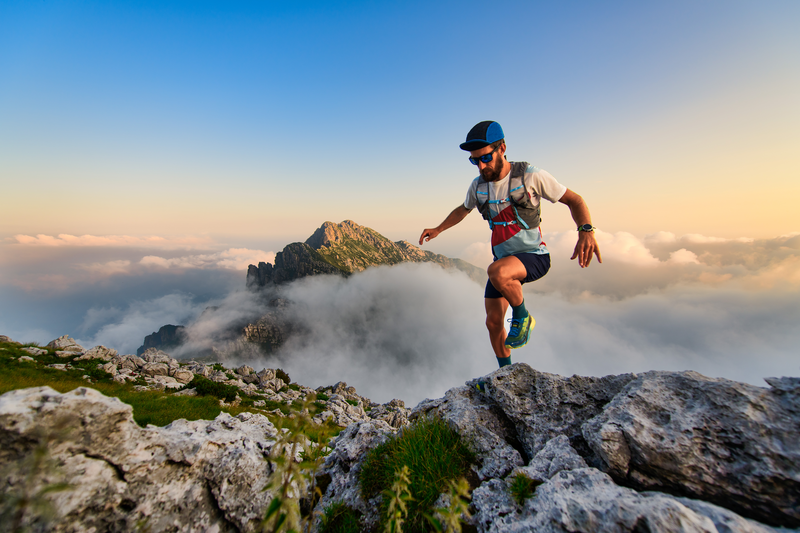
95% of researchers rate our articles as excellent or good
Learn more about the work of our research integrity team to safeguard the quality of each article we publish.
Find out more
PERSPECTIVE article
Front. Psychiatry , 18 August 2022
Sec. Neuroimaging
Volume 13 - 2022 | https://doi.org/10.3389/fpsyt.2022.958688
This article is part of the Research Topic Insights in Neuroimaging and Stimulation: 2022 View all 7 articles
Tourette syndrome (TS) is characterized by multiple motor and vocal tics, and high-comorbidity rates with other neuropsychiatric disorders. Obsessive compulsive disorder (OCD), attention deficit hyperactivity disorder (ADHD), autism spectrum disorders (ASDs), major depressive disorder (MDD), and anxiety disorders (AXDs) are among the most prevalent TS comorbidities. To date, studies on TS brain structure and function have been limited in size with efforts mostly fragmented. This leads to low-statistical power, discordant results due to differences in approaches, and hinders the ability to stratify patients according to clinical parameters and investigate comorbidity patterns. Here, we present the scientific premise, perspectives, and key goals that have motivated the establishment of the Enhancing Neuroimaging Genetics through Meta-Analysis for TS (ENIGMA-TS) working group. The ENIGMA-TS working group is an international collaborative effort bringing together a large network of investigators who aim to understand brain structure and function in TS and dissect the underlying neurobiology that leads to observed comorbidity patterns and clinical heterogeneity. Previously collected TS neuroimaging data will be analyzed jointly and integrated with TS genomic data, as well as equivalently large and already existing studies of highly comorbid OCD, ADHD, ASD, MDD, and AXD. Our work highlights the power of collaborative efforts and transdiagnostic approaches, and points to the existence of different TS subtypes. ENIGMA-TS will offer large-scale, high-powered studies that will lead to important insights toward understanding brain structure and function and genetic effects in TS and related disorders, and the identification of biomarkers that could help inform improved clinical practice.
Tourette syndrome (TS) is characterized by multiple, persistent motor and vocal tics, and affects approximately 0.6–1% of children worldwide (1). Tics are often preceded by premonitory urges and resemble voluntary actions but are patterned and repetitive and may also be voluntarily suppressed. These characteristics suggest that neural networks and brain regions associated with both voluntary and involuntary motor behavior and affective processes may be involved. There is no cure for TS and efforts to develop novel pharmacological treatments are hampered by our limited understanding of the neurobiology and brain structural and functional deficits that underlie the disorder. With 90% of patients with TS presenting comorbid with other neuropsychiatric disorders, our efforts to understand and treat this disorder are further complicated. Most frequent comorbid disorders in TS are found along the impulsive–compulsive spectrum and include obsessive compulsive disorder (OCD up to 50% of patients with TS), attention deficit hyperactivity disorder (ADHD up to 54%), and autism spectrum disorders (ASDs up to 20%). Major depressive disorder (MDD up to 26%) and anxiety disorders (AXDs up to 36%) are also often found comorbid with TS, especially in the clinical settings (1–3).
From a genetic perspective, TS is a complex disorder; multiple genes interact with environmental factors to lead to the onset of symptoms (4, 5). Recent multi-site studies have identified genome-wide significant susceptibility variants and pathways that implicate ligand-gated ion channel signaling (highlighting the role of GABA), immune, cell adhesion, and transsynaptic signaling processes in TS (6–9). From a pathophysiology perspective, the quest for the anatomical structure and brain circuits that underlie TS and tackle its clinical heterogeneity has proven challenging, impeded by low sample size and efforts mostly fragmented across multiple sites (10, 11). Here, we present the scientific premise, perspectives, and key goals that have motivated the recent establishment of the ENIGMA-TS (Enhancing NeuroImaging Genetics through Meta-Analysis for TS) working group. Leveraging an international network of collaborators, existing collections of data, and established infrastructure and pipelines for large-scale neuroimaging and genetics studies, ENIGMA-TS will help close major gaps in understanding brain structure and function in TS, help dissect the basis of the heterogeneity of its clinical presentation, and explore the underlying links between TS and its frequently comorbid disorders.
To date, structural and functional neuroimaging studies for TS have been limited in size and have produced mixed results that are sometimes not replicated across studies [reviewed in (1, 5, 12–16)]. In general, abnormal development and/or maintenance of cortico-striato-thalamo-cortical (CSTC) circuits are implicated (1, 11, 17). Several studies report lower prefrontal cortical thickness in patients with TS (12, 18–22). Beyond the prefrontal cortex, structural alterations have been documented in many other brain areas, involving most brain structures associated with sensorimotor processing (13, 16, 23–29). The largest multicenter structural magnetic resonance imaging (MRI) study of TS to date (103 patients with TS and 103 matched controls), found lower white matter volume bilaterally in the orbital and medial prefrontal cortex, and larger gray matter volume in the posterior thalamus, hypothalamus, and midbrain in patients with TS (12) (Figure 1A). Similarly, studies of the functional neuroanatomy of tic disorders have been very limited in size. Resting-state functional MRI (rsfMRI) can be used to assess an idle “ticcing” state and inform on the brain networks associated with the manifestation of tics, irrespective of specific cognitive (task-related) demands (11). TS rsfMRI studies show reduced long-range connectivity and increased short-distance connectivity associated with motor processing (30, 31). Overall, these findings could reflect the aberrant or “immature” brain development of individuals with TS. However, these reports are based on a handful of patients and controls, and much larger studies are required to provide definitive evidence.
