- 1CSGA, Team “Molecular Mechanisms of Flavor Perception”, University of Burgundy Franche-Comté, CNRS, Institut Agro, Dijon, France
- 2Faculty of Biotechnologies (BioTech), ITMO, Saint Petersburg, Russia
The sensory perception of food is a complex phenomenon involving the integration of different stimuli (aroma, taste, trigeminal sensations, texture and visual). Flavor compounds activate odorant, taste and trigeminal chemoreceptors, generating a depolarization of the sensory neurons and then the consciousness of food flavor perception. Recent studies are increasingly highlighting the importance of perireceptor events, which include all the molecular events surrounding the receptors, in the modulation of flavor perception. These events affect the quantity and quality of flavor compounds in the environment of chemoreceptors. They include the metabolization of flavor compounds by enzymes present in biological fluids (saliva and mucus) and the oronasal epithelia and noncovalent interactions with binding proteins. Perireceptor mechanisms have been extensively studied in insects and mammals, demonstrating the importance of the entailed processes in the termination of the chemical signal. In humans, research is in full swing. Here, we reviewed the perireceptor mechanisms recently reported in vitro, in biological fluids and in cells and in vivo in humans. These studies indicate that perireceptor mechanisms likely have an important contribution to flavor perception. This mini-review focuses on recent pioneering studies that are paving the way for this new research area. It also suggests that new approaches taking into account the real conditions of food consumption will be required in the future to accurately address this question.
Introduction
Food flavor is one of the most important food attributes involved in the acceptance or rejection of a food. It corresponds to three modalities, namely, aroma, taste and trigeminal perceptions. Flavor compounds are chemicals belonging to different chemical families that activate chemosensory receptors in the oral and nasal cavities. These receptors include olfactory and trigeminal receptors (OR and TR, respectively) in the nasal and oral cavities and gustatory receptors (GR) in the oral cavity (Patapoutian et al., 2009; Su et al., 2009; Yarmolinsky et al., 2009). Before receptor binding, flavor compounds are released in saliva, where they can interact with numerous salivary proteins, including enzymes (Canon et al., 2018). Aroma compounds reach the olfactory receptors directly via the orthonasal pathway or via the retronasal pathway after being released in the gaseous phase in the oral cavity during food oral processing (Ruijschop et al., 2009; Ployon et al., 2017). In the nasal cavity, they diffuse into the olfactory mucus, which contains peripheral proteins that can interact with them, in the vicinity of olfactory receptors (Heydel et al., 2013). These so-called “perireceptor events” have been mainly studied in the field of olfaction. Numerous studies have pointed out that molecular mechanisms occurring near the OR modulate the quality and quantity of odorants that bind to the OR, modulating the termination of the chemical signal (Heydel et al., 2013, 2019; Thiebaud et al., 2013). Recent studies in humans have also shown that molecular and enzymatic mechanisms targeting flavor compounds take place in the oral cavity as soon as food enters the mouth, impacting not only the retro-olfaction but also the taste and trigeminal perceptions of flavor (Schwartz et al., 2021b; Canon et al., 2021). These molecular mechanisms, which are related to host physiology, are thought to play an important role in flavor perception.
Peripheral mechanisms impacting flavor compounds can be separated into two types, whether they rely on catalysis or interaction without catalysis (Heydel et al., 2013; Canon et al., 2018). First, the noncatalytic scavenging of flavor compounds by perireceptor proteins modifies the concentrations of the free flavor compounds in the biological fluid (saliva or nasal mucus). This can impact the amount of flavor compounds available to interact with chemosensory receptors, either positively (e.g., proteins that facilitate the transport of a hydrophobic compound to its receptor) or negatively (e.g., proteins that scavenge an odorant so that it is unavailable for its receptor), depending on the affinity of the perireceptor protein for the flavor compounds. Second, interactions with xenobiotic metabolizing enzymes (XME) present in fluids and epithelia lead to the production of flavor metabolites, consequently decreasing the quantity of flavor compounds in the vicinity of receptors (Munoz-Gonzalez et al., 2021a). Additionally, it affects quality by generating metabolites, which can activate additional chemoreceptors depending on their structure (Ijichi et al., 2019). These two mechanisms are believed to modulate the chemosensory response (Figure 1).
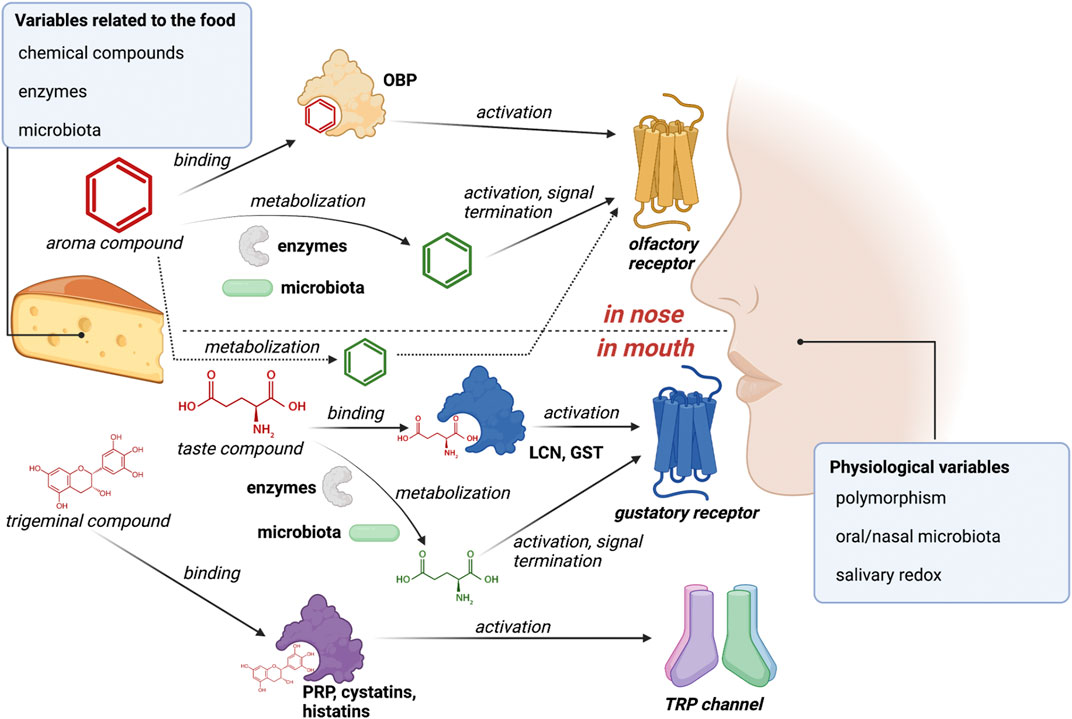
FIGURE 1. Flavor compounds subject to perireceptor events occurring in the oral and nasal cavities and involving human proteins and microorganisms. Abbreviations are as follows: GST, glutathione transferase; LCN, lipocalin; OBP, odorant-binding protein; PRP, proline-rich protein.
