T. forsythia can attach to and invade the oral squamous cell carcinoma cell line H357, likely mediated by NanH sialidase (22). Evidence of T. forsythia's invasion of buccal epithelium has been demonstrated in an in vivo study (56). Interestingly, the bacterium has been found to have no effect on apoptosis in oral epithelial OKF6/hTERT-2 cells, in contrast to P. gingivalis and oral commensal Streptococcus gordonii and Streptococcus sanguinis (57). In the human oral keratinocyte cell line HOK-16B, T. forsythia induces the production of IL-24 mediated by reactive oxygen species (ROS) (58), as well as the activation of IL-1α production through nucleotide-binding oligomerization domain-like receptor protein 10 (59). Additionally, in human oral keratinocytes, T. forsythia stimulates the production of IL-1Rα, IL-8, and vascular endothelial growth factor (VEGF) (35). While T. forsythia slightly inhibited epithelial cell migration as measured in a scratch assay, this inhibition was markedly lower compared to that induced by P. gingivalis and Prevotella nigrescens (60).
4.2 Polymorphonuclear leukocytes
Polymorphonuclear leukocytes (PMNs), integral to the innate immune system, play a crucial role in eliminating invading pathogens through diverse mechanisms such as phagocytosis, ROS production, and neutrophil extracellular trap (NET) formation (61). PMNs represent the predominant immune cell type in the gingival crevice, and their continuous transmigration into the sulcus is essential for maintaining oral health (62). The ability to deactivate neutrophil function is considered a key strategy employed by periodontal pathogens to promote dysregulated inflammation (63, 64). Despite its importance, the specific interaction between T. forsythia and PMNs remains poorly investigated. A study demonstrated that PMNs can phagocytose non-opsonized T. forsythia, leading to ROS production (65). Additionally, T. forsythia has been shown to adhere to and invade dental follicle mesenchymal stem cells, resulting in diminished PMN chemotaxis, phagocytic activity, and NET formation induced by these cells (66). Such alterations in PMN function may contribute to enhanced survival of oral pathogens.
4.3 Macrophages
Macrophages, as phagocytic tissue-resident cells of the innate immune system, are ubiquitous in almost all tissues and undertake various functions, including phagocytosis, pathogen presentation, clearance of cellular debris, and regulation of tissue homeostasis (67–69). T. forsythia, along with its OMVs and LPS, elicits the production of diverse pro-inflammatory mediators, notably IL-1β, TNF-α, IL-6, and IL-8, in human U937 macrophages (10, 34, 46, 50, 70).
In murine macrophages, T. forsythia prompts the dose-dependent production of IL-6, MCP-1, and IL-23 (71). Furthermore, the co-infection of murine macrophages with P. gingivalis and T. forsythia synergistically enhances the production of IL-6, albeit not of other cytokines (71). One study has documented T. forsythia's capability to induce macrophage apoptosis through the activation of caspase-1, concomitant with the release of danger signals, particularly fibronectin and heat-shock protein 60 (72).
4.4 Mesenchymal stromal cells
Mesenchymal stromal cells (MSCs) are widely distributed throughout dental tissues (73). These cells express pattern-recognition receptors and can be activated by various bacterial components (74). Similar to other MSCs, they possess immunomodulatory properties and can influence the activity of various cells. Therefore, dental MSCs are crucial for maintaining tissue homeostasis and play a significant role in the progression of periodontitis (75, 76). Several studies have demonstrated that T. forsythia induces the gene and protein expression of IL-6, IL-8, and MCP-1 in MSCs derived from the gingiva and periodontal ligament (10, 34, 70). A similar effect has been observed with T. forsythia LPS and OMVs (46, 50).
5 Discussion and perspectives
The current understanding of T. forsythia's pathogenicity mechanisms and host-interaction does not conclusively establish its capability to manipulate the host immune system and classify it as a keystone pathogen, similar to P. gingivalis, which is known to significantly influence host-microbiome interactions even when present at low abundance (8, 77). Various virulence factors of P. gingivalis are known to subvert the immune system. For instance, gingipain proteases inhibit local IL-8 accumulation, causing “local chemokine paralysis” that inhibits PMN migration and consequently reduces bacterial killing, contributing to bacterial overgrowth (78, 79). Gingipain proteases also impair T-cell function and tissue colonization through adhesion to epithelial cells (80). Additionally, P. gingivalis affects the host immune response by manipulating complement and TLR2 signaling (81). Further research into T. forsythia's virulence factors and their mode of action is necessary to understand if and how this bacterium can manipulate the host immune system.
Our recent studies have shown contrasting effects of P. gingivalis and T. forsythia wild-type bacteria on gene expression and corresponding content of various pro-inflammatory mediators in human macrophages and gingival and periodontal-ligament derived MSCs. While infection with T. forsythia induced both gene expression and protein content, infection with P. gingivalis resulted in increased gene expression but low levels of cytokines (10, 82). This observation suggests that P. gingivalis proteases have a greater ability to degrade host proteins than T. forsythia enzymes. Animal studies further support this, demonstrating that unlike P. gingivalis, subcutaneous infection of mice with T. forsythia does not inhibit neutrophil migration to the infected site (83).
