- 1Agriculture and Food, RISE Research Institutes of Sweden AB, Gothenburg, Sweden
- 2Division of Engineering Materials, Department of Industrial and Materials Science, Chalmers University of Technology, Gothenburg, Sweden
As life expectancy increases so do age related problems such as swallowing disorders, dysphagia, which affects 10%–30% of people over 65 years old. For dysphagia patients the texture and rheological properties of the food, and the bolus, is critical to avoid choking and pneumonia. Texture modified foods, timbals, are often served to these patients due to their ease of swallowing. The main concern with these foods is that they do not look visually alike the food they replace, which can decrease the patient’s appetite and lead to reduced food intake and frailty. This study aims to improve both the visual appearance of texturized food as well as the energy density and fiber content of the timbal formulation. 3D scanning and additive manufacturing (3D Printing) were used to produce meals more reminiscent of original food items, increasing their visual appeal. Rheology was used to ensure the original flow profile was maintained as the timbal was reformulated by reducing starch contents and partially replacing with dietary fibers. The amount of starch was reduced from 8.7 wt% in the original formulation to 3.5 wt% and partially replaced with 3 wt% citrus fiber, while maintaining properties suitable for both swallowing and 3D printing. The resulting formulation has improved nutritional properties, while remaining suitable for constructing visually appealing meals, as demonstrated by 3Dprinting a chicken drumstick from a model generated with 3D scanning.
Introduction
The number of people aged above 60 years old is predicted to double by 2050 and triple by 2100 whilst the population above 80 years of age is projected to triple by 2050 and increase more than sevenfold by 2100 (United Nations, 2015). To prevent age related problems such as frailty and achieve a good quality of life at high ages, preventing malnutrition is crucial. However swallowing disorders, known as dysphagia, is a common problem affecting 10%–30% of the population above 65 years old (Barczi et al., 2000). Dysphagia can also affect younger patients, often after intensive care treatments (Zuercher et al., 2019). There is no cure for dysphagia, but it is managed by modifying food texture to reduce the need of chewing, easy forming of a cohesive bolus and prevent it from entering the airways (Waqas et al., 2017; Qazi et al., 2019; Raheem et al., 2021; Stading, 2021). Depending on the severity of dysphagia different textures are needed (Wendin et al., 2010; Stading, 2021). The focus in this study was timbales which are moist, smooth, and short in texture, similar to an omelet. Timbals are commonly given to dysphagia patients when they first show signs of dysphagia, in care facilities as well as in home-care. They are also commercially available in frozen form and are prepared from pureed food reformulated with starch and egg. Even if there is an increased knowledge regarding dysphagia, and there are existing products on the market dysphagia is often associated with a decrease in appetite which results in risk of malnutrition and frailty (Raheem et al., 2021). The visual appeal, often associated with the recognition of traditional meals, as well as flavor of the texturized foods have been found to be important (Okkels et al., 2018). It is also hypothesized that texture-modified foods which resembles the original meals could encourage eating together and thereby breaking social isolation adding to an improved quality of life. To allow the patient to recognize what they are eating and thereby encourage food intake, shaping of the timbales to resemble the original food items has been suggested. Another aspect is to formulate the timbales to be as energy dense as possible due to the low amounts commonly eaten by the patients (Goldman et al., 2014). Many thickeners have been explored for texturized dysphagia foods such as microcrystalline cellulose and hydrocolloids (Raheem et al., 2021). The dietary fiber intake in the population above 50 years in the United States is below the recommended daily value, and an even lower intake has been found in adults above 60 years old with impaired cognitive functions (Prokopidis et al., 2022). Dietary fibers are associated with reduction in cardiovascular disease and an important constituent for a healthy diet to prevent constipation (Brownlee, 2011; Raheem et al., 2021; Prokopidis et al., 2022). A dietary fiber which has been increasingly used is micro fibrillated cellulose commercially available as e.g., citrus fibers, which is a side stream from the juice industry and consist of both insoluble and soluble fibers (Lundberg et al., 2014; Korus et al., 2020; Bruno et al., 2021; Huang et al., 2021). It forms a swollen network in water; however, the behavior depends on the fiber size and pectin content (Korus et al., 2020; Bruno et al., 2021; Huang et al., 2021; Qi et al., 2021; Putri et al., 2022).
Additive manufacturing, commonly known as 3D-printing offers a unique possibility to automate production of visually appealing and naturally looking texture-modified foods, as well as to personalize the food for an individual patient’s needs. It allows for quick variation in the presentation of the food design for increased palatability (Wegrzyn et al., 2012; Liu et al., 2017; Dick et al., 2021a; Dick et al., 2021b; Xing et al., 2022). 3D-printing is a prototyping technique which has become more mainstream within many sectors in recent years. The technological development has led to an increased interest also within the food sector to prepare specialty foods or make use of side streams (Holland et al., 2018; Dick et al., 2019; Derossi et al., 2020; García-Segovia et al., 2020; He et al., 2020; Chen et al., 2022b; Xing et al., 2022). The 3D printing technology is based on a bottom-up approach where a product is produced in a layer-by-layer fashion based on a computer aided design file (CAD) instead of using e.g., a mold. The description of the 3D shape which is 3D-printed is called a CAD file. It can be prepared in a range of ways, one way being simple shapes such as the cube or more refined pre-prepared shapes used herein in the internal software of the printer. Another method more commonly utilized within medicine, and now starting to appear within food 3D printing is 3D light scanning (Goyanes et al., 2016; Kim et al., 2017). 3D scanning allows for a digital rendition of an object; the resulting mesh can be used 3D printer for more natural looking products.
