- 1Department of Chemical and Biomedical Engineering, Columbia, MO, United States
- 2Division of Food, Nutrition and Exercise Science, The University of Missouri, Columbia, MO, United States
With the increased interest in plant-based foods, a growing demand for high protein plant-based alternatives has spiked the food industry. Consumer acceptance of a food product is influenced by the natural flavor or through the addition of familiar flavors, which influence the purchase of the food product. There is a wide variety of soy-based products available on the market, with soymilk being one of the most popular among vegetarian diets. Soybean products have been known for their beany or malty flavor, having advantage in certain types of food products like tofu yet experiencing market resistance among certain population groups that are unfamiliar with the beany flavor of soybean. Improving the flavor profile might significantly change consumer perceptions toward soy-based food products. In the current study, chocolate and vanilla flavors were added to newly developed varieties of soybean milk (high oleic and tofu line) to investigate the impact of flavoring components on the physicochemical characteristics of novel soybean varieties. Soymilk with flavoring components were spray-dried, and the resultant powders were subjected to physical, reconstitution, rheological, particle, and thermal property analysis. Experimental analysis and statistical data showed that powder properties were improved with the addition of flavoring components. Obtained product yield (57%–66%), flowability (good to passable), wettability (2.52–1.34 min), dispersibility (83%–95%), solubility (75%–86%), viscosity (3.22–10.35 mPa s), thermal stability (≥15°C), particle size distribution (improved homogeneity), and morphology (smooth and spherical). The obtained results would be of interest to food processors and food product developers looking for plant-based alternatives.
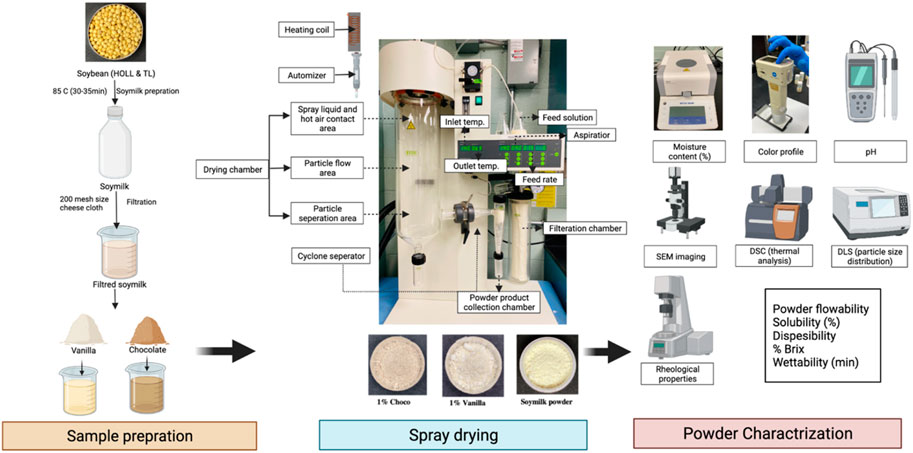
GRAPHICAL ABSTRACT | Graphical representation of flavoring component integration in soymilk and spray dried to investigate the impact of flavoring components on the physicochemical parameters of a soymilk powder.
Highlights
1. Spray drying of the soymilk with the chocolate and vanilla as flavors
2. Analyzing the physicochemical properties of flavored soymilk powder
3. The rheological, thermal, and particle properties of soymilk powder were explored
4. The market potential and applications of a flavored soymilk powder
1 Introduction
Soybean (Glycine max) is a leguminous plant cultivated worldwide due to its high nutritious value. Soybean is highly rich in protein (36%–46%), lipid (18%–25%), and carbohydrate (25%–30%), and some micronutrients, which is considered a nutrition-rich food source for vegetarian population (Rizzo and Baroni, 2018) (Isanga and Zhang, 2008). Due to its nutritional profile, soybean is also referred to as the “king of beans,”. Soybean is extensively grown all over the world; based on data, the United States (48.19%), Brazil (43.96%), and Argentina (7.61%) are the topmost producer of soybean, followed by China and India (Voora et al., 2020). The soybean production trend has continuously inclined from 2012 to 2020, and it was reported that the overall soybean market reached USD 143.23 billion in the year 2017 (Voora et al., 2020). More than a thousand soybean varieties have developed in the last few years all over the world. However, traditionally grown soybean comprises a high amount of linoleic acid (54%), saturated fatty acid (9%–22%) but less in oleic acid (11%), which is responsible for increasing the low-density lipoprotein (LDL) cholesterol in our body (Rizzo and Baroni, 2018) (Taha, 2020). A new variety of soybeans has been developed with high oleic and low linoleic acid content (HOLL) to reduce cholesterol accumulation and add more sustainability to the soy processing industry. The newly developed high oleic (HO) Soybean variety contains 75%–80% oleic acid and reduced linoleic acid, which reduces the risk of cardiovascular disease by increasing the high-density lipoprotein (HDL) (Piccinin et al., 2019). The annual demand for soybean oil with the trans-fat label is rising, which has resulted in a 9-billion-pound increase in demand for high oleic soybean. Due to high market demand, production of high oleic soybean and commodity soybean is increasing, creating a new opportunity to commercialize high oleic soybean. Additionally, in the current environment, restaurants, soybean processing businesses, and consumers are shifting to high oleic soybeans to obtain a nutrient-dense diet (High Oleic Soybeans, 2022). Furthermore, the tofu line (TF) soybean is also a recently introduced variety of soybean that is highly rich in protein and considered a good source of raw material for developing fermented soy-based products (USDA ARS, 1997). The reason for employing these novel soybean varieties is that they have an improved nutritional profile and the potential to develop nutritious soy-based food products on a commercial scale.
One of the most enticing reasons for vegetarians’ interest in soy and soy cuisine is the high protein content of soybeans (Sharma et al., 2014). With the rise in vegetarianism, a variety of soy-based food products have become more readily available in the market. Several soy-based products are available in the market, including soymilk, soy-yogurt, tofu, tamari, tempeh, soy flour, soy protein, etc. Many of these soy-based products are perishable commodities that are vulnerable to changes in environmental conditions. Most importantly, these soy-based products have a beany, malty, or painty flavor because of enzymatic reaction during processing, that influences consumer acceptance of soy-based products (Yang et al., 2015). The intensity of beany flavor could be slightly improved by soaking, microwave cooking, pressure cooking, and thermal processing methods (Yu et al., 2017). However, soy-based food products contain some beany flavor which still influences the consumer acceptability due to their taste preference. Soymilk is one of the highly consumed soy-based food and one of the best alternatives for a vegan/vegetarian/lactose intolerance diet. So, the flavor profile of soymilk/soy-based food products could be further improved by adding flavoring components, which will also influence the customer acceptability for soy-based food products. Converting soymilk into powdered form helps to extend its shelf life, reduce transportation costs, make it easier to handle, and it can be used for developing reconstituted beverages (Assadpour and Jafari, 2019).
Spray drying is one of the most efficient and successful forms of drying technology. It converts the liquid feed solution into a dried powder at a rapid evaporation rate, making it one of the most cost-effective forms of drying. Spray drying is a one-step drying process that is utilized extensively in the flavoring, cosmetic, pharmaceutical, and food sectors (Sosnik and Seremeta, 2015). The spray dryer is fitted with a spray nozzle with a specified size (0.15–2 mm) of the nozzle tip, which converts the feed solution into mist and transports it to the drying chamber (Ousset et al., 2018). Mini spray dryer is integrated with a two-fluid nozzle, in which compressed gas (air or N2) disperses the feed solution into the fine droplets inside the drying chamber (Spray Dryer Manual). Hot air encounters the mist in the drying chamber and converts it to powder form (S. Singh and Dixit, 2014). Spray-dried powder is drawn into the collection chamber via the aspirator by a co-current, counter-current, or mixed airflow within the drying chamber. In the co-current flow, drying materials come into contact with the cooler air, which is mainly used for thermosensitive materials, and in counter-current flow, hot air is exposed to the drying materials (Raffin et al., 2006).
During the drying process, spray dryers’ parameters such as inlet temperature, feed flow rate, and aspirator can be optimized, which helps to improve product yield and other physicochemical properties of dried powder (P. Singh et al., 2022). Furthermore, other parameters such as outlet temperature, pressure drop, relative humidity (%), and airflow rate change impact powder quality, which can be adjusted by changing the filter, connecting the dehumidifier, and optimization the spray drying parameters.
In the current study, HO soymilk (HOSM) combined with flavoring elements (1%vanilla and 1% chocolate) was spray-dried to investigate the impact of flavoring components on the physicochemical properties of soymilk powder. The flavoring components mask the beany and malty flavor of soymilk powder but does impact the powder quality of final product. Many factors might contribute to changes in physicochemical qualities, including composition of flavoring component, processing conditions, and masking products. Derived from a previous study on optimizing spray drying parameters for soymilk powder, the inlet temperature was set at 140°C, the aspirator at 35 m3/h, and the feed rate at 5 mL/min (P. Singh et al., 2022). Furthermore, some trial experiments were carried out with higher concentrations (2% and 4%) of flavoring components. Non-etheless, it was observed that a higher concentration of flavoring components had an adverse impact on the yield of soymilk powder. It could be caused by stickiness and hygroscopic behavior of flavoring component due to its dextrose base, which results in the accumulating powder particles in the cyclone separator area (Nishad et al., 2017). Taking this into consideration, a lower concentration of vanilla and chocolate flavor (1%) was added to enhance the flavor profile of soymilk powder. In this experiment, TF soymilk (TLSM) and HOSM were used to study the behavior of flavoring components in the soymilk powder. A total of six sets of experiments were performed in triplicates using HOSM and TLSM as a control sample mixed with vanilla and chocolate flavors. After spray drying, soymilk powder from all combinations was used for analyzing its physical, reconstitution, rheological, particle, and thermal parameters to study the impact of flavoring components on powder properties of spray-dried soymilk powder. In this study, variables were outlet temperature, run time, and thermal efficiency (%) of the drying process, as well as powder characterization parameters like product yield (%), color value (L*, a*, b*, chroma, hue angle, whitening index), flowability (Carr Index, Hausner ratio), water solubility (%), dispersibility, moisture content (%), water activity (Aw), and wettability (min) and viscosity. Furthermore, particle size distribution, thermal degradation behavior, and morphology of the HOSM and TLSM were studied in combination with the flavoring components.
2 Materials and methods
2.1 Soymilk sample preparation
To prepare the soymilk sample, 125 g of soybean was soaked in 1,000 mL of water in 0.1% of NaHCO3 solution overnight (14 ± 2 hr) at room temperature (25°C). Then it was thoroughly washed with drinking water to remove the residue of NaHCO3 from the surface of the soaked soybean. Soaked soybean and 1,500 mL of water was added to the SoyJoy G4soymilk maker (SoyaJoy brand procured from the Amazon, United States), and machine was run on soaked bean soymilk making mode. During the soymilk making, processing temperature reached 80°C, and the soymilk-making time was 30 ± 5 min. After soymilk preparation, it was filtered using a muslin cheese cloth and passed through the vacuum filter using Whatman filter paper (Fisher brand, Filter No. P8, United States) to prepare the diluted feed solution to make it suitable for spray drying processing. Flavoring components vanilla (1%) and chocolate (1%) were thoroughly mixed with the soymilk samples. Vanilla (pure vanilla extract in dextrose base) and chocolate (cocoa in dextrose base combined with cinnamon and nutmeg) flavoring components manufactured in Cook’s Flavorings were purchased from Amazon, United States. After sample preparation total solid content of the feed solution was measured to calculate the product yield of the spray drying process. The average % total solid in soymilk (TL and HO) was 4.56% ± 0.09% which increases to 5.56% with the addition of flavoring components (1%).