In the present paper, we highlight the current research being carried out on human peripheral mechanisms of flavor perception.
Peripheral mechanisms impacting aroma molecules in the nasal cavity
Peripheral mechanisms have been characterized in the nasal cavity, showing the ability of some proteins to interact with odorant (or aroma) compounds. Odorant-binding proteins (OBPs) represent the main group of these proteins. Their structure is characterized by a hydrophobic cavity, named the “calyx”, allowing the binding of aroma compounds and the transport of these hydrophobic compounds up to their respective olfactory receptors through the hydrophilic olfactory mucus (Pelosi and Knoll, 2022). OBP2A, found in the olfactory mucus of humans (Lacazette, 2000), is able to bind a large variety of odorant compounds. Very recently, a research team pointed to a polymorphism in OBP2A gene expression correlated with physiological variations in olfactory performance (Sollai et al., 2019, 2022).
Concerning metabolization events, studies to date have pointed to the role of nasal XME (also called OME for odorant-metabolizing enzymes) in the protection of the olfactory neuroepithelium as well as in the biotransformation of odorants. XME are present in the olfactory epithelium and the nasal mucus (Kornbausch et al., 2022). They are classed as phase I (activation of the odorant mainly through oxidation), phase II (functionalization through transfer of polar groups such as glutathione or UDP-glucuronic acid, not necessarily consecutive of phase I) and phase III (excretion of the metabolite out of the epithelium to the mucus when the previous phase occurs within the cell) enzymes. Phase I XMEs include cytochrome P450, flavin monooxygenases, epoxide hydrolases, aldehyde dehydrogenase, and carboxyl esterase (Heydel et al., 2013; Thiebaud et al., 2013). Glutathione transferases (Schwartz et al., 2020a) and uridine diphosphate glucuronate transferases (UGTs) (Leclerc et al., 2002; Neiers et al., 2021) are conjugation phase II enzymes, and multidrug resistance-associated proteins (MDRs) are phase III transporters (Kudo et al., 2010; Thiebaud et al., 2011).
In rodents, approaches using electroolfactometry or behavioral studies have shown the importance of metabolic events for signal termination (Robert-Hazotte et al., 2019; Neiers et al., 2021), while studies in humans linking nasal metabolism and odorant perception are just emerging. It has been shown that COVID-19-associated loss of smell is related to the UGT2A1/UGT2A2 locus (Shelton et al., 2022). This confirms the importance of UGT present in the olfactory epithelium in humans for odorant perception. Accordingly, Ijichi and coworkers demonstrated in vivo metabolic activities in the nasal cavity, such as methylation, ester hydrolysis and aldehyde reduction, depending on the odorant substrates, and showed that the odorant metabolites significantly affected odor perception (Ijichi et al., 2019). Furthermore, the conversion of odorants by nasal metabolism was found to be lower in patients with olfactory dysfunctions, suggesting the importance of odorant enzymatic conversion in odorant perception (Ijichi et al., 2022). Competition between two odorant molecules for one XME, such as the dicarbonyl-xylulose reductase (DCXR), results in a competitive metabolism impacting the olfactory process (Robert-Hazotte et al., 2022).
Aroma molecules interactions with saliva and oral mucosa
Saliva and oral mucosa both contain various proteins contributing to perireceptor events (Denny et al., 2008; Sivadasan et al., 2015; Canon et al., 2018; Schwartz et al., 2021b). Aroma can bind to salivary proteins such as mucin and alpha-amylase via hydrophobic effects (Pages-Helary et al., 2014), thus modulating the amount of aroma compounds released in the mouth and available to activate the OR. Aroma persistence corresponds to the prolonged release of aroma from the mouth (Muñoz-González et al., 2019). It is driven by the adsorption of aroma compounds onto the thin layer of salivary proteins covering the oral mucosa (Ployon et al., 2020; Munoz-Gonzalez et al., 2021a), which is called the mucosal pellicle (Cabiddu et al., 2020). It was suggested that aroma compounds interact with the mucosal pellicle proteins via hydrophobic effects due to their hydrophobic properties (Aybeke et al., 2019) before being released progressively, resulting in aroma persistence (Table 1).
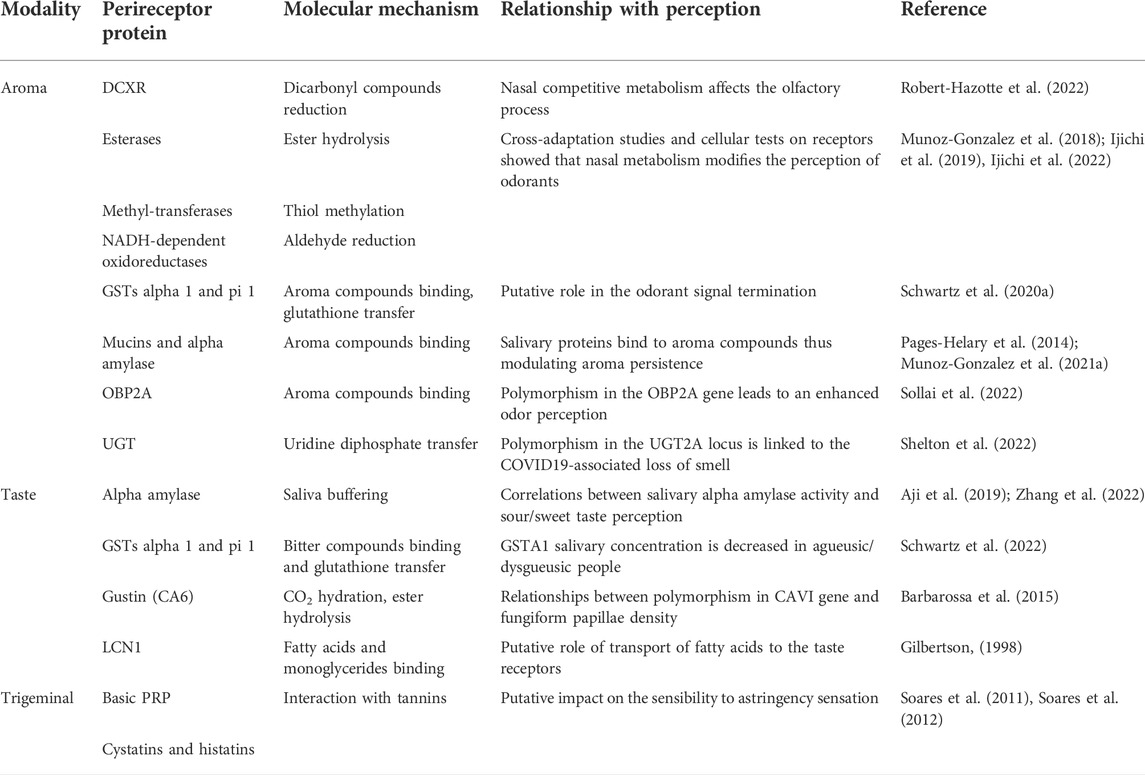
TABLE 1. Olfactory, gustatory and trigeminal perireceptor proteins involved in the modulation of flavor perception in human. Abbreviations are as follows: CA6, carbonic anhydrase 6; DCXR, dicarbonyl-xylulose reductase; GST, glutathione transferase; LCN1, lipocalin 1; OBP2A, odorant-binding protein 2A; PRP, proline-rich protein; UGT, UDP-glucuronosyl transferase.