Figure 1. Brain regions that have been implicated in TS and related disorders (A) volumetric MRI studies of key brain regions for TS and related disorders. TS was associated with larger subcortical volumes of thalamus and hypothalamus (12, 29). OCD in children was associated with larger subcortical volumes of thalamus, while OCD in adults was associated with larger subcortical volumes of pallidum and smaller subcortical volumes of hippocampus (35). ADHD was associated with smaller subcortical volumes of the caudate, putamen, amygdala, and nucleus accumbens (39). ASD was associated with smaller subcortical volumes of the pallidum, putamen, amygdala, and nucleus accumbens (41). MDD was associated with smaller subcortical volumes of hippocampus (55). (B) TS–ADHD–ASD GWAS and TS–OCD GWAS cross-disorder tissue specificity analysis, testing 30/53 tissue types from GTEx v7 tissue expression atlas (61). Significant enrichment of gene expression in corresponding tissue under Bonferroni correction (p < 1.67 × 10–3 for 30 tissues tested and p < 9.43 × 10–4 for 53 tissues tested). The green label indicates enrichment of gene expression in TS–ADHD–ASD Tissue Specificity Analysis. The red label indicates enrichment of gene expression in both TS–OCD and TS–ADHD–ASD tissue specificity analysis (Created with BioRender.com).
Small sample sizes have entailed low-statistical power and hampered the dissection of TS subtypes (on average less than 30 cases and 30 controls as reviewed in (11)). Furthermore, differences in analytic approaches have also contributed to inconsistent findings and limited reproducibility. ENIGMA (32) is an unprecedented worldwide initiative to tackle the crisis of reproducibility that comes from underpowered studies. Analyzing diverse worldwide samples (more than 40 countries and 1,400 investigators), ENIGMA has pioneered the identification of genetic markers that underlie cortical measures and subcortical volumes through neuroimaging genome-wide association studies (GWAS) (33, 34). Disorder-focused ENIGMA working groups have identified brain profiles for multiple psychiatric disorders and conditions based on an analysis of large worldwide datasets (32). These include the largest neuroimaging studies for multiple disorders that are highly correlated to TS: OCD (N = 5,423) (35–38) ADHD (N = 3,762) (39, 40), ASD (N = 3,222) (41), and MDD (N = 10,327) (42, 43) (Figure 1A).
The ENIGMA–TS working group was formed to address the need for large-scale studies to understand brain structure and function in TS and tackle clinical heterogeneity. The effort was motivated not only by ENIGMA but also TS–EUROTRAIN, an earlier collaborative consortium funded by the European Union (2012–2016), which represented an international network of researchers from 12 different sites in academia and industry aiming to understand the neurobiology of TS (5, 44–50). With a strong basis of collaboration, investigators from 23 sites and 12 countries (namely, Australia, Germany, Denmark, France, Italy, the Netherlands, Norway, Poland, Spain, the United Kingdom, China, and the United States) have already joined ENIGMA–TS and are currently working to pool and harmonize previously collected TS neuroimaging data with an initial goal of analysis for 1,000 TS individuals and a corresponding number of controls. The data mostly represent diverse European and European American ancestry and one cohort from China. There is an open and ongoing call for additional investigators to join ENIGMA–TS and information on how to join is available on the ENIGMA-TS website.1 Indeed, through our publications, conference presentations, our website, and access to the ENIGMA network, our goal is to draw additional membership, further expanding our sample size and representation of diverse populations in our studies.
The ENIGMA–TS working group uses standardized protocols for the processing and analysis of imaging data, to determine the reliability of effects across datasets, and identify common trends of low effect size that may not reach statistical significance in any individual study (37, 51, 52). We will use previously standardized methods to harmonize structural MRI, diffusion tensor imaging (DTI), and rsfMRI data across different sites, scanners, and imaging protocols (41, 53–55). In addition to greatly enhanced statistical power, data pooling allows novel comparisons across demographics (e.g., sex). In particular, our proposed analyses will have sufficiently large sample sizes to examine the effects of comorbidity and uncover different TS brain phenotypes or dimensions. Integrating with genetics, we will shed light on the links between TS and related disorders and underlying the brain structure and function (Figure 1B).
We can specify some hypotheses based on prior work, especially for structural MRI, which was the only case where sample sizes exceeded 100. The two largest previous structural studies in TS both found evidence for increased thalamic volume, especially in the posterior thalamus (12, 15). Several studies identified lower gray or white matter in the orbito-frontal cortex in TS (12, 18–20, 22, 56, 57). Others identified case–control differences in TS relating to the primary motor cortex (13). Studies of striatal volumes in TS have shown inconsistent results (12, 15), as have white matter DTI and functional connectivity rsfMRI results, but mostly included much smaller sample sizes compared to what we will achieve (13). However, rsfMRI has been demonstrated to contain diagnostically relevant information in TS (58). Several structural MRI reports suggest effects of age, sex, and comorbidity, supporting our plans to include such information in our models. In addition, both similarities and differences have been noted with the spectrum of childhood onset disorders that often occur comorbid with TS. For example, higher thalamic volume was also noted in the pediatric OCD (36, 59–62), whereas lower thalamic volume has been reported in ADHD (63–65). Lower gray or white matter volumes in the orbito-frontal cortex was seen in TS (9, 31, 58, 66–69) and also in the ADHD and OCD (18). Our hypotheses strongly motivate cross-disorder analyses, which we will also present later in this article.
The TS has a complex and heterogeneous genetic basis, with both common and rare variants contributing to risk (6–9, 70–78) (Figure 2A). The largest TS copy number variant (CNV) analysis performed to date (2,434 TS cases and 4,093 matched controls) (9), identified the first two genome-wide significant rare loci for TS (namely, NRXN1 deletions, and CNTN6 duplications) (9). The largest family exome-sequencing study for TS to date (800 trios), also pointed to de novo mutations that contribute to TS risk (8, 70), implicating two high-confidence TS risk genes, WWC1 and CELSR3. On the contrary, focusing on common genetic variants, a large GWAS by Yu et al. (6) and follow-up studies showed that ligand-gated ion channel signaling, immune, cell adhesion, and transsynaptic-signaling processes are involved in TS (7). Analysis of an even larger GWAS for TS bringing together all major consortia working on TS genetics in a study of more than 12,000 patients is currently underway, promising novel insights into the genetics of TS by uncovering additional genes and pathways that underlie disease risk. Already, intermediate results point to two additional novel candidates for TS (79).
Figure 2. Genetics vs. brain structure in TS (A) network of the GO: Biological processes (GO:BP) terms from key genes previously implicated in TS as reviewed in the text. Enrichment analysis of the genes implicated in TS was performed with the ToppFun function in Toppgene (https://toppgene.cchmc.org/). Terms with p < 0.05 after FDR correction were considered statistically significant. Related GO:BP terms including the same genes were collapsed into a single term. Cytoscape (https://cytoscape.org/) was used for network visualization. The following genes were included: ASH1L (72), CD180 (7), CDH26 (7), CELSR3 (8, 70), CNTN6 (9), COL8A1 (71), CTNNA3 (93), FLT3 (7), GABBR2 (7), GABRG1 (7), GRIK4 (7), HCN1 (7), HDAC9 (7), HDC (74–76), IL12A (7), KIF26B (72), NCAM2 (7), NCR1 (7), NLRP7 (7), NRXN1 (9), NTM (7), ROBO2 (7), SLITRK1 (77, 78), WWC1 (8, 70). (B) Associations between TS PRS calculated based on the latest TS GWAS meta-analysis (79) and volume of 14 sub-cortical brain structures in UK Biobank. Each part was measured separately in the left hemisphere and the right hemisphere of the brain. Linear regression was performed with age, sex, genotyping batch, and top 10 PCs used as covariates in the analysis. The asterisk (*) indicates a significant association after multiple testing correction using the FDR method (p < 0.05). (C) Exploring the genetic architecture of TS and related comorbidities via genomic structural equation modeling. The path graph shows loads and corresponding standard errors in parenthesis.
