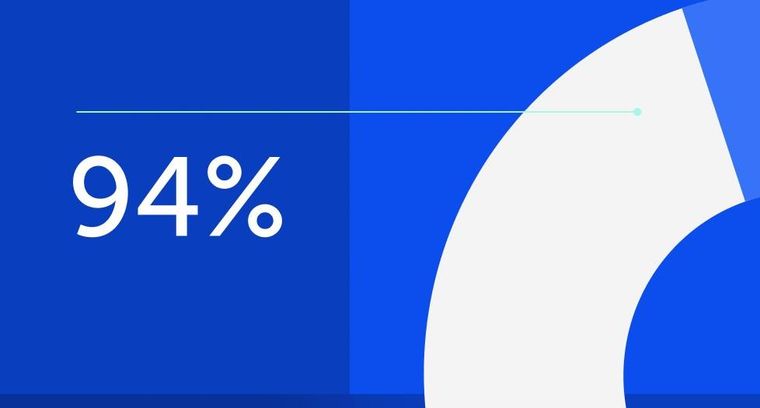
94% of researchers rate our articles as excellent or good
Learn more about the work of our research integrity team to safeguard the quality of each article we publish.
Find out more
ORIGINAL RESEARCH article
Front. Oral. Health, 28 February 2022
Sec. Oral Infections and Microbes
Volume 3 - 2022 | https://doi.org/10.3389/froh.2022.847914
This article is part of the Research TopicWomen in Oral Infections and Microbes: 2021View all 5 articles
Porphyromonas gingivalis is a key pathogen of periodontitis, an inflammatory disease that affects the tooth-supporting tissues. The aim of the present study was to investigate the effects of the flavanone eriodictyol on P. gingivalis-induced reactive oxygen species (ROS) production by gingival keratinocytes and the inflammatory response of macrophages. Porphyromonas gingivalis and H2O2 acted synergistically to induce ROS production by keratinocytes. The presence of eriodictyol significantly attenuated ROS production in a dose-dependent manner. We used a macrophage model to show that eriodictyol decreases the secretion of IL-1β, IL-6, IL-8, and TNF-α induced by P. gingivalis. Evidence has been brought that this anti-inflammatory property of eriodictyol may be related to its ability to prevent the activation of the NF-κB signaling pathway by P. gingivalis. This periodontal pathogen was also found to be a potent inducer of matrix metalloproteinase (MMP) production by macrophages, including MMP-2, MMP-8, and MMP-9. Eriodictyol dose-dependently inhibited the production of all three MMPs. Lastly, eriodictyol inhibited the catalytic activity of both MMP-9 and P. gingivalis collagenase. In conclusion, eriodictyol may be a potential therapeutic agent for preventing and/or treating periodontal disease due to its antioxidant, anti-inflammatory, and anti-proteinase properties.
Periodontal disease is a current public health problem worldwide that alters the quality of life of patients. More specifically, periodontitis, a destructive form of periodontal disease, affects nearly 10% of the USA adult population [1]. Periodontitis is defined as an inflammatory disorder of the periodontium triggered by specific anaerobic Gram-negative bacteria, called periodontal pathogens, that colonize the subgingival areas [2, 3]. If left untreated, periodontitis can lead to the destruction of tooth-supporting connective tissue and alveolar bone, leading to tooth loss. In addition to the damage caused in the oral cavity, the etiological factors of periodontitis have additional impacts on the whole human body. Associations between periodontitis and cardiovascular diseases, type 2 diabetes, neurodegenerative diseases, and adverse pregnancy outcomes have been reported by numerous studies [4–6].
In a healthy periodontium, the immune response of mucosal and infiltrating immune cells triggered by periodontal pathogens in the dental biofilm is moderate and protective [7, 8]. More specifically, macrophages and monocytes play a key role in host defenses against periodontal infections and contribute to the initiation of an adaptive immune response to periodontal pathogens such as P. gingivalis [9, 10]. However, if periodontal pathogens proliferate and increase in number, a state of dysbiosis develops in the subgingival sites, inducing an exaggerated inflammatory response, which leads to the secretion of cytokines and matrix metalloproteinases (MMPs) that sustain the chronic inflammatory reaction and modulate connective tissue and alveolar bone destruction [11]. The NF-κB signaling pathway, which consists of the NF-κB p50/p65 heterodimer and is inhibited by the suppressive protein inhibitor kappa B (IκB), plays a central role inflammatory mediator secretion [2, 7, 8]. Moreover, reactive oxygen species (ROS) production by resident and inflammatory cells of the periodontium is drastically increased during periodontitis and antioxidant host defense system cannot balance this process resulting in an oxidative stress condition and tissue damage [12].
Porphyromonas gingivalis, an anaerobic Gram-negative bacterium, is a key pathogen in chronic periodontitis [13, 14]. The presence of this bacterium in subgingival biofilm is associated with gingival bleeding, an increase in the depth of periodontal pockets, and an intensification of bone resorption [14]. Owing to the contribution of its numerous virulence factors [14–17], P. gingivalis can invade the gingival mucosa where it can elicit an inflammatory response following macrophage infiltration [18, 19].
