- 1Facultad de Ingenieria, Universidad de La Sabana, Bogotá, Colombia
- 2Departamento de Innovación, Drycol S.A.S, Madrid, Colombia
Production of probiotics at industrial scale to be used as food supplements involves diverse kinds of processes such as selection of microorganisms, 2) fermentation, 3) drying, and the use of non-food grade commercial culture mediums that should be washed after obtaining the ideal cell concentration. Then, food grade culture medium for probiotics that do not need to be washed and protect the microorganisms through the gastrointestinal tract, is proposed as a low-cost alternative to grow probiotics to be used for incorporation in food. In this way, an experimental design was carried out to obtain the operative conditions for spray drying food-grade culture medium at industrial scale. The factors evaluated were the inlet and outlet temperature and a specific heat treatment before the drying process. As response variables the physical properties of the food-grade medium in powder and the viability count of the probiotic Limosilactobacillus fermentum K73 were evaluated. The viability of four different known probiotics (Limosilactobacillus fermentum, Lactobacillus delbruekii, Lacticaseibacillus rhamnosus and Enterococcus faecium) was proved which resulted after 10 h in 10.6, 10.2, 10.1, and 11.2 logCFU/ml, respectively. Also, in vitro digestions of the probiotics in this food-grade medium were carried out and compared with digestions of the probiotics in the commercial medium MRS, demonstrating that the proposed medium protect the probiotics of the gastrointestinal conditions, allowing them to arrive at the intestine at 7.3, 6.8, 7.5 and 7 logCFU/mL, for L. fermentum, L. delbruekii, L. rhamnosus and E. faecium, respectively. Finally, the optimal conditions of the spray drying process were used to reproduce the whey food-grade medium at a large-scale spray dryer (dimensions), this to validate the medium performance in the production of powdered probiotics as L. fermentum.
Introduction
Nowadays whey is one of the most valuables secondary bioresources obtained from the production of cheese. However, the companies that reuse or sell this product in the world are just a minority. This situation leaves whey still to be a contaminant which should be treated before disposed. Sweet whey from cheese production is composed of around 0.6% of serum proteins, 0.45% of minerals, 4.5% of lactose and aminoacids and vitamins in lower concentration (WHEY PROCESSING, 2022). These characteristics make this whey to be suitable for the growth of probiotics and the development of new products (Tsermoula et al., 2021).
In the last decade, the world probiotic market was valued at 34.1 billion of dollars, and it is estimated to reach 73.9 billion by 2030 (https://www.alliedmarketresearch.com/probiotics-market). This created the need of scale up the probiotic production processes, rising the costs of production and the difficulty of carry out some of the processes contemplated in the upstream and downstream to obtain the commercial probiotics (Huang et al., 2016). Among them, the selection of the growth medium for producing commercial amounts of probiotics depends on costs, the efficiency of cell production, and ease of harvest. For microorganism’s production on an industrial scale, the cell concentration and time are taken to reach that cell amount has a significant impact on the cost. De Man, Rogosa and Sharpe agar MRS broth and Reinforced Clostridial Agar RCM are the conventional medium for probiotic production, but its high cost, the additional concentration and washing process that should be carried for their toxicity for human ingestion, make them unrentable for the industry (Huang et al., 2016).
On the other hand, whey had been evaluated as a culture medium for probiotics because of it contains carbohydrates, fat, casein protein, vitamins, minerals and low production cost (Drgalić et al., 2005; Koutinas et al., 2009; Huang et al., 2017; Krunić et al., 2019). Nevertheless, this matrix does not have free amino acids and small peptides that decreasing the possibility for a microorganism to grow. Therefore, a source of these compounds must be added to supplement the matrix and feed adequately the microorganism to grow. Yeast extract is the stock option used to add the missing compounds and improve the presence of nitrogen, but it can also be used as a mixture of alanine, serine, isoleucine, and cysteine to such propose (Aragón-Rojas et al., 2018; Goveas and Sajankila, 2020; Huynh et al., 2020). Studies had shown that its physicochemical composition provides the probiotics with high concentration of not only nitrogen sources but prebiotics to promote their growth, as also the environment needed to survive on long-term. A lot of culture media produced at lab scale could be found in literature (Burgain et al., 2013; Huang et al., 2017; Sharma et al., 2017; Aragón-Rojas et al., 2018; Krunić et al., 2019), however, there are not works that evaluate their reproducibility and functionality at higher scales.
One of the processes to help probiotics to use nutrients and proteins of whey is the thermal treatment. In this case, thermal treatment of whey denatures the globular proteins which result in the unfolding of the tertiary structure of proteins and altering the hydrophobic and covalent interaction of the protein structure, this change in the structure allows the aminoacids to be more available for be used by probiotics (Abd El-Salam and El-Shibiny, 2015; Aragón-Rojas et al., 2018; Krunić et al., 2019). In addition, the denaturalization of whey proteins facilitates the entrapment of microorganism in the whey structure, the above conferees protection to the microorganisms from encapsulation processes (Doherty et al., 2012; Bosnea et al., 2014; Pereira et al., 2018).
Powder production could be carried out by spray drying. This technique is used to transform liquid substances into powders quickly and efficiently. The speed of the process and the drying time is short, consequently it allows the drying of temperature-sensitive products, even without degradation. In addition, this technique is also widely used to improve the preservation of the product in dry solid form (Geranpour et al., 2020). Thus, the aim of this study was to scale-up a food-grade culture medium composed of whey and evaluate its protection for probiotics against gastrointestinal conditions using an in vitro digestion model.
