- 1Institute of Food Science and Nutrition, University of Sargodha, Sargodha, Pakistan
- 2Department of Zoology, University of Sargodha, Sargodha, Pakistan
The issue of household waste management is a pervasive global concern. Household waste, especially food waste, comprises a reservoir of compounds with potential economic value, among which bioactive compounds prominently feature. These bioactive compounds, derived from natural chemical substances inherent in waste from animal and protein sources, offer nutritional benefits. Extracted bioactive compounds find versatile applications in various domains including textiles, cosmetics, nutraceuticals, food additives, and the agriculture sector. The extraction of such compounds is achieved through a diversity of techniques, including conventional methods alongside non-conventional green approaches such as extraction methods using enzymes, microwaves, or supercritical fluids. However, there is an increasing need for green and sustainable approaches, leading to bioactive-rich extracts with low environmental impact. This review thoroughly summarizes the origins, kinds, and characteristics of bioactive chemicals found in food waste. It also addresses the most promising and innovative methodologies for the recovery of functional bioactive compounds from waste materials that have been published recently. New technologies can meaningfully manage materials from household food waste. These bioactive compounds are valuable for food industrial applications.
1 Introduction
Any item that is discarded from the home environment as part of residents’ regular activities is referred to as “household waste” or “domestic garbage”. It comprises significant portion of municipal solid waste (MSW) and is made up of a variety of materials including glass, metal, paper, plastic, food scraps, and textile fragments. Over 60 tons of rubbish are produced every second in the world’s households, amounting to two billion tons of waste annually (Maalouf and Mavropoulos, 2023). The collection, transportation, and treatment of household garbage are a global issue (Mostaghimi and Behnamian, 2023a). Earlier studies have established the correlation between trash generation and population size as well as average income or level of living. The quantity and composition of trash can also be impacted by variables that include the environment, ways of life, levels of education, cultural and religious views, and public and social attitudes (Yu et al., 2023).
Food waste (FW) comprises a significant portion of household waste and is defined by the Food and Agriculture Organization of the United Nations (FAO) as food that is produced in smaller quantities or of greater superiority as a result of choices and actions made by food vendors, convenience stores, and consumers (Poonam Sharma et al., 2021a; Delgado, Rodriguez, and Staszewska, 2023). According to FAO data from 2019 and a UN Environment Programme Food Waste Index study, almost 14% of the world’s food, valued at $400 billion annually, is wasted post-harvest until it reaches stores, and 17% more is wasted by consumers, primarily in households and retail establishments (Tanyanyiwa and Tongowona, 2023). A third of the world’s annual food production manifests as FW, which could serve 1.26 billion malnourished people (Liu et al., 2023a). Inappropriate food waste management and disposal errors can have serious environmental consequences. An estimated 3.3 billion tons of carbon dioxide equivalent is released, due to insufficient handling of FW, into the environment annually, accounting for 8% of all human greenhouse gas emissions (Bhatia et al., 2023). Food valorization is an innovative approach to reducing waste and providing new economic opportunities by producing value-added goods based on the bioactive ingredients of foods. These beneficial substances, which also add value to the products, include polyphenols, vitamins, minerals, and prebiotics (Liu et al., 2023a). Utilizing FW’s ability to extract these molecules may open the door to the development of premium, functional food ingredients, cosmetics, and nutritional supplements. This method seeks to lessen FW and promote creative revenue streams, in addition to aiding the development of a circular economy. It is crucial to consider the accessibility of these leftovers through time, their techno-economic prospect, and the environmental evaluation of profit and costs based on their life span to assure the sustainability of the environment and economy of future FW valorization (P. Roy et al., 2023).
This paper aims to provide a comprehensive overview of the various types of household FW commonly encountered from fruit, vegetables, cereals, pulses, and dairy. Valuable bioactive compounds like polyphenols, dietary fibers, proteins, vitamins, and minerals are extracted from these FWs by different extraction techniques. The potential applications of biologically active compounds in the food sector include food additives, nutraceuticals, and single cell proteins. Feasible conventional and nonconventional methods along with using AI and robotics for transforming FW into value-added products are also explored here. Moreover, this review offers recommendations for sustainability concerns in waste valorization and directions for future research on improving the utilization of FW, as well as stimulating interest in its conversion and reuse.
2 Traditional methods to manage household waste
Due to their extreme heterogeneity, household or municipal wastes must first be properly sorted and fractionated before undergoing any significant treatment. According to a number of research findings, households account for 50%–80% of solid trash produced in developing nations, with markets and commercial zones accounting for the another 10%–15% of municipal waste (S. Khan et al., 2022). These waste materials, encompassing garden waste, kitchen waste, plastic cans and bags, timber, metallic waste, cardboard, synthetic rubbers, animal hides, power cells, inert compounds, fabric waste, paint containers, building debris, and numerous other indeterminate items, necessitate a sorting and separation process to deliver essential data on the quality of sorting for any prospective application (M. Singh, Singh, and Singh, 2024). This process is a crucial step in solid waste management. Traditional techniques of food waste (FW) management encompass animal feeding, landfilling, composting, incineration, combustion, and similar measures (Zhu et al., 2023). A hierarchy of recommended waste management techniques is provided by the EC’s waste management plan. The five steps of waste management, from most to least preferred, are avoiding waste generation, reusing materials, recycling materials, recovering energy from waste, and safely disposing of waste (Mostaghimi and Behnamian, 2023a). These methods lessen the production of waste, increase resource efficiency, preserve a non-ecofriendly energy mix, develop new markets and businesses, and lessen risks to human health and the environment. Overall, the hierarchy directs waste management procedures and promotes the use of more ecologically friendly and sustainable methods (Teng et al., 2023). Centralized waste management systems have two main weaknesses: the need to frequently transport waste over long distances, and the difficulty of sorting waste at large plants (Bernat, 2023). Home waste treatment can facilitate energy and monetary savings by circumventing the challenges of extensive waste management systems (Jayanandini, 2023). Various waste management methods may be implemented in a home, contingent on the amount of space, time, and financial resources available. Regretfully, the majority of these—open burning, anaerobic digestion, and composting—only permit the processing of organic waste (Hui et al., 2023). Composting is the most used waste management technique in homes. There are many different methods and tools available, ranging from the most affordable homemade boxes to sophisticated yet rather costly automated composters. The process of composting gives the soil the chance to absorb the nutrients included in the biomass and gives access to high-quality fertilizer. However, it requires time (Waqas et al., 2023). In addition, microbes eat the energy included in the waste, so it is lost from the viewpoint of the householders (Jouhara et al., 2017). Energy recovery from methane combustion is possible by anaerobic digestion, but this requires substantial investment and a sizable area to install the digester. It may also only be readily introduced in warm climates as microbes need a certain temperature to thrive. The digester must be heated in cold and mild temperatures, which presents some challenges. The homeowner receives useful fertilizer as a byproduct; organic waste contains nutrients that may be recycled (Glasser, 2023; Kumar et al., 2023). Another popular technique for handling domestic garbage is combustion to create heat, which happens between the fuel and the oxidant. The fuel may be solid, liquid, or gaseous. Fuel and oxidants undergo chemical reactions when ignited, and eventually the process becomes self-sustaining due to the heat produced by the reactions (Liang, 2023; Obi et al., 2023). Contemporary nations forbid the open burning of mixed garbage due to the significant harm it causes both the environment and public health (Oyebode, 2024). In certain situations, biomass burning could make sense, provided the right circumstances are met. A sizable space is required for the disposal of garbage. Finding a “free” location is challenging—not just in suburban areas but also away from populated areas (Santos and Mendes, 2024). The lengthening of trash transportation routes to disposal sites results in higher waste management expenses. A contemporary landfill is a costly, intricate technological structure that spans several dozen hectares and is just a few tens of meters deep. The landfill’s base is made up of many layers in addition to the earth, and leachate neutralization and collection are required (Un, 2023). Treatment is then required for the collected leachate. Perforated 0.7-meter-diameter ferrous-beton rings are used to attach vertical gas wells (Petruk et al., 2016). Like composting, controlled incineration facilities are used for incineration. Contemporary incinerators include large stacks and combustion chambers that are purpose built. To achieve full combustion, they must supply air, high combustion temperatures, extended residence durations, and effective waste mixing (Reich et al., 2023). To comply with pollution regulations, they also feature effective flue gas cleaning systems. The Waste Incineration Directive of the EU has specifications for them (Dal Pozzo, Capecci, and Cozzani, 2023). The waste categories that are burned include municipal solid waste (MSW), industrial waste, toxic waste, clinical waste, and sludge from sewage treatment plants (Ogunkunle et al., 2024).
Although these conventional techniques may result in the loss of important biomaterials recovered from food waste streams, they may tangentially boost economic gains. It was projected that traditional and unplanned FW management would result in the depletion of critical resources, such as nutrients and bioactive substances, as well as negative environmental effects. To comply with international regulatory frameworks for food security, integrated and developing home and FW management has been supported (Sarker et al., 2024a).
3 Recent trends of household waste management
The integration of artificial intelligence (AI), robotics, the internet of things (IoT), and big data and analytics into waste management has revolutionized the sector (Rane, 2023). AI enhances operational efficiency by automating waste management processes, such as scheduling pickups, trucking, and sorting. Robotics, powered by detectors, machine vision, and AI, automate trash segregation operations and ensure material-specific disposal (Sivashanmugam et al., 2023). Timely waste collection and disposal is made possible by IoT-based waste management with real-time waste bin tracking. Big data insights into location and bin emptying frequency enable waste collection businesses to distinguish between different forms of waste and guarantee timely collection (Melakessou et al., 2020). Cutting-edge recycling technologies improve waste stream quality and operating efficiency. Examples include material scanners and autonomous waste-picking devices. Developments in decomposition technologies make use of organic materials’ natural breakdown processes to nourish the soil with healthy nutrients and stop soil erosion (Rajput et al., 2024). Furthermore, the process of waste management has been greatly enhanced by the incorporation of blockchain technology, bringing transparency and traceability to the waste management value chain to enable responsible waste management. These advances, coupled with the principles of the circular economy which ensure minimum waste by reusing and repurposing existing products, are paving the way for a more sustainable future (Gupta and Shankar, 2024; Kandpal et al., 2024).
The management of household waste by its valorization in the production of biofuels, biomethane production, bio-alcohol production, biohydrogen production, production of bio-materials, animal feed, and the recovery of value-added components and bioactive compounds is a promising strategy for achieving sustainable and zero-waste development of the agri-food sector. Food and agri-food utilize waste byproducts for biofuel production, a significant strategy due to rising energy costs and environmental concerns (Venugopal, 2022). Biofuels are produced using bio-based technologies such as anaerobic digestion, aerobic digestion, and microbial fermentation (Anoopkumar et al., 2023). Adsorption, which is an economical and environmentally beneficial process, is utilized for wastewater treatment, with lignocellulosic waste products acting as substitutes for activated carbon synthesis (H. H. Shah et al., 2023). Extracted components from food waste (FW) containing sugars, proteins, fibers, pectin, lipids, polysaccharides, taste compounds, and phytochemicals are employed as food additives, nutraceuticals, pharmaceuticals, and cosmetics. This increases food availability, extends shelf life, and minimizes landfill waste (Aqilah et al., 2023). Polymers made from renewable organic sources, known as “bio-based polymers”, diminish reliance on fossil fuels and are produced from agro-industrial waste through combined fermentation and enzymatic processes. Enzymes, produced from these wastes, have significant industrial applications due to their high specificity (J. Yadav et al., 2023). The dried biomass of diverse microbial species—single-cell protein (SCP)—can serve as a protein supplement. SCP is produced by microorganisms that convert low-protein organic substrates into high-protein products (U. Shah and Hajoori, 2022). The aerobic degradation of FW produces pathogen-free bio-fertilizers and soil conditioners, reducing solid waste handling and disposal burdens and providing social and environmental benefits (Lirikum et al., 2022). The success of this valorization procedure is affected by variables such as temperature, ventilation, moisture levels, pH, carbon-to-nitrogen ratio, particle dimensions, and level of compaction. However, the viability of composting relies on the greenhouse-gas emissions produced during the decomposition of organic matter (Azis et al., 2022).
4 Sources of household food waste generation
4.1 Cereals and pulses
Cereals, which include rice and grains such as wheat, rye, millet, corn, and buckwheat are an important part of the food pyramid and serve as the primary source of energy (M. A. Shah, Sunooj, and Mir, 2023). Throughout the processing phase, cereals and legumes produce by-products such as germ and bran. The residual cereal matter in malt processing is generally considered a byproduct and is commonly disposed of as refuse. Rice husks can be used for a variety of purposes, including building materials, nutrients, substrates for decomposition techniques, and producing fiber and feedstock (R. Yadav et al., 2020). The byproduct of barley processing contains a significantly higher quantity of vitamin E (2.7 times more) than the entire barley grain. In addition, it provides an abundance of different bioactive substances, including phenolics, phytates, and insoluble food fiber (Raj et al., 2023). Phenolic chemicals are also produced because of cellulase’s enzymatic degradation of rice bran and husk. Value-added products can be produced by growing flat mushrooms, extracting pectin, and making livestock feed from the leftover shells of cocoa processing. Furthermore, it is possible to obtain a second-generation bioethanol from coconut husk (Freitas et al., 2021; Liu et al., 2023a).
4.2 Fruits and vegetables
Nutrient-rich fruit and vegetable wastes are often handled via landfill and composting, which harms the environment (Singha and Singha, 2024). Another strategy is hydrolyzing the cellulose and starch in these wastes to create sweet fermentables that can be used to yield alcohol and hydrogen (Aruwajoye et al., 2020). Innovations such as single-cell proteins, oils, pigments, polyphenols, and biofuels can be produced by microbial processing. Fermentation can also produce lactic and succinic acids (Chavan et al., 2022). Such strategies can maximize the resource’s potential for sustainable use while addressing environmental issues (Almalki et al., 2023). Figure 1 illustrates different sources of household waste and bioactive compounds present in those waste materials.
4.3 Dairy
Worldwide, approximately 128 million tons of milk production is lost annually, attributed to factors like processing, microbial spoilage, and inadequate handling. Dairy goods, characterized by their intricate organic makeup of proteins, fats, sugars, and food supplements, are especially susceptible to spoilage and fungal contamination (Pantoja et al., 2022). In the manufacturing of cheese, for each kilogram of the product, 9 kg of whey is discarded (Liu et al., 2023a). The wastewater from milk processing, containing significant amounts of carbohydrates, lipids, proteins, and nitrogen concentrations of 14–830 mg/L, can produce substances such as nucleic acids, urea, proteins, and NO−2, NO−3, and NH+4 when milk proteins are dissociated. This dairy refuse, abundant in organic content, has the potential to be transformed into value-added items through microbial-facilitated methods (Kusmayadi et al., 2022). Filamentous fungi can also decompose the intricate carbohydrates present in dairy waste into monosaccharides, contributing to the creation of superior biomass for animal feed and single-cell proteins, deemed safe for human consumption (T. A. Singh et al., 2022).
4.4 Edible oil and fats
Oxidative or hydrolytic spoilage is a primary factor leading to the degradation of edible oils and their subsequent disposal (Fazli et al., 2022). The emergence of polar molecules in used cooking oil, such as free short-chain fatty acids, mono- and diglycerides, aldehydes, ketones, polymers, and cyclic and aromatic compounds, renders it unfit for human consumption after undergoing multiple deep-frying cycles (Cárdenas et al., 2021; Liu et al., 2023a). Historically, the oil processing industry has released its effluents into the soil and groundwater, resulting in the creation of oily films on aquatic surfaces. This has presented a significant hazard to the survival of marine organisms and caused blockages in sewage and drains due to the emulsification of organic matter, as well as oil methanization, contributing to the greenhouse effect. Nevertheless, innovative methods now exist that employ microbial cells to degrade organic substances in effluents, leading to the production of high-quality products such as bio-based zwitterionic biosurfactant (Khorram et al., 2023). For example, Pseudomonas aeruginosa has demonstrated the ability to generate biosurfactants, including rhamnolipid and sophorolipid, along with biodiesel production through lipase and liquid hydrocarbon biofuels (Gaur et al., 2022). Residues from edible oil, such as tocopherols, sterols, and squalene, are now extracted and utilized as raw materials in diverse industries, including the creation of single-cell oil/protein for food as well as in medicinal formulations and cosmetics in the form of soap stalk (Liu et al., 2023a).