Natural molecules isolated from plants may be promising alternatives to synthetic chemicals for the management of periodontal disease [20, 21]. Eriodictyol {Figure 1; [(S)-2-(3,4-dihydroxyphenyl)-5,7-dihydroxy-2,3-dihydrochromen-4-one]} is a flavonoid belonging to the flavanone subclass and is mainly found in the traditional medicinal plant yerba santa (Eriodictyon californicum) as well as in citrus fruits and vegetables [22, 23]. Studies carried out over the past decade have shown that eriodictyol exibits a number of therapeutic properties, including antioxidant and anti-inflammatory effects [22, 23]. The therapeutic properties of eriodictyol appear to depend on its capacity to modulate several cell signaling pathways [22, 23]. Although a recent study showed that food supplementation with eriodictyol could prevent periodontal disease in a mouse model [24], to the best of our knowledge, there are no reports in the literature that have addressed the exact underlying mechanisms of the potential beneficial effect of eriodictyol on periodontitis. In the present study, we investigated the effects of eriodictyol on P. gingivalis-induced ROS production by gingival keratinocytes and on the inflammatory response of macrophages.
Eriodictyol {Figure 1; [(S)-2-(3,4-dihydroxyphenyl)-5,7-dihydroxy-2,3-dihydrochromen-4-one]} was prepared at the USDA-ARS Horticultural Research Laboratory (Fort Pierce, Florida, USA). A stock solution was prepared in dimethylsulfoxide (DMSO) at a concentration of 1 M and was kept at 4°C in the dark. The final concentration of DMSO in the assays described below was ≤ 0.1% and had no effects on gingival keratinocytes and macrophages.
Porphyromonas gingivalis ATCC 33277 was cultivated in an anaerobic chamber (80% N2, 10% CO2, 10% H2) at 37°C in Todd-Hewitt broth (THB; Becton Dickinson and Company, Sparks, MD, USA) containing 0.001% (w/v) hemin and 0.0001% (w/v) vitamin K.
The previously characterized B11 human gingival keratinocyte cell line [25] was cultivated in keratinocyte serum-free medium (K-SFM; Life Technologies Inc., Burlington, ON, Canada) supplemented with 50 μg/mL of bovine pituitary extract, 5 ng/mL of human epidermal growth factor, 100 μg/mL of penicillin G-streptomycin, and 0.5 μg/mL of amphotericin B. Gingival keratinocytes (3 × 105 cells/well) were seeded in black wall, clear flat bottom 96-well microplates (Greiner Bio-One North America, Monroe, NC, USA) and were incubated overnight at 37°C in a 5% CO2 atmosphere. ROS production was measured using a fluorometric assay to monitor the oxidation of 2′,7′-dichlorofluorescein-diacetate (DCF-DA; Sigma-Aldrich Canada Co., Oakville, ON, Canada) into a fluorescent compound. DCF-DA was freshly prepared in DMSO at a concentration of 40 mM. The cells were washed with Hank's balanced salt solution (HBSS; HyClone Laboratories, Logan, UT, USA) and were incubated for a further 30 min in the presence of 100 μM DCF-DA in HBSS. Excess DCF-DA was discarded, and the keratinocytes were washed with HBSS. The cells were then treated with P. gingivalis cells at a multiplicity of infection (MOI) of 100 either in the presence or absence of hydrogen peroxide (H2O2; 1 mM) and in the absence or presence of eriodictyol (40, 80, or 160 μM) in HBSS. H2O2 was used as an inducer of oxidative stress [26, 27]. The fluorescence emission indicating ROS production was then recorded every 20 min for 3 h at 37°C using a Synergy 2 microplate reader (BioTek Instruments, Winooski, VT, USA) with a 485 nm excitation filter and a 528 nm emission filter. Assays were carried out in triplicate in three independent experiments, and the means ± standard deviations were calculated.
To assess the effect of eriodictyol on the adhesion of P. gingivalis to gingival keratinocytes (B11 cell line), the bacteria were first labeled with fluorescein isothiocyanate (FITC). Briefly, a 24-h culture (10 mL) was harvested by centrifugation (9,000 × g for 5 min), washed once in 10 mM phosphate-buffered saline (PBS; pH 7.2), and suspended in 0.5 M sodium bicarbonate buffer (pH 8) containing 0.03 mg/mL of FITC. The bacterial suspension was incubated in the dark at 37°C for 30 min with constant shaking. The bacteria were then washed three times by centrifugation (9,000 × g for 5 min) and were suspended in 10 mL of K-SFM. Gingival keratinocytes cultured as described above were seeded at 1 × 106 cells/mL in sterile black wall, clear flat bottom microplates (Greiner Bio-One North America Inc.) and were incubated overnight at 37°C in a 5% CO2 atmosphere. The medium was then removed by aspiration, and the confluent cell monolayers were pre-incubated with eriodictyol (40, 80, or 160 μM; in K-SFM) for 30 min at 37°C in a 5% CO2 atmosphere. FITC-labeled P. gingivalis was added at an MOI of 1,000, and the monolayers were incubated for a further 4 h at 37°C in a 5% CO2 atmosphere. Un-adhered bacteria were then removed, and the wells were washed twice with PBS. Relative fluorescence units (RFU) corresponding to the level of adhered bacteria were determined using a Synergy 2 microplate reader (BioTek Instruments) at an excitation wavelength of 495 nm and an emission wavelength of 525 nm. Wells with no P. gingivalis were used as controls to measure basal auto-fluorescence. Control wells without eriodictyol were used to determine 100% adherence values. Assays were performed in triplicate in three independent experiments, and the means ± standard deviations were calculated.