Materials and Methods
Materials
Sweet whey powder (Saputo, protein 11%, fat 1.5%, lactose 69.5% (w/w)) and Yeast Extract were purchased from DYM S.A.S. (Colombia). Food-grade non-purified soy lecithin was bought from BellChem. S.A.S (Colombia) and Maltodextrin 1910 was obtained from INTERKROL S.A. (Colombia). Pepsin from porcine gastric mucosa (P6887), Pancreatic Lipase (L3126) and Pancreatin (P7545), both from porcine pancreas, as well as Ox-Bile (70,168), were purchased from Sigma-Aldrich (Saint Louis, MO, United States) and used in the in vitro assays. Analytical grade salts used in the electrolyte solutions were purchased from Panreac (Barcelona, Spain). MRS broth and agar were obtained from scharlau (Spain)
Strains
Probiotic Limosilactobacillus fermentum K73 (GenBank KP784433) was isolated from Colombian typical food. Strains Lactobacillus delbrukkii ssp. lactis (ATCC 12315), Enterococcus faecium (ATCC 35667) and L. rhamnosus (ATCC 7469), were obtained from ATCC. All the strains were maintained in -80 °C until its use.
Production of Dried Food-Grade Culture Medium for Probiotics
A Response Surface experimental design was carried out to find the optimal condition to obtain food-grade culture medium powder by spray drying (Mobile MinorTM, GEA) with a evaporation water capacity of 5 kg/h. The formulation of the food-grade culture medium was described previously by Aragon- rojas et al.(Aragón-Rojas et al., 2018). Briefly, 2.2 g/L of yeast extract was added to a mixture of 80 g/L of whey powder in distilled water. The numerical factors considered for the experimental design (Table 1) were: the inlet and outlet temperatures of the drying process, and as a categorical factor the previous thermal treatment of the culture medium mixture was considered, this thermal treatment was carried out at 121°C and 15 psi. The response variables were the physical properties of the powder such as moisture content, water activity and solubility, and the viability of the probiotic L. fermentum in the reconstituted media. For the last, the powder was reconstituted with distilled water to reach a concentration of 10% of solids (p/p), following by an adjustment of pH to 5.5 with HCL 1 M, and a thermal treatment at 121°C for 15 min, to guarantee the denaturalization of the proteins which confers protection to the microorganism as proposed by Aragón-Rojas et al. (2018). before inoculation with L. fermentum. The measurement of viability of the probiotic was carried out after 24 h of batch fermentations at 37 °C.
Design Expert® software (version 10.0.1.0) (Stat-Ease Inc., MN, United States) was used for statistical analysis, using Response Surface Experimental RSE design optimization. Design Expert® software’s graphical-numerical optimization method was used for optimizing response, using desirability criteria (Ricaurte et al., 2018): low content of moisture and water activity, and high solubility and viability.
Physical Properties of Dried Food-Grade Culture Medium
Water activity (Aw) was measured with an AquaLab4 device (Decagon Devices, Inc., United States). Moisture content (%H) was measured using an EM 120-HR moisture analyzer (Precisa Gravimetrics AG, Dietikon, Switzerland). Solubility was measured counting the time in which 1 g solubilizated completely on 100 ml of deionized water.
Viability Cell Count
Viability of probiotics were measured by cell count methodology on plate agar MRS after culturing during 24 h at 37°C. The cell counts are expressed as log CFU/ml and the change in bacterial cycles was calculated as the cell count before the experiment minus the cell counts after the experiment.
Scale-Up of Optimal Conditions of the Culture Media Drying Process
The scale-up of the drying process was carried out with the optimal conditions found by the RSE methodology, in a spray dryer PSA150 with a water evaporation capacity of 150 L/h (Vibrasec, Colombia). Briefly, 20 L of the culture medium prepared as mentioned above without the thermal treatment, were placed in the dryer’s feed tank and impulse at 18 Hz to the drying chamber. Here the inlet temperature was maintained constant at 150°C and the outlet temperature at 90°C. Then to validate the optimal conditions, the medium in powder was reconstituted in distilled water in a concentration to reach 10% of solids (p/p) and subjected to thermal treatment before inoculating the probiotic L. fermentum. After 24 h of fermentation, viability was measured by plate count methodology on MRS agar.
Growth Assay With Different Probiotic Strains
To validate the functionality of the food-grade culture medium in powder, batch fermentations with different probiotics were carried out and compared with the fermentation on MRS broth using the powder produced under the optimal conditions of the experimental design. Here, liquid culture medium was prepared by a mixture of the powder in a concentration of 8–10% (p/v) in solids in distilled water, followed by an autoclaving sterilization. MRS broth was prepared according to manofacturer’s instructions. The media were inoculated in a 10% v/v proportion and fermentations were performed at 37°C for 24 h. After fermentation viability was measured by plate count in MRS agar.