T. forsythia may potentially affect oral antimicrobial defense through its proteases. Miropin, for instance, degrades neutrophil elastases and cathepsin G, impairing antibacterial neutrophil function (84). Moreover, T. forsythia, along with other red-complex bacteria, degrades cathelicidin, an essential antimicrobial peptide in the oral cavity (85). Additionally, T. forsythia inhibits complement activation at several stages through the metalloproteinase karylisin (29). Interestingly, a clinical study has shown a negative correlation between the prevalence and levels of T. forsythia in subgingival plaque and PMN activities such as phagocytosis and superoxide anion production (86).
There are indications that T. forsythia may alter the host response to other bacteria. Recent studies suggest that T. forsythia may scavenge NOD-2 ligands secreted by F. nucleatum, resulting in diminished activation of oral epithelial cells (87). T. forsythia also inhibits the invasion of P. gingivalis into oral epithelial cells (88) and synergistically augments alveolar bone loss with F. nucleatum in an experimental periodontitis model in mice (89). However, periodontitis models in mice primarily use ligature and infection with P. gingivalis, with limited studies using T. forsythia.
Translating available research data on T. forsythia's interaction with the host immune system is challenging. Most knowledge is based on in-vitro and sometimes mice studies using a model of mono-infection with T. forsythia, while the subgingival plaque contains numerous microorganisms, and host responses involve an orchestrated response of various cell types that is difficult to mimic in the laboratory.
Moreover, it's widely acknowledged that bacterial virulence is heavily influenced by ecological conditions and can be significantly altered within biofilms. For instance, the growth and virulence of various strains of P. gingivalis are contingent upon factors such as temperature, pH, and iron availability (90–92). While ecological conditions in the subgingival pocket may drive the selection of specific T. forsythia strains, data in this regard are limited.
Recent research has uncovered differences in the activation of the chemokine protein IP-10 by THP-1 macrophages between laboratory-adapted T. forsythia ATCC 43037 and clinical isolates (93). Notably, one clinical isolate elicited a notably higher response than T. forsythia ATCC 43037 (93). Additionally, another study highlighted a substantial increase in the prevalence of T. forsythia bspA and prtH genotypes in periodontitis patients compared to healthy individuals. While the underlying reason for this observation remains unclear, it is conceivable that factors such as increased GCF flow and alterations in its composition in periodontitis might drive the selection of specific T. forsythia strains or influence the gene expression and virulence of this bacterium (94).
In summary, T. forsythia expresses several unique virulence factors that affect host defense and immune response (Figure 2). However, it remains unclear if T. forsythia has the potential to manipulate the host response, influence host-microbiome interactions, and promote a dysbiotic state. New studies focusing on the modification of oral biofilm by T. forsythia, the activity of T. forsythia proteases, and their clinical relevance, as well as their effects on the microbial community-host interaction, are necessary.
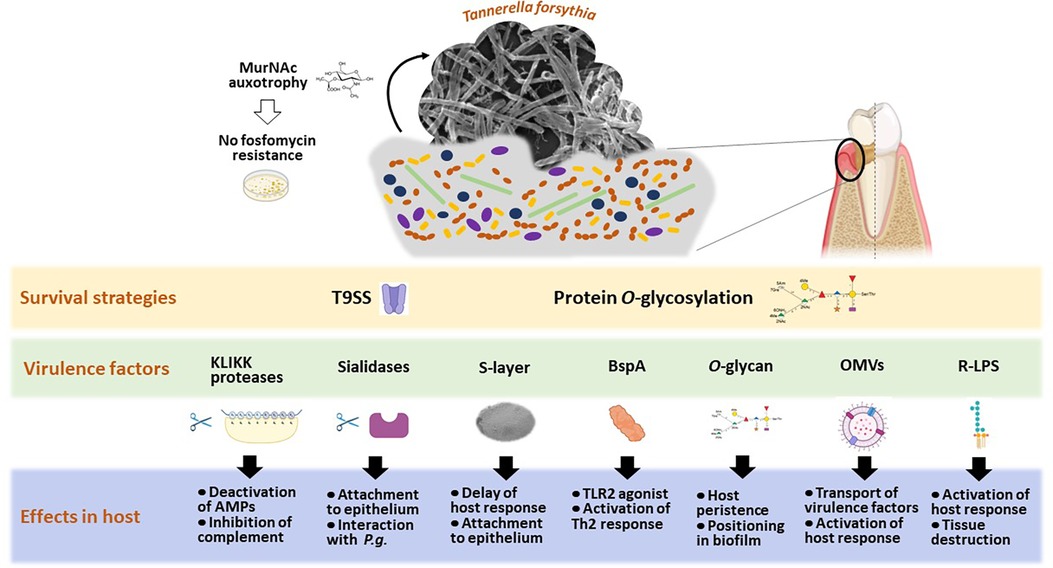
Figure 2. Scheme of Tannerella forsythia's host interaction strategies, illustrating the most investigated means of survival and known virulence factors leading to measurable effects in diverse host cells. Created with Biorender.