U937 human monocytes (CRL-1593.2; American Type Culture Collection, Manassas, VA, USA) were cultivated in Roswell Park Memorial Institute 1640 medium (RPMI; Life Technologies Inc.) supplemented with 10% heat-inactivated fetal bovine serum (FBS) and 100 μg/mL of penicillin G/streptomycin at 37°C in a 5% CO2 atmosphere. Prior to stimulation with P. gingivalis, the monocytes (1 × 106 cells/mL) were differentiated into adherent macrophage-like cells by incubating them in RPMI-10% FBS containing 100 ng/mL of phorbol myristic acid (PMA; Sigma-Aldrich Canada Co.) for 48 h in 12-well microplates. After PMA treatment, the adherent macrophage-like cells were washed to remove remaining PMA and non-adherent cells. The differentiated cells were further incubated in RPMI-10% FBS without PMA for 24 h. Then, the adherent macrophage-like cells were maintained in RPMI-1% FBS and incubated overnight at 37°C in a 5% CO2 atmosphere. The macrophage-like cells were pre-treated for 2 h with eriodictyol (80, 160, or 320 μM) in RPMI-1% FBS prior to stimulating them with P. gingivalis cells at an MOI of 100. After a 24-h incubation at 37°C in a 5% CO2 atmosphere, the culture medium supernatants were collected and were kept at −20 °C until used. To exclude any effects resulting from a loss of cell viability, the cytotoxicity of eryodictyol at the concentrations used was assessed with an MTT (3-[4,5-diethylthiazol-2-yl]-2,5diphenyltetrazolium bromide) colorimetric assay (Roche Diagnostics, Laval, QC, Canada) performed according to the manufacturer's protocol. Cells incubated in culture medium in the absence of eriodictyol and bacteria were used as controls. Enzyme-linked immunosorbent assay (ELISA) kits (R&D Systems, Minneapolis, MN, USA; Invitrogen, Thermo Fisher Scientific Inc., Waltham, MA, USA) were used to determine IL-1β, IL-6, IL-8, TNF-α, MMP-2, MMP-8, and MMP-9 concentrations according to the manufacturers' protocols. Assays were performed in triplicate in three independent experiments, and the means ± standard deviations were calculated.
The human monoblastic leukemia cell line U937 3xκ B-LUC, a subclone of the U937 cell line stably transfected with a luciferase gene linked to a promoter of three NF-κB-binding sites [28], was used to investigate the effect of eriodictyol on P. gingivalis-induced activation of the NF-κB signaling pathway. These cells were cultivated in RPMI-10% FBS, 100 μg/mL of penicillin G/streptomycin, and 75 μg/mL of hygromycin B at 37°C in a humidified incubator with a 5% CO2 atmosphere. The U937 3xκB-LUC monocytes were seeded (105 cells/well) in the wells of black wall, black bottom, 96-well microplates (Greiner Bio-One North America) and were pre-incubated with eriodictyol (80, 160, or 320 μM) for 30 min. The monocytes were then stimulated with P. gingivalis at an MOI of 100 for 6 h. Wells containing monocytes without bacteria or eriodictyol were used as controls. Bright-Glo reagent (Promega Corporation, Durham, NC, USA) was used according to the manufacturer's protocol to measure luciferase activity and determine NF-κB activation. Luminescence was monitored using a Synergy 2 microplate reader. Assays were carried out in triplicate in three independent experiments, and the means ± standard deviations were calculated.
The effect of eriodictyol (80, 160, and 320 μM) on MMP activity was assessed using an MMP-9 Inhibitor Screening Fluorometric Assay Kit (Abcam Inc., Toronto, ON, Canada) according to the manufacturer's protocol. MMP-9 activity was monitored by recording fluorescence with a 328 nm excitation filter and a 420 nm emission filter for 20 min. N-isobutyl-N-(4-methoxyphenylsulfonyl)-glycylhydroxamic acid (NNGH; 1 mM) was used as a control inhibitor of MMP-9 activity. Assays were performed in triplicate in three independent experiments, and the means ± standard deviations were calculated.
To determine the effect of eriodictyol on the collagenase activity of P. gingivalis, a 48-h culture was centrifuged at 10,000 × g for 10 min. The culture supernatant was incubated at 37°C for 3 h with eriodictyol (80, 160, or 320 μM) and 100 μg/mL of fluorogenic DQ substrate collagen (Molecular Probes, Eugene, OR, USA). The fluorescence corresponding to collagen degradation was measured every 30 min using a Synergy 2 microplate reader with a 495 nm excitation filter and a 525 emission filter. Leupeptin (1 μM) was used as a positive inhibitor control. Assays were performed in triplicate in three independent experiments, and the means ± standard deviations were calculated.
A one-way ANOVA with a post-hoc Bonferroni multiple comparison test was used to analyze the data. Results were considered statistically significant at p < 0.05, p < 0.01, or p < 0.001.
The production of ROS by gingival keratinocytes may cause deleterious effects on the oral epithelium. It was first shown that P. gingivalis (MOI = 1,000) time-dependently increased the production of ROS by gingival keratinocytes. Following a 3-h contact, we observed a 4.3-fold increase in ROS compared to untreated control cells (Figure 2). However, in the presence of eriodictyol, at all the concentrations tested (40, 80, and 160 μM), P. gingivalis-induced ROS production was significantly attenuated, with a 24.0–30.6% reduction after the 3-h contact (Figure 2). The ability of eriodictyol to impair ROS production by gingival keratinocytes treated with P. gingivalis combined with H2O2, a well-known inducer of oxidative stress [26, 27], was also investigated. As reported in Figure 3, P. gingivalis and H2O2 acted synergistically to induce ROS production by gingival keratinocytes. More specifically, following a 3-h contact, the P. gingivalis + H2O2 combination induced a 19.5-fold higher production of ROS compared to the amount of ROS produced by cells treated with P. gingivalis or H2O2 alone. Eriodictyol attenuated ROS production, with a 160 μM concentration causing a 52.9% inhibition after a 3-h contact.