In-Vitro Digestion
Following the fermentations, an in vitro digestion assay was performed following the INFOGEST protocol proposed by Minekus et al. (Minekus et al., 2014) with some modifications by Mat et al. (2016). Table 2 shows the preparation of the simulated salivary (SSF), gastric (SGF) and intestinal (SIF) fluids. A sample of 5 ml of the fermentation was added to a 5 ml of SSF and the pH was adjusted to 7 with NaOH 1 M. The oral phase was put in a shaker at 100 rpm and 37°C for 2 min. After the oral phase, SGF was added to the mixture, including pepsin in a final concentration of 2000 U/ml; then pH was modified to reach a pH of 3 with HCl 1 M, and the mixture was at constant shaking at 100 rpm and 37°C for 2 h. When the gastric phase is over, it is mixed with SIF, bile extract 10 mM in the final concentration, pancreatin and pancreatic lipase, with a final concentration of 100 U/mL of trypsin and 2000 U/ml of lipase in the final mixture. pH was arranged to reach 7 with NaOH 1 M. Finally, the intestinal phase was shacked at 100 rpm and 37°C during 2 h.
At the end of each phase (oral, gastric, and intestinal) aliquots were taken and a viability assay was carried out in MRS agar plates. Viable colonies were count and results were expressed in log CFU/ml.
Scale Production of L. fermentum
As proposed before, culture medium in powder was prepared to carry out a fermentation of 80 L with an inoculum of 10% v/v. To encapsulate L. fermentum after 10 h of fermentation, wall material composed of whey and maltodextrin as proposed by Aragon-Rojas (Aragón-Rojas et al., 2020) was added to the probiotic fermentation until reach a mixture with 40% of solids. Later that mixture was feed to the industrial dryer used in 2.6 at inlet temperature of 175 and 80°C of outlet temperature, with a feed rate of 13 Hz. Viability of encapsulated probiotic was measured releasing it from the powder, briefly, 1 g of powder was diluted in 9 ml on an aqueous solution of peptone 2% (p/v), first letting it hydratate for 15 min and the vortexing for 10 min. After releasing, probiotics in the solution were cultured in agar plates of MRS and colony counts were carried out after 24 h. Finally in vitro digestion of encapsulated probiotics was carried out following the INFOGEST 1.0 protocol exposed before.
Statistical Analysis
The statistical analysis was performed using the response surface analysis for optimization in the Design Expert Software Version 10.1.0 (Stat-Ease Inc., MN, United States). A statistical significance test was used in the total error criteria with a confidence level of 95%. The significant terms in the model were found through analysis of variance (ANOVA). The fit of the model was evaluated by the R2 value.
Results and Discussion
Scale-Up of Drying Process of Food-Grade Culture Medium
An experimental design was carried out with inlet (Tin) and outlet (Tout) temperatures of the spray drying process and a thermal treatment (TT) as the factors to be evaluated, the response variables are presented in Table 3. In this case, response variables were the physical properties of the medium in powder as water activity, moisture content and time of solubilization, additional the ability to growth the microorganism was also considered to be a useful response variable measured in logCFU/ml. The RSE models obtained were accepted with a significance value under 0.05 (p < 0.05). In this way, linear models represented the influence of the factors on water activity, moisture content and solubility. As for the growth of the microorganism in the culture medium was a “FI model” which represents the influence of factors. All the models were obtained with a non-significative lack of fit (p > 0.05) (Table 4) and with a R2 between 0.75 and 0.8 for all the models.
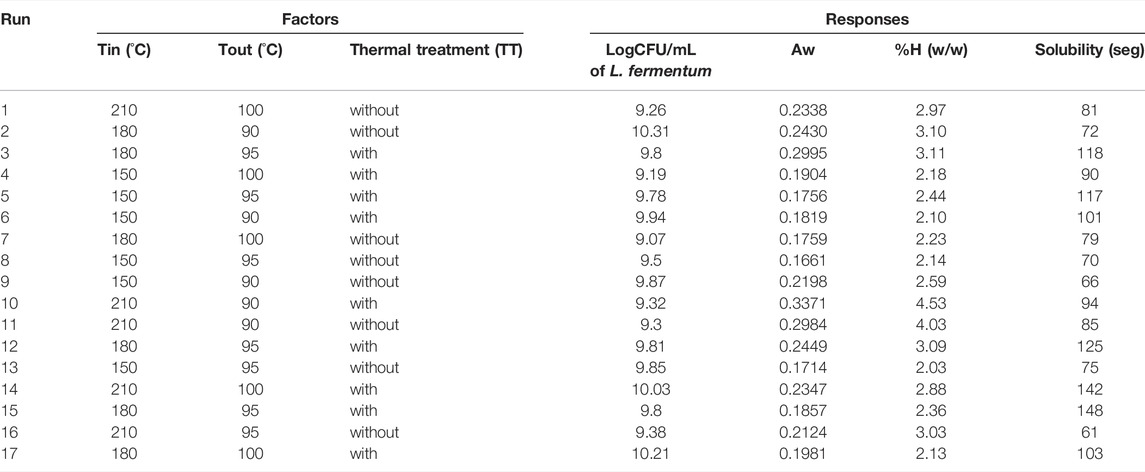
TABLE 3. Spray drying experimental design of the food-grade culture medium and its response variables.
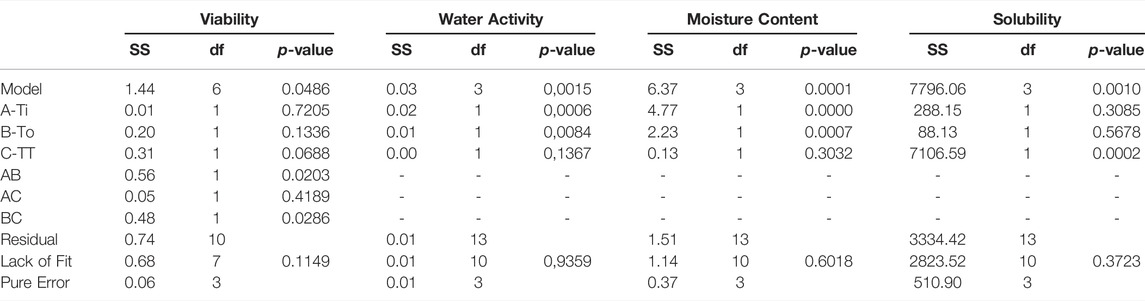
TABLE 4. ANOVA results for the adjusted variables to response optimization design: Viability (log UFC/ml), water activity, moisture content (%) and solubility (s).