4.5 Meat, poultry, and eggs
A positive strategy for utilizing byproducts from slaughterhouses involves cultivating lactic acid bacteria, which can be incorporated into probiotic goods (Sar et al., 2022). Slaughterhouse remnants serve as a rich reservoir of nutrients that can be harnessed to generate diverse value-added items, including biogas, hemoglobin for both food and non-food applications, biomass, and methane (Selvamuthukumaran, 2024a). These outputs include fish feed, fertilizers, binders, blood sausages, blood cakes, blood puddings, blood clots, and a sustainable energy substitute derived from the anaerobic digestion of wastewater (Purkait et al., 2023). Biodiesel has been derived from chicken manure biochar through pseudo-catalytic transesterification reactions and eggshells using homogeneous catalysts for transesterification of triglycerides with methanol. This approach holds significant promise in the development of pharmaceutical and cosmetic products (Wang et al., 2020).
4.6 Seafoods and aquatic life
Oceanic ecosystems, contributing approximately 20% of the global food supply, produce substantial byproducts owing to the suboptimal quality of marine organisms; only 40% of protein from crustaceans and 75% of tuna fillets are deemed usable (Ahern et al., 2021). This byproduct, encompassing materials like shrimp exoskeletons, crab carapaces, prawn remnants, fish scales, and the skeletal structures of crustaceans, is abundant in valuable compounds such as amino acids, cellulose, and calcium carbonate (Machineni, 2023). Traditional disposal methods can cause environmental harm, and chitin can contribute to contamination. Therefore, a biological remedy for this waste is crucial for environmental protection and bioeconomic revenue generation. Valuable substances like astaxanthin, a pigment found in crustacean waste, and chitosan, a biopolymer with antibacterial properties, can be derived from this waste (S. Singh et al., 2022). Furthermore, marine-derived glycosaminoglycans are higher quality than those obtained from land-based sources. Enzymatic hydrolysis can transform the waste products of seafood processing into compounds with biological activity and health benefits (Ozogul et al., 2021; V. C; Roy VikashChandra et al., 2023).
5 Bioactive compounds in food waste
Food and natural substances contain potent components and bioactive compounds that can improve health outcomes (Mateus et al., 2023). For instance, edibles can provide dietary fiber, vitamins, minerals, polyphenols, and other health-promoting substances (Kusumasari et al., 2024). The identification and extraction of these bioactive molecules enables the development of novel functional ingredients and nutritional supplements, which reduce waste and enhance sustainability. The identification and extraction of these bioactive molecules enable the development of novel functional ingredients and nutritional supplements which reduce waste and enhance sustainability. (Mahmud et al., 2024).
5.1 Polyphenols
Health-beneficial polyphenols can be extracted from food waste (FW). Plants require phenolic compounds for a variety of molecular and physiological processes, including signaling, defense mechanisms, auxin transport mediation, and scavenging of free radicals. These substances have anti-inflammatory, antibacterial, antioxidant, and anticarcinogenic qualities that help human health in addition to greatly enhancing the health of plants. In plants, phenolic compounds act as UV filters, signal compounds, attractants, structural polymers, antioxidants, and defensive response chemicals (Koraqi et al., 2023). Apple peel and other fruit waste contain flavonoids such as quercetin glycosides which have antioxidant properties similar to vitamin C (Dhakal et al., 2024; Hamauzu 2024). Carrots have high anthocyanin content and strong antioxidant properties, which may enhance gastrointestinal health and lower the risk of cardiovascular disease (Ikram et al., 2024). Pomelo peel, often discarded after its fruit is consumed, is rich in flavonoids and phenolic acids (Abiq et al., 2024). Grape skin and seed are the main sources of resveratrol, a renowned antioxidant (Almanza-Oliveros et al., 2024). In addition, cranberry pomace, the neglected byproduct of cranberries, has beneficial polyphenolic compounds such as anthocyanins and proanthocyanidins (Boateng and Clark, 2024). Blueberry byproducts, including peel, pulp, seed, and stems, have higher levels of phenolic compounds, especially anthocyanins, than the fruit’s flesh (Cruz et al., 2024; Luo, 2024). Lastly, soluble coffee production waste contains about 6% polyphenols and 4% tannins (de Oliveira et al., 2024). Table 1 shows the significant phenolic compounds present in different household food wastes.
5.2 Proteins
Proteins, which are essential macromolecules for various biological processes, are abundant in FW, including premium-quality proteins similar to those in meat, dairy, and eggs (Kishore, Pal, and Sarkar, 2024). Poultry and meat processing waste contain substantial muscle tissue, a rich protein source, while dairy processing waste may contain whey, a high-quality protein source (R. Khan et al., 2024). FW proteins can be classified into animal and plant-based sources, with plant-derived byproducts such as wheat bran and tofu residue being significant protein sources due to their nutritional value (Liu et al., 2023a). The byproducts resulting from the preparation or processing of meat, fish, and dairy also encompass significant quantities of protein. For example, residual dairy materials may house whey protein, a premium and complete protein source, while waste from fish processing is a reservoir of valuable biomolecules, including enzymes, functional proteins, bioactive peptides, and oils rich in omega-3 fatty acids (Frias et al., 2020). Poultry byproducts—lean meat, skins, heads, and feet—present a sustainable source of animal protein. The safe extraction and utilization of protein from FW require the development of innovative processing technologies and the implementation of sound manufacturing practices to ensure the safety and quality of the derived protein (V. C. Roy VikashChandra et al., 2023).
5.3 Dietary fiber
Dietary fiber, the non-digestible segment of plant material, is pivotal in human health and is present in discarded FW from fruit, vegetables, and grain, as well as the byproducts of food processing. There are two categories of fiber: soluble, which readily dissolves in water and helps control blood sugar levels, and insoluble, which facilitates consistent bowel movements (Patil et al., 2024). FW contains considerable fiber, with amounts varying by food type and discarded portion. For instance, banana peel contains high levels of insoluble dietary fiber and is a source of xylo-oligosaccharides. Other sources of xylo-oligosaccharides include guava pomegranate pomace and orange pomace (Chatterjee and Mazumder, 2023). Jerusalem artichoke residues, typically discarded, can be used to extract high-purity soluble dietary fiber. Notwithstanding its substantial dietary fiber content, garlic peel is frequently discarded because of its limited soluble dietary fiber content (Liu et al., 2023a). Nevertheless, adjusting the composition of dietary fiber derived from garlic peel has the potential to improve its solubility. Fructo-oligosaccharides, found in plants like banana peel, Jerusalem artichokes, garlic, and cassava waste, are excellent soluble dietary fiber sources (Liu et al., 2023a; M; Martins et al., 2023). Oat bran is a significant source of β-glucan and dietary fiber, while household FW, particularly orange peel, is a rich source of pectin. The oat bran segment serves as an abundant reservoir of β-glucan and dietary fiber, with no less than 5.5% weight of β-glucan and 16.0% dry-weight dietary fiber, with at least one-third being soluble (Selvamuthukumaran, 2024a). Domestic FW has attracted attention for its pectin content, and various types of FW are abundant in pectin. Judith and colleagues discovered that the pectin yield from orange peel was exceptionally high, reaching 247 mg/g dry weight and positioning it as an optimal source of dietary fiber (Morais et al., 2020). The research also highlighted that apple waste is the second-largest pectin source, following citrus fruits (Selvamuthukumaran, 2024a).
5.4 Vitamins and minerals
Vitamins and minerals, essential micronutrients for human health, are abundant in FW from overproduction or spoilage. FW from fruits and vegetables is abundant in vitamins C and B-complex, and carotenoids, precursors of vitamin A, are found in discarded carrot and pumpkin bark and peel. Tomato waste, particularly skin, has high lycopene content, which is a carotenoid. Similarly, shrimp waste, a byproduct of this major international crustacean commodity, contains astaxanthin, a valuable carotenoid with antioxidant and anti-inflammatory properties, which is used in nutritional supplements, functional foods, cosmetics, and livestock feed (Liu et al., 2023a). FW offers a potential solution to reduce waste and recover essential nutrients (Talan et al., 2021). The recovery of these nutrients could enable the fortification of food or the production of nutritional supplements which could improve nutrition and decrease dependence on conventional sources. The detection of essential minerals such as calcium, sodium, iron, magnesium, potassium, and zinc are encouraging, but more research is required to understand the nutritional value of vitamins in FW and develop effective extraction methods. (Kussmann, Cunha, and Berciano, 2023). Fruit, vegetables, grains, dairy products, and meat leftovers are common dietary sources of these minerals (Rao, Bast, and De Boer, 2021). For instance, fruit and vegetable peels have high calcium and potassium content, and dairy product waste is rich in calcium and phosphorus. Thus, FW offers the potential to recover essential nutrients and reduce waste, promoting a more fair and sustainable food system (Direito et al., 2021). Table 2 signifies the bioactive compounds and value-added products that can be obtained from different domestic waste sources.
6 Traditional and novel techniques for the isolation of bioactive compounds
The choice of extraction method for removing chemicals from fruit and vegetable waste (FVW) is crucial, as it determines the outcome: it influences the diversity and number of bioactive compounds that can be obtained. In other words, the optimal utilization of FVW depends on the optimal extraction method (Fărcaş et al., 2019). The choice of solvent, which mainly depends on the polarity of the target compound, affects the effectiveness of traditional extraction techniques (Chojnacka and Moustakas, 2024). Other factors to consider are molecular compatibility, mass transfer efficiency, co-solvent use, environmental impact, human toxicity, and economic feasibility. To optimize the extraction of bioactive compounds, common methods such as Soxhlet extraction, hydro-distillation, and maceration need to account for these parameters (Carrasco-Sandoval et al., 2021; R; Martins et al., 2023a).
Soxhlet extraction, a method for isolating lipids, was developed in 1879 by the German chemist Franz Ritter von Soxhlet (R. Martins et al., 2023b). It is widely used method for extracting valuable compounds from natural sources, serving as a standard for evaluating newer extraction techniques (Nuralın, 2024). The procedure is as follows. A thimble with a small amount of dried material is placed in a distillation flask filled with a chosen solvent. The solvent evaporates and condenses, dissolving the desired components from the solid plant material in the thimble (Ibrahim et al., 2024). The target compounds accumulate in the main flask liquid, which automatically returns to the flask when it reaches a certain level. The solvent is then continuously recycled to the thimble, and this cycle is repeated until the extraction is complete (Jagannath and Biradar, 2019).
Maceration is a widely used low-cost technique for extracting bioactive compounds and essential oils from plants (Ranjha et al., 2020). It is a technique that enhances the extraction of bioactive compounds and essential oils from plant materials by accelerating the diffusion and dilution of concentrated liquids. Maceration involves three steps: grinding the plant material, dissolving it in a solvent in a vessel, and filtering and pressing the liquid. Hydro distillation is an older method that does not require organic solvents and can be applied to fresh plant material (Ibrahim et al., 2024). It consists of placing the plant material in a specific still chamber, adding water, and boiling the mixture (Hikal et al., 2021). By applying steam to the plant material instead of immersing it, this method extracts the desired bioactive molecules that require steam and hot water to be released. However, hydro distillation may also result in the loss of some volatile chemicals that are sensitive to high temperatures (Nguyen et al., 2023). Conventional extraction suffers from several disadvantages, such as prolonged extraction time, expensive and high-quality solvents, considerable solvent loss, limited extraction selectivity, and thermal degradation of heat-sensitive compounds (T. Wang et al., 2024).
Non-conventional extraction methods are innovative and alternative approaches that overcome the inherent limitations of conventional methods (Osorio-Tobón, 2020). These advanced techniques, developed in the last five decades, offer promising prospects for efficient and selective extraction. Some of the most notable and applicable of these techniques are enzyme-assisted extraction, microwave-assisted extraction, ultrasound-assisted extraction, and pulsed electric field extraction (M. Sharma et al., 2024).
6.1 Super-critical fluid extraction
Supercritical fluid extraction (SFE) is an environmentally friendly method for extracting bioactive compounds from natural sources. SFE uses supercritical carbon dioxide (SC-CO2) or other fluids that are economical and efficient. By carefully regulating the temperature and pressure of the fluids, this technique allows for selective extraction using various fluids. SFE is an effective solvent extraction technique that offers advantages over traditional solvent extraction methods, such as increased efficiency and environmental friendliness for the successful extraction of polyphenols, flavonoids, oils, and phenolics from a variety of plant materials. (Ortiz-Sanchez et al., 2024). Employing supercritical fluid extraction to recover bioactive components from orange peel waste (OPW), extracted hesperidin—a flavonoid with anti-inflammatory and antioxidant qualities—in significant quantities (8.18 g/kg OPW db.). SFE has the potential to improve the bioactive chemical output and quality of food waste (Figure 2).
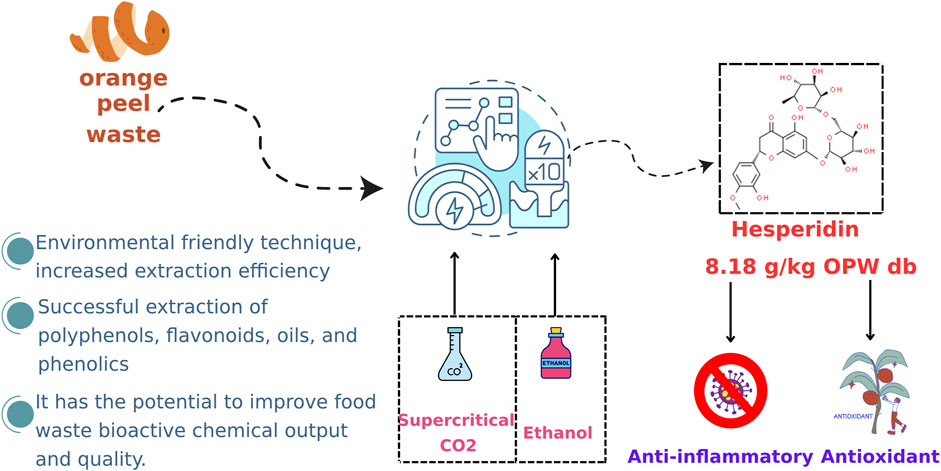
Figure 2. Bioactive compounds extracted from orange-peel waste through super-critical fluid extraction (SFE).
6.2 Sub-critical water extraction
The process of extracting phenolic compounds by subcritical water extraction (SWE) has become increasingly popular. When water is heated to between 100 °C and 374 °C under pressure and keeps its liquid condition below the critical pressure of 22 MPa, it is referred to as subcritical water. Gbashi et al. (2017) investigated the effectiveness of SWE in extracting polyphenolic compounds from Terminalia chebula Retz. fruit. The study revealed that the amount of gallic and ellagic acid extracted increased with subcritical water temperature up to 180°C, while the highest amount of corilagin was obtained at 120°C. The experiment involved subjecting the samples to temperatures of 100 °C–220°C for 20 min; at 160°C, the reaction times ranged from 10 to 50 min (Kim et al., 2017), Enhancing subcritical water hydrolysis in pumpkin leaves produces certain phenolic chemicals. The hydrolyzate of pumpkin leaves include the primary phenolic compounds gentisic, ferulic, p-coumaric, and caffeic acids (Figure 3). Mayanga-Torres et al. (2017) extracted total phenolic components from two typical coffee waste leftovers (powder and defatted cake) using subcritical water and semi-continuous flow conditions. The largest amount of total phenolic compounds (26.64 mg GAE/g of coffee powder) was recovered at 200°C and 22.5 MPa.
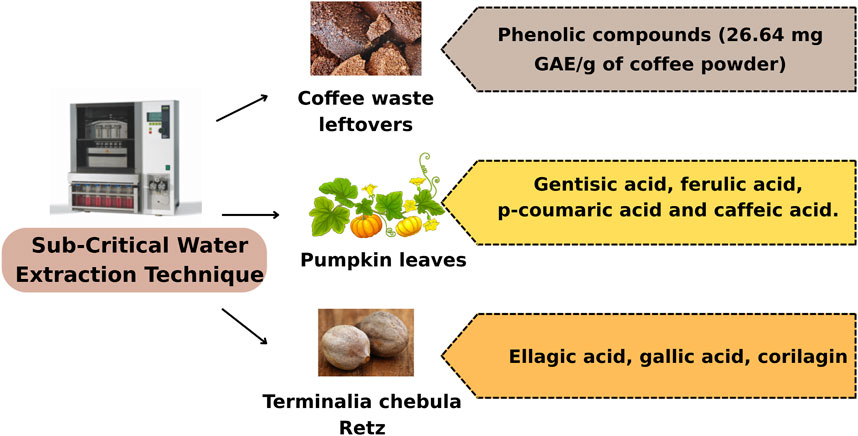
Figure 3. Extraction of biologically active compounds from plant waste employing subcritical water extraction.
