- 1Department of Agricultural and Environmental Sciences, Tennessee State University, Nashville, TN, United States
- 2Paul G. Allen School for Global Health, College of Veterinary Medicine, Washington State University, Pullman, WA, United States
- 3Department of Microbiology and Immunology, Virginia Commonwealth University School of Medicine, Richmond, VA, United States
- 4Tamarack Biotics LLC Pleasant Ave Fresno, Fresno, CA, United States
Introduction: Coxiella burnetii is a zoonotic Gram-negative obligate intracellular bacterial pathogen and the causative agent of query (Q) fever in humans. Contamination of milk by C. burnetii, as a consequence of livestock infection, is a significant public health concern. Effective methods to inactivate C. burnetii in milk are a critical aspect of food safety. Implementation of non-thermal UV-C processing technologies in the dairy industry can effectively preserve the sensory and nutritional quality of raw milk products while ensuring their safety, making them a viable alternative to traditional high-temperature short-time (HTST) pasteurization methods.
Methods: Optical light attenuation factors, such as the absorption, scattering, and reflection by skim milk (SM) were evaluated using a spectrophotometer. SM inoculated with an avirulent strain of C. burnetii was irradiated using a collimated beam device equipped with a low-pressure UV-C 254 nm lamp at doses from 0 to 12 mJ/cm2. Optical properties were considered for the evaluation of the delivered UV-C dose. The pasteurization treatment was conducted using a lab scale HTST pasteurizer (72°C/15 s). The verification studies were conducted using Escherichia coli ATCC 25922 inoculated in a phosphate buffer (transparent fluid) and humic acid (opaque fluid). Salmonella enterica serovar Muenchen ATCC BAA 1674 inoculated in SM was tested for its suitability as a surrogate for C. burnetii, a bacterium that requires specialized equipment and expertise for experimentation.
Results and Discussion: Absorption, reduced scattering coefficient, and the reflectance of SM at 254 nm were measured as 19 ± 0.3/cm, 26 ± 0.5/cm, and 10.6%, respectively. The UV-C results showed a log-linear inactivation of C. burnetii in SM with the UV-C sensitivity (D10) value of 4.1 ± 0.04 mJ/cm2. The results of HTST pasteurization revealed that C. burnetii was heat-sensitive with a D value of 1.75 min. Salmonella Muenchen showed similar UV inactivation kinetics and is, thereby, suggested as a suitable surrogate to C. burnetii for the pilot-scale UV-C processing studies of SM.
Introduction
Coxiella burnetii is a zoonotic Gram-negative obligate intracellular bacterial pathogen and the causative agent of query (Q) fever in humans (Eldin et al., 2017). Shedding of C. burnetii in milk and other secretions and excretions of infected cows, goats, and sheep is a significant concern for public health (Enright et al., 1957a; Shaw and Voth, 2019; Wittwer et al., 2022). The majority of human infections are asymptomatic or emerge as acute Q fever—a flu-like illness of differing severities, with symptoms which may include fever, chills, headache, fatigue, malaise, myalgia, arthralgia, and a cough (CFSPH, 2017). In some cases, C. burnetii can cause severe syndromes, including reproductive losses and pneumonia, which are also life-threatening in people with pre-existing conditions, including heart valve abnormalities (CFSPH, 2017). During the life cycle, C. burnetii transitions between a replicative large cell variant (LCV) and a non-replicative small-cell variant (SCV) that accumulates in the stationary phase (Coleman et al., 2004). The SCV has an unusual spore-like structure with highly condensed chromatin. C. burnetii is highly resistant to environmental factors, including heat, making it one of the significant and most resistive bacteria in milk (Codex Alimentarius, 2004; Roest et al., 2013).
Following World War II, there was an increase in the occurrence of Q-fever in European and North American people who were consuming raw milk and its products (Wegener, 1957; Marmion and Stoker, 1958). As a result, investigations into the thermal resistance of C. burnetii were initiated that led to pasteurization recommendations in 1957 by US researchers, which became the international standards (Enright et al., 1957a; Enright et al., 1957b; Codex Alimentarius Commission, 2004; Cerf and Condron, 2006). These studies indicated that the current thermal pasteurization conditions effectively reduced C. burnetii levels, even beyond the 5 Log10 reduction required by the Codex Alimentarius (Enright et al., 1957b; Codex Alimentarius Commission, 2004; Wittwer et al., 2022). However, the temperatures used in thermal pasteurization may significantly reduce the milk quality, including alterations in the sensorial and nutritional profile of the product, protein denaturation, and undesired changes to milk fat globules (Garcia-Amezquita et al., 2009; Cappozzo, Koutchma and Barnes, 2015; Gunter-ward et al., 2018). Furthermore, the high operational cost of high temperature short time (HTST) pasteurization is not feasible for small-scale dairy units. To preserve the benefits of raw dairy products, without compromising their safety, warrants the study of non-thermal technology for the processing of dairy products (Gunter-ward et al., 2018).