The influence of the thermal treatment before the drying process in the production of the powdered medium was analyzed to observe if it influenced the response variables. The above is due to the high cost of this thermal process and the inconvenience of carrying out for industrial quantities. In this case, performing it before the drying process was only significant (p < 0.05) in for the growth of L. fermentum and the solubility of the medium after dried. Inlet and outlet temperature were key factors on the physical properties of the powder, having a significative (p < 0.05) effect on the moisture content, solubility, and water activities.
The optimization process yielded 17 solutions with a desirability between 0.726 and 0.873. The solution chosen for this design was the one with the highest desirability which was without the use of the thermal treatment over the liquid medium before the drying process, an inlet temperature of 150°C and an outlet temperature of 90°C. In Table 5 are presented the results expected for the response variables expected if the optimal conditions above are used for the process.
The optimal conditions were used to scale up the drying process to an industrial spray drying process. Here, 20 L of liquid culture medium were dried with the optimal conditions found at pilot scale (Table 5) in a spray dryer with a drying chamber with a water evaporation capacity of 150 L/h. One factor ANOVA was carried out to establish the differences between the industrial and pilot process. It could be observed that there was not a significant difference between the values of the physical properties water activity and solubility obtained for the culture medium processed at pilot and industrial scale. However, for moisture content there is a significant difference between the powder production at pilot and industrial scales. The above could be caused by the atomization type of the industrial process, that through the disc, the drop size reach measures near 20–30 μm, which increase the removal of the water in a 50% approx. (Assadpour and Jafari, 2019). Although the difference in the moisture content, both products meet the requirements established on the International Standard Organization, which indicates that the maximum moisture content permitted on a dried milk product is 4.5% (WHEY PROCESSING, 2022).
Validation of the Dried Cultured Medium With Probiotic Strains
The selection of the growth medium for producing commercial amounts of probiotics depends on costs, the efficiency of cell production, and ease of harvest. For microorganism’s production on an industrial scale, the cell concentration and time are taken to reach that cell amount has a significant impact on the cost. MRS broth and RCM are the conventional medium for probiotic production, but its high cost make them unrentable for the industry. For this reason, lactic growth medium is used for its variety of presentations and its physicochemical compounds.
Therefore, to validate that de powdered culture medium is functional for the growth of probiotics, the ATCC strains Enterococcus faecium, Lactobacillus delbrukii, Limosilactobacillus fermentum and Lacticaseibacillus rhamnosus were grown on the reconstituted culture medium. After 10 h of fermentation the viability count was 9.6, 10.2, 10.6 and 9.8 logCFU/mL, respectively. This demonstrated the usefulness of the functionality of the food-grade culture medium in powder. This is in accordance with the results found by Drgalić et al. (2005) where whey was used as the only compound of the medium and viability of commercial probiotics was higher than 7 log CFU/ml. Also, (Jantzen et al. (2013) used whey supplemented with yeast extract to grow L. reuteri with a final slurry concentration of 9 log CFU/g after 24 h. As found by Sharma et al., whey supplemented with oat allowed probiotic L. plantarum to grow until reach 10.9 log CFU/ml in 10 h, proving the efficacy of growing probiotics on whey supplemented with yeast extract. In recent studies, a solution of 30% of sweet whey (%p/p) was used to grow L. casei and P. feudenreichii, in this case the viability of the probiotics was of 9.2 and 9.6 log CFU/ml, respectively (Huang et al., 2017), supporting the results obtained for the food-grade culture medium proposed in this study.
Each one of the strains evaluated presents an affinity for one or more compounds that increases the viability of the growth of the bacteria. For the Lactobacillus delbrueckii, glucose and amino acids can improve its growth (Li et al., 2016). On the other hand, Lacticaseibacillus rhamnosus is affected significantly with the presence of carbohydrates as lactulose, galactooligosaccharide, and fructooligosaccharide (Kneifel et al., 2000). For Lactobacillus Fermentum, the presence of lactose improves the production of the microorganism, as the use of milk-based products as host has a positive impact on its growth (Riaz et al., 2010; Rezvani et al., 2017). Enterococcus Faecium presents an increase in its production if there is a source of nitrogen present on the medium. Yang et al. (2018) studied the effect of the medium, pH, and temperature of growth for different probiotic strains, including E. Faecium, where it was concluded that the growth of the strain increases when is inoculated on a medium with high concentrations of yeast extract or peptone, both nitrogen promotors. For these reasons it can be said that the efficacy of the medium K73 is due to its specific chemical composition, that provides more nutrients and an optimal environment for the strains to grow.
