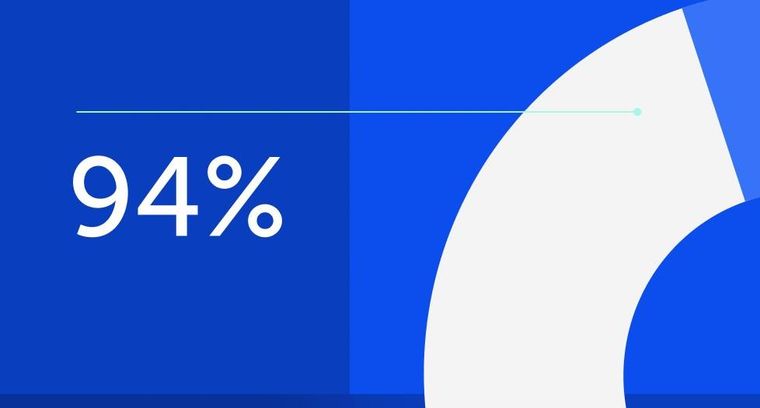
94% of researchers rate our articles as excellent or good
Learn more about the work of our research integrity team to safeguard the quality of each article we publish.
Find out more
ORIGINAL RESEARCH article
Front. Food. Sci. Technol., 15 February 2023
Sec. Food Biotechnology
Volume 3 - 2023 | https://doi.org/10.3389/frfst.2023.1012121
There is widespread interest in the immunostimulatory effects of food-derived substances that are expected to contribute to improving human health. Broccoli (Brassica oleracea var. italica) has been shown to contain potent polysaccharides for developing food materials with immunomodulatory function. In this study, immunostimulatory activity of previously uncharacterized broccoli polysaccharides was assessed using a silkworm (Bombyx mori) immunological assay (using muscle contraction as an index) across 10 commercial and 17 experimental cultivars of broccoli. For the commercial cultivars, there was a significant variation in specific activity (units/mg, measured by the silkworm-based immunological assay). For the experimental cultivars, a cultivar “winter dome” showed the highest activity. We further purified the immunostimulatory polysaccharides of “winter dome” by gel filtration. Using the silkworm-based assay, we found that the peak fraction (“Fraction II”) contained the active substance with higher specific activity than previously reported active substances (e.g., glucans). The substance appeared a heterogeneous molecular weight of more than 270 kDa. The major sugar composition of Fraction II was glucose, suggesting that glucose homopolymer is responsible for the activity. Further, Fraction II induced cytokine productions both in the silkworm (production of active B. mori paralytic peptide (BmPP) in vivo) and mouse (production of TNFα in vitro) immune system. These results suggest that glucose homopolymer of broccoli induces cytokines both in insect and mammalian immune systems, providing molecular insights to our understanding about how plant-derived substances interact with animal immune system.
Innate immunity is a mechanism that rapidly eliminates foreign or unwanted substances (e.g., bacteria, fungi, viruses, and cancer cells) from the body without the use of antibodies, and its molecular design is evolutionarily conserved across phyla ranging from invertebrates to mammals (Miyashita et al., 2012; Miyashita et al., 2014; Miyashita et al., 2015; Miyashita et al., 2018; Miyashita et al., 2019). Enhancing immune function with orally available substances may contribute to human health in terms of preventing unwanted microbial infections. It also contributes to ensuring food quality and safety, which is currently regulated by guidelines (Bondoc, 2016), by defining substances that affect animal physiology. We have previously reported that the Bombyx mori paralytic peptide (BmPP) pathway, which induces muscle contraction, is one of the innate immune pathways that responds to xenobiotics in the silkworm, B. mori (Ishii et al., 2008; Ishii et al., 2010). BmPP is a multifunctional peptide hormone that induces both muscle contraction and activation of circulating immune cells (i.e., hemocytes) in the silkworm. Utilizing this feature of BmPP, we previously developed an experimental method to quantify the muscle contraction activity (as a marker of BmPP activation) and proposed a methodology to search for natural products that stimulate innate immunity using the silkworm (Dhital et al., 2011; Fujiyuki et al., 2012). Although the immunoregulatory functions of polysaccharides have long been of interest in the research field (Horsfall and Dimond, 1957; BolouriMoghaddam and Van den Ende, 2012; Shi et al., 2021; Wang et al., 2021; Zhang et al., 2021; Cai et al., 2022; Taoerdahong et al., 2022), our empirical knowledge about immunostimulatory function of plant-derived polysaccharides when administered in animal body remains fragmentary. Thus we have applied the silkworm model to search for compounds with potential to human application, given the conserved feature of innate immune system between invertebrate and vertebrate animals.