UV-C irradiation technology has been considered one of the most promising technologies for pathogen inactivation in milk and other beverages due to its low energy consumption, better or at least equal nutrient retention, and the fact that it does not generate any chemical by-products (Patras et al., 2021; Pendyala et al., 2022; Vashisht et al., 2022). In addition, the authors demonstrated that a uniform dose delivery will not alter the quality of the products. In a 2016 decision from the European Union commission, UV-treated (1045 J/L) milk was approved to be marketed with an extended shelf-life (EFSA Panel on Dietetic Products, Nutrition and Allergies, 2016). The antimicrobial properties of UV-C irradiation at 254 nm have been extensively studied against vegetative bacteria, bacterial spores, viruses, fungi, algae, and protozoa (Malayeri et al., 2016). Importantly, Little, Kishimoto, and Canonico (1980) studied the effect of UV irradiation on C. burnetii in suspensions. They reported the inactivation of C. burnetii (108 organisms per mL) at UV treatment conditions of 600 μW/cm2 for 15 s at a distance of 10 cm and penetration depth of 1 mm. In this study, however, the authors did not include measurements of the optical attenuation coefficients of their test fluid, nor did they report the average dose used. Moreover, since the ability to directly enumerate viable C. burnetii via a colony-forming unit (CFU) assay has only been recently made possible (Sanchez et al., 2018), the authors could only indirectly measure the effect of UV treatment on C. burnetii through detection of serum antibodies. Bolton and Linden (2003) reported a standard method for estimating UV-C sensitivity in absorbing fluids. However, in addition to absorbance, some fluids (e.g., milk) can scatter UV-C photons, which needs to be considered to calculate the average delivered dose/fluence and, thereby, UV-C sensitivity. Therefore, the objectives for this study were to 1) develop a method for dose measurement and estimate microbial UV-C sensitivity in skim milk (SM); 2) evaluate the UV-C sensitivity of C. burnetii in SM; 3) conduct a comparative HTST pasteurization study; and 4) identify a bacterial surrogate with similar UV-C sensitivity to C. burnetii for further continuous UV-C system validation on pilot scale.
Materials and methods
Bacterial culture conditions and enumeration
A chloramphenicol-resistant strain of the avirulent C. burnetii Nine Mile II isolate (NMII; RSA439; clone 4) was propagated in acidified citrate cysteine medium-2 (ACCM-2), as described previously (Omsland et al., 2011; Sanchez, Vallejo-Esquerra and Omsland, 2018). C. burnetii was stored at −80°C in ACCM-2 supplemented with 10% DMSO. For the enumeration of microbial counts, the samples were diluted serially in ACCM-2 inorganic salts before plating on solid (final 0.25% w/vol. agarose) ACCM-2 supplemented with 500 μM tryptophan and 1.5 μg/mL chloramphenicol. The plates were incubated for 9–10 days in a tri-gas incubator at 37°C with 5% CO2 and 5% O2 (Sanchez, Vallejo-Esquerra and Omsland, 2018). To correlate the number of viable bacteria as measured by the plate count method to the total number of bacteria used for inoculation, C. burnetii genome equivalents (GEs) were quantified via the detection of the C. burnetii gene CBU1206 using a CFX96 real-time PCR detection system (Bio-Rad Laboratories, Hercules, CA) (Beare et al., 2012; Sanchez, Vallejo-Esquerra and Omsland, 2018). C. burnetii GEs were extrapolated from a standard curve prepared using recombinant CBU1206. A non-pathogenic strain of Escherichia coli ATCC 25922 and Salmonella enterica serovar Muenchen ATCC BAA 1674 were obtained from the American Type Culture Collection (ATCC) and propagated in Tryptic Soy Broth (TSB) and harvested as reported earlier (Pendyala et al., 2021; Vashisht et al., 2021). To enumerate the microbial population, appropriate dilutions in peptone water (in 0.1% PW) were plated in duplicate onto Tryptic Soy Agar (Oxoid Ltd., Basingstoke, United Kingdom) plates and incubated for 24 h at 37°C. UV-C sensitivity of C. burnetii was hypothesized to be in the range similar to Escherichia coli and Salmonella (Gopisetty et al., 2019; Vashisht et al., 2021).
Preparation of test fluid microbial suspensions
Ultra-high-temperature (UHT) processed SM from Parmalat, Canada; humic acid (adjusted pH to 7.0) from Agricultural Services of America, Inc., Florida, United States; and phosphate buffer saline (PBS, pH 7.0) were used as test fluids. To remove ACCM-2 and DMSO from the test samples, C. burnetii stocks were washed twice with phosphate-buffered saline (Becton Dickinson, New Jersey, United States) using centrifugation at 3000 g for 15 min. The test samples were prepared by spiking SM with microorganisms (C. burnetii, E. coli, or S. Muenchen) at a concentration of >108 CFU/mL for testing by UV-C irradiation or HTST pasteurization. Each strain was separately inoculated in a sample and treated independently.