In Vitro Digestion of Probiotic Strains
It is well known that to be considered as a probiotic, microorganism must comply with five basic requirements, as is shown on Lin et al. (2007). LABs are considered as common microorganisms that have beneficial effects on human health and, therefore, the general candidates to be probiotics. One of the requirements to carry the name of probiotics is that microorganisms arrive in the intestine at least in a concentration of 6 log CFU/g. For this reason, an in vitro digestion to compare the protective effect of the food-grade culture medium was carried out. In this assay, 1 ml aliquot of each gastrointestinal phase was used to measure viability by count plate in agar MRS. As shown in Figure 1 (solid bars), the reduction on probiotics viability after digestion was only between 2 and 3 cycles, allowing to arrive in the intestine at 7.3, 6.8, 7.5 and 7 log CFU/mL, for L. fermentum, L. delbruekii, L. rhamnosus and E. faecium, respectively. On the contrary, probiotics growth in MRS had a reduction of 3–4 cycles on viability. Although MRS is a medium selective for the growth of Lactobacillii, it does not have in its composition structures that enhance the protection of the microorganism against the GI conditions (Figure 1, stripe bars). However, the proposed food-grade medium incorporates whey concentrate, which is composed by lactose and globular proteins as β-lactoglobulin, α-lactalbumin, bovine serum albumin, and immunoglobulins. These molecules not only serve as food to microorganisms but also enhance the protection against the interaction of the gastrointestinal enzymes, salts, and changing pH.
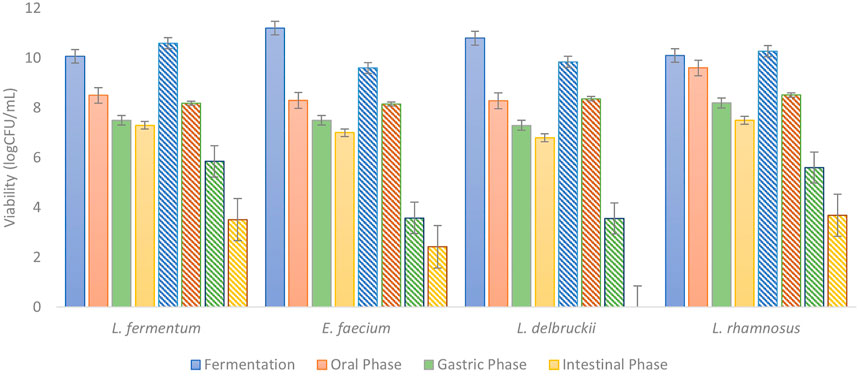
FIGURE 1. Viability of probiotics during in vitro digestion. Solid bars represent the viability after each digestion phase of probiotics cultured in the food-grade medium. Stripe bars represent the viability after each digestion phase of probiotics cultured in MRS.
Similar results were obtained by Jantzen et al. (2013) were microorganism L. reuteri where growth in MRS and then in vitro digested. In this case a reduction of 88% in probiotic viability was observed after 3 h of intestinal phase, like the 72–100% of viability reduction found in this study after digestion.Madureira et al. (2011) compared a fermentation in a cheese whey matrix and MRS, and their potential as protective matrices through the gastrointestinal tract, this time a reduction of 6 log cycles was found for probiotic strains in MRS at the end of intestinal phase, while probiotic in whey cheese matrix just had a reduction of 2 log cycles in the simulation of their pass through the gastrointestinal tract. The above is in accordance with the results obtained in this study, making whey a suitable matrix to not only fabricate food-grade culture media to obtain high concentrations of probiotics but also that protect them of the conditions in the gastrointestinal tract, allowing them to reach the intestine, which is their active site, in adequate concentrations.
The above was in accordance with the results obtained Saito et al. (2014), presented a viability study for two strains of Lactobacillus, where was suspended on a soy yogurt and summited an in vitro digestion test. It concluded that the high survival of the strain on the gastric-intestinal phases was the high number of polysaccharides and prebiotic compounds.Senaka Ranadheera et al. (2013) presents those dairy foods rich in milk fat, such as ice cream, were found to be more effective in enhancing the survivability and bile acid tolerance of probiotics, due to the presence of polysaccharides and fermentable sugars, such as glucose and fructose.Corcoran et al. (2005) have also studied the effect of sugars such as glucose and fructose on the survival of lactobacilli in acidic environments. These authors found that the survival of L. rhamnosus GG exposed to simulated gastric phase was significantly high in the presence of glucose and fructose, and that viability varied among species. The investigation associated this result with the chemical composition of cereals with the presence of soluble sugars, and to different polysaccharides that act as a prebiotic, such as inulin. Therefore, the medium K73, with its high presence of fermentable sugar (lactose in 51.64%), and with the addition of nitrogen, lipids, and prebiotic sources, making it a highly recommended growth medium for lactobacilli strains compared to the MRS.
Industrial Production of Encapsulated Probiotics
The production of probiotics in powder for different food applications involves different processes such as centrifugation to concentrate cells and encapsulation which allow the microorganisms to arrive in adequate concentration at the intestine and be called probiotics. In the concentration step the main processes are centrifugation and wash of the medium on which probiotics were grown, the last due to the toxicity of the medium for human ingestion. These are high-cost processes that could be eliminated when using a food-grade medium as the proposed one in this study.
The above was proved by the industrial production of encapsulated L. fermentum in powder. For this 80 L of probiotic on food-grade medium was obtained after 10 h of fermentation. Later, it was mixed with wall material composed of whey and maltodextrin to obtain a solution of 40% of solids (p/v). This solution was fed to the industrial spray dryer at 15 Hz with an inlet temperature of 180°C and an outlet temperature of 80°C. After the probiotic in powder was obtained a release was carried out to verify the concentration of the probiotic encapsulated, which was of 6.8 log CFU/ml. To prove the efficacy of encapsulation enhancing the protection against the GIT the probiotic in powder was in vitro digested, these results showed how wall material and culture medium protect the probiotic against the gastrointestinal conditions allowing the probiotic to arrive in the intestine with just 1 cycle reduction in viability.