The most applied method is direct extrusion of a paste from a syringe onto a build plate (Sun et al., 2015). In direct extrusion-based 3D printing the formulation of the paste from a rheological perspective becomes of uttermost importance. The paste needs to be shear thinning to allow for flow through a narrow syringe with a high enough yield stress to allow for the printed structure to hold itself up on the build plate. On the same time, the paste needs to match previous formulations that have been found suitable for dysphagia patients when it comes to rheological properties as well as nutritional (Wegrzyn et al., 2012; Liu et al., 2017; García-Segovia et al., 2020; He et al., 2020; Dick et al., 2021b; In et al., 2021; Jeon et al., 2021; Chen et al., 2022a). Printability of different pastes, and what is commonly known as the printability window, is most often assessed through rheological characterization of the viscoelastic and flow behavior coupled with image analysis. To understand the effect of parameter changes onto a printed item a standard such as a cube is often used (Liu et al., 2019). Work has also been done to theoretically correlate parameters with the rheological behavior. However, it is a function of machine parameters such as the nozzle size and the exact formulation which needs to be considered for the “printability window” (Liu et al., 2019).
The aim of the present study was to adapt the timbal composition to reduce the amount of starch and partially replace it with citrus fiber to increase the fiber content. Rheological characterization has been utilized to match the flow properties with the original timbals and the printability of the different formulations has been assessed. The formulation which was found to match the rheological properties to that of the original timbal recipe was then printed into a shrimp to assess the printability. Herein 3D scanning has been done of food items, such as a chicken drumstick. As a proof of concept, the 3D scanned chicken drumstick has been printed with a commercial food 3D printer, Foodini.
Materials and methods
Timbal formulations were prepared based on a modified version of the original recipe “Grundrecept grönsakstimbal med omelett-och timbalbas” from the cookbook “Mätt rätt och slätt” published by Findus Sverige AB (Findus Sweden and Findus Special Foods, 1999). The formulations were modified, and the starch replaced with citrus fibers to a varying degree according to Table 1. The timbals were prepared by mixing powders of egg white, yolk and starch kindly provided by Findus Sverige AB (Malmö, Sweden) together with salt and/or citrus fiber. The citrus fiber, Herba Cusine MultiTextur (Herbafood Ingredients GmbH, Werder, Germany) containing 85% fiber and 15% pectin was purchased from Åsö AB (Åtvidaberg, Sweden). The dry mixture was whisked into tomato puree containing 93% water together with rape seed oil. The formulations were stirred until the mixture was completely smooth, thereafter stored at 4 °C overnight. The timbal formulations were filled in stainless steel capsules with 1.5 mm nozzles and printed at room temperature into a cube (40x40x40 mm) using a Foodini printer (Natural Machines, Barcelona, Spain). The printer settings were kept the same for all formulations, see Table 2. The printed timbal cubes and other 3D printed samples were baked in a commercial steam oven (Convotherm-Elektrogeräte GmbH, Eglfing, Germany) at 120°C and full steam for 15 min. The timbale cubes were photographed before and after baking using a Dino-light (Dino-light Europe, Almare, Netherlands), Table 3. Its internal software was used to measure the dimensions of the cube.

TABLE 3. Yield stress as measured by the oscillation stress (kPa) at cross-over point of storage and loss modulus in the amplitude sweep.
3D scanning was preformed using GOM Atos Triple Scan II (Zeiss, Germany) with camera pixels of 2 × 8,000,000 at the working distance of 830 mm. The measuring area was 320 × 240 × 240 mm with a point spacing of .104 mm. The GOM Inspect Pro 2021 (Zeiss, Germany) was used for the 3D scanning and initial mesh edits. An STL file was exported and further processed using Fusion 360 and Meshmixer (Autodesk, San Rafael, United States) before printing.
The rheological properties of the different formulations were characterized using a HR30 (TA Instruments, Newcastle, United States) equipped with parallel x-hatched plates 20 mm in diameter and a gap of 1 mm with a water-controlled Peltier plate. The tests were carried out at 20°C in triplicates for each measurement type and sample. Flow curves were obtained at shear rates of .1–100 s−1 with 5 points per decade using steady state sensing with a max equilibrium time of 10 s and sample period of 5 s. The viscoelastic properties were characterized using amplitude sweeps and mechanical spectra. The stress sweeps were performed at 1 Hz with an applied stress between 1 and 10,000 Pa, the yield stress was defined as the cross over modulus with respect to the applied oscillation stress. The mechanical spectra were carried out with an applied stress of 5 Pa (found to be within the linear viscoelastic limit for all samples) for frequencies between 0.1 and 30 Hz with 10 points per decade. To conduct parameter fitting of the flow properties TRIOS software version 5.4.0.300 was utilized and the Herschel-Bulkley model was fitted to the flow curve
where σ is the shear stress, γ ˙shear rate, K is a consistency index and n is a shear thinning index. Note that K and n are like the corresponding parameters in the Power Law model, but not exactly equal. Microstructure of the citrus fiber was determined for 10% solutions which were smeared onto a microscope slide with a cover slip placed on top. The samples were analyzed using a light microscope (LM), BX53F2 from Olympus (Tokyo, Japan) equipped with a CMOS camera, CAM-SC50 using a 4x, 10x, and 20x objective in brightfield and with crossed polarizers.
Results
To increase the fiber content but retain the energy density and rheological properties, starch in the original recipe was replaced in parts with citrus fibers. The rheological behavior and printability were examined with respect to included wt% of citrus fiber to the original formulation. First, the microstructure of the citrus fiber was characterized at 10 wt% using light microscopy (LM), see Figure 1. The bright field micrographs Figure 1(1a–c) shows that the citrus fibers are well dispersed in the water solution. They had swollen to form a network structure, but individual fibers were still visible. Figure 1(1a–c) show a varying fiber length from more than 200 µm down to below 10 µm. In Figure 1(2a-c) the crystallinity of the same sample visualized using cross-polarized light, and the citrus fibers are clearly mainly crystalline. In comparison between the brightfield and the polarized micrographs it is shown that the citrus fiber material also contain some amorphous segments and pectin. The citrus fibers were intended to increase the viscosity and yield stress of the formulation to replace the gelatinized starch, providing shape stability to the printed structures.