2.2 Spray drying parameters
HOSM and TLSM mixed with vanilla and chocolate flavors were spray-dried using a B-290 mini spray dryer 65 × 110 × 70 cm (W × H × D) (Mini Spray Dryer B-290 procured from the BUCHI Corporation) (B-290 operation Manual, 2020). A 0.7 cm peristaltic pump was attached to the spray dryer in order to maintain peristaltic flow inside the drying chamber, which contains two titanium fluid nozzles with a 0.15 mm tip size. The inlet temperature was kept at 140°C, the aspirator at 35 m3/h, and the feed flow rate at 5 mL/min throughout the drying process. The drying and collecting chambers were made of 3.3 borosilicate glass to withstand thermal shock. Spray dryer glass chambers are coated with perfluoroalkoxy polymer to prevent contamination and acidic reactions (Arpagaus and Schwartzbach, 2008). The soymilk mixed with flavoring components was spray-dried in triplicate to calculate the thermal efficiency (%) of drying process and product yield (%) of collected powder. Prior to starting the experiment spray dryer was run for 10 min with double distilled water to make sure that system is working properly and reached a steady state.
2.3 Thermal efficiency of spray drying process
The outlet temperature of the mini spray dryer B-290 outlet pipe was monitored with a thermocouple sensor to determine the thermal efficiency of the spray drying process. The sum of all heat and mass transmission during spray dying results directly influences the outlet temperature. Other spray dryer variables, such as inlet temperature, aspirator and feed rate, and the qualities of the spray-dried material, do affect the outlet temperature (Santos et al., 2017). Thermal efficiency (ɳ) is the ratio of heat consumed to heat supplied (Cheng et al., 2018).
Where ɳ represent thermal efficiency (%) of drying process, T1 inlet temperature °C, T2 outlet temperature °C, and Ta is room temperature °C.
2.4 Product yield (%)
The product yield (%) of the spray dried sample was determined by using Sasikumar and Singh methods (Singh P. et al., 2022). The initial solid mass in feed solution and recovered mass of dried powder was measured to calculate the product yield (%) of spray dried powder.
2.5 Powder characterization parameters
2.5.1 Color value
The bare human eye can detect a substantial change in color between two or more samples, but an accurate color profile may be determined by using some techniques. The color value of soy-milk powder was measured using a colorimeter (Konika Minolta® CR-410, Ramsey, New Jersey, United States). The calorimeter was calibrated with the white plate before taking the color value of soymilk with the flavoring component. The color values L* (lightness), a* (redness coefficient), and b* (yellowness coefficient) were recorded to calculate the chromatic index, whitening index, and hue angle for spray-dried soymilk powder with and without flavoring component (Ogolla et al., 2019). All the color value were measured in quadruple (n = 4)
Color value L* ranges from 0 to 100, in which 0 color value indicates the sample is darker in color; however, 100 color value signifies the lightness of the product. Likewise, chromaticity values a* and b* vary from positive to negative here a* = red,-a* = green, b* = yellow, and -b* = blue (Milovanovic et al., 2020). Furthermore, ∆E indicates the difference in color profile between the soymilk added with the flavoring agents (1% chocolate and 1% vanilla) with respect to the control sample (plain soymilk from TLSM and HOSM).
2.5.2 Flowability
Flowability, which measures the flow properties of spray-dried milk powder, was determined using the Carr index (CI) value. Similarly, the cohesiveness of the spray-dried powder was estimated based on the Hausner ratio (HR) value. Carr index and Hausner ratio were calculated using the bulk density and tapped density of spray-dried powder. To determine the bulk density of spray-dried soymilk powder, 1 g of the powder was poured into the 10 mL graduated cylinder, and its bulk volume was recorded. Then, it was allowed to be tapped by using an electronic benchtop laboratory shaker (Tekmar VXR JANKE, and KUNKEL VX9, United States) at 1,250 rpm for 15 min to record the tapped volume of soymilk powder. Bulk density and tapped density were calculated by dividing mass by bulk volume (bulk density) and tapped volume (tapped density). Bulk density (ρb) and tapped density (ρt) values were used to calculate the CI and HR (Ogolla et al., 2019).
Soymilk powder with a Carr index value below 25% is considered to have an acceptable flowability, whereas a value above 25% indicates poor flow behavior. Similarly, less than 1.2 HR value indicates less cohesiveness and good flowable powder; however, more than 1.2 HR value reflects highly cohesive and less flowable food powder (Nekkanti et al., 2009). To measure the porosity of spray-dried soymilk, sample particle density/true density was recorded using Ultrapycnometer 1,000 series (Quantachrome brand) which was nitrogen gas operated system (Jinapong et al., 2008). To determine the true density of spray dried soymilk powder, a large cell (135 cm3) was calibrated with the volume set to 70.699cc using the UltraPyc mode. A 1 g sample was placed inside of an empty cell and multiple purge flow mode (n = 3). At the end, the average volume and density were recorded, along with the standard deviation.
2.5.3 Dispersibility
The dispersibility of the spray-dried powder sample was measured by the Jinapong method with slight modifications (Jinapong et al., 2008). To start with the experiment, 10 mL of distilled water was taken in 50 mL of the beaker at room temperature (25°C) then 1 g of soymilk powder was added to it followed by vigorously stirring for 15–20 s (back and forth movement) using a glass rod. Then reconstituted soymilk powder was passed through the 212 μm sieve (Ogolla et al., 2019). The filtered sieved liquid solution was transferred to the aluminum disc, and it was dried in an oven at 105°C for approximately 1 h (with continuous monitoring until it was thoroughly dried). The dried weight of the soymilk sample was recorded to calculate the %total solid (%TS) content in soymilk (Zungur Bastıoğlu et al., 2016)
Here x indicates the amount of powder taken and y is moisture content (%) of powder sample. Moisture content (%) of spray-dried soymilk powder was determined using a moisture analyzer (METTLER TOLEDO HE53). Approximately 2 g of soymilk powder was uniformly spread on the aluminum disc and then allowed to heat at 105°C for 5 ± 2 min to the moisture meter to display the moisture content present in soymilk powder (Operation instructions Moisture Analyzer HE53, 2023).
2.5.4 Water solubility
To determine the water solubility of the soymilk sample, approximately 1 g of powder sample was dissolved in 10 mL distilled water and vigorously mixed to allow the solubilized soymilk sample to dissolve in the aqueous solution. The dissolved sample was incubated in a water bath at 37°C with continuous stirring and subsequently centrifuged for 10 min at 5,000 rpm. Afterward, the supernatant was dispensed to the pre-weighted aluminum disc and allowed to dry at 105°C in the oven until a constant weight was obtained (Kha et al., 2010).
2.5.5 Wettability
The wettability of spray-dried soymilk samples was determined using the Jinapong method with the same modifications. A glass funnel was placed on a stand to determine wettability, and a 250 mL beaker filled with 100 mL distilled water was kept below the funnel at a distance of 10 cm. Then, with the stopwatch set, 0.1 g of soymilk powder was passed through the funnel, and the wetting time was recorded based on the visual appearance until all of the powder had penetrated the water solution (Jinapong et al., 2008).
2.5.6 Refractive index, pH, and water activity
The pH of reconstituted (5%) soymilk (w/v) solution was tested using Lab® Expert Pro-ISM Metter Toledo TM pH meter. A buffer solution with a pH of 7.00 was used to calibrate the pH meter. The water activity (aw) of spray-dried soymilk powder was evaluated using an AquaLab CX-2 water activity meter at 25°C ± 1°C. The concentration of reconstituted soymilk solution was measured by %brix value using a refractometer with model number HI96800. All the values were recorded in triplicate.
2.5.7 Thermal analysis of spray-dried soymilk powder
The thermal profile of soymilk powder was investigated with a TA Instruments Q20 Differential Scanning Calorimeter (Q20 DSC) connected to a refrigerated chilling system (RCS 90). RCS 90 cools the system to −80°C and stabilizes the DSC system at flange temperature (−70°C). A sample of 9 ± 1 mg soymilk powder was sealed in an aluminum pan and heated at 10°C/min from 30°C to 200°C. To study the change in thermal characteristics of soymilk powder in the thermal scan curve, the flow rate of nitrogen gas was constant at 50 mL/min. The thermogram was studied using Q series software (Q20-0985-DSC Q20) to observe the thermal degradation behavior of soymilk powder.
2.5.8 Rheological behavior of soymilk powder
The rheological behavior of flavored soymilk powder was measured at room temperature (25°C) using an Anton-Paar MCR-302 rheometer with a cone plate measuring system. The diameter of the cone was 50 mm, forming a 1° angle with the surface of the plat. Using a micropipette, 0.75 mL of the soymilk sample was carefully applied to the plat. To measure the viscosity of the soymilk sample with and without flavor, 5% of spray-dried samples were reconstituted with distilled water. All experiments were carried out in triplicate at room temperature (25°C) at shear rates ranging from 0.1 to 100 s-1.
2.5.9 Particle size distribution and zeta potential of soymilk powder
DLS (Dynamic Light Scattering) Delsa Nano Submicron Particle Size and Zeta Potential particle Analyzer, recruited from Beckman Coulter California, United States, was used to determine the particle size distribution and Zeta Potential of soymilk powder. Powder particle was diluted at 5000-folds and particle distribution analysis parameters were viscosity (0.8878 mPa-s), refractive index (1.3328), temperature (25°C ± 1°C), scattering angle (15°), and signal intensity (3,500 ± 500 counts per second (cps). The particle size distribution properties were measured by the instrument approximately 50 replicates, and the graphs and data displayed represent the average value.
2.5.10 Scanning electron microscopy (SEM) of flavored soymilk powder
The SEM imaging of flavored soymilk powder was performed using a Scanning Electron Microscope (FEI Quanta 600F ESEM) in a high vacuum, as described by Singh et al. (2022).
2.6 Crude fat and crude protein content analysis
The crude fat (%) content in HOSM was analyzed by ether extraction (AOAC Official Method 920.39 (A). Crude protein content was measured by using Kjeldahl method (AOAC Official Method 984.13 (A-D), 2006). The analysis was performed at Agricultural Experimental Station Chemical Laboratories at the University of Missouri, Columbia College of Agricultural Food and Natural Resource.
