- 1Department of Food Science and Nutrition, University of Ghana, Accra, Ghana
- 2Department of Food Process Engineering, University of Ghana, Accra, Ghana
- 3Department of Food Science and Nutrition, University of Minnesota, St. Paul, MN, United States
Tigernuts and millet are indigenous underutilized crops in West Africa that have versatile applications in food processing. These crops are rich in fermentable carbohydrates, resistant starch, fiber, and micronutrients, making them ideal candidates for pre- and probiotic (synbiotic) foods. This study utilized whole tigernuts in a dairy–millet-based fermented beverage called brukina, turned it to a synbiotic, and assessed the functional and physicochemical profiles, microbial quality, and shelf stability of the beverage. The tigernut–millet agglomerate was prepared by incorporating cellulose-hydrolyzed tigernut fibrous (TNF) cake and non-hydrolyzed TNF (10% and 15%, respectively) into millet and allowing to ferment for 12 and 24 h. Brukina produced from composite tigernut milk: dairy in a ratio of 40%:60% was inoculated with the probiotic Lacticaseibacillus casei after pasteurization. The beverage was analyzed for physicochemical, proximate, and functional properties and microbiological stability at 5°C and 25°C. The obtained data were subjected to analysis of variance (ANOVA) in Minitab version 17 using a general linear model to determine the variability, interactions, and significance of the measured product characteristics. The agglomerate water absorption capacity (l/g) ranged from 0.70 ± 0.17 to 0.89 ± 0.17, bulk density (g/l) from 0.55 ± 0.04 to 0.63 ± 0.00, and swell index (%) from 1.62 ± 0.08 to 1.80 ± 0.06. The agglomerate prepared from dough and fermented for 12 h had excellent functional characteristics and was selected for synbiotic brukina production. Moisture content of the product decreased (p < 0.001) with tigernut incorporation ranging from 78.85% to 70.45%, while sodium, phosphorus, protein, total carbohydrate, and crude fiber increased with tigernut incorporation (p < 0.05). Synbiotic brukina supported the growth of L. casei attaining 11 log CFU/mL with a corresponding increase in lactic acid production and was microbiologically safe at 5°C and 25°C for 5 days compared to unpasteurized and uninoculated probiotic control (p < 0.05). The addition of whole tigernuts and L. casei to brukina enhanced its nutritional content with a shelf stability of 3 days.
Introduction
Cyperus esculentus L. (tigernut) is a tropical crop grown in Southern Europe, Asia, and Africa. In Ghana, tigernut remains classified as a minor crop, which is not captured in official records (SRID Agriculture in Ghana and Facts and Figures, 2010). Enhancing the usability of indigenous plant products like tigernut and millet in a developing country aligns with SDG 2 (Zero Hunger) and SDG 12 (Responsible Consumption and Production), and it can play a significant role in curbing the problem of food and nutritional insecurity. Sánchez-Zapata et al. (2012) explored the use of tigernuts as ingredients in existing foods. The authors incorporated tigernut cake (the fibrous remains after juicing tigernut) into sausage and used the tigernut milk as a carbon source for the development of probiotic products. Tigernut has high nutritional value, and its tubers contain pleasantly flavored oil, much like olive and sweet almond oil (Kim et al., 2007; Yu et al., 2022). Tigernut has long been recognized for its nutritional and health-related benefits. It contains approximately 50% digestible carbohydrates, 10% protein, and 9% dietary fiber, which consists mainly cellulose and lignin (Arafat et al., 2009; Munekata et al., 2021). It has a good micronutrient profile including zinc, magnesium, potassium, calcium, and vitamins C and E (Arafat et al., 2009; Munekata et al., 2021). Tigernut is consumed mainly for its perceived aphrodisiac properties and nourishment as a ready-to-eat (RTE) snack (Asante et al., 2014). Tigernut is highly fibrous, making it a suitable composite material for the production of yogurt, and its yogurt quality is comparable to that of dairy yogurt (Sanful, 2009). Fermentation of dietary fibers in the colon generates short-chain fatty acids such as propionate, which has been shown to inhibit the activity of hydroxy-3-methylglutarylCoA reductase, the limiting enzyme for cholesterol synthesis (Xu et al., 2020). When dietary fiber bonds with bile acids, it prevents reabsorption in the liver, therefore inhibiting cholesterol synthesis (Xu et al., 2020).
Millet is one of the underutilized grains in Africa. In Ghana, it is a staple for the Northern locales (Adebiyi et al., 2016). It is rich in protein, vitamins, essential minerals, and fiber content, which makes it a good candidate for products such as infant food, snacks, and dietary enhancements. Moreover, millet cuisines have progressed toward becoming a part of daily meals in homes and restaurants (Xu et al., 2020). Both millet and tigernut fibers are readily fermentable by colonic microorganisms, such as bifidobacteria and lactobacilli, as carbon sources, making them a decent source of prebiotic. Millet is a key ingredient in the popular fermented dairy beverage known as brukina in Ghana but consumed in various parts of West Africa (Adebiyi et al., 2016). Brukina is a nutritious snack, widely consumed and thus a good candidate for product development with enhanced nutrition and safety.
Different authors have explored the incorporation of tigernuts into existing food products. Jimoh and Kole (2022) developed a tigernut fruit blend and studied its nutritional profile, sensory properties, and shelf life. Sanful (2009) also developed yoghurt from composite dairy and tigernut milk. All these works prove the feasibility of tigernut in developing nutritious affordable food products. However, none of these authors developed a synbiotic product that had incorporated tigernut fiber and a suitable probiotic to further enhance the nutritional and functional properties of developed food products. Although tigernuts have desirable properties to use in food formulations, its fiber is difficult to swallow, thus limiting its use in foods. Furthermore, the tigernut milk extract is rich in starch, which causes gelation when heated above 60°C (da Costa Neto et al., 2019). This property limits its functional use in foods like dairy products requiring pasteurization as a legal requirement.
The incorporation of tigernut milk in food requires further research to improve the physicochemical and textural properties of such products. The study presents novel ways of making use of underutilized and affordable whole crops to enhance functional properties, nutritional and microbiological profiles, and stability of brukina beverage.
Materials and methods
A brown variety of tigernut tubers (Cyperus esculentus L.) (50 kg) was obtained from Twifo Praso in the Central Region of Ghana. Pearl millet and dairy milk powder were also obtained from a local market in Accra. Tigernut and millet were manually sorted to remove the broken pieces and any other physical contaminants before storage at ambient temperature. The probiotic bacteria (Lacticaseibacillus casei) strain isolated from human saliva was obtained from the microbiology research laboratory of the Department of Nutrition and Food Science, University of Ghana. The cultures were stored at −80°C in de Man Rogosa Sharpe (MRS) broth (Sigma-Aldrich Co. Ltd., Dorset, UK) containing 10% (v/v) of glycerol.