Measurement of optical properties
The optical properties of SM inoculated with test micro-organism were measured using a double beam Cary 100 spectrophotometer (Varian, United States) equipped with a 6-inch single integrating sphere (Labsphere, DRA-CA-30, United States) to calculate scattered light at a 254 nm wavelength (Shenoy and Pal, 2008). Thin quartz cuvettes (0.08 mm path length) were prepared and used to measure total transmittance and total reflectance values. The transmittance and reflectance (diffuse reflectance) of light were collected by the integrating sphere when the sample was placed at the entrance and exit ports, respectively (Gunter-ward et al., 2018). The amount of light transmitted and reflected by the quartz cuvettes was also quantified and considered to estimate the absorption, scattering coefficients, and reflectance. The refractive index (RI) of SM was measured at 20°C, using a digital refractometer from Schmidt + Haensch GmbH & Co., at nine distinct wavelengths ranging from ultraviolet to the visible range, i.e., 365 nm–706 nm. For each measurement, 0.3 mL of SM was placed in the measurement compartment. Refractive index data were compared with Milli-Q water (control). A fifth-order polynomial fit was then used to calculate the refractive index at 254 nm. A higher-order polynomial model was selected as it fitted the experimental data well with a R2 value of 0.99. For a SM sample, a control was run to check the system calibration. The refractive index of Milli-Q water was found to be 1.376, which is in excellent agreement with the scientific literature, indicating that the system was well-calibrated (Schiebener et al., 1990). The accuracy of the measurements is within 0.002% at each wavelength (data not shown). Data were reported as means ± standard deviation. All measurements were carried out in duplicates (technical replicates) with three biological replicates. The inverse adding doubling (IAD) program (GitHub, CA, United States, 94107) was used to quantify absorption and reduced scattering coefficients by applying total transmittance, reflectance, and the refractive index as input values (Prahl, 1999). IAD is a command-line program which uses the inverse adding doubling method and includes the Fresnel reflection at the surface and corrections along with integrating sphere experiments. The inverse adding-doubling method is an accurate solution of the radiative transport equation for all albedos, all optical depths, and all phase functions, this technique can be applied to any fluid medium for which the radiative transport equation is valid. This method is applicable to homogeneous turbid slabs with any optical thickness, albedo, or phase function. The optical properties are obtained by iterating an adding-doubling solution of the radiative transport equation (Eq 2) until the calculated values of the reflection and transmission match the measured ones. From the obtained optical properties, Ultraviolet transmittance (UVT, %/cm), which indicates the fraction of the incident light transmitted through a fluid over a 1 cm path-length, was calculated as per Eq 1.
where A represents the absorbance (base10) of the test fluid at 254 nm for a 1-cm path.
UV system, dose calculation, and test fluid treatment
The collimated beam apparatus (Pendyala et al., 2019) was used to irradiate test fluids (Figure 1). The system design followed the recommendations of Bolton and Linden (2003) and utilized a low-pressure mercury vapor arc lamp, primarily emitting at 253.7 nm (positioned at 18.05 cm from the surface). Irradiance at the position of the fluid surface was measured using a calibrated radiometer ILT1700 with SED240 detectors, each equipped with a quartz W diffuser and an NS254 spectral filter to ensure that only 254 nm radiation was measured (International Light Technologies, Peabody, MA, United States). The delivered UV-C dose (fluence) was calculated as the product of the volume average of the fluence rate in the sample and the exposure time by assuming a perfect mixing of the sample by the stir bar (12.7 x 3.2 mm). A measure of 2 mL of the microbial suspension (tested in SM, humic acid, or PBS) was treated in 10-mL beakers (optical path length of 6 mm) at UV dose ranges from 0 to 17 mJ/cm2 (n = 3). Since SM scatters UV-C light, the scattering factor (
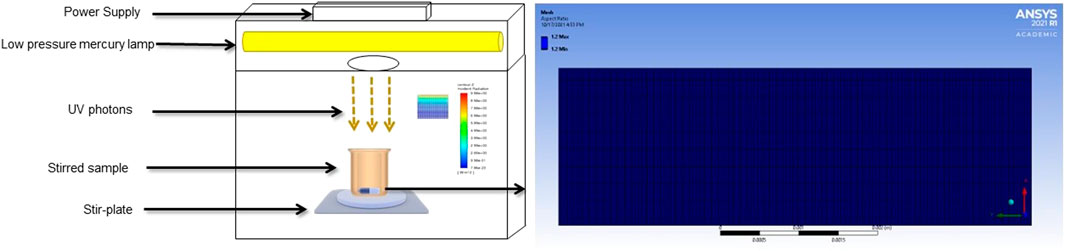
FIGURE 1. Collimated beam system and computational fluid dynamics analysis (radiation profile) conducted using the Fluent program.
where
Then, the average UV fluence rate (
CFD simulation was carried out with the commercial code Ansys 2021 R1. Fluent software was used for drawing geometry (2D) and generating the mesh, while Ansys Fluent v12.1 (Ansys Inc., Canonsburg, PA, United States) was used for solving the radiation fields. The mesh resolution nodes and elements were 3643 and 36000, respectively. Figure 1 shows the schematic representation of the geometry of the system adopted for the numerical simulations. This technique used the discrete ordinates irradiation model; the fluid (2 mL) in a 10-mL beaker was exposed to UV incident radiation coming from a near collimated beam apparatus. In the near collimated beam apparatus, the local disinfection depends on the local fluence rate and kinetic rate constants. Modeling the collimated beam photo-reactor, therefore, involves two sub-models, the intensity of the radiation field and UV reaction kinetics.