Bringing together TS GWAS and ENIGMA data, Mufford et al. performed the first study aiming to map TS genes to brain structure (80) using summary statistics from the Yu et al. TS GWAS (6) and the ENIGMA GWAS of subcortical volumes (30,717 individuals), we examined the genetic pleiotropy (the same SNP affecting two traits) and concordance (the agreement in SNP effect directions across these two traits). We found significant pleiotropy between TS and putaminal and caudate volumes, independent of the direction of effect, and significant concordance between TS and lower thalamic volume. It should be noted that this analysis associates TS with smaller thalamus, whereas the two largest previous studies in TS suggested an opposite effect. This discordance emphasizes the need for a much larger TS neuroimaging study, such as the one to be undertaken by ENIGMA–TS.
Furthermore, preparing for the ENIGMA–TS analysis, we asked whether the polygenic risk score (PRS) based on the recent Tsetsos et al. TS GWAS (79) correlates with brain structures in neuroimaging data from 29,798 individuals from the UK Biobank (UKB) (Figure 2B). We observed that increase in the genetic risk of TS was significantly associated with decrease in right putamen (beta: -0.0175, adj.p: 0.0069) and left pallidum (beta: -0.0137, adj.p: 0.043) volumes. We also found significant correlations between TS PRS and bilateral thalamic volume (beta: -0.0132 to -0.0138). ENIGMA–TS will pursue further analysis based on the most up-to-date TS GWAS and also the latest ENIGMA and UKB MRI GWAS datasets. In ENIGMA, more than 50,000 people from diverse populations from around the world have been assessed and analyzed with GWAS and whole-brain MRI, including over 10,000 with DTI and over 10,000 with rsfMRI. UKB data on approximately 10,000 individuals have also been recently integrated with ENIGMA (34, 81), while an even larger UKB dataset is now available. ENIGMA has now identified multiple genetic variants determining brain structure, namely, intracranial volume (ICV) (82) and subcortical volumes (33, 34, 83, 84). Our recent work also provides genetic determinants for regional and global measures of cortical surface area and thickness (34, 85). These rich datasets will be leveraged to gain insights into the brain structural measures that correlate with TS genetic risk.
With 90% of patients with TS presenting with additional neuropsychiatric comorbidities, understanding the molecular, pathophysiological, and neuroanatomical underpinnings of TS should also extend to investigating relationships to other comorbid disorders, with ADHD, ASD, OCD, MDD, and AXD being among the most prevalent (1–3). We recently performed the largest cross-disorder meta-analysis for TS, ADHD, ASD, and OCD, analyzing 124,000 samples and 6.8 million single nucleotide polymorphisms (SNPs) (66, 86). We showed that the hypothalamus-pituitary-adrenal (HPA) gland axis—and thus stress response—plays an important role in the shared pathophysiology of the studied disorders. Given the high comorbidity of TS to MDD and AXD, ENIGMA–TS will extend the cross-disorder analysis to include these additional comorbidities. Figure 2C shows our exploratory factor analysis of the genetic correlation matrix produced from multivariable LD Score Regression (LDSR), across the TS, ADHD, ASD, and OCD datasets described in Yang et al. (66) and large-scale GWAS on MDD and AXD (142,646 and 17,310 individuals, respectively) (87, 88). To do this, we used the R package, GenomicSEM (89). Identified factors highlight shared genetic liability across the studied disorders. We would like to point out the existence of a TS + OCD factor that is anticorrelated to ADHD and a shared liability factor with contributions across TS, ADHD, ASD, and MDD. Based on such analyses, we will pursue additional GWAS meta-analysis aiming to identify genetic susceptibility loci of different genetic factors along this phenotypic spectrum. We will then seek to correlate brain structure differences for the studied disorders to the genetic variants that underlie the brain structure in ENIGMA and UKB GWAS.
At a pathophysiological level, the association of tics with psychiatric comorbidities may result from the disruption of several cortico-basal ganglia loops. For instance, several behavioral and neuroimaging studies suggest the involvement of partly overlapping, albeit still separate, fronto-striatal circuits in both TS and ADHD (18, 22, 90). Although studies that compare TS to OCD have not been reported so far, the presence of OCD in patients with TS was found to be associated with volume reduction in the caudate nucleus (14), and lower cortical thickness in the ventromedial prefrontal cortex and hippocampus (22).
ENIGMA recently created pipelines that allowed a first cross-disorder analysis of cortical and subcortical brain structure across three of the disorders that appear often comorbid in TS (ADHD, ASD, and OCD) (54). Structural T1-weighted brain MRI scans of controls (n = 5,827) and individuals with ADHD (n = 2,271), OCD (n = 2,323), and ASD (n = 1,777) from 151 datasets worldwide were analyzed using standardized ENIGMA processing protocols. Subcortical volume and regional cortical thickness differences were examined in a mega-analytical framework (54). Analyses were performed separately for children, adolescents, and adults using linear mixed-effects models controlling for age, sex, and site (and ICV for subcortical measures). Lifespan dynamics were found in the pairwise findings: Children with ADHD compared with those with OCD had smaller hippocampal volumes, possibly influenced by IQ. Children and adolescents with ADHD had smaller ICV than controls and those with OCD or ASD. Adults with ASD showed thicker frontal cortices compared with controls and other clinical groups. No OCD-specific alterations across age groups—or surface area alterations among all disorders in childhood and adulthood—were observed. Furthermore, differences between medicated and unmedicated patients, and effects of duration of illness and age of onset were identified (54). Through collaboration with the relevant ENIGMA working groups, ENIGMA–TS will extend this work across TS, and ADHD, OCD, ASD, MDD, and AXD, analyzing a combined worldwide dataset of unprecedented power. Our work will yield brain maps of the main effects of each disorder, in children, adolescents, and adults, ranking brain metrics for effect sizes, and detection of metrics with common and disease-specific brain alterations.
Recent work supports a continuity of behavioral disorders–related traits across the population, with patients being at one extreme of the distribution. For instance, Demontis et al. found that ADHD symptoms in the general population are determined by largely the same genetic factors as those associated with a clinical diagnosis of ADHD (91). Robinson et al. showed that similar continuity from the general population to the clinical phenotype exists for ASD-related traits (92); also confirmed by others (93) and for OCD symptoms (94). In a similar manner, a recent study showed that PRS from the Yu et al. TS GWAS (6) predicted the presence of tics in a general population cohort (95). Such continuity thus allows us to extend the case–control findings to large population cohorts and to study the underlying mechanisms in more detail in terms of the roles of specific symptom domains and brain regions. More broadly, it also allows us to move toward the identification of diagnostic and prognostic biomarkers. ENIGMA–TS will seek to explore the value of the TS genetic and neuroimaging biomarkers that will be identified based on our studies to predict related symptoms in population-based cohorts. To do this, we will analyze large population studies (ABCD and Generation R cohorts) (96–100) for which symptoms and behavioral traits related to TS and its highly comorbid disorders of interest and genomic and MRI measures are available.