6.3 Enzyme assisted extraction
Enzyme-assisted extraction (EAE) uses water as a solvent rather than organic solvents, so it is thought to be a more environmentally benign method of extracting oil and bioactive chemicals. The extraction efficiency of lycopene from the peel portion of tomato processing waste is greatly increased by using enzyme mixes with cellulolytic and pectinolytic activity. Moreover, this technology has become economically viable for mass production thanks to the affordable prices of food-grade enzyme products on the market (Prokopov et al., 2017; Costa et al., 2020). The study examined the effectiveness of the enzyme-assisted extraction of sativoside, a plant-derived bioactive compound, for its use in the food and nutraceutical sectors. It found that enzyme preparations, either singly or in mixtures, could increase the extraction of bioactive compounds from plant cells by breaking down the cell walls and facilitating extraction. Enzyme-assisted extraction, which uses mild processing conditions and enzymes that can catalyze reactions in water, could be an alternative to conventional solvent-based extraction methods. Figure 4 demonstrates the working procedure of EAE to extract bioactive compounds.
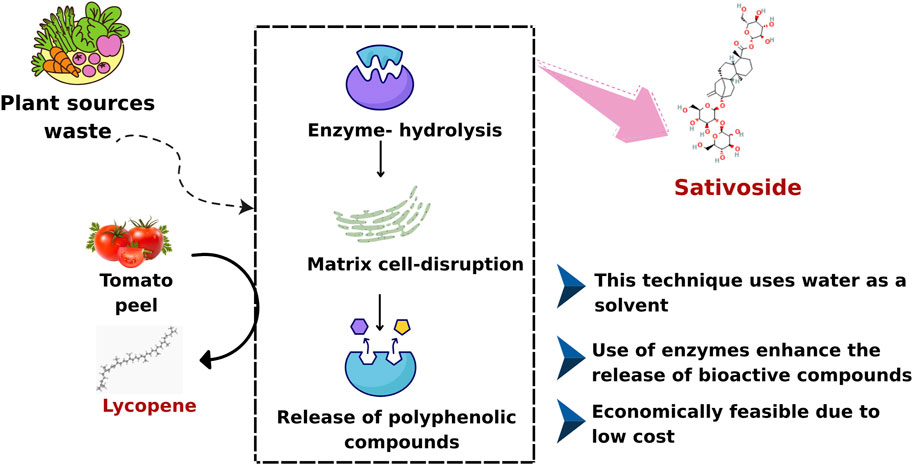
Figure 4. Extraction of bioactive compounds from plant sources waste through enzyme-assisted extraction.
Costa et al. (2020) examined the efficacy of EAE for extracting sativoside, a plant-derived bioactive compound, for application in the food and nutraceutical sectors. They found that enzyme preparations, either alone or in combination, could enhance the extraction of bioactive compounds from plant cells by facilitating cell disruption and extraction. EAE, which uses enzymes to catalyze reactions in water and mild processing conditions, could be an alternative to conventional solvent-based extraction methods.
6.4 Ultrasound assisted extraction
Ultrasound-assisted extraction (UAE) is a simple and effective method for extracting bioactive compounds from plants. The extraction yield depends on the plant type and ultrasonic frequency used (Jiménez-Moreno et al., 2020; Koraqi et al., 2023). High-performance liquid chromatography enables the identification of the extracted chemicals. Water-soluble polysaccharides from Agaricus bisporus by-products were extracted by UAE by Kaleem et al. (2019) and Aguiló-Aguayo et al. (2017). They reported the presence of β-Glucan with different particle sizes of 1.01 and 0.98 g/100 g dry bulk, respectively. The optimal extraction conditions were 15 min of extraction time, 100 m of amplitude, and 80% ethanol for precipitation, resulting in an extraction yield of 4.7%. Koraqi et al. (2023) and Sun et al. (2024) investigated the UAE of polyphenols from Pleurotus citrinopileatus mushroom with water and ethanol as solvents. They found that the optimal extraction parameters for water and ethanol were 44°C, 14 min, and 20 mL/g and 39°C, 13 min, and 20 mL/g, respectively. Koraqi et al., 2024 also demonstrated that UAE enhanced the yield and antioxidant activity of polyphenols over conventional methods (Figure 5).
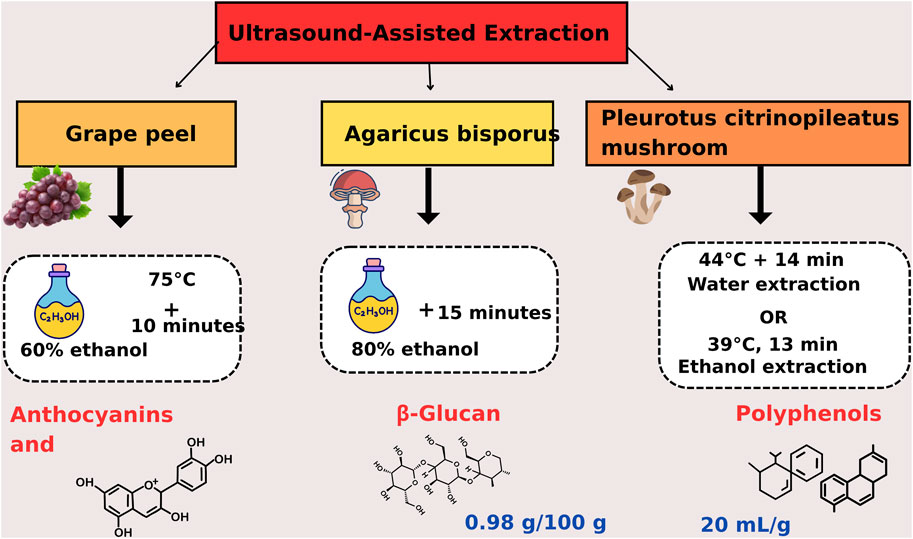
Figure 5. Extraction of bioactive compounds from peel, Agaricus bisporus, and Ieutrotus mushroom through ultrasound-assisted extraction.
6.5 Microwave-assisted extraction
Microwave-assisted extraction (MAE) is an innovative extraction technique that utilizes both solvent and microwave irradiation to extract plant metabolites. The main advantage of MAE over conventional methods such as Soxhlet and UAE is its high speed of extraction (Shen et al., 2023). Several studies have investigated the optimal conditions for MAE of different plant extracts. For example, Sonar and Rathod (2020) used water as a solvent to extract mangiferin from Mangifera indica leaves and found that the best parameters were 5 min of extraction time, 1:20 solid-to- ratio, and 272 W of microwave power, yielding 55 mg/g of mangiferin. Likewise, Filip et al. (2017) applied a response surface method to optimize the MAE of polyphenols from basil (Ocimum basilicum L.), obtaining 0.849 g of catechin equivalents/100 g of total flavonoids and 4.299 g of GAE/100 g of total polyphenols using 50% ethanol, 442 W of microwave power, and 15 min of extraction time (Ranasinghe et al., 2024). MAE of bioactive components from defatted date seeds has been an improvement.
MAE is a recommended method for extracting bioactive compounds from plant materials due to advantages such as high extraction efficiency, short extraction time, low solvent consumption, and high extraction selectivity (Figure 6). The optimal conditions for MAE were determined to be 600 W of power, 5 min of extraction time, 50% of ethanol concentration, and 30 mL/g solvent-to-solid ratio. These conditions achieved the highest yields of total phenolic content (TPC) (13.86 mg GAE/g), total flavonoid content (TFC) (4.32 mg QE/g), and antioxidant activity (DPPH) (62.31%) (Begić et al., 2020).
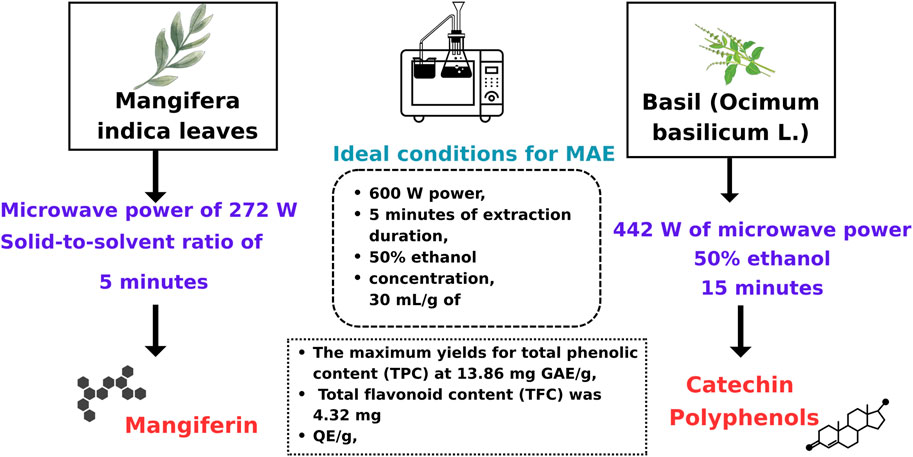
Figure 6. Extraction of bioactive compounds from Mangifera indica and basil leaf through microwave-assisted extraction.
6.6 Deep eutectic solvents
Deep eutectic solvents (DESs) are a new type of environmentally friendly and biodegradable solvent that have been recently applied to the extraction of phenolic compounds (Zainal-Abidin et al., 2017). These are formed by heating and mixing hydrogen bond donors (HBDs) and acceptors (HBAs) to a specific temperature (Abbasi, Farooq, and Anderson, 2021). DESs have the ability to dissolve phenolic compounds and exhibit lower melting points than their individual components (Wong et al., 2024). Choline chloride (ChCl) is a widely used and affordable salt for the preparation of DESs due to its non-toxicity (Elhamarnah et al., 2024). Urea, ethylene glycol, and glycerol are common hydrogen bond donors (HBDs) in DESs, along with alcohols, amino acids, carboxylic acids, and sugars. DESs are typically low-cost, easy to synthesize, and biodegradable (Lim et al., 2024). Density, viscosity, and conductivity are some of the unique properties of DESs that depend on their composition (Zhang et al., 2024). These properties can be modified using different HBAs and HBDs. DESs are usually less conductive, more viscous, and denser than water (Mu et al., 2023).
7 Utilization of bioactive compounds for food application
7.1 Nutraceuticals
Nutraceuticals are compounds extracted or purified from food sources that have pharmacological effects and confer health benefits beyond basic nutrition. These compounds, such as anti-inflammatory agents, cardio-protectives, and antioxidants, play a vital role in disease prevention and health promotion (Liu et al., 2023a). Plant-based food waste is a sustainable source of bioactive components that can replace synthetic chemicals in various applications. These components, found in different plant residues, have potential benefits for human and environmental health (Petrovic and Barbinta-Patrascu, 2023). Resveratrol-rich grape skins, a by-product of winemaking, exhibit antioxidant activity and anti-inflammatory effects that contribute to liver detoxification and cellular protection (Chedea et al., 2021a). Mango peel, a rich source of phenolic compounds and vitamins, has demonstrated potential benefits in lowering the risk factors of diseases such as cancer, cataracts, Alzheimer’s, and Parkinson’s (Chedea et al., 2021a). Additionally, caffeine extraction from waste tea leaves is a promising technique for recycling tea by-products (Debnath, Haldar, and Purkait, 2021). Recent studies have indicated that food waste can be a valuable source of diverse bioactive compounds that can confer health benefits to humans and animals. Algae and food waste contain various bioactive substances with potential health effects. Algal polysaccharides exhibit immunomodulatory and anti-allergic activities, while food waste monosaccharides possess antithrombotic, antiviral, and antioxidant properties (S. Ghosh et al., 2022). Moreover, xylo-oligosaccharides (XOS) derived from fruit pomace have prebiotic properties that stimulate the proliferation of beneficial gut microbiota (Marim and Gabardo, 2021). Galacto-oligosaccharides (GOS) and other bioactive compounds derived from dairy byproducts and potato peel also have prebiotic properties and antiparasitic activities (Ben-Othman, Jõudu, and Bhat, 2020; Gonçalves et al., 2023). Furthermore, fructo-oligosaccharides (FOS) obtained from different plant byproducts have demonstrated health benefits, such as better glycemic regulation, immune enhancement, antioxidant activity, and carcinogen suppression (Rahim et al., 2021). Banana extracts enriched with bioactive compounds exhibit anti-leishmanial activity and have potential applications in the therapy of protozoan infections (Poonam Sharma et al., 2021a; Liu et al., 2023a). The use of these various bioactive compounds from food waste supports sustainability, enhances health, and reduces environmental damage (Ribeiro et al., 2022; Liu et al., 2023a).
7.2 Food additives
Additives used in food are chemicals that are used to improve the taste, texture, or other sensory aspects of food. Overripe berries, undesirable fruits, peels that are thrown away, pomace, and seeds are examples of byproducts that provide untapped potential for creative antioxidant food additions (Nirmal et al., 2023). Recent research has proven the usefulness of discarded olive oil extract rich in polyphenols as an antioxidant in lamb patties, inhibiting both protein and lipid oxidation, retaining color, and extending shelf life by up to as 3 days. (Serra, Salvatori, and Pastorelli, 2021). Apple peel extract is effective in preventing peroxides and thiobarbituric acid-reactive compounds from oxidizing chilled minced rainbow fish (Taskaya, Ozturk-Kerimoglu, and Serdaroglu, 2024). Apple byproducts and strawberry tree extracts suppress polyphenol oxidase and peroxidase to reduce enzymatic browning, a frequent problem in fresh food, and improve consumer acceptability (V. F. R. Martins et al., 2024).
Bioactive substances found in food waste, such as terpenoids, peptides, polysaccharides, and phenolic compounds, function as organic antibacterial agents for food preservation. Shrimp and organic leafy greens have less bacterial contamination when treated with olive leaf extract (Bodie et al., 2024). Meat products that include bioactive compounds have been shown to have improved quality and longer shelf life (Ivane et al., 2024). These compounds have also been demonstrated to have antibacterial activity against microbes that cause fish spoilage. The antibacterial activity of pomegranate peel extract against S. aureus and B. cereus shows promise when applied to chicken products (Viacava, Marcovich, and Ansorena, 2024).
Although commercial colorants are primarily synthetic, natural sources such as carotenoids and anthocyanins are also present. Anthocyanins are present in berries, particularly blackberry remnants, purple sweet potatoes, crimson kale, and black carrots, and distillery byproducts provide important natural colorants and nutraceuticals (A. Ghosh et al., 2024). Carotenoids may be found in citrus peel and pomace which can act as natural colorants and prolong the shelf life of food and beverages by protecting them against pollutants, infections, and unwanted tastes (Saini et al., 2022).
Pectin is an example of a water-insoluble fiber that functions as a food that is good for the health of the gut microbiota (Baky et al., 2022). Citrus peel, which is abundant in pectin, is a great way of extracting it and using it as a gelling agent in meat, baked goods, and confections. Unlike sorghum protein, avocado byproducts, which are 30% waste product, provide edible protein with better potential to absorb water and oil and to scavenge free radicals. Avocado seed-derived starch exhibits favorable properties that make it a promising ingredient for biodegradable food packaging materials or as a thickening or gelling agent or medicinal carrier [179, 180]. These creative methods show how food waste (FW) may be turned into useful resources to improve the sustainability and quality of food (Teshome et al., 2023).
7.3 Biosurfactants
The utilization of biosurfactants, which are derived from microorganisms, has had a significant upsurge in the global market, amounting US$30.64 billion in value, thus highlighting their importance in various industries (Aslam et al., 2024; P; Singh, Patil, and Rale, 2019). Despite their traditional higher costs than synthetic counterparts, it has been demonstrated that the use of FW as a substrate for biosurfactant manufacture is sustainable and cost-effective. FW, due to its abundance and richness in nutrients, particularly excels in rhamnolipid and sophorolipid biosurfactant production. Life cycle assessments have confirmed the environmental benefits of biosurfactants, with waste whey, used kitchen oil, and soy molasses being considered promising FW sources. Future endeavors in this direction involve optimizing FW treatment, biosurfactant purification, and the overall production life cycle to enhance environmental applications and emphasize cost reduction (Jumbo et al., 2022; Sulaiman, 2024).
7.4 Single-cell proteins
Single-cell proteins (SCPs) are a broad class of proteins derived from live or dried cell biomass in microbial cultures. They can be refined, edible, or in their raw form. SCPs are a multipurpose ingredient that may be used in the place of meals high in protein for both human and animal consumption. It has a significant potential for converting organic resources and stands out from other protein sources. With an amino acid content higher than that of soybeans and fast microbial growth, SCP is resistant to environmental stressors (Wang et al., 2020; Ibáñez, Garrido-Chamorro and Barreiro, 2023).
