- 1Department of Chinese Medicine, The University of Hong Kong-Shenzhen Hospital, Shenzhen, China
- 2School of Chinese Medicine, The University of Hong Kong, Pokfulam, Hong Kong SAR, China
Depression is clinically defined as a mood disorder with persistent feeling of sadness, despair, fatigue, and loss of interest. The pathophysiology of depression is tightly regulated by the biosynthesis, transport and signaling of neurotransmitters [e.g., serotonin, norepinephrine, dopamine, or γ-aminobutyric acid (GABA)] in the central nervous system. The existing antidepressant drugs mainly target the dysfunctions of various neurotransmitters, while the efficacy of antidepressant therapeutics is undermined by different adverse side-effects. The present review aimed to dissect the molecular mechanisms underlying the antidepressant activities of herbal medicines toward the development of effective and safe antidepressant drugs. Our strategy involved comprehensive review and network pharmacology analysis for the active compounds and associated target proteins. As results, 45 different antidepressant herbal medicines were identified from various in vivo and in vitro studies. The antidepressant mechanisms might involve multiple signaling pathways that regulate neurotransmitters, neurogenesis, anti-inflammation, antioxidation, endocrine, and microbiota. Importantly, herbal medicines could modulate broader spectrum of the cellular pathways and processes to attenuate depression and avoid the side-effects of synthetic antidepressant drugs. The present review not only recognized the antidepressant potential of herbal medicines but also provided molecular insights for the development of novel antidepressant drugs.
1 Introduction
Depression is a common mental disease that seriously affects 5% of adults worldwide, especially postpartum women (1, 2). Diagnostic and statistical manual of mental disorders (DSM-5) divides depression disorder into eight categories: disruptive mood dysregulation disorder, major depressive disorder (including major depressive episode), persistent depressive disorder (dysthymia), premenstrual dysphoric disorder, substance/medication-induced depressive disorder, depressive disorder due to another medical condition, other specified depressive disorder, and unspecified depressive disorder (3). Patients with depression usually suffer from symptoms such as depressed mood, anxiety, loss of interest, lack of energy, pessimism, disappointment, self-denial and even suicidal thoughts, while 41% of depressed mothers may intend to harm their babies (4). Depression not only represents an ongoing medical challenge but also has emerged as a financial burden for global healthcare systems, for example, annual cost of nearly $210.5 billion in the United States (5). The existing treatments mainly alleviate depressive symptoms so that the remission rate is less than 60% (6). Most of antidepressant drugs cause different apparent adverse side-effects, resulting in the average withdrawal incidence rate of 56% (7, 8). Depression is well-known to be a multifactorial mental disease and exhibit various symptoms including sadness, anxiety, anger and irritability. Synthetic antidepressants are challenged by efficacy and severe side effects. Current first-line antidepressants like SSRIs and SNRIs are designed to specifically target the actions of serotonin and noradrenaline so that SSRIs and SNRIs may not be effective against depression as the result of multiple other causes (9). Thus, single-target therapies may fail in the treatment of multifactorial disease.
Nevertheless, 2.39–40% of patients in different countries and regions alternatively used herbal medicines (10–13). Encouragingly, traditional Chinese medicine (TCM) has achieved the effective use of herbal medicines to treat depression over thousands of years (14). Therefore, herbal medicines may serve as a rich source for the development of novel antidepressant therapies. These results stimulated us to examine the current understanding on the pathology of depression, the pharmacology of the existing antidepressant drugs and the antidepressant activity of herbal medicines toward the development of novel effective and safe antidepressant drugs.