Thermal challenge studies
The thermal challenge studies were conducted using a laboratory-scale HTST pasteurizer (Kontopodi et al., 2022). The pasteurizer consists of three main sections (as shown in Figure 2), a pre-heating section (77°C), a holding section (72°C for 15 s), and a cooling unit. The temperature was monitored using a probe. SM inoculated with C. burnetii was directly fed into the system using a peristaltic pump (Watson–Marlow). The pump was calibrated before the thermal challenge studies. The clean-in-place (CIP) procedure was conducted before any thermal challenge studies. The cleanliness and sterility of the HTST system were ensured by performing a CIP procedure before and after use. This CIP consisted of flushing the system with sterile water, followed by 0.1 N HCl, sterile water again, 0.1 N NaOH, and a final rinse with sterile water. Flushing was performed at 180 mL/min. SM was then pumped through the heating time, where it achieved the pasteurization temperature of 72°C at a flow rate of 90 mL/min at a 15-s holding time. At the end of the holding section, SM passed through a cooling coil. In this phase, SM was cooled to a final temperature of 4°C ± 1. A 10 mL of sample was then collected for enumeration and plating.
Data analysis and statistics
To assess the inactivation of E. coli or C. burnetii, the log-linear model available in the GInaFiT tool (a freeware add-in for Microsoft Excel) (Geeraerd, Valdramidis, and Van Impe, 2005) was used to fit the experimental data, and the goodness-of-fit parameters, including R2, root-mean-square error, and rate constants, were evaluated. Inactivation kinetics is expressed as follows:
where
A balanced design with four replicates randomized in the experimental order was performed for each treatment. Data were reported as means ± one standard deviation from the mean and significance level set to 0.05 (5%).
Results and discussion
Optical properties and average fluence rate estimation
Data on optical properties indicate that SM strongly absorbs and scatters UV-C light (Table 1). From the measured optical data, it is apparent that UV-C light has minimal transmission through SM due to presence of aromatic amino acids and other UV-C absorbing organic solutes (Gunter-ward et al., 2018). Light scattering by casein micelles causes skim milk to appear turbid and opaque. The average fluence rate or incident irradiance through the test fluid suspension was calculated by substituting the experimental Petri factor, reflection factor, water factor, divergence factor, and the scattering factor in Eq.3.
Verification of the scattering factor and the UV dose distribution in SM
To verify the estimated scattering factor from CFD, comparative microbial (E. coli) inactivation studies in SM and humic acid with the same UV-C absorbance as SM without scattering were conducted. Humic acid is a stronger absorber of UV light and has been used as a surrogate fluid (Pendyala et al., 2019). The data show that there is no significant difference in the microbial inactivation kinetics in both SM and diluted humic acid (Figure 3) and confirms the accuracy of the calculated scattering factor. The dose distribution throughout the fluid domain is a crucial parameter to estimate the UV-C sensitivity of microorganisms. The efficient dose distribution conditions, provided to all microbial particles, result in the log-linear inactivation kinetics in mono-microbial populations. To check the UV dose distribution in SM under standard experimental stir bar mixing conditions, a comparative study with a high UV-C transparent fluid phosphate-buffered saline (PBS) was conducted at the same experimental UV-C doses. The experimental data show that there is no significant difference (p >0.05) between the microbial inactivation kinetics with D10 values ranging from 3.20 to 3.32 mJ/cm2 and the shown log-linear inactivation kinetics in the three different test fluids (Table 2). Therefore, these results indicated that the experimental mixing conditions distributed the UV-C dose efficiently in opaque test fluids.
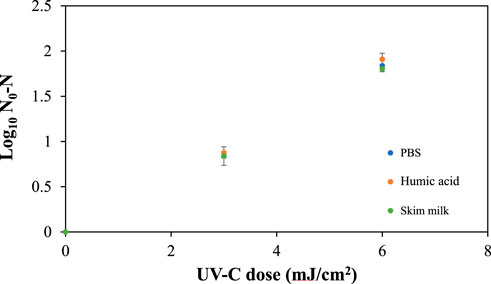
FIGURE 3. Verification of collimated beam dose delivery using E. coli in skim milk, PBS, and humic acid. Triplicate irradiations were performed for each dose; all replicates shown on plot, and values shown are averages of duplicate plating of each irradiated sample. Error bars represent range of data.