Conversion of aroma compounds into metabolites by oral XMEs occurs in both saliva (Buettner, 2002b, 2002a; Bader et al., 2018a; Munoz-Gonzalez et al., 2018) and the oral mucosa (Giebultowicz et al., 2009; Mallery et al., 2011; Fabrini et al., 2014; Ployon et al., 2020). Ex vivo or in vivo studies reported various reactions occurring within seconds, such as thiol methylation, aldehyde/ketone reduction or ester hydrolysis (Munoz-Gonzalez et al., 2018, 2019; Ijichi et al., 2019). In addition, Muñoz-Gonzàlez et al. demonstrated that salivary aldehyde reduction was enhanced by nicotinamide adenine dinucleotide (NADH) (Munoz-Gonzalez et al., 2018). This suggests the implication of salivary NAD(P)H-dependent enzymes such as aldehyde dehydrogenase (Giebultowicz et al., 2009), in accordance with similar results obtained on olfactory cleft mucus (Ijichi et al., 2022). The metabolization of aldehydes was also observed using an in vitro model of oral mucosa (Ployon et al., 2020). From a sensory point of view, it has been shown that the newly created metabolites can modulate the activation of olfactory receptors and therefore their perception, thus suggesting that the metabolites are perceived as part of the odor quality of substrates present in food (Ijichi et al., 2019). It has also been demonstrated that the intensity of metabolized compounds decreases more quickly than that of nonmetabolized compounds (Munoz-Gonzalez et al., 2021a).
Salivary antioxidant capacity (SAC) appears to be an important feature influencing aroma compound metabolism (Munoz-Gonzalez et al., 2018, 2019) and aroma release (Munoz-Gonzalez et al., 2021b). The SAC can be defined as the sum of the antioxidant species, including small chemicals but also antioxidant proteins and cofactors. SAC can be imbalanced by pathological states such as obesity, leading to considerable interindividual variability (Schwartz et al., 2020b). Obese people have a higher antioxidant capacity, which leads to a higher reducing power of saliva and a lower aroma release (Piombino et al., 2014). Food can also modify the SAC by carrying oxidative or antioxidative molecules, but further studies are needed to better understand the level of involvement of SAC in flavor perception.
Taste compounds and salivary proteins
For aroma compounds, most of the tastant molecules are released from food during chewing. They are solubilized in saliva and reach the taste receptors located on the tongue surface. Additionally, saliva plays numerous roles, including mucosa protection, lubrication and initiation of digestion (Dawes et al., 2015). Among the 3000 salivary proteins identified in human saliva (Denny et al., 2008; Sivadasan et al., 2015), some are involved in taste perception (Fábián et al., 2015). Different studies have demonstrated a link between the salivary proteome and taste perception, mainly by analyzing the composition of salivary proteins with regard to individual taste sensitivity (Dsamou et al., 2012; Mounayar et al., 2013; Bader et al., 2018b; Stolle et al., 2018). The molecular links between these proteins and taste perception have been established in only a few cases and could involve direct (e.g., transport or metabolization of the tastants) or indirect (e.g., modulating the taste bud density) pathways.
Some enzymes have a demonstrated role in specific taste modalities, such as alpha-amylase, which is highly associated with sweet perception (Rodrigues et al., 2017; Aji et al., 2019). In an acidic environment, salivary alpha-amylase activity is also positively correlated with salivary buffering capacity, which is responsible for a lower intensity of sour perception (Zhang et al., 2022). Similarly, carbonic anhydrase 6 (gustin) is involved in salivary buffering capacity and is related to gustatory and olfactory disorders when expressed at low levels in human parotid saliva (Henkin et al., 1999). A correlation between gustin polymorphism and fungiform papillae density was established, indicating that gustin probably influences taste perception (Barbarossa et al., 2015).
Some enzymes able to metabolize odorants are also involved in tastant metabolism. Indeed, a recent study revealed the ability of two salivary glutathione transferase isoforms (GSTA1 and GSTP1) to metabolize bitter compounds such as isothiocyanates (Schwartz et al., 2022). Interestingly, the salivary enzymatic content can be modulated by the diet. For example, GST salivary activity is increased by a diet rich in broccoli or coffee (Sreerama et al., 1995). Additionally, in a diet rich in bitter compounds, salivary proteins reduce bitter sensitivity and then increase the acceptability of the diet for consumers (Martin et al., 2019). Interestingly, it was shown that the SAC, which modulates the concentration of oxidoreductant cofactors, also modulates taste perception (Walliczek-Dworschak et al., 2017; Zhu et al., 2021).
To date, the lipocalin LCN1 is the only protein proposed to play a transporter role toward tastant molecules. This protein was proposed to solubilize free fatty acids and monoglycerides to allow their detection by taste receptors in the aqueous environment of the oral cavity (Gilbertson, 1998), but this hypothesis needs further verification.
Trigeminal compounds and salivary proteins
Trigeminal sensation corresponds to tactile (somatosensation), proprioceptive and nociceptive afferences to the face and mouth, which are mediated by the trigeminal nerve. Many of the transduction channels that convert thermal, mechanical or chemical stimuli into electrical activity are transient receptor potential (TRP) channels. They are expressed by sensory neurons embedded in the oral mucosae, but some of them are also expressed by keratinocytes, which may release signal molecules acting on the sensory neurons in response to noxious thermal stimuli (Patapoutian et al., 2009). A similar mechanism could also occur in the perception of astringency (Canon et al., 2021), in parallel with the activation of mechanoreceptors following the aggregation of the mucosal pellicle (Ployon et al., 2018). Regarding TRP channels, some appear as polymodal transducers, as they can be activated by stimuli of different natures. For instance, TRPV1 is activated by capsaicin and heat, TRPM8 is activated by cold and menthol, and TRPA1 is activated by a variety of noxious stimuli, including cold temperatures, pungent natural compounds and environmental irritants (Patapoutian et al., 2009).