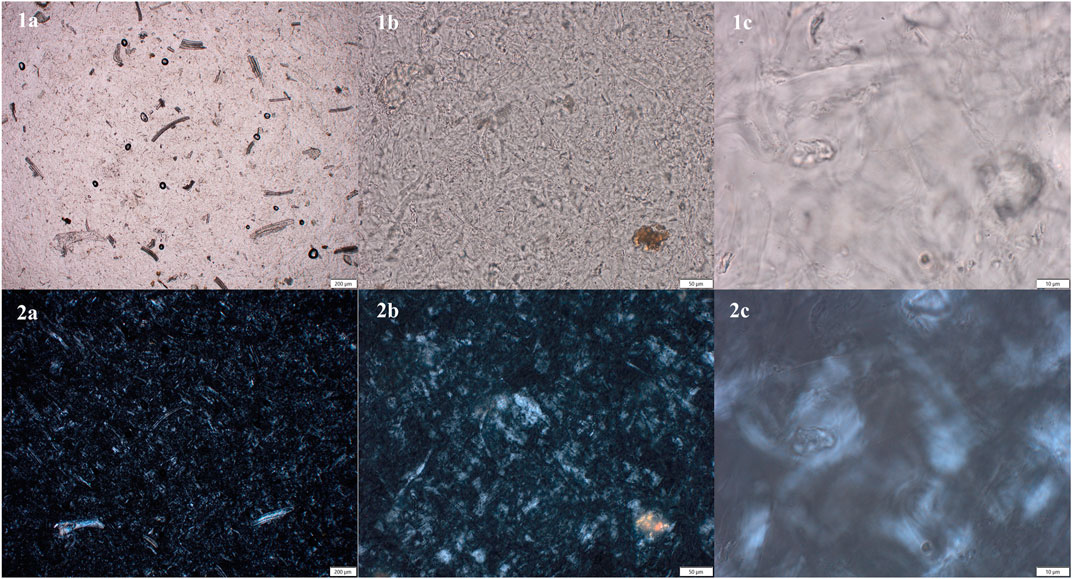
FIGURE 1. Light microscope micrographs of citrus fiber 10 wt% mixed with water using bright field, 1a–c, and polarized light 2a–c. The scale bars are for a 200 μm, b 50 µm c 10 µm.
Citrus fibers were added in increased concentration from 1.5–9 wt% as described in Table 1. The rheological properties of the different formulations were characterized with respect to their flow and viscoelastic properties, see Figures 2, 3. The substitution of starch with 1.5 wt% citrus fiber reduced viscosity and stress response. Increased amounts of citrus fibers increased the viscosity and flow curve further but did not greatly alter the shear thinning behavior, displayed non-significant difference in the shear thinning index (n), Table 2. The viscosity of the original formulation was found to lay somewhere between that of the modified formulations with 3–6 wt% added citrus fibers. A comparison of the yield stress, gained from a Herschel-Bulkley fit to the flow curve, showed that the yield stress of the original formulation was found to lay between that of the modified formulations with 3–6 wt% added citrus fibers. The flow curve at 9 wt% showed wall-slip and stable measurements were not feasible above 1 s−1, these results were removed from the graph and excluded from the analysis. Additional assessment of the viscoelastic properties of the formulations, Figures 3A–C with mechanical spectra and stress sweeps showed that the storage modulus and Tan δ of the original formulation was found to lay between that of 3–6 wt% added fiber. By assessing the yield stress from the stress sweep, as the crossover between the storage and loss moduli with respect to oscillation stress, Table 3, the value of the original formulation was closest to that of the 6 wt% citrus fiber. In the stress sweep, Figure 3C, the storage modulus within the linear viscoelastic region matched between the original formulation and 3 wt% of citrus fiber, but that the 3 wt% could not withstand the same deformation. The 6 wt% citrus fiber had similar yield stress, but the storage modulus and viscosity were higher.
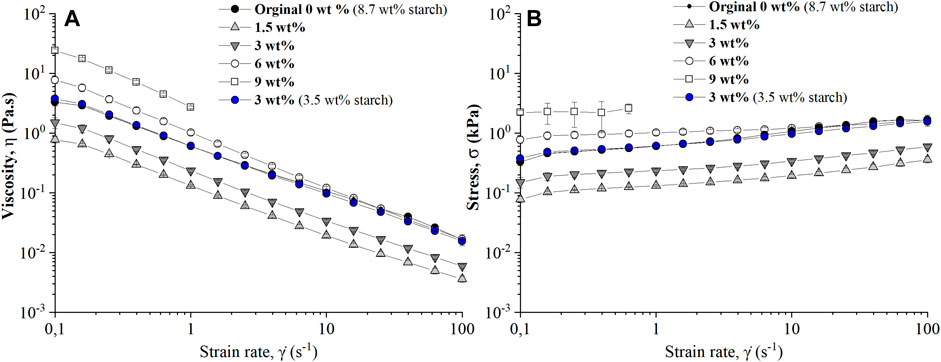
FIGURE 2. Flow curves of the different formulations (A) viscosity (Pa.s) vs. shear rate (1/s) and (B) Stress (kPa) vs. shear rate (1/s).