2 Current understanding of depression
The causes of depression are complex, including genetic conditions, endocrine, mental state, living habits, and health status (15–17). Although the pathogenesis is complicated and remains elusive, several hypothesis/theories have been proposed to explain clinical manifestations from different perspectives. The pathology of depression was summarized in Figure 1 and elaborated as follows:
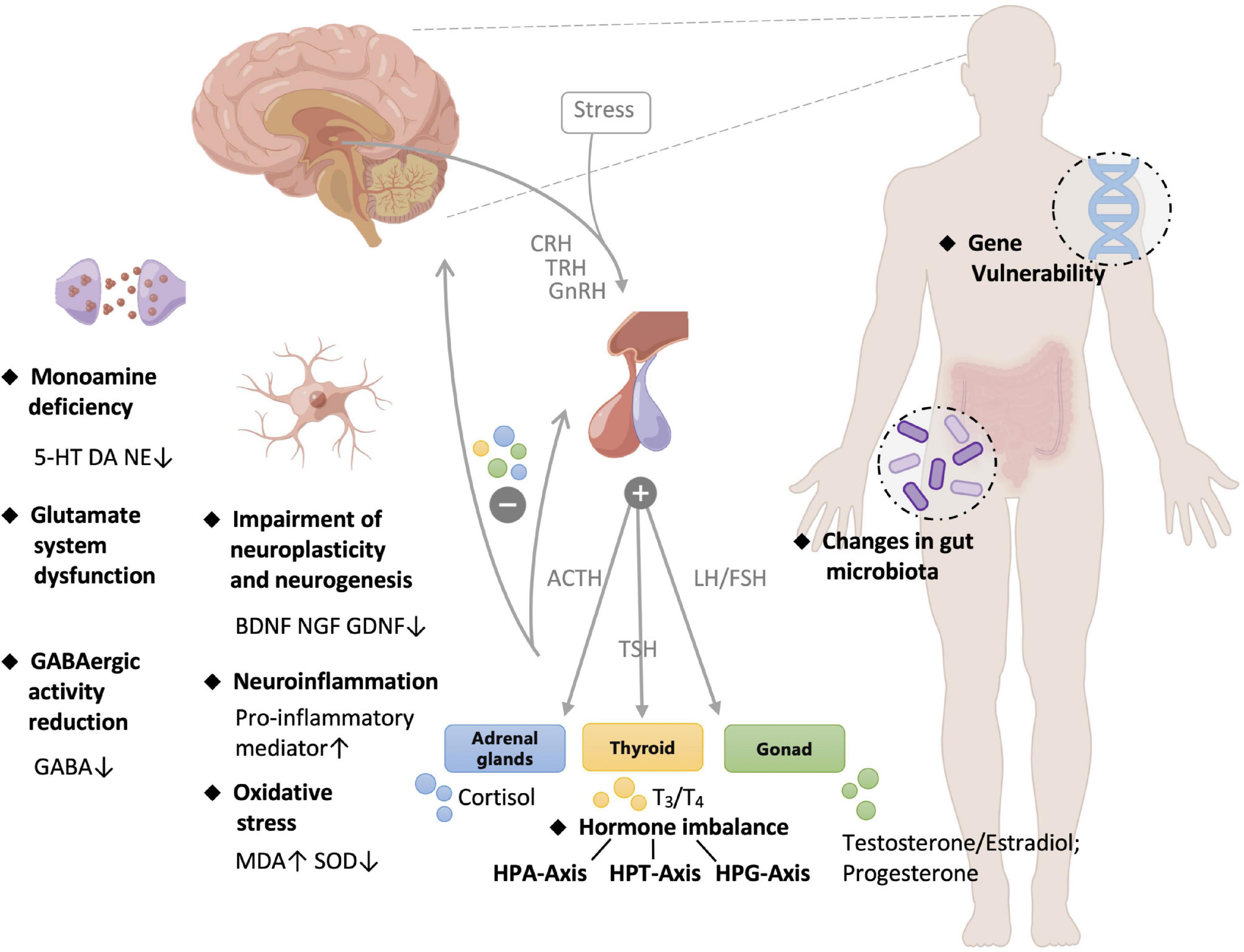
Figure 1. Pathology of depression. 5-HT, 5-hydroxytryptamine; DA, dopamine; NE, norepinephrine; GABA, gamma-aminobutyric acid; BDNF, brain derived neurotrophic factor; NGF, nerve growth factor; MDA, malondialdehyde; SOD, superoxide dismutase; CRH, corticotropin-releasing hormone; TRH, thyrotropin-releasing hormone; GnRH, gonadotropin-releasing hormone; ACTH, adrenocorticotropic hormone; TSH, thyroid stimulating hormone; LH, luteinizing hormone; FSH, follicle-stimulating hormone; HPA, hypothalamus-pituitary-adrenal; HPT, hypothalamic-pituitary-thyroid; HPG, hypothalamic–pituitary–gonadal.