2.7 Experimental design and statistical analysis
The experiment was planned by using a full factorial design using 2*3 model. Here independent variable has two categorical level (HOSM and TLSM), and the other independent variable has three categorical level (control, chocolate, and vanilla). To determine the effect of flavoring components on HOSM and TLSM powder properties, six sets of experiments were performed in triplicate. A total of 6 sets of experiments (triplicate) were performed by setting the inlet temperature (140°C), aspirator (35 m3/h), and feed rate (5 mL/min) are shown in Table 1. In this experimental design, concentration of vanilla and chocolate flavor were kept constant. Before starting the experiment, some pre-trials were carried out by mixing higher concentrations (2% and 4%) of flavors to find the impact of flavoring components on soymilk powder, but due to its sticky behavior lower concentration were decided for final combination (1% Chocolate and 1% Vanilla). One-way analysis of variance (ANOVA) and Tukey’s honestly significant difference test (Tukey’s HSD) was determined by using fit model the significant difference between the mean value of physical, reconstitution, particle, and thermal properties of spray-dried soymilk powder. All the statistical analysis was done using JMP 14.0.0 software (FEAST lab), and all the results were expressed in average ±standard deviation.
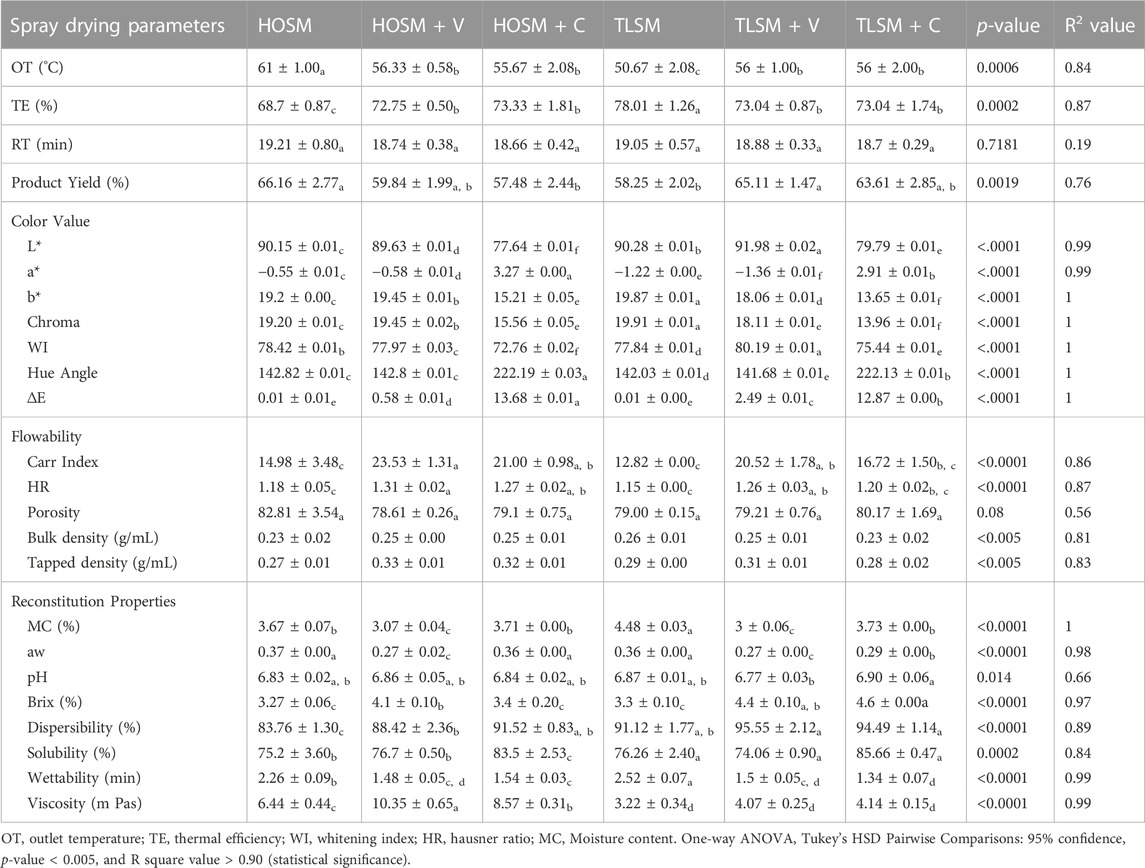
TABLE 1. Effect of change in flavoring components on spray drying parameters and physicochemical properties of a spray-dried soymilk powder.
3 Results and discussion
3.1 Spray drying parameters
Spray drying technology is used to generate specific ingredients with extended shelf life. Spray drying of soymilk with flavoring agent was performed at an inlet temperature (140°C), aspirator (35 m3/h), and feed flow rate (5 mL/min). Physicochemical properties of the soymilk powder depend on spray drying parameters. However, the outlet temperature is the maximum temperature to which the powder is exposed during the drying process, influencing the powder quality. Outlet temperature depends on the amount of heat and mass exchanged during the drying process recorded from the spray drying panel. Outlet temperature increases with increase in inlet temperature, the humidity of drying gas, aspirator, and the viscosity of feed solution and decrease with an increase in feed flow rate and spray gas flow rate (Piñón-Balderrama et al., 2020). Furthermore, outlet temperature increases with the rise in the total solid content in the feed solution and inversely influences the moisture content (%) of the collected powder (Goula and Adamopoulos, 2004); (Özdikicierler et al., 2019). In the current study, an insignificant change in outlet temperature was recorded with the increase in %TSS of the HOSM and TLSM (4.56%). With the addition of flavoring agents in powder form (1% vanilla and 1% chocolate) the %TSS increased to 5.56% in the feed solution. In addition, the rate of heat and mass transfer during the drying process influence the OT which is difficult to precisely monitor throughout the drying process (Patel et al., 2015). Furthermore, the fluctuations in the outlet temperature are influenced by, % Relative Humidity (47%–52%), pressure drop (−70 to −90 mbar) and surrounding atmospheric temperature (23–25°C). However, a slightly higher outlet temperature range was observed for HOSM, which insignificantly decreases with flavor addition. On the other hand, a marginally lower outlet temperature range was recorded for TLSM, which increases with the addition of flavoring agents.
Thermal efficiency is a measuring parameter for spray drying performance and cost-effectiveness of the processing. The thermal efficiency of the process is the amount of energy required to dry the feed solution by evaporating the moisture (Cheng et al., 2018). Increase in outlet temperature and an airflow rate of the spray dryer adversely influences the thermal efficiency (%) of the drying process. The thermal efficiency of the process in the current study varied from 68.70% to 78.01%, as calculated using the reflected outlet temperature, inlet temperature (140°C), and atmospheric temperature (±24°C). The concentration of the feed solution, heat and mass transfer, pressure drop, and surrounding relative humidity influence the thermal efficiency of the process in response to changes in the outlet temperature. Thermal efficiency (%) for HOSM was 68.7 ± 0.87 which slightly improved with the addition of vanilla (72.75 ± 0.50) and chocolate (73.33 ± 1.81) as flavoring components. Higher thermal efficiency was recorded for TLSM (78.01%), which slightly decreases with the increase in viscosity of feed solution with the add-on of flavoring agents (73.04%). On the other hand, a marginally lower thermal efficiency range was observed for HOSM, which increased with the addition of flavoring agents. Statistical evidence shows that p-value <0.005 and R2 value > 0.8 indicate the significant difference in outlet temperature and thermal efficiency during the spray drying process of a different combination of soymilk. The product yield of spray-dried soymilk powder ranged from 57% to 66%; in the case of TLSM powder, product yield improved with a flavoring component (Table 1). However, slightly less powder recovered for HOSM mixed with flavor.
3.2 Color value
The color value is one of the quantitative representations used to determine consumers’ acceptability of food products. The visual appearance of food products strongly influences marketing because any visual defects impact consumer acceptability (Pathare et al., 2013). The color value of food products is influenced by changes in temperature, feed rate, moisture content, and other processing conditions. The color loss indicates microbial contamination and deterioration of the quality of food products (Chang et al., 2018). A slight color change is not visible by unaided eyes, so to avoid this problem, color value L*, a* and b* was recorded using a colorimeter. However, the inlet temperature, feed rate, and airflow rate were kept constant throughout the drying process, but the addition of a flavoring agent substantial impact the color profile of soymilk powder. Complement in soymilk with vanilla flavor does not demonstrate any significant change in color value L*, a*, b*, and Hue angle, but it does improve the whitening index (WI) slightly (Figure 1). Non-etheless, TLSM showed a marginally higher range of Lightness (L*), yellowness (b*), and redness (a*) but a marginally lower WI and Hue angle. However, the addition of chocolate flavor dramatically changed the color value (L*, a*, b*, Chroma, WI, and Hue angle) profile of soymilk powder. Color value L* of soymilk powder decreases (90–77), color value a* increases (−0.55–3.27) (Table:1), and color value b decrease with the addition of chocolate flavor (V. K. Singh et al., 2014). Soymilk powder showed the upper range of Lightness value which was close to the lightness value of camel milk and cheese powder (Koca et al., 2015) (Kalal et al., 2018) and slightly higher than other commercial milk products (Milovanovic et al., 2020).
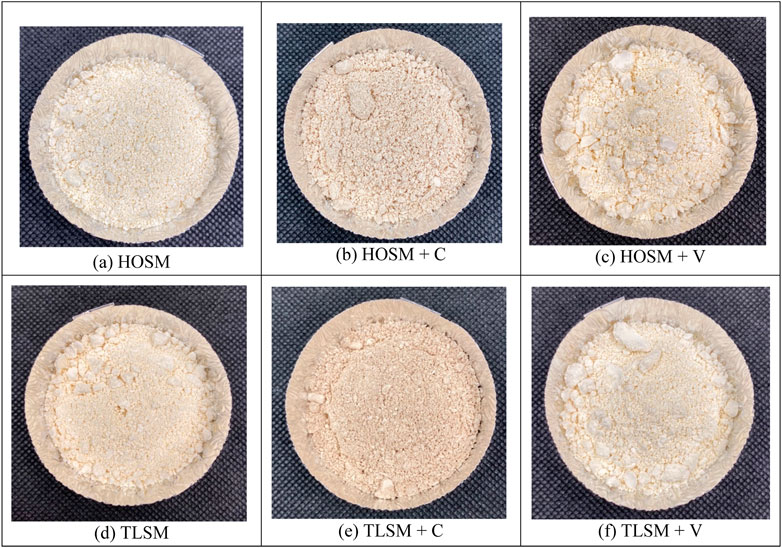
FIGURE 1. Spray dried soymilk powder with and without flavoring components (A) HOSM (B) HOSM + C (C) HOSM + V (D) TLSM (E) TLSM + C and (F) TLSM + V.
Furthermore, the chroma value decreased from 19.2 to 15.6 for HOSM and from 19.9 to 13.4 for TLSM powder, while the whitening index value decreased marginally. Nevertheless, a significant change in hue angle is observed, which changes from 142.8 to 222.2 for HOSM and from 142.7 to 222.1 for TLSM (Table:1) (Pathare et al., 2013). Although based on experimental data and statistical evidence data, it was observed that HOSM and TLSM do not show any significant changes in color profile. R2 value was >0.9 and p-value <0.0001, which indicates the statistical significance of the data and substantial shift in color profile with the addition of flavoring agents (vanilla and chocolate) (Table 1). Moreover, the HOSM added with the vanilla flavor had an ∆E value of 0.58 ± 0.01 that indicated there was no noticeable difference in the color profile, while the HOSM added with the chocolate flavor had an ∆E value of 13.68 ± 0.01 indicating the significant increase in color tending towards brownish like chocolate milk. Similarly, when the TLSM was mixed with vanilla flavor, the ∆E value was 2.49 ± 0.01, and the color difference increased with the addition of chocolate flavor (12.87 ± 0.00) (Figure 1) (Table 1).