Experimental design
Using Minitab 17, the study employed a mixture design with extreme vertices and two process variables: enzyme treatment of tigernut fibrous cake (TNF) and fermentation time (12 and 24 h). The objective of the study was to investigate the suitability of tigernuts and millet as prebiotic sources in probiotic-based products. The major components of the product formulation were tigernut fibrous cake and wet-milled millet flour. A total of 20 formulations (Ocloo et al., 2014) were generated for the agglomerate formulation process. To ensure the nutritional quality of the product, the fiber content was considered a constraint. The study used the fiber nutritional requirement for processed food products, setting a range of 1.5–3 g of fiber per 100 kcal of processed food (Ioniță-Mîndrican et al., 2022). Based on the highly fibrous nature of the tigernut cake, eight optimized formulations were selected. The rationale behind this selection was to ensure that the fiber content of the tigernut cake component in the chosen formulations fell within the specified range of 1.5–3 g. In addition to the eight optimized selections, a standard control was included requiring no fermentation of the millet (Table 1).
Tigernut milk extraction
The tigernuts were sorted to remove contaminated, defective tubers, and whole tubers were washed with clean sterile water afterward. The tigernuts were then soaked for 12 h and blended with water in a ratio of 1:7 (w/v), and the obtained slurry was divided into two samples labeled A and B. Sample A was enzymatically treated at 55°C with cellulase for 6 h to reduce the starch. The slurries obtained from samples A and B were filtered to obtain the tigernut milk and further homogenized in the colloid mill. The preparation of tigernut milk and cake is presented in Figure 1.
Agglomerate preparation
The millet was sorted, washed, and soaked for 12 h. Using the disc attrition mill (Model SK-30-SS Attrition Mill), the millet was milled to obtain flour. The flour was divided into two equal parts and labeled A and B. Sample A was mixed with TNF in a ratio of 10:90 and 15:85. Both samples were mixed with 1% water and kneaded to form a compact dough. The dough was divided into two parts: one was allowed to spontaneously ferment for 12 h and the other for 24 h. Only the dough fermented for 12 h was used for the preparation of beverage and functional analysis based on the outcome of the functional analysis, which is comparable to the control (data not shown). Further preparation was carried out on four agglomerate formulations fermented for 12 h only (Table 1, selected formulations for beverage preparation). The fermented dough was then rolled on a sieve with a pore size of 2 mm to form uniform balls (agglomerate) and steam-cooked for 30 min (Figure 2) (Baidoo and Parry-Hanson Kunadu, 2018).
Preparation of composite milk
Using Sanful’s method (Sanful, 2009) with slight modifications, powdered milk was reconstituted by adding 140 g powdered milk to 540 mL potable water. Composite tigernut–dairy milk was prepared in a ratio of 60:40. The mixture was pasteurized for 15 min at 62°C. The pasteurized milk (800 mL) was allowed to cool to 43°C and spontaneously fermented in 1,000-mL Erlenmeyer flasks at 37°C for 10 h. The fermented milk was then pasteurized at 72°C for 30 min and allowed to cool.
Probiotic inoculum preparation
The frozen L. casei in vials was activated on MRS agar and subsequently cultured into MRS broth anaerobically at 37°C for 12 h. L. casei was subsequently cultured in sterile reconstituted dairy milk at 37°C for 20 h. The culture was used as an inoculum at 1% (v/v) in each composite tigernut–dairy milk for the beverages (Rozada-Sánchez et al., 2008). The preparation of the composite brukina beverage is depicted in Figure 3. Half of the fermented dairy–tigernut milk composite that did not undergo further pasteurization and probiotic inoculation was used as a control (unpasteurized and uninoculated control).
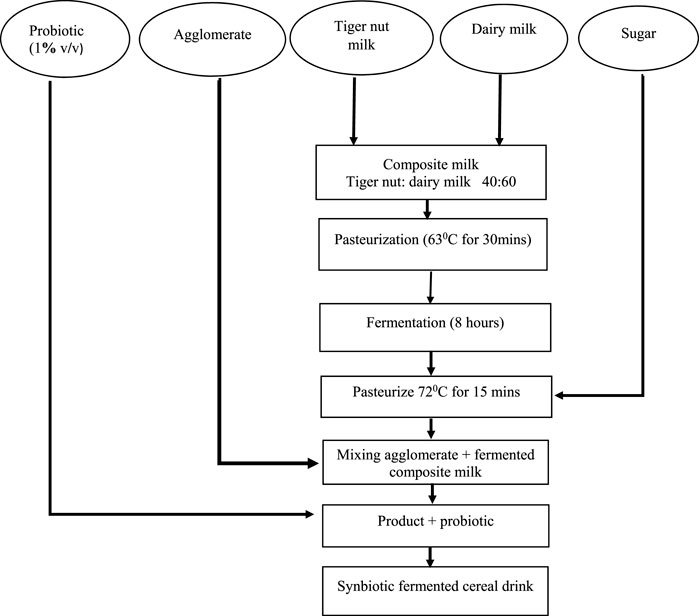
FIGURE 3. Flow diagram for the processing of fermented synbiotic cereal–tiger nut-based dairy beverage.
Functional properties of the agglomerate
The bulk density was determined in triplicate. The agglomerate sample was put into a 10-ml measuring cylinder and gently agitated continuously until a constant volume was obtained (Ocloo et al., 2014).
The water absorption capacity was determined in triplicate; 5.0 g of the sample was weighed and mixed with 20 ml of distilled water and allowed to stand for 30 min at a room temperature of 28°C. The mixture was then centrifuged (Centurion Scientific centrifuge K3 Series) at 1,512 RCF for 20 min. The excess water was decanted from the centrifuge tubes into a measuring cylinder, and the volume was determined. The water absorption capacity was calculated as volume (water absorbed/g of flour) (Ocloo et al., 2014).
For the swelling index, 5 g of each sample was placed in a graduated measuring cylinder containing 100 ml of distilled water at room temperature. It was tapped gently to eliminate air, and the initial volume was recorded. It was subsequently swirled around and allowed to stand for 5 h, and the final volume was recorded.
Determination of pH and titratable acidity
pH was determined using a digital pH meter (Mettler Toledo SevenCompact). For the titratable acidity, three drops of phenolphthalein were added to 15 ml of the sample in a beaker. The mixture was titrated against 0.1 N NaOH until the solution turned pink. The volume of NaOH used was recorded, and the titratable acidity was calculated (Feldsine et al., 2002).
Proximate analysis
Moisture content, crude protein, total ash, crude fiber, and carbohydrate contents were determined using standard methods (AOAC, 1997). The moisture content of the synbiotic beverage was determined using the oven drying method at 105°C for 5 h. The protein content was also determined by employing the micro-Kjeldahl method. Total ash was determined by igniting 2 g product at 550°C for 4 h using a muffle furnace. The crude fiber was determined using the digestion method, and the carbohydrate content was estimated by calculating the difference [100 − (% water + % protein + % fat + % ash + % crude fiber)]. The crude fat was determined using the standard Soxhlet extraction method with diethyl ether as the solvent (AOAC, 2000).
Mineral analysis of synbiotic beverage
Based on the results of the proximate composition, sample C was excluded in further mineral analysis. A wet digestion method using a closed-vessel microwave digester (ETHOS-EZ Milestone) was used for the digestion of synbiotic brukina for mineral analysis. Approximately 0.3 g of the sample was digested with nitric acid and sulfuric acid in a ratio of 2:1. The digested sample was transferred into a 50-ml volumetric flask; distilled water was added to achieve the final volume of 50 ml. Sodium (Na), potassium (K), magnesium (Mg), calcium (Ca), zinc (Zn), iron (Fe), phosphorus (P), manganese (Mn), and copper (Cu) were all determined in triplicate with the use of the PerkinElmer Atomic Absorption spectrophotometer (Model AA 220FS, Massachusetts, United States) (AOAC, 2005).