The activation of these different receptors is modulated by salivary composition. For instance, it has been reported that basic proline-rich proteins (bPRPs), which are able to bind and scavenge tannins (Canon et al., 2011, 2013, 2015), protect the mucosal pellicle from aggregation (Ployon et al., 2018) and increase liking of astringent solution in rats (Glendinning, 1992). Other families of salivary proteins, such as histatins and cystatins, have been reported to interact with and aggregate tannins (Soares et al., 2011, 2012); thus, salivary composition is likely to be linked with astringency sensibility. Structurally unrelated cysteine-modifying agents, such as cinnamaldehyde, isothiocyanates or allicin, activate TRPA1 via covalent modification of cysteine residues (Hinman et al., 2006; Macpherson et al., 2007). Some of these compounds, in particular isothiocyanates, are metabolized by GSTs (Schwartz et al., 2022), suggesting a possible impact on the activation of TRPA1. Moreover, activation of mechanoreceptors depends on the lubrification of the oral cavity, which depends on saliva and its composition (Bongaerts et al., 2007; Yakubov et al., 2015).
In return, the activation of trigeminal receptors may lead to changes in the composition and flow of saliva, affecting its properties. Indeed, mechanical stimulation during food chewing is known to modify salivary flow and composition (Engelen and Van Der Bilt, 2008). The consumption of capsaicin-rich foods, which activate TRPV1, stimulates the secretion of saliva and nasal mucus, increasing the removal of capsaicin (Bessac and Jordt, 2008; Brooks, 2011). TRPA1 deficiency leads to decreased MUC5AC secretion at the pulmonary level (Caceres et al., 2009). Similar mechanisms could occur in the oral cavity, impacting MUC5B secretion. Salivatory responses vary greatly between individuals, affecting responses to astringent stimuli and thus influencing the overall acceptability of polyphenol-rich foods (Dinnella et al., 2009, 2011).
Nasal and oral microbiota and their impact on flavor
The role of the oral and nasal microbiota and their relationships with chemoperception represents an increasingly studied topic of research. The bacterial diversity of the nasal microbiota differs during life stages from childhood to adulthood. A cross-sectional study focusing on this transition shows that puberty has a major impact on the composition of the nasal microbiota. Significant differences are present in the nasal microbiota diversity, showing that Proteobacteria and Firmicutes are predominant in prepubertal children, while Actinobacteria are predominant in adults (Oh et al., 2012). Biswas and coworkers studied the association between olfactory dysfunction and nasal bacterial communities. No significant differences were observed in bacterial diversity among the three cohorts; however, the relative numbers of Corynebacterium spp. and Streptococcus spp. were significantly different in people with olfactory loss (Biswas et al., 2020). Butyrate-producing Faecalibacterium or Porphyromonas have been strongly associated with reduced olfactory function (Koskinen et al., 2018).
The oral cavity is a niche for over 700 microbial species, including bacteria, fungi and viruses (Lamont et al., 2018). This oral microbiota was shown to modulate both taste and aroma perception through several recently reviewed mechanisms (Schwartz et al., 2021a). The production and processing of tongue biofilm metabolites play an important role in taste modulation. This can be explained by two potential mechanisms of perireceptor modulation: first, bacteria can prevent access of taste molecules to taste receptors; second, bacterial metabolism produces compounds that can impact taste receptor activation and taste sensitivity (Fluitman et al., 2021; Leung and Covasa, 2021). Oral bacteria consuming sugars and amino acids reduce the availability of these compounds around taste buds (Gardner et al., 2020). Conversely, bacteria such as Veillonella, Lactobacillus and Actinomyces synthesize organic acids and short-chain fatty acids, thus increasing their concentration within the saliva (Takahashi, 2015). Feng and coworkers found that increased proportions of Actinomyces and Firmicutes in saliva were associated with reduced taste sensitivity, while increased taste sensitivity was the result of higher proportions of Bacteroides on the tongue membrane (Feng et al., 2018).
Oral microorganisms have been shown to metabolize precursor compounds present in foods to generate aroma molecules in the mouth. Glycoside conjugates are metabolized by bacteria such as Prevotella and Veillonella species associated with increased glycoside hydrolysis (Parker et al., 2020). This reaction leads to the release of aroma compounds such as terpenes, aromatic derivatives or alcohols (Mayr et al., 2014; Munoz-Gonzalez et al., 2014). Cysteine conjugates are metabolized to their corresponding thiols by C-S lyases of oral anaerobes such as Fusobacterium nucleatum (Starkenmann et al., 2008a; Neiers et al., 2022). Thiol release participates in the flavor of some vegetables and fruits (Starkenmann et al., 2008b) but also in the typicity of Sauvignon white wine (Tominaga et al., 1998). Furthermore, the oral microbiota was shown to be related to scarce olfactory performance and neophobia (Valentino et al., 2022).
As the microbiota composition seems to be associated with the physiopathological state of the individual, these states are also linked to olfactogustative modifications. The investigation of microbiota and their linkages with chemoperception is a challenging task, and more studies are needed to clarify these relationships.
Conclusion
Perireceptor events occurring in the mouth and nose appear to be very diverse based on the growing literature on the topic. They encompass various molecular events related to host physiology and health status. In the future, several challenges will be addressed, and a deeper understanding of these events in link with flavor perception will be attained. These challenges include the study of perireceptor events as close as possible to the real conditions of food consumption using in vivo studies and the integration of the different types of perireceptor events to consider crossmodal mechanisms. In this regard, special emphasis must be placed on the dynamics of perception and the different molecular partners. This will shed light on the metabolic images of flavor compounds. These partners include compounds from the human oral/nasal sphere but probably also molecules and microorganisms from the food, adding additional variables to this complex network of interactions that modulates receptor activation and in fine flavor perception.
Author contributions
All authors listed have made a substantial, direct, and intellectual contribution to the work and approved it for publication.
Funding
MS acknowledges grants from the Région Bourgogne Franche-Comté (ANER MétabolArôme Mathieu Schwartz).
Conflict of interest
The authors declare that the research was conducted in the absence of any commercial or financial relationships that could be construed as a potential conflict of interest.
Publisher’s note
All claims expressed in this article are solely those of the authors and do not necessarily represent those of their affiliated organizations, or those of the publisher, the editors and the reviewers. Any product that may be evaluated in this article, or claim that may be made by its manufacturer, is not guaranteed or endorsed by the publisher.