Figure 2. Time- and dose-dependent effects of eriodyctiol on ROS production by gingival keratinocytes treated with P. gingivalis (MOI = 100). Results are expressed as the means ± SD of triplicate assays from three independent experiments. All values obtained in the presence of eriodictyol are significantly different from P. gingivalis-infected cells not treated with eriodictyol (p < 0.05).
Figure 3. Time- and dose-dependent effects of eriodictyol on ROS production by gingival keratinocytes stimulated with P. gingivalis + H2O2. Results are expressed as the means ± SD of triplicate assays from three independent experiments. All values obtained in the presence of eriodictyol are significantly different from keratinocytes stimulated with P. gingivalis + H2O2 in the absence of eriodictyol (p < 0.05).
Analyses were then conducted to determine the ability of eriodictyol to inhibit the adhesion of P. gingivalis to gingival keratinocytes (B11 cell line) as a potential mechanism involved in the inhibition of P. gingivalis-induced ROS production. Eriodictyol dose-dependently reduced the adherence of FITC-labeled P. gingivalis to gingival keratinocytes (Figure 4). More specifically, in the presence of 160 μM eriodictyol, adherence was inhibited by 30.8%.
Figure 4. Dose-dependent effect of eriodictyol on the adherence of P. gingivalis to gingival keratinocytes. Results are expressed as the means ± SD of triplicate assays from three independent experiments. *Values are significantly different from those of the adherence assay performed in the absence of eriodictyol (p < 0.05).
To investigate the anti-inflammatory effect of eriodictyol, macrophages were pretreated for 2 h with various concentrations (80, 160, or 320 μM) of this flavanone before being challenged with P. gingivalis cells at an MOI of 100. To exclude the possibility that the reduction in levels may have resulted from cell toxicity, we evaluated the viability of macrophages that had been treated for 24 h with eriodictyol. No obvious cytotoxic effects were detected with eriodictyol at a final concentration of 320 μM. Cell viability was ≥92% compared with the untreated control (data not shown). The secretion of IL-6, IL-8, TNF-α, and IL-1β increased significantly when the macrophages were stimulated with P. gingivalis compared to untreated cells (Figure 5). More specifically, the secretion of IL-6, IL-8, TNF-α, and IL-1β increased 17.4-, 8.5-, 138.5-, and 4.2-fold, respectively. Eriodictyol, at all the concentrations tested, significantly decreased the P. gingivalis-induced secretion of the four cytokines. In the presence of 160 μM eriodictyol, the secretion of IL-6, IL-8, TNF-α, and IL-1β decreased by 99.9, 70.8, 95.2, and 78.5%, respectively.
Figure 5. Dose-dependent effect of eriodictyol on the secretion of IL-1β (A), IL-6 (B), IL-8 (C), and TNF-α (D) by macrophages stimulated with P. gingivalis (MOI = 100) for 24 h. Results are expressed as the means ± SD of triplicate assays for three independent experiments. #Significant increase (p < 0.001) compared to cells not stimulated with P. gingivalis. *Significant decrease (p < 0.001) compared to P. gingivalis-stimulated cells.
The same protocol was used to assess the effect of eriodictyol on MMP production by P. gingivalis-treated macrophages. The secretion of MMP-2, MMP-8, and MMP-9 increased significantly when the macrophages were challenged with P. gingivalis compared with untreated cells (Figure 6). Eriodictyol dose-dependently reduced the production of all three MMPs. At a concentration of 160 μM, eriodictyol reduced the P. gingivalis-induced production of MMP-2, MMP-8, and MMP-9 by 36.9, 99.9, and 82.5%, respectively.
Figure 6. Dose-dependent effect of eriodictyol on the secretion of MMP-2 (A), MMP-8 (B), and MMP-9 (C) by macrophages stimulated with P. gingivalis (MOI = 100) for 24 h. Results are expressed as the means ± SD of triplicate assays for three independent experiments. #Significant increase (p < 0.001) compared to cells not stimulated with P. gingivalis. *Significant decrease (p < 0.001) compared to P. gingivalis-stimulated cells.
An alternative model was used to assess the anti-inflammatory effect of eriodictyol. Since NF-κB is a transcription factor implicated in inflammatory processes leading to the production of cytokines and MMPs, we evaluated the effect of eriodictyol on NF-κB activation using the U937 3xκ B-LUC monocytic cell line. As shown in Figure 7, eriodictyol dose-dependently reduced P. gingivalis-modulated NF-κB activation. At a concentration of 160 μM, eriodictyol reduced NF-κB activation by 80.7%.
Figure 7. Dose-dependent effect of eriodictyol on P. gingivalis (MOI = 100)-induced NF-κB activation. A value of 100% was assigned to the activation obtained with P. gingivalis in the absence of test compounds. Results are expressed as the means ± SD of triplicate assays for three independent experiments. #Significant increase (p < 0.01) compared to cells not stimulated with P. gingivalis. *Significant decrease (p < 0.01) compared to P. gingivalis-stimulated cells.
Eriodictyol was further studied for its ability to inhibit MMP activity. We used MMP-9 as a model and showed that eriodictyol inhibits the catalytic activity of MMP-9 in a dose- and time-dependent manner (Figure 8). After the 20-min incubation time, eriodictyol at 80, 160, and 320 μM inhibited MMP-9 activity by 19.9, 27.4, and 58.0%, respectively.
Figure 8. Dose- and time-dependent effects of eriodictyol on the activity of MMP-9. Results are expressed as the means ± SD of triplicate assays from three independent experiments. All values obtained in the presence of eriodictyol are significantly different from the assay performed in the absence of eriodictyol (p < 0.05).