Microbiological assessments
Samples were analyzed for determining the counts of aerobic bacteria, yeast and molds, Staphylococcus aureus, Escherichia coli, and lactobacilli. A measure of 10 g of each sample was transferred into 90 mL of 0.1% sterile buffered peptone water (Oxoid, Hampshire) and homogenized in a stomacher for 1 minute. Appropriate serial dilutions were prepared from homogenates. Yeast and molds were enumerated on malt extract agar by spread plating appropriate dilutions on pre-poured plates. Inoculated plates were incubated at 25°C for 3–5 days before enumeration. S. aureus was enumerated by pour plating on mannitol salt agar (MSA, Oxoid CM0085). Inoculated plates were incubated at 37°C for 48 h. Yellow colonies with yellow zones surrounding them were counted and recorded as colony-forming units per ml of sample (CFU/ml). E. coli was enumerated on MacConkey agar with 24 h incubation at 37°C. Lactobacilli were enumerated on de Man Rogosa Sharpe agar (MRS Oxoid CM036) by using the pour plate overlay method. Plates were incubated at 37°C for 48 h before enumeration (Maselli and Hekmat, 2016).
Determination of accelerated shelf life using the Arrhenius model
Brukina beverages in plastic bottles with a net weight of 350 mL were stored at critical temperature variations of 5°C, 25°C, and 35°C. The analysis was carried out periodically every 24 h from days 0 to 5, to get five points of observation. Two parameters were used in the shelf life study: the log CFU/mL of L. casei and pH value of the product. The result data of each parameter were plotted against time (day) to generate linear equations. The three equations obtained for three conditions of product storage temperature are depicted using the following equations (Pulungan et al., 2018):
The choice of reaction order for a parameter is carried out by comparing the regression value (R2) of each linear equation at the same temperature. The reaction order with a larger R2 value is the order of reactions used by that parameter.
From Equation 2, k0 represents the deteriorating constant. It is the factor that determines the quality of a product kept at a constant temperature.
Based on the Arrhenius equation (Eq. 3) and the calculation of k, the shelf life of fermented cereal–tiger nut-based drink can be determined using the first-order kinetics:
Statistical analysis
The obtained data were analyzed using analysis of variance (ANOVA). The physicochemical parameters, functional properties, the L. casei growth, lactic acid production, and the developed agglomerate were used for the analysis of variance. The level of significance was set at p < 0.05. A general linear model was used to determine the interactions and variability between the groups influenced by the time, temperature, enzymatic treatment, and compositional blend. The statistical analysis was carried out using Minitab version 17.
Results and discussion
Swell index, water absorption capacity, and bulk density of the composite agglomerate
The compositional blend of 15% and 10% TNF agglomerates for the production of synbiotic brukina did not have any significant effect on the swell index and water absorption capacity (p = 0.422) and (p = 0.640), respectively (Table 2). Bulk density (BD) values for 10% and 15% TNF agglomerates, with and without enzyme hydrolysis, were 0.55–0.63 g/mL and 0.59–0.63 g/mL, respectively (Table 2). The observed difference in BD was significantly higher (p = 0.009) than that in the control. Similar bulk density values of 0.59 g/mL (Adejuyitan, 2011) and 0.56 g/mL (Chinma et al., 2009) have been reported for TNF-based products. This observed difference in the bulk density is associated with the varying starch composition of the agglomerate from varying sources, which influences structural differences.
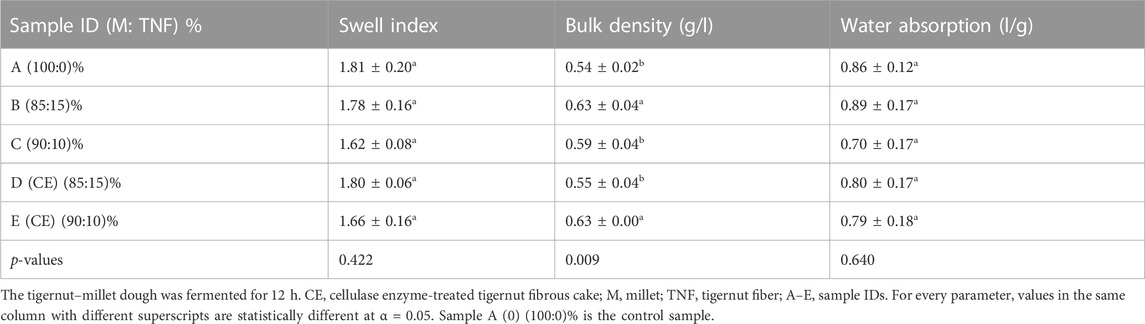
TABLE 2. Functional properties of cellulase- and non-cellulase-hydrolyzed tigernut fibrous cake-based millet agglomerate fermented for 12 h.
Steam cooking at atmospheric pressure leads to the absorption of water by the agglomerate until gel formation (Zarski et al., 2021). The high-water environment and heating are ideal for starch pasting and gelatinization, leading to starch granule swelling and losing crystallinity through the absorption of water. The overall effect is the creation of a gelatinized starch product. The linear amylose and available amylopectin align to form hydrogen bonding which expels water when cooled (Zarski et al., 2021). For this reason, the formed agglomerate does not absorb much moisture and has little capability to swell further. The similarity in the swell index and water absorption among all samples was expected as these functional properties are characteristics of starches found in all the agglomerate ingredients, as shown in Table 2. According to Wang et al. (2003), hydrolysis has been found to cause changes in the swelling power of starch morsels. Amylopectin plays a critical role in starch granule swelling and water-holding ability. Once the amylopectin structure is disrupted, an intact linkage cannot be formed, and the damaged chains tend to dissolve because they no longer can entrap water.
Proximate composition of the synbiotic brukina
The proximate composition of the fermented synbiotic brukina samples determined included moisture, ash, protein, fat, carbohydrate, and caloric content. The values were reported on a percentage dry-matter basis except for moisture content values. Table 3 shows the proximate composition of the synbiotic brukina samples made from millet–TNF dough fermented for 12 h.
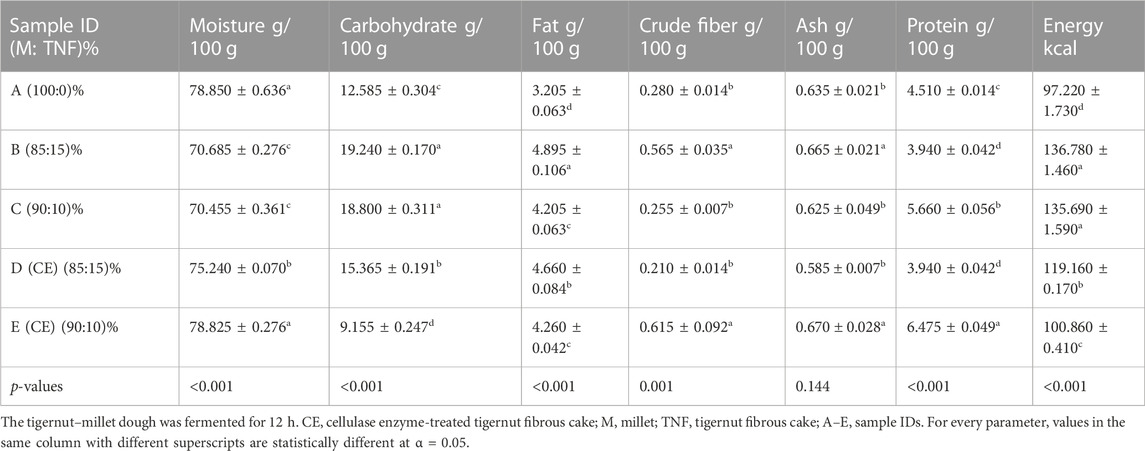
TABLE 3. Proximate composition of synbiotic cereal–tigernut-based brukina with varying compositions of cellulase- and non-cellulase-treated tigernut fibrous cake.