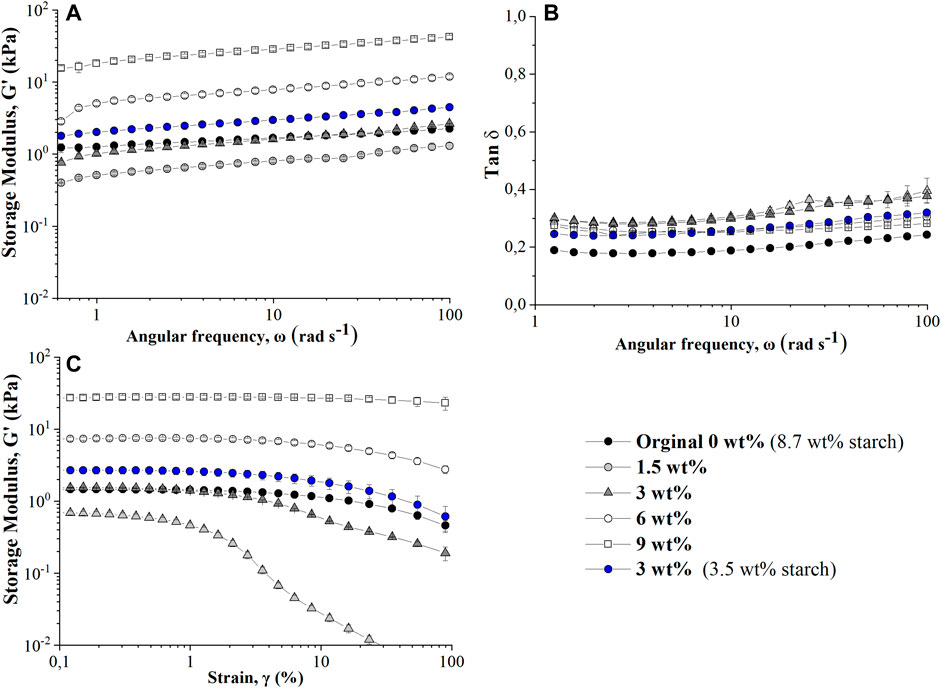
FIGURE 3. Characterization of the viscoelastic properties with regards to the different formulations (A) storage modulus (kPa) vs. angular frequency (B) tan δ vs. angular frequency (C) storage modulus (kPa) vs. strain (%).
The rheological assessment of both the flow and viscoelastic properties showed that it was not possible to entire mimic the behavior of the timbal original formulation only through the removal of starch and addition of citrus fiber. An additional formulation using 3 wt% citrus fiber and 3.5 wt% starch was therefore evaluated, Table 1. The rheological behavior was found to be more alike that of the original formulation, Figures 2, 3. Herschel-Bulkley fit showed close similarity of 3 wt% citrus fiber and 3.5 wt% starch to the original formulation both with a yield stress near 0.27 kPa, Table 2. The shear thinning index were near 0.4 indicating a shear thinning behavior of the pastes, did not significantly differ between the considered formulations, Table 2. The yield stress from the mechanical spectra, defined as the crossover modulus in the stress sweep showed a slightly higher value of the original formulation with 8.7 wt% starch than that of 3 wt% citrus fiber and 3.5 wt% starch, Table 3.
To assess the effect of the formulation onto the printing parameters, these were optimized to be suitable for the original formulation, Printing parameters were optimized for the original formulation, Supplementary Table S1, it was applied to cubes printed in 40 × 40 × 40xmm cube, Figure 4. The printing was used as a validation to assess if our rheological characterization could be used to predict printability. The cubes were photographed before and after printing and the visual representation of the effect of yield behavior is shown in Figure 4. The formulation with 3 wt% citrus fiber had a too low yield stress, spread out during baking whereas the formulation with higher yield stress such as the 6 wt% retained its shape during baking. Both the original formulation, 6 wt%, and 3 wt% with 3.5 wt% starch formulations were similar or have shrunk slightly after baking.
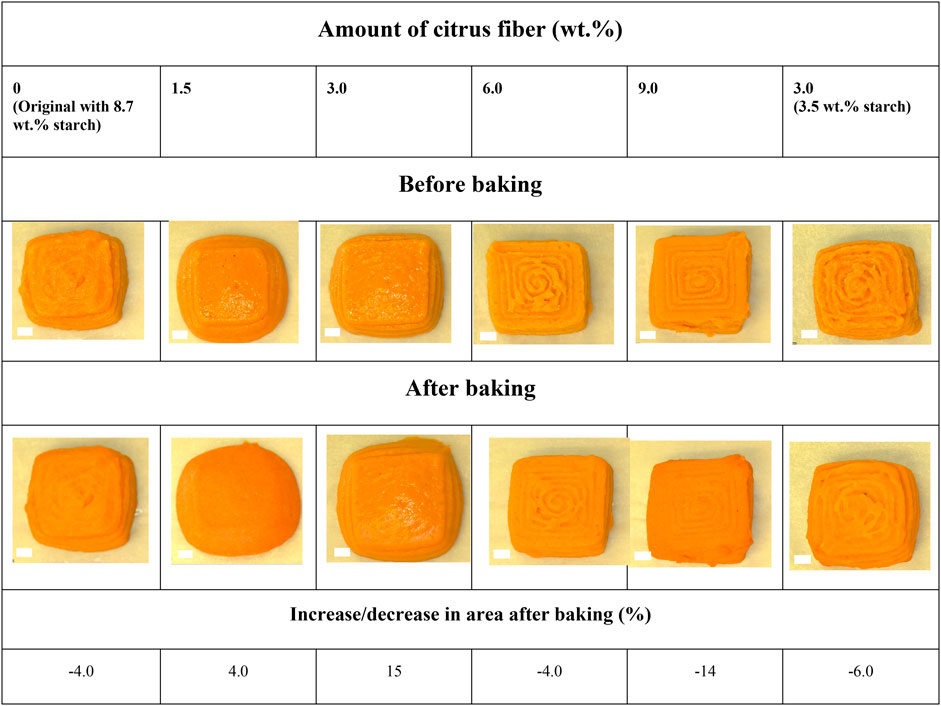
FIGURE 4. 3D-printed cubes using the original and five modified formulations before and after baking, the white scale bar to the left in each image correspond to 5 mm. A comparison of the area in respect to after baking was conducted and is shown in the bottom row.