Using the silkworm-based assay system, we have demonstrated yeast β-glucans (Fujiyuki et al., 2012) and acidic broccoli (Brassica oleracea var. italica) polysaccharides (Urai et al., 2017) are immunostimulatory agents, as they activated BmPP in the hemolymph and induced muscle contraction of silkworms. In broccoli, an immunostimulatory effect of pectin-like acidic polysaccharides was demonstrated with a specific activity of 130 units/mg (units were quantitatively determined by the silkworm-based assay as described previously and in this study) (Urai et al., 2017). In the literature, not only acidic polysaccharides, neutral polysaccharides have also been reported to have immunostimulatory effects using cell-based assay in vitro (e.g., spleen cell proliferation, production of nitric oxide, etc.) (Ni et al., 2010; Yi et al., 2012; Li et al., 2014; Zhang et al., 2020). These reports concur the long-acknowledged concept in immunology that some polysaccharides are recognized as immunoreactive pattern molecule by the immune system across organismal phyla (Horsfall and Dimond, 1957; Leung et al., 2006; BolouriMoghaddam and Van den Ende, 2012; Li et al., 2014; Li and Wang, 2015; Ohta et al., 2016; Urai et al., 2017). More recently, high molecular weight (approximately 300 kDa) acidic polysaccharide from lepidopteran insect species (Antheraea yamamai and B. mori) has been shown to stimulate innate immunity of mammals (Ohta et al., 2016; Ali et al., 2018), which is in line with our proposal to screen for immunostimulatory polysaccharides by making the most of the polysaccharide sensing function conserved in the silkworm.
In our previous study, we reported that the hot water extract of the edible part of broccoli showed higher immunostimulatory activity than other plants to the silkworm (Urai et al., 2017). In the study, the active component was first precipitated by ethanol, and then subjected to diethylaminoethyl (DEAE)-cellulose column chromatography and taken its bound fraction (Urai et al., 2017). The suggested chemical structure was a pectin-like acidic polysaccharide (Urai et al., 2017). On the other hand, some non-acidic (neutral) polysaccharides in different plant materials have recently been reported to have physiological effects on the innate immune system (Ni et al., 2010; Yi et al., 2012; Li et al., 2014; Zhang et al., 2020), but there are no such reports for broccoli. In the present study, we examined whether there are bioactive neutral polysaccharides in the flow-through fraction of DEAE-cellulose column chromatography using the hot water extract of broccoli. We also compared across broccoli cultivars for the immunostimulatory activity, and we further purified the active substance by a gel-filtration column chromatography and tested whether the immunoreactive effect of the substance is common in invertebrates and mammals.
The total immunostimulatory activity (measured by the silkworm-based muscle contraction assay described in the method section) of each part of broccoli is shown in Table 1 (see Figure 1A, B for graphical details of broccoli parts and sample preparation). For every 100 g (wet weight) of start material, the hot-water extract of buds contained 1,700,000 ± 450,000 units (n = 3), that of stems contained 40,000 ± 20,000 units (n = 3), and that of stalk contained 6,600 ± 2,100 units (n = 3). The actual wet weight of each individual broccoli used in the experiment was 120 ± 5.8 g for buds, 150 ± 23 g for stems, and 84 ± 13 g for stalk. Thus, majority of the activity existed in the buds such that 97% for buds, 2.8% for stems, and 0.26% for stalk (see Table 1 for the results from three experimental replicates).
TABLE 1. Total immunostimulatory activity found in the hot-water extracts of broccoli (per 100 g wet weight of each part).
FIGURE 1. (A) The buds, stems, and stalk of broccoli. The picture represents the three parts of broccoli (buds, stems, and stalks) as described in the Materials and Methods section. In this study, broccoli was broken down into these three parts and hot water extracts were prepared separately. The immunostimulatory activity of each part is summarized in Table 1. (B) The scheme of the neutral polysaccharide preparation from broccoli. Shown in the panel is a simplified scheme for the preparation of neutral polysaccharides from broccoli: PCI, phenol-chloroform-isoamyl alcohol; DEAE, diethylaminoethyl. For a detailed description of each step, please refer to the Materials and Methods section. (C) Immunostimulatory effect (silkworm muscle contraction assay) of the neutral polysaccharides of broccoli. The graph shows the dose-dependent immunostimulatory effect of a neutral polysaccharide sample prepared from broccoli cultivar No. 1 (see also Table 2 for a list of results for other cultivars). Three independent experimental replicates (replicate #1, replicate #2, and replicate #3) are shown in the panel. The vertical axis represents the C value (see Materials and Methods for how we determined the C values), and the horizontal axis represents the sample dose used in the assay. (D) The broccoli neutral polysaccharides stimulate mammalian immune cells. The graph shows the immunostimulatory activity of neutral polysaccharide from broccoli on mouse macrophage cells. The vertical axis represents the TNF-alpha concentration detected in the culture medium of mouse macrophage cells (pg/mL). Each plot represents each technical replicate for the measurement. Experimental details are described in the method section. There was a statistically significant difference between the two groups (Student’s t-test p-value = .00013). (E) Immunostimulatory activity of different broccoli (and cauliflower) cultivars. Each bar chart represents the amount of immunostimulatory activity per weight (Units/mg), with an error bar that represents standard deviation. Each cultivar names are demonstrated at the bottom of the chart.