The moisture content of synbiotic brukina ranged from 78.85% to 70.45%. The values obtained were comparatively lower than 81.71%–86.45% reported in Awonorin and Udeozor (2014) and 92.44% reported in Badau et al. (2015), but higher than 62.8%–73.5% reported in Musa and Hamza (2013) for tigernut milk. Samples containing 15% and 10% TNF without cellulase hydrolysis recorded lower moisture content (p < 0.001) than the samples containing 10% and 15% hydrolyzed TNF-incorporated drinks, as shown in Table 3. TNF without cellulase hydrolysis has a high fiber content. Dietary fiber has the capacity to absorb and hold water molecules by binding them through hydrogen bonding and other interactions (Wong et al., 2022). The hygroscopic nature allows it to trap moisture, reducing the amount of free water available in the sample. The hydrolysis of TNF with cellulose and fermentation of the starch component in the TNF–millet samples led to increased tightly packed crystalline structure due to the preferential cellulose hydrolysis of amorphous regions within the biopolymers of the TNF and millet. The hydrogen bonds formed within the crystalline starch molecule in the product prevented water absorption into the formed gel, making enough moisture available, hence the observed high moisture in the product with hydrolyzed TNF incorporation. The observed moisture for a beverage is good since moisture of approximately 70% will provide enough free water. Lactobacillus casei is a mesophilic bacterium that grows optimally under high-to-moderate moisture conditions depending on the pH, temperature, and nutrient availability in its environment. Synbiotic samples with tigernuts had higher carbohydrate contents (p < 0.001) than control samples without tigernuts. The carbohydrate content of the samples ranged from 9.15% to 18.85%, which was higher than 8.5% reported in Frimpong (2016). This observation as shown in Table 3 was the same for the fat (p < 0.001) caloric content (p < 0.001) of the product. The observation can be explained from the physical and chemical changes that occur during processing. Heating starch-containing ingredients in the presence of moisture causes swelling and gelatinization of starch. This process increases digestibility and availability of starch, hence higher carbohydrate concentration. High temperatures also aid in hydrolysis of complex carbohydrates making them more easily digestible and available (Eliass and on, 2017). The incorporation of 40% tigernut milk and 10%–15% fiber into the beverage samples increased the fat content of the product. This characteristic can be attributed to the fact that tigernut is rich in fatty acids (Liu et al., 2019). The fat levels were within the range of 3.2%—4.8%. Tigernut fats, most of which are oleic acid, and monounsaturated fats (Eteshola and Oraedu, 1996) are known to help with weight loss, reduce the risk of heart disease, and decrease inflammation (Adeniyi et al., 2013). The crude fiber in all samples, except samples C and D, showed a significant increase in fiber content (p = 0.001) compared to control sample A but for samples C and D. However, the value range (0.21%–0.62%) was higher than 0.20% reported in Belewu and Belewu (2007) in tigernut-based products. The fiber in food means carbohydrates not digested or absorbed in the human small intestine (Lupton, 2010). Fiber increases fecal output and reduces the fecal pH by producing short-chain fatty acids in the colon, which reduces the incidence of colon cancer. It binds to bile to prevent its reabsorption in the liver, leading to inhibition of the synthesis of cholesterol, hence preventing diabetes, heart diseases, obesity, and certain degenerative diseases (Chen et al., 1984; Mann and Cummings, 2009).
The increase in protein and carbohydrate, crude fiber, and fat contents, as observed in the product, affirms the fact that the tigernut is an excellent composite material to be used in combination with cereals for the development of probiotic beverage (Eliasson, 2017). Carbohydrates contribute to the taste and flavor of the products (Eliasson, 2017), and it also serves as a carbon source for the probiotic microorganism L. casei in the product.
Mineral composition of synbiotic burkina samples
Table 4 shows the mineral profile of brukina samples. It was observed that the mineral content of the fermented synbiotic brukina samples was high in general. They showed no significant differences across all the minerals examined (p > 0.05) except for phosphorus (p = 0.002), sodium (p = 0.003), and calcium (p = 0.018). None exceeded the daily recommended mineral intake level despite the observed high mineral content. The differences in the minerals examined for various synbiotic brukina samples can be attributed to the factors such as the processing method employed in the product development and incorporation of whole tigernut in the product. Tigernut and millet are high in minerals such as magnesium, potassium, and phosphorous (Nalini et al., 2018), which contributed to the high mineral content observed in both ash and mineral profiles in Table 3 and Table 4, respectively.
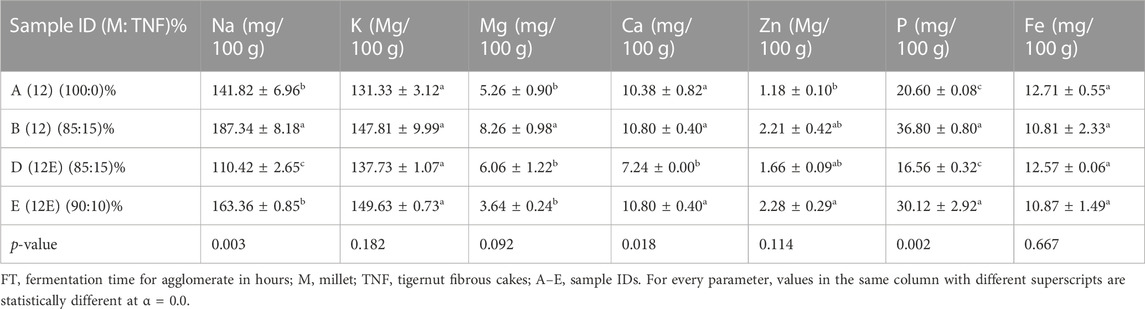
TABLE 4. Mineral composition of synbiotic composite cereal–tigernut-based brukina with 10% and 15% of cellulase- and non-cellulase-treated TNF.