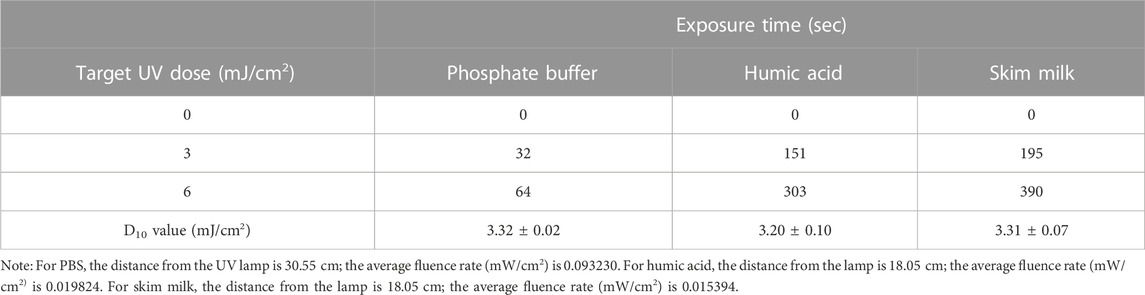
TABLE 2. Exposure times to achieve the target UV dose for the inactivation of E. coli for UV dose validation.
UV inactivation of C. burnetii and the identification of a bacterial surrogate for validation studies
SM inoculated with C. burnetii at approximately 108 CFU/mL was exposed to known UV-C doses of 0, 3, 6, and 12 mJ/cm2 in a stirred collimated beam UV system. The data revealed a >3 log10 reduction of C. burnetii at a maximum dose of 12 mJ/cm2 (Figure 4). The inactivation kinetics of C. burnetii were fitted into a log-linear model with a low (0.35) root-mean-square error (RMSE) and a higher (0.92) R2 value (Table 3). The D10 value and kinetic constant (kmax) of C. burnetii in SM were estimated as 4.1 ± 0.04 mJ/cm2 and 0.56 cm2/mJ, respectively (Table 3). Little, Kishimoto, and Canonico (1980) demonstrated the inactivation of C. burnetii in suspension and within guinea pig peritoneal macrophages by UV-C irradiation. The authors reported that C. burnetii was inactivated at a UV irradiance of 600 μW/cm2 for 15 s at a distance of 10 cm in the suspension and macrophages. According to our presented data on the D10 value of C. burnetii, the performance criterion of 5 log10 reductions, as demanded by the Codex Alimentarius, can be achieved at a UV-C dose of 20.5 mJ/cm2.
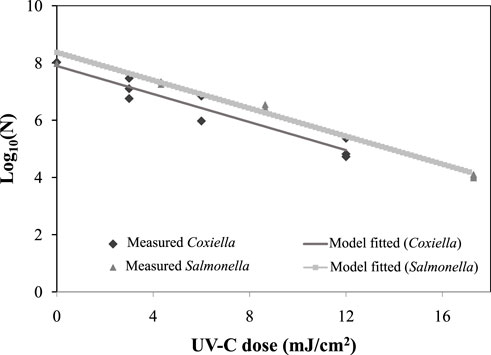
FIGURE 4. UV-C Inactivation of C. burnetii and Salmonella enterica serovar Muenchen in SM Triplicate irradiations were performed for each dose; all replicates shown on plot, and values shown are averages of duplicate plating of each irradiated sample.
While the inactivation of C. burnetii is a key consideration for milk processing, the cultivation of C. burnetii requires specialized equipment and expertise. Therefore, to facilitate the validation of pilot-scale UV-C systems for the inactivation of bacterial pathogens in milk, we sought to identify a bacterial surrogate for C. burnetii. Based on the D10 value of C. burnetii (4.1 ± 0.04 mJ/cm2), the Salmonella strain Muenchen ATCC BAA 1674 reported with D10 values ranging from 3.9 to 4.3 mJ/cm2 in the phosphate-buffered saline (Gopisetty et al., 2019; Pendyala et al., 2021; Vashisht et al., 2021) was selected for UV-C inactivation studies with these organisms in SM (Figure 4). The results show log linear inactivation kinetics with RMSE 0.1913 and R2 >0.98. Interestingly, the data show Kmax (0.56) and D10 values (4.1 mJ/cm2) similar to C. burnetii, indicative of their suitability as surrogates for C. burnetii for UV-C inactivation studies in SM. It should be noted that this experiment was designed to evaluate the UV-C sensitivity of C. burnetii using a collimated beam system. Considering the UV-C light distribution in SM, the attenuation of UV-C intensity with the fluid depth, the collimated beam system will not adequately achieve a 99.999% reduction in C. burnetii. In contrast, the system can be used to quantify the D10 value (dose required for 1 log reduction of test micro-organism. Further studies will be conducted using a UV-C continuous flow system to achieve atleast 8 log10 reduction of C. burnetii in milk. For UV-C inactivation studies, Salmonella can be a suitable surrogate for C. burnetii which does not need specialized equipment. Salmonella can be easily propagated in TSB, enumerated, and subsequently plated on TSA plates. These plates are typically incubated at 37°C for a duration of 18–24 h. Based on these characteristics, Salmonella can be an ideal surrogate, particularly when validating UV systems at commercial flow-rates. In our forthcoming field testing, we plan to utilize Salmonella as a surrogate for C. burnetii.