2.1 Monoamine hypothesis
Joseph J. Schildkraut proposed monoamine hypothesis as early as in 1965. The monoamine hypothesis describes that depression is resulted from the abnormal transmission of monoamine neurotransmitters, including synaptic deprivation of monoamine neurotransmitters, and dysfunctions of monoamine transporter and receptors (18, 19). Monoamine theory guided scientists to develop a number of antidepressant drugs including monoamine oxidase inhibitor isoniazid isopropylhydrazide although the drug was originally used to tuberculosis (6). Indeed, 80% of the antidepressant drugs that were approved by the United States Food and Drug Administration (FDA) target monoamine transmitter systems (20). The therapeutic effects of such drugs somehow approved monoamine hypothesis. The tricyclic drug tianeptine is known to promote serotonin reuptake and exhibit similar antidepressant effect as selective serotonin reuptake inhibitor (SSRI). However, some patients feel worse after taking tianeptine (21). Such clinical phenomena challenged monoamine hypothesis. The changes in monoamine levels appear to be the consequences other than the causes of depression.
2.2 Glutamatergic hypothesis and GABAergic deficit hypothesis
Glutamate is an excitatory amino acid that plays an essential role in cognitive functions such as learning and memory. Clinical studies observed a higher level of plasma glutamate in patients with depression (22). Indeed, N-methyl-D-aspartate receptor (NMDA-R) antagonists showed the potency of relieving depression symptoms (23). Thus, the glutamate hypothesis was proposed to highlight the elevation of glutamate between synapses as the causes of mental and emotional disorders. Accordingly, plasma glutamate level of patients is positively correlated with the severity of the disease (24). The inhibition of glutamate receptors became a therapeutic target for the development of novel antidepressant drugs. Interestingly, glutamate supplement exhibited antidepressant effects in some cases (25).
On the other hand, γ-aminobutyric acid (GABA) is synthesized from glutamate. Unlike glutamate, GABA is an inhibitory neurotransmitter. Under physiological conditions, the excitatory glutamate and the inhibitory GABA form a balance in the brains. GABA prevents the neurotoxicity of excess glutamate and termination of stress response (26). Depression patients and animal models suffered from the decreased levels of GABA and GABA-A receptor expression. Brexanolone alleviated postpartum depression by increasing GABA level and motivating the GABA-A receptor, suggesting the GABAergic deficit hypothesis (27, 28). Thus, depression may be caused by different pathological changes while the excitatory-inhibitory imbalance should be the common cause.
2.3 Hormone dysregulation
Hypothalamic-pituitary-adrenal (HPA) axis mainly regulates stress response. Under negative emotions or stress, HPA axis remains active. The hypersecretion of cortisol (corticosterone in rodents) causes neuronal damage and structural disturbances in the hippocampus, resulting in depression symptoms (29). Down-regulation of receptor induces the weakening of negative feedback while aggravates HPA axis excitement, forming a vicious circle. Similarly, the depression process involves other hormone systems, such as hypothalamic-pituitary-gonadal (HPG) axis and hypothalamic–pituitary–thyroid (HPT) axis.
Two third of depression patients are female, largely due to the frequent fluctuation of sex hormones in addition to environmental and genetic factors (3, 30). Both aging men and women are prone to mood disorders with the change of corresponding sex hormone levels, but exhibit different clinical outcomes (31). Females respond to stress in more sensitive manner than males as the sex hormones decline (32). Possibly due to the more influential role of estrogen in mood regulation, women usually become emotionally fragile during the low-estrogen period (33). Estrogen not only modulates cognition and emotion in the brain, but also exhibits neuroprotective effect (33, 34). Surprisingly, males with higher estrogen level tend to suffer from depression (35). Thus, caution is needed to address hormone dysregulation in depression in both sexes.