Microbial quality, growth of L. casei in probiotic brukina, and shelf life of synbiotic brukina
E. coli, S. aureus, and yeast and molds were not detected in the pasteurized products sampled before and after inoculation with L. casei as depicted in Table 5 and Figures 4A,B. However, changes in the L. casei count in the synbiotic products were observed during storage at 5°C and 25°C, as shown in Table 6. L. casei attained 11 log CFU/mL counts in the brukina samples (A, B, D, and E) after 4 days for the storage at 5°C and 25°C. L. casei growth paralleled aerobic plate count (APC) growth in pasteurized and probiotic inoculated brukina at 5°C and 25°C (Figures 4A,B, respectively). The high count of microbes in the unpasteurized samples reflects the microbiological quality of raw materials that are used as spontaneously fermented products. All the samples showed a similar trend of L. casei growth with time. After day 4, products A, B, D, and E stored at 5°C recorded the final pH of 3.54, 3.56, 3.39, and 3.53, respectively, and the most considerable reduction in pH were recorded for all the products. The same trend was observed in the sample stored at 25°C. The observed trend of log increase in L. casei at refrigeration temperature is consistent with the result obtained in Parra et al. (2013) using a cereal blend where the viability of L. casei during 28 days of refrigeration storage survived and attained the highest populations after 7 days of storage in a cereal-based diet. Furthermore, higher survival of L. casei than that of Lactobacillus acidophilus has been found in varying functional fermented products and non-fermented products that do not contain milk (Kyung et al., 2005). The growth of L. casei depended on its ability to utilize the nutrients available in the growth medium for its survival. Corcoran et al. (2005) observed that the survival of lactobacilli in acidic environments is enhanced in the presence of metabolizable sugars that allow cell membrane proton pumps to operate and prevent the lowering of intracellular pH. L. casei is known to be more resistant to acidic conditions in a grain diet than other lactobacilli (Charal et al., 2002). The composite nature of the product, with cellulase hydrolyzed starch, made metabolizable sugars readily available for L. casei metabolic activity in samples kept at different storage temperatures. The initial pH range (4.41–4.75) of the products which resulted from the initial fermentation of both the agglomerate and the composite milk used for the product preparation also influenced the growth of the probiotic microorganism in the product. The beverage supported L. casei metabolic activity and growth, noting that L. casei possesses mechanisms to counteract the detrimental effects of low pH, such as the production of acid-tolerant enzymes, acidic amylase, acidic protease, and acidic phosphatase, and the maintenance of an internal pH homeostasis (Castro-López et al., 2022), hence the observed trends of increasing lactic acid production with corresponding growth in L. casei. For a probiotic-based ready-to-eat dairy-based product, the shelf life is defined by either pH changes (because of the live probiotic activity) or the probiotic count in the product with varying environmental temperature conditions (Kortei et al., 2020). The shelf life was estimated by transforming the pH using the regression of log shelf life versus temperature. The linear regression of pH as expressed by the Arrhenius model predicted the product’s shelf life as 5 days for the sample kept at 5°C, 4 days for 25°C, and 3 days for 35°C, as shown in Table 7. However, for uncertainty factors (Tano-Debrah et al., 2019), since only pH was considered a chemical parameter in the Arrhenius model, the shelf life was determined as 3 days to cater for possibilities of temperature abuse during storage.
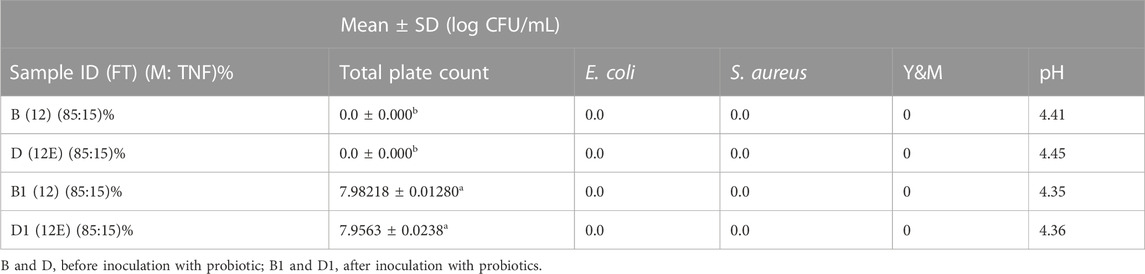
TABLE 5. pH and bacteriological quality of pasteurized composite cereal–tigernut-based brukina with 15% of cellulase- and non-cellulase-treated TNF before and after probiotic inoculation.
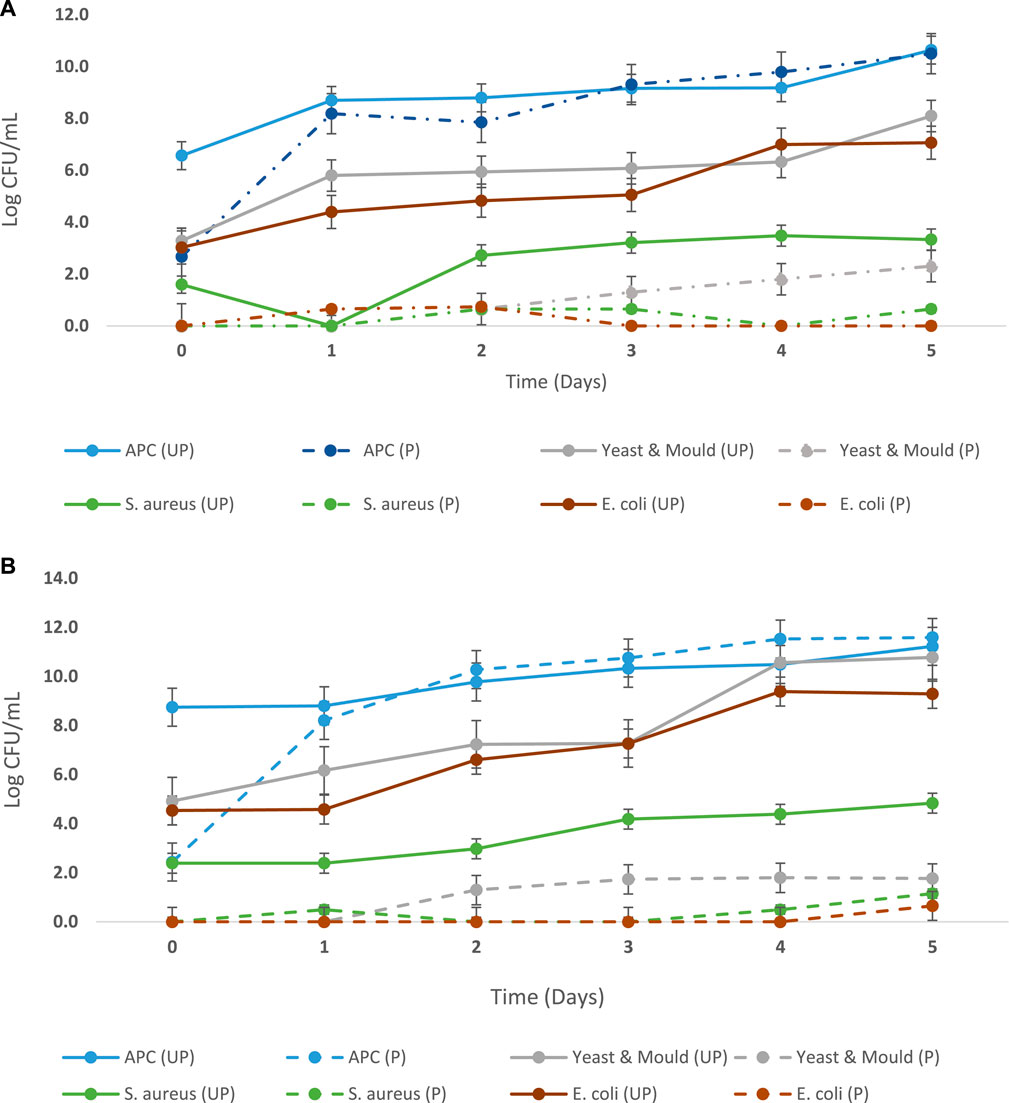
FIGURE 4. (A) Microbiological quality of pasteurized and Lacticaseibacillus casei-inoculated brukina (P) and unpasteurized and L. casei-uninoculated brukina after production (UP) stored at 5°C for 5 days. Pasteurization and inoculation with L. casei significantly reduced aerobic plate count (APC, p = 0.0003), yeast and mold (p = 0.018), S. aureus (p = 0.0007), and E. coli (p < 0.0001) over the storage time. (B) Microbiological quality of pasteurized and L. casei-inoculated brukina (p) and unpasteurized and L. casei-uninoculated brukina after production (UP) stored at 25°C for 5 days. Pasteurization and inoculation with L. casei significantly reduced aerobic plate count (APC, p = 0.0004), yeast and mold (p = 0.019), S. aureus (p = 0.0007), and E. coli (p < 0.0001) over the storage time.