Immunostimulatory activities were determined by the silkworm-based muscle contraction assay. SD: Standard Deviation. There was a significant difference (Analysis of variance (ANOVA); p = .00011) across the three parts (values were obtained from experiments using 120 g Buds, 150 g Stems, and 84 g Stalk). The activity found in the buds was significantly higher than other parts (post hoc t-test; p = .00026 vs. stems, and p = 4.2 × 10−5 vs. Stalk).
For the cultivar set #1, the immunostimulatory activity of DEAE flow-through fraction (i.e., non-acidic polysaccharide fraction) was determined for each cultivar using the silkworm muscle contraction assay (Figure 1C for cultivar No. 1, and others listed in Table 2). As shown in Table 2, samples from all cultivars showed the activity but with different specific activity (i.e., amount of activity per sugar amount), ranging from a maximum of 13,000 units/mg sugar (cultivar No. 1) to a minimum of 840 units/mg sugar (cultivar No. 3). We further assessed the immunostimulatory activity of the sample from cultivar No. 1 on mouse macrophage cells. As demonstrated in Figure 1D, the TNF-alpha concentration in the culture medium of mouse macrophage cells was higher when treated by the sample (mean = 585 pg/mL, sd = 77 pg/mL) than when treated by the vehicle (mean = 77 pg/mL, sd = 4.2 pg/mL). The difference was statistically significant (p = .00013 in Student’s t-test).
TABLE 2. List of broccoli cultivars and their immunostimulatory activities used for cultivar set #1 (ten commercial cultivars). There was a significant difference across cultivars; Akaike’s Information Criterion (AIC) for full model (AICfull = −272.8) was lower than that for the null model (AICnull = −256.8).
Since the detailed information for each cultivar was not available for the cultivar set #1, we then cultured different cultivars (cultivar set #2) that are provided with published information (see Materials and Methods section for details). The immunostimulatory activities of non-acidic polysaccharide fraction (i.e., DEAE flow-through fraction) were determined for the cultivar set #2 (15 broccoli and 2 cauliflower cultivars, Figure 1E). The immunostimulatory activity per sugar weight (i.e., specific activity) distributed between 260 and 11000 units/mg, with the cultivar “winter dome” showing the highest specific activity (Figure 1E). We thus decided to use “winter dome” cultivar for the following analysis.
Using the cultivar “winter dome”, we purified the immunostimulatory substance. By the gel filtration column chromatography (we pooled Fraction II as shown in Figure 2A), the specific activity increased by 3.6-fold compared to the loaded DEAE flow-through fraction. The yield of activity was 50% (Figure 2A; Table 3). As shown in Figure 2A, the activity appeared on different positions of the gel filtration, indicating that polysaccharides with different molecular sizes greater than 270 kDa shows the immunostimulatory effect.
FIGURE 2. (A) Elution profile of Sephacryl S-1000 gel filtration column chromatography. The elution profile of the gel filtration column chromatography is shown in the figure. The x-axis represents elution volume (mL), and the y-axes represent sugar concentration (µg/mL) of each fraction (the left axis) and the contraction value (the right axis). Method details for the chromatography, sugar quantification, and contraction value estimation are described in the method section. The black circles represent the sugar concentrations, and the red squares represent the contraction values. As shown in the chart, we pooled fractions into three fractions: Fr. I, II, and III. In this study, Fr. II was used for further analysis. Please also see Table 2 for the purification table. (B) Sugar component analysis of the purified immunostimulatory neutral polysaccharide (Fr. II). Shown in the figure is the HPLC chart of sugar component analysis. The x-axis represents retention time (minutes). The red chart represents the HPLC pattern from ABEE-labelled sugar (monosaccharide) mixture. Each peak is annotated as described in the method section. The black chart represents the HPLC pattern from ABEE-labelled hydrolyzed sample (Fr. II). As shown in the figure, Fr. II showed a signal from ABEE-labelled glucose, and other monosaccharides were under the detection limit. See the method section for technical details for this experiment. (C) Activation of Paralytic Peptide (BmPP) in the response to broccoli-derived neutral polysaccharide (Fr. II). Silkworm hemolymph samples were run on an SDS-polyacrylamide gel and the active BmPP was detected by immunoblot analysis using anti-PP antibody. Hemolymph samples were collected 3 min after either the sample (Fr. II) or the vehicle (saline) injection. The gel image before cropping is shown in Supplementary Figure S1 (please see the supplementary material). (D) Immunostimulatory effect of the purified fraction (Fr. II) on mouse macrophage cells. Mouse macrophage cells (RAW264.7) were exposed to the sample and measured for TNF-α amount in the culture medium. The y-axis represents the TNF-α concentration (pg/mL). Same experiment was performed using the buffer that we used for the gel filtration column chromatography when we obtained Fr. II (Buffer Control), where neither of the three replicates induced TNF-α production by RAW.264.7 cells. N.D., not detected. There was a statistically significant difference between the two groups (p = .0035, Student’s t-test).