Comparative HTST pasteurization using SM inoculated with C. burnetii
The physical properties of SM and heat exchanger parameters are shown in Table 4. Starting with a C. burnetii population of 3.81 x 108 ± 0.21 CFU/mL (5.38 x 108 ± 0.31 GE/mL), the heat-dependent log10 reduction during a holding time of 15 s at 72°C resulted in 8 log10 reduction of C. burnetii in SM, with a D-value of 1.75 s. It was lower as compared to the value reported in the studies of Enright et al., 1957a and Enright et al., 1957b, where the D value was 1.88 s at 72°C. A recent study by Wittwer et al. (2022) on Coxiella isolates M, WDK299, and WDK1188 indicated that, in general, all isolates were more susceptible to heat over a temperature ranging from 60°C to 65°C with holding times from 15 to 25 s. For the highest temperature of 65°C, the D-value was reported as 5.1–7.6 s, with a predicted reduction of approximately 10.5 log10 at 72.4°C for 15 s.
Conclusion
In this study, we developed methods to determine the optical attenuation properties of a highly scattering fluid (SM) and demonstrated the UV-C sensitivity of an avirulent strain of C. burnetii in SM. Our experiments indicated a D10 value of 4.1 ± 0.04 mJ/cm2 for C. burnetii in SM and predicted a UV-C dose of 20.5 mJ/cm2 for a 5 log10 reduction (linear part of model), meeting the requirement set by the Codex Alimentarius. This validation test should be conducted in a flow through UV system, and collimated systems should not be used for verification. These findings are crucial for the development of non-thermal UV-C pasteurization systems for the processing of SM as they provide valuable insights into UV-C sensitivity and the required doses to effectively reduce C. burnetii ontamination. Additionally, we identified the Salmonella strain Muenchen ATCC BAA 1674 as a suitable surrogate for UV-C treatment validation studies, which enables further investigation and the validation of UV-C pasteurization methods. The results showed that the D10 value of Salmonella was 4.1 mJ/cm2, which was similar to C. burnetii, reflecting its suitability as a surrogate. In comparison, the HTST pasteurization study showed >8 log10 reduction of C. burnetii in SM. The presented data are critical for the development of non-thermal UV-C pasteurization systems for the processing of milk. Immediate future studies need to be focused on the evaluation of the quality parameters of UV-C processed milk so that an optimal dose for microbial inactivation with the minimal effect on these parameters can be reported.
Data availability statement
The raw data supporting the conclusion of this article will be made available by the authors, without undue reservation.
Author contributions
BP: conceptualization, methodology, investigation, visualization, and writing–original draft; PV: methodology, writing–original draft, investigation, and visualization; F-CC: resources and investigation; SS: methodology, investigation, and visualization; BC: conceptualization and funding resources; AO: methodology, supervision, and original–draft review; AP: conceptualization, methodology, supervision, and original–draft review. All authors contributed to the article and approved the submitted version.
Acknowledgments
The authors thank Drs. Bob Heinzen and Paul Beare, Rocky Mountain Laboratories, NIAID, NIH, for sharing the chloramphenicol-resistant mutant of C. burnetii used in this project.
Conflict of interest
Author BC was employed by Tamarack Biotics LLC, Pleasant Ave, Fresno.
The remaining authors declare that the research was conducted in the absence of any commercial or financial relationships that could be construed as a potential conflict of interest.
The authors AP and AO declared that they were an editorial board member of Frontiers at the time of submission. This had no impact on the peer review process and the final decision.
Publisher’s note
All claims expressed in this article are solely those of the authors and do not necessarily represent those of their affiliated organizations, or those of the publisher, the editors, and the reviewers. Any product that may be evaluated in this article, or claim that may be made by its manufacturer, is not guaranteed or endorsed by the publisher.
References
Beare, P. A., Larson, C. L., Gilk, S. D., and Heinzen, R. A. (2012). Two systems for targeted gene deletion in Coxiella burnetii. Appl. Environ. Microbiol. 78 (13), 4580–4589. doi:10.1128/AEM.00881-12
Bolton, J. R., and Linden, K. G. (2003). Standardization of methods for fluence (UV dose) determination in bench-scale UV experiments. J. Environ. Eng. 129 (3), 209–215. doi:10.1061/(asce)0733-9372(2003)129:3(209)
Cappozzo, J. C., Koutchma, T., and Barnes, G. (2015). Chemical characterization of milk after treatment with thermal (HTST and UHT) and nonthermal (turbulent flow ultraviolet) processing technologies. J. Dairy Sci. 98 (8), 5068–5079. doi:10.3168/jds.2014-9190
Cerf, O., and Condron, R. (2006). Coxiella burnetii and milk pasteurization: an early application of the precautionary principle? Epidemiol. Infect. 134 (5), 946–951. doi:10.1017/S0950268806005978
Codex Alimentarius Commission (2004). Draft code of hygienic practice for milk and milk products at step 8, Report of the thirty-sixth session of the codex committee on food hygiene. Rome: Codex Alimentarius Commission Twenty-seventh Session, 44–82.