Given that P. gingivalis may act in concert with MMPs to cause the destruction of periodontal connective tissue, we assessed the effect of eriodictyol on the collagenase activity of this periodontal pathogen. We showed that eriodictyol inhibits the degradation of type I collagen by P. gingivalis in a dose- and time-dependent manner. After a 4-h incubation, the lowest concentration (80 μM) of eriodictyol inhibited collagen degradation by 9.6% while the highest concentration (320 μM) inhibited collagen degradation by 30.1% (Figure 9).
Figure 9. Dose- and time-dependent effects of eriodictyol on the degradation of collagen by P. gingivalis. Results are expressed as the means ± SD of triplicate assays from three independent experiments. *Values are significantly different from the assay performed in the absence of eriodictyol (p < 0.05).
Periodontitis is a chronic inflammatory disease associated with an overproduction of cytokines and MMPs, which mediate connective tissue destruction and bone resorption. It results from a dysbiosis of the commensal oral microbiota, resulting in the overgrowth of a specific group of Gram-negative bacteria, including P. gingivalis [11, 29]. Oxidative stress and the inflammatory response mediated by periodontal pathogens are major contributors to periodontitis [2, 7, 30, 31]. Consequently, host modulation therapies involving antioxidant and anti-inflammatory compounds are a promising avenue for the treatment of this disease [32]. The search for novel antioxidant and anti-inflammatory agents is an active area of research as traditional therapies are often associated with undesirable side effects. In this regard, plant polyphenols are interesting bioactive molecules given their ability to act on multiple inflammatory targets [20, 21]. The aim of the present study was to investigate the effects of the flavanone eriodictyol on P. gingivalis-induced ROS production by gingival keratinocytes and on the inflammatory response of macrophages.
Oxidative stress resulting from an imbalance between oxidative and anti-oxidative episodes has been associated with various inflammatory diseases, including periodontitis [30, 31, 33]. More specifically, elevated levels of ROS originating from oxidative stress have been detected in the saliva and gingival crevicular fluid of periodontitis subjects [34–36]. These excessive amounts of ROS may cause damage to DNA, proteins, and lipids, and may also induce an inflammatory response in resident and immune cells in diseased periodontal sites [37, 38]. Given that the oral mucosa is in close contact with periodontal pathogens, we used a gingival keratinocyte model to show that P. gingivalis cells induce the production of intracellular ROS. This agrees with the results of Wang et al. [39], who reported that stimulating gingival epithelial cells with P. gingivalis induced the production of ROS, which caused the phosphoactivation of JAK2 and led to the secretion of the pro-inflammatory cytokines IL-6 and IL-8. Interestingly, we showed that P. gingivalis and H2O2, when used together, act in synergy to produce significantly higher amounts of ROS. To the best of our knowledge, this is the first time that such a synergistic effect has been reported. The presence of eriodictyol during keratinocyte stimulation with P. gingivalis ± H2O2 significantly inhibited ROS production in a dose-dependent manner. The fact that eriodictyol was also shown to inhibit the adhesion of P. gingivalis to gingival keratinocytes may explain, at least in part, its ability to attenuate ROS production. The antioxidant activity of eriodictyol has been previously reported in studies using various cell models, including mesangial cells [40], macrophages [41], and microglial cells [42]. Given that a periodontal therapy that improves the status of oxidative stress has been shown to be beneficial [43], eriodictyol may be of interest for such a therapeutic approach.
Macrophages, which are key cells of the innate immune system, play an essential role in the overall inflammatory response. During the onset and progression of periodontitis, macrophages are found in high numbers in diseased periodontal sites [9, 10]. These leukocytes modulate the inflammation process by secreting a variety of chemical agents, including cytokines, that can amplify the inflammatory response [9, 10]. We showed that eriodictyol attenuates the production of IL-1β, IL-6, IL-8, and TNF-α by P. gingivalis-stimulated macrophages. Eriodictyol may thus be a promising molecule for modulating the inflammatory process that occurs during periodontitis. Studies aimed to investigate the ability of eriodictyol to up-regulate the secretion of anti-inflammatory cytokines such as IL-4 and IL-10 may further support the anti-inflammatory potential of eriodictyol.
NF-κB is considered the main mediator of the immune response and is a central pathway by which host cells respond to stress and bacterial challenges [44]. Consequently, NF-κB is a key target for anti-inflammatory compounds as inhibiting it may impair pro-inflammatory cytokines secretion [45]. We used the U937 3xκ BLUC cell line to show that P. gingivalis cells activate the NF-κB signaling pathway and that eriodictyol almost completely prevents this activation. It can be hypothesized that inhibition of NF-κB activation may be related to the ability of eriodictyol to prevent phosphorylation and subsequent degradation of IκB. Our results agree with the study of Lee [46], who reported that eriodictyol reduces mRNA expression and secretion of pro-inflammatory cytokines in a murine macrophage model. This anti-inflammatory property involves the blockage of NF-κB activation.
Macrophages, which make up an important part of the inflammatory infiltrate in active periodontal lesions, are major producers of MMPs when they are triggered by periodontal pathogens [10]. Given their action on a large array of extracellular matrix proteins such as laminin, fibronectin, and collagen, MMPs contribute to periodontal tissue destruction [47, 48]. In the present study, we showed that P. gingivalis cells act as a potent inducer of MMP production, including MMP-2,−8, and−9, by macrophages. Eriodictyol inhibited the production of all three MMPs, suggesting that the use of this flavanone may be a potentially effective strategy for the prevention and control of periodontitis.