After acid hydrolysis and ABEE labeling of Fr. II, we performed an HPLC analysis, where it showed a single peak derived from ABEE-labeled glucose. Other monosaccharides were below the detection limit (Figure 2B). This result indicates that the chemical structure of the non-acidic polysaccharide present in Fr. II is glucose homopolymer, which is a neutral polysaccharide, that is, likely to not bind to DEAE cellulose column. We also observed an induction of active BmPP in response to an intra-haemocoel administration of Fr. II to the silkworm (Figure 2C), confirming that Fr. II stimulates the innate immune system via cytokine production.
We further assessed the immunostimulatory effect of the broccoli neutral polysaccharides (Fraction II) on mammalian cells. As shown in Figure 2D, TNF-alpha concentration in the culture medium of mouse macrophage cells was much higher when treated by Fraction II (mean = 205 pg/mL, sd = 30 pg/mL) than when treated by the buffer used for gel filtration (under detection limit for all three replicates). The difference was statistically significant (p = 0.0035, Student’s t-test).
In the present study, we first examined the non-acidic polysaccharide fraction (the flow-through fraction of DEAE cellulose column chromatography using the hot water/PCI extract of broccoli as a start material) for its immunostimulatory activity. The results suggest the presence of neutral polysaccharides that act on innate immunity in the broccoli. In addition, in the present study, we compared the amount of activity across three edible parts (buds, stems, and stalk) to understand the distribution of the active substance. The results suggest that the buds contain the majority of active molecule (see Table 1). However, relatively large variation (large standard deviations) was observed for the stems and the stalk, and care should be taken in estimating their actual percentages. Yet, in any case, the results indicate that broccoli buds have potential applications as ingredients for functional food. This is the first report for bioactive neutral polysaccharides of broccoli that induces muscle contraction in the silkworm in vivo (i.e., activation of the insect cytokine BmPP) and cytokine release from mouse macrophage cells in vitro.
In this study, we further purified the active substance and obtained the information about its chemical structure based on the molecular size estimation in gel filtration column chromatography and the results of sugar composition analysis. The results suggest that the active polysaccharide of broccoli (“winter dome” cultivar) is neutral glucose homopolymer with the molecular size over 270 kDa. In plants and fungi other than broccoli, polysaccharides from edible materials such as Cordyceps militaris (Zhang et al., 2020), Mosla chinensis (Li et al., 2014), Panax ginseng (Ni et al., 2010), and Dimocarpus longan (Yi et al., 2012) have been reported to have immunostimulatory effects. Nevertheless, information about their chemical structure was scarce, and this study provides information about how we can purify the active polysaccharide and about their chemical structure. Currently, we do not know whether the bioactive polysaccharides from the above species share the chemical structure (i.e., glucose homopolymer) or not.
In the present study, we have also compared the immunostimulatory activity (i.e., silkworm muscle contraction) of the DEAE flow-through fractions obtained from 27 cultivars (cultivar set #1 and #2). The results demonstrated differences among the cultivars for the specific activity (i.e., amount of activity per sugar quantity), and whether this difference is due to the amount of active substance or to the presence of active substances with different chemical structures needs further investigation. The DEAE flow-through fraction of the cultivar “winter dome”, which showed the highest specific activity (Figure 1E; care should be taken in interpreting the values, considering the large standard deviations shown in Figure 1E), was further purified by gel filtration. As a result, we found that the active substance in the purified fraction (Fr. II) showed heterogeneous molecular weights larger than 270 kDa and a specific activity of 32,000 Units/mg. We have been using silkworm muscle contraction as an indicator to identify innate immune activators in various food materials, and this value (i.e., 32,000 Units/mg) seems outstanding. For example, the specific activity of the neutral polysaccharide fraction of broccoli is more than 300 times higher than that of curdlan, which has a β-(1,3)-glucan (glucose homopolymer) structure and has been regarded as one of the most active polysaccharides for immunostimulatory effect in the silkworm assay system. There may be a unique chemical structure of glucose homopolymer that shows such outstanding specific activity. Also, comprehensive gene expression analysis using RNA sequencing techniques in silkworm and mouse immune cells to confirm the multifaceted effects of broccoli glucose homopolymers would be a promising future direction for this research.
Two groups of eukaryotes, fungi and plants, possess large amounts of polysaccharides in a structure called the cell wall. Polysaccharide recognition in eukaryotes plays an important role in defense against pathogenic fungi. Lectins and complement proteins are known as molecules that recognize polysaccharides in fungi (Snarr et al., 2017). It has been reported that α-(1,3)-glucan produced by Histoplasma capsulatum contributes to virulence by hiding immunostimulatory β-glucans from detection by host phagocytes (Rappleye et al., 2007). In fact, in our assay system for measuring immune activation ability using silkworm muscle contraction as an indicator, we reported that the strength of activity varied depending on the origin and chemical structure of β-glucans (Fujiyuki et al., 2012). Further investigation will broaden our understanding of the interaction between diverse polysaccharide substances and the animal immune system.