References
Aji, G. K., Warren, F. J., and Roura, E. (2019). Salivary α-amylase activity and starch-related sweet taste perception in humans. Chem. Senses 44, 249–256. doi:10.1093/chemse/bjz010
Aybeke, E. N., Ployon, S., Brule, M., De Fonseca, B., Bourillot, E., Morzel, M., et al. (2019). Nanoscale mapping of the physical surface properties of human buccal cells and changes induced by saliva. Langmuir 35, 12647–12655. doi:10.1021/acs.langmuir.9b01979
Bader, M., Dunkel, A., Wenning, M., Kohler, B., Medard, G., del Castillo, E., et al. (2018a). Dynamic proteome alteration and functional modulation of human saliva induced by dietary chemosensory stimuli. J. Agric. Food Chem. 66, 5621–5634. doi:10.1021/acs.jafc.8b02092
Bader, M., Stolle, T., Jennerwein, M., Hauck, J., Sahin, B., and Hofmann, T. (2018b). Chemosensate-induced modulation of the salivary proteome and metabolome alters the sensory perception of salt taste and odor-active thiols. J. Agric. Food Chem. 66, 7740–7749. doi:10.1021/acs.jafc.8b02772
Barbarossa, I. T., Melis, M., Mattes, M. Z., Calò, C., Muroni, P., Crnjar, R., et al. (2015). The gustin (CA6) gene polymorphism, rs2274333 (A/G), is associated with fungiform papilla density, whereas PROP bitterness is mostly due to TAS2R38 in an ethnically-mixed population. Physiol. Behav. 138, 6–12. doi:10.1016/j.physbeh.2014.09.011
Bessac, B. F., and Jordt, S.-E. (2008). Breathtaking TRP channels: TRPA1 and TRPV1 in airway chemosensation and reflex control. Physiology 23, 360–370. doi:10.1152/physiol.00026.2008
Biswas, K., Wagner Mackenzie, B., Ballauf, C., Draf, J., Douglas, R. G., and Hummel, T. (2020). Loss of bacterial diversity in the sinuses is associated with lower smell discrimination scores. Sci. Rep. 10, 16422. doi:10.1038/s41598-020-73396-3
Bongaerts, J. H. H., Rossetti, D., and Stokes, J. R. (2007). The lubricating properties of human whole saliva. Tribol. Lett. 27, 277–287. doi:10.1007/s11249-007-9232-y
Brooks, S. M. (2011). Perspective on the human cough reflex. Cough 7, 10. doi:10.1186/1745-9974-7-10
Buettner, A. (2002a). Influence of human saliva on odorant concentrations. 2. aldehydes, alcohols, 3-alkyl-2-methoxypyrazines, methoxyphenols, and 3-hydroxy-4, 5-dimethyl-2(5H)-furanone. J. Agric. Food Chem. 50, 7105–7110. doi:10.1021/jf020714o
Buettner, A. (2002b). Influence of human salivary enzymes on odorant concentration changes occurring in vivo. 1. Esters and thiols. J. Agric. Food Chem. 50, 3283–3289. doi:10.1021/jf011586r
Cabiddu, G., Maes, P., Hyvrier, F., Olianas, A., Manconi, B., Brignot, H., et al. (2020). Proteomic characterization of the mucosal pellicle formed in vitro on a cellular model of oral epithelium. J. Proteomics 222, 103797. doi:10.1016/j.jprot.2020.103797
Caceres, A. I., Brackmann, M., Elia, M. D., Bessac, B. F., del Camino, D., D’Amours, M., et al. (2009). A sensory neuronal ion channel essential for airway inflammation and hyperreactivity in asthma. Proc. Natl. Acad. Sci. U. S. A. 106, 9099–9104. doi:10.1073/pnas.0900591106
Canon, F., Ballivian, R., Chirot, F., Antoine, R., Sarni-Manchado, P., Lemoine, J., et al. (2011). Folding of a salivary intrinsically disordered protein upon binding to tannins. J. Am. Chem. Soc. 133, 7847–7852. doi:10.1021/ja200534f
Canon, F., Belloir, C., Bourillot, E., Brignot, H., Briand, L., Feron, G., et al. (2021). Perspectives on astringency sensation: An alternative hypothesis on the molecular origin of astringency. J. Agric. Food Chem. 69, 3822–3826. doi:10.1021/acs.jafc.0c07474
Canon, F., Neiers, F., and Guichard, E. (2018). Saliva and flavor perception: Perspectives. J. Agric. Food Chem. 66, 7873–7879. doi:10.1021/acs.jafc.8b01998
Canon, F., Paté, F., Cheynier, V., Sarni-Manchado, P., Giuliani, A., Pérez, J., et al. (2013). Aggregation of the salivary proline-rich protein IB5 in the presence of the tannin EgCG. Langmuir 29, 1926–1937. doi:10.1021/la3041715
Canon, F., Ployon, S., Mazauric, J.-P., Sarni-Manchado, P., Réfrégiers, M., Giuliani, A., et al. (2015). Binding site of different tannins on a human salivary proline-rich protein evidenced by dissociative photoionization tandem mass spectrometry. Tetrahedron 71, 3039–3044. doi:10.1016/j.tet.2014.11.013
Dawes, C., Pedersen, A. M., Villa, A., Ekstrom, J., Proctor, G. B., Vissink, A., et al. (2015). The functions of human saliva: A review sponsored by the world workshop on oral medicine VI. Arch. Oral Biol. 60, 863–874. doi:10.1016/j.archoralbio.2015.03.004
Denny, P., Hagen, F. K., Hardt, M., Liao, L., Yan, W., Arellanno, M., et al. (2008). The proteomes of human parotid and submandibular/sublingual gland salivas collected as the ductal secretions. J. Proteome Res. 7, 1994. doi:10.1021/pr700764j
Dinnella, C., Recchia, A., Fia, G., Bertuccioli, M., and Monteleone, E. (2009). Saliva characteristics and individual sensitivity to phenolic astringent stimuli. Chem. Senses 34, 295–304. doi:10.1093/chemse/bjp003
Dinnella, C., Recchia, A., Tuorila, H., and Monteleone, E. (2011). Individual astringency responsiveness affects the acceptance of phenol-rich foods. Appetite 56, 633–642. doi:10.1016/j.appet.2011.02.017
Dsamou, M., Palicki, O., Septier, C., Chabanet, C., Lucchi, G., Ducoroy, P., et al. (2012). Salivary protein profiles and sensitivity to the bitter taste of caffeine. Chem. Senses 37, 87–95. doi:10.1093/chemse/bjr070
Engelen, L., and Van Der Bilt, A. (2008). Oral physiology and texture perception of semisolids. J. Texture Stud. 39, 83–113. doi:10.1111/j.1745-4603.2007.00132.x
Fábián, T., Beck, A., Fejérdy, P., Hermann, P., and Fábián, G. (2015). Molecular mechanisms of taste recognition: Considerations about the role of saliva. Int. J. Mol. Sci. 16, 5945–5974. doi:10.3390/ijms16035945
Fabrini, R., Bocedi, A., Camerini, S., Fusetti, M., Ottaviani, F., Passali, F. M., et al. (2014). Inactivation of human salivary glutathione transferase P1-1 by hypothiocyanite: A post-translational control system in search of a role. PLoS One 9, e112797. doi:10.1371/journal.pone.0112797