To evaluate the printability in a more complex geometry, the formulation with 3 wt% citrus fiber and 3.5 wt% starch was printed in a shape of a shrimp, Figure 5. For the shrimp the parameters were adjusted to retain a higher resolution of the print with a decreased flow speed but increased printing speed, Supplementary Table S1. The geometry is clearly printed with high control of the deposition during printing of the antenna and shell, and it was found to be shape stable also after baking. Additionally, as a proof of concept, a simplified version of the 3D scanned chicken drumstick was printed, Figure 6, using the printing parameters of the standard cubes.
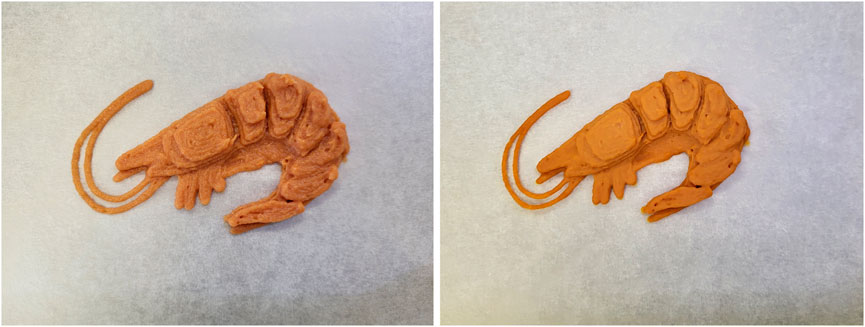
FIGURE 5. 3D printed Shrimp of the forumulation with 3 wt% citrus fiber and 3.5 wt% starch shown before (left) and after (right) baking. The shrimp design was selected from the internal libray of the Foodini and printed with the ingrient mousse-cream and is approximatly 5 cm in length.
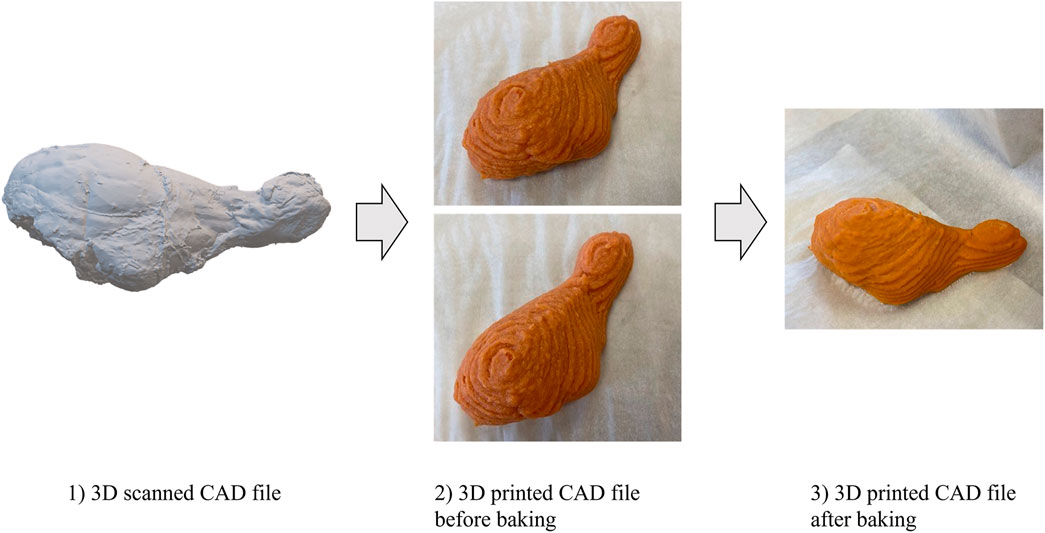
FIGURE 6. The CAD file of a 3D scanned chicken drumstick 1) before baking 2) and after baking 3). The dimension of the 3D printed drumstick was 4 cm × 10 cm.
Discussion
The rheological properties, Figures 2, 3, showed that citrus fibers cannot totally replace starch in a timbal formulation because of the high quantity needed to achieve the desired yield stress. However, starch content could be reduced from 8.7 wt% to 3.5 wt% through the inclusion of 3 wt% citrus fiber to give a similar rheological behavior to the original formulation. Fibers have well-known health benefits when incorporated in a varied diet (Korus et al., 2020; Raheem et al., 2021; Prokopidis et al., 2022). In the case where an ordinary diet is mainly replaced with modified-texture food it is an even more important consideration, as low intake of fiber and little physical activity are risk factors that can result in problems related to for example digestion (Yurtdaş et al., 2020). The addition of the fiber needs to be made with the rheological properties in mind for the ease of chew and swallow to not risk the patient’s health.