Data availability statement
The original contributions presented in the study are included in the article, further inquiries can be directed to the corresponding author.
Author contributions
CS: Conceptualization, Formal Analysis, Funding acquisition, Supervision, Writing – review & editing. OA: Conceptualization, Formal Analysis, Funding acquisition, Supervision, Writing – review & editing.
Funding
The author(s) declare financial support was received for the research, authorship, and/or publication of this article.
This work was funded by the Austrian Science Fund FWF, projects P 20605-B, P 24317-B and P 33618-B (to CS) and P34642-B (to OA and CS). The funder had no role in the design of the study; in the collection, analyses, or interpretation of data; in the writing of the manuscript, or in the decision to publish the results.
Conflict of interest
The authors declare that the research was conducted in the absence of any commercial or financial relationships that could be construed as a potential conflict of interest.
The author(s) declared that they were an editorial board member of Frontiers, at the time of submission. This had no impact on the peer review process and the final decision.
Publisher's note
All claims expressed in this article are solely those of the authors and do not necessarily represent those of their affiliated organizations, or those of the publisher, the editors and the reviewers. Any product that may be evaluated in this article, or claim that may be made by its manufacturer, is not guaranteed or endorsed by the publisher.
References
1. Belibasakis GN, Belstrom D, Eick S, Gursoy UK, Johansson A, Kononen E. Periodontal microbiology and microbial etiology of periodontal diseases: historical concepts and contemporary perspectives. Periodontol 2000. (2023):1–17. doi: 10.1111/prd.12473 [Epub ahead of print].36661184
2. Socransky S, Haffajee A, Cugini M, Smith C, Kent R. Microbial complexes in subgingival plaque. J Clin Periodontol. (1998) 25(2):134–44. doi: 10.1111/j.1600-051X.1998.tb02419.x
3. Zaura E, Pappalardo VY, Buijs MJ, Volgenant CMC, Brandt BW. Optimizing the quality of clinical studies on oral microbiome: a practical guide for planning, performing, and reporting. Periodontol 2000. (2021) 85(1):210–36. doi: 10.1111/prd.12359
4. Abusleme L, Hoare A, Hong BY, Diaz PI. Microbial signatures of health, gingivitis, and periodontitis. Periodontol 2000. (2021) 86(1):57–78. doi: 10.1111/prd.12362
5. Lamont RJ, Hajishengallis G. Polymicrobial synergy and dysbiosis in inflammatory disease. Trends Mol Med. (2015) 21(3):172–83. doi: 10.1016/j.molmed.2014.11.004
6. Van Dyke TE, Bartold PM, Reynolds EC. The nexus between periodontal inflammation and dysbiosis. Fron Immunol. (2020) 11:511. doi: 10.3389/fimmu.2020.00511
7. Griffen AL, Beall CJ, Campbell JH, Firestone ND, Kumar PS, Yang ZK, et al. Distinct and complex bacterial profiles in human periodontitis and health revealed by 16S pyrosequencing. ISME J. (2012) 6(6):1176–85. doi: 10.1038/ismej.2011.191
8. Hajishengallis G, Lamont RJ. Beyond the red complex and into more complexity: the polymicrobial synergy and dysbiosis (PSD) model of periodontal disease etiology. Mol Oral Microbiol. (2012) 27(6):409–19. doi: 10.1111/j.2041-1014.2012.00663.x
9. Tomek MB, Neumann L, Nimeth I, Koerdt A, Andesner P, Messner P, et al. The S-layer proteins of Tannerella forsythia are secreted via a type IX secretion system that is decoupled from protein O-glycosylation. Mol Oral Microbiol. (2014) 29(6):307–20. doi: 10.1111/omi.12062
10. Braun ML, Tomek MB, Grünwald-Gruber C, Nguyen PQ, Bloch S, Potempa JS, et al. Shut-down of type IX protein secretion alters the host immune response to Tannerella forsythia and Porphyromonas gingivalis. Front Cell Infect Microbiol. (2022) 12:835509. doi: 10.3389/fcimb.2022.835509
11. Benedyk M, Marczyk A, Chruscicka B. Type IX secretion system is pivotal for expression of gingipain-associated virulence of Porphyromonas gingivalis. Mo Oral Microbiol. (2019) 34(6):237–44. doi: 10.1111/omi.12268