Feng, Y., Licandro, H., Martin, C., Septier, C., Zhao, M., Neyraud, E., et al. (2018). The associations between biochemical and microbiological variables and taste differ in whole saliva and in the film lining the tongue. Biomed. Res. Int. 1, 2838052. doi:10.1155/2018/2838052
Fluitman, K. S., van den Broek, T. J., Nieuwdorp, M., Visser, M., Ijzerman, R. G., and Keijser, B. J. F. (2021). Associations of the oral microbiota and Candida with taste, smell, appetite and undernutrition in older adults. Sci. Rep. 11, 23254. doi:10.1038/s41598-021-02558-8
Gardner, A., So, P. W., and Carpenter, G. H. (2020). Intraoral microbial metabolism and association with host taste perception. J. Dent. Res. 99, 739–745. doi:10.1177/0022034520917142
Giebultowicz, J., Wolinowska, R., Sztybor, A., Pietrzak, M., Wroczynski, P., and Wierzchowski, J. (2009). Salivary aldehyde dehydrogenase: Activity towards aromatic aldehydes and comparison with recombinant ALDH3A1. Molecules 14, 2363–2372. doi:10.3390/molecules14072363
Gilbertson, T. A. (1998). Gustatory mechanisms for the detection of fat. Curr. Opin. Neurobiol. 8, 447–452. doi:10.1016/S0959-4388(98)80030-5
Glendinning, J. I. (1992). Effect of salivary proline-rich proteins on ingestive responses to tannic acid in mice. Chem. Senses 17, 1–12. doi:10.1093/chemse/17.1.1
Henkin, R. I., Martin, B. M., and Agarwal, R. P. (1999). Decreased parotid saliva gustin/carbonic anhydrase VI secretion: An enzyme disorder manifested by gustatory and olfactory dysfunction. Am. J. Med. Sci. 318, 380–391. doi:10.1097/00000441-199912000-00005
Heydel, J.-M., Coelho, A., Thiebaud, N., Legendre, A., Bon, A.-M. L., Faure, P., et al. (2013). Odorant-binding proteins and xenobiotic metabolizing enzymes: Implications in olfactory perireceptor events. Anat. Rec. 296, 1333–1345. doi:10.1002/ar.22735
Heydel, J. M., Faure, P., and Neiers, F. (2019). Nasal odorant metabolism: Enzymes, activity and function in olfaction. Drug Metab. Rev. 51, 224–245. doi:10.1080/03602532.2019.1632890
Hinman, A., Chuang, H., Bautista, D. M., and Julius, D. (2006). TRP channel activation by reversible covalent modification. Proc. Natl. Acad. Sci. U. S. A. 103, 19564–19568. doi:10.1073/pnas.0609598103
Ijichi, C., Wakabayashi, H., Sugiyama, S., Hayashi, K., Ihara, Y., Nishijima, H., et al. (2022). Odorant metabolism of the olfactory cleft mucus in idiopathic olfactory impairment patients and healthy volunteers. Int. Forum Allergy Rhinol. 12, 293–301. doi:10.1002/alr.22897
Ijichi, C., Wakabayashi, H., Sugiyama, S., Ihara, Y., Nogi, Y., Nagashima, A., et al. (2019). Metabolism of odorant molecules in human nasal/oral cavity affects the odorant perception. Chem. Senses 44, 465–481. doi:10.1093/chemse/bjz041
Kornbausch, N., Debong, M. W., Buettner, A., Heydel, J.-M., and Loos, H. (2022). Odorant metabolism in humans. Angew. Chem. Int. Ed. Engl. 61, 202202866. doi:10.1002/anie.202202866
Koskinen, K., Reichert, J. L., Hoier, S., Schachenreiter, J., Duller, S., Moissl-Eichinger, C., et al. (2018). The nasal microbiome mirrors and potentially shapes olfactory function. Sci. Rep. 8, 1296. doi:10.1038/s41598-018-19438-3
Kudo, H., Doi, Y., and Fujimoto, S. (2010). Expressions of the multidrug resistance-related proteins in the rat olfactory epithelium: A possible role in the phase III xenobiotic metabolizing function. Neurosci. Lett. 468, 98–101. doi:10.1016/j.neulet.2009.10.073
Lacazette, E., Gachon, A. M., and Pitiot, G. (2000). A novel human odorant-binding protein gene family resulting from genomic duplicons at 9q34: Differential expression in the oral and genital spheres. Hum. Mol. Genet. 9, 289–301. doi:10.1093/hmg/9.2.289
Lamont, R. J., Koo, H., and Hajishengallis, G. (2018). The oral microbiota: Dynamic communities and host interactions. Nat. Rev. Microbiol. 16, 745–759. doi:10.1038/s41579-018-0089-x
Leclerc, S., Heydel, J.-M., Amossé, V., Gradinaru, D., Cattarelli, M., Artur, Y., et al. (2002). Glucuronidation of odorant molecules in the rat olfactory system. Activity, expression and age-linked modifications of UDP-glucuronosyltransferase isoforms, UGT1A6 and UGT2A1, and relation to mitral cell activity. Brain Res. Mol. Brain Res. 107, 201–213. doi:10.1016/S0169-328X(02)00455-2
Macpherson, L. J., Dubin, A. E., Evans, M. J., Marr, F., Schultz, P. G., Cravatt, B. F., et al. (2007). Noxious compounds activate TRPA1 ion channels through covalent modification of cysteines. Nature 445, 541–545. doi:10.1038/nature05544
Mallery, S. R., Budendorf, D. E., Larsen, M. P., Pei, P., Tong, M., Holpuch, A. S., et al. (2011). Effects of human oral mucosal tissue, saliva, and oral microflora on intraoral metabolism and bioactivation of black raspberry anthocyanins. Cancer Prev. Res. 4, 1209–1221. doi:10.1158/1940-6207.CAPR-11-0040
Martin, L. E., Kay, K. E., and Torregrossa, A.-M. (2019). Bitter-induced salivary proteins increase detection threshold of quinine, but not sucrose. Chem. Senses 44, 379–388. doi:10.1093/chemse/bjz021
Mayr, C. M., Parker, M., Baldock, G. A., Black, C. A., Pardon, K. H., Williamson, P. O., et al. (2014). Determination of the importance of in-mouth release of volatile phenol glycoconjugates to the flavor of smoke-tainted wines. J. Agric. Food Chem. 62, 2327–2336. doi:10.1021/jf405327s
Mounayar, R., Septier, C., Chabanet, C., Feron, G., and Neyraud, E. (2013). Oral fat sensitivity in humans: Links to saliva composition before and after stimulation by oleic acid. Chem. Percept. 6, 118–126. doi:10.1007/s12078-013-9152-1
Munoz-Gonzalez, C., Brule, M., Feron, G., and Canon, F. (2019). Does interindividual variability of saliva affect the release and metabolization of aroma compounds ex vivo? The particular case of elderly suffering or not from hyposalivation. J. Texture Stud. 50, 36–44. doi:10.1111/jtxs.12382