To increase the appetite of texture modified foods for dysphagia 3D printing has been suggested to increase attractiveness and produce more realistic looking foods by many authors (Dick et al., 2019; Dick et al., 2021a; Dick et al., 2021b; Theocharidou et al., 2022; Xing et al., 2022). The addition of fibers to food intended for dysphagia has not yet been conducted but is a nessary next step. Before patient trials are conducted, exhaustive testing is required to optimize the formulation. In 3D printing of foods through direct extrusion a directive value for the yield stress of .3 kPa has previously been found suitable to retain the geometry of the construct (Liu et al., 2017). The original formulation had a measured mean yield stress of 0.27 kPa, Table 2, thus near the suggested value for printability. The formulation with 3 wt% of citrus fiber and 3.5 wt% starch had a mean yield stress value of 0.25 kPa, Table 2, but was still able to retain its shape Figure 4. The viscosity and shear thinning index was also in a close range between the original formulation and that of the 3 wt% of citrus fiber with 3.5 wt% starch, Figure 2; Table 2. The inclusion of the citrus fiber however led to a slightly increased storage modulus in comparison to the original formulation, Figure 2. The formulations with 1.5 and 3 wt% citrus fiber added but without starch had mean yield stress values of .09 and .16 kPa, Table 2. From the visual analysis of the printed cubes, Figure 4, it was seen that the samples with 1.5 and 3 wt% citrus fiber could not hold its shape after printing and that a mean yield stress value of 0.16 kPa was below the limit to retain the shape at the build plate. The formulation with 6 wt% citrus fiber had a mean yield stress value of 0.83 kPa, Table 2, nearly three times the original formulation. From the printed cubes, Figure 4, it was seen that the formulation with 6 wt% resulted in more details and a neatly printed cube. Further tests are needed of the mechanical properties of the baked timbals to see if there is a reinforcing effect of the citrus fiber. In addition, sensory tests are needed to evaluate how the increased elasticity of the formulation affects the perception of the timbals.
3D scanning and 3D printing were used to show a future view of how dysphagia food can be prepared allowing for personalization, Figure 6. 3D printing and 3D scanning have during a long time been of high interest within the medical industry, both for drug formulation and tissue engineering (Melchels et al., 2011; Goyanes et al., 2016). As the technology develops and the personalization of food comes closer to that of medicine it is natural that 3D printing of food becomes incorporated to some extent within the specialty food sector, which could be both regarding shape, texture, or nutritional content (Severini and Derossi, 2016; Liu et al., 2017; Ueland et al., 2020). Herein as a proof of concept a 3D scanned chicken drumstick and the resulting CAD file is shown together with the 3D printed result, Figure 6. It can be seen in Figure 6 that the 3D printed timbal version was highly simplified, this was partially due to the rheological behavior of the formulation so that all details could not be fully retained but mainly to reduce printing time. The printing time was limited to 15 min. The present results highlight the importance of rheological measurements of both flow properties and viscoelasticity for the formulation for 3D-printed foods. The importance of rheological assessment of the texture-modified food has previously been showed by others (Wendin et al., 2010; Waqas et al., 2017; Raheem et al., 2021; Stading, 2021; Theocharidou et al., 2022; Xing et al., 2022), but not for 3D printed timbales.
Conclusion
The study shows proof of concept of how to use 3D printing to create a visually attractive, yet texture-modified meal based on reconstituted pureed foods, called timbales. 3D scanning was used to create a proof-of-concept chicken drumstick from a timbal formula with increased fiber content. The original timbale formulation was altered through the reduction of starch from 8.7 wt% to 3.5 wt% by the addition of 3 wt% citrus fiber. The rheological profile was retained by balancing the reduction of starch with citrus fiber. The rheological profile was assessed both through oscillatory measurements and flow sweeps. In the flow sweeps the stress response was fitted with the Herschel- Bulkley model. The shear thinning index and yield stress were the two values compared between the original and altered formulations. The values for yield stress were of the original and altered timbale formulation were found to be slightly below the value found in literature, 0.3 kPa, desired for food printing. The formulation with 3.5 wt% starch and 3 wt% citrus fiber had a mean yield stress of 0.25 kPa but was still able to retain the printed test cube, shrimp and chicken drumstick after printed. The timbal formulation with only added 1.5 wt% or 3 wt% citrus fiber and no starch were not able to hold their shape after cubes had been printed. The starch could not be entirely removed but the reduction in wt% of starch allowed for a more energy dense formulation to be made.
Data availability statement
The original contributions presented in the study are included in the article/Supplementary Material, further inquiries can be directed to the corresponding author.
Author contributions
AA wrote the main part of the manuscript, MS and EH aided with revision and draft. The conceptualization of the article was conducted by EH, MS, and AA, as well as discussion regarding the experimental results. MS contributed with funding. The main part of the experimental work was conducted by EH and AA. CÖ conducted the experimental work with regards to microscopy and AM conducted parts of the experimental work within rheology and 3D printing.
Funding
This study was supported by the Sweden’s Innovation Agency Vinnova.
Acknowledgments
MS and AA acknowledge support from the KP Nanocellulose platform from RISE Research institute of Sweden AB. Lars- Olof Ingmarsson at RISE AB is acknowledged for the help with 3D scanning.
Conflict of interest
The authors declare that the research was conducted in the absence of any commercial or financial relationships that could be construed as a potential conflict of interest.
Publisher’s note
All claims expressed in this article are solely those of the authors and do not necessarily represent those of their affiliated organizations, or those of the publisher, the editors and the reviewers. Any product that may be evaluated in this article, or claim that may be made by its manufacturer, is not guaranteed or endorsed by the publisher.