Stress is known to increase cortisol level and subsequently decrease the release of thyroid stimulating hormone (TSH) (36). Patients with bipolar II depression and anxiety disorder exhibit a lower TSH level and less response to thyrotropin-releasing hormone (TRH), while emotion also influences thyroid hormones (37, 38). People with thyroid disease are commonly associated with mood disorders (39, 40). Hyperthyroidism induces anxiety and irritability, whereas hypothyroidism causes depression. Consequently, thyroid supplementation may be used in the clinical treatment of depression (41).
2.4 Neurogenesis and neuroplasticity hypothesis
Depression is an emotional disease and may show signs at the cell and organ levels. Neuroanatomy studies revealed that hippocampus volume appeared to be reduced in the brains of depression patients (42). Bipolar patients was found to have less gray matter volume (43). Such changes may be caused by the decline of neurotrophic factors, such as brain-derived neurotropic factor (BDNF), nerve growth factor (NGF), and glia-derived neurotropic factor (GDNF) (44).
2.5 Miscellaneous theories
Scientists proposed several other conjectures of depression including inflammation theory, gut microbiota theory, glial pathology theory, epigenetic theory, infection theory, and “dys-stress” theory (45, 46). These theories together provided a comprehensive perspectivity to explain the depression mechanisms.
2.6 Current antidepressants and limitations
FDA-approved antidepressant drugs for adults are divided into seven categories: selective serotonin reuptake inhibitors (SSRIs), serotonin and norepinephrine reuptake inhibitors (SNRIs), tricyclic and tetracyclic antidepressants (TCAs and TeCAs), atypical antidepressants, monoamine oxidase inhibitors (MAOIs), N-methyl D-aspartate (NMDA) antagonist, neuroactive steroid, gamma-aminobutyric acid (GABA)-A receptor positive modulator (20). TCAs and MAOIs belong to the first generation of antidepressants with relatively strong short-term efficacy and low price (47). However, due to the severe side effects, these drugs are not considered the first choice for treating depression. SSRIs and SNRIs are considered as the first-line medications in clinical practice although side effects exist (9).
Most of synthetic antidepressant drugs are known to frequently cause severe side effects and exhibit symptoms including dizziness, nausea, weight change, sexual dysfunction, and apathy (48). The classification and common side effects of antidepressants are shown in the Table 1.
Indeed, the existing antidepressants are limited by different other factors including efficacy, patient compliance, withdrawal reaction and recurrence. As for efficacy, antidepressants often need at least 2 weeks to take effect (49). Many patients may feel the improvement of symptoms after taking medication but are not satisfactory with the overall effect while some patients may be getting even worse (6, 50). As for patient compliance, compliance with antidepressants is extremely poor. Quite a portion of patients are unwilling to follow antidepressant treatment (51). The fear of side effects is a key reason for poor compliance. As for the withdrawal reaction, more than half of the patients experience withdrawal symptoms, including gastrointestinal symptoms, flu-like symptoms, sleep disorders, sensory disorders, movement disorders, and emotional disorders (8). Some patients may have severe symptoms. Finally, the recurrence is also an important problem. Patients may be considered as fully cured by antidepressant treatment but more likely have depression again than normal people (52). Indeed, a quarter of patients relapse depression (53). Therefore, there is a strong need for other complementary or alternative therapies. It is believed that herbal remedies possess better potential than different physiotherapies and psychotherapies.
3 Herbal medicines for the treatment of depression
Herbal ingredients are often used in combination. Presumably, different ingredients may act on several mechanisms in a coordinated manner. For example, hypericin, hyperforin, and eriodictyol may contribute to the antidepressant effects of Hypericum perforatum L. by targeting different mechanisms (54–57). On the other hand, some ingredients may act on more than one target. For example, puerarin not only acts on the 5-HT system and neurotransmitters but also regulates antioxidant and anti-inflammatory pathways, remodels gut microbiota, and modulates the HPA-axis (58–64). In this review, major ingredients and the related antidepressant mechanisms were searched from the recent literatures via PubMed and Google Scholar and summarized in Table 2 and Figure 2. In fact, different active compounds might act on one or several target proteins involved in the regulation of neurotransmitter function, HPA axis, BDNF signaling pathway, anti-inflammatory response, oxidative stress, intestinal microbiota and ferroptosis.