12. Posch G, Pabst M, Brecker L, Altmann F, Messner P, Schäffer C. Characterization and scope of S-layer protein O-glycosylation in Tannerella forsythia. J Biol Chem. (2011) 286(44):38714–24. doi: 10.1074/jbc.M111.284893
13. Veith PD, Scott NE, Reynolds EC. Characterization of the O-glycoproteome of Tannerella forsythia. mSphere. (2021) 6(5):e0064921. doi: 10.1128/mSphere.00649-21
14. Veith PD, Shoji M, Scott NE, Reynolds EC. Characterization of the O-glycoproteome of Porphyromonas gingivalis. Microbiol Spectr. (2022) 10(1):e0150221. doi: 10.1128/spectrum.01502-21
15. Settem RP, Honma K, Nakajima T, Phansopa C, Roy S, Stafford GP, et al. A bacterial glycan core linked to surface (S)-layer proteins modulates host immunity through Th17 suppression. Mucosal Iimmunol. (2013) 6(2):415–26. doi: 10.1038/mi.2012.85
16. Bloch S, Tomek MB, Friedrich V, Messner P, Schäffer C. Nonulosonic acids contribute to the pathogenicity of the oral bacterium Tannerella forsythia. Interface Focus. (2019) 9(2):20180064. doi: 10.1098/rsfs.2018.0064
17. Wyss C. Dependence of proliferation of Bacteroides forsythus on exogenous N-acetylmuramic acid. Infect Immun. (1989) 57(6):1757–9. doi: 10.1128/iai.57.6.1757-1759.1989
18. Hottmann I, Borisova M, Schäffer C, Mayer C. Peptidoglycan salvage enables the periodontal pathogen Tannerella forsythia to survive within the oral microbial community. Microb Physiol. (2021): 31(2):123–34. doi: 10.1159/000516751
19. Gisin J, Schneider A, Nägele B, Borisova M, Mayer C. A cell wall recycling shortcut that bypasses peptidoglycan de novo biosynthesis. Nat Chem Biol. (2013) 9(8):491–3. doi: 10.1038/nchembio.1289
20. Varki A. Sialic acids in human health and disease. Trends Mol Med. (2008) 14(8):351–60. doi: 10.1016/j.molmed.2008.06.002
21. Jennings MP, Day CJ, Atack JM. How bacteria utilize sialic acid during interactions with the host: snip, snatch, dispatch, match and attach. Microbiology. (2022) 168(3):001157. doi: 10.1099/mic.0.001157
22. Frey AM, Satur MJ, Phansopa C, Parker JL, Bradshaw D, Pratten J, et al. Evidence for a carbohydrate-binding module (CBM) of Tannerella forsythia NanH sialidase, key to interactions at the host-pathogen interface. Biochem J. (2018) 475(6):1159–76. doi: 10.1042/BCJ20170592
23. Honma K, Mishima E, Sharma A. Role of Tannerella forsythia NanH sialidase in epithelial cell attachment. Infec Immun. (2011) 79(1):393–401. doi: 10.1128/IAI.00629-10
24. Honma K, Ruscitto A, Frey AM, Stafford GP, Sharma A. Sialic acid transporter NanT participates in Tannerella forsythia biofilm formation and survival on epithelial cells. Microb Pathogen. (2016) 94:12–20. doi: 10.1016/j.micpath.2015.08.012
25. Frey AM, Satur MJ, Phansopa C, Honma K, Urbanowicz PA, Spencer DIR, et al. Characterization of Porphyromonas gingivalis sialidase and disruption of its role in host-pathogen interactions. Microbiology. (2019) 165(11):1181–97. doi: 10.1099/mic.0.000851
26. Hamlet SM, Ganashan N, Cullinan MP, Westerman B, Palmer JE, Seymour GJ. A 5-year longitudinal study of Tannerella forsythia prtH genotype: association with loss of attachment. J Periodontol. (2008) 79(1):144–9. doi: 10.1902/jop.2008.070228
27. Song Q, Zhang X, Li N, Shen J, Cheng J. A propeptide-independent protease from Tannerella sp.6_1_58FAA_CT1 displays trypsin-like specificity. J Basic Microbiol. (2017) 57(1):50–6. doi: 10.1002/jobm.201600486
28. Ksiazek M, Mizgalska D, Eick S, Thøgersen IB, Enghild JJ, Potempa J. KLIKK Proteases of Tannerella forsythia: putative virulence factors with a unique domain structure. Front Microbiol. (2015) 6:312. doi: 10.3389/fmicb.2015.00312
29. Jusko M, Potempa J, Karim AY, Ksiazek M, Riesbeck K, Garred P, et al. A metalloproteinase karilysin present in the majority of Tannerella forsythia isolates inhibits all pathways of the complement system. J Immunol. (2012) 188(5):2338–49. doi: 10.4049/jimmunol.1101240