Supplementary Material
The Supplementary Material for this article can be found online at: https://www.frontiersin.org/articles/10.3389/frfst.2022.1058641/full#supplementary-material
References
Barczi, S. R., Sullivian, P. A., and Robbins, J. A. (2000). How should dysphagia care of older adults differ? Establishing optimal practice patterns. Semin. Speech Lang. 21, 347–361. doi:10.1055/s-2000-8387
Brownlee, I. A. (2011). The physiological roles of dietary fibre. Food Hydrocoll. 25, 238–250. doi:10.1016/j.foodhyd.2009.11.013
Bruno, E., Lupi, F. R., Martin-Piñero, M. J., Girimonte, R., Baldino, N., Muñoz, J., et al. (2021). Influence of different dispersing systems on rheological and microstructural properties of citrus fiber suspensions. LWT--Food Sci. Technol. 152, 112270. doi:10.1016/j.lwt.2021.112270
Chen, J., Sun, H., Mu, T., Blecker, C., Richel, A., Richard, G., et al. (2022a). Effect of temperature on rheological, structural, and textural properties of soy protein isolate pastes for 3D food printing. J. Food Eng. 323, 110917. doi:10.1016/j.jfoodeng.2021.110917
Chen, Y., Zhang, M., Sun, Y., and Phuhongsung, P. (2022b). Improving 3D/4D printing characteristics of natural food gels by novel additives: A review. Food Hydrocoll. 123, 107160. doi:10.1016/j.foodhyd.2021.107160
Derossi, A., Paolillo, M., Caporizzi, R., and Severini, C. (2020). Extending the 3D food printing tests at high speed Material deposition and effect of non-printing movements on the final quality of printed structures. J. Food Eng. 275, 109865. doi:10.1016/j.jfoodeng.2019.109865
Dick, A., Bhandari, B., and Prakash, S. (2019). 3D printing of meat. Meat Sci. 153, 35–44. doi:10.1016/j.meatsci.2019.03.005
Dick, A., Bhandari, B., and Prakash, S. (2021a). Effect of reheating method on the post-processing characterisation of 3D printed meat products for dysphagia patients. LWT—Food Sci. Technol. 150, 111915. doi:10.1016/j.lwt.2021.111915
Dick, A., Dong, X., Bhandari, B., and Prakash, S. (2021b). The role of hydrocolloids on the 3D printability of meat products. Food Hydrocoll. 119, 106879. doi:10.1016/j.foodhyd.2021.106879
Findus Sweden ABFindus Special Foods (1999). Grundrecept grönsakstimbal med omelett-och timbalbas, Special Foods Kokbok recept Mätt rätt och slätt. Available at: https://cdn.nomadfoodscdn.com/-/media/project/foodservices/sweden/special-foods-se/bestall-material/special-foods-kokbok-recept-matt-ratt-slatt.pdf (Acessed November 15, 2022).
García-Segovia, P., García-Alcaraz, V., Balasch-Parisi, S., and Martínez-Monzó, J. (2020). 3D printing of gels based on xanthan/konjac gums. Innov. Food Sci. Emerg. Technol. 64, 102343. doi:10.1016/j.ifset.2020.102343
Goldman, A. M., BradMojet, J., and Kremer, S. (2014). Meeting the food needs of the aging population – implications for food science and technology. The International Union of Food Science and Technology (IUFoST). Available at: https://iufost.org/sites/default/files/documents/sibs/IUF.SIB.Meeting%20the%20Food%20Needs%20of%20the%20Ageing%20Population.pdf (Accessed September 24, 2022).
Goyanes, A., Det-Amornrat, U., Wang, J., Basit, A. W., and Gaisford, S. (2016). 3D scanning and 3D printing as innovative technologies for fabricating personalized topical drug delivery systems. J. Control. Release. 234, 41–48. doi:10.1016/j.jconrel.2016.05.034
He, C., Zhang, M., and Fang, Z. (2020). 3D printing of food: Pretreatment and post-treatment of materials. Crit. Rev. Food Sci. Nutr. 60, 2379–2392. doi:10.1080/10408398.2019.1641065
Holland, S., Foster, T., MacNaughtan, W., and Tuck, C. (2018). Design and characterisation of food grade powders and inks for microstructure control using 3D printing. J. Food Eng. 220, 12–19. doi:10.1016/j.jfoodeng.2017.06.008
Huang, J., Liao, J., Qi, J., Jiang, W., and Yang, X. (2021). Structural and physicochemical properties of pectin-rich dietary fiber prepared from citrus peel. Food Hydrocoll. 110, 106140. doi:10.1016/j.foodhyd.2020.106140
In, J., Jeong, H., Song, S., and Min, S. C. (2021). Determination of material requirements for 3D gel food printing using a fused deposition modeling 3D printer. Foods 10, 2272. doi:10.3390/foods10102272
Jeon, W. Y., Yu, J. Y., Kim, H. W., and Park, H. J. (2021). Production of customized food through the insertion of a formulated nanoemulsion using coaxial 3D food printing. J. Food Eng. 311, 110689. doi:10.1016/j.jfoodeng.2021.110689
Kim, H. W., Bae, H., and Park, H. J. (2017). Classification of the printability of selected food for 3D printing: Development of an assessment method using hydrocolloids as reference material. J. Food Eng. 215, 23–32. doi:10.1016/j.jfoodeng.2017.07.017
Korus, J., Juszczak, L., Witczak, M., and Ziobro, R. (2020). Effect of citrus fiber on the rheological properties of dough and quality of the gluten-free bread. Appl. Sci. 10, 6633. doi:10.3390/app10196633
Liu, Z., Bhamdari, B., Prakash, S., Manthial, S., and Zhang, M. (2019). Linking rheology and printability of a multicomponent gel system of carrageenan-xanthan-starch in extrusion based additive manufacturing. Food Hydrocoll. 87, 413–424. doi:10.1016/j.foodhyd.2018.08.026
Liu, Z. B., Zhang, M., Bhandari, B., and Wang, Y. C. (2017). 3D printing: Printing precision and application in food sector. Trends Food Sci. Technol. 69, 83–94. doi:10.1016/j.tifs.2017.08.018