FW is a prime example of a fermentation substrate for the synthesis of SCP. Using a two-stage fermentation method, Yarrowia lipolytica has a high protein concentration (38.8% ± 0.2% w/w biomass dry weight), highlighting the effectiveness of FW in SCP conversion [192]. The volatile fatty acids of FW fermentation broth are highlighted by photosynthetic bacteria as a potential substitute since they depend on the bioavailability of carbon sources. With a noteworthy carbon source conversion capacity of 0.99 mg-biomass/mg-chemical oxygen requirement, strategic carbon source control improves SCP production (2088.4 mg/L) (Liu et al., 2023a; Bangar et al., 2023).
Wastewater from coffee production, which is high in proteins, sugars, and salts, is an important substrate for SCP bioconversion. To produce SCPs with a protein concentration of 37.4%–39%, yeast effectively assimilates glucose, mannose, and fructose. This makes yeast an affordable source of protein for animal feed additives (Salazar-López et al., 2022). The potential utilization of byproducts to create SCPs for supplemental usage in a variety of culinary goods is demonstrated by the seamless integration of SCPs from fermented vegetable remnants into wheat flour bread without comprising sensory characteristics (Liu et al., 2023a).
7.5 Organic fertilizers
FW is composted and vermicomposted to create organic fertilizers, which are considered an environmentally beneficial substitute for their chemical equivalents and also function as sources of animal nourishment (Dutta and Kumar 2021). Growing mushrooms with FW reduces environmental impacts while enhancing crop yield and altering the bacterial populations in the soil (Huang et al., 2023). As a byproduct of anaerobic digestion, biogas waste from FW is rich in nutrients and trace elements that promote plant development, making it a great organic fertilizer or soil conditioner (Pooja Sharma et al., 2023). FW and organic waste are mixed in digesters during anaerobic digestion, with microbes turning them into nutrient-rich anaerobic digestate. This substance can initially harm plants, but eventually makes the soil better for growing them; it can help ryegrass grow more than regular fertilizers by 5%–30% (Battista et al., 2022). While FW compost helps with the chelator-enhanced phytoremediation of metal-contaminated soils, the anaerobic fermentation of kitchen waste creates volatile fatty acids with soil-cleaning capabilities for vanadium and chromium-contaminated soils (Nasir et al., 2023; Ng, 2023).
Organic fertilizers are produced using a variety of techniques, such as chemical hydrolysis and anaerobic digestion, and they may be found in a range of forms, such as soil conditioners, composts, and organic fertilizers (Pigoli et al., 2021). Liquid organic fertilizers improve overall performance by providing targeted application, quick plant absorption, reduced volume needs, and streamlined breakdown processes (Vejan et al., 2021). As demonstrated by the culture of Chlorella for the manufacture of biodiesel, the incorporation of methane-oxidizing bacteria increases the potency of organic fertilizer and promotes microalgae development (Helen La, 2022).
7.6 Bioplastics
Bioplastics are a form of plastic material derived from sustainable sources such as starch, plant oils, and microorganisms. They are considered an eco-friendly alternative to traditional plastics as they have a significantly lower impact on the environment. Although the global production of bioplastics was only 1% in 2019—equivalent to 3.8 million tons—it is expected to grow in the future (Farghali et al., 2023). The transition from oil-based synthetic plastics to bioplastics not only fits with sustainability objectives but also helps alleviate worries about global warming because bioplastics take less energy to produce (57 MJ/kg) than synthetic plastics (77 MJ/kg) (Schirmeister and Mülhaupt, 2022).
FW may be bio-converted into bioplastics, which is an efficient waste management technique. For successful deployment, pretreatment techniques that improve the biological and physicochemical characteristics of FW are necessary (Sreekala, Ismail, and Nathan, 2022). Commonly employed hydrolysis methods include enzymatic, chemical, bio, and physical. After being refined with sugarcane molasses, Alcaligenes sp. NCIM 5085 produced an astounding 70.89% of high molecular weight polyhydroxybutyrate (PHB) (Liu et al., 2023a). A different strain, Halomonas campaniensis LS21, was grown in FW and produced 70% PHB by using the cellulose, proteins, starch, and fatty acids found in the waste. Significant commercial potential exists for biopolymers such as polyhydroxyalkanoates (PHA), polybutylene succinate (PBS), polylactic acid (PLA), and polyvinyl alcohol (PVA). Nevertheless, there are still issues with the commercialization of bio-composites, such as elevated processing expenses, shortage of raw input, and lackluster cost competitiveness, which call for deliberate progress (Mujtaba et al., 2022).
7.7 Animal feed
FW is a particularly interesting substitute source material for making animal feed (Dou et al., 2024). Plant leftovers, which are usually more than 80% wet, require an extra drying stage. The periodic variations in the waste’s composition necessitate feed makers making regular adjustments to their compositions. Dried fish meal contains mostly carbohydrates (65%), followed by crude fiber (13%), ash (8%), ether extract (2%), and crude protein (12%) (Dou et al., 2024). Pigs primarily obtain their energy from carbohydrates; however, high-fiber concentrations can impair digestion and energy availability (Valente Junior et al., 2024). Vegetable waste may be used in diets, but it is necessary to supplement it with hay from Cenchrus ciliaris, a mineral combination, and regular salt (Maeresera, 2020). FW may be transformed into safe, high-quality animal feed products with increased value thanks to modern processing technology (Rohini et al., 2020). Compared to heating and ensiling, extrusion, pelletizing, and dehydration result in extended shelf life. Although there is a balanced nutritional content in livestock feed made from fermented, heat-treated FW, problems arise when it comes to sheep and cattle leftovers in raw FW. These drawbacks are mitigated, particularly for ruminants, by administering FW-derived feed. Protein-rich FW has become an important resource for making fish feed pellets, reducing the quantity of waste disposed in landfills. When supplemented with yeast, bromelain, and papain, FW derived from plants can increase grass carp growth by up to 63.6% while also improving immunity (Liu et al., 2023a; Nath et al., 2023) (Figure 7).
8 Challenges and future perspectives
While several food waste (FW) conversion technologies have been established at the laboratory scale, there are still obstacles and constraints to overcome before these technologies can be sustainably implemented at the commercial level (Sarker et al., 2024a; Grossi, Lange, and Amaral, 2024). Future research must address these concerns. The majority of the technologies discussed above are still in the research and development stage, and a major barrier is the high extraction costs due to expensive equipment, solvents, and energy. For the successful industrial implementation of FW conversion, some of the challenges to be addressed include the low efficiency of the extraction processes, the poor stability of natural bioactive compounds compared to their synthetic analogs, and difficulties in managing the residual streams that may have adverse impacts on the environment or human health if not properly treated or utilized. Therefore, it is not yet possible to conclude that FW-related problems may be successfully resolved by using these technologies alone (S. AlQahtani et al., 2024; Sarker et al., 2024a).
8.1 Sustainability concerns in FW conversion
Future difficulties will mostly consist of increasing extraction operation efficiency and creating new, ecologically friendly techniques (Sarker et al., 2024a; Goria et al., 2024). Further investigation will also be necessary to ascertain the safety and bioavailability of the extracted components as well as to find new uses for these products. The ultimate objective is to recycle and revitalize waste motivated by the pressing issue of worldwide FW production (Ali et al., 2024; D’Alessandro et al., 2024). Researchers must weigh the advantages of the extra goods acquired against the price of large-scale FW conversion (S. Roy and Roy, 2024).
8.2 Future research priorities for FW conversion
The development of targeted extraction techniques for various FW sources or bioactive components of interest will be essential in the coming years. Utilizing green solvents as much as is feasible is crucial to reducing effects on the environment and public health (A. Sharma and Lee, 2024). Setting particular requirements for value-added goods arising from FW conversion may be challenging yet valuable, given the absence of a unified norm for FW sources. Additionally, an attempt should be made to close the quality difference between the original product and value-added goods produced by FW. Replacing present products with value-added commodities created from FW is one possible area of research (Bechir et al., 2024). Further life cycle assessment studies on produce that are derived from FW are needed. In addition to exploiting waste and uncovering value, future studies on FW should focus on employing FW as an economical and high-grade source of value-added products (V. Kumar and Verma, 2024).
9 Conclusion
In conclusion, bioactive compound inherent in the chemical substances in household food waste provide significant nutritional advantages. These compounds can be extracted through a variety of techniques, encompassing both traditional methods and innovative green approaches such as enzymatic, microwave, or supercritical fluid extractions. The versatility of these extracted bioactive compounds is demonstrated by their wide-ranging applications across numerous sectors, including textiles, cosmetics, nutraceuticals, food additives, and agriculture. Furthermore, the integration of AI, robotics, IoT, and big data into waste management has revolutionized the sector, enhancing operational efficiency, automating processes, and ensuring timely waste collection and disposal. Although there are advantages to these approaches, challenges persist such as elevated extraction expenses, suboptimal yields, and safety considerations. In order to unlock the complete potential of domestic food waste, an efficiently organized waste management system at the household level is imperative. Further research is necessary to enhance the efficiency of these procedures.
Author contributions
FS: conceptualization, validation, and writing–review and editing. ZA: visualization and writing–original draft. MA: validation and writing–review and editing. KM: conceptualization and writing–original draft. AG: validation and writing–review and editing.
Funding
The author(s) declare that no financial support was received for the research, authorship, and/or publication of this article.
Acknowledgments
The authors are highly obliged to their department and institute for access to journals, books, and valuable database.
Conflict of interest
The authors declare that the research was conducted in the absence of any commercial or financial relationships that could be construed as a potential conflict of interest.
Publisher’s note
All claims expressed in this article are solely those of the authors and do not necessarily represent those of their affiliated organizations, or those of the publisher, the editors, and the reviewers. Any product that may be evaluated in this article, or claim that may be made by its manufacturer, is not guaranteed or endorsed by the publisher.
References
Abbasi, N. M., Farooq, M. Q., and Anderson, J. L. (2021). Investigating the variation in solvation interactions of choline chloride-based deep eutectic solvents formed using different hydrogen bond donors. ACS Sustain. Chem. Eng. 9 (35), 11970–11980. doi:10.1021/acssuschemeng.1c04375
Abidin, Z., Hamdi, M., Hayyan, M., Hayyan, A., and Jayakumar, N. S. (2017). New horizons in the extraction of bioactive compounds using deep eutectic solvents: a review. Anal. Chim. Acta 979, 1–23. doi:10.1016/j.aca.2017.05.012
Adlin, W. N. A. B. W., Yi Mian, V. J., Huap, A. C., and Lee, W.-C. (2020). Characterization of biosurfactants produced by bacillus subtilis using fresh and waste cooking oil enriched medium. J. Asian Sci. Res. 10 (3), 156–164. doi:10.18488/journal.2.2020.103.156.164
Aguiló-Aguayo, I., Walton, J., Viñas, I., and Tiwari, B. K. (2017). Ultrasound assisted extraction of polysaccharides from mushroom by-products. Lwt 77, 92–99. doi:10.1016/j.lwt.2016.11.043
Ahern, M., Thilsted, S., Oenema, S., and Kühnhold, H. (2021). The role of aquatic foods in sustainable healthy diets. U. N. Nutr. Discuss. Pap.
Ajlouni, S., Premier, R., and Tow, W. W. (2020). Improving extraction of lycopene from tomato waste by-products using ultrasonication and freeze drying. World J. Adv. Res. Rev. 5 (2), 177–185. doi:10.30574/wjarr.2020.5.2.0044
Akram, M. U., Yılmaz, R., Salantur, A., and Uygun, U. (2023). Bioactive compounds in lipids of selected wheat genotypes. Int. J. Food Sci. Technol. 58 (11), 6049–6058. doi:10.1111/ijfs.16713
Alancay, M., Lobo, M., and Sammán, N. (2023). Physicochemical and structural characterization of whey protein concentrate–tomato pectin conjugates. J. Sci. Food Agric. 103 (11), 5242–5252. doi:10.1002/jsfa.12612
Ali, S., Yan, Q., Sun, H., and Irfan, M. (2024). Techno-economic analysis of biogas production from domestic organic wastes and locally sourced material: the moderating role of social media based-awareness. Environ. Sci. Pollut. Res. 31 (4), 6460–6480. doi:10.1007/s11356-023-31543-z
Almalki, F. A., Alsamhi, S. H., Sahal, R., Hassan, J., Hawbani, A., Rajput, N. S., et al. (2023). Green IoT for eco-friendly and sustainable smart cities: future directions and opportunities. Mob. Netw. Appl. 28 (1), 178–202. doi:10.1007/s11036-021-01790-w
AlQahtani, S., Shahrah, E. K., Ahmed, A. E., and AlQahtani, M. A. (2024). “Sustainable management of food waste in Saudi arabia,” in Food and nutrition security in the kingdom of Saudi arabia, vol. 2: macroeconomic policy and its implication on food and nutrition security (Berlin, Heidelberg: Springer), 215–239.
Anoopkumar, A. N., Aneesh, E. M., Madhavan, A., Binod, P., Kumar Awasthi, M., Kuddus, M., et al. (2023). Advanced food waste valorization techniques for bioenergy production: a path in the direction of environmental sustainability. R. Soc. Chem. 78. doi:10.1039/BK9781837670093-00264
Aqilah, N. M. N., Rovina, K., Wen, X. L. F., and Vonnie, J. M. (2023). A review on the potential bioactive components in fruits and vegetable wastes as value-added products in the food industry. Molecules 28 (6), 2631. doi:10.3390/molecules28062631
Arifah, S. M., Budiastuti, M. T. S., and Sih Dewi, W., (2022). Identification of soybean husk and cow manure metabolites after vermicomposting. Int. J. Adv. Sci. Eng. Inf. Technol. 12 (4), 1620. doi:10.18517/ijaseit.12.4.13581
Aruwajoye, G. S., Kassim, A., Saha, A. K., and Kana, E. B. G. (2020). Prospects for the improvement of bioethanol and biohydrogen production from mixed starch-based agricultural wastes. Energies 13 (24), 6609. doi:10.3390/en13246609
Aslam, M. Z., Firdos, S., Li, Z., Wang, X., Liu, Y., Qin, X., et al. (2022). Detecting the mechanism of action of antimicrobial peptides by using microscopic detection techniques. Foods 11 (18), 2809. doi:10.3390/foods11182809
Aslam, R., Mobin, M., Zehra, S., and Aslam, J. (2024). “Biosurfactants production utilizing microbial resources,” in Industrial applications of biosurfactants and microorganisms (Amsterdam, Netherlands: Elsevier), 39–58.