30. Koneru L, Ksiazek M, Waligorska I, Straczek A, Lukasik M, Madej M, et al. Mirolysin, a LysargiNase from Tannerella forsythia, proteolytically inactivates the human cathelicidin, LL-37. Biol Chem. (2017) 398(3):395–409. doi: 10.1515/hsz-2016-0267
31. Koziel J, Karim AY, Przybyszewska K, Ksiazek M, Rapala-Kozik M, Nguyen KA, et al. Proteolytic inactivation of LL-37 by karilysin, a novel virulence mechanism of Tannerella forsythia. J Innate Immun. (2010) 2(3):288–93. doi: 10.1159/000281881
32. Sekot G, Posch G, Oh YJ, Zayni S, Mayer HF, Pum D, et al. Analysis of the cell surface layer ultrastructure of the oral pathogen Tannerella forsythia. Arch Microbiol. (2012) 194(6):525–39. doi: 10.1007/s00203-012-0792-3
33. Ye X, Paul B, Mo J, Reynolds EC, Ghosal D, Veith PD. Ultrastructural and glycoproteomic characterization of Prevotella intermedia: insights into O-glycosylation and outer membrane vesicles. MicrobiologyOpen. (2024) 13(2):e1401. doi: 10.1002/mbo3.1401
34. Sekot G, Posch G, Messner P, Matejka M, Rausch-Fan X, Andrukhov O, et al. Potential of the Tannerella forsythia S-layer to delay the immune response. J Dent Res. (2011) 90(1):109–14. doi: 10.1177/0022034510384622
35. Bloch S, Zwicker S, Bostanci N, Sjöling A, Bostrom EA, Belibasakis GN, et al. Immune response profiling of primary monocytes and oral keratinocytes to different Tannerella forsythia strains and their cell surface mutants. Mol Oral Microbiol. (2018) 33(2):155–67. doi: 10.1111/omi.12208
36. Sakakibara J, Nagano K, Murakami Y, Higuchi N, Nakamura H, Shimozato K, et al. Loss of adherence ability to human gingival epithelial cells in S-layer protein-deficient mutants of Tannerella forsythensis. Microbiology. (2007) 153(Pt 3):866–76. doi: 10.1099/mic.0.29275-0
37. Tomek MB, Maresch D, Windwarder M, Friedrich V, Janesch B, Fuchs K, et al. A general protein O-glycosylation gene cluster encodes the species-specific glycan of the oral pathogen Tannerella forsythia: o-glycan biosynthesis and immunological implications. Front Microbiol. (2018) 9:2008. doi: 10.3389/fmicb.2018.02008
38. Settem RP, Honma K, Sharma A. Neutrophil mobilization by surface-glycan altered th17-skewing bacteria mitigates periodontal pathogen persistence and associated alveolar bone loss. PloS One. (2014) 9(9):e108030. doi: 10.1371/journal.pone.0108030
39. Chinthamani S, Settem RP, Honma K, Kay JG, Sharma A. Macrophage inducible C-type lectin (Mincle) recognizes glycosylated surface (S)-layer of the periodontal pathogen Tannerella forsythia. PloS One. (2017) 12(3):e0173394. doi: 10.1371/journal.pone.0173394
40. Bloch S, Thurnheer T, Murakami Y, Belibasakis GN, Schäffer C. Behavior of two Tannerella forsythia strains and their cell surface mutants in multispecies oral biofilms. Mol Oral Microbiol. (2017) 32(5):404–18. doi: 10.1111/omi.12182
41. Hajishengallis G, Martin M, Sojar HT, Sharma A, Schifferle RE, DeNardin E, et al. Dependence of bacterial protein adhesins on toll-like receptors for proinflammatory cytokine induction. Clin Diagn Lab Immunol. (2002) 9(2):403–11. doi: 10.1128/cdli.9.2.403-411.2002
42. Myneni SR, Settem RP, Connell TD, Keegan AD, Gaffen SL, Sharma A. TLR2 Signaling and Th2 responses drive Tannerella forsythia-induced periodontal bone loss. J Immunol. (2011) 187(1):501–9. doi: 10.4049/jimmunol.1100683
43. Myneni SR, Settem RP, Sojar HT, Malone JP, Loimaranta V, Nakajima T, et al. Identification of a unique TLR2-interacting peptide motif in a microbial leucine-rich repeat protein. Biochem Biophys Res Commun. (2012) 423(3):577–82. doi: 10.1016/j.bbrc.2012.06.008
44. Mahalakshmi K, Krishnan P, Chandrasekaran SC. Detection of Tannerella forsythia bspA and prtH genotypes among periodontitis patients and healthy subjects-A case-control study. Arch Oral Biol. (2018) 96:178–81. doi: 10.1016/j.archoralbio.2018.09.012
45. Lee HR, Jun HK, Choi BK. Tannerella forsythia BspA increases the risk factors for atherosclerosis in ApoE(−/−) mice. Oral Dis. (2014) 20(8):803–8. doi: 10.1111/odi.12214
46. Friedrich V, Gruber C, Nimeth I, Pabinger S, Sekot G, Posch G, et al. Outer membrane vesicles of Tannerella forsythia: biogenesis, composition, and virulence. Mol Oral Microbiol. (2015) 30(6):451–73. doi: 10.1111/omi.12104