We have presented the background, rationale, and perspectives that support the establishment of the ENIGMA–TS working group and motivate our mission. Our large-scale, high-powered studies, integrating data from multiple countries, have the potential to offer a major breakthrough in the quest to understand brain structure and function and genetic effects in TS and correlated disorders. ENIGMA’s global approach offers higher power to detect factors that underlie TS onset and disease progression and test the generalizability of brain biomarkers in diverse samples across the globe. ENIGMA–TS already has partnerships with 12 countries while the ENIGMA reference neuroimaging GWAS includes samples from more than 40 countries around the world.
For the first time, ENIGMA–TS will undertake a large-scale cross-disorder study of brain structure and function and genetic susceptibility across TS and often comorbid OCD, ADHD, ASD, MDD, and AXD. We aim to identify biomarkers for disease subtypes that cut across diagnostic boundaries, lifting a major barrier in truly understanding factors that drive high comorbidity in patients with TS. Although our initial studies include mostly European datasets, we will make every effort to extend analyses to include representation from more diverse datasets through ENIGMA-available resources, public databases, and through our open call for additional collaborations.
To date, all major progress in understanding the genetics of TS and other neuropsychiatric disorders has been realized thanks to international collaborative efforts. Through ENIGMA–TS, we will seek to replicate this success to understand brain structure and function in TS and related disorders bringing together investigators working on the genetics and neuroimaging of these common disorders. Our joint work can offer far-reaching implications for future research, including the identification of robust multimodal markers of disease burden, and may ultimately lead to new therapies, improved patient management, and improved quality of life for patients and their families.
Publicly available datasets were analyzed in this study. This data can be found here: https://www.med.unc.edu/pgc/.
PP designed the study and experiments, coordinated the study and data integration and analysis, and wrote the manuscript. YJ performed the experiments, analyzed and interpreted the data, and wrote the manuscript. KM-V, BF, OH, NJ, PT, and KB designed the study and experiments, contributed to the data, interpreted the results, and wrote the manuscript. All authors contributed data and methods, contributed to results interpretation, analyzed data, and wrote the manuscript.
This work was supported by NIMH grant no. 1R01MH126213 and NSF IIS grant no. 1715202 to PP, the Innovative Medicines Initiative 2 Joint Undertaking (grant no. 777394) to NF, the NIH (grant nos. R01MH118217 and K01MH104592) to DG, the NIH (grant nos. R01MH126213, R01MH116147, and P41EB015922) to NJ, the VI-PPIT-US from the University of Seville (grant no. USE-18817-A) to JM-R, the Lundbeck Foundation, the Dagmar Marshall Foundation, the Bøhmske Foundation, the Carpenter Jørgen Holm, and wife Elisa born Hansen Memorial Foundation, the Queen Louise’s Children’s Hospital Foundation, and the King Christian X Foundation to NM, the NIH (grant nos. R01MH126213, R01MH116147, and P41EB015922) to PT and ST.
Authors IN and SR were employed by the company Forschungszentrum Jülich GmbH.
The remaining authors declare that the research was conducted in the absence of any commercial or financial relationships that could be construed as a potential conflict of interest.
All claims expressed in this article are solely those of the authors and do not necessarily represent those of their affiliated organizations, or those of the publisher, the editors and the reviewers. Any product that may be evaluated in this article, or claim that may be made by its manufacturer, is not guaranteed or endorsed by the publisher.
1. Robertson MM, Eapen V, Singer HS, Martino D, Scharf JM, Paschou P, et al. Gilles de la Tourette syndrome. Nat Rev Dis Prim. (2017) 3:16097. doi: 10.1038/nrdp.2016.97
2. Robertson MM. A personal 35 year perspective on Gilles de la Tourette syndrome: prevalence, phenomenology, comorbidities, and coexistent psychopathologies. Lancet Psychiatry. (2015) 2:68–87. doi: 10.1016/S2215-0366(14)00132-1
3. Hirschtritt ME, Lee PC, Pauls DL, Dion Y, Grados MA, Illmann C, et al. Lifetime prevalence, age of risk, and genetic relationships of comorbid psychiatric disorders in Tourette syndrome. JAMA Psychiatry. (2015) 72:325–33. doi: 10.1001/jamapsychiatry.2014.2650
4. Paschou P. The genetic basis of Gilles de la Tourette syndrome. Neurosci Biobehav Rev. (2013) 37:1026–39. doi: 10.1016/j.neubiorev.2013.01.016
5. Georgitsi M, Willsey AJ, Mathews CA, State M, Scharf JM, Paschou P. The genetic etiology of Tourette syndrome: large-scale collaborative efforts on the precipice of discovery. Front Neurosci. (2016) 10:351. doi: 10.3389/fnins.2016.00351
6. Yu D, Sul JH, Tsetsos F, Nawaz MS, Huang AY, Zelaya I, et al. Interrogating the genetic determinants of Tourette’s syndrome and other tiC disorders through genome-wide association studies. Am J Psychiatry. (2019) 176:217–27. doi: 10.1176/appi.ajp.2018.18070857
7. Tsetsos F, Yu D, Sul JH, Huang AY, Illmann C, Osiecki L, et al. Synaptic processes and immune-related pathways implicated in Tourette syndrome. Transl Psychiatry. (2021) 11:56. doi: 10.1038/s41398-020-01082-z
8. Wang S, Mandell JD, Kumar Y, Sun N, Morris MT, Arbelaez J, et al. De novo sequence and copy number variants are strongly associated with Tourette disorder and implicate cell polarity in pathogenesis. Cell Rep. (2018) 24:3441.e–54.e. doi: 10.1016/j.celrep.2018.08.082
9. Huang AY, Yu D, Davis LK, Sul JH, Tsetsos F, Ramensky V, et al. Rare copy number variants in NRXN1 and CNTN6 increase risk for Tourette syndrome. Neuron. (2017) 94:1101.e–11.e. doi: 10.1016/j.neuron.2017.06.010
10. Paschou P, Müller-Vahl K. Editorial: the neurobiology and genetics of Gilles de la Tourette syndrome: new avenues through large-scale collaborative projects. Front Psychiatry. (2017) 8:197. doi: 10.3389/fpsyt.2017.00197
11. Martino D, Ganos C, Worbe Y. Neuroimaging applications in Tourette’s syndrome. Int Rev Neurobiol. (2018) 143:65–108. doi: 10.1016/bs.irn.2018.09.008
12. Greene DJ, Williams AC, Koller JM, Schlaggar BL, Black KJ. Brain structure in pediatric Tourette syndrome. Mol Psychiatry. (2017) 22:972–80. doi: 10.1038/mp.2016.194
13. Greene DJ, Black KJ, Schlagger BJ. Neurobiology and functional anatomy of tic disorders. In: D Martino, JF Leckman editors. Tourette Syndrome. New York, NY: Oxford University Press (2013). p. 238–75. doi: 10.1093/med/9780199796267.003.0012
14. Bloch MH, Leckman JF, Zhu H, Peterson BS. Caudate volumes in childhood predict symptom severity in adults with Tourette syndrome. Neurology. (2005) 65:1253–8. doi: 10.1212/01.wnl.0000180957.98702.69
15. Miller AM, Bansal R, Hao X, Sanchez-Pena JP, Sobel LJ, Liu J, et al. Enlargement of thalamic nuclei in tourette syndrome. Arch Gen Psychiatry. (2010) 67:955–64. doi: 10.1001/archgenpsychiatry.2010.102
16. Wan X, Zhang S, Wang W, Su X, Li J, Yang X, et al. Gray matter abnormalities in Tourette syndrome: a meta-analysis of voxel-based morphometry studies. Transl Psychiatry. (2021) 11:287. doi: 10.1038/s41398-021-01394-8
17. Mink JW. Neurobiology of basal ganglia and Tourette syndrome: basal ganglia circuits and thalamocortical outputs. Adv Neurol. (2006) 99:89–98.