Azis, F. A., Rijal, M., Suhaimi, H., and Emeroylariffion Abas, Pg (2022). Patent landscape of composting technology: a review. Inventions 7 (2), 38. doi:10.3390/inventions7020038
Baky, M. H., Mohamed, S., Ezzelarab, N., Shao, P., Elshahed, M. S., and Mohamed, A. F. (2022). Insoluble dietary fibers: structure, metabolism, interactions with human microbiome, and role in gut homeostasis. Crit. Rev. Food Sci. Nutr. 64, 1–15. doi:10.1080/10408398.2022.2119931
Balli, D., Cecchi, L., Pieraccini, G., Innocenti, M., Benedettelli, S., and Mulinacci, N. (2022). What’s new on total phenols and γ-oryzanol derivatives in wheat? a comparison between modern and ancient varieties. J. Food Compos. Analysis 109, 104453. doi:10.1016/j.jfca.2022.104453
Bangar, S. P., Chaudhary, V., Kajla, P., Balakrishnan, G., and Phimolsiripol, Y. (2023). Strategies for upcycling food waste in the food production and supply chain. Trends Food Sci. Technol. 143, 104314. doi:10.1016/j.tifs.2023.104314
Battista, F., Strazzera, G., Valentino, F., Gottardo, M., Villano, M., Matos, M., et al. (2022). New insights in food waste, sewage sludge and green waste anaerobic fermentation for short-chain volatile fatty acids production: a review. J. Environ. Chem. Eng. 10 (5), 108319. doi:10.1016/j.jece.2022.108319
Bechir, S., Lachi, O., Taouzinet, L., Messaoudene, L., Allam, A., Madani, K., et al. (2024). Exploring the environmental and economic impact of fruits and vegetable loss quantification in the food industry. Environ. Sci. Pollut. Res. 31 (4), 5221–5241. doi:10.1007/s11356-023-31311-z
Begić, S., Horozić, E., Alibašić, H., Bjelić, E., Seferović, S., Kozarević, E. C., et al. (2020). Antioxidant capacity and total phenolic and flavonoid contents of methanolic extracts of urtica dioica L. By different extraction techniques. Int. Res. J. Pure Appl. Chem. 21 (23), 207–214. doi:10.9734/irjpac/2020/v21i2330319
Ben-Othman, S., Jõudu, I., and Bhat, R. (2020). Bioactives from agri-food wastes: present insights and future challenges. Molecules 25 (3), 510. doi:10.3390/molecules25030510
Bernat, K. (2023). Post-consumer plastic waste management: from collection and sortation to mechanical recycling. Energies 16 (8), 3504. doi:10.3390/en16083504
Bettio, G., Zardo, L. C., Rosa, C. A., and Záchia Ayub, M. A. (2020). Bioconversion of ferulic acid into aroma compounds by newly isolated yeast strains of the Latin american biodiversity. Biotechnol. Prog. 37 (1), e3067. doi:10.1002/btpr.3067
Bhat, I.Ul H., and Bhat, R. (2021). Quercetin: a bioactive compound imparting cardiovascular and neuroprotective benefits: scope for exploring fresh produce, their wastes, and by-products. Biology 10 (7), 586. doi:10.3390/biology10070586
Bhatia, L., Jha, H., Sarkar, T., and Sarangi, P. K. (2023). Food waste utilization for reducing carbon footprints towards sustainable and cleaner environment: a review. Int. J. Environ. Res. Public Health 20 (3), 2318. doi:10.3390/ijerph20032318
Boateng, I. D., and Clark, K. (2024). Trends in extracting agro-byproducts’ phenolics using non-thermal technologies and their combinative effect: mechanisms, potentials, drawbacks, and safety evaluation. Food Chem. 437, 137841. doi:10.1016/j.foodchem.2023.137841
Bodie, A. R., Wythe, L. A., Dittoe, D. K., Rothrock, M. J., Bryan, C. A. O. ’, and Ricke, S. C. (2024). Alternative additives for organic and natural ready-to-eat meats to control spoilage and maintain shelf life: current perspectives in the United States. Foods 13 (3), 464. doi:10.3390/foods13030464
Bulkan, G., Yudhanti, G. T., Sitaram, S., Millati, R., Wikandari, R., and Taherzadeh, M. J. (2022). Inhibitory and stimulatory effects of fruit bioactive compounds on edible filamentous fungi: potential for innovative food applications. Fermentation 8 (6), 270. doi:10.3390/fermentation8060270
Caballero, S., Mereles, L., Burgos-Edwards, A., Nelson, A., Coronel, E., Rocio, V., et al. (2021). Nutritional and bioactive characterization of sicana odorifera naudim vell. seeds by-products and its potential hepatoprotective properties in swiss albino mice. Biology 10 (12), 1351. doi:10.3390/biology10121351
Caponio, G. R., Calasso, M., Tamma, G., Gambacorta, G., and De Angelis, M. (2023). Promising application of grape pomace and its agri-food valorization: source of bioactive molecules with beneficial effects. Sustainability 15 (11), 9075. doi:10.3390/su15119075
Cárdenas, J., Orjuela, A., Sánchez, D. L., Narváez, P. C., Katryniok, B., and Clark, J. (2021). Pre-treatment of used cooking oils for the production of green chemicals: a review. J. Clean. Prod. 289, 125129. doi:10.1016/j.jclepro.2020.125129
Carrasco-Sandoval, J., Rebolledo, P., Peterssen-Fonseca, D., Fischer, S., Wilckens, R., Aranda, M., et al. (2021). A fast and selective method to determine phenolic compounds in quinoa (Chenopodium quinoa will) seeds applying ultrasound-assisted extraction and high-performance liquid chromatography. Chem. Pap. 75, 431–438. doi:10.1007/s11696-020-01313-z
Carreira-Casais, A., Carpena, M., Pereira, A. G., Chamorro, F., Soria-Lopez, A., Perez, P. G., et al. (2021). Critical variables influencing the ultrasound-assisted extraction of bioactive compounds—a review. Chem. Proc. 5 (1), 50. doi:10.3390/CSAC2021-10562
Carullo, G., Ramunno, A., Sommella, E., De Luca, M., Lucia Belsito, E., Frattaruolo, L., et al. (2022). Ultrasound-assisted extraction, chemical characterization, and impact on cell viability of food wastes derived from southern Italy autochthonous citrus fruits. Antioxidants 11 (2), 285. doi:10.3390/antiox11020285
Celano, R., Docimo, T., Lisa Piccinelli, A., Gazzerro, P., Tucci, M., Sanzo, R.Di, et al. (2021). Onion peel: turning a food waste into a resource. Antioxidants 10 (2), 304. doi:10.3390/antiox10020304
Çelik, Ö., and Temiz, H. (2020). Traditional yogurt dilemma; rich flavor vs. microbial safety: an investigation on volatile aroma profiles, chemical, and microbiological qualities of traditional yogurts, J. central Eur. Agric. 21 (3), 461–475. doi:10.5513/jcea01/21.3.2813
Chatterjee, B., and Mazumder, D. (2023). A critical review of the advances in valorizing agro-industrial wastes through mixed culture fermentation. J. Environ. Chem. Eng. 12, 111838. doi:10.1016/j.jece.2023.111838
Chavan, S., Yadav, B., Atmakuri, A., Tyagi, R. D., Wong, J. W. C., and Drogui, P. (2022). Bioconversion of organic wastes into value-added products: a review. Bioresour. Technol. 344, 126398. doi:10.1016/j.biortech.2021.126398
Chedea, V. S., Lucia Tomoiagǎ, L., Macovei, Ş. O., Claudiu Mǎgureanu, D., Iliescu, M. L., Bocsan, I. C., et al. (2021a). Antioxidant/pro-Oxidant actions of polyphenols from grapevine and wine by-products-base for complementary therapy in ischemic heart diseases. Front. Cardiovasc. Med. 8, 750508. doi:10.3389/fcvm.2021.750508
Chojnacka, K., and Moustakas, K. (2024). Anaerobic digestate management for carbon neutrality and fertilizer use: a review of current practices and future opportunities. Biomass Bioenergy 180, 106991. doi:10.1016/j.biombioe.2023.106991
Costa, J. R., Tonon, R. V., Cabral, L., Gottschalk, L., Pastrana, L., and Pintado, M. E. (2020). Valorization of agricultural lignocellulosic plant byproducts through enzymatic and enzyme-assisted extraction of high-value-added compounds: a review. ACS Sustain. Chem. Eng. 8 (35), 13112–13125. doi:10.1021/acssuschemeng.0c02087
Cruz, M. A. A. S., Pedro, P. S. C., Araújo-Lima, C. F., Freitas-Silva, O., and Teodoro, A. J. (2024). Hybrid fruits for improving health—a comprehensive review. Foods 13 (2), 219. doi:10.3390/foods13020219
D’Alessandro, C., Szopik-Depczyńska, K., Tarczyńska-Łuniewska, M., Silvestri, C., and Ioppolo, G. (2024). Exploring circular economy practices in the healthcare sector: a systematic review and bibliometric analysis. Sustainability 16 (1), 401. doi:10.3390/su16010401
Debnath, B., Haldar, D., and Purkait, M. K. (2021). Potential and sustainable utilization of tea waste: a review on present status and future trends. J. Environ. Chem. Eng. 9 (5), 106179. doi:10.1016/j.jece.2021.106179
Delgado, A., Rodriguez, R., and Staszewska, A. (2023). Tackling food waste in the tourism sector: towards a responsible consumption trend. Sustainability 15 (17), 13226. doi:10.3390/su151713226
Dhakal, M., Ghimire, S., Karki, G., Deokar, G. S., Al-Asmari, F., and Nirmal, N. P. (2024). “Fruit waste: potential bio-resource for extraction of nutraceuticals and bioactive compounds,” in Bioactive extraction and application in food and nutraceutical industries (Berlin, Heidelberg: Springer), 299–332.
Direito, R., Rocha, J., Sepodes, B., and Eduardo-Figueira, M. (2021). From Diospyros kaki L. (Persimmon) phytochemical profile and health impact to new product perspectives and waste valorization. Nutrients 13 (9), 3283. doi:10.3390/nu13093283
Dong-Shin, K., Kim, M.-Bo, and Lim, S.-B. (2017). Enhancement of phenolic production and antioxidant activity from buckwheat leaves by subcritical water extraction. Prev. Nutr. Food Sci. 22 (4), 345–352. doi:10.3746/pnf.2017.22.4.345
Dou, Z., Dierenfeld, E. S., Wang, X., Chen, X., and Shurson, G. C. (2024). A critical analysis of challenges and opportunities for upcycling food waste to animal feed to reduce climate and resource burdens. Resour. Conservation Recycl. 203, 107418. doi:10.1016/j.resconrec.2024.107418
Dutta, S., and Kumar, M. S. (2021). Potential of value-added chemicals extracted from floral waste: a review. J. Clean. Prod. 294, 126280. doi:10.1016/j.jclepro.2021.126280
Elhamarnah, Y., Qiblawey, H., and Nasser, M. (2024). A review on deep eutectic solvents as the emerging class of green solvents for membrane fabrication and separations. J. Mol. Liq. 398, 124250. doi:10.1016/j.molliq.2024.124250
Fărcaş, A. C., Socaci, S. A., and Diaconeasa, Z. M. (2019). Introductory chapter: from waste to new resources. Food Preserv. Waste Exploitation 2, 3. doi:10.5772/intechopen.89442
Farghali, M., Mohamed, I. M. A., Osman, A. I., and Rooney, D. W. (2023). Seaweed for climate mitigation, wastewater treatment, bioenergy, bioplastic, biochar, food, pharmaceuticals, and cosmetics: a review. Environ. Chem. Lett. 21 (1), 97–152. doi:10.1007/s10311-022-01520-y
Fazli, M. M., Zanganeh, H., and Hassanzadazar, H. (2022). Effect of heating on disposal point of main edible oils available in Iran market. Food Sci. Nutr. 10 (12), 4394–4402. doi:10.1002/fsn3.3033
Feng, M., Lu, X., Wang, L., Zhang, J., Yang, S., Shi, C., et al. (2019). Preparation of the catalytic chitin/zn composite by combined ionic liquid–inorganic salt aqueous solution from shrimp shells. Acs Sustain. Chem. Eng. 7, acssuschemeng.9b00418. doi:10.1021/acssuschemeng.9b00418
Freitas, L. C., Barbosa, J. R., da Costa, A. L. C., Wariss Figueiredo Bezerra, F., Pinto, R. H. H., and de Carvalho Junior, R. N. (2021). From waste to sustainable industry: how can agro-industrial wastes help in the development of new products? Resour. Conservation Recycl. 169, 105466. doi:10.1016/j.resconrec.2021.105466
Frias, J. M., O’Neill, G., Burgess, K., and Barry-Ryan, C. (2020). 49th. Annual food science and technology conference: book of abstracts. Dublin, Ireland: IFSTI.
Galasso, C., Orefice, I., Pellone, P., Cirino, P., Miele, R., Ianora, A., et al. (2018). On the neuroprotective role of astaxanthin: new perspectives? Mar. Drugs 16 (8), 247. doi:10.3390/md16080247
García-Vargas, M. C., María del, M. C., and Castro, E. (2020). Avocado-derived biomass as a source of bioenergy and bioproducts. Appl. Sci. 10 (22), 8195. doi:10.3390/app10228195
Gaur, V. K., Sharma, P., Sirohi, R., Varjani, S., Taherzadeh, M. J., Chang, J.-S., et al. (2022). Production of biosurfactants from agro-industrial waste and waste cooking oil in a circular bioeconomy: an overview. Bioresour. Technol. 343, 126059. doi:10.1016/j.biortech.2021.126059
Gbashi, S., Ayodeji Adebo, O., Piater, L., Edwin Madala, N., and Njobeh, P. B. (2017). Subcritical water extraction of biological materials. Sep. Purif. Rev. 46 (1), 21–34. doi:10.1080/15422119.2016.1170035
Ghosh, A., Nadeem, F., Choudhary, A. K., Grace Manzeke-Kangara, M., Manzeke-Kangara, M. G., Joy, E. J. M., et al. (2024). OPEN ACCESS EDITED BY. Divers. Agri-Food Prod. Syst. Nutr. Secur. 162. doi:10.3389/fnut.2023.1078667
Ghosh, S., Sarkar, T., Pati, S., Abdul Kari, Z., Atan Edinur, H., and Chakraborty, R. (2022). Novel bioactive compounds from marine sources as a tool for functional food development. Front. Mar. Sci. 9, 832957. doi:10.3389/fmars.2022.832957
Glasser, D. (2023). Science AMA series: I’m professor david glasser, winner of the inaugural harry oppenheimer gold medal and director of materials and process synthesis at UNISA. I’m here with professor diane hildebrandt to talk about waste to energy conversion. AMA!. Authorea. doi:10.15200/winn.147913.31470
Gonçalves, A. C., Nunes, A. R., Falcão, A., Alves, G., and Silva, L. (2021). Dietary effects of anthocyanins in human health: a comprehensive review. Pharmaceuticals 14 (7), 690. doi:10.3390/ph14070690
Gonçalves, D. A., González, A., Roupar, D., Teixeira, J. A., and Nobre, C. (2023). How prebiotics have been produced from agro-industrial waste: an overview of the enzymatic technologies applied and the models used to validate their health claims. Trends Food Sci. Technol. 135, 74–92. doi:10.1016/j.tifs.2023.03.016
Gonçalves, L. A., Lorenzo, J. M., and Antonio Trindade, M. (2021). Fruit and agro-industrial waste extracts as potential antimicrobials in meat products: a brief review. Foods 10 (7), 1469. doi:10.3390/foods10071469
Górecka, D., Wawrzyniak, A., Jędrusek-Golińska, A., Dziedzic, K., Hamułka, J., Kowalczewski, P. Ł., et al. (2020). Lycopene in tomatoes and tomato products. Open Chem. 18 (1), 752–756. doi:10.1515/chem-2020-0050
Goria, K., Singh, H. M., Singh, A., Kothari, R., and Tyagi, V. V. (2024). Insights into biohydrogen production from algal biomass: challenges, recent advancements and future directions. Int. J. Hydrogen Energy 52, 127–151. doi:10.1016/j.ijhydene.2023.03.174
Grossi, L. B., Lange, L. C., and Amaral, M. C. S. (2024). Transition pathway towards more sustainable waste management practices for end-of-life reverse osmosis membranes: challenges and opportunities in Brazil. J. Clean. Prod. 435, 140571. doi:10.1016/j.jclepro.2024.140571
Gupta, R., and Shankar, R. (2024). Managing food security using blockchain-enabled traceability system. Benchmarking Int. J. 31 (1), 53–74. doi:10.1108/bij-01-2022-0029
Hardoko, H., Budi Sasmito, B., Puspitasari, Y. E., Afandi, H. M., and Maulia, N. (2017). Study of glucosamine production from shrimp shells by fermentation using trichoderma harzianum. J. Exp. Life Sci. 7 (2), 115–121. doi:10.21776/ub.jels.2017.007.02.10
Hikal, W. M., Hussein, A. H. S.-Al A., Tkachenko, K. G., Bratovcic, A., Szczepanek, M., and Rodriguez, R. M. (2021). Sustainable and environmentally friendly essential oils extracted from pineapple waste. Biointerface Res. Appl. Chem. 12 (5), 6833–6844. doi:10.33263/BRIAC125.68336844
Hoskin, R. T., Grace, M. H., Xiong, J., and Lila, M. A. (2023). Spray-drying microencapsulation of blackcurrant and cocoa polyphenols using underexplored plant-based protein sources. J. Food Sci. 88 (6), 2665–2678. doi:10.1111/1750-3841.16590
Huang, C., Han, X., Luo, Q., Nie, Y., Kang, M., Chen, Y., et al. (2023). Agro-based spent mushroom compost substrates improve soil properties and microbial diversity in greenhouse tomatoes. Agronomy 13 (9), 2291. doi:10.3390/agronomy13092291
Hui, C. X., Dan, Ge, Alamri, S., and Toghraie, D. (2023). Greening smart cities: an investigation of the integration of urban natural resources and smart city technologies for promoting environmental sustainability. Sustain. Cities Soc. 99, 104985. doi:10.1016/j.scs.2023.104985
Hunter, S., Gutiérrez Blanco, E., and Borrion, A. (2021). Expanding the anaerobic digestion map: a review of intermediates in the digestion of food waste. Sci. Total Environ. 767, 144265. doi:10.1016/j.scitotenv.2020.144265
Hussain, M., Saeed, F., Niaz, B., Ali, I., and Tufail, T. (2022). Biochemical and structural characterization of ferulated arabinoxylans extracted from nixtamalized and non-nixtamalized maize bran. Foods 11 (21), 3374. doi:10.3390/foods11213374
Hussain, S., Jõudu, I., and Bhat, R. (2020). Dietary fiber from underutilized plant resources—a positive approach for valorization of fruit and vegetable wastes. Sustainability 12 (13), 5401. doi:10.3390/su12135401
Ibáñez, A., Garrido-Chamorro, S., and Barreiro, C. (2023). Microorganisms and climate change: a not so invisible effect. Microbiol. Res. 14 (3), 918–947. doi:10.3390/microbiolres14030064
Ibrahim, M. F., Mohamad, N., Fairus, M. J. M., Jenol, M. A., and Aziz, S. A. (2024). Chemical substitutes from agricultural and industrial by-products: bioconversion, bioprocessing, and biorefining. Essent. Oil Pineapple Wastes, 103–121.