Periodontal tissue destruction has been associated with the presence of high levels of active MMP-9 in gingival tissue, saliva, and gingival crevicular fluid [47–49]. Moreover, this MMP may be a practical marker for assessing the clinical severity of periodontitis [50]. We thus used MMP-9 as an MMP model to investigate the ability of eriodictyol to inhibit the catalytic activity of MMPs. We showed that eriodictyol markedly inhibits MMP-9 activity.
Eriodictyol, by inhibiting both the production and the activity of MMPs, offers a promising perspective for developing novel and innovative therapies for treating pathologic conditions characterized by excessive MMP activity such as in periodontitis. In addition to MMPs, the collagenase activity of P. gingivalis may also participate in periodontal tissue breakdown. Type I collagen makes up ~60% of the periodontal tissue volume. In the present study, we showed that eriodictyol reduces collagen degradation by P. gingivalis, suggesting that it may contribute to attenuating the tissue destruction process.
Given that periodontitis is a complex disease initiated by a polymicrobial biofilm colonizing the subgingival sites, a limitation of this study is the fact that a single periodontal pathogen grown in planktonic culture was used to stimulate gingival keratinocytes and macrophages. Nevertheless, eriodictyol, incorporated into mouthrinses, toothpastes, gels, or local drug delivery systems, may represent a promising therapeutic agent for the prevention and/or treatment of periodontal disease due to its antioxidant, anti-inflammatory, and anti-MMP properties.
The original contributions presented in the study are included in the article/supplementary material, further inquiries can be directed to the corresponding author.
DG, PMMH, and DPS conceived and designed the experiments. PMMH performed the experimental assays and the statistical analysis. JM analyzed and provided the test compound for the study. DG drafted the manuscript. DG, PMMH, JM, and DPS reviewed and edited the manuscript. All authors contributed to the article and approved the submitted version.
PMMH was supported by a scholarship provided by São Paulo Research Foundation (FAPESP; Grant #2018/16540-8 and #2019/15343-7). This study was funded by the Laboratoire de Contrôle microbiologique of Université Laval (2021-12).
The authors declare that the research was conducted in the absence of any commercial or financial relationships that could be construed as a potential conflict of interest.
All claims expressed in this article are solely those of the authors and do not necessarily represent those of their affiliated organizations, or those of the publisher, the editors and the reviewers. Any product that may be evaluated in this article, or claim that may be made by its manufacturer, is not guaranteed or endorsed by the publisher.
We wish to thank S. Groeger and J. Meyle (Justus-Liebig-University Giessen, Germany), for providing the B11 keratinocyte cell line. We also thank R. Blomhoff and H. Carlsen (University of Oslo, Norway) for their generous gift of the U937 3xκB-LUC cell line. The technical assistance of K. Vaillancourt is acknowledged.
1. Eke PI, Borgnakke WS, Genco RJ. Recent epidemiologic trends in periodontitis in the USA. Periodontol 2000. (2020) 82:257–67. doi: 10.1111/prd.12323
2. Hajishengallis G. Immunomicrobial pathogenesis of periodontitis: keystones, pathobionts, and host response. Trends Immunol. (2014) 35:3–11. doi: 10.1016/j.it.2013.09.001
3. Kononen E, Gursoy M, Gursoy UK. Periodontitis: a multifaceted disease of tooth-supporting tissues. J Clin Med. (2019) 8:1135. doi: 10.3390/jcm8081135
4. Bui FQ, Almeida-da-Silva CLC, Huynh B, Trinh A, Liu J, Woodward J, et al. Association between periodontal pathogens and systemic disease. Biomed J. (2019) 42:27–35. doi: 10.1016/j.bj.2018.12.001
5. Kumar PS. From focal sepsis to periodontal medicine: a century of exploring the role of the microbiome in systemic disease. J Physiol. (2017) 595:465–76. doi: 10.1113/JP272427
6. Meurman JH, Bascones-Martinez A. Oral infections and systemic health – more than just links to cardiovascular diseases. Oral Health Prev Dent. (2021) 19:441–8. doi: 10.3290/j.ohpd.b1993965
7. Liu YCG, Lerner UH, Teng YTA. Cytokine responses against periodontal infection: protective and destructive roles. Periodontol 2000. (2010) 52:163–206. doi: 10.1111/j.1600-0757.2009.00321.x
8. Yamamoto M, Aizawa R. Maintaining a protective state for human periodontal tissue. Periodontol 2000. (2021) 86:142–56. doi: 10.1111/prd.12367
9. Zappa U, Reinking-Zappa M, Graf H, Espeland M. Cell populations and episodic periodontal attachment loss in humans. J Clin Periodontol. (1991) 18:508–15. doi: 10.1111/j.1600-051X.1991.tb00082.x
10. Wang W, Zheng C, Yang J, Li B. Intersection between macrophages and periodontal pathogens in periodontitis. J Leukoc Biol. (2021) 110:577–83. doi: 10.1002/JLB.4MR0421-756R