18. Draganski B, Martino D, Cavanna AE, Hutton C, Orth M, Robertson MM, et al. Multispectral brain morphometry in Tourette syndrome persisting into adulthood. Brain. (2010) 133:3661–75. doi: 10.1093/brain/awq300
19. Fahim C, Yoon U, Das S, Lyttelton O, Chen J, Arnaoutelis R, et al. Somatosensory-motor bodily representation cortical thinning in Tourette: effects of tic severity, age and gender. Cortex. (2010) 46:750–60. doi: 10.1016/j.cortex.2009.06.008
20. Müller-Vahl KR, Kaufmann J, Grosskreutz J, Dengler R, Emrich HM, Peschel T. Prefrontal and anterior cingulate cortex abnormalities in Tourette syndrome: evidence from voxel-based morphometry and magnetization transfer imaging. BMC Neurosci. (2009) 10:47. doi: 10.1186/1471-2202-10-47
21. Sowell ER, Kan E, Yoshii J, Thompson PM, Bansal R, Xu D, et al. Thinning of sensorimotor cortices in children with Tourette syndrome. Nat Neurosci. (2008) 11:637–9. doi: 10.1038/nn.2121
22. Worbe Y, Gerardin E, Hartmann A, Valabrégue R, Chupin M, Tremblay L, et al. Distinct structural changes underpin clinical phenotypes in patients with Gilles de la Tourette syndrome. Brain. (2010) 133(Pt 12):3649–60. doi: 10.1093/brain/awq293
23. Ganos C, Roessner V, Münchau A. The functional anatomy of Gilles de la Tourette syndrome. Neurosci Biobehav Rev. (2013) 37:1050–62. doi: 10.1016/j.neubiorev.2012.11.004
24. Greene DJ, Schlaggar BL, Black KJ. Neuroimaging in Tourette syndrome: research highlights from 2014 to 2015. Curr Dev Disord Rep. (2015) 2:300–8. doi: 10.1007/s40474-015-0062-6
25. Hashemiyoon R, Kuhn J, Visser-Vandewalle V. Putting the pieces together in Gilles de la Tourette syndrome: exploring the link between clinical observations and the biological basis of dysfunction. Brain Topogr. (2017) 30:3–29. doi: 10.1007/s10548-016-0525-z
26. Liu Y, Wang J, Zhang J, Wen H, Zhang Y, Kang H, et al. Altered Spontaneous brain activity in children with early Tourette syndrome: a resting-state fMRI study. Sci Rep. (2017) 7:4808. doi: 10.1038/s41598-017-04148-z
27. Neuner I, Schneider F, Shah NJ. Functional neuroanatomy of tics. Int Rev Neurobiol. (2013) 112:35–71. doi: 10.1016/B978-0-12-411546-0.00002-0
28. Worbe Y, Lehericy S, Hartmann A. Neuroimaging of tic genesis: present status and future perspectives. Mov Disord. (2015) 30:1179–83. doi: 10.1002/mds.26333
29. Kim S, Greene DJ, D’andrea CB, Bihun EC, Koller JM, O’reilly B, et al. Hippocampal volume in provisional tic disorder predicts tic severity at 12-month follow-up. J Clin Med. (2020) 9:1715. doi: 10.3390/jcm9061715
30. Church JA, Fair DA, Dosenbach NUF, Cohen AL, Miezin FM, Petersen SE, et al. Control networks in paediatric Tourette syndrome show immature and anomalous patterns of functional connectivity. Brain. (2009) 132(Pt 1):225–38. doi: 10.1093/brain/awn223
31. Worbe Y, Malherbe C, Hartmann A, Pélégrini-Issac M, Messé A, Vidailhet M, et al. Functional immaturity of cortico-basal ganglia networks in Gilles de la Tourette syndrome. Brain. (2012) 135(Pt 6):1937–46. doi: 10.1093/brain/aws056
32. Thompson PM, Jahanshad N, Ching CRK, Salminen LE, Thomopoulos SI, Bright J, et al. ENIGMA and global neuroscience: a decade of large-scale studies of the brain in health and disease across more than 40 countries. Transl Psychiatry. (2020) 10:100. doi: 10.1038/s41398-020-0705-1
33. Satizabal CL, Adams HHH, Hibar DP, White CC, Knol MJ, Stein JL, et al. Genetic architecture of subcortical brain structures in 38,851 individuals. Nat Genet. (2019) 51:1624–36. doi: 10.1038/s41588-019-0511-y
34. Grasby KL, Jahanshad N, Painter JN, Colodro-Conde L, Bralten J, Hibar DP, et al. The genetic architecture of the human cerebral cortex. Science. (2020) 367:eaay6690. doi: 10.1126/science.aay6690
35. Boedhoe PSW, Schmaal L, Abe Y, Ameis SH, Arnold PD, Batistuzzo MC, et al. Distinct subcortical volume alterations in pediatric and adult OCD: a worldwide meta- and mega-analysis. Am J Psychiatry. (2017) 174:60–9. doi: 10.1176/appi.ajp.2016.16020201
36. Weeland CJ, Kasprzak S, de Joode NT, Abe Y, Alonso P, Ameis SH, et al. The thalamus and its subnuclei—a gateway to obsessive-compulsive disorder. Transl Psychiatry. (2022) 12:70. doi: 10.1038/s41398-022-01823-2
37. van den Heuvel OA, Boedhoe PSW, Bertolin S, Bruin WB, Francks C, Ivanov I, et al. An overview of the first 5 years of the ENIGMA obsessive–compulsive disorder working group: the power of worldwide collaboration. Hum Brain Mapp. (2022) 43:23–36. doi: 10.1002/hbm.24972
38. Boedhoe PSW, Heymans MW, Schmaal L, Abe Y, Alonso P, Ameis SH, et al. An empirical comparison of meta– and mega-analysis with data from the ENIGMA obsessive-compulsive disorder working group. Front Neuroinform. (2019) 12:102. doi: 10.3389/fninf.2018.00102
39. Hoogman M, Bralten J, Hibar DP, Mennes M, Zwiers MP, Schweren LSJ, et al. Subcortical brain volume differences in participants with attention deficit hyperactivity disorder in children and adults: a cross-sectional mega-analysis. Lancet Psychiatry. (2017) 4:310–9. doi: 10.1016/S2215-0366(17)30049-4
40. Postema MC, Hoogman M, Ambrosino S, Asherson P, Banaschewski T, Bandeira CE, et al. Analysis of structural brain asymmetries in attention-deficit/hyperactivity disorder in 39 datasets. J Child Psychol Psychiatry Allied Discip. (2021) 62:1202–19. doi: 10.1111/jcpp.13396
41. Van Rooij D, Anagnostou E, Arango C, Auzias G, Behrmann M, Busatto GF, et al. Cortical and subcortical brain morphometry differences between patients with autism spectrum disorder and healthy individuals across the lifespan: results from the ENIGMA ASD working group. Am J Psychiatry. (2018) 175:359–69. doi: 10.1176/appi.ajp.2017.17010100