Lundberg, B., Pan, X., White, A., Chau, H., and Hotchkiss, A. (2014). Rheology and composition of citrus fiber. J. Food Eng. 125, 97–104. doi:10.1016/j.jfoodeng.2013.10.021
Melchels, F., Wiggenhauser, P. S., Warne, D., Barry, M., Ong, F. R., Chong, W. S., et al. (2011). CAD/CAM-assisted breast reconstruction. Biofabrication 3, 034114. doi:10.1088/1758-5082/3/3/034114
Okkels, S. S. L., Saxosen, M., Bügel, S., Olsen, A., Klausen, T. W., and Beck, A. M. (2018). Acceptance of texture-modified in-between-meals among old adults with dysphagia. Clin. Nutr. ESPEN 25, 126126–132132. doi:10.1016/j.clnesp.2018.03.119
Prokopidis, K., Giannos, P., Ispoglou, T., Witard, O. C., and Isanejad, M. (2022). Dietary fiber intake is associated with cognitive function in older adults: Data from the national health and nutrition examination survey. Am. J. Med. 135, 257–262. doi:10.1016/j.amjmed.2022.03.022
Putri, N. I., Celus, M., Audenhove, V. J., Nanseera, R. P., Loey, V. A., and Hendrickx, M. (2022). Functionalization of pectin-depleted residue from different citrus by-products by high pressure homogenization. Food Hydrocoll. 129, 107638. doi:10.1016/j.foodhyd.2022.107638
Qazi, W. M., Ekberg, O., Wiklund, J., Kotze, R., and Stading, M. (2019). Assessment of the food swallowing process using bolus visualisation and manometry simultaneously in a device that models human swallowing. Dysphagia 34, 821–833. doi:10.1007/s00455-019-09995-8
Qi, J., Song, L., Zeng, W., and Liao, J. (2021). Citrus fiber for the stabilization of O/W emulsion through combination of Pickering effect and fiber-based network. J. Food Chem. 343, 128523. doi:10.1016/j.foodchem.2020.128523
Raheem, D., Carrascosa, C., Ramos, F., Saraiva, A., and Raposo, A. (2021). Maltitol: Analytical determination methods, applications in the food industry, metabolism and health impacts. Int. J. Environ. Res. Public Health 18, 5227. doi:10.3390/ijerph17145227
Severini, C., and Derossi, A. (2016). Could the 3D printing technology be a useful strategy to obtain customized nutrition? J. Clin. Gastroenterol. 50, S175–S178. doi:10.1097/MCG.0000000000000705
Stading, M. (2021). Bolus rheology of texture-modified food: Effect of degree of modification. J. Texture Stud. 52, 540–551. doi:10.1111/jtxs.12598
Sun, J., Peng, Z., Zhou, W., Fuh, J. Y. H., Hong, G. S., and Chiu, A. (2015). A review on 3D printing for customized food fabrication. Procedia Manuf. 1, 308–319. doi:10.1016/j.promfg.2015.09.057
Theocharidou, A., Mourtzinos, I., and Ritzoulis, C. (2022). The role of guar gum on sensory perception, on food function, and on the development of dysphagia supplements – a review. Food Hydrocoll. Health 2, 100053. doi:10.1016/j.fhfh.2022.100053
Ueland, Ø., Altintzoglou, T., Kirkhus, B., Lindberg, D., Rognså, G. H., Rosnes, J. T., et al. (2020). Perspectives on personalised food. Trends Food Sci. Technol. 102, 169–177. doi:10.1016/j.tifs.2020.05.021
United Nations (2015). World population prospects: The 2015 revision, key findings and advance table. Available at: https://population.un.org/wpp/publications/files/key_findings_wpp_2015.pdf (Acessed November 27, 2022).
Waqas, M. Q., Wiklund, J., Altskär, A., Ekberg, O., and Stading, M. (2017). Shear and extensional rheology of commercial thickeners used for dysphagia management. J. Texture Stud. 48, 507–517. doi:10.1111/jtxs.12264
Wegrzyn, T. F., Golding, M., and Archer, R. H. (2012). Food layered manufacture: A new process for constructing solid foods. Trends Food Sci. Technol. 27, 66–72. doi:10.1016/j.tifs.2012.04.006
Wendin, K., Ekman, S., Bülow, M., Ekberg, O., Johansson, D., Rothenberg, E., et al. (2010). Objective and quantitative definitions of modified food textures based on sensory and rheological methodology. Food Nutr. Res. 54, 5134. doi:10.3402/fnr.v54i0.5134
Xing, X., Chitrakar, B., Hati, S., Xie, S., Li, H., Li, C., et al. (2022). Development of black fungus-based 3D printed foods as dysphagia diet: Effect of gums incorporation. Food Hydrocoll. 123, 107173. doi:10.1016/j.foodhyd.2021.107173
Yurtdaş, G., Acar-Tek, N., Akbulut, G., Cemali, Ö., Arslan, N., Beyaz Coşkun, A., et al. (2020). Risk factors for constipation in adults: A cross-sectional study. J. Am. Coll. Nutr. 39, 713–719. doi:10.1080/07315724.2020.1727380
Keywords: rheology, 3D printing, additive manufacturing, dysphagia, citrus fiber, 3D-scanning
Citation: Ahlinder A, Höglund E, Öhgren C, Miljkovic A and Stading M (2023) Towards attractive texture modified foods with increased fiber content for dysphagia via 3D printing and 3D scanning. Front. Food. Sci. Technol. 2:1058641. doi: 10.3389/frfst.2022.1058641
Received: 30 September 2022; Accepted: 30 December 2022;
Published: 18 January 2023.
Edited by:
Suzana Caetano Da Silva Lannes, University of São Paulo, BrazilReviewed by:
Amit Baran Das, Tezpur University, IndiaFabio Licciardello, University of Modena and Reggio Emilia, Italy
Copyright © 2023 Ahlinder, Höglund, Öhgren, Miljkovic and Stading. This is an open-access article distributed under the terms of the Creative Commons Attribution License (CC BY). The use, distribution or reproduction in other forums is permitted, provided the original author(s) and the copyright owner(s) are credited and that the original publication in this journal is cited, in accordance with accepted academic practice. No use, distribution or reproduction is permitted which does not comply with these terms.
*Correspondence: Astrid Ahlinder, YXN0cmlkLmFobGluZGVyQHJpLnNl