11. Lamont RJ, Koo H, Hajishengallis G. The oral microbiota: dynamic communities and host interactions. Nature Rev Microbiol. (2018) 16:745–59. doi: 10.1038/s41579-018-0089-x
12. Wang Y, Andrukhov O, Rausch-Fan X. Oxidative stress and antioxidant system in periodontitis. Front Physiol. (2017) 8:910. doi: 10.3389/fphys.2017.00910
13. Curtis MA, Diaz PI, Van Dyke TE. The role of the microbiota in periodontal disease. Periodontol 2000. (2020) 83:14–25. doi: 10.1111/prd.12296
14. How KY, Song KP, Chan KG. Porphyromonas gingivalis: an overview of periodontopathogenic pathogen below the gum line. Front Microbiol. (2016) 7:53. doi: 10.3389/fmicb.2016.00053
15. Dahlen G, Basic A, Bylund J. Importance of virulence factors for the persistence of oral bacteria in the inflamed gingival crevice and in the pathogenesis of periodontal disease. J Clin Med. (2019) 8:1339. doi: 10.3390/jcm8091339
16. Mysak J, Podzimek S, Sommerova P, Lyuya-Mi Y, Bartova J, Janatova T, et al. Porphyromonas gingivalis: major periodontopathic pathogen overview. J Immunol Res. (2014) 2014:476068. doi: 10.1155/2014/476068
17. Hu W, Zhou W, Wang H, Liang S. Roles of Porphyromonas gingivalis and its virulence factors in periodontitis. Adv Protein Chem Struct Biol. (2020) 120:45–84. doi: 10.1016/bs.apcsb.2019.12.001
18. Ji S, Choi YS, Choi Y. Bacterial invasion and persistence: critical events in the pathogenesis of periodontitis? J Periodontal Res. (2015) 50:570–85. doi: 10.1111/jre.12248
19. Groeger SE, Meyle J. Epithelial barrier and oral bacterial infection. Periodontol 2000. (2015) 69:46–67. doi: 10.1111/prd.12094
20. Bunte K, Hensel A, Beikler T. Polyphenols in the prevention and treatment of periodontal disease: a systematic review of in vivo, ex vivo and in vitro studies. Fitoterapia. (2019) 132:30–9. doi: 10.1016/j.fitote.2018.11.012
21. Fernandez-Rojas B, Gutierrez-Venegas G. Flavonoids exert multiple periodontic benefits including anti-inflammatory, periodontal ligament-supporting, and bone-preserving effects. Life Sci. (2018) 209:435–54. doi: 10.1016/j.lfs.2018.08.029
22. Deng Z, Hassan S, Rafiq M, Li H, He Y, Cai Y, et al. Pharmacological activity of eriodictyol: the major natural polyphenolic flavanone. Evid-Based Complementary. Altern Med. (2020) 2020:6681352. doi: 10.1155/2020/6681352
23. Islam A, Islam MS, Rahman MK, Uddin MN, Akanda MR. The phamacological and biological roles of eriodictyol. Arch Pharm Res. (2020) 43:582–92. doi: 10.1007/s12272-020-01243-0
24. Carvalho JS, Ramadan D, de Paiva Gonçalves V, Maquera-Huacho PM, Assis RP, Lima TFO, et al. Impact of citrus flavonoid supplementation on inflammation in lipopolysaccharide-induced periodontal disease in mice. Food Funct. (2021) 12:5007–17. doi: 10.1039/D0FO03338C
25. Gröeger S, Michel J, Meyle J. Establishment and characterization of immortalized human gingival keratinocyte cell lines. J Periodontal Res. (2008) 43:604–14. doi: 10.1111/j.1600-0765.2007.01019.x
26. Lee JH, Yoo JY, Kim HB, Yoo HI, Song DY, Min SS, et al. Neuregulin1 attenuates H2O2-induced reductions in EAAC1 protein levels and reduces H2O2-induced oxidative stress. Neurotox Res. (2019) 35:401–9. doi: 10.1007/s12640-018-9965-4
27. Sun X, Jia H, Xu Q, Zhao C, Xu C. Lycopene alleviates H2O2-induced oxidative stress, inflammation and apoptosis in bovine mammary epithelial cells via the NFE2L2 signaling pathway. Food Funct. (2019) 10:6276. doi: 10.1039/C9FO01922G
28. Carlsen H, Moskaug JØ, Fromm SH, Blomhoff R. In vivo imaging of NF-kappa B activity. J Immunol. (2002) 168:1441–6. doi: 10.4049/jimmunol.168.3.1441
29. Hajishengallis G, Lamont RJ. Beyond the red complex and into more complexity: the polymicrobial synergy and dysbiosis (PSD) model of periodontal disease etiology. Mol Oral Microbiol. (2012) 27:409–19. doi: 10.1111/j.2041-1014.2012.00663.x
30. Chapple ILC. Reactive oxygen species and antioxidants in inflammatory diseases. J Clin Periodontol. (1997) 24:287–96. doi: 10.1111/j.1600-051X.1997.tb00760.x
31. Chapple IL, Matthews JB. The role of reactive oxygen and antioxidant species in periodontal tissue destruction. Periodontol 2000. (2007) 43:160–232. doi: 10.1111/j.1600-0757.2006.00178.x
32. Sulijaya B, Takahashi N, Yamazaki K. Host modulation therapy using anti-inflammatory and antioxidant agents in periodontitis: a review of clinical translation. Arch Oral Biol. (2019) 105:72–80. doi: 10.1016/j.archoralbio.2019.07.002
33. Pizzino G, Irrera N, Cucinotta M, Pallio G, Mannino F, Arcoraci V. Oxidative stress: harms and benefits for human health. Oxid Med Cell Longev. (2017) 2017:8416763. doi: 10.1155/2017/8416763
34. Tothova L, Celec P. Oxidative stress and antioxidants in the diagnosis and therapy of periodontitis. Front Physiol. (2017) 8:1055. doi: 10.3389/fphys.2017.01055
35. Georgiou AC, Cornejo Ulloa P, van Kessel GMH, Crielaard W, van der Wall SV. Reactive oxygen species can be traced locally and systemically in apical periodontitis: A systematic review. Arch Oral Biol. (2021) 129:105167. doi: 10.1016/j.archoralbio.2021.105167