The encapsulation of probiotics in powder by spray drying is an effective strategy to enhance the survival of probiotics through their pass in the gastrointestinal tract. On the other hand, wall material is an important factor in the delivery of probiotics in the intestine, among them whey powders and polysaccharides have proven to have a protective effect for probiotics allowing them to reach the intestine in concentration greater than 6 log CFU/g. This has been demonstrated by Moayyedi and collaborators (Moayyedi et al., 2018), in their study probiotic L. rhamnosus was encapsulated by spray drying using when, inulin and Persian gum as wall material, in this case this allowed to survive the microorganism with a reduction of only 2 cycles in its viability, having a final concentration after intestinal phase of 8.88 logCFU/ml. Similar results were obtained in a synbiotic preparation of L. casei and tuna oil (Eratte et al., 2017), probiotic reach the intestine at 6.5 logCFU/ml at the end of the in vitro digestion, being in this case whey protein and gum Arabic the wall material. Also, whey as wall material is not only used as encapsulating agent with spray drying technique but also with ionotropic extrusion and layer by layer encapsulation as used by de Araújo Etchepare et al. (2020) where ionic extrusion was used to prepare the initial beads containing probiotics and then they were coated with a layer of WPC, which protected the microorganisms L. acidophilus and allowed to reach the intestine in a concentration of 9.19 logCFU/g.
Conclusion
Whey concentrate supplemented with yeast extract is a suitable matrix to grow probiotics as a food-grade medium which do not need to be washed to be used in the food industry. Also, the food-grade medium in powder allows to growth a variety of different probiotics, and its powder state and its physical properties as high rate of solubility, moisture content and water activity, allows it to be prepared and preserved for long periods of time. It is important to highlight the ability of this medium to protect microorganism from the gastrointestinal conditions, and its versatility to be part of the wall material of microcapsules of probiotics.
Data Availability Statement
The original contributions presented in the study are included in the article/Supplementary Material, further inquiries can be directed to the corresponding author.
Author Contributions
MXQ-C and KB contributed to conception and design of the study. KB, VB and MR performed the experimentation. KB and MC wrote the first draft of the manuscript. KB and MXQ-C edited the manuscript, performed the analysis and organized the database. All authors contributed to manuscript revision, read, and approved the submitted version.
Conflict of Interest
Author MR was employed by the company Drycol S.A.S.
The remaining authors declare that the research was conducted in the absence of any commercial or financial relationships that could be construed as a potential conflict of interest.
Publisher’s Note
All claims expressed in this article are solely those of the authors and do not necessarily represent those of their affiliated organizations, or those of the publisher, the editors and the reviewers. Any product that may be evaluated in this article, or claim that may be made by its manufacturer, is not guaranteed or endorsed by the publisher.
Acknowledgments
Researchers are grateful to Universidad de La Sabana and MinCiencias for the funding of the project ING 223-2019 in which this study was developed.
Abbreviations
Tin, inlet temperature; Tout, outlet temperature; Aw, water activity; SSF, simulated salivary fluid; SGF, simulated gastric fluid; SIF, simulated intestinal fluid.
References
Abd El-Salam, M. H., and El-Shibiny, S. (2015). Preparation and Properties of Milk Proteins-Based Encapsulated Probiotics: a Review. Dairy Sci. Technol. 95, 393–412. doi:10.1007/S13594-015-0223-8/TABLES/1
Aragón-Rojas, S., Hernández-Álvarez, A. J., Mainville, I., Arcand, Y., and Quintanilla-Carvajal, M. X. (2020). Effect of the Carrier Material, Drying Technology and Dissolution Media on the Viability of Lactobacillus Fermentum K73 during Simulated Gastrointestinal Transit. Food Funct. 11, 2339–2348. doi:10.1039/c9fo01091b
Aragón-Rojas, S., Ruiz-Pardo, R. Y., Hernández-Sánchez, H., and Quintanilla-Carvajal, M. X. (2018). Optimization of the Production and Stress Resistance of the probioticLactobacillus fermentumK73 in a Submerged Bioreactor Using a Whey-Based Culture Medium. CyTA - J. Food 16, 1064–1070. doi:10.1080/19476337.2018.1527785