Munoz-Gonzalez, C., Brule, M., Martin, C., Feron, G., and Canon, F. (2021a). Molecular mechanisms of aroma persistence: From noncovalent interactions between aroma compounds and the oral mucosa to metabolization of aroma compounds by saliva and oral cells. Food Chem. 131467. doi:10.1016/j.foodchem.2021.131467
Muñoz-González, C., Canon, F., Feron, G., Guichard, E., and Pozo-Bayón, M. (2019). Assessment wine aroma persistence by using an in vivo PTR-ToF-MS approach and its relationship with salivary parameters. Molecules 24, 1277. doi:10.3390/molecules24071277
Munoz-Gonzalez, C., Feron, G., Brule, M., and Canon, F. (2018). Understanding the release and metabolism of aroma compounds using micro-volume saliva samples by ex vivo approaches. Food Chem. 240, 275–285. doi:10.1016/j.foodchem.2017.07.060
Munoz-Gonzalez, C., Feron, G., and Canon, F. (2021b). Physiological and oral parameters contribute prediction of retronasal aroma release in an elderly cohort. Food Chem. 342, 128355. doi:10.1016/j.foodchem.2020.128355
Munoz-Gonzalez, C., Feron, G., Guichard, E., Rodriguez-Bencomo, J. J., Martin-Alvarez, P. J., Moreno-Arribas, M. V., et al. (2014). Understanding the role of saliva in aroma release from wine by using static and dynamic headspace conditions. J. Agric. Food Chem. 62, 8274–8288. doi:10.1021/jf503503b
Neiers, F., Gourrat, K., Canon, F., and Schwartz, M. (2022). Metabolism of cysteine conjugates and production of flavor sulfur compounds by a carbon–sulfur lyase from the oral anaerobe Fusobacterium nucleatum. J. Agric. Food Chem. 70, 9969–9979. doi:10.1021/acs.jafc.2c01727
Neiers, F., Jarriault, D., Menetrier, F., Briand, L., and Heydel, J.-M. (2021). The odorant metabolizing enzyme UGT2A1: Immunolocalization and impact of the modulation of its activity on the olfactory response. PLoS ONE 16, e0249029. doi:10.1371/journal.pone.0249029
Oh, J., Conlan, S., Polley, E. C., Segre, J. A., and Kong, H. H. (2012). Shifts in human skin and nares microbiota of healthy children and adults. Genome Med. 4, 77. doi:10.1186/gm378
Pages-Helary, S., Andriot, I., Guichard, E., and Canon, F. (2014). Retention effect of human saliva on aroma release and respective contribution of salivary mucin and alpha-amylase. Food Res. Int. 64, 424–431. doi:10.1016/j.foodres.2014.07.013
Parker, M., Onetto, C., Hixson, J., Bilogrevic, E., Schueth, L., Pisaniello, L., et al. (2020). Factors contributing to interindividual variation in retronasal odor perception from aroma glycosides: The role of odorant sensory detection threshold, oral microbiota, and hydrolysis in saliva. J. Agric. Food Chem. 68, 10299–10309. doi:10.1021/acs.jafc.9b05450
Patapoutian, A., Tate, S., and Woolf, C. J. (2009). Transient receptor potential channels: Targeting pain at the source. Nat. Rev. Drug Discov. 8, 55–68. doi:10.1038/nrd2757
Pelosi, P., and Knoll, W. (2022). Odorant‐binding proteins of mammals. Biol. Rev. Camb. Philos. Soc. 97, 20–44. doi:10.1111/brv.12787
Piombino, P., Genovese, A., Esposito, S., Moio, L., Cutolo, P. P., Chambery, A., et al. (2014). Saliva from obese individuals suppresses the release of aroma compounds from wine. PLoS One 9, e85611. doi:10.1371/journal.pone.0085611
Ployon, S., Brule, M., Andriot, I., Morzel, M., and Canon, F. (2020). Understanding retention and metabolization of aroma compounds using an in vitro model of oral mucosa. Food Chem. 318, 126468. doi:10.1016/j.foodchem.2020.126468
Ployon, S., Morzel, M., Belloir, C., Bonnotte, A., Bourillot, E., Briand, L., et al. (2018). Mechanisms of astringency: Structural alteration of the oral mucosal pellicle by dietary tannins and protective effect of bPRPs. Food Chem. 253, 79–87. doi:10.1016/j.foodchem.2018.01.141
Ployon, S., Morzel, M., and Canon, F. (2017). The role of saliva in aroma release and perception. Food Chem. 226, 212–220. doi:10.1016/j.foodchem.2017.01.055
Robert-Hazotte, A., Faure, P., Ménétrier, F., Folia, M., Schwartz, M., Le Quéré, J.-L., et al. (2022). Nasal odorant competitive metabolism is involved in the human olfactory process. J. Agric. Food Chem. 70, 8385–8394. doi:10.1021/acs.jafc.2c02720
Robert-Hazotte, A., Faure, P., Neiers, F., Potin, C., Artur, Y., Coureaud, G., et al. (2019). Nasal mucus glutathione transferase activity and impact on olfactory perception and neonatal behavior. Sci. Rep. 9, 3104. doi:10.1038/s41598-019-39495-6
Rodrigues, L., Costa, G., Cordeiro, C., Pinheiro, C., Amado, F., and Lamy, E. (2017). Salivary proteome and glucose levels are related with sweet taste sensitivity in young adults. Food Nutr. Res. 61, 1389208. doi:10.1080/16546628.2017.1389208
Ruijschop, R. M. A. J., Boelrijk, A. E. M., de Graaf, C., and Westerterp-Plantenga, M. S. (2009). Retronasal aroma release and satiation: A review. J. Agric. Food Chem. 57, 9888–9894. doi:10.1021/jf901445z
Schwartz, M., Brignot, H., Feron, G., Hummel, T., Zhu, Y., von Koskull, D., et al. (2022). Role of human salivary enzymes in bitter taste perception. Food Chem. 386, 132798. doi:10.1016/j.foodchem.2022.132798
Schwartz, M., Canon, F., Feron, G., Neiers, F., and Gamero, A. (2021a). Impact of oral microbiota on flavor perception: From food processing to in-mouth metabolization. Foods 10, 2006. doi:10.3390/foods10092006
Schwartz, M., Menetrier, F., Heydel, J. M., Chavanne, E., Faure, P., Labrousse, M., et al. (2020a). Interactions between odorants and glutathione transferases in the human olfactory cleft. Chem. Senses 45, 645–654. doi:10.1093/chemse/bjaa055
Schwartz, M., Neiers, F., Charles, J. P., Heydel, J. M., Munoz-Gonzalez, C., Feron, G., et al. (2021b). Oral enzymatic detoxification system: Insights obtained from proteome analysis to understand its potential impact on aroma metabolization. Compr. Rev. Food Sci. Food Saf. 20, 5516–5547. doi:10.1111/1541-4337.12857
Schwartz, M., Neiers, F., Feron, G., and Canon, F. (2020b). The relationship between salivary redox, diet, and food flavor perception. Front. Nutr. 7, 612735. doi:10.3389/fnut.2020.612735