42. Schmaal L, Hibar DP, Sämann PG, Hall GB, Baune BT, Jahanshad N, et al. Cortical abnormalities in adults and adolescents with major depression based on brain scans from 20 cohorts worldwide in the ENIGMA major depressive disorder working group. Mol Psychiatry. (2017) 22:900–9. doi: 10.1038/mp.2016.60
43. Opel N, Thalamuthu A, Milaneschi Y, Grotegerd D, Flint C, Leenings R, et al. Brain structural abnormalities in obesity: relation to age, genetic risk, and common psychiatric disorders: evidence through univariate and multivariate mega-analysis including 6420 participants from the ENIGMA MDD working group. Mol Psychiatry. (2021) 26:4839–52. doi: 10.1038/s41380-020-0774-9
44. Forde NJ, Kanaan AS, Widomska J, Padmanabhuni SS, Nespoli E, Alexander J, et al. TS-EUROTRAIN: a European-wide investigation and training network on the etiology and pathophysiology of Gilles de la Tourette syndrome. Front Neurosci. (2016) 10:384. doi: 10.3389/fnins.2016.00384
45. Kanaan AS, Gerasch S, García-García I, Lampe L, Pampel A, Anwander A, et al. Pathological glutamatergic neurotransmission in Gilles de la Tourette syndrome. Brain. (2017) 140:218–34. doi: 10.1093/brain/aww285
46. Forde NJ, Ronan L, Zwiers MP, Alexander-Bloch AF, Faraone SV, Oosterlaan J, et al. No association between cortical gyrification or intrinsic curvature and attention-deficit/hyperactivity disorder in adolescents and young adults. Front Neurosci. (2017) 11:218. doi: 10.3389/fnins.2017.00218
47. Fan S, van den Heuvel OA, Cath DC, van der Werf YD, de Wit SJ, de Vries FE, et al. Mild white matter changes in un-medicated obsessive-compulsive disorder patients and their unaffected siblings. Front Neurosci. (2016) 9:495. doi: 10.3389/fnins.2015.00495
48. Gerasch S, Kanaan AS, Jakubovski E, Müller-Vahl KR. Aripiprazole improves associated comorbid conditions in addition to tics in adult patients with Gilles de la Tourette syndrome. Front Neurosci. (2016) 10:416. doi: 10.3389/fnins.2016.00416
49. Haense C, Müller-Vahl KR, Wilke F, Schrader C, Capelle HH, Geworski L, et al. Effect of deep brain stimulation on regional cerebral blood flow in patients with medically refractory tourette syndrome. Front Psychiatry. (2016) 7:118. doi: 10.3389/fpsyt.2016.00118
50. Lessov-Schlaggar CN, Rubin JB, Schlaggar BL. The fallacy of univariate solutions to complex systems problems. Front Neurosci. (2016) 10:267. doi: 10.3389/fnins.2016.00267
51. Hoogman M, van Rooij D, Klein M, Boedhoe P, Ilioska I, Li T, et al. Consortium neuroscience of attention deficit/hyperactivity disorder and autism spectrum disorder: the ENIGMA adventure. Hum Brain Mapp. (2022) 43:37–55. doi: 10.1002/hbm.25029
52. Kong XZ, Mathias SR, Guadalupe T, Abé C, Agartz I, Akudjedu TN, et al. Mapping cortical brain asymmetry in 17,141 healthy individuals worldwide via the ENIGMA consortium. Proc Natl Acad Sci USA. (2018) 115:E5154–63. doi: 10.1073/pnas.1718418115
53. Hoogman M, Muetzel R, Guimaraes JP, Shumskaya E, Mennes M, Zwiers MP, et al. Brain imaging of the cortex in ADHD: a coordinated analysis of large-scale clinical and population-based samples. Am J Psychiatry. (2019) 176:531–42. doi: 10.1176/appi.ajp.2019.18091033
54. Boedhoe PSW, van Rooij D, Hoogman M, Twisk JWR, Schmaal L, Abe Y, et al. Subcortical brain volume, regional cortical thickness, and cortical surface area across disorders: findings from the ENIGMA ADHD ASD, and OCD working groups. Am J Psychiatry. (2020) 177:834–43. doi: 10.1176/appi.ajp.2020.19030331
55. Schmaal L, Veltman DJ, Van Erp TGM, Smann PG, Frodl T, Jahanshad N, et al. Subcortical brain alterations in major depressive disorder: findings from the ENIGMA major depressive disorder working group. Mol Psychiatry. (2016) 21:806–12. doi: 10.1038/mp.2015.69
56. Cheng B, Braass H, Ganos C, Treszl A, Biermann-Ruben K, Hummel FC, et al. Altered intrahemispheric structural connectivity in Gilles de la Tourette syndrome. NeuroImage Clin. (2014) 4:174–81. doi: 10.1016/j.nicl.2013.11.011
57. Peterson BS, Staib L, Scahill L, Zhang H, Anderson C, Leckman JF, et al. Regional brain and ventricular volumes in Tourette syndrome. Arch Gen Psychiatry. (2001) 58:427–40. doi: 10.1001/archpsyc.58.5.427
58. Greene DJ, Church JA, Dosenbach NUF, Nielsen AN, Adeyemo B, Nardos B, et al. Multivariate pattern classification of pediatric Tourette syndrome using functional connectivity MRI. Dev Sci. (2016) 19:581–98. doi: 10.1111/desc.12407
59. Atmaca M, Yildirim H, Ozdemir H, Tezcan E, Kursad Poyraz A. Volumetric MRI study of key brain regions implicated in obsessive-compulsive disorder. Prog Neuro Psychopharmacol Biol Psychiatry. (2007) 31:46–52. doi: 10.1016/j.pnpbp.2006.06.008
60. Kim JJ, Lee MC, Kim J, Kim IY, Kim SI, Han MH, et al. Grey matter abnormalities in obsessive-compulsive disorder: statistical parametric mapping of segmented magnetic resonance images. Br J Psychiatry. (2001) 179:330–4. doi: 10.1192/bjp.179.4.330
61. Weeland CJ, Vriend C, van der Werf Y, Huyser C, Hillegers M, Tiemeier H, et al. Thalamic subregions and obsessive-compulsive symptoms in 2,500 children from the general population. J Am Acad Child Adolesc Psychiatry. (2022) 61:321–30. doi: 10.1016/j.jaac.2021.05.024
62. Weeland CJ, White T, Vriend C, Muetzel RL, Starreveld J, Hillegers MHJ, et al. Brain morphology associated with obsessive-compulsive symptoms in 2,551 children from the general population. J Am Acad Child Adolesc Psychiatry. (2021) 60:470–8. doi: 10.1016/j.jaac.2020.03.012
63. Ivanov I, Bansal R, Hao X, Zhu H, Kellendonk C, Miller L, et al. Morphological abnormalities of the thalamus in youths with attention deficit hyperactivity disorder. Am J Psychiatry. (2010) 167:397–408. doi: 10.1176/appi.ajp.2009.09030398