Ikram, A., Rasheed, A., Ahmad Khan, A., Khan, R., Ahmad, M., Bashir, R., et al. (2024). Exploring the health benefits and utility of carrots and carrot pomace: a systematic review. Int. J. Food Prop. 27 (1), 180–193. doi:10.1080/10942912.2023.2301569
Ivane, N. M. A., Wang, W., Ma, Q., Wang, J., Liu, Y., Haruna, S. A., et al. (2024). RETRACTED: the effect of different Capsicum annuum components on the quality and shelf-life of chicken breast meat. Food control. 159, 110263. doi:10.1016/j.foodcont.2023.110263
Jagannath, A., and Biradar, R. (2019). Comparative evaluation of soxhlet and ultrasonics on the structural morphology and extraction of bioactive compounds of lemon (citrus limon L.) peel. J. Food Chem. Nanotechnol. 5 (3), 56–64. doi:10.17756/jfcn.2019-072
Jayanandini, G. (2023). “Effective solid waste segregation for sustainable disposal: model for coimbatore city, India.” In Proceedings of the 2023 9th International Conference on Advanced Computing and Communication Systems (ICACCS), 1:431–436. Coimbatore, India, March 17-18, 2023
Jiménez-Moreno, N., Esparza, I., Bimbela, F., Gandía, L. M., and Ancín-Azpilicueta, C. (2020). Valorization of selected fruit and vegetable wastes as bioactive compounds: opportunities and challenges. Crit. Rev. Environ. Sci. Technol., 50 (20), 2061–2108. doi:10.1080/10643389.2019.1694819
Johari, S. A. M., Ayoub, M., Inayat, A., Ullah, S., Uroos, M., Naqvi, S. R., et al. (2022). Utilization of dairy scum waste as a feedstock for biodiesel production via different heating sources for catalytic transesterification. Chembioeng Rev. 9 (6), 605–632. doi:10.1002/cben.202200003
Jouhara, H., Czajczyńska, D., Ghazal, H., Krzyżyńska, R., Anguilano, L., Reynolds, A. J., et al. (2017). Municipal waste management systems for domestic use. Energy 139, 485–506. doi:10.1016/j.energy.2017.07.162
Jumbo, R. B., Coulon, F., Cowley, T., Azuazu, I., Atai, E., Bortone, I., et al. (2022). Evaluating different soil amendments as bioremediation strategy for wetland soil contaminated by crude oil. Sustainability 14 (24), 16568. doi:10.3390/su142416568
Junior, V., Teixeira, D., Genova, J. L., Kim, S. W., Saraiva, A., and Rocha, G. C. (2024). Carbohydrases and phytase in poultry and pig nutrition: a review beyond the nutrients and energy matrix. Animals 14 (2), 226. doi:10.3390/ani14020226
Kaleem, M., Ahmad, A., Rai, M. A., and Raja, G. K. (2019). Ultrasound-assisted phytochemical extraction condition optimization using response surface methodology from perlette grapes (vitis vinifera). Processes 7 (10), 749. doi:10.3390/pr7100749
Kandpal, V., Jaswal, A., Gonzalez, E. D. R. S., and Agarwal, N. (2024). “Circular economy principles: shifting towards sustainable prosperity,” in Sustainable energy transition: circular economy and sustainable financing for environmental, social and governance (ESG) practices (Berlin, Heidelberg: Springer), 125–165.
Khan, R., Brishti, F. H., Arulrajah, B., Goh, Y. M., Rahim, M. H. A., Karim, R., et al. (2024). Mycoprotein as a meat substitute: production, functional properties, and current challenges-a review. Int. J. Food Sci. Technol. 59 (1), 522–544. doi:10.1111/ijfs.16791
Khan, S., Anjum, R., Turab Raza, S., Nazir, A. B., and Ihtisham, M. (2022). Technologies for municipal solid waste management: current status, challenges, and future perspectives. Chemosphere 288, 132403. doi:10.1016/j.chemosphere.2021.132403
Khorram, A. G., Fallah, N., Nasernejad, B., Afsham, N., Esmaelzadeh, M., and Vatanpour, V. (2023). Electrochemical-based processes for produced water and oily wastewater treatment: a review. Chemosphere 338, 139565. doi:10.1016/j.chemosphere.2023.139565
Kim, M. Y., Lee, B. W., Lee, H.-Un, Lee, Yu Y., Lee, J. Y., Woo, K. S., et al. (2019). Phenolic compounds and antioxidant activity in sweet potato after heat treatment. J. Sci. Food Agric. 99 (15), 6833–6840. doi:10.1002/jsfa.9968
Kishore, A., Pal, B., and Sarkar, P. (2024). Camelids for sustainability: a socio-economic perspective. Asian J. Environ. Ecol. 23 (1), 53–72. doi:10.9734/ajee/2024/v23i1521
Koraqi, H., Anka, T. P., Khalid, W., Kumar, N., and Pareek, S. (2023). Optimization of experimental conditions for bioactive compounds recovery from raspberry fruits (rubus idaeus L.) by using combinations of ultrasound-assisted extraction and deep eutectic solvents. Appl. Food Res. 3 (2), 100346. doi:10.1016/j.afres.2023.100346
Koraqi, H., Qazimi, B., Khalid, W., Stanoeva, J. P., Sehrish, A., Siddique, F., et al. (2023). Optimized conditions for extraction, quantification and detection of bioactive compound from nettle (urtica dioica L.) using the deep eutectic solvents, ultra-sonication and liquid chromatography-mass spectrometry (LC-DAD-ESI-MS/MS). Int. J. Food Prop. 26 (1), 2171–2185. doi:10.1080/10942912.2023.2244194
Koraqi, H., Yuksel Aydar, A., Khalid, W., Ercisli, S., Rustagi, S., Ramniwas, S., et al. (2024). Ultrasound-assisted extraction with natural deep eutectic solvent for phenolic compounds recovery from rosa damascene mill.: experimental design optimization using central composite design. Microchem. J. 196, 109585. doi:10.1016/j.microc.2023.109585
Kumar, A., Bhardwaj, S., and Samadder, S. R. (2023). Evaluation of methane generation rate and energy recovery potential of municipal solid waste using anaerobic digestion and landfilling: a case study of dhanbad, India. Waste Manag. Res. 41 (2), 407–417. doi:10.1177/0734242X221122494
Kumar, J. (2021). “Microbial hydrolysed feather protein as a source of amino acids and protein in the diets of animals including poultry,” in Advances in poultry nutrition research (London, England: IntechOpen). doi:10.5772/intechopen.96925
Kumar, V., and Verma, P. (2024). Pulp-paper industry sludge waste biorefinery for sustainable energy and value-added products development: a systematic valorization towards waste management. J. Environ. Manag. 352, 120052. doi:10.1016/j.jenvman.2024.120052
Kusmayadi, A., Lu, P.-H., Huang, C.-Yu, Leong, Y. K., Yen, H.-W., and Chang, J.-S. (2022). Integrating anaerobic digestion and microalgae cultivation for dairy wastewater treatment and potential biochemicals production from the harvested microalgal biomass. Chemosphere 291, 133057. doi:10.1016/j.chemosphere.2021.133057
Kussmann, M., Henrique Abe Cunha, D., and Berciano, S. (2023). Bioactive compounds for human and planetary health. Front. Nutr. 10, 1193848. doi:10.3389/fnut.2023.1193848
Kusumasari, S., Syabana, M. A., Pamela, V. Y., and Meindrawan, B. (2024). Potential use of food waste in food processing to add nutritional value. In E3S Web Conf. 483:02006. doi:10.1051/e3sconf/202448302006
La, H., and Patrick, A. J. (2022). Role of biochar in the removal of organic and inorganic contaminants from waste gas streams. BioChar Appl. Bioremediation Contam. Syst. 89. doi:10.1515/9783110734003-005
Liang, J. (2023). Characteristics of hazardous waste and recovery. Highlights Sci. Eng. Technol. 59, 91–96. doi:10.54097/hset.v59i.10065
Liaqat, H., Jin Kim, K., Park, S., Jung, S. K., Park, S. H., Lim, S., et al. (2021). Antioxidant effect of wheat germ extracts and their antilipidemic effect in palmitic acid-induced steatosis in hepg2 and 3t3-l1 cells. Foods 10 (5), 1061. doi:10.3390/foods10051061
Liaqat, I., Ali, S., Butt, A., Iqbal Durrani, A., Zafar, U., Saleem, S., et al. (2022). Purification and characterization of keratinase from bacillus licheniformis dcs1 for poultry waste processing. J. Oleo Sci. 71 (5), 693–700. doi:10.5650/jos.ess21426
Lim, E., Pang, J.-S. Y., and Von Lau, Ee (2024). A comparative study of thermophysical properties between choline chloride-based deep eutectic solvents and imidazolium-based ionic liquids. J. Mol. Liq. 395, 123895. doi:10.1016/j.molliq.2023.123895
Lirikum, L. N. K., Thyug, L., Mozhui, L., and Mozhui, L. (2022). Vermicomposting: an eco-friendly approach for waste management and nutrient enhancement. Trop. Ecol. 63 (3), 325–337. doi:10.1007/s42965-021-00212-y
Liu, Q., Zhao, J., Liu, S., Fan, Y., Mei, J., Liu, X., et al. (2021). Positive intervention of insoluble dietary fiber from defatted rice bran on hyperlipidemia in high fat diet fed rats. J. Food Sci. 86 (9), 3964–3974. doi:10.1111/1750-3841.15812
Liu, Z., de Souza, T. S. P., Holland, B., Dunshea, F., Barrow, C., and Suleria, H. A. R. (2023a). Valorization of food waste to produce value-added products based on its bioactive compounds. Processes 11 (3), 840. doi:10.3390/pr11030840
Lodu, F. D., Suwitari, Ni K. E., and Suariani, L. (2022). Response of native chickens (3-10 weeks) fed on diets substituated with graded levels of sweet potato fermentation. Seas. Sustain. Environ. Agric. Sci. (1) 6, 58–63. doi:10.22225/seas.6.1.4887.58-63
Luo, X. (2024). “Natural antioxidants from fruit by-products for active packaging applications,” in Biobased packaging materials: sustainable alternative to conventional packaging materials (Berlin, Heidelberg: Springer), 163–192.
Lyu, F., Luiz, S. F., Perdomo Azeredo, D. R., Cruz, A. G., Ajlouni, S., and Senaka Ranadheera, C. (2020). Apple pomace as a functional and healthy ingredient in food products: a review. Processes 8 (3), 319. doi:10.3390/pr8030319
Maalouf, A., and Mavropoulos, A. (2023). Re-assessing global municipal solid waste generation. Waste Manag. Res. 41 (4), 936–947. doi:10.1177/0734242X221074116
Machineni, L. (2023). Waste to wealth and health: bio-recovery and applications of chitin and its derivatives. Int. J. Environ. Technol. Manag. 26 (3–5), 341–359. doi:10.1504/ijetm.2023.130779
Maeresera, T. (2020). Comparative assessment of the effects of horse manure and urea as nitrogen sources on seed yield, forage production and nutritional quality of Cenchrus ciliaris post seed harvesting. Botsw. Univ. Agric. Nat. Resour.
Mahmud, N., Valizadeh, S., Oyom, W., and Tahergorabi, R. (2024). Exploring functional plant-based seafood: ingredients and health implications. Trends Food Sci. Technol. 144, 104346. doi:10.1016/j.tifs.2024.104346
Maqbool, Z., Khalid, W., Taimoor Atiq, H., Koraqi, H., Javaid, Z., Alhag, S. K., et al. (2023). Citrus waste as source of bioactive compounds: extraction and utilization in health and food industry. Molecules 28 (4), 1636. doi:10.3390/molecules28041636
Marim, A. V. C., and Gabardo, S. (2021). Xylooligosaccharides: prebiotic potential from agro-industrial residue, production strategies and prospects. Biocatal. Agric. Biotechnol. 37, 102190. doi:10.1016/j.bcab.2021.102190
Martínez-Gutiérrez, E. (2023). Study of influence of extraction method on the recovery bioactive compounds from peel avocado. Molecules 28 (6), 2557. doi:10.3390/molecules28062557
Martínez-Meza, Y., Pérez-Jiménez, J., Rocha-Guzmán, N. E., Rodriguez-Garcia, M. E., Alonzo-Macías, M., and Reynoso-Camacho, R. (2021). Modification on the polyphenols and dietary fiber content of grape pomace by instant controlled pressure drop. Food Chem. 360, 130035. doi:10.1016/j.foodchem.2021.130035
Martins, M., Ávila, P. F., Poletto, P., and Goldbeck, R. (2023). “Polysaccharide degradation for oligosaccharide production with nutraceutical potential for the food industry,” in Polysaccharide degrading biocatalysts (Amsterdam, Netherlands: Elsevier), 335–363.