Assadpour, E., and Jafari, S. M. (2019). Advances in Spray-Drying Encapsulation of Food Bioactive Ingredients: From Microcapsules to Nanocapsules. Annu. Rev. Food Sci. Technol. 10, 103–131. doi:10.1146/annurev-food-032818-121641
Bosnea, L. A., Moschakis, T., and Biliaderis, C. G. (2014). Complex Coacervation as a Novel Microencapsulation Technique to Improve Viability of Probiotics under Different Stresses. Food Bioprocess Technol. 7, 2767–2781. doi:10.1007/S11947-014-1317-7/FIGURES/10
Burgain, J., Gaiani, C., Cailliez-Grimal, C., Jeandel, C., and Scher, J. (2013). Encapsulation of Lactobacillus Rhamnosus GG in Microparticles: Influence of Casein to Whey Protein Ratio on Bacterial Survival during Digestion. Innovative Food Sci. Emerg. Technol. 19, 233–242. doi:10.1016/j.ifset.2013.04.012
Corcoran, B. M., Stanton, C., Fitzgerald, G. F., and Ross, R. P. (2005). Survival of Probiotic Lactobacilli in Acidic Environments Is Enhanced in the Presence of Metabolizable Sugars. Appl. Environ. Microbiol. 71, 3060–3067. doi:10.1128/AEM.71.6.3060-3067.2005
de Araújo Etchepare, M., Nunes, G. L., Nicoloso, B. R., Barin, J. S., Moraes Flores, E. M., de Oliveira Mello, R., et al. (2020). Improvement of the Viability of Encapsulated Probiotics Using Whey Proteins. LWT 117, 108601. doi:10.1016/J.LWT.2019.108601
Doherty, S. B., Auty, M. A., Stanton, C., Ross, R. P., Fitzgerald, G. F., and Brodkorb, A. (2012). Survival of Entrapped Lactobacillus Rhamnosus GG in Whey Protein Micro-beads during Simulated Ex Vivo Gastro-Intestinal Transit. Int. Dairy J. 22, 31–43. doi:10.1016/J.IDAIRYJ.2011.06.009
Drgalic, I., Tratnik, L., and Bozanic, R. (2005). Growth and Survival of Probiotic Bacteria in Reconstituted Whey. Lait 85, 171–179. doi:10.1051/LAIT:2005009
Eratte, D., Dowling, K., Barrow, C. J., and Adhikari, B. P. (2017). In-vitro Digestion of Probiotic Bacteria and Omega-3 Oil Co-microencapsulated in Whey Protein Isolate-Gum Arabic Complex Coacervates. Food Chem. 227, 129–136. doi:10.1016/j.foodchem.2017.01.080
Geranpour, M., Assadpour, E., and Jafari, S. M. (2020). Recent Advances in the Spray Drying Encapsulation of Essential Fatty Acids and Functional Oils. Trends Food Sci. Technol. 102, 71–90. doi:10.1016/j.tifs.2020.05.028
Goveas, L. C., and Sajankila, S. P. (2020). Effect of Yeast Extract Supplementation on Halotolerant Biosurfactant Production Kinetics Coupled with Degradation of Petroleum Crude Oil by Acinetobacter Baumannii OCB1 in Marine Environment. Bioresour. Technol. Rep. 11, 100447. doi:10.1016/J.BITEB.2020.100447
Huang, S., Cauty, C., Dolivet, A., le Loir, Y., Chen, X. D., Schuck, P., et al. (2016). Double Use of Highly Concentrated Sweet Whey to Improve the Biomass Production and Viability of Spray-Dried Probiotic Bacteria. J. Funct. Foods 23, 453–463. doi:10.1016/j.jff.2016.02.050
Huang, S., Méjean, S., Rabah, H., Dolivet, A., le Loir, Y., Chen, X. D., et al. (2017). Double Use of Concentrated Sweet Whey for Growth and Spray Drying of Probiotics: Towards Maximal Viability in Pilot Scale Spray Dryer. J. Food Eng. 196, 11–17. doi:10.1016/j.jfoodeng.2016.10.017
Huynh, D., Kaschabek, S. R., and Schlömann, M. (2020). Effect of Inoculum History, Growth Substrates and Yeast Extract Addition on Inhibition of Sulfobacillus Thermosulfidooxidans by NaCl. Res. Microbiol. 171, 252–259. doi:10.1016/J.RESMIC.2020.08.004
Jantzen, M., Göpel, A., and Beermann, C. (2013). Direct Spray Drying and Microencapsulation of probioticLactobacillus Reuterifrom Slurry Fermentation with Whey. J. Appl. Microbiol. 115, 1029–1036. doi:10.1111/jam.12293
Kneifel, W., Rajal, A., and Kulbe, K. D. (2000). In Vitrogrowth Behaviour of Probiotic Bacteria in Culture Media with Carbohydrates of Prebiotic Importance. Microb. Ecol. Health Dis. 12, 27–34. doi:10.1080/089106000435563
Koutinas, A. A., Papapostolou, H., Dimitrellou, D., Kopsahelis, N., Katechaki, E., Bekatorou, A., et al. (2009). Whey Valorisation: a Complete and Novel Technology Development for Dairy Industry Starter Culture Production. Bioresour. Technol. 100, 3734–3739. doi:10.1016/J.BIORTECH.2009.01.058
Krunić, T. Ž., Obradović, N. S., and Rakin, M. B. (2019). Application of Whey Protein and Whey Protein Hydrolysate as Protein Based Carrier for Probiotic Starter Culture. Food Chem. 293, 74–82. doi:10.1016/J.FOODCHEM.2019.04.062
Li, C., Zhang, G. F., Mao, X., Wang, J. Y., Duan, C. Y., Wang, Z. J., et al. (2016). Growth and Acid Production of Lactobacillus Delbrueckii Ssp. Bulgaricus ATCC 11842 in the Fermentation of Algal Carcass. J. Dairy Sci. 99, 4243–4250. doi:10.3168/JDS.2015-10700
Lin, W.-H., Yu, B., Jang, S.-H., and Tsen, H.-Y. (2007). Different Probiotic Properties for Lactobacillus Fermentum Strains Isolated from Swine and Poultry. Anaerobe 13, 107–113. doi:10.1016/j.anaerobe.2007.04.006