The future direction of food supplements using polysaccharides is likely to involve further research to better understand the specific health benefits of different types of polysaccharides and how they can be used to improve the nutritional value of food products [also see recent reviews in this field (Lomartire and Gonçalves, 2022; Qiu et al., 2022; Tounsi et al., 2022)]. However, the lack of information and human clinical trials limits the wide use of polysaccharides in functional foods. For example, seaweed has been used for therapeutic purposes for centuries, but only recently have advanced technologies made it possible to extract and study the potential properties of its bioactive compounds (Lomartire and Gonçalves, 2022). Additionally, research will likely focus on developing new methods for extracting and purifying polysaccharides from natural sources, such as fruits, vegetables, and grains, in order to make them more suitable for use as food supplements. The present study would be one of those attempts. As more research is conducted on the health benefits of polysaccharides, it is expected that we will see an increase in the number of food products that contain polysaccharides as functional ingredients.
Ten commercial broccoli cultivars (No. 1–10) provided by Sakata Seed Corporation (Yokohama, Japan) were used in the first part of this study. For the cultivar No. 1, we performed two experiments using different batches of broccoli (one experiment for comparing three parts of broccoli, another for preparation of DEAE flow-through fraction). The experiments and plant sample collection complied with guidelines of Teikyo University.
Searching for the start material for purification and further characterization (e.g., sugar composition analysis), we used fifteen cultivars of broccoli (B. oleracea var. italica) and two cultivars of cauliflower (B. oleracea var. botrytis) provided by the National Agriculture and Food Research Organization (Tsukuba, Japan) (Takahashi et al., 2021). Spring crops were used in this study. The list of these cultivars is shown in Figure 1E. The experiments and plant sample collection complied with guidelines of Teikyo University.
We performed a hot-water extraction as described previously (Fujiyuki et al., 2012). Broccoli (Replicate #1, cultivar No.1) was divided into two edible parts (small flower buds, and stems) and an inedible part (stalks) with scissors, boiled for 2 min (at the boiling point in the laboratory), and then immersed in ice-cold water (ca. 0°C) to cool well. Figure 1A is a photographic description of each part of the broccoli. The small flower buds were then separated from the stems using scissors, and the weight of buds, stems, and stalks were measured. Fifteen grams of each part was taken and homogenized using a polytron with 30 mL of Milli-Q water. The homogenate sample was then autoclaved for 15 min at 121°C to perform a hot-water extraction, and the supernatant was collected (8,590 g for 10 min at 4°C).
Immunomodulatory activity was measured using a silkworm-based assay as previously reported (Urai et al., 2017; Li et al., 2022). In this assay, we measure silkworm muscle contraction as an index of BmPP (insect cytokine) level. Muscle specimens were prepared by rearing 5th instar silkworms on artificial diet (Silkmate 2S, Nihon Nosan Kogyo, Tokyo, Japan) for 5 days since the last ecdysis (Urai et al., 2017). The sample solution (50 µl) was injected into the muscle specimens using a tuberculin syringe (1 mL) with a 27-gauge needle, and the body length was monitored for 10 min. The contraction value [C = (x-y)/x] was calculated for the length of the silkworm at the beginning of the experiment [x (cm)] and the length at the maximum contraction [y (cm)]. The contraction values (C-values) for various sample doses (6–10 doses for each sample, and one silkworm was used for each dose) were plotted on a graph, and the sample volume with a C-value of .15 was estimated, and its activity was determined to be 1 unit.
Please see Figure 1B for a simplified scheme of the whole process. Two volumes of PCI (phenol/chloroform/isoamyl alcohol) were added to the hot water extract of broccoli, and the aqueous layer was collected after centrifugation (8,590 g, 5 min at 4°C). Two volumes of ethanol were added to the aqueous layer, and the precipitate formed by centrifugation (8,590 g, 10 min at 4°C) was dissolved in 10 mM Tris-HCl (pH 7.9) buffer, and loaded to a DEAE cellulose column (GE Healthcare, bed volume = 20 mL) equilibrated by the same buffer, and we collected the flow-through fraction (Figure 1B).
The flow-through fraction from DEAE column chromatography (we used “winter dome” cultivar for this experiment) was concentrated by ethanol precipitation and then applied to a gel filtration column (Sephacryl S-1000, diameter = 15 mm, height = 1080 mm, bed volume = 190 mL). The column was equilibrated by 10 mM Tris/HCl (pH = 7.9) buffer in advance and the same buffer was used for elution. The fraction size was 2 mL/fraction, and each fraction was subjected to sugar quantification (duplicate experiment) and silkworm muscle contraction assay (the c-value for each fraction was obtained from a duplicate assay). Sugar quantification was performed according to a previously reported method (Urai et al., 2017). Active fractions were pooled to obtain three fractions (fractions #30-#45 as Fraction I, fractions #46-#80 as Fraction II, and fractions #81-#100 as Fraction III as shown in Figure 2A). We used Fraction II for further characterization, as the fraction showed an increased specific activity (please also see the purification table as shown in Table 2).