3.3 Flowability
Flowability is one of the essential attributes which determine the flow behavior of spray-dried powder. Flowability and cohesiveness of soymilk powder were measured using Carr index (CI) and Hausner ratio (HR) values. The flowability of spray-dried powder is influenced by changes in particle size, particle size distribution, moisture content, hygroscopic nature, interstitial air, and processing temperature (Seth et al., 2017) (Wang et al., 2015). Lower the CI and HR values are an indication of the powder flowability with desirable cohesiveness and vice versa. Calculated CI values for HOSM and TLSM (14.98 & 12.82, respectively) demonstrate the good flowing properties. The addition of vanilla and chocolate flavors to soymilk tends to raise the CI value, which reduces the flowability of soymilk powder. Changing the flowability from good to passable might be due to a change in particle morphology and the surface composition with the addition of a flavoring component. Similarly, the Hauser ratio value rises with the addition of a flavoring ingredient to the soymilk, indicating a decrease in flowability and an increase in the cohesiveness of spray-dried soymilk powder. However, the flowability of soymilk powder undesirably decreased with the addition of flavoring agents. Non-etheless, it falls under the fair to a passable flowing category according to the flowability chart, which seems to be similarly flowable to goat milk powder (Reddy et al., 2014) and has improved flowing properties than instant soymilk powder (Jinapong et al., 2008), milk protein concentrate (McSweeney et al., 2020), spray-dried sauce powder (Wang et al., 2015).
The porosity of HOSM powder slightly decreases with the addition of flavoring component, which changes from 82.81 to 78.61 (vanilla) and 79.10 (chocolate). However, porosity does not significantly change in with flavoring component for TLSM powder 79 to 79.21 (vanilla) and 80.17 (chocolate), which is similar to the behavior observed for the spray dried sugar cane juice powder with maltodextrin as drying aid (Nishad et al., 2017). Greater porosity indicates the higher efficiency of entrapping the air inside the particle, which accounts for the more porous and less dense behavior of spray-dried soy powder (Syll et al., 2013).
3.4 Reconstitution properties: Dispersibility, solubility, and wettability
Dispersibility of soymilk powder is one of the another determining factors in defining consumer acceptability. The dispersibility of spray-dried soymilk powder is influenced by drying factors such as inlet temperature, aspirator, pressure drop and change in relative humidity during spray drying process as well as moisture content, and the hygroscopic nature of spray-dried soymilk powder (Zungur Bastıoğlu et al., 2016) (Ogolla et al., 2019). After adding vanilla and chocolate flavors, the dispersibility of HOSM powder increased from 83.76% to 88.42% (vanilla) and 91.25% (chocolate), respectively. However, TLSM demonstrated a greater range of dispersibility (91.25%), which was further enhanced by the addition of a flavoring agent (95.55%–94.49%). The obtained dispersibility of HOSM and TLSM powder was higher than soy powder (Syll et al., 2013), and quite similar to spray-dried soymilk powder (≥90%), higher than whole milk powder (≥85%) (A. Sharma et al., 2012), and goat milk powder (87.20%) (Reddy et al., 2014). The measured moisture content of HOSM was 3.67% which slightly declined with the addition of vanilla flavor and was nearly identical with chocolate flavor. However, the moisture content of TLSM powder was marginally higher than the HOSM, 4.48%, but it also reduced after adding the flavoring component (vanilla and chocolate). The higher moisture content of soymilk powder could be attributed to its hygroscopic nature of soymilk powder. The obtained P and R2 values for dispersibility were <0.0001 and 0.89, respectively. Similarly, the p-value was <0.0001and the R2 value was 1, indicating the significant difference on moisture content of soymilk with and without flavoring components. That the linear regression model was statistically significant (Table 1).
The solubility of soymilk powder defines its ability to dissolve in the aqueous solution. The solubility of a food powder is directly related to its particle size, moisture content, stickiness, amount of fat, and protein content (Zungur Bastıoğlu et al., 2016). Both HOSM and TLSM powder had similar ranges of solubility (75.2% & 76.26%), which shows the similarity with the solubility of high protein content spray-dried cheese powder (∼36%) (Koca et al., 2015), yogurt powder (36.09%) (Koç et al., 2014) (Santos et al., 2018) and soy protein isolates (Boatright and Hettiarachchy, 1995). This slightly lower solubility of soymilk powder is due to the high protein (∼37–38%) content in soybean (Osthoff et al., 2010) (Tayade and Pardeshi, 2014). The measured crude protein content of the high oleic soybean and spray dried HOSM powder was 38.76% ± 0.3% and 43.54% ± 0.1% respectively (AOAC official method).
The solubility of spray dried soymilk powder is affected by powder composition as well as the alteration in protein structure which could be due to polymerization or denaturation caused by thermal processes, Maillard, or oxidation reactions (Koca et al., 2015) (Sirison et al., 2017) (Niyibituronsa et al., 2018). Based on the above results solubility of soymilk powder was higher than the soy protein isolates (SPI), which was approximately 30%–40% (Sirison et al., 2017). The vanilla flavor does not show any significant impact on solubility. However, chocolate flavor helps to improve the solubility of both HOSM and TLSM powder (88.42% & 85.66%) which was comparable to the solubility of spray-dried sweetened yogurt (Seth et al., 2017), mulberry powder (Fazaeli et al., 2012).
Wettability is another reconstitution property of spray-dried soymilk powder that indicates the dissolution rate. Wettability is affected by various factors, including particle size, density, porosity, and protein content (Koç et al., 2014). In the current study, obtained wetting time of soymilk powder was 2.26 (HOSM) and 2.52 min (TLSM), which shows the similar wettability to goat milk and cow milk powder (Reddy et al., 2014). The wettability of soymilk powder improved with the addition of flavoring component wetting time reduced from >2 min to <1.67 min.
3.5 Water activity (aw), pH, and refractive index
Lowering the water activity and moisture content leads to the higher shelf life of spray-dried soymilk powder. As the water activity increases, a higher amount of free water is available, which is vulnerable to microbial contamination (Fazaeli et al., 2012). The water activity of HOSM and TLSM powders was 0.36 and 0.37, respectively, and decreased with flavoring components (vanilla and chocolate), indicating that the shelf life of soymilk powder improves with the addition of flavoring components. The water activity of soymilk powder with flavoring component was close to spray-dried melon seed powder (Zungur Bastıoğlu et al., 2016), and spray-dried yogurt powder (Seth et al., 2017), skim milk, and whole milk powder (Schuck et al., 2005). Regression analysis data showed a 0.98 R2 value and <0.0001 p-value, indicating the statistically significant of the model. The pH value of freshly prepared soymilk powder was 6.78 ± 0.04, and the pH values of 5% reconstituted HOSM and TLSM powder were 6.83 and 6.87, respectively, which insignificantly changed with the addition flavoring component. Obtained p-value and R2 values were 0.014 and 0.66, respectively, which was reasonable to support the statistical significance. Degree Brix indicates the amount of sugar content present in an aqueous solution. Reconstituted HOSM and TLSM powder (5%) shows 3.27 ± 0.03 %Brix. Adding the flavoring component enhances amplifies its sugar level, increasing the %Brix from 4.10 to 4.6 for vanilla and 3.4 to 4.6 for chocolate flavor (Table 1). The refractive index value indicates how strongly light travels through the aqueous solution, and it directly varies with the amount of dissolved sugar content. It demonstrates the likeness with %Bris, which is statistically significant with <0.0001 p-value and 0.97 R2 value.
3.6 Rheological properties of soymilk
Newtonian fluid properties were observed in reconstituted soymilk samples with and without a flavoring agent. The 5% reconstituted HOSM (6.43 ± 0.44 mPa s) viscosity was higher than that of TLSM 3.22 ± 0.34 mPa s. The viscosity of soymilk was increased with the addition of flavoring components. In the case of HOSM, viscosity increases to 10.35 ± 0.65 mPa s with the vanilla flavor and 8.56 ± 0.31 mPa s with the chocolate flavor. Similar viscosity of TLSM was increased by 4.07 ± 0.2 mPa s with vanilla and 4.14 ± 0.15 mPa s with chocolate flavor (Table 1). Obtained viscosity of whole milk was 2.81 ± 0.36 mPa s, while cow milk had a viscosity of 2.04 mPa s, and camel milk had a viscosity of 1.72 mPa s (Ogolla et al., 2019), which seems close to the viscosity of soymilk 6.62 mPa s (Sethi et al., 2016). The high viscous nature of reconstituted HOSM could be due to the smaller particle size and high-fat content spray-dried soymilk powder (23.44% ± 0.58%) measured by (AOAC Official method). A similar value of fatty acid content (22%–24%) was found in spray dried soymilk powder of other varieties of soybean (Liang and Hartel, 2004) (Osthoff et al., 2010) (Fujii, 2017). Based on the study performed by Liang it was observed that free fat present in the milk sample interacted with the chocolate powder which led to the decrease in viscosity of the sample. (Liang and Hartel, 2004). In the current study, we observed a slight increase in viscosity with the addition of chocolate powder which might be due to the composition of the chocolate powder used. However, the chocolate flavor used in the current study contained some amount of sugar mixed with a trace of another flavoring agent, indicating an upsurge in the viscous nature of soymilk. Similarly, HOSM (5%) reconstituted with water showed higher viscosity than TLSM with similar concentrations. The addition of chocolate and vanilla flavors increases the viscosity of reconstituted soymilk, which could be due to its dextrose content. However, the addition of vanilla flavor showed a slightly more viscous nature than the chocolate flavor. Obtained p-value and R2 value for the viscosity of soymilk samples with and without flavoring component was <0.0001 & 0.99, respectively.