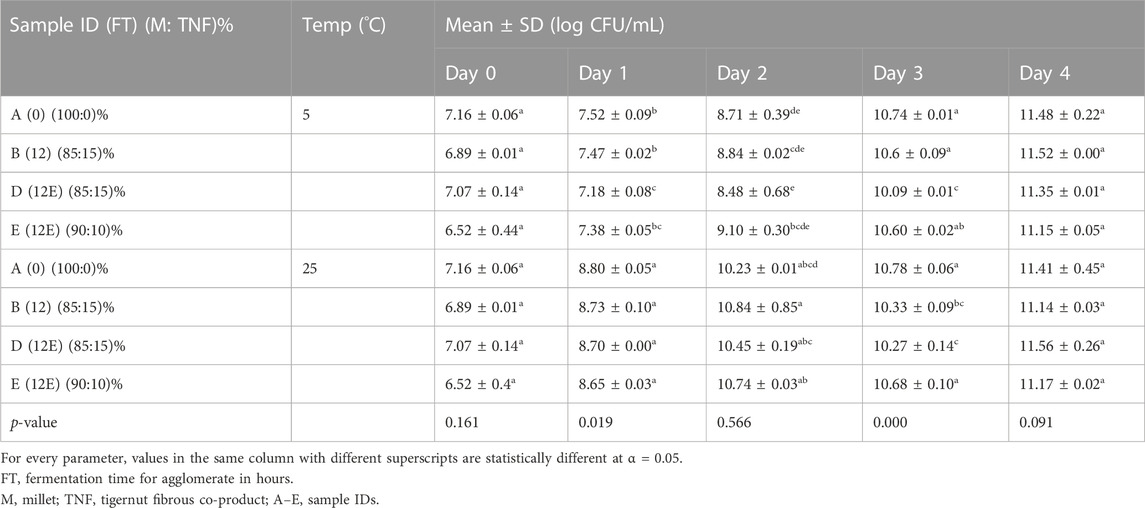
TABLE 6. Probiotic L. casei of synbiotic composite cereal–tigernut-based brukina with 10% and 15% of cellulase and non-cellulase-treated TNF stored at two varying temperatures 5°C and 25°C for 4 days.
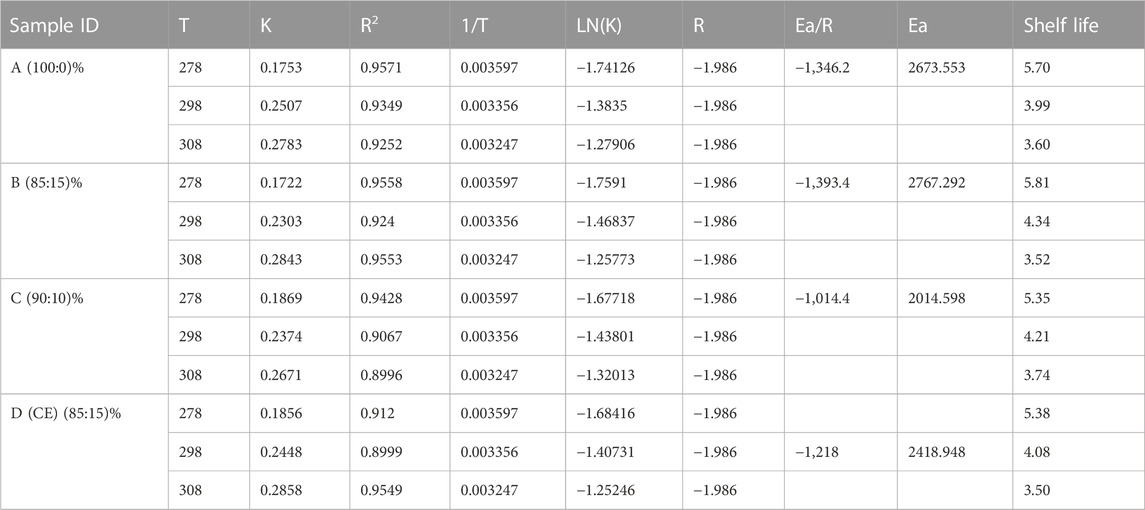
TABLE 7. Shelf life of synbiotic composite cereal–tigernut-based brukina with 10% and 15% of cellulase- and non-cellulase-treated TNF stored for 5 days at 5°C, 25°C, and 35°C.
Conclusion
A novel synbiotic brukina was developed incorporating tigernut milk, tigernut fibrous cake, enzyme hydrolysis, and inoculation with L. casei to improve the functional properties and nutritional quality and stability of brukina. Synbiotic brukina exhibited promising results in supporting the growth of L. casei, with a significant 1 log increase in probiotic count observed over a 4-day period under both refrigerated (5°C) and room temperature (25°C) conditions. While all formulations had comparable functional properties as the control, the agglomerate formulation that incorporated 10% TNF and enzyme hydrolyzed dough had superior macro- and micronutrient profiles and is thus recommended for commercialization or used in nutrition interventions. The high fiber content ranging from 0.21% to 0.62% indicates potential benefits in terms of increased fecal output, reduced fecal pH, and enhanced protective gut microbes. The utility organisms (yeast and molds) and pathogen indicator microorganism (E. coli) were reduced to undetectable levels after pasteurization and L. casei inoculation, and their numbers remained low throughout the storage period. This buttresses the microbiological safety of the novel beverage, which is one of the major challenges faced with the traditional brukina beverage. The shelf life of 3 days was limited by sourness of the product from high concentration of the probiotic, L. casei, at 35°C. At chilled temperatures, the product is stable for at least 4 days.
Data availability statement
The original contributions presented in the study are included in the article/Supplementary material; further inquiries can be directed to the corresponding author.
Author contributions
AP-HK conceptualized the study, supervised the work, and wrote the final draft. SY also contributed to the conceptualization of the work, analyzed the samples, collated and analyzed the data, and wrote the original draft. LD analyzed the samples and edited the first draft of the manuscript. FS supervised the work. AO supervised the work and edited the final manuscript. GA supervised the work and supported with the sample analysis. All authors contributed to the article and approved the submitted version.
Acknowledgments
The authors thank the Environmental Chemistry Laboratory of CSIR-Water Research Institute for their support in mineral analysis, the Food Chemistry and Mycotoxin Laboratory of CSIR-Food Research Institute for their support in proximate and vitamin analyses, and Richard Bayitse for providing the cellulase enzyme for this research.