Martins, R., Barbosa, A., Advinha, B., Sales, H., Pontes, R., and Nunes, J. (2023). Green extraction techniques of bioactive compounds: a state-of-the-art review. Processes 11 (8), 2255. doi:10.3390/pr11082255
Martins, V. F. R., Pintado, M. E., Morais, R. M. S. C., and Morais, A. M. M. B. (2024). Recent highlights in sustainable bio-based edible films and coatings for fruit and vegetable applications. Foods 13 (2), 318. doi:10.3390/foods13020318
Mateus, A. R. S., Pena, A., Sendón, R., Almeida, C., Almeida Nieto, G., Khwaldia, K., et al. (2023). By-products of dates, cherries, plums and artichokes: a source of valuable bioactive compounds. Trends Food Sci. Technol. 131, 220–243. doi:10.1016/j.tifs.2022.12.004
Mayanga-Torres, P. C., Lachos-Perez, D., Rezende, C. A., Prado, J. M., Ma, Z., Tompsett, G. T., et al. (2017). Valorization of coffee industry residues by subcritical water hydrolysis: recovery of sugars and phenolic compounds. J. Supercrit. Fluids 120, 75–85. doi:10.1016/j.supflu.2016.10.015
Melakessou, F., Paul, K., Alnaffakh, N., Faye, S., and Khadraoui, D. (2020). Heterogeneous sensing data analysis for commercial waste collection. Sensors 20 (4), 978. doi:10.3390/s20040978
Mendoza, C., Patricia, M., Omar Navarro-Cortez, R., Hernández-Uribe, J. P., Bello-Pérez, L. A., and Vargas-Torres, A. (2022). Sweet potato color variety and flour production drying method determine bioactive compound content and functional properties of flour. J. Food Process. Preserv. 46 (10). doi:10.1111/jfpp.16852
Meneguzzo, F., Ciriminna, R., Zabini, F., and Pagliaro, M. (2020). Review of evidence available on hesperidin-rich products as potential tools against covid-19 and hydrodynamic cavitation-based extraction as a method of increasing their production. Processes 8 (5), 549. doi:10.3390/pr8050549
Miah, T., Demoro, P., Nduka, I., De Luca, F., Abate, S., and Arrigo, R. (2023). Orange peel biomass-derived carbon supported cu electrocatalysts active in the co2-reduction to formic acid. Chemphyschem 24 (7), e202200589. doi:10.1002/cphc.202200589
Miron, A., Sarbu, A., Zaharia, A., Sandu, T., Iovu, H., Claudiu Fierascu, R., et al. (2022). A top-down procedure for synthesizing calcium carbonate-enriched chitosan from shrimp shell wastes. Gels 8 (11), 742. doi:10.3390/gels8110742
Morais, E. S., Costa Lopes, A. M. da, Freire, M. G., Freire, C. S. R., Coutinho, J. A. P., and Silvestre, A. J. D. (2020). Use of ionic liquids and deep eutectic solvents in polysaccharides dissolution and extraction processes towards sustainable biomass valorization. Molecules 25 (16), 3652. doi:10.3390/molecules25163652
Mostaghimi, K., and Behnamian, J. (2023a). Waste minimization towards waste management and cleaner production strategies: a literature review. Environ. Dev. Sustain. 25 (11), 12119–12166. doi:10.1007/s10668-022-02599-7
Mu, L., Gao, J., Zhang, Q., Kong, F., Zhang, Yu, Ma, Z., et al. (2023). Research progress on deep eutectic solvents and recent applications. Processes 11 (7), 1986. doi:10.3390/pr11071986
Mujtaba, M., Lipponen, J., Ojanen, M., Puttonen, S., and Vaittinen, H. (2022). Trends and challenges in the development of bio-based barrier coating materials for paper/cardboard food packaging; a review. Sci. Total Environ. 851, 158328. doi:10.1016/j.scitotenv.2022.158328
Nagarajan, J., Kay, H. P., Prasad K, N., Ramakrishnan, N., Aldawoud, T. M. S., Galanakis, C. M., et al. (2020). Extraction of carotenoids from tomato pomace via water-induced hydrocolloidal complexation. Biomolecules 10 (7), 10–1019. doi:10.3390/biom10071019
Nasir, A., Khan, M. I., Asif, M., Nawaz, M. F., and Ahmad, I. (2023). Farmyard manure enhances phytoremediation and mitigates Pb, Cd, and drought stress in ryegrass. Sustainability 15 (21), 15319. doi:10.3390/su152115319
Nassef, S. L., Asael, M. A., and Abd-El-Motaleb, N. M. (2023). Production of novel healthy barely flakes by using naked barley, naked oats and unused baladi bread (sahla). Food Technol. Res. J. 1 (2), 64–76. doi:10.21608/ftrj.2023.291271
Nath, P. C., Ojha, A., Debnath, S., Sharma, M., Kumar Nayak, P., Sridhar, K., et al. (2023). Valorization of food waste as animal feed: a step towards sustainable food waste management and circular bioeconomy. Animals 13 (8), 1366. doi:10.3390/ani13081366
Nemati, A., Alizadeh Khaledabad, M., and Ghasempour, Z. (2020). Optimization of orange juice formulation through using lactose-hydrolyzed permeate by rsm methodology. Food Sci. Nutr. 8 (8), 4584–4590. doi:10.1002/fsn3.1774
Ng, C. C., and Uvarajan, T. (2023). Roles and significance of chelating agents for potentially toxic elements (PTEs) phytoremediation in soil: a review. J. Environ. Manage 341, 117926. doi:10.1016/j.jenvman.2023.117926
Nguyen, T. L., Ora, A., Häkkinen, S. T., Ritala, A., Räisänen, R., Kallioinen-Mänttäri, M., et al. (2023). Innovative extraction technologies of bioactive compounds from plant by-products for textile colorants and antimicrobial agents. Biomass Convers. Biorefinery, 1–30. doi:10.1007/s13399-023-04726-4
Nirmal, N. P., Chandra Khanashyam, A., Mundanat, A. S., Shah, K., Sajith Babu, K., Thorakkattu, P., et al. (2023). Valorization of fruit waste for bioactive compounds and their applications in the food industry. Foods 12 (3), 556. doi:10.3390/foods12030556
Novelina, A. A., Nerishwari, K., Hapsari, S., and Hari, P. D. (2022). Utilization of avocado seed powder (persea americana mill.) as a mixture of modified cassava flour in making cookies. Iop Conf. Ser. Earth Environ. Sci. 1059 (1), 012060. doi:10.1088/1755-1315/1059/1/012060
Nugrahaningtyas, K. D., Lukitawati, R., Aulia Mukhsin, S., Fadlulloh, Z., Sabiilagusti, A. I., Budiman, A. W., et al. (2022). Conversion of waste cooking oil into green diesel using ni/mor and cu/mor catalysts. J. Phys. Conf. Ser. 2190 (1), 012037. doi:10.1088/1742-6596/2190/1/012037
Nuralın, L. (2024). Investigation of ziziphus jujube seeds as a new source of taxifolin and silibinin with three different extraction methods. Microchem. J. 198, 110137. doi:10.1016/j.microc.2024.110137
Obi, O. E., Mong, O. O., Nleonu, E. C., Kalu, P. N., Onyeocha, C. E., Ndubuisi, C. O., et al. (2023). Determination of combustion characteristics of densified biomass fuels from agricultural and domestic wastes. J. Energy Res. Rev. 15 (2), 26–34. doi:10.9734/jenrr/2023/v15i2304
Ogunkunle, O., Laseinde, O. T., Oloyede, C. T., and Jekayinfa, S. O. (2024). “Biodiesel production from municipal waste,” in Waste valorization for bioenergy and bioproducts (Amsterdam, Netherlands: Elsevier), 283–313.
Oliveira, A. de, Moreira, T. F. M., Silva, B. P., Oliveira, G., Teixeira, V. M. C., Watanabe, L. S., et al. (2024). Characterization and bioactivities of coffee husks extract encapsulated with polyvinylpyrrolidone. Food Res. Int. 178, 113878. doi:10.1016/j.foodres.2023.113878
Oliveira, B. E. de, Contini, L. R. F., Abla Garcia, V., Cili, L. P., Leite das Chagas, E. G., Andreo, M. A., et al. (2022). Valorization of grape by-products as functional and nutritional ingredients for healthy pasta development. J. Food Process. Preserv. 46 (12), 46. doi:10.1111/jfpp.17245
Ortiz-Sanchez, M., Carlos, A. C. A., and Solarte-Toro, J. C. (2024). Orange peel waste as a source of bioactive compounds and valuable products: insights based on chemical composition and biorefining. Biomass 4 (1), 107–131. doi:10.3390/biomass4010006
Osorio-Tobón, J. F. (2020). Recent advances and comparisons of conventional and alternative extraction techniques of phenolic compounds. J. Food Sci. Technol. 57, 4299–4315. doi:10.1007/s13197-020-04433-2
Oyebode, O. J. (2024). Promoting integrated and sustainable solid waste management system in A developing city for public health and cleaner environment. J. Harbin Eng. Univ. 45 (01).
Ozogul, F., Cagalj, M., Šimat, V., Ozogul, Y., Tkaczewska, J., Hassoun, A., et al. (2021). Recent developments in valorisation of bioactive ingredients in discard/seafood processing by-products. Trends Food Sci. Technol. 116, 559–582. doi:10.1016/j.tifs.2021.08.007
Paesa, M., Ancín-Azpilicueta, C., Velderrain-Rodríguez, G. R., Martín Belloso, O., Gualillo, O., Osada, J., et al. (2022). Anti-inflammatory and chondroprotective effects induced by phenolic compounds from onion waste extracts in atdc-5 chondrogenic cell line. Antioxidants (12) 11, 2381. doi:10.3390/antiox11122381
Paesa, M., Nogueira, D. P., Velderrain-Rodríguez, G. R., Esparza, I., Jiménez-Moreno, N., Mendoza, G., et al. (2022). Valorization of onion waste by obtaining extracts rich in phenolic compounds and feasibility of its therapeutic use on colon cancer. Antioxidants 11 (4), 733. doi:10.3390/antiox11040733
Pantoja, L. S. G., Amante, E. R., Rodrigues, A. M. da C., and Luiza, H. M. da S. (2022). World scenario for the valorization of byproducts of Buffalo milk production chain. J. Clean. Prod. 364, 132605. doi:10.1016/j.jclepro.2022.132605
Panzella, L., Moccia, F. D., Nasti, R., Marzorati, S., Verotta, L., and Napolitano, A. (2020). Bioactive phenolic compounds from agri-food wastes: an update on green and sustainable extraction methodologies. Front. Nutr. 7, 60. doi:10.3389/fnut.2020.00060
Patil, M. S., Bavaskar, K. R., Rane, B. R., Jain, A. S., and Shirkhedkar, A. A. (2024). “Introduction to dietary fibers,” in Food supplements and dietary fiber in health and disease (Florida 32905 USA: Apple Academic Press), 3–47.
Pazla, R., Jamarun, N., Elhaida’s, E., Elhaida’s, A., Yanti, G., and Ikhlas, Z. (2023). The impact of replacement of concentrates with fermented tithonia (tithonia diversifolia) and avocado waste (persea americana miller) in fermented sugarcane shoots (saccharum officinarum) based rations on consumption, digestibility, and production perform. Adv. Animal Veterinary Sci. 11 (3). doi:10.17582/journal.aavs/2023/11.3.394.403
Petrovic, S. M., and Barbinta-Patrascu, M.-E. (2023). Organic and biogenic nanocarriers as bio-friendly systems for bioactive compounds’ delivery: state-of-the art and challenges. Materials 16 (24), 7550. doi:10.3390/ma16247550
Petruk, V., Stalder, F., Ishchenko, V., Vasylkivskyi, I., Petruk, R., Turchyk, P., et al. 2016. Household waste management. The European experience. Nilan-Ltd, Ukraine, ВНТУ.
Pigoli, A., Zilio, M., Tambone, F., Mazzini, S., Schepis, M., Meers, E., et al. (2021). Thermophilic anaerobic digestion as suitable bioprocess producing organic and chemical renewable fertilizers: a full-scale approach. Waste Manag. 124, 356–367. doi:10.1016/j.wasman.2021.02.028
Polivanova, O. B., Gins, E. M., Moskalev, E. A., Voinova, M. S., Koroleva, A. K., Semenov, A.Zh., et al. (2021). Quality evaluation, phytochemical characteristics and estimation of beta-carotene hydroxylase 2 (chy2) alleles of interspecific potato hybrids. Agronomy 11 (8), 1619. doi:10.3390/agronomy11081619
Pozzo, D., Alessandro, S. C., and Cozzani, V. (2023). Techno-economic impact of lower emission standards for waste-to-energy acid gas emissions. Waste Manag. 166, 305–314. doi:10.1016/j.wasman.2023.05.013
Prokopov, T., Georgieva, M., Nikolova, M., Atanasov, D., and Taneva, D. (2021). Drying characteristics of onion processing waste. Ser. Ii - For. • Wood Ind. • Agric. Food Eng. (1) 14 (63), 193–200. doi:10.31926/but.fwiafe.2021.14.63.1.17
Prokopov, T., Nikolova, M., Dobrev, G., and Taneva, D. (2017). Enzyme-assisted extraction of carotenoids from Bulgarian tomato peels. Acta Aliment. 46 (1), 84–91. doi:10.1556/066.2017.46.1.11
Purkait, M. K., Duarah, P., and Das, P. P. (2023). Recovery of bioactives from food wastes. Florida, US: CRC Press.
Putra, N. R., Rizkiyah, D. N., Qomariyah, L., Aziz, A. H. A., Veza, I., and Yunus, M. A. C. (2023). Experimental and modeling for catechin and epicatechin recovery from peanut skin using subcritical ethanol. J. Food Process Eng. 46 (3). doi:10.1111/jfpe.14275
Rahim, M. A., Saeed, F., Khalid, W., Hussain, M., and Anjum, F. M. (2021). Functional and nutraceutical properties of fructo-oligosaccharides derivatives: a review. Int. J. Food Prop. 24 (1), 1588–1602. doi:10.1080/10942912.2021.1986520
Raj, R., Shams, R., Kumar Pandey, V., Dash, K. K., Singh, P., and Bashir, O. (2023). Barley phytochemicals and health promoting benefits: a comprehensive review. J. Agric. Food Res. 14, 100677. doi:10.1016/j.jafr.2023.100677
Rajput, A., Singh, V., Kumar, S., Chaubey, C., and Kumar, P. (2024). Chapter–11 sustainable farming practices: balancing productivity and environmental health. A Compr. Explor. Soil, Water, Air Pollut. Agric. 186.
Ranasinghe, M., Sivapragasam, N., Hussein, M., Airouyuwa, J. O., Manikas, I., Sundarakani, B., et al. (2024). Valorizing date seeds in biscuits: a novel approach to incorporate bioactive components extracted from date seeds using microwave-assisted extraction. Resour. Environ. Sustain. 15, 100147. doi:10.1016/j.resenv.2023.100147
Rane, N. (2023). Integrating leading-edge artificial intelligence (AI), Internet of things (IOT), and Big data technologies for smart and sustainable architecture, engineering and construction (AEC) industry: challenges and future directions. Eng. Constr. (AEC) Industry Challenges Future Dir. doi:10.2139/ssrn.4616049
Ranjha, M. M. A. N., Amjad, S., Ashraf, S., Khawar, L., Safdar, M. N., Jabbar, S., et al. (2020). Extraction of polyphenols from apple and pomegranate peels employing different extraction techniques for the development of functional date bars. Int. J. Fruit Sci. 20 (Suppl. 3), S1201–S1221. doi:10.1080/15538362.2020.1782804
Rao, M., Bast, A., and De Boer, A. (2021). Valorized food processing by-products in the EU: finding the balance between safety, nutrition, and sustainability. Sustainability 13 (8), 4428. doi:10.3390/su13084428
Reich, J., Nolte, M., Lagodny, P., Unger, P., Meyer, C., Guder, C., et al. (2023). “Circular economy through technology for waste-to-energy,” in Circular economy adoption: catalysing decarbonisation through policy instruments (Berlin, Heidelberg: Springer), 331–377.
Ribeiro, T. B., Voss, G. B., Coelho, M. C., and Pintado, M. E. 2022. “Food waste and by-product valorization as an integrated approach with zero waste: future challenges.” In Future Foods. (Cambridge, Massachusetts, United States: Academic Press), 569–596. doi:10.1016/b978-0-323-91001-9.00017-7
Rico, D., Peñas, E., Peña’s, M. del C. G., Martínez-Villaluenga, C., Dilip, K., Birsan, R. I., et al. (2020). Sprouted barley flour as a nutritious and functional ingredient. Foods 9 (3), 296. doi:10.3390/foods9030296
Rocha, R., Pinela, J., Abreu, R. M. V., Añibarro-Ortega, M., Pires, T. C. S. P., Luísa Saldanha, A., et al. (2020). Extraction of anthocyanins from red raspberry for natural food colorants development: processes optimization and in vitro bioactivity. Processes 8 (11), 1447. doi:10.3390/pr8111447
Rohini, C., Geetha, P. S., Vijayalakshmi, R., Mini, M. L., and Pasupathi, E. (2020). Global effects of food waste. J. Pharmacogn. Phytochemistry 9 (2), 690–699.
Roy, P., Mohanty, A. K., Dick, P., and Misra, M. (2023). A review on the challenges and choices for food waste valorization: environmental and economic impacts. ACS Environ. Au 3 (2), 58–75. doi:10.1021/acsenvironau.2c00050
Roy, S., and Roy, R. N. (2024). Appraising and overcoming the barriers of RFID implementation in a process industry in New Zealand. Int. J. Intelligent Enterp. 11 (1), 1–20. doi:10.1504/ijie.2023.10056444
Roy, V. C., Rakibul Islam, Md, Sultana, S., Yeasmin, M., Park, J.-S., Lee, H.-J., et al. 2023a. “Trash to treasure: an up-to-Date understanding of the valorization of seafood by-products, targeting the major bioactive compounds.” Mar. Drugs, 21 (9): 485.