64. Seidman LJ, Biederman J, Liang L, Valera EM, Monuteaux MC, Brown A, et al. Gray matter alterations in adults with attention-deficit/hyperactivity disorder identified by voxel based morphometry. Biol Psychiatry. (2011) 69:857–66. doi: 10.1016/j.biopsych.2010.09.053
65. Xia S, Li X, Kimball AE, Kelly MS, Lesser I, Branch C. Thalamic shape and connectivity abnormalities in children with attention– deficit/hyperactivity disorder. Psychiatry Res Neuroimaging. (2012) 204:161–7. doi: 10.1016/j.pscychresns.2012.04.011
66. Yang Z, Wu H, Lee PH, Tsetsos F, Davis LK, Yu D, et al. Investigating shared genetic basis across Tourette syndrome and comorbid neurodevelopmental disorders along the impulsivity-compulsivity spectrum. Biol Psychiatry. (2021) 90:317–27. doi: 10.1016/j.biopsych.2020.12.028
67. Hatton SN, Huynh KH, Bonilha L, Abela E, Alhusaini S, Altmann A, et al. White matter abnormalities across different epilepsy syndromes in adults: an ENIGMA-Epilepsy study. Brain. (2020) 143:2454–73. doi: 10.1093/brain/awaa200
68. Fortin JP, Parker D, Tunç B, Watanabe T, Elliott MA, Ruparel K, et al. Harmonization of multi-site diffusion tensor imaging data. Neuroimage. (2017) 161:149–70. doi: 10.1016/j.neuroimage.2017.08.047
69. Adhikari BM, Jahanshad N, Shukla D, Glahn DC, Blangero J, Reynolds RC, et al. Heritability estimates on resting state fMRI data using ENIGMA analysis pipeline. Pac Symp Biocomput. (2018) 23:307–18. doi: 10.1142/9789813235533_0029
70. Willsey AJ, Fernandez TV, Yu D, King RA, Dietrich A, Xing J, et al. De novo coding variants are strongly associated with Tourette disorder. Neuron. (2017) 94:486.e–99.e. doi: 10.1016/j.neuron.2017.04.024
71. Nag A, Bochukova EG, Kremeyer B, Campbell DD, Muller H, Valencia-Duarte AV, et al. CNV analysis in Tourette syndrome implicates large genomic rearrangements in COL8A1 and NRXN1. PLoS One. (2013) 8:e59061. doi: 10.1371/journal.pone.0059061
72. Liu S, Tian M, He F, Li J, Xie H, Liu W, et al. Mutations in ASH1L confer susceptibility to Tourette syndrome. Mol Psychiatry. (2020) 25:476–90. doi: 10.1038/s41380-019-0560-8
73. Sundaram SK, Huq AM, Wilson BJ, Chugani HT. Tourette syndrome is associated with recurrent exonic copy number variants. Neurology. (2010) 74:1583–90. doi: 10.1212/WNL.0b013e3181e0f147
74. Castellan Baldan L, Williams KA, Gallezot JD, Pogorelov V, Rapanelli M, Crowley M, et al. Histidine decarboxylase deficiency causes Tourette syndrome: parallel findings in humans and mice. Neuron. (2014) 81:77–90. doi: 10.1016/j.neuron.2013.10.052
75. Karagiannidis I, Dehning S, Sandor P, Tarnok Z, Rizzo R, Wolanczyk T, et al. Support of the histaminergic hypothesis in tourette syndrome: association of the histamine decarboxylase gene in a large sample of families. J Med Genet. (2013) 50:760–4. doi: 10.1136/jmedgenet-2013-101637
76. Ercan-Sencicek AG, Stillman AA, Ghosh AK, Bilguvar K, O’Roak BJ, Mason CE, et al. L-Histidine decarboxylase and Tourette’s Syndrome. N Engl J Med. (2010) 362:1901–8. doi: 10.1056/nejmoa0907006
77. Abelson JF, Kwan KY, O’Roak BJ, Baek DY, Stillman AA, Morgan TM, et al. Sequence variants in SLITRK1 are associated with Tourette’s syndrome. Science. (2005) 310:317–20.
78. Karagiannidis I, Rizzo R, Tarnok Z, Wolanczyk T, Hebebrand J, Nöthen MM, et al. Replication of association between a SLITRK1 haplotype and Tourette Syndrome in a large sample of families. Mol Psychiatry. (2012) 17:665–8. doi: 10.1038/mp.2011.151
79. Tsetsos F, Topaloudi A, Jain P, Yang Z, Yu D, Kolovos P, et al. Genome-wide association study identifies two novel loci for Gilles de la Tourette Syndrome. medRxiv [Preprint]. (2021). doi: 10.1101/2021.12.11.21267560
80. Mufford M, Cheung J, Jahanshad N, van der Merwe C, Ding L, Groenewold N, et al. Concordance of genetic variation that increases risk for Tourette syndrome and that influences its underlying neurocircuitry. Transl Psychiatry. (2019) 9:120. doi: 10.1038/s41398-019-0452-3
81. Elliott LT, Sharp K, Alfaro-Almagro F, Shi S, Miller KL, Douaud G, et al. Genome-wide association studies of brain imaging phenotypes in UK Biobank. Nature. (2018) 562:210–6. doi: 10.1038/s41586-018-0571-7
82. Adams HHH, Hibar DP, Chouraki V, Stein JL, Nyquist PA, Rentería ME, et al. Novel genetic loci underlying human intracranial volume identified through genome-wide association. Nat Neurosci. (2016) 19:1569–82. doi: 10.1038/nn.4398
83. Hibar DP, Stein JL, Renteria ME, Arias-Vasquez A, Desrivières S, Jahanshad N, et al. Common genetic variants influence human subcortical brain structures. Nature. (2015) 520:224–9. doi: 10.1038/nature14101
84. Hibar DP, Adams HHH, Jahanshad N, Chauhan G, Stein JL, Hofer E, et al. Novel genetic loci associated with hippocampal volume. Nat Commun. (2017) 8:13624. doi: 10.1038/ncomms13624
85. Stein JL, Medland SE, Vasquez AA, Hibar DP, Senstad RE, Winkler AM, et al. Identification of common variants associated with human hippocampal and intracranial volumes. Nat Genet. (2012) 44:552–61. doi: 10.1038/ng.2250
86. Yang Z, Wu H, Lee PH, Tsetsos F, Davis LK, Yu D, et al. Cross-disorder GWAS meta-analysis for attention deficit/hyperactivity disorder, autism spectrum disorder, obsessive compulsive disorder, and Tourette syndrome. bioRxiv [Preprint]. (2019). doi: 10.1101/770222
87. Wray NR, Ripke S, Mattheisen M, Trzaskowski M, Byrne EM, Abdellaoui A, et al. Genome-wide association analyses identify 44 risk variants and refine the genetic architecture of major depression. Nat Genet. (2018) 50:668–81. doi: 10.1038/s41588-018-0090-3