Madureira, A. R., Amorim, M., Gomes, A. M., Pintado, M. E., and Malcata, F. X. (2011). Protective Effect of Whey Cheese Matrix on Probiotic Strains Exposed to Simulated Gastrointestinal Conditions. Food Res. Int. 44, 465–470. doi:10.1016/J.FOODRES.2010.09.010
Mat, D. J. L., le Feunteun, S., Michon, C., and Souchon, I. (2016). In Vitro Digestion of Foods Using pH-Stat and the INFOGEST Protocol: Impact of Matrix Structure on Digestion Kinetics of Macronutrients, Proteins and Lipids. Int. Food Res. J. 88, 226–233. doi:10.1016/J.FOODRES.2015.12.002
Minekus, M., Alminger, M., Alvito, P., Ballance, S., Bohn, T., Bourlieu, C., et al. (2014). A Standardised Staticin Vitrodigestion Method Suitable for Food - an International Consensus. Food Funct. 5, 1113–1124. doi:10.1039/C3FO60702J
Moayyedi, M., Eskandari, M. H., Rad, A. H. E., Ziaee, E., Khodaparast, M. H. H., and Golmakani, M.-T. (2018). Effect of Drying Methods (Electrospraying, Freeze Drying and Spray Drying) on Survival and Viability of Microencapsulated Lactobacillus Rhamnosus ATCC 7469. J. Funct. Foods 40, 391–399. doi:10.1016/j.jff.2017.11.016
Pereira, R. N., Teixeira, J. A., Vicente, A. A., Cappato, L. P., da Silva Ferreira, M. V., da Silva Rocha, R., et al. (2018). Ohmic Heating for the Dairy Industry: a Potential Technology to Develop Probiotic Dairy Foods in Association with Modifications of Whey Protein Structure. Curr. Opin. Food Sci. 22, 95–101. doi:10.1016/J.COFS.2018.01.014
Rezvani, F., Ardestani, F., and Najafpour, G. (2017). Growth Kinetic Models of Five Species of Lactobacilli and Lactose Consumption in Batch Submerged Culture. Braz. J. Microbiol. 48, 251–258. doi:10.1016/j.bjm.2016.12.007
Riaz, S., Nawaz, S. K., and Hasnain, S. (2010). Bacteriocins Produced by L. Fermentum and L .Acidophilus Can Inhibit Cephalosporin Resistant E .Coli. Braz. J. Microbiol. 41, 643–648. doi:10.1590/S1517-83822010000300015
Ricaurte, L., Hernández-Carrión, M., Moyano-Molano, M., Clavijo-Romero, A., and Quintanilla-Carvajal, M. X. (2018). Physical, Thermal and Thermodynamical Study of High Oleic Palm Oil Nanoemulsions. Food Chem. 256, 62–70. doi:10.1016/j.foodchem.2018.02.102
Saito, V. S. T., dos Santos, T. F., Vinderola, C. G., Romano, C., Nicoli, J. R., Araújo, L. S., et al. (2014). Viability and Resistance of Lactobacilli Isolated from Cocoa Fermentation to Simulated Gastrointestinal Digestive Steps in Soy Yogurt. J. Food Sci. 79, M208–M213. doi:10.1111/1750-3841.12326
Senaka Ranadheera, C., Evans, C. A., Adams, M. C., and Baines, S. K. (2013). Production of Probiotic Ice Cream from Goat's Milk and Effect of Packaging Materials on Product Quality. Small Ruminant Res. 112, 174–180. doi:10.1016/j.smallrumres.2012.12.020
Sharma, P., Trivedi, N., and Gat, Y. (2017). Development of Functional Fermented Whey-Oat-Based Product Using Probiotic Bacteria. 3 Biotech. 7, 272. doi:10.1007/S13205-017-0906-3
Tsermoula, P., Khakimov, B., Nielsen, J. H., and Engelsen, S. B. (2021). WHEY - the Waste-Stream that Became More Valuable Than the Food Product. Trends Food Sci. Technol. 118, 230–241. doi:10.1016/J.TIFS.2021.08.025
WHEY PROCESSING (2022). Dairy Processing Handbook. AvaliableAt: https://dairyprocessinghandbook.tetrapak.com/chapter/whey-processing (accessed 224, 22).
Keywords: whey, food-grade medium, in vitro digestion, probiotics, scale-up and design, spray—drying
Citation: Bauer Estrada K, Caldas Abril M, Bonilla Bravo V, Ruiz M and Quintanilla-Carvajal MX (2022) Whey as Food-Grade Culture Medium on an Industrial Scale That Protects Probiotics During In Vitro Digestion. Front. Food. Sci. Technol. 2:894761. doi: 10.3389/frfst.2022.894761
Received: 12 March 2022; Accepted: 09 May 2022;
Published: 30 May 2022.
Edited by:
Isabel Hernando, Universitat Politècnica de València, SpainReviewed by:
Graciela Liliana Garrote, National University of La Plata, ArgentinaPanagiotis Kandylis, Aristotle University of Thessaloniki, Greece
Copyright © 2022 Bauer Estrada, Caldas Abril, Bonilla Bravo, Ruiz and Quintanilla-Carvajal. This is an open-access article distributed under the terms of the Creative Commons Attribution License (CC BY). The use, distribution or reproduction in other forums is permitted, provided the original author(s) and the copyright owner(s) are credited and that the original publication in this journal is cited, in accordance with accepted academic practice. No use, distribution or reproduction is permitted which does not comply with these terms.
*Correspondence: María Ximena Quintanilla-Carvajal, maria.quintanilla1@unisabana.edu.co