Russo, C., Maugeri, A., Lombardo, G. E., Musumeci, L., Barreca, D., Rapisarda, A., et al. (2021). The second life of citrus fruit waste: a valuable source of bioactive compounds. Molecules 26 (19), 5991. doi:10.3390/molecules26195991
Sabra, A., Netticadan, T., and Wijekoon, C., 2021. "Grape bioactive molecules, and the potential health benefits in reducing the risk of heart diseases", Food Chem. X, 12:100149. doi:10.1016/j.fochx.2021.100149
Sahradyan, S. I., and Ghazaryan, A. 2023. "Preparation of functional cookies with red grape pomace bio-dust", Agriscience Technol.:2, 204–208. doi:10.52276/25792822-2023.2-204.2-204
Saini, R. K., Ranjit, A., Sharma, K., Prasad, P., Shang, X., Gowda, K. G. M., et al. (2022). Bioactive compounds of citrus fruits: a review of composition and health benefits of carotenoids, flavonoids, limonoids, and terpenes. Antioxidants 11 (2), 239. doi:10.3390/antiox11020239
Salazar-López Julieta, N., Barco-Mendoza, G. A., Shain Zuñiga-Martínez, B., Abraham Domínguez-Avila, J., Robles-Sánchez, R. M., et al. (2022). Single-cell protein production as a strategy to reincorporate food waste and agro by-products back into the processing chain. Bioengineering 9 (11), 623. doi:10.3390/bioengineering9110623
Samota, M. K., Kaur, M., Sharma, M., Sarita, V. K., Thakur, J., Rawat, M., et al. (2023). Hesperidin from citrus peel waste: extraction and its health implications. Qual. Assur. Saf. Crops Foods 15 (2), 71–99. doi:10.15586/qas.v15i2.1256
Santos, B., and Mendes, C. (2024). GIS-based approach for optimizing biowaste collection services in rural small sized municipalities. Waste Manag. Bull. 2 (1), 9–20. doi:10.1016/j.wmb.2023.12.001
Santos, L. G. dos, and Martins, V. G. (2022). Recovery of phenolic compounds from purple onion peel using bio-based solvents: thermal degradation kinetics and color stability of anthocyanins. J. Food Process. Preserv. 46 (12). doi:10.1111/jfpp.17161
Sar, T., Harirchi, S., Ramezani, M., Bulkan, G., Akbas, M. Y., Pandey, A., et al. (2022). Potential utilization of dairy industries by-products and wastes through microbial processes: a critical review. Sci. Total Environ. 810, 152253. doi:10.1016/j.scitotenv.2021.152253
Sarker, A., Ahmmed, R., Ahsan, S. M., Rana, J., Kumar Ghosh, M., and Nandi, R. (2024a). A comprehensive review of food waste valorization for the sustainable management of global food waste. Sustain. Food Technol. 2, 48–69. doi:10.1039/d3fb00156c
Schirmeister, C. G., and Mülhaupt, R. (2022). Closing the carbon loop in the circular plastics economy. Macromol. Rapid Communications43 13, 2200247. doi:10.1002/marc.202200247
Selvamuthukumaran, M. (2024a). Wealth out of food processing waste: ingredient recovery and valorization. Florida, US: CRC Press.
Serra, V., Salvatori, G., and Pastorelli, G. (2021). Dietary polyphenol supplementation in food producing animals: effects on the quality of derived products. Animals 11 (2), 401. doi:10.3390/ani11020401
Shah, H. H., Amin, M., Pepe, F., Mancusi, E., and Fareed, A. G. (2023). Overview of environmental and economic viability of activated carbons derived from waste biomass for adsorptive water treatment applications. Environ. Sci. Pollut. Res., 1–26. doi:10.1007/s11356-023-30540-6
Shah, M. A., Sunooj, K. V., and Ahmad Mir, S. (2023). Cereal-based food products. Berlin, Heidelberg: Springer Nature.
Shah, U., and Hajoori, M. (2022). Production of single cell protein from fruit waste. Available at: https://www.ijraset.com/research-paper/production-of-single-cell-protein-from-fruit-waste#:∼:text=The%20bioconversion%20of%20fruit%20waste,for%20single%20cell%20protein%20production.
Shahidi, F., Danielski, R., and Silva, G. R. B.Da (2022). Effect of processing on the preservation of bioactive compounds in traditional and exotic fruits: a review. J. Food Bioact. 18. doi:10.31665/jfb.2022.18308
Sharma, A., and Lee, B.-S. (2024). Toxicity test profile for deep eutectic solvents: a detailed review and future prospects. Chemosphere 350, 141097. doi:10.1016/j.chemosphere.2023.141097
Sharma, M., Bains, A., Goksen, G., Sridhar, K., Sharma, M., Amin, M. K., et al. (2024). Bioactive polysaccharides from aegle marmelos fruit: recent trends on extraction, bio-techno functionality, and food applications. Food Sci. Nutr. doi:10.1002/fsn3.4026
Sharma, N., Tiwari, V., Vats, S., Kumari, A., Chunduri, V., Kaur, S., et al. (2020). Evaluation of anthocyanin content, antioxidant potential and antimicrobial activity of black, purple and blue colored wheat flour and wheat-grass juice against common human pathogens. Molecules 25 (24), 5785. doi:10.3390/molecules25245785
Sharma, P., Bano, A., Verma, K., Yadav, M., Varjani, S., Singh, S. P., et al. (2023). Food waste digestate as biofertilizer and their direct applications in agriculture. Bioresour. Technol. Rep. 23, 101515. doi:10.1016/j.biteb.2023.101515
Sharma, P., Gaur, V. K., Sirohi, R., Varjani, S., Kim, S. H., and Wong, J. W. C. (2021a). Sustainable processing of food waste for production of bio-based products for circular bioeconomy. Bioresour. Technol. 325, 124684. doi:10.1016/j.biortech.2021.124684
Sharma, R., Mokhtari, S., Jafari, S. M., and Sharma, S. (2021). Barley-based probiotic food mixture: health effects and future prospects. Crit. Rev. Food Sci. Nutr. 62 (29), 7961–7975. doi:10.1080/10408398.2021.1921692
Shen, L., Pang, S., Zhong, M., Sun, Y., Qayum, A., Liu, Y., et al. (2023). A comprehensive review of ultrasonic assisted extraction (UAE) for bioactive components: principles, advantages, equipment, and combined technologies. Ultrason. Sonochemistry 101, 106646. doi:10.1016/j.ultsonch.2023.106646
Singh, M., Singh, M., and Singh, S. K. (2024). Tackling municipal solid waste crisis in India: insights into cutting-edge technologies and risk assessment. Sci. Total Environ. 917, 170453. doi:10.1016/j.scitotenv.2024.170453
Singh, P., Patil, Y., and Rale, V. (2019). Biosurfactant production: emerging trends and promising strategies. J. Appl. Microbiol. 126 (1), 2–13. doi:10.1111/jam.14057
Singh, S., Negi, T., Sagar, N. A., Kumar, Y., Tarafdar, A., Sirohi, R., et al. (2022). Sustainable processes for treatment and management of seafood solid waste. Sci. Total Environ. 817, 152951. doi:10.1016/j.scitotenv.2022.152951
Singh, T. A., Sharma, M., Sharma, M., Sharma, G. D., Kumar Passari, A., and Bhasin, S. (2022). Valorization of agro-industrial residues for production of commercial biorefinery products. Fuel 322, 124284. doi:10.1016/j.fuel.2022.124284
Singha, R., and Singha, S. (2024). “Composting for a sustainable future: turning waste into nutrient-rich soil,” in Water-soil-plant-animal nexus in the era of climate change (Hershey, Pennsylvania: IGI Global), 279–297.
Sivashanmugam, S., Rodriguez, S., Rahimian, F. P., Elghaish, F., and Dawood, N. (2023). Enhancing information standards for automated construction waste quantification and classification. Automation Constr. 152, 104898. doi:10.1016/j.autcon.2023.104898
Sonar, M. P., and Rathod, V. K. (2020). Optimization study of marmelosin (imperatorin) extraction from aegle marmelos using three phase partitioning. J. Biol. Act. Prod. Nat. 10 (5), 418–428. doi:10.1080/22311866.2020.1816215
Sreekala, A. G. V., Ismail, M. H. B., and Nathan, V. K. (2022). Biotechnological interventions in food waste treatment for obtaining value-added compounds to combat pollution. Environ. Sci. Pollut. Res. 29 (42), 62755–62784. doi:10.1007/s11356-022-21794-7
Sulaiman, I. M. (2024). Diagnosis of pathogenic microorganisms causing infectious diseases. Florida, US: CRC Press.
Sun, M., Zhuang, Y., Gu, Y., Zhang, G., Fan, X., and Ding, Y. (2024). A comprehensive review of the application of ultrasonication in the production and processing of edible mushrooms: drying, extraction of bioactive compounds, and post-harvest preservation. Ultrason. Sonochemistry 102, 106763. doi:10.1016/j.ultsonch.2024.106763
Sypka, M., Jodłowska, I., and Białkowska, A. (2021). Keratinases as versatile enzymatic tools for sustainable development. Biomolecules 11 (12), 1900. doi:10.3390/biom11121900
Talan, A., Tiwari, B., Yadav, B., Tyagi, R. D., Woon-Chung Wong, J., and Drogui, P. (2021). Food waste valorization: energy production using novel integrated systems. Bioresour. Technol. 322, 124538. doi:10.1016/j.biortech.2020.124538
Tanyanyiwa, S., and Tongowona, G. (2023). Enhancing food security through fisheries for rural communities around lake mutirikwi in Zimbabwe. Available at: https://www.intechopen.com/online-first/88454.
Tapia-Quirós, P., Montenegro-Landívar, M. F., Reig, M., Vecino, X., Luis Cortina, J., Saurina, J., et al. (2022). Recovery of polyphenols from agri-food by-products: the olive oil and winery industries cases. Foods 11 (3), 362. doi:10.3390/foods11030362
Tasinov, O., Dincheva, I., Badjakov, I., Kiselova-Kaneva, Y., Galunska, B., Nogueiras, R., et al. (2021). Phytochemical composition, anti-inflammatory and er stress-reducing potential of sambucus ebulus l. fruit extract. Plants 10 (11), 2446. doi:10.3390/plants10112446
Taskaya, A., Ozturk-Kerimoglu, B., and Serdaroglu, M. (2024). Plum (prunus domestica) extract acts as a natural antioxidant in minced sardine muscles during frozen storage. J. Aquatic Food Prod. Technol. 33 (1), 19–32. doi:10.1080/10498850.2023.2276839
Teng, Z.-lu, Guo, C., Zhao, Q., and Mubarik, M. S. (2023). Antecedents of green process innovation adoption: an AHP analysis of China’s gas sector. Resour. Policy 85, 103959. doi:10.1016/j.resourpol.2023.103959
Teshome, E., Teka, T. A., Nandasiri, R., Rout, J. R., Voukang Harouna, D., Astatkie, T., et al. (2023). Fruit by-products and their industrial applications for nutritional benefits and health promotion: a comprehensive review. Sustainability 15 (10), 7840. doi:10.3390/su15107840
Toledo, N. M. V. de, Mondoni, J., dos Santos Harada-Padermo, S., Vela-Paredes, R., Berni, P., Selani, M. M., et al. (2019). Characterization of apple, pineapple, and melon by-products and their application in cookie formulations as an alternative to enhance the antioxidant capacity. J. Food Process. Preserv. 43 (9). doi:10.1111/jfpp.14100
Torres, M. D., Fradinho, P., Rodríguez, P., Falqué, E., Santos, V., and Domínguez, H. (2020). Biorefinery concept for discarded potatoes: recovery of starch and bioactive compounds. J. Food Eng. 275, 109886. doi:10.1016/j.jfoodeng.2019.109886
Un, C. (2023). A sustainable approach to the conversion of waste into energy: landfill gas-to-fuel technology. Sustainability 15 (20), 14782. doi:10.3390/su152014782
Vejan, P., Khadiran, T., Abdullah, R., and Ahmad, N. (2021). Controlled release fertilizer: a review on developments, applications and potential in agriculture. J. Control. Release 339, 321–334. doi:10.1016/j.jconrel.2021.10.003
Venugopal, V. (2022). Green processing of seafood waste biomass towards blue economy. Curr. Res. Environ. Sustain. 4, 100164. doi:10.1016/j.crsust.2022.100164
Viacava, G. E., Marcovich, N. E., and Ansorena, M. R. (2024). “Studies on nanostructure functionalized materials for active and intelligent food packaging,” in Nanostructured materials for food packaging applications (Amsterdam, Netherlands: Elsevier), 477–511.
Vilas-Boas, A. A., Campos, D. A., Nunes, C., Ribeiro, S. M. R., Oliveira, A., Pintado, M., et al. (2020). Polyphenol extraction by different techniques for valorisation of non-compliant Portuguese sweet cherries towards a novel antioxidant extract. Sustainability 12 (14), 5556. doi:10.3390/su12145556
Walling, J. G., Sallam, A. H., Steffenson, B. J., Henson, C. A., Vinje, M. A., and Mahalingam, R. (2022). Quantitative trait loci impacting grain β-glucan content in wild barley (Hordeum vulgare ssp. spontaneum) reveals genes associated with cell wall modification and carbohydrate metabolism. Crop Sci. 62 (3), 1213–1227. doi:10.1002/csc2.20734
Wang, S., An, Z., and Wang, Z.-Wu. 2020. “Bioconversion of methane to chemicals and fuels by methane-oxidizing bacteria.” In Adv. Bioenergy, 5:169–247. Elsevier, doi:10.1016/bs.aibe.2020.04.005
Wang, S., Yan, W., and Zhao, F. (2020). Recovery of solid waste as functional heterogeneous catalysts for organic pollutant removal and biodiesel production. Chem. Eng. J. 401, 126104. doi:10.1016/j.cej.2020.126104
Wang, T., Zhu, Li, Mei, Li, and Kanda, H. (2024). Extraction and separation of natural products from microalgae and other natural sources using liquefied dimethyl ether, a green solvent: a review. Foods 13 (2), 352. doi:10.3390/foods13020352
Waqas, M., Hashim, S., Humphries, U. W., Ahmad, S., Noor, R., Shoaib, M., et al. (2023). Composting processes for agricultural waste management: a comprehensive review. Processes 11 (3), 731. doi:10.3390/pr11030731
Wong, Y. S., Yusoff, R., and Cheng Ngoh, G. (2024). Phenolic compounds extraction by assistive technologies and natural deep eutectic solvents. Rev. Chem. Eng. 40 (2), 229–246. doi:10.1515/revce-2022-0043
Yadav, J., Ray, S., Soni, M., and Kashyap, B. K. (2023). “Microbial fermentation system for the production of biopolymers and bioenergy from various organic wastes and by-products,” in Current research trends and applications in waste management (Berlin, Heidelberg: Springer), 307–339.
Yadav, R., Yadav, N., Saini, P., Kaur, D., and Kumar, R. (2020). Potential value addition from cereal and pulse processed by-products: a review. Sustain. Food Waste Manag. Concepts Innovations, 155–176.
Yu, S., Qi, Z., Jian, Li H., Ma, W., Sun, Y., Wang, X., et al. (2023). Development of an extended STIRPAT model to assess the driving factors of household carbon dioxide emissions in China. J. Environ. Manag. 325, 116502. doi:10.1016/j.jenvman.2022.116502
Zhang, S., Gai, Z., Gui, T., Chen, J., Chen, Q., and Li, Y. (2021). Antioxidant effects of protocatechuic acid and protocatechuic aldehyde: old wine in a new bottle. Evidence-Based Complementary Altern. Med. 2021, 6139308–6139319. doi:10.1155/2021/6139308
Zhu, X., Xu, Y., Zhen, G., Lu, X., Xu, S., Zhang, J., et al. (2023). Effective multipurpose sewage sludge and food waste reduction strategies: a focus on recent advances and future perspectives. Chemosphere 311, 136670. doi:10.1016/j.chemosphere.2022.136670
Keywords: waste management, food waste, biological active compounds, value-added products, waste valorization, phenolic compounds, extraction, artificial intelligence
Citation: Siddique F, Ali Z, Arshad M, Mubeen K and Ghazala A (2024) Exploration of novel eco-friendly techniques to utilize bioactive compounds from household food waste: special reference to food applications. Front. Food. Sci. Technol. 4:1388461. doi: 10.3389/frfst.2024.1388461
Received: 19 February 2024; Accepted: 27 March 2024;
Published: 03 May 2024.
Edited by:
Waseem Khalid, Government College University, PakistanReviewed by:
Muhammad Abdul Rahim, Times Institute Multan, PakistanHyrije Koraqi, University for Business and Technology (UBT), Albania
Copyright © 2024 Siddique, Ali, Arshad, Mubeen and Ghazala. This is an open-access article distributed under the terms of the Creative Commons Attribution License (CC BY). The use, distribution or reproduction in other forums is permitted, provided the original author(s) and the copyright owner(s) are credited and that the original publication in this journal is cited, in accordance with accepted academic practice. No use, distribution or reproduction is permitted which does not comply with these terms.
*Correspondence: Farzana Siddique, farzana.siddique@uos.edu.pk; Muhammad Arshad, arshad.sarwar@uos.edu.pk
†ORCID: Farzana Siddique, https://orcid.org/0000-0002-6610-2494; Zoha Ali, https://orcid.org/0009-0009-0495-6011; Muhammad Arshad, https://orcid.org/0000-0001-5501-6956; Kinza Mubeen, https://orcid.org/0009-0009-5173-0250; Afsheen Ghazala, https://.org/0009-0003-8803-7368