3.7 Particle size distribution and zeta potential of HOSM and TLSM soymilk powder
The maximum distribution of the particle recorded at average particle size range 2.528 ± 4.11 μm for HOSM. However, with the addition of flavoring components such as chocolate (1.387 ± 0.6 μm), and vanilla (0.687 ± 0.36 μm) high particle distribution was observed at a slightly smaller average particle size range. Particle size distribution was measured at refractive index value 1.3328 (nD), viscosity 0.8878 (cp) of 5000-fold diluted soymilk powder. Particle size distribution were calculated using D10, D50 and D90, indicating a 10% and 90% smaller particle diameter range (McSweeney et al., 2020). The polydispersibility Index (PDI) measures the homogeneity of the particle (Kanojia et al., 2016) was 1.053 and 3.55, for the HOSM and TLSM respectively, indicating the comparatively broader range of particle size distribution for TLSM (Table 2). However, the addition of flavoring components slightly improves the size uniformity of the powder particles, but the PDI value still indicates the broader range of particle size. The maximum distribution of particles for TLSM was recorded at an average particle size of 4.148 ± 6.70 μm which decreases with the addition of flavoring components like chocolate (2.9 ± 5.1 μm) and vanilla (2.56 ± 2.94 μm). Other particle properties such as average hydrodynamic diameter, span, and PDI decrease with the addition of flavoring components in TLSM (Table 2). The addition of chocolate slightly increases the particle homogeneity but still indicates the broader range of PDI value (2.917), but it drastically improves with the addition of vanilla flavor (0.501). Zeta potential, which reflects the sonic strength of powder particles in an aqueous solution, was measured at −26.85 mV for HOSM and −35.39 mV for TLSM, which indicates the moderately stabilized range of soy powder in an aqueous solution (Rao et al., 2011). The value of zeta potential increases with the addition of chocolate flavor, which represents the high stability of the particle in an aqueous solution. However, the addition of vanilla flavor does not show any significant effect on HOSM (−13.53 mV), but its stability decreases with the interaction with TLSM (−46.36 mV). The particle size of HOSM and TLSM showed similarity with the soymilk prepared from the commercially available variety of the soybean (Sivanandan et al., 2010).
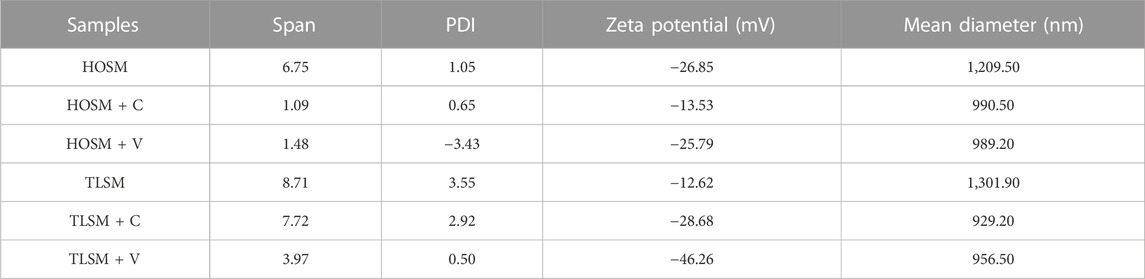
TABLE 2. Particle size distribution and Zeta potential of TLSM and HOSM powder with flavoring components.
3.8 differential scanning calorimetry (DSC)
Thermal degradation behavior and glass transition temperature of the functional component of HOSM and TLSM were determined using DSC. The endothermic peak for HOSM has obtained at 82.60°C ± 7.07°C and for TLSM at 78.73°C ± 6.78°C temperature. This slightly higher degradation peak for HOSM showed its thermal stability, the reason might be its higher content of oleic acid and temperature tolerance (P. Singh et al., 2022). However, the thermal stability of the soymilk powder was improved with the addition of flavoring components. After the addition of flavoring components such as chocolate and vanilla degradation peak was shifted to ≥15 °C. This degradation peak was obtained to be similar to the first peak of why protein maltodextrin (Agustinisari et al., 2020), camel milk (Ogolla et al., 2019) and moderately higher than soy protein isolated, soymilk (Renkema et al., 2000) (Z.-S. Liu et al., 2004). Spray-dried soymilk powder was performed at a temperature range of 30–180°C, and the experiment duration was 18 ± 2 min. Glass transition temperature (Tg) for HOSM and TLSM were 54.18 ± 7.33°C and 56.86°C ± 6.01°C respectively, with no significant difference. Glass transition temperature of soymilk powder increases with the addition of flavoring components. Furthermore, specific heat capacity (Cp) which denotes the heat required to raise the temperature 1°C, was approximately 2.01 ± 0.09 (J/g °C) for HOSM and 2.10 ± 0.55 (J/g °C) for TLSM. From the thermal analysis data, it was observed that there was less heat required to degrade the soymilk in combination with flavoring components. Similarly, the heat flow during thermal degradation was 0.78 ± 0.22 for HOSM and 0.31 ± .12 TLSM (Figures 2A, B).
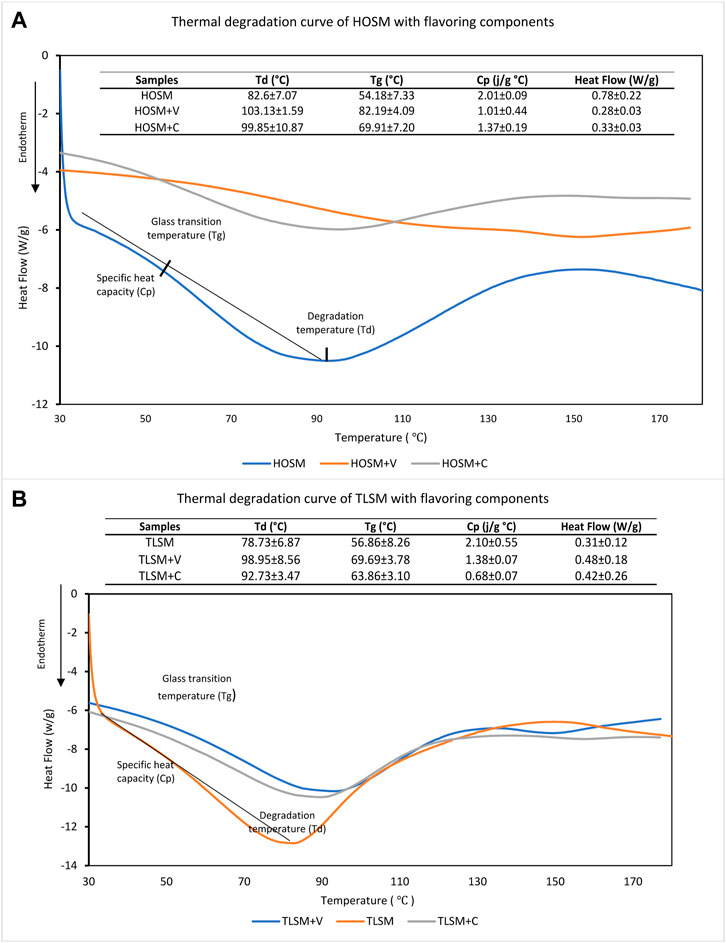
FIGURE 2. Thermal degradation curve for soymilk (A) HOSM (B) TLSM powder with the flavoring components.
3.9 Scanning electron microscopy imaging of soymilk powder
The microstructure of spray-dried soymilk powder was demonstrated using scanning electron microscopy. The SEM imaging of spray-dried soymilk powder in interaction with flavoring components was performed at magnifications of ×1,000, ×5,000, and ×150,00. Image analysis was performed by using ImageJ which is an open-source software developed by the National Institutes of Health (NIH) (Kumari and Rana, 2015). The ImageJ was used to evaluate the area, minimum, maximum, and average length of the powder particles by analyzing the 100–120 random-size particles (Party et al., 2021). The particle size of HOSM powder was 2.65 ± 1.87 μm, which does not significantly change with the addition of flavoring components vanilla (2.66 ± 2.15 μm) and chocolate (2.46 ± 1.24 μm). A similar behavior was observed for the TLSM but the size of the TLSM powder particle was marginally smaller, 2.30 ± 1.57 μm, as compared to HOSM. The SEM imaging with lower magnification (×1,000) represents the slight fusion and agglomeration behavior of spray-dried soymilk powder. TLSM and HOSM powder marginally sticked with each other; non-etheless, after adding the flavoring components, particles were dispersed but still smaller particle was clustered with each other (Figures 3 A, D, G) and (Figures 4 A, D, G). At ×5,000 magnification, the power particle appears as a slightly wrinkled sphere with concavity on the surface (Figure three a, d and g). At ×5,000 magnification, soymilk powder particles appear almost spherical with slight concavity on the surface (Figures 3 B, E, H). The addition of vanilla flavor marginally improved the morphology of the powder particle (Figures 3 and 4 E, H). Furthermore, powder particles appear substantially spherical in combination with chocolate flavor.
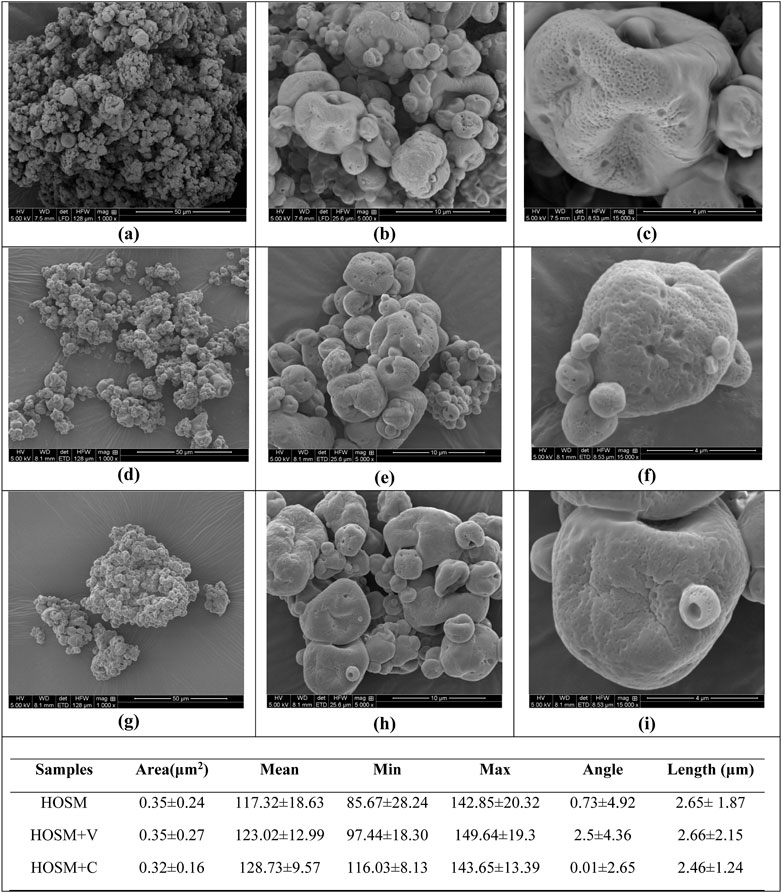
FIGURE 3. SEM images of spray dried HOSM powder at magnification (A) ×1,000 (B) ×5,000 (C) ×15,000 and flavoring components such as vanilla (D–F) and chocolate (G–I) at the same magnification.