36. Acquier AB, De Couto Pita AK, Busch L, Sanchez GA. Parameters of oxidative stress in saliva from patients with aggressive and chronic periodontitis. Redox Rep. (2017) 22:119–26. doi: 10.1080/13510002.2016.1198104
37. Pisoschi AM, Pop A. The role of antioxidants in the chemistry of oxidative stress: a review. Eur J Med Chem. (2015) 97:55–74. doi: 10.1016/j.ejmech.2015.04.040
38. De Oliveira-Marques V, Cyrne L, Marinho HS, Antunes F. A quantitative study of NF-kappaB activation by H2O2: relevance in inflammation and synergy with TNF-alpha. J Immunol. (2007) 178:3893–902. doi: 10.4049/jimmunol.178.6.3893
39. Wang H, Zhou H, Duan X, Jotwani R, Vuddaraju H, Liang S, et al. Porphyromonas gingivalis-induced reactive oxygen species activate JAK2 and regulate production of inflammatory cytokines through c-Jun. Infect Immun. (2014) 82:4118–26. doi: 10.1128/IAI.02000-14
40. Bai J, Wang Y, Zhu X, Shi J. Eriodictyol inhibits high glucose-induced extracellular matrix accumulation, oxidative stress, and inflammation glomerular mesangial cells. Phytother Res. (2019) 33:2775–82. doi: 10.1002/ptr.6463
41. Mokdad-Bzeouich I, Mustapha N, Sassi A, Bedoui A, Ghoul M, Ghedira K, et al. Investigation of immunomodulatory and anti-inflammatory effects of eriodictyol through its cellular and antioxidant activity. Cell Stress Chaperones. (2016) 21:773–81. doi: 10.1007/s12192-016-0702-8
42. He P, Yan S, Wen X, Zhang S, Liu Z, Liu X, et al. Eriodictyol alleviates lipopolysaccharide-triggered oxidative stress and synaptic dysfunctions in BV-2 microglial cells and mouse brain. J Cell Biochem. (2019) 120:14756–70. doi: 10.1002/jcb.28736
43. Bostanci V, Toker H, Senel S, Ozdemir H, Aydin H. Effect of chronic periodontitis on serum and gingival crevicular fluid oxidant and antioxidant status in patients with familial Mediterranean fever before and after periodontal treatment. J Periodontol. (2014) 85:706–12. doi: 10.1902/jop.2013.130230
44. Liu T, Zhang L, Joo D, Sun S. NF-κB signaling in inflammation. Signal Transduct Target Ther. (2017) 2:17023. doi: 10.1038/sigtrans.2017.23
45. Gupta SC, Sundaram C, Reuter S, Aggarwal BB. Inhibiting NF-κB activation by small molecules as a therapeutic strategy. Biochim Biophys Acta. (2010) 1799:775–87. doi: 10.1016/j.bbagrm.2010.05.004
46. Lee JK. Anti-inflammatory effects of eriodictyol in lipopolysaccharide-stimulated Raw 264.7 murine macrophages. Arch Pharm Res. (2011) 34:671–9. doi: 10.1007/s12272-011-0418-3
47. Cavalla F, Hernandez-Rios P, Sorsa T, Biguetti C, Hernandez M. Matrix metalloproteinases as regulators of periodontal inflammation. Int J Mol Sci. (2017) 18:440. doi: 10.3390/ijms18020440
48. Boelen GJ, Boute L, d'Hoop J, EzEldeen M, Lambrichts I, Opdenakker G. Matrix metalloproteinases and inhibitors in dentistry. Clin Oral Investig. (2019) 23:2823–35. doi: 10.1007/s00784-019-02915-y
49. Kim HD, Sukhbaatar M, Shin M, Ahn YB, Yoo WS. Validation of periodontitis screening model using sociodemographic, systemic, and molecular information in a Korean population. J Periodontol. (2014) 85:1676–83. doi: 10.1902/jop.2014.140061
Keywords: eriodictyol, macrophage, keratinocyte, Porphyromonas gingivalis, periodontal disease
Citation: Maquera-Huacho PM, Spolidorio DP, Manthey JA and Grenier D (2022) Eriodictyol Suppresses Porphyromonas gingivalis-Induced Reactive Oxygen Species Production by Gingival Keratinocytes and the Inflammatory Response of Macrophages. Front. Oral. Health 3:847914. doi: 10.3389/froh.2022.847914
Received: 03 January 2022; Accepted: 31 January 2022;
Published: 28 February 2022.
Edited by:
Fabian Cieplik, University Medical Center Regensburg, GermanyReviewed by:
Yüksel Korkmaz, Johannes Gutenberg University of Mainz, GermanyCopyright © 2022 Maquera-Huacho, Spolidorio, Manthey and Grenier. This is an open-access article distributed under the terms of the Creative Commons Attribution License (CC BY). The use, distribution or reproduction in other forums is permitted, provided the original author(s) and the copyright owner(s) are credited and that the original publication in this journal is cited, in accordance with accepted academic practice. No use, distribution or reproduction is permitted which does not comply with these terms.
*Correspondence: Daniel Grenier, ZGFuaWVsLmdyZW5pZXJAZ3JlYi51bGF2YWwuY2E=
Disclaimer: All claims expressed in this article are solely those of the authors and do not necessarily represent those of their affiliated organizations, or those of the publisher, the editors and the reviewers. Any product that may be evaluated in this article or claim that may be made by its manufacturer is not guaranteed or endorsed by the publisher.
Research integrity at Frontiers
Learn more about the work of our research integrity team to safeguard the quality of each article we publish.