Sugar composition analysis was performed using 4-aminobenzoic acid ethyl ester (ABEE) labeling method as previously reported (Urai et al., 2017). After acid hydrolysis of the purified polysaccharide fraction (fraction II), the ABEE-labeled samples were analyzed by an HPLC using a reversed-phase column. Monosaccharide standards were used as control, and each monosaccharide was identified based on elution time. To further validate the peak annotation, the samples were mixed with ABEE labeled glucose samples and analyzed by HPLC, where we confirmed that the peaks overlapped for each sample.
We followed the previously reported method for the detection of active BmPP (Miyashita et al., 2015). Fifth instar (day 5) silkworm larvae were injected with 50 µl of the fraction II (from the gel filtration column chromatography) or saline (Vehicle). After 3-min incubation, hemolymph was collected, by cutting the silkworm abdominal legs directly, into 1.5 mL tubes preheated in a heat block at 100°C (1 tube/larva). After 10 min of heat treatment at 100°C, samples were spun at 10,000 g for 10 min and the supernatants were collected. An equal volume of blood from three animals in each group was pooled, and 15 µl of the pooled blood was applied to each lane for electrophoresis. Western blotting was performed to examine the bands detected by anti-paralytic peptide (BmPP) antibody.
We maintained RAW264.7 cells, a murine macrophage-derived cell line at 37 °C with 5% CO2 using RPMI-1640 (Thermo Fisher Scientific K.K., Tokyo, Japan) with 10% fetal bovine serum (FBS). When performing the TNF-alpha induction assay, we inoculated RAW264.7 cells (1✕105 cells/well) in RPMI-1640 (+10% FBS) on a 96-well flat-bottom plate, and cultured at 37°C for 24 h. We then removed the medium, and immediately put the sample (i.e., 10 times diluted DEAE flow-through fraction) or the buffer used for the chromatography (10 mM Tris-HCl, pH = 7.9) suspended in RPMI 1640 (non-FBS) medium, and cultured the cells for 6 h. We collected the cell culture supernatant and measured the TNF-α concentration using Mouse TNF-α Quantikine ELISA Kit MTA00B (R&D SYSTEMS).
The original contributions presented in the study are included in the article/Supplementary Materials, further inquiries can be directed to the corresponding authors.
Study conception and design: AM and KS. Acquisition of data: AM, KK, TT, AO, and HN. Analysis and interpretation of data: AM, KK, AO, and KS. Drafting of manuscript: AM. Critical revision: AM, MT, and KS.
We thank Genome Pharmaceuticals Institute, Co. Ltd., for technical supports, and also thank Sakata Seed Corporation for providing materials. This work was supported by a JSPS KAKENHI (Grant# 20K16253 and 22K15461) to AM, and a JSPS KAKENHI (Grant# 21H02733) to KS.
Author KK, TT, HN, and KS were employed by the company Genome Pharmaceuticals Institute Co., Ltd. and author AO was employed by the company Nihon Berumu Co., Ltd.
The remaining authors declare that the research was conducted in the absence of any commercial or financial relationships that could be construed as a potential conflict of interest.
All claims expressed in this article are solely those of the authors and do not necessarily represent those of their affiliated organizations, or those of the publisher, the editors and the reviewers. Any product that may be evaluated in this article, or claim that may be made by its manufacturer, is not guaranteed or endorsed by the publisher.
The Supplementary Material for this article can be found online at: https://www.frontiersin.org/articles/10.3389/frfst.2023.1012121/full#supplementary-material.
ABEE, 4-aminobenzoic acid ethyl ester; BmPP, Bombyx mori paralytic peptide; DEAE, diethylaminoethyl; TNF, tumor necrosis factor; HPLC, high performance liquid chromatography; AIC, akaike’s information criterion.
Ali, M. F. Z., Yasin, I. A., Ohta, T., Hashizume, A., Ido, A., Takahashi, T., et al. (2018). The silkrose of Bombyx mori effectively prevents vibriosis in penaeid prawns via the activation of innate immunity. Sci. Rep. 8 (1), 8836. doi:10.1038/s41598-018-27241-3
Bolouri Moghaddam, M. R., and Van den Ende, W. (2012). Sugars and plant innate immunity. J. Exp. Bot. 63 (11), 3989–3998. doi:10.1093/jxb/ers129
Bondoc, I. (2016). European regulation in the veterinary sanitary and food safety area, a component of the European policies on the safety of food products and the protection of consumer interests: A 2007 retrospective. Part One: The role of enropean institutions in laying dawn and passing laws specific to the veterinary sanitary and food safety area. Universul Juridic 2016, 12–15. Supliment.