3.1 Regulation of neurotransmitter function
3.1.1 Targeting monoamine neurotransmitters system
Monoamine neurotransmitters include serotonin (5-HT), noradrenaline (NE), and dopamine (DA). 5-HT is an indole neurotransmitter and induces a happy mood in the brain (65). As two types of catecholamines. NE is an excitatory neurotransmitter and alerts people by producing excitement and anger, whereas DA is called the happiness hormone (66). The deficiency of these neurotransmitters results in apathy and the lack of energy. Unlike chemically synthesized antidepressants, herbal medicines may exhibit a broad spectrum of effects on the activity of multiple neurotransmitters. As a key herbal medicine antidepressant, hyperforin derived from St. John’s wort simultaneously inhibits the reabsorption of 5-HT, NE, and DA with similar effectiveness (54). Protopine reduces the reuptake of 5-HT and NE via inhibiting the transporter (67). Apigenin, luteolin, and quercetin from Cayratia japonica inhibit the activities of MAO-A and MAO-B (68). Highly like the current antidepressants, herbal medicines target 5-HT receptors as the main antidepressant mechanism. Puerarin derived from Radix puerariae acts not only as the antagonist for 5-HT2C and 5-HT2A receptors but also as the agonist for 5-HT1A receptor (58, 59).
3.1.2 Targeting GABAergic system
GABA receptors have long been therapeutic targets for anxiety disorders. The current antidepressants improve depression in mice via regulating the GABA system and enhancing the activity of GABAnergic neurons (69). Anxiety and depression often co-exist and influence each other in clinical practice (70). GABA-A receptor positive modulator Zulresso was approved by FDA in 2019 as a treatment for postpartum depression (71). The bark of Magnolia officinalis is well-documented for treating depression in traditional Chinese medicine formulations, while honokiol and magnolol are considered as the active ingredients. Indeed, magnolol treatment reversed the depressive symptoms in rats after chronic unpredictable mild stress (CUMS). Following the treatment, CUMS rats performed equally well in the tests for sucrose preference, locomotor activity, and forced swimming test compared with the rats in the control group, indicating that magnolol may be equally effective as Fluoxetine hydrochloride (72). Honokiol and magnolol positively regulate GABA-A receptors, especially δ-containing receptors (73). It was recently found that GABA-B receptor inhibitors might be potential antidepressant drugs (74). Interestingly, GABA-B1 receptor knockout mice appeared to more anxious than wild breeds. Presumably, GABA-B receptor positive allosteric agents are anxiolytic whereas the antagonists could be antidepressants (75). Nevertheless, both inhibitors and agonists were found to exhibit an antidepressant effect (76).
3.1.3 Targeting L-glutamate signaling pathway
Glutamate receptors include ionotropic and metabotropic forms for rapidly transmitting excitation and widely affecting neural function by coupling with G protein, receptively (77). Depressive symptoms could be relieved by N-methyl-d-aspartate (NMDA) receptor antagonists, group I metabotropic glutamate receptor (mGluR1 and mGluR5) antagonists, and positive modulators of α-amino-3-hydroxy-5-methyl-4-isoxazole propionic acid (AMPA) receptors (78). L-Theanine from Camellia sinensis share similar structure with glutamate and binds to several glutamate receptors, thereby blocking the action of glutamate and reducing glutamate excitotoxicity (79). After treatment for 8 weeks, L-theanine improved depressive symptoms including anxiety, sleep disturbance, and cognitive impairment in MDD patients (80).
3.2 Regulation of HPA-axis
The HPA-axis involves three hormones [i.e., corticotropin-releasing hormone (CRH), adrenocorticotropic hormone (ACTH), and cortisol] and mainly mediates stress in the human body (81). As a stress hormone, cortisol affects the levels of neurotransmitters such as 5-HT. Anti-glucocorticoid therapy benefits the brain’s reward mechanisms and alleviates depression (82). Many herbs, such as Scutellaria baicalensis, Phellodendron phellodendri, and Chuanxiong, are known to induce significant reduction of plasma corticosterone levels in depressed mice (83). Based on radiometric ligand-binding assays, icariin could restore the down-regulation of glucocorticoid receptor in social defeat mouse model of depression (84). Several flavonoids (e.g., hypericin, hyperoside, isoquercitrin, and miquelianin) from St. John’s wort significantly reduced the levels of ACTH and corticosterone in rats, and could achieve better effects than imipramine positive control (85).