Conflict of interest
The author(s) AP-HK declared that they were an editorial board member of Frontiers, at the time of submission. This had no impact on the peer review process and the final decision.
The authors declare that the research was conducted in the absence of any commercial or financial relationships that could be construed as a potential conflict of interest.
Publisher’s note
All claims expressed in this article are solely those of the authors and do not necessarily represent those of their affiliated organizations, or those of the publisher, the editors, and the reviewers. Any product that may be evaluated in this article, or claim that may be made by its manufacturer, is not guaranteed or endorsed by the publisher.
Supplementary material
The Supplementary Material for this article can be found online at: https://www.frontiersin.org/articles/10.3389/frfst.2023.1228643/full#supplementary-material
References
Adebiyi, J. A., Obadina, A. O., Adebo, O. A., and Kayitesi, E. (2016). Fermented and malted millet products in Africa: expedition from traditional/ethnic foods to industrial value-added products. Crit. Rev. Food Sci. Nutr., 1–12. doi:10.1080/10408398.2016.1188056
Adejuyitan, J. A. (2011). Tigernut processing: its food uses and health benefits. Am. J. Food Technol. 6 (3), 197–201. doi:10.3923/ajft.2011.197.201
Adeniyi, T. A., Adeonipekun, P. A., and Omotayo, A. E. (2013). Investigating the phytochemicals and antimicrobial properties of three sedge (Cyperaceae) species. Int. J. Trop. Med. 8 (4), 92–98. doi:10.36478/ijtmed.2013.92.98
AOAC (2005). AOAC official method 999.10 lead, Cadmium, zinc, Copper, and Iron in foods Atomic absorption Spectrophotometry after microwave digestion. Gaithersburg, MD. Edition 18.
AOAC (2000). “Fat (crude) or ether extract in Animal feed,” in Official methods of analysis of AOAC International.
AOAC (1997). “Official methods of analysis of the association of official Analytical Chemists (method 945.46),” in Official methods of analysis of the association of official Analytical Chemists.
Arafat, S. M., Gaafar, A. M., Basuny, A. M., and Nassef, S. L. (2009). Chufa tubers (Cyperus esculentus L.): as a New source of food. World Appl. Sci. J. 7 (2), 151–156.
Asante, F. A., Oduro, I., Ellis, W. O., and Saalia, F. K. (2014). Effect of planting period and site on the chemical composition and milk acceptability of tigernut (Cyperus Esculentus L) tubers in Ghana. Am. J. Food Nutr. 2 (3), 49–54. doi:10.12691/ajfn-2-3-3
Awonorin, S. O., and Udeozor, L. O. (2014). Chemical properties of tiger nut-Soy milk extract. IOSR J. Environ. Sci. Toxicol. Food Technol. 8 (3), 87–98. doi:10.9790/2402-08318798
Badau, M. H., Bristone, C., Igwebuike, J. U., and Igwegbe, A. O. (2015). Production and evaluation of yoghurt from mixtures of cow milk, milk extract from soybean and tiger nut. World J. Dairy and Food Sci. 10 (2), 159–169. doi:10.5829/idosi.wjdfs.2015.10.2.94216
Baidoo, W., and Parry-Hanson Kunadu, A. (2018). Using low technology to improve the safety of informal-vended brukina–A fermented milk and millet smoothie in Ghana. Afr. J. Food, Agric. Nutr. Dev. 18 (3), 13646–13663. doi:10.18697/ajfand.83.17620
Belewu, M. A., and Belewu, K. Y. (2007). Comparative physico-chemical evaluation of tiger-nut, soybean, and coconut milk sources. Int. J. Agric. Biol. 5 (785), e787.
Castro-López, C., Romero-Luna, H. E., García, H. S., Vallejo-Cordoba, B., González-Córdova, A. F., and Hernández-Mendoza, A. (2022). Key stress Response mechanisms of probiotics during their Journey through the digestive System: A review. Probiotics Antimicrob. Proteins 15, 1250–1270. doi:10.1007/s12602-022-09981-x
Charalampopoulos, D., Wang, R., Pandiella, S. S., and Webb, C. (2002). Application of cereals and cereal components in functional foods: A review. Int. J. Food Microbiol. 79, 131–141. doi:10.1016/s0168-1605(02)00187-3
Chen, W. J. L., Anderson, J. W., and Jennings, D. (1984). Propionate may mediate the Hypocholesterolemic effects of certain Soluble plant fibers in cholesterol-Fed Rats. Exp. Biol. Med. 175 (2), 215–218. doi:10.3181/00379727-175-41791
Chinma, C. E., Adewuyi, O., and Abu, J. O. (2009). Effect of germination on the chemical, functional and pasting properties of flour from brown and yellow varieties of tigernut (Cyperus esculentus). Food Res. Int. 42 (8), 1004–1009. doi:10.1016/j.foodres.2009.04.024
Corcoran, B. M., Stanton, C., Fitzgerald, G. F., and Ross, R. P. (2005). Survival of probiotic lactobacilli in acidic environments is enhanced in the presence of metabolizable sugars. Appl. Environ. Microbiol. 71, 3060–3067. doi:10.1128/AEM.71.6.3060-3067.2005
da Costa Neto, J. J. G., Gomes, T. L. M., Justo, T. F., Pereira, K. S., Amaral, P. F. F., Rocha Leão, M. H. M., et al. (2019). Microencapsulation of tiger nut milk by lyophilization: morphological characteristics, shelf life and microbiological stability. Food Chem. 284, 133–139. doi:10.1016/j.foodchem.2019.01.110
Eliasson, A. C. (2017). “Starch: physicochemical and functional aspects,” in Carbohydrates in food. Third Edition.
Eteshola, E., and Oraedu, A. C. I. (1996). Fatty acid compositions of tigernut tubers (Cyperus esculentus L.), baobab seeds (Adansonia digitata L.), and their mixture. JAOCS, J. Am. Oil Chemists’ Soc. 73, 255–257. doi:10.1007/bf02523905
Feldsine, P., Abeyta, C., and Andrews, W. H.AOAC International Methods Committee (2002). AOAC INTERNATIONAL methods Committee Guidelines for Validation of Qualitative and Quantitative food microbiological official methods of analysis. J. AOAC Int. 85 (5), 1187–1200. doi:10.1093/jaoac/85.5.1187
Frimpong, R. Y. (2016). Hysicochemical and sensory evaluation of Biscuits made from Defatted tiger nut and Wheat composite flour [Thesis]. University of Ghana.