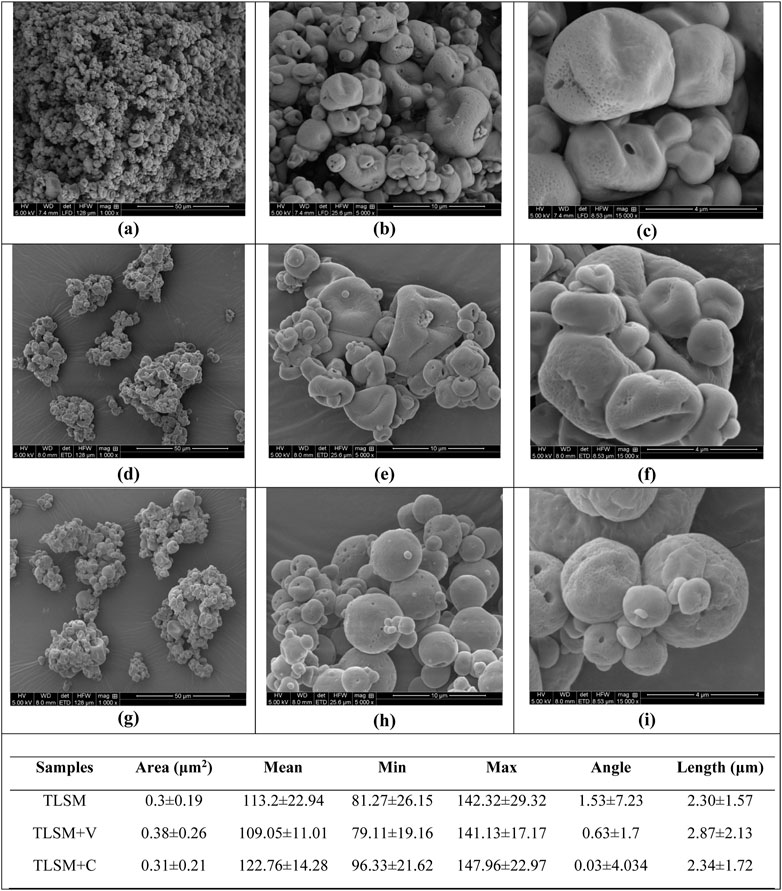
FIGURE 4. SEM images of spray dried TLSM powder at magnification (A) ×1,000 (B) ×5,000 (C) ×15,000 and flavoring components such as vanilla (D–F) and chocolate (G–I) at the same magnification.
The spherical nature of powder particles is affected by changes in oil content, as oil content decreases, particle sphericity increases (Erbay and Koca, 2015). There was no significant difference was recorded in either variety of soymilk powder, however HOSM appears slightly less sticky and wrinkled. In the case of TLSM powder, smaller particles are grooved into larger powder particles. The stickiness of the powder particles improved with the addition of chocolate flavor; however, the vanilla flavor does not show any significant change in the particle morphology. Spray drying process takes place in the different stages; In the initial stage of drying surface of the droplet saturated with the solvent and drying takes place by diffusion of solvent from the outer surface of droplet. In the following stage, the surface water level falls, and there is no longer enough moisture to keep the droplet surface saturated (Ravichandran and Krishnaswamy, 2021) causing the formation of dried shell on the surface.
The rate of evaporation decreases while the thickness of the shell increases. Particle size and morphology are directly influenced by the rate of evaporation and drying kinetics. So, the slight concavity of the surface is due to the high rate of evaporation and heat and mass transfer in the drying chamber during the spray drying process (Stevanović, 2017) (W. Liu et al., 2010) (Zbicinski et al., 2022). The shrinkage on the surface of the spherical particle might be due to the relatively low concentration of total solid in the feed solution. A slight increase in the concentration of feed solution improves the particle sphericity by forming a thicker shell on the surface of the particle (Lourenço et al., 2020) (Ousset et al., 2018). At 150,00x morphology of spray-dried soymilk powder particles appears primarily smooth with some coalescence. (Figures 3 and 4 C, F). Scanning electron microscopic images of soymilk powder attribute the similar quality to spray-dried powder particles such as cheese powder (Koca et al., 2015), spray-dried solid dispersion (Ousset et al., 2018), soymilk powder (Osthoff et al., 2010).
3.10 Statistical analysis of the data
Statistical analysis data for analyzing the effect of flavoring components on both HOSM and TLSM. The fit model data for effect test and summary of fit are represented in Tables 3, 4. Based on the analysis of the variance (ANOVA), and Tukey’s HSD data statistically significant p-value (<0.005), R square (>0.90), and F ratio (>1). The sum of the square values (model, error, and corrected total), root mean square error (RMSE) and mean of response (MOR).
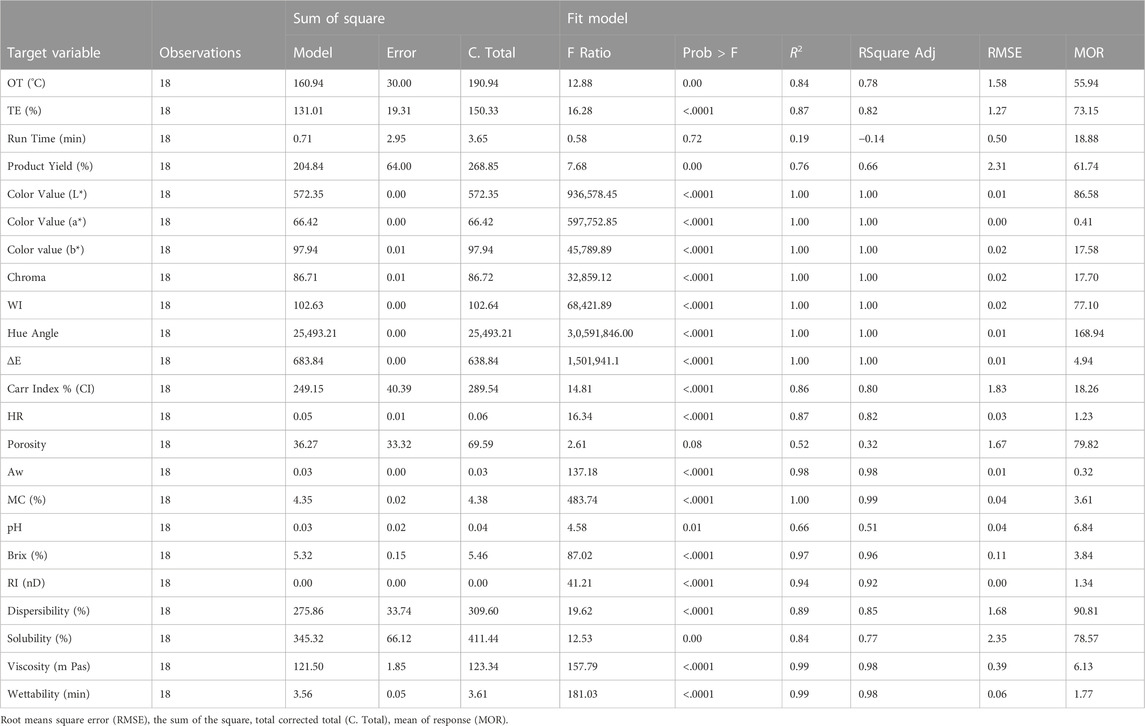
TABLE 3. Analysis of variance (ANOVA) table for full factorial design showing factors and their effect on response.
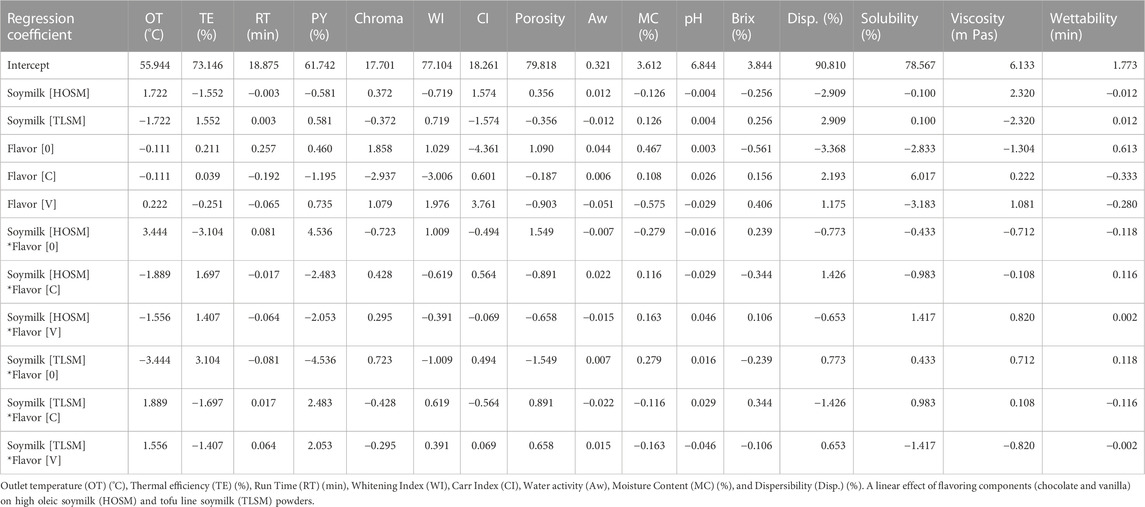
TABLE 4. Regression equation coefficients for spray drying response parameters of soymilk powder with flavoring components.
Were analyzed to find the statistical significance of the experimental model. Most of the variable’s data show the statistically significant with >0.005 p-values, <0.90 R square values, >1 F value, and less root means square error and desirable mean values (Table 3 and Table 4). However, the run time of the spray dryer, the porosity of the powder, and the H value of the reconstituted soymilk represent no significant change observed with the addition of flavoring components.
4 Conclusion
The experimental investigation of the effect of flavoring components on spray-dried soymilk powder was analyzed. Soymilk powder exhibits beany flavor, influencing the consumer acceptability of plant-based milk powder. Adding flavoring components to the soymilk could help improve the flavor profile of soymilk powder. In the current study, vanilla and chocolate flavor were added to the soymilk solution and passed through the spray dryer. The drying process was performed to study the effect of physicochemical properties of soymilk powder in combination with flavoring components. Spray drying parameters like outlet temperature ranged from 50 to 61°C and thermal efficiency 68%–78% throughout the drying process. According to the experimental data, the thermal efficiency and outlet temperature of the drying process slightly differed between the two soybean varieties (TL and HO). However, the addition of flavoring components mildly improves the thermal efficiency of the process by decreasing the outlet temperature. Furthermore, there was no significant amount of change recorded for the run time of the process with and without flavoring components. Physical properties of the soymilk powder, such as flowability, decrease with the addition of flavoring components, and drastic changes in color profile were recorded with chocolate flavor addition. A slight decrease in moisture content (4.48%–3%) and water activity (0.37–0.27) was recorded for both HOSM and TLSM with the flavoring components. Reconstitution powder parameters of HOSM and TLSM, such as dispersibility (83.76–95.55), solubility (74.06%–85.66%), %brix (3.27–4.6), were improved with the addition of flavoring components. There was a higher range of viscosity recorded for reconstituted (5%) TLSM (6.44–10.35 m Pas) as compared to HOSM (3.22–4.14 m Pas). However, in both cases, the viscosity increased with the addition of flavoring component. Obtained particle size was in microscopic range (≤10 μm), and the polydispersibility index (PDI) was 1.053–3.55, which indicate the polydisperse nature of particles in both varieties of soymilk powder. However, the PDI values decrease with the addition of flavoring components, indicating that particle size uniformity improves with the addition of flavoring components. The highest peak of thermal degradation was recorded at 78.73°C for TLSM and 82.60°C for HOSM, which became more stable with the addition of flavoring components by shifting degradation peak ≥15°C. Furthermore, there was no significant difference in the morphological properties observed in HOSM and TLSM powder. However, particle sphericity was highly improved with the chocolate flavor, but there was no significant impact was seen on the particle structure with the addition of vanilla flavor.