3.3 Regulation of BDNF signaling pathway
Brain-derived neurotropic factor is known to regulate the growth and function of neuron cells and thereby plays an important role in the regulation of learning and memory (86). Stress reduces the level of BDNF in the brain, leading to atrophy and cell loss in hippocampus and prefrontal cortex, suggesting the link of BDNF with depression (87). Indeed, most of antidepressant drugs could booster the expression of BDNF (88). Peony glycosides from Radix Paeoniae Alba increased the BDNF mRNA level in the brain and improved depressive-behaviors in CUMS-induced mouse model of depression (89). Traditional Chinese medicine formulation PAPZ of four ingredients (i.e., Radix Ginseng, Radix Angelicae Sinensis, Radix Polygalae, and Semen Ziziphi Spinosae) increased the protein expression of BDNF and alleviated the depressive behavior in corticosterone-challenged mice (90). Esculetin from Cichorium intybus L. activated BDNF/TrkB pathway in LPS-depressed mice by increasing BDNF expression (91). BDNF was also found to enhance the function of 5-HT transporter and reduce the level of 5-HT in the synaptic cleft, indicating a need to investigate the cross-talks between different systems (92).
3.4 Regulation of anti-inflammatory response
Depression patients generally show marked increase in pro-inflammatory cytokines (e.g., CRP, IL-3, IL-6, and IL-12) (93). Indeed, anti-inflammatory drugs like celecoxib could effectively relieve the symptoms of depression (94). Many herbal medicines are well-documented for anti-inflammatory properties and potential in the treatment of depression in the inflammatory model of depression (95). Crocus sativus L. (Saffron) is an important medicinal ingredient and also a common spice in North African, Mediterranean, and Middle Eastern countries. As one of the main components, crocin improved depressive symptoms and reduced the expression of inflammatory cytokines (e.g., IL-1β, IL-18, and TNF-α) in the hippocampus of LPS-depressed mice (96). The cellular experiments found that crocin skewed the polarization of glial BV-2 cells from the inflammatory M1 phenotype to the M2 phenotype by inhibiting the NF-kB and NLRP3 signaling pathway (96). Esculetin as a coumarin compound in various plants exhibited strong anti-inflammatory effect, reduced the levels of IL-1β, IL-6, and TNF-α in serum and hippocampus, and down-regulated the hippocampal expression of iNOS and COX-2 in LPS-depressed mice (91). Moreover, BDNF exhibits anti-inflammatory effect, suggesting that the increase in BDNF level also represents an anti-inflammatory mechanism (97).
3.5 Regulation of oxidative stress
Oxidative stress is implicated in various neurodegenerative diseases including AD and PD (98). Depression patients often suffer from cognitive impairment, likely as the result of oxidative stress (99). The antioxidant system is likely disturbed in people with depression (100). Interestingly, 5-HT deficiency appeared to be associated with altered expression of antioxidant enzymes (101). Many herbal medicines are well-known for antioxidative effects and may relieve depression symptoms through antioxidant activity. Eriodictyol is a bitter-masking flavanone, a flavonoid derived from Eriodictyon californicum. Eriodictyol reduced oxidative damage, prevented cell apoptosis, induced glutathione synthesis, and reduced ROS production in H2O2-treated PC12 cells (57). On the other hand, eriodictyol profoundly ameliorated sucrose preference, reduced immobility time in forced swimming test and feeding latency in novelty-suppressed feeding test in LPS- and CUMS-induced rat model of depression (102). Turmeric is one of the raw materials of curry as a spice, and curcumin in it can restore the effects of oxidative stress and prevent depression caused by CUMS (103, 104). Polyphenols are found in many fruits and vegetables, and it has been suggested that diet therapy may be used to relieve depression (105).