Ioniță-Mîndrican, C. B., Ziani, K., Mititelu, M., Oprea, E., Neacșu, S. M., Moroșan, E., et al. (2022). Therapeutic benefits and dietary Restrictions of fiber intake: A state of the Art review. Nutrients 14 (13), 2641. doi:10.3390/nu14132641
Jimoh, M. O., and Kole, O. F. (2022). Quality acceptability of wine produced from fermented tiger nut drink fortified with coconut milk. Res. J. Food Sci. Nutr. 7 (2), 30–38. doi:10.31248/rjfsn2021.135
Kim, M., No, S., and Yoon, S. H. (2007). Stereospecific analysis of fatty acid composition of Chufa (Cyperus esculentus L .) tuber oil. J. Am. Oil Chemists’ Soc. 84, 1079–1080. doi:10.1007/s11746-007-1131-8
Kortei, N. K., Annan, T., Quansah, L., Aboagye, G., Akonor, P., and Tettey, C. (2020). Microbiological quality evaluation of ready-to-eat mixed vegetable salad, food ingredients and some water samples from a restaurant in Accra: A case study. Afr. J. Food, Agric. Nutr. Dev. 20 (06), 16669–16688. doi:10.18697/ajfand.94.18805
Kyung, Y. Y., Woodams, E. E., and Hang, Y. D. (2005). Fermentation of beet juice by beneficial lactic acid bacteria. LWT - Food Sci. Technol. 38, 73–75. doi:10.1016/j.lwt.2004.04.008
Liu, X. X., Liu, H. M., Li, J., Yan, Y. Y., Wang, X. D., Ma, Y. X., et al. (2019). Effects of various oil extraction methods on the structural and functional properties of starches isolated from tigernut (Cyperus esculentus) tuber meals. Food Hydrocoll. 95, 262–272. doi:10.1016/j.foodhyd.2019.04.044
Lupton, J. (2010). Codex definition of dietary fibre and issues requiring resolution. Diet. Fibre New Front. Food Health.
Mann, J. I., and Cummings, J. H. (2009). Possible implications for health of the different definitions of dietary fibre. Nutr. Metabolism Cardiovasc. Dis. 19 (3), 226–229. doi:10.1016/j.numecd.2009.02.002
Maselli, L., and Hekmat, S. (2016). Microbial vitality of probiotic milks supplemented with cereal or pseudocereal grain flours. J. Food Res. 5 (2), 41. doi:10.5539/jfr.v5n2p41
Munekata, P. E. S., Pérez-Álvarez, J. Á., Pateiro, M., Viuda-Matos, M., Fernández-López, J., and Lorenzo, J. M. (2021). Satiety from healthier and functional foods. Trends Food Sci. Technol. 113, 397–410. doi:10.1016/j.tifs.2021.05.025
Musa, A. A., and Hamza, A. (2013). Comparative analysis of locally prepared ‘kunun aya’ (tiger-nut milk) consumed by students of Kaduna State University, Kaduna Nigeria. Sci. World J. 8 (2), 13–18.
Nalini, P., Abraham R, J. J., Appa Rao, V., Narendra Babu, R., Nobal Rajkumar, T., Rajkumar, R., et al. (2018). Shelf-life of ready-to-eat Retort processed Pepper Chicken. Int. J. Curr. Microbiol. Appl. Sci. 7, 832–840. doi:10.20546/ijcmas.2018.703.097
Ocloo, F. C. K., Okyere, A. A., and Asare, I. K. (2014). Physicochemical, functional and pasting properties of flour produced from gamma irradiated tiger nut (Cyperus esculentus L.). Radiat. Phys. Chem. 103, 9–15. doi:10.1016/j.radphyschem.2014.05.010
Parra, K., Ferrer, M., Piñero, M., Barboza, Y., and Medina, L. M. (2013). Use of Lactobacillus acidophilus and Lactobacillus casei for a potential probiotic legume-based fermented product using pigeon pea (Cajanus cajan). J. Food Prot. 76 (2), 265–271. doi:10.4315/0362-028X.JFP-12-138
Pulungan, M. H., Sukmana, A. D., and Dewi, I. A. (2018). Shelf-life prediction of apple brownies using accelerated method. IOP Conf. Ser. Earth Environ. Sci. 131, 012019. doi:10.1088/1755-1315/131/1/012019
Rozada-Sánchez, R., Sattur, A. P., Thomas, K., and Pandiella, S. S. (2008). Evaluation of Bifidobacterium spp. for the production of a potentially probiotic malt-based beverage. Process Biochem. 43, 848–854. doi:10.1016/j.procbio.2008.04.002
Sánchez-Zapata, E., Pérez-Alvarez, J. A., and Fernández-López, J. (2012). Effects of tiger nut (Cyperus esculentus) milk liquid co-products on the quality of pork burgers. Int. J. Food Sci. Technol. 47 (10), 2198–2204. doi:10.1111/j.1365-2621.2012.03089.x
Sanful, R. E. (2009). The use of tiger-nut (Cyperus esculentus), cow milk and their composite as substrates for yoghurt production. Pak. J. Nutr. 8 (6), 755–758. doi:10.3923/pjn.2009.755.758
SRID Agriculture in Ghana, Facts and Figures (2010). in Accra, Ghana: Statistics, research, and Information Directorate. Source Available at: http://gis4agricgh.net/POLICIES/AGRICULTURE-IN-GHANA-FF-2010.pdf.
Tano-Debrah, K., Saalia, F. K., Ghosh, S., and Hara, M. (2019). Development and sensory shelf-life Testing of KOKO Plus: A food supplement for improving the nutritional profiles of traditional Complementary foods. Food Nutr. Bull. 40 (3), 340–356. doi:10.1177/0379572119848290
Wang, Y. J., Truong, V. D., and Wang, L. (2003). Structures and rheological properties of corn starch as affected by acid hydrolysis. Carbohydr. Polym. 52 (3), 327–333. doi:10.1016/s0144-8617(02)00323-5
Wong, K. Y., Thoo, Y. Y., Tan, C. P., and Siow, L. F. (2022). Moisture absorption behavior and thermal properties of sucrose replacer mixture containing inulin or polydextrose. Appl. Food Res. 2 (1), 100089. doi:10.1016/j.afres.2022.100089
Xu, W., Lin, L., Liu, A., Zhang, T., Zhang, S., Li, Y., et al. (2020). l -Theanine affects intestinal mucosal immunity by regulating short-chain fatty acid metabolism under dietary fiber feeding. Food Funct. 11 (9), 8369–8379. doi:10.1039/d0fo01069c
Yu, Y., Lu, X., Zhang, T., Zhao, C., Guan, S., Pu, Y., et al. (2022). Tiger nut (Cyperus esculentus L.): nutrition, processing, function and applications. Foods 11 (4), 601. doi:10.3390/foods11040601
Keywords: brukina, tigernut, probiotic, prebiotic, fermentation, synbiotic
Citation: Yeboah S, Ohemeng A, Donkor L, Saalia FK, Annor GA and Kunadu AP-H (2023) Characterization of physicochemical and microbial quality, functional properties, and shelf stability of fermented tigernut-based probiotic beverages. Front. Food. Sci. Technol. 3:1228643. doi: 10.3389/frfst.2023.1228643
Received: 31 May 2023; Accepted: 13 July 2023;
Published: 28 September 2023.
Edited by:
António Raposo, Universidade Lusófona Research Center for Biosciences and Health Technologies, PortugalReviewed by:
Cid Marcos Gonçalves Andrade, State University of Maringá, BrazilVishu Chaudhary, Chandigarh University, India
Muhammad Hazwan Hamzah, Putra Malaysia University, Malaysia
Copyright © 2023 Yeboah, Ohemeng, Donkor, Saalia, Annor and Kunadu. This is an open-access article distributed under the terms of the Creative Commons Attribution License (CC BY). The use, distribution or reproduction in other forums is permitted, provided the original author(s) and the copyright owner(s) are credited and that the original publication in this journal is cited, in accordance with accepted academic practice. No use, distribution or reproduction is permitted which does not comply with these terms.
*Correspondence: Angela Parry-Hanson Kunadu, YXBhcnJ5LWhhbnNvbkB1Zy5lZHUuZ2g=