47. Lim Y, Kim HY, Han D, Choi BK. Proteome and immune responses of extracellular vesicles derived from macrophages infected with the periodontal pathogen Tannerella forsythia. J Extracell Vesicles. (2023) 12(12):e12381. doi: 10.1002/jev2.12381
48. Cecil JD, O'Brien-Simpson NM, Lenzo JC, Holden JA, Singleton W, Perez-Gonzalez A, et al. Outer membrane vesicles prime and activate macrophage inflammasomes and cytokine secretion in vitro and in vivo. Front Immunol. (2017) 8:1017. doi: 10.3389/fimmu.2017.01017
49. Bodet C, Chandad F, Grenier D. Inflammatory responses of a macrophage/epithelial cell co-culture model to mono and mixed infections with Porphyromonas gingivalis, Treponema denticola, and Tannerella forsythia. Microbes Infect. (2006) 8(1):27–35. doi: 10.1016/j.micinf.2005.05.015
50. Posch G, Andrukhov O, Vinogradov E, Lindner B, Messner P, Holst O, et al. Structure and immunogenicity of the rough-type lipopolysaccharide from the periodontal pathogen Tannerella forsythia. Clin Vacc Immunol. (2013) 20(6):945–53. doi: 10.1128/CVI.00139-13
51. Bodet C, Grenier D. Synergistic effects of lipopolysaccharides from periodontopathic bacteria on pro-inflammatory cytokine production in an ex vivo whole blood model. Mol Oral Microbiol. (2010) 25(2):102–11. doi: 10.1111/j.2041-1014.2010.00566.x
52. Groeger S, Meyle J. Oral mucosal epithelial cells. Front Immunol. (2019) 10:208. doi: 10.3389/fimmu.2019.00208
53. Mishima E, Sharma A. Tannerella forsythia invasion in oral epithelial cells requires phosphoinositide 3-kinase activation and clathrin-mediated endocytosis. Microbiology. (2011) 157(Pt 8):2382–91. doi: 10.1099/mic.0.048975-0
54. Inagaki S, Onishi S, Kuramitsu HK, Sharma A. Porphyromonas gingivalis vesicles enhance attachment, and the leucine-rich repeat BspA protein is required for invasion of epithelial cells by Tannerella forsythia. Infect Immun. (2006) 74(9):5023–8. doi: 10.1128/IAI.00062-06
55. Onishi S, Honma K, Liang S, Stathopoulou P, Kinane D, Hajishengallis G, et al. Toll-like receptor 2-mediated interleukin-8 expression in gingival epithelial cells by the Tannerella forsythia leucine-rich repeat protein BspA. Infect Immun. (2008) 76(1):198–205. doi: 10.1128/iai.01139-07
56. Leung NM, Chen R, Rudney JD. Oral bacteria in plaque and invading buccal cells of young orthodontic patients. Am J Orthod Dentofac. (2006) 130(6):698e11–8. doi: 10.1016/j.ajodo.2006.05.028
57. White T, Alimova Y, Alves VTE, Emecen-Huja P, Al-Sabbagh M, Villasante A, et al. Oral commensal bacteria differentially modulate epithelial cell death. Arch Oral Biol. (2020) 120:104926. doi: 10.1016/j.archoralbio.2020.104926
58. Ko YK, An SJ, Han NY, Lee H, Choi BK. Regulation of IL-24 in human oral keratinocytes stimulated with Tannerella forsythia. Mol Oral Microbiol. (2019) 34(5):209–18. doi: 10.1111/omi.12265
59. Lee SJ, Choi BK. Involvement of NLRP10 in IL-1alpha induction of oral epithelial cells by periodontal pathogens. Innate Immun. (2017) 23(7):569–77. doi: 10.1177/1753425917722610
60. Laheij AM, de Soet JJ, Veerman EC, Bolscher JG, van Loveren C. The influence of oral bacteria on epithelial cell migration in vitro. Med Inflamm. (2013) 2013:154532. doi: 10.1155/2013/154532
61. Chapple ILC, Hirschfeld J, Kantarci A, Wilensky A, Shapira L. The role of the host-neutrophil biology. Periodontol 2000. (2023) 00:1–47. doi: 10.1111/prd.12490
62. Rijkschroeff P, Loos BG, Nicu EA. Oral polymorphonuclear neutrophil contributes to oral health. Cur Oral Health Rep. (2018) 5(4):211–20. doi: 10.1007/s40496-018-0199-6
63. Miralda I, Uriarte SM. Periodontal pathogens’ strategies disarm neutrophils to promote dysregulated inflammation. Mol Oral Microbiol. (2021) 36(2):103–20. doi: 10.1111/omi.12321
64. Prucsi Z, Plonczynska A, Potempa J, Sochalska M. Uncovering the oral dysbiotic microbiota as masters of neutrophil responses in the pathobiology of periodontitis. Front Microbiol. (2021) 12:729717. doi: 10.3389/fmicb.2021.729717