Shelton, J. F., Shastri, A. J., Fletez-Brant, K., Aslibekyan, S., Auton, A., et al. The 23andMe COVID-19 Team. (2022). The UGT2A1/UGT2A2 locus is associated with COVID-19-related loss of smell or taste. Nat. Genet. 54, 121–124. doi:10.1038/s41588-021-00986-w
Sivadasan, P., Gupta, M. K., Sathe, G. J., Balakrishnan, L., Palit, P., Gowda, H., et al. (2015). Human salivary proteome--a resource of potential biomarkers for oral cancer. J. Proteomics 127, 89–95. doi:10.1016/j.jprot.2015.05.039
Soares, S., Mateus, N., and de Freitas, V. (2012). Interaction of different classes of salivary proteins with food tannins. Food Res. Int. 49, 807–813. doi:10.1016/j.foodres.2012.09.008
Soares, S., Vitorino, R., Osório, H., Fernandes, A., Venâncio, A., Mateus, N., et al. (2011). Reactivity of human salivary proteins families toward food polyphenols. J. Agric. Food Chem. 59, 5535–5547. doi:10.1021/jf104975d
Sollai, G., Melis, M., Magri, S., Usai, P., Hummel, T., Tomassini Barbarossa, I., et al. (2019). Association between the rs2590498 polymorphism of Odorant Binding Protein (OBPIIa) gene and olfactory performance in healthy subjects. Behav. Brain Res. 372, 112030. doi:10.1016/j.bbr.2019.112030
Sollai, G., Melis, M., Tomassini Barbarossa, I., and Crnjar, R. (2022). A polymorphism in the human gene encoding OBPIIa affects the perceived intensity of smelled odors. Behav. Brain Res. 427, 113860. doi:10.1016/j.bbr.2022.113860
Sreerama, L., Hedge, M. W., and Sladek, N. E. (1995). Identification of a class 3 aldehyde dehydrogenase in human saliva and increased levels of this enzyme, glutathione S-transferases, and DT-diaphorase in the saliva of subjects who continually ingest large quantities of coffee or broccoli. Clin. Cancer Res. 1, 1153–1163.
Starkenmann, C., Le Calve, B., Niclass, Y., Cayeux, I., Beccucci, S., and Troccaz, M. (2008a). Olfactory perception of cysteine-S-conjugates from fruits and vegetables. J. Agric. Food Chem. 56, 9575–9580. doi:10.1021/jf801873h
Starkenmann, C., Troccaz, M., and Howell, K. (2008b). The role of cysteine and cysteine-S conjugates as odour precursors in the flavour and fragrance industry. Flavour Fragr. J. 23, 369–381. doi:10.1002/ffj.1907
Stolle, T., Grondinger, F., Dunkel, A., and Hofmann, T. (2018). Quantitative proteomics and SWATH-MS to elucidate peri-receptor mechanisms in human salt taste sensitivity. Food Chem. 254, 95–102. doi:10.1016/j.foodchem.2018.01.160
Su, C.-Y., Menuz, K., and Carlson, J. R. (2009). Olfactory perception: Receptors, cells, and circuits. Cell 139, 45–59. doi:10.1016/j.cell.2009.09.015
Takahashi, N. (2015). Oral microbiome metabolism: From “who are they?” To “what are they doing?”. J. Dent. Res. 94, 1628–1637. doi:10.1177/0022034515606045
Thiebaud, N., Menetrier, F., Belloir, C., Minn, A.-L., Neiers, F., Artur, Y., et al. (2011). Expression and differential localization of xenobiotic transporters in the rat olfactory neuro-epithelium. Neurosci. Lett. 505, 180–185. doi:10.1016/j.neulet.2011.10.018
Thiebaud, N., Veloso Da Silva, S., Jakob, I., Sicard, G., Chevalier, J., Menetrier, F., et al. (2013). Odorant metabolism catalyzed by olfactory mucosal enzymes influences peripheral olfactory responses in rats. PLoS One 8, e59547. doi:10.1371/journal.pone.0059547
Tominaga, T., des Gachons, C. P., and Dubourdieu, D. (1998). A new type of flavor precursors in vitis vinifera L cv Sauvignon blanc: S-Cysteine conjugates. J. Agric. Food Chem. 46, 5215–5219. doi:10.1021/jf980481u
Valentino, V., De Filippis, F., Menghi, L., Gasperi, F., and Ercolini, D. (2022). Food Neophobia and scarce olfactory performances are linked to oral microbiota. Food Res. Int. 111092. doi:10.1016/j.foodres.2022.111092
Walliczek-Dworschak, U., Schöps, F., Feron, G., Brignot, H., Hähner, A., and Hummel, T. (2017). Differences in the density of fungiform papillae and composition of saliva in patients with taste disorders compared to healthy controls. Chem. Senses 42, 699–708. doi:10.1093/chemse/bjx054
Yakubov, G. E., Macakova, L., Wilson, S., Windust, J. H. C., and Stokes, J. R. (2015). Aqueous lubrication by fractionated salivary proteins: Synergistic interaction of mucin polymer brush with low molecular weight macromolecules. Tribol. Int. 89, 34–45. doi:10.1016/j.triboint.2014.12.025
Yarmolinsky, D. A., Zuker, C. S., and Ryba, N. J. P. (2009). Common sense about taste: From mammals to insects. Cell 139, 234–244. doi:10.1016/j.cell.2009.10.001
Zhang, D., Jiang, H., Chen, J., and Wang, X. (2022). Buffering capacity of saliva influences the perception of acid-related sensory properties. Food Qual. Prefer. 97, 104454. doi:10.1016/j.foodqual.2021.104454
Keywords: flavor, enzymes, aroma, taste, trigeminal, perireceptor events, odorant
Citation: Boichot V, Muradova M, Nivet C, Proskura A, Heydel J-M, Canivenc-Lavier M-C, Canon F, Neiers F and Schwartz M (2022) The role of perireceptor events in flavor perception. Front. Food. Sci. Technol. 2:989291. doi: 10.3389/frfst.2022.989291
Received: 08 July 2022; Accepted: 03 October 2022;
Published: 19 October 2022.
Edited by:
José S. Câmara, Universidade da Madeira, PortugalReviewed by:
Zheng Qiao, University of Colorado Anschutz Medical Campus, United StatesCopyright © 2022 Boichot, Muradova, Nivet, Proskura, Heydel, Canivenc-Lavier, Canon, Neiers and Schwartz. This is an open-access article distributed under the terms of the Creative Commons Attribution License (CC BY). The use, distribution or reproduction in other forums is permitted, provided the original author(s) and the copyright owner(s) are credited and that the original publication in this journal is cited, in accordance with accepted academic practice. No use, distribution or reproduction is permitted which does not comply with these terms.
*Correspondence: Mathieu Schwartz, bWF0aGlldS5zY2h3YXJ0ekBpbnJhZS5mcg==