3.6 Modulation of intestinal microbiota
The enteric nervous system (ENS) is known to control gastrointestinal behavior via the actions of neurons and neurotransmitters in a manner independent of central nervous system (CNS) input, thereby also known as the “second brain” (106). Indeed, intestinal flora directly produces neurotransmitters (e.g., serotonin and GABA), and regulates brain functions and emotion through the microbiota–gut–brain (MGB or BGM) axis (107, 108). Gut microbiota in the large intestine synthesize various short-chain fatty acids (SCFAs) as the major metabolites for modulating the levels of neurotransmitters and neurotrophic factors and directly affecting brain functions (109, 110). Probiotics Allobaculum and Bifidobacterium were considerably reduced in the gut of depressed patients (111). Interestingly, traditional Chinese medicine formulation Kaixinsan could increase the relative abundance of Allobaculum and Bifidobacterium in the gut of CUMS mice (112). The concurrent use of antibiotics decreased the antidepressant effect of Kaixinsan, suggesting the link of Allobaculum and Bifidobacterium with depression (112). Moreover, puerarin reversed stress-induced disruption of gut microflora via increasing the level of beneficial bacteria and decreasing the inflammatory bacteria in CUMS mouse (113). Collectively, herbal medicines might exhibit antidepressant activity by affecting gut microbiota.
3.7 Regulation of ferroptosis
Ferroptosis describes iron-mediated oxidative cell death, largely due to the toxicity from dramatical increase in the level of intracellular iron ions (114, 115). Ferroptosis has emerged as a hot target for cancer therapy in the past decade. Lipid peroxidation is hyperactive in the depressed population than in the normal population and tightly associated with ferroptosis, suggesting a new therapeutic target (116). A recent analysis of hippocampal proteomes identified the hyperactivation of ferroptosis pathway in CUMS mice (117). Interestingly, traditional Chinese medicine formulation Xiaoyaosan was shown to substantially reduce the total iron and ferrous content in the hippocampus from CUMS mouse model, possibly by regulating PEBP1-GPX4-mediated ferroptosis (118). Galangin, a polyphenolic compound from Alpinia officinarum, also inhibited ferroptosis in the hippocampus by activating the SLC7A11/GPX4 axis (119). Iron chelators and lipophilic antioxidants were suggested for preventing ferroptosis (120). Considering the number, chemical diversity and potency, herbal products represent a rich source for the discovery of new ferroptosis-targeting antidepressants.
3.8 Pathway enrichment analysis
The potent active compounds were further examined through network pharmacology analysis while the target proteins were accordingly predicted (Figures 3, 4). Specifically, the prediction and screening of potential depression-related targets were performed using Similarity Ensemble Approach (SEA) at https://sea.bkslab.org, the Search Tool for Interactions of Chemicals (STITCH) at http://stitch.embl.de, SwissTargetPrediction at http://www.swisstargetprediction.ch, Therapeutic Target Database (TTD) at http://db.idrblab.net/ttd, Comparative Toxicogenomics Database (CTD) at http://ctdbase.org, PharmGKB at https://www.pharmgkb.org, DisGeNET at https://www.disgenet.org, and GeneCards at https://www.genecards.org. Kyoto Encyclopedia of Genes and Genomes (KEGG) Pathway Enrichment of selected targets were performed using the online bioinformatics tool DAVID at http://david.ncifcrf.gov. Interestingly, the pathway enrichment analysis suggests that active herbal compounds mainly target serotonergic synapse pathway (KEGG: map04726) and dopaminergic synapse pathway (KEGG: map04728) in relation to depression. As shown in Figure 3, eight targets (i.e., APP, CASP3, PRKCA, MAOA, ALOX12, ALOX15, ALOX5, and CYP2C19) were enriched for regulating serotonergic synapse pathway, whereas the most related compounds were curcumin from Curcuma longa L. and baicalein from Scutellaria baicalensis Georgi. As shown in Figure 4, eight targets (i.e., SLC6A3, AKT1, PRKCA, FOS, MAOA, DRD1, DRD2, and DRD5) were enriched for regulating the dopaminergic synapse pathway whereas neferine from Nelumbinis semen was mostly studied.