65. Moriguchi K, Hasegawa Y, Higuchi N, Murakami Y, Yoshimura F, Nakata K, et al. Energy dispersive spectroscopy-scanning transmission electron microscope observations of free radical production in human polymorphonuclear leukocytes phagocytosing non-opsonized Tannerella forsythia. Microsc Res Tech. (2017) 80(6):555–62. doi: 10.1002/jemt.22819
66. Hieke C, Kriebel K, Engelmann R, Muller-Hilke B, Lang H, Kreikemeyer B. Human dental stem cells suppress PMN activity after infection with the periodontopathogens Prevotella intermedia and Tannerella forsythia. Sci Rep. (2016) 6:39096. doi: 10.1038/srep39096
67. Gordon S, Taylor PR. Monocyte and macrophage heterogeneity. Nat Rev Immunol. (2005) 5(12):953–64. doi: 10.1038/nri1733
68. Ogle ME, Segar CE, Sridhar S, Botchwey EA. Monocytes and macrophages in tissue repair: implications for immunoregenerative biomaterial design. Exp Biol Med. (2016) 241(10):1084–97. doi: 10.1177/1535370216650293
69. Parihar A, Eubank TD, Doseff AI. Monocytes and macrophages regulate immunity through dynamic networks of survival and cell death. J Innate Immun. (2010) 2(3):204–15. doi: 10.1159/000296507
70. Kendlbacher FL, Bloch S, Hager-Mair FF, Bacher J, Janesch B, Thurnheer T, et al. Multispecies biofilm behavior and host interaction support the association of Tannerella serpentiformis with periodontal health. Mol Oral Microbiol. (2023) 38(2):115–33. doi: 10.1111/omi.12385
71. Tamai R, Deng X, Kiyoura Y. Porphyromonas gingivalis with either Tannerella forsythia or Treponema denticola induces synergistic IL-6 production by murine macrophage-like J774.1 cells. Anaerobe. (2009) 15(3):87–90. doi: 10.1016/j.anaerobe.2008.12.004
72. Jun HK, Jung YJ, Choi BK. Treponema denticola, Porphyromonas gingivalis, and Tannerella forsythia induce cell death and release of endogenous danger signals. Arch Oral Biol. (2017) 73:72–8. doi: 10.1016/j.archoralbio.2016.09.010
73. Chen Y, Huang H, Li G, Yu J, Fang F, Qiu W. Dental-derived mesenchymal stem cell sheets: a prospective tissue engineering for regenerative medicine. Stem Cell Res Ther. (2022) 13(1):38. doi: 10.1186/s13287-022-02716-3
74. Andrukhov O. Toll-Like receptors and dental mesenchymal stromal cells. Front Oral Health. (2021) 2:648901. doi: 10.3389/froh.2021.648901
75. Andrukhov O, Behm C, Blufstein A, Rausch-Fan X. Immunomodulatory properties of dental tissue-derived mesenchymal stem cells: implication in disease and tissue regeneration. World J Stem Cells. (2019) 11(9):604–17. doi: 10.4252/wjsc.v11.i9.604
76. Poblano-Perez LI, Castro-Manrreza ME, Gonzalez-Alva P, Fajardo-Orduna GR, Montesinos JJ. Mesenchymal stromal cells derived from dental tissues: immunomodulatory properties and clinical potential. Int J Mol Sci. (2024) 25(4):1986. doi: 10.3390/ijms25041986
77. Darveau RP, Hajishengallis G, Curtis MA. Porphyromonas gingivalis as a potential community activist for disease. J Dent Res. (2012) 91(9):816–20. doi: 10.1177/0022034512453589
78. Darveau RP, Belton CM, Reife RA, Lamont RJ. Local chemokine paralysis, a novel pathogenic mechanism for Porphyromonas gingivalis. Infec Immun. (1998) 66(4):1660–5. doi: 10.1128/iai.66.4.1660-1665.1998
79. Hajishengallis G, Lamont RJ. Breaking bad: manipulation of the host response by Porphyromonas gingivalis. Eur J Immunol. (2014) 44(2):328–38. doi: 10.1002/eji.201344202
80. Katz J, Yang QB, Zhang P, Potempa J, Travis J, Michalek SM, et al. Hydrolysis of epithelial junctional proteins by Porphyromonas gingivalis gingipains. Infect Immun. (2002) 70(5):2512–8. doi: 10.1128/IAI.70.5.2512-2518.2002
81. Maekawa T, Krauss JL, Abe T, Jotwani R, Triantafilou M, Triantafilou K, et al. Porphyromonas gingivalis manipulates complement and TLR signaling to uncouple bacterial clearance from inflammation and promote dysbiosis. Cell Host Microbe. (2014) 15(6):768–78. doi: 10.1016/j.chom.2014.05.012