4.1 Future market potential of the study
The plant-based milk market is exponentially growing due to its tremendous health benefits, ethical concern, and sustainable food system. The current value of the plant-based milk market is USD 13.24 billion, which is expected to reach USD 30.79 billion (2031) (Plant-Based Milk Market, 2021). The addition of flavoring components to soymilk powder could improve its physicochemical properties and extend its shelf life by preventing it from being impacted by changes in environmental conditions. Plant-based milk powder is an important alternative to animal-based milk for creating a sustainable food system. Developed soymilk powder could be directly used for human consumption or to create reconstituted soy-based beverages. It could be an excellent lactose-intolerance diet supplement and a viable substitute for animal-based milk powder. Sensory evaluation of soy-based products prepared from flavored soymilk will be performed in the future prospects of the study. Even though, the plant-based milk has several health benefits, the major disadvantage are the beany and malty flavors impacting the taste profile. The food industry trend shows an increase in demand for flavor-enhanced plant-based milks to match the physicochemical characteristics and taste preferences of dairy-based milk. The study for flavor enhancement of plant-based milk opens the opportunities to improving the flavor profile of plant-based milk as well as studying the effect of flavoring components on the physicochemical properties of powdered milk. Future recommendations could address the gaps in identifying new and natural ingredients as flavoring agents that does not influence the viscosity of the plant-based milk; design equipment/techniques for flavor infusion to improve the thermal stability and for enhancing flavor, overall improving the sustainability, and contributing to the plant based healthy food sector. Developed formulation might be used to make alternative plant based infant foods, smoothies, baked goods, pudding, whipped topping, coffee creamer, and other food products.
Data availability statement
The original contributions presented in the study are included in the article/supplementary material, further inquiries can be directed to the corresponding author.
Author contributions
PS: Experimental design, spray drying, physicochemical characterization, data analysis, writing the original draft. KK: Project administration, conceptualization, funding acquisition, supervision, reviewing, and editing. All authors contributed to the article and approved the submitted version.
Funding
Funding for this project comes from MSMC Project No. MSMC00065151.
Acknowledgments
Special thanks to Missouri Soybean Merchandising Council (MSMC) for funding this project.
Conflict of interest
The authors declare that the research was conducted in the absence of any commercial or financial relationships that could be construed as a potential conflict of interest.
Publisher’s note
All claims expressed in this article are solely those of the authors and do not necessarily represent those of their affiliated organizations, or those of the publisher, the editors and the reviewers. Any product that may be evaluated in this article, or claim that may be made by its manufacturer, is not guaranteed or endorsed by the publisher.
References
Agustinisari, I., Mulia, K., and Nasikin, M. (2020). The effect of eugenol and chitosan concentration on the encapsulation of eugenol using whey protein–maltodextrin conjugates. Appl. Sci. 10 (9), 3205. Article 9. doi:10.3390/app10093205
Arpagaus, C., and Schwartzbach, H. (2008). Scale-up from bench-top research to laboratory production. Scale-up from the Büchi Mini Spray Dryer B-290 to the Niro MOBILE MINORTM. Available at: https://www.bcluae.com/upload/product/B-290_Scale-up_B-290_Niro_MOBILE_MINOR_en_01.pdf (Retrieved March 17, 2022).
Assadpour, E., and Jafari, S. M. (2019). Advances in spray-drying encapsulation of food bioactive ingredients: From microcapsules to nanocapsules. Annu. Rev. Food Sci. Technol. 10 (1), 103–131. doi:10.1146/annurev-food-032818-121641
B-290 operation Manual (2020). Technical data overview and materials used, B-290 mini spray dryer operation manual. Available at: https://assets.buchi.com/image/upload/v1605800030/pdf/Operation-Manuals/OM_093001_B-290_en.pdf (Retrieved June 7, 2022). 20-23
Boatright, W. l., and Hettiarachchy, N. s. (1995). Spray-dried soy protein isolate solubility, gelling characteristics, and extractable protein as affected by antioxidants. J. Food Sci. 60 (4), 806–809. doi:10.1111/j.1365-2621.1995.tb06234.x
Chang, L. S., Karim, R., Abdulkarim, S. M., Yusof, Y. A., and Ghazali, H. M. (2018). Storage stability, color kinetics and morphology of spray-dried soursop (Annona muricata L.) powder: Effect of anticaking agents. Int. J. Food Prop. 21 (1), 1937–1954. doi:10.1080/10942912.2018.1510836
Cheng, F., Zhou, X., and Liu, Y. (2018). Methods for improvement of the thermal efficiency during spray drying. E3S Web Conf. 53, 01031. doi:10.1051/e3sconf/20185301031
Erbay, Z., and Koca, N. (2015). Effects of whey or maltodextrin addition during production on physical quality of white cheese powder during storage. J. Dairy Sci. 98 (12), 8391–8404. doi:10.3168/jds.2015-9765
Fazaeli, M., Emam-Djomeh, Z., Kalbasi Ashtari, A., and Omid, M. (2012). Effect of spray drying conditions and feed composition on the physical properties of black mulberry juice powder. Food Bioprod. Process. 90 (4), 667–675. doi:10.1016/j.fbp.2012.04.006
Fujii, T. (2017). Coagulation and rheological behaviors of soy milk colloidal dispersions. Biosci. Biotechnol. Biochem. 81 (4), 680–686. doi:10.1080/09168451.2017.1282810
Goula, A. M., and Adamopoulos, K. G. (2004). Spray drying of tomato pulp: Effect of feed concentration. Dry. Technol. 22 (10), 2309–2330. doi:10.1081/DRT-200040007
High Oleic Soybeans (2022). United soybean board. Available at https://www.unitedsoybean.org/high-oleic-soybeans/ (Retrieved November 30, 2022).
Isanga, J., and Zhang, G.-N. (2008). Soybean bioactive components and their implications to health—a review. Food Rev. Int. 24 (2), 252–276. doi:10.1080/87559120801926351
Jinapong, N., Suphantharika, M., and Jamnong, P. (2008). Production of instant soymilk powders by ultrafiltration, spray drying and fluidized bed agglomeration. J. Food Eng. 84 (2), 194–205. doi:10.1016/j.jfoodeng.2007.04.032
Kalal, A., Hiregoudar, S., Nidoni, U., C T, R., and Naik, N. (2018). Effect of spray drying and drying aids on bitter gourd juice powder properties. Agric. Res. J. 55, 318. doi:10.5958/2395-146X.2018.00057.1
Kanojia, G., Willems, G.-J., Frijlink, H. W., Kersten, G. F. A., Soema, P. C., and Amorij, J.-P. (2016). A Design of Experiment approach to predict product and process parameters for a spray dried influenza vaccine. Int. J. Pharm. 511 (2), 1098–1111. doi:10.1016/j.ijpharm.2016.08.022
Kha, T. C., Nguyen, M. H., and Roach, P. D. (2010). Effects of spray drying conditions on the physicochemical and antioxidant properties of the Gac (Momordica cochinchinensis) fruit aril powder. J. Food Eng. 98 (3), 385–392. doi:10.1016/j.jfoodeng.2010.01.016
Koç, B., Sakin-Yılmazer, M., Kaymak-Ertekin, F., and Balkır, P. (2014). Physical properties of yoghurt powder produced by spray drying. J. Food Sci. Technol. 51 (7), 1377–1383. doi:10.1007/s13197-012-0653-8
Koca, N., Erbay, Z., and Kaymak-Ertekin, F. (2015). Effects of spray-drying conditions on the chemical, physical, and sensory properties of cheese powder. J. Dairy Sci. 98 (5), 2934–2943. doi:10.3168/jds.2014-9111
Kumari, R., and Rana, N. (2015). Particle size and shape analysis using imagej with customized tools for segmentation of particles. doi:10.17577/ijertv4is110211
Liang, B., and Hartel, R. W. (2004). Effects of milk powders in milk chocolate. J. Dairy Sci. 87 (1), 20–31. doi:10.3168/jds.S0022-0302(04)73137-9
Liu, W., Wu, W. D., Selomulya, C., and Chen, X. D. (2010). Uniform chitosan microparticles prepared by a novel spray-drying technique. Int. J. Chem. Eng. 2011, 1–7. doi:10.1155/2011/267218
Liu, Z.-S., Chang, S. K. C., Li, L.-T., and Tatsumi, E. (2004). Effect of selective thermal denaturation of soybean proteins on soymilk viscosity and tofu’s physical properties. Food Res. Int. 37 (8), 815–822. doi:10.1016/j.foodres.2004.04.004
Lourenço, S. C., Moldão-Martins, M., and Alves, V. D. (2020). Microencapsulation of pineapple peel extract by spray drying using maltodextrin, inulin, and Arabic gum as wall matrices. Foods 9 (6), 718. Article 6. doi:10.3390/foods9060718
McSweeney, D. J., Maidannyk, V., Montgomery, S., O’Mahony, J. A., and McCarthy, N. A. (2020). The influence of composition and manufacturing approach on the physical and rehydration properties of milk protein concentrate powders. Foods 9 (2), 236. doi:10.3390/foods9020236
Milovanovic, B., Djekic, I., Miocinovic, J., Djordjevic, V., Lorenzo, J. M., Barba, F. J., et al. (2020). What is the color of milk and dairy products and how is it measured? Foods 9 (11), 1629. Article 11. doi:10.3390/foods9111629
Nekkanti, V., Muniyappan, T., Karatgi, P., Hari, M. S., Marella, S., and Pillai, R. (2009). Spray-drying process optimization for manufacture of drug–cyclodextrin complex powder using design of experiments. Drug Dev. Industrial Pharm. 35 (10), 1219–1229. doi:10.1080/03639040902882264
Nishad, J., Selvan, C. J., Mir, S. A., and Bosco, S. J. D. (2017). Effect of spray drying on physical properties of sugarcane juice powder (Saccharum officinarum L.). J. Food Sci. Technol. 54 (3), 687–697. doi:10.1007/s13197-017-2507-x
Niyibituronsa, M., Onyango, A. N., Gaidashova, S., Imathiu, S., Uwizerwa, M., Ochieng, E. P., et al. (2018). The effect of different processing methods on nutrient and isoflavone content of soymilk obtained from six varieties of soybean grown in Rwanda. Food Sci. Nutr. 7 (2), 457–464. doi:10.1002/fsn3.812
Ogolla, J. A., Kulig, B., Bădulescu, L., Okoth, M. W., Esper, G., Breitenbach, J., et al. (2019). Influence of inlet drying air temperature and milk flow rate on the physical, optical and thermal properties of spray-dried camel milk powders. Food Bioprocess Technol. 12 (5), 751–768. doi:10.1007/s11947-019-2243-5
Operating Instructions Moisture Analyzer HE53 (2023). Available at: https://pim-resources.coleparmer.com/instruction-manual/mettler-he53-moisture-balance-im.pdf (Retrieved January 28, 2023). 52
Osthoff, G., Hugo, A., van Wyk, P., de Wit, M., and Meyer, S. (2010). Characterization of a spray-dried soymilk powder and changes observed during storage. Food Sci. Technol. Int. 16 (2), 169–178. doi:10.1177/1082013209353236
Ousset, A., Meeus, J., Robin, F., Schubert, M. A., Somville, P., and Dodou, K. (2018). Comparison of a novel miniaturized screening device with büchi B290 mini spray-dryer for the development of spray-dried solid dispersions (SDSDs). Processes 6 (8), 129. Article 8. doi:10.3390/pr6080129
Özdikicierler, O., Dirim, N., and Pazır, F. (2019). Modeling and optimization of the spray drying parameters for soapwort (Gypsophila Sp.) extract. Food Sci. Biotechnol. 28 (5), 1409–1419. doi:10.1007/s10068-019-00573-0
Party, P., Bartos, C., Farkas, Á., Szabó-Révész, P., and Ambrus, R. (2021). Formulation and in vitro and in silico characterization of “nano-in-micro” dry powder inhalers containing meloxicam. Pharmaceutics 13 (2), 211. doi:10.3390/pharmaceutics13020211
Patel, B. B., Patel, J. K., Chakraborty, S., and Shukla, D. (2015). Revealing facts behind spray dried solid dispersion technology used for solubility enhancement. Saudi Pharm. J. 23 (4), 352–365. doi:10.1016/j.jsps.2013.12.013
Pathare, P. B., Opara, U. L., and Al-Said, F. A.-J. (2013). Colour measurement and analysis in fresh and processed foods: A review. Food Bioprocess Technol. 6 (1), 36–60. doi:10.1007/s11947-012-0867-9
Piccinin, E., Cariello, M., De Santis, S., Ducheix, S., Sabbà, C., Ntambi, J. M., et al. (2019). Role of oleic acid in the gut-liver Axis: From diet to the regulation of its synthesis via stearoyl-CoA desaturase 1 (SCD1). Nutrients 11 (10), 2283. doi:10.3390/nu11102283
Piñón-Balderrama, C. I., Leyva-Porras, C., Terán-Figueroa, Y., Espinosa-Solís, V., Álvarez-Salas, C., and Saavedra-Leos, M. Z. (2020). Encapsulation of active ingredients in food industry by spray-drying and Nano spray-drying technologies. Processes 8 (8), 889. Article 8. doi:10.3390/pr8080889
Plant-based Milk Market (2022). Available at: https://www.futuremarketinsights.com/reports/plant-based-milk-market (Retrieved March 17, 2022).