Coleman, S. A., Fischer, E. R., Howe, D., Mead, D. J., and Heinzen, R. A. (2004). Temporal analysis of Coxiella burnetii morphological differentiation. J. Bacteriol. 186 (21), 7344–7352. doi:10.1128/jb.186.21.7344-7352.2004
EFSA Panel on Dietetic Products,Nutrition and Allergies (NDA) (2016). Safety of UV-treated milk as a novel food pursuant to Regulation (EC) No 258/97. EFSA J. 14 (1), 4370. doi:10.2903/j.efsa.2016.4370
Eldin, C., Mélenotte, C., Mediannikov, O., Ghigo, E., Million, M., Edouard, S., et al. (2017). From Q fever to coxiella burnetii infection: A paradigm change. Clin. Microbiol. Rev. 30 (1), 115–190. doi:10.1128/cmr.00045-16
Enright, J. B., Sadler, W. W., and Thomas, R. C. (1957a). Pasteurization of milk containing the organism of Q fever. Am. J. public health nations health 47 (6), 695–700. doi:10.2105/ajph.47.6.695
Enright, J. B., Sadler, W. W., and Thomas, R. C. (1957b). Thermal inactivation of Coxiella burnetii and its relation to pasteurization of milk (No. 517). Washington, DC: US Department of Health, Education, and Welfare, Public Health Service.
Garcia-Amezquita, L. E., Primo-Mora, A. R., Barbosa-Cánovas, G. V., and Sepulveda, D. R. (2009). Effect of nonthermal technologies on the native size distribution of fat globules in bovine cheese-making milk. Innovative Food Sci. Emerg. Technol. 10 (4), 491–494. doi:10.1016/j.ifset.2009.03.002
Geeraerd, A. H., Valdramidis, V. P., and Van Impe, J. F. (2005). GInaFiT, a freeware tool to assess non-log-linear microbial survivor curves. Int. J. food Microbiol. 102 (1), 95–105. doi:10.1016/j.ijfoodmicro.2004.11.038
Gopisetty, V. V. S., Patras, A., Pendyala, B., Kilonzo-Nthenge, A., Ravi, R., Pokharel, B., et al. (2019). UV-C irradiation as an alternative treatment technique: study of its effect on microbial inactivation, cytotoxicity, and sensory properties in cranberry-flavored water. Innovative food Sci. Emerg. Technol. 52, 66–74. doi:10.1016/j.ifset.2018.11.002
Gunter-Ward, D. M., Patras, A., Bhullar, M. S., Kilonzo-Nthenge, A., Pokharel, B., and Sasges, M. (2018). Efficacy of ultraviolet (UV-C) light in reducing foodborne pathogens and model viruses in skim milk. J. Food Process. Preserv. 42 (2), e13485. doi:10.1111/jfpp.13485
Kontopodi, E., Boeren, S., Stahl, B., van Goudoever, J. B., van Elburg, R. M., and Hettinga, K. (2022). High-temperature short-time preserves human milk's bioactive proteins and their function better than pasteurization techniques with long processing times. Front. Pediatr. 9, 798609. doi:10.3389/fped.2021.798609
Little, J. S., Kishimoto, R. A., and Canonico, P. G. (1980). In vitro studies of interaction of rickettsia and macrophages: effect of ultraviolet light on coxiella burnetii inactivation and macrophage enzymes. Infect. Immun. 27 (3), 837–841. doi:10.1128/iai.27.3.837-841.1980
Malayeri, A. H., Mohseni, M., Cairns, B., Bolton, J. R., Chevrefils, G., Caron, E., et al. (2016). Fluence (UV dose) required to achieve incremental log inactivation of bacteria, protozoa, viruses and algae. IUVA News 18 (3), 4–6.
Marmion, B. P., and Stoker, M. G. P. (1958). The epidemiology of Q fever in Great Britain; an analysis of the findings and some conclusions. Br. Med. J. 2 (5100), 809–816. doi:10.1136/bmj.2.5100.809
Omsland, A., Beare, P. A., Hill, J., Cockrell, D. C., Howe, D., Hansen, B., et al. (2011). Isolation from animal tissue and genetic transformation of Coxiella burnetii are facilitated by an improved axenic growth medium. Appl. Environ. Microbiol. 77 (11), 3720–3725. doi:10.1128/AEM.02826-10
Patras, A., Bhullar, M. S., Pendyala, B., and Crapulli, F. (2021). Ultraviolet treatment of opaque liquid foods: from theory to practice. Therapeutics. B978-0-08-100596-5.22941-7. ISBN: 9780128157817.