Cai, B., Zhao, X., Luo, L., Wan, P., Chen, H., and Pan, J. (2022). Structural characterization, and in vitro immunostimulatory and antitumor activity of an acid polysaccharide from Spirulina platensis. Int. J. Biol. Macromol. 196, 46–53. doi:10.1016/j.ijbiomac.2021.12.062
Dhital, S., Hamamoto, H., Urai, M., Ishii, K., and Sekimizu, K. (2011). Purification of innate immunostimulant from green tea using a silkworm muscle contraction assay. Drug Discov. Ther. 5 (1), 18–25. doi:10.5582/ddt.v5.1.18
Fujiyuki, T., Hamamoto, H., Ishii, K., Urai, M., Kataoka, K., Takeda, T., et al. (2012). Evaluation of innate immune stimulating activity of polysaccharides using a silkworm (Bombyx mori) muscle contraction assay. Drug Discov. Ther. 6 (2), 88–93. doi:10.5582/ddt.2012.v6.2.88
Horsfall, J. G., and Dimond, A. E. (1957). Interactions of tissue sugar, growth substances, and disease susceptibility. Z. für Pflanzenkrankh. Pflanzenpathol. Pflanzenschutz 64 (7/10), 415–421.
Ishii, K., Hamamoto, H., Kamimura, M., Nakamura, Y., Noda, H., Imamura, K., et al. (2010). Insect cytokine paralytic peptide (PP) induces cellular and humoral immune responses in the silkworm Bombyx mori. J. Biol. Chem. 285 (37), 28635–28642. doi:10.1074/jbc.M110.138446
Ishii, K., Hamamoto, H., Kamimura, M., and Sekimizu, K. (2008). Activation of the silkworm cytokine by bacterial and fungal cell wall components via a reactive oxygen species-triggered mechanism. J. Biol. Chem. 283 (4), 2185–2191. doi:10.1074/jbc.M705480200
Leung, M. Y. K., Liu, C., Koon, J. C. M., and Fung, K. P. (2006). Polysaccharide biological response modifiers. Immunol. Lett. 105 (2), 101–114. doi:10.1016/j.imlet.2006.01.009
Li, J-E., Nie, S-P., Xie, M-Y., and Li, C. (2014). Isolation and partial characterization of a neutral polysaccharide from Mosla chinensis Maxim. cv. Jiangxiangru and its antioxidant and immunomodulatory activities. J. Func. Foods 6, 410–418. doi:10.1016/j.jff.2013.11.007
Li, P., and Wang, F. (2015). Polysaccharides: Candidates of promising vaccine adjuvants. Drug Discov. Ther. 9 (2), 88–93. doi:10.5582/ddt.2015.01025
Li, W., Miyashita, A., and Sekimizu, K. (2022). Peanut triacylglycerols activate innate immunity both in insects and mammals. Scis Rep. 12 (1), 7464–7469. doi:10.1038/s41598-022-11494-0
Lomartire, S., and Gonçalves, A. M. (2022). Novel technologies for seaweed polysaccharides extraction and their use in food with therapeutically applications—a review. Foods 11 (17), 2654. doi:10.3390/foods11172654
Miyashita, A., Iyoda, S., Ishii, K., Hamamoto, H., Sekimizu, K., and Kaito, C. (2012). Lipopolysaccharide O-antigen of enterohemorrhagic Escherichia coli O157: H7 is required for killing both insects and mammals. FEMS Microbiol. Lett. 333 (1), 59–68. doi:10.1111/j.1574-6968.2012.02599.x
Miyashita, A., Kizaki, H., Kawasaki, K., Sekimizu, K., and Kaito, C. (2014). Primed immune responses to gram-negative peptidoglycans confer infection resistance in silkworms. J. Biol. Chem. 289 (20), 14412–14421. doi:10.1074/jbc.M113.525139
Miyashita, A., Lee, T. Y. M., McMillan, L. E., Easy, R., and Adamo, S. (2018). Terminal reproductive investment, physiological trade-offs and pleiotropic effects: Their effects produce complex immune/reproductive interactions in the cricket Gryllus texensis. bioRxiv 2018, 499236. doi:10.1101/499236
Miyashita, A., Lee, T. Y. M., McMillan, L. E., Easy, R., and Adamo, S. A. (2019). Immunity for nothing and the eggs for free: Apparent lack of both physiological trade-offs and terminal reproductive investment in female crickets (Gryllus texensis). PloS one 14 (5), e0209957. doi:10.1371/journal.pone.0209957
Miyashita, A., Takahashi, S., Ishii, K., Sekimizu, K., and Kaito, C. (2015). Primed immune responses triggered by ingested bacteria lead to systemic infection tolerance in silkworms. PLoS One 10 (6), e0130486. doi:10.1371/journal.pone.0130486
Ni, W., Zhang, X., Wang, B., Chen, Y., Han, H., Fan, Y., et al. (2010). Antitumor activities and immunomodulatory effects of ginseng neutral polysaccharides in combination with 5-fluorouracil. J. Med. Food 13 (2), 270–277. doi:10.1089/jmf.2009.1119
Ohta, T., Kusano, K., Ido, A., Miura, C., and Miura, T. (2016). Silkrose: A novel acidic polysaccharide from the silkmoth that can stimulate the innate immune response. Carbohydr. Polym. 136, 995–1001. doi:10.1016/j.carbpol.2015.09.070