Raffin, R. P., Jornada, D. S., Ré, M. I., Pohlmann, A. R., and Guterres, S. S. (2006). Sodium pantoprazole-loaded enteric microparticles prepared by spray drying: Effect of the scale of production and process validation. Int. J. Pharm. 324 (1), 10–18. doi:10.1016/j.ijpharm.2006.06.045
Rao, S., Song, Y., Peddie, F., and Evans, A. M. (2011). Particle size reduction to the nanometer range: A promising approach to improve buccal absorption of poorly water-soluble drugs. Int. J. Nanomedicine 6, 1245–1251. doi:10.2147/IJN.S19151
Ravichandran, K. S., and Krishnaswamy, K. (2021). Sustainable food processing of selected North American native berries to support agroforestry. Crit. Rev. Food Sci. Nutr. 0 (0), 1–26. doi:10.1080/10408398.2021.1999901
Reddy, R. S., Ramachandra, C. T., Hiregoudar, S., Nidoni, U., Ram, J., and Kammar, M. (2014). Influence of processing conditions on functional and reconstitution properties of milk powder made from Osmanabadi goat milk by spray drying. Small Ruminant Res. 119 (1), 130–137. doi:10.1016/j.smallrumres.2014.01.013
Renkema, J. M. S., Lakemond, C. M. M., de Jongh, H. H. J., Gruppen, H., and van Vliet, T. (2000). The effect of pH on heat denaturation and gel forming properties of soy proteins. J. Biotechnol. 79 (3), 223–230. doi:10.1016/S0168-1656(00)00239-X
Rizzo, G., and Baroni, L. (2018). Soy, soy foods and their role in vegetarian diets. Nutrients 10 (1), 43. doi:10.3390/nu10010043
Santos, G., Maurício, A. C., Sencadas, V., Santos, J. D., Fernandes, M. H., and Gomes, P. S. (2017). Spray drying: an overview. Biomaterials-Physics and Chemistry-New Edition. doi:10.5772/intechopen.72247
Santos, G. dos, Nogueira, R. I., and Rosenthal, A. (2018). Powdered yoghurt produced by spray drying and freeze drying: A review. Braz. J. Food Technol. 21. doi:10.1590/1981-6723.12716
Schuck, P., Méjean, S., Dolivet, A., and Jeantet, R. (2005). Thermohygrometric sensor: A tool for optimizing the spray drying process. Innovative Food Sci. Emerg. Technol. 6 (1), 45–50. Scopus. doi:10.1016/j.ifset.2004.10.001
Seth, D., Mishra, H. N., and Deka, S. C. (2017). Functional and reconstitution properties of spray-dried sweetened yogurt powder as influenced by processing conditions. Int. J. Food Prop. 20 (7), 1603–1611. doi:10.1080/10942912.2016.1214965
Sethi, S., Tyagi, S. K., and Anurag, R. K. (2016). Plant-based milk alternatives an emerging segment of functional beverages: A review. J. Food Sci. Technol. 53 (9), 3408–3423. doi:10.1007/s13197-016-2328-3
Sharma, A., Jana, A. H., and Chavan, R. S. (2012). Functionality of milk powders and milk-based powders for end use applications—a review. Compr. Rev. Food Sci. Food Saf. 11 (5), 518–528. doi:10.1111/j.1541-4337.2012.00199.x
Sharma, S., Kaur, M., Goyal, R., and Gill, B. S. (2014). Physical characteristics and nutritional composition of some new soybean (Glycine max (L.) Merrill) genotypes. J. Food Sci. Technol. 51 (3), 551–557. doi:10.1007/s13197-011-0517-7
Singh, P., Bilyeu, L., and Krishnaswamy, K. (2022). Improving process sustainability by optimizing spray drying parameters: High oleic soymilk using response surface methodology. Food Bioprocess Technol. 15, 833–851. doi:10.1007/s11947-021-02726-8
Singh, P., Bilyeu, L., and Krishnaswamy, K. (2022). Spray drying process optimization: Drought resistant variety (W82) soymilk powder using response surface methodology (RSM). LWT, 113760. doi:10.1016/j.lwt.2022.113760
Singh, S., and Dixit, D. (2014). A review on spray drying: Emerging technology in food industry. 4, 8.
Singh, V. K., Pandey, S., Pare, A., and Singh, R. B. (2014). Optimization of process parameters for the production of spray dried Ber (Ziziphus jujube L.) powder. J. Food Sci. Technol. 51 (12), 3956–3962. doi:10.1007/s13197-012-0897-3
Sirison, J., Matsumiya, K., Samoto, M., Hidaka, H., Kouno, M., and Matsumura, Y. (2017). Solubility of soy lipophilic proteins: Comparison with other soy protein fractions. Biosci. Biotechnol. Biochem. 81 (4), 790–802. doi:10.1080/09168451.2017.1282808
Sivanandan, L., Toledo, R. T., and Singh, R. K. (2010). Rheological and ultrastructural properties and particle size distribution of soymilk as affected by processing methods. Int. J. Food Prop. 13 (3), 580–598. doi:10.1080/10942910902716943
Sosnik, A., and Seremeta, K. P. (2015). Advantages and challenges of the spray-drying technology for the production of pure drug particles and drug-loaded polymeric carriers. Adv. Colloid Interface Sci. 223, 40–54. doi:10.1016/j.cis.2015.05.003
Stevanović, M. (2017). “Chapter 11—polymeric micro- and nanoparticles for controlled and targeted drug delivery,” in Nanostructures for drug delivery. Editors E. Andronescu, and A. M. Grumezescu (Elsevier), 355–378. doi:10.1016/B978-0-323-46143-6.00011-7
Syll, O., Khalloufi, S., and Schuck, P. (2013). Dispersibility and morphology of spray-dried soy powders depending on the spraying system. Dairy Sci. Technol. 93 (4), 431–442. doi:10.1007/s13594-013-0112-y
Taha, A. Y. (2020). Linoleic acid–good or bad for the brain? Npj Sci. Food 4 (1), 1. doi:10.1038/s41538-019-0061-9
Tayade, P. T., and Pardeshi, I. L. (2014). Comparative investigation on spray dried powder from soymilk and sprouted soybean milk. Int. J. Agric. Eng. 7 (2), 410–416. doi:10.15740/has/ijae/7.2/410-416
USDA ARS (2022). Online magazine. 45, 11, Available at: https://agresearchmag.ars.usda.gov/1997/nov/tofu/ (Retrieved June 6, 2022).
Wang, W., Dufour, C., and Zhou, W. (2015). Impacts of spray-drying conditions on the physicochemical properties of soy sauce powders using maltodextrin as auxiliary drying carrier. CyTA - J. Food 13 (4), 1–8. doi:10.1080/19476337.2015.1014430
Yang, A., Smyth, H., Chaliha, M., and James, A. (2015). Sensory quality of soymilk and tofu from soybeans lacking lipoxygenases. Food Sci. Nutr. 4 (2), 207–215. doi:10.1002/fsn3.274
Yu, H., Liu, R., Hu, Y., and Xu, B. (2017). Flavor profiles of soymilk processed with four different processing technologies and 26 soybean cultivars grown in China. Int. J. Food Prop. 20 (3), S2887–S2898. doi:10.1080/10942912.2017.1382507
Zbicinski, I., Ciesielski, K., and Ge, B. (2022). Mechanism of particle agglomeration for single and multi-nozzle atomization in spray drying: A review. Processes 10 (4), 727. Article 4. doi:10.3390/pr10040727
Keywords: HOSM (high oleic soymilk), TLSM (tofu line soymilk), SEM (scanning electron microscopy), DSC (differential scanning calorimetry), ANOVA (analysis of variance)
Citation: Singh P and Krishnaswamy K (2023) The influence of flavoring components on the physicochemical properties of spray-dried high oleic (HO) and tofu line (TL) soymilk powder. Front. Food. Sci. Technol. 3:1070453. doi: 10.3389/frfst.2023.1070453
Received: 14 October 2022; Accepted: 16 February 2023;
Published: 02 March 2023.
Edited by:
Minwei Xu, North Dakota State University, United StatesReviewed by:
Ali Ubeyitogullari, University of Arkansas, United StatesDimas Rahadian Aji Muhammad, Sebelas Maret University, Indonesia
Copyright © 2023 Singh and Krishnaswamy. This is an open-access article distributed under the terms of the Creative Commons Attribution License (CC BY). The use, distribution or reproduction in other forums is permitted, provided the original author(s) and the copyright owner(s) are credited and that the original publication in this journal is cited, in accordance with accepted academic practice. No use, distribution or reproduction is permitted which does not comply with these terms.
*Correspondence: K. Krishnaswamy, krishnaswamyk@umsystem.edu