Pendyala, B., Patras, A., Gopisetty, V. V. S., Sasges, M., and Balamurugan, S. (2019). Inactivation of Bacillus and Clostridium spores in coconut water by ultraviolet light. Foodborne pathogens Dis. 16 (10), 704–711. doi:10.1089/fpd.2019.2623
Pendyala, B., Patras, A., Gopisetty, V. V. S., and Sasges, M. (2021). UV-C inactivation of microorganisms in a highly opaque model fluid using a pilot scale ultra-thin film annular reactor: validation of delivered dose. J. Food Eng. 294, 110403. doi:10.1016/j.jfoodeng.2020.110403
Pendyala, B., Patras, A., Gopisetty, V. V. S., Vashisht, P., and Ravi, R. (2022). Inactivation of B. Cereus spores in whole milk and almond milk by serpentine path coiled tube UV-C system: numerical simulation of flow field, lipid peroxidation and volatiles analysis. Food Res. Int. 160, 111652. doi:10.1016/j.foodres.2022.111652
Prahl, S. (1999). Optical property measurements using the inverse adding-doubling program, 9205. St. Vincent Hospital: Oregon Medical Laser Center, 1–53.
Roest, H. I., Bossers, A., van Zijderveld, F. G., and Rebel, J. M. (2013). Clinical microbiology of Coxiella burnetii and relevant aspects for the diagnosis and control of the zoonotic disease Q fever. Vet. Q. 33 (3), 148–160. doi:10.1080/01652176.2013.843809
Sanchez, S. E., Vallejo-Esquerra, E., and Omsland, A. (2018). Use of axenic culture tools to study Coxiella burnetii. Curr. Protoc. Microbiol. 50 (1), e52. doi:10.1002/cpmc.52
Schiebener, P., Straub, J., Levelt Sengers, J. M. H., and Gallagher, J. S. (1990). Refractive index of water and steam as function of wavelength, temperature and density. J. Phys. Chem. reference data 19 (3), 677–717. doi:10.1063/1.555859
Shaw, E. I., and Voth, D. E. (2019). Coxiella burnetii: A pathogenic intracellular acidophile. Microbiology 165 (1), 1–3. doi:10.1099/mic.0.000707
Shenoy, M. R., and Pal, B. P. (2008). Method to determine the optical properties of turbid media. Appl. Opt. 47 (17), 3216–3220. doi:10.1364/AO.47.003216
The Center for Food Security and Public Health (CFSPH) (2017). Q fever [fact sheet]. Available at: https://www.cfsph.iastate.edu/Factsheets/pdfs/q_fever.pdf.
Vashisht, P., Pendyala, B., Gopisetty, V. V. S., and Patras, A. (2021). Modeling and validation of delivered fluence of a continuous dean flow pilot scale UV system: monitoring fluence by biodosimetry approach. Food Res. Int. 148, 110625. doi:10.1016/j.foodres.2021.110625
Vashisht, P., Pendyala, B., Patras, A., Gopisetty, V. V. S., and Ravi, R. (2022). Design and efficiency evaluation of a mid-size serpentine Dean flow UV-C system for the processing of whole milk using computational fluid dynamics and biodosimetry. J. Food Eng. 335, 111168. doi:10.1016/j.jfoodeng.2022.111168
Wegener, K. H., and Fever, Q. (1957). Its signification regarding milk hygiene. Bibliographic study with contribution to the question of its occurrence in cattle herds in Schleswig-Holstein. Kiel. Milchwirtsch. Forschungsberichte 9, 509–535.
Keywords: high-temperature short-time pasteurization, Coxiella burnetii, skim milk, UV-C sensitivity, collimated beam apparatus
Citation: Pendyala B, Vashisht P, Chen F-C, Sanchez SE, Comstock B, Omsland A and Patras A (2023) Evaluating the UV-C sensitivity of Coxiella burnetii in skim milk using a bench-scale collimated beam system and comparative thermal sensitivity study by high-temperature short-time pasteurization. Front. Food. Sci. Technol. 3:1251069. doi: 10.3389/frfst.2023.1251069
Received: 30 June 2023; Accepted: 26 September 2023;
Published: 23 November 2023.
Edited by:
Vermont Punongbayan Dia, The University of Tennessee, Knoxville, United StatesReviewed by:
Joshua B. Gurtler, Agricultural Research Service (USDA), United StatesSandra Guerrero, University of Buenos Aires, Argentina
Copyright © 2023 Pendyala, Vashisht, Chen, Sanchez, Comstock, Omsland and Patras. This is an open-access article distributed under the terms of the Creative Commons Attribution License (CC BY). The use, distribution or reproduction in other forums is permitted, provided the original author(s) and the copyright owner(s) are credited and that the original publication in this journal is cited, in accordance with accepted academic practice. No use, distribution or reproduction is permitted which does not comply with these terms.
*Correspondence: Ankit Patras, YXBhdHJhc0B0bnN0YXRlLmVkdQ==