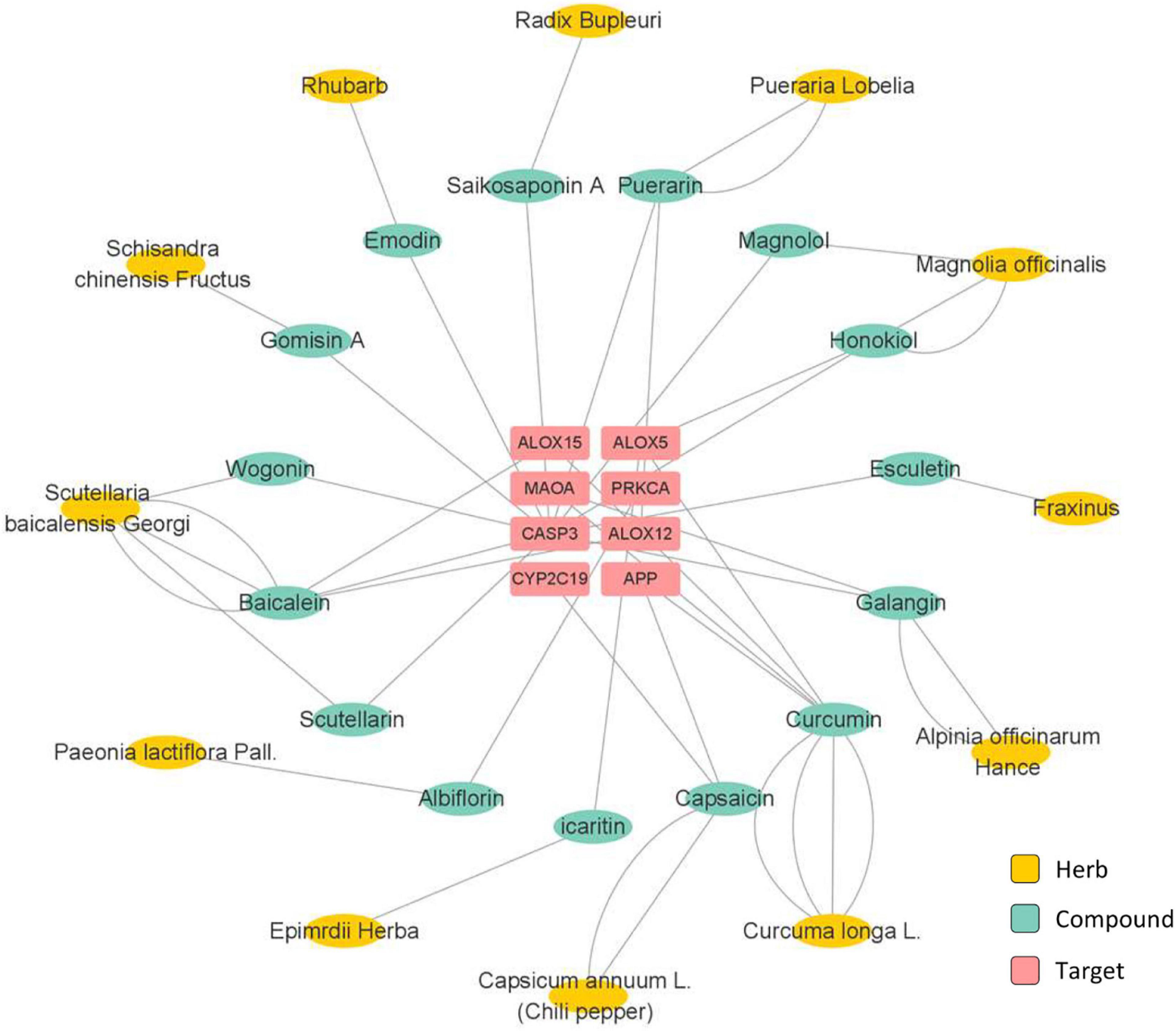
Figure 3. Network pharmacology analysis of herbs and the active compounds for targeting serotonergic synapse pathway.