82. Kendlbacher FL, Bloch S, Hager-Mair FF, Schäffer C, Andrukhov O. Red-complex bacteria exhibit distinctly different interactions with human periodontal ligament stromal cells compared to Fusobacterium nucleatum. Arch Oral Biol. (2024):106004. doi: 10.1016/j.archoralbio.2024.106004. [Epub ahead print].38776586
83. Gosling PT, Gemmell E, Carter CL, Bird PS, Seymour GJ. Immunohistological analysis of Tannerella forsythia-induced lesions in a murine model. Oral Microb Immunol. (2005) 20(1):25–30. doi: 10.1111/j.1399-302X.2004.00188.x
84. Ksiazek M, Mizgalska D, Enghild JJ, Scavenius C, Thogersen IB, Potempa J. Miropin, a novel bacterial serpin from the periodontopathogen Tannerella forsythia, inhibits a broad range of proteases by using different peptide bonds within the reactive center loop. J Biol Chem. (2015) 290(1):658–70. doi: 10.1074/jbc.M114.601716
85. Puklo M, Guentsch A, Hiemstra PS, Eick S, Potempa J. Analysis of neutrophil-derived antimicrobial peptides in gingival crevicular fluid suggests importance of cathelicidin LL-37 in the innate immune response against periodontogenic bacteria. Oral Mircobiol Immunol. (2008) 23(4):328–35. doi: 10.1111/j.1399-302X.2008.00433.x
86. Carvalho RP, Mesquita JS, Bonomo A, Elsas PX, Colombo AP. Relationship of neutrophil phagocytosis and oxidative burst with the subgingival microbiota of generalized aggressive periodontitis. Oral Microb Immunol. (2009) 24(2):124–32. doi: 10.1111/j.1399-302X.2008.00484.x
87. Settem RP, Ruscitto A, Chinthamani S, Honma K, Sharma A. Tannerella forsythia scavenges Fusobacterium nucleatum secreted NOD2 stimulatory molecules to dampen oral epithelial cell inflammatory response. Mol Oral Microbiol. (2024) 39(2):40–6. doi: 10.1111/omi.12429
88. Saito A, Inagaki S, Ishihara K. Differential ability of periodontopathic bacteria to modulate invasion of human gingival epithelial cells by Porphyromonas gingivalis. Microb Pathoge. (2009) 47(6):329–33. doi: 10.1016/j.micpath.2009.09.012
89. Settem RP, El-Hassan AT, Honma K, Stafford GP, Sharma A. Fusobacterium nucleatum and Tannerella forsythia induce synergistic alveolar bone loss in a mouse periodontitis model. Infect Immun. (2012) 80(7):2436–43. doi: 10.1128/iai.06276-11
90. Curtis MA, Zenobia C, Darveau RP. The relationship of the oral microbiotia to periodontal health and disease. Cell Host Microbe. (2011) 10(4):302–6. doi: 10.1016/j.chom.2011.09.008
91. Kesavalu L, Holt SC, Ebersole JL. In vitro environmental regulation of Porphyromonas gingivalis growth and virulence. Oral Microb Immunol. (2003) 18(4):226–33. doi: 10.1034/j.1399-302x.2003.00071.x
92. Sharaf S, Hijazi K. Modulatory mechanisms of pathogenicity in Porphyromonas gingivalis and other periodontal pathobionts. Microorganisms. (2022) 11(1):15. doi: 10.3390/microorganisms11010015
93. Chinthamani S, Settem RP, Honma K, Stafford GP, Sharma A. Tannerella forsythia strains differentially induce interferon gamma-induced protein 10 (IP-10) expression in macrophages due to lipopolysaccharide heterogeneity. Pathogens Dis. (2022) 80(1):ftac008. doi: 10.1093/femspd/ftac008
Keywords: host response, inflammatory mediators, protein O-glycosylation, S-layer, Tannerella forsythia, virulence factors
Citation: Schäffer C and Andrukhov O (2024) The intriguing strategies of Tannerella forsythia's host interaction. Front. Oral. Health 5:1434217. doi: 10.3389/froh.2024.1434217
Received: 17 May 2024; Accepted: 20 May 2024;
Published: 30 May 2024.
Edited by:
Georgios N. Belibasakis, Karolinska Institutet (KI), SwedenReviewed by:
George Hajishengallis, University of Pennsylvania, United States© 2024 Schäffer and Andrukhov. This is an open-access article distributed under the terms of the Creative Commons Attribution License (CC BY). The use, distribution or reproduction in other forums is permitted, provided the original author(s) and the copyright owner(s) are credited and that the original publication in this journal is cited, in accordance with accepted academic practice. No use, distribution or reproduction is permitted which does not comply with these terms.
*Correspondence: Oleh Andrukhov, b2xlaC5hbmRydWtob3ZAbWVkdW5pd2llbi5hYy5hdA==