Qiu, S-M., Aweya, J. J., Liu, X., Liu, Y., Tang, S., Zhang, W., et al. (2022). Bioactive polysaccharides from red seaweed as potent food supplements: A systematic review of their extraction, purification, and biological activities. Carbohydr. Polym. 275, 118696. doi:10.1016/j.carbpol.2021.118696
Rappleye, C. A., Eissenberg, L. G., and Goldman, W. E. (2007). Histoplasma capsulatumα-(1,3)-glucan blocks innate immune recognition by the β-glucan receptor. PNAS 104 (4), 1366–1370. doi:10.1073/pnas.0609848104
Shi, Y., Ye, Y-F., Zhang, B-W., Liu, Y., and Wang, J-H. (2021). Purification, structural characterization and immunostimulatory activity of polysaccharides from Umbilicaria esculenta. Int. J. Biol. Macromol. 181, 743–751. doi:10.1016/j.ijbiomac.2021.03.176
Snarr, B. D., Qureshi, S. T., and Sheppard, D. C. (2017). Immune recognition of fungal polysaccharides. J. Fungi 3 (3), 47. doi:10.3390/jof3030047
Takahashi, M., Ohishi, M., Sato, F., Okada, K., and Sasaki, H. (2021). Enlarging broccoli (Brassica oleracea L. var. italica) heads by extending the growing period and sparse planting to increase floret yield. Hort. J. 90 (1), 75–84. doi:10.2503/hortj.utd-241
Taoerdahong, H., Zhou, K., Yang, F., and Dong, C-X. (2022). Structure, immunostimulatory activity, and the effect of ameliorating airway inflammation of polysaccharides from Pyrus sinkiangensis Yu. Int. J. Biol. Macromol. 195, 246–254. doi:10.1016/j.ijbiomac.2021.11.176
Tounsi, L., Hentati, F., Hlima, H. B., Barkallah, M., Smaoui, S., Fendri, I., et al. (2022). Microalgae as feedstock for bioactive polysaccharides. Int. J. Biol. Macromol. 221, 1238–1250. doi:10.1016/j.ijbiomac.2022.08.206
Urai, M., Kataoka, K., Nishida, S., and Sekimizu, K. (2017). Structural analysis of an innate immunostimulant from broccoli, Brassica oleracea var. italica. Drug Discov. Ther. 11 (5), 230–237. doi:10.5582/ddt.2017.01044
Wang, Y-J., Wan, D-L., Li, Q-M., Zha, X-Q., and Luo, J-P. (2021). Structural characteristics and immunostimulatory activities of a new polysaccharide from Dendrobium fimbriatum Hook. Food & Func. 12 (7), 3057–3068. doi:10.1039/d0fo03336g
Yi, Y., Zhang, M-W., Liao, S-T., Zhang, R-F., Deng, Y-Y., Wei, Z-C., et al. (2012). Structural features and immunomodulatory activities of polysaccharides of longan pulp. Carbohydr. Polym. 87 (1), 636–643. doi:10.1016/j.carbpol.2011.08.034
Zhang, J., Ma, N., Ma, G., Zhong, L., Pei, F., Hu, Q., et al. (2021). Characterization of soy protein isolate/Flammulina velutipes polysaccharide hydrogel and its immunostimulatory effects on RAW264. 7 cells. Food Chem Toxicol 151, 112126. doi:10.1016/j.fct.2021.112126
Keywords: silkworm, immunity, broccoli, polysaccharides, biochemistry
Citation: Miyashita A, Kataoka K, Tsuchida T, Ogasawara AA, Nakajima H, Takahashi M and Sekimizu K (2023) High molecular weight glucose homopolymer of broccoli (Brassica oleracea var. italica) stimulates both invertebrate and mammalian immune systems. Front. Food. Sci. Technol. 3:1012121. doi: 10.3389/frfst.2023.1012121
Received: 05 August 2022; Accepted: 27 January 2023;
Published: 15 February 2023.
Edited by:
Seyed Mohammad Taghi Gharibzahedi, Technical University of Berlin, GermanyReviewed by:
Ivan Luzardo-Ocampo, Institute of Neurobiology, National Autonomous University of Mexico, MexicoCopyright © 2023 Miyashita, Kataoka, Tsuchida, Ogasawara, Nakajima, Takahashi and Sekimizu. This is an open-access article distributed under the terms of the Creative Commons Attribution License (CC BY). The use, distribution or reproduction in other forums is permitted, provided the original author(s) and the copyright owner(s) are credited and that the original publication in this journal is cited, in accordance with accepted academic practice. No use, distribution or reproduction is permitted which does not comply with these terms.
*Correspondence: Kazuhisa Sekimizu, c2VraW1penVAbWFpbi50ZWlreW8tdS5hYy5qcA==
Disclaimer: All claims expressed in this article are solely those of the authors and do not necessarily represent those of their affiliated organizations, or those of the publisher, the editors and the reviewers. Any product that may be evaluated in this article or claim that may be made by its manufacturer is not guaranteed or endorsed by the publisher.
Research integrity at Frontiers
Learn more about the work of our research integrity team to safeguard the quality of each article we publish.