88. Otowa T, Hek K, Lee M, Byrne EM, Mirza SS, Nivard MG, et al. Meta-analysis of genome-wide association studies of anxiety disorders. Mol Psychiatry. (2016) 21:1391–9. doi: 10.1038/mp.2015.197
89. Grotzinger AD, Rhemtulla M, de Vlaming R, Ritchie SJ, Mallard TT, Hill WD, et al. Genomic structural equation modelling provides insights into the multivariate genetic architecture of complex traits. Nat Hum Behav. (2019) 3:513–25. doi: 10.1038/s41562-019-0566-x
90. Plessen KJ, Royal JM, Peterson BS. Neuroimaging of tic disorders with co-existing attention-deficit/hyperactivity disorder. Eur Child Adolesc Psychiatry. (2007) 16:60–70. doi: 10.1007/s00787-007-1008-2
91. Demontis D, Walters RK, Martin J, Mattheisen M, Als TD, Agerbo E, et al. Discovery of the first genome-wide significant risk loci for attention deficit/hyperactivity disorder. Nat Genet. (2019) 51:63–75. doi: 10.1038/s41588-018-0269-7
92. Bralten J, Van Hulzen KJ, Martens MB, Galesloot TE, Arias Vasquez A, Kiemeney LA, et al. Autism spectrum disorders and autistic traits share genetics and biology. Mol Psychiatry. (2018) 23:1205–12. doi: 10.1038/mp.2017.98
93. Robinson EB, St Pourcain B, Anttila V, Kosmicki JA, Bulik-Sullivan B, Grove J, et al. Genetic risk for autism spectrum disorders and neuropsychiatric variation in the general population. Nat Genet. (2016) 48:552–5. doi: 10.1038/ng.3529
94. Bralten J, Widomska J, Witte WD, Yu D, Mathews CA, Scharf JM, et al. Shared genetic etiology between obsessive-compulsive disorder, obsessive-compulsive symptoms in the population, and insulin signaling. Transl Psychiatry. (2020) 10:121. doi: 10.1038/s41398-020-0793-y
95. Abdulkadir M, Mathews CA, Scharf JM, Yu D, Tischfield JA, Heiman GA, et al. Polygenic risk scores derived from a Tourette syndrome genome-wide association study predict presence of tics in the avon longitudinal study of parents and children cohort. Biol Psychiatry. (2019) 85:298–304. doi: 10.1016/j.biopsych.2018.09.011
96. Casey BJ, Cannonier T, Conley MI, Cohen AO, Barch DM, Heitzeg MM, et al. The adolescent brain cognitive development (ABCD) study: imaging acquisition across 21 sites. Dev Cogn Neurosci. (2018) 32:43–54. doi: 10.1016/j.dcn.2018.03.001
97. Barch DM, Albaugh MD, Avenevoli S, Chang L, Clark DB, Glantz MD, et al. Demographic, physical and mental health assessments in the adolescent brain and cognitive development study: rationale and description. Dev Cogn Neurosci. (2018) 32:55–66. doi: 10.1016/j.dcn.2017.10.010
98. Volkow ND, Koob GF, Croyle RT, Bianchi DW, Gordon JA, Koroshetz WJ, et al. The conception of the ABCD study: from substance use to a broad NIH collaboration. Dev Cogn Neurosci. (2018) 32:4–7. doi: 10.1016/j.dcn.2017.10.002
99. Kooijman MN, Kruithof CJ, van Duijn CM, Duijts L, Franco OH, van IJzendoorn MH, et al. The generation R study: design and cohort update 2017. Eur J Epidemiol. (2016) 31:1243–64. doi: 10.1007/s10654-016-0224-9
Keywords: Tourette syndrome, neuroimaging, genetics, ENIGMA, brain MRI
Citation: Paschou P, Jin Y, Müller-Vahl K, Möller HE, Rizzo R, Hoekstra PJ, Roessner V, Mol Debes N, Worbe Y, Hartmann A, Mir P, Cath D, Neuner I, Eichele H, Zhang C, Lewandowska K, Munchau A, Verrel J, Musil R, Silk TJ, Hanlon CA, Bihun ED, Brandt V, Dietrich A, Forde N, Ganos C, Greene DJ, Chu C, Grothe MJ, Hershey T, Janik P, Koller JM, Martin-Rodriguez JF, Müller K, Palmucci S, Prato A, Ramkiran S, Saia F, Szejko N, Torrecuso R, Tumer Z, Uhlmann A, Veselinovic T, Wolańczyk T, Zouki J-J, Jain P, Topaloudi A, Kaka M, Yang Z, Drineas P, Thomopoulos SI, White T, Veltman DJ, Schmaal L, Stein DJ, Buitelaar J, Franke B, van den Heuvel O, Jahanshad N, Thompson PM and Black KJ (2022) Enhancing neuroimaging genetics through meta-analysis for Tourette syndrome (ENIGMA-TS): A worldwide platform for collaboration. Front. Psychiatry 13:958688. doi: 10.3389/fpsyt.2022.958688
Received: 31 May 2022; Accepted: 18 July 2022;
Published: 18 August 2022.
Edited by:
Roberto Canitano, Siena University Hospital, ItalyReviewed by:
Michael H. Bloch, Yale University, United StatesCopyright © 2022 Paschou, Jin, Müller-Vahl, Möller, Rizzo, Hoekstra, Roessner, Mol Debes, Worbe, Hartmann, Mir, Cath, Neuner, Eichele, Zhang, Lewandowska, Munchau, Verrel, Musil, Silk, Hanlon, Bihun, Brandt, Dietrich, Forde, Ganos, Greene, Chu, Grothe, Hershey, Janik, Koller, Martin-Rodriguez, Müller, Palmucci, Prato, Ramkiran, Saia, Szejko, Torrecuso, Tumer, Uhlmann, Veselinovic, Wolańczyk, Zouki, Jain, Topaloudi, Kaka, Yang, Drineas, Thomopoulos, White, Veltman, Schmaal, Stein, Buitelaar, Franke, van den Heuvel, Jahanshad, Thompson and Black. This is an open-access article distributed under the terms of the Creative Commons Attribution License (CC BY). The use, distribution or reproduction in other forums is permitted, provided the original author(s) and the copyright owner(s) are credited and that the original publication in this journal is cited, in accordance with accepted academic practice. No use, distribution or reproduction is permitted which does not comply with these terms.
*Correspondence: Peristera Paschou, cHBhc2Nob3VAcHVyZHVlLmVkdQ==
Disclaimer: All claims expressed in this article are solely those of the authors and do not necessarily represent those of their affiliated organizations, or those of the publisher, the editors and the reviewers. Any product that may be evaluated in this article or claim that may be made by its manufacturer is not guaranteed or endorsed by the publisher.
Research integrity at Frontiers
Learn more about the work of our research integrity team to safeguard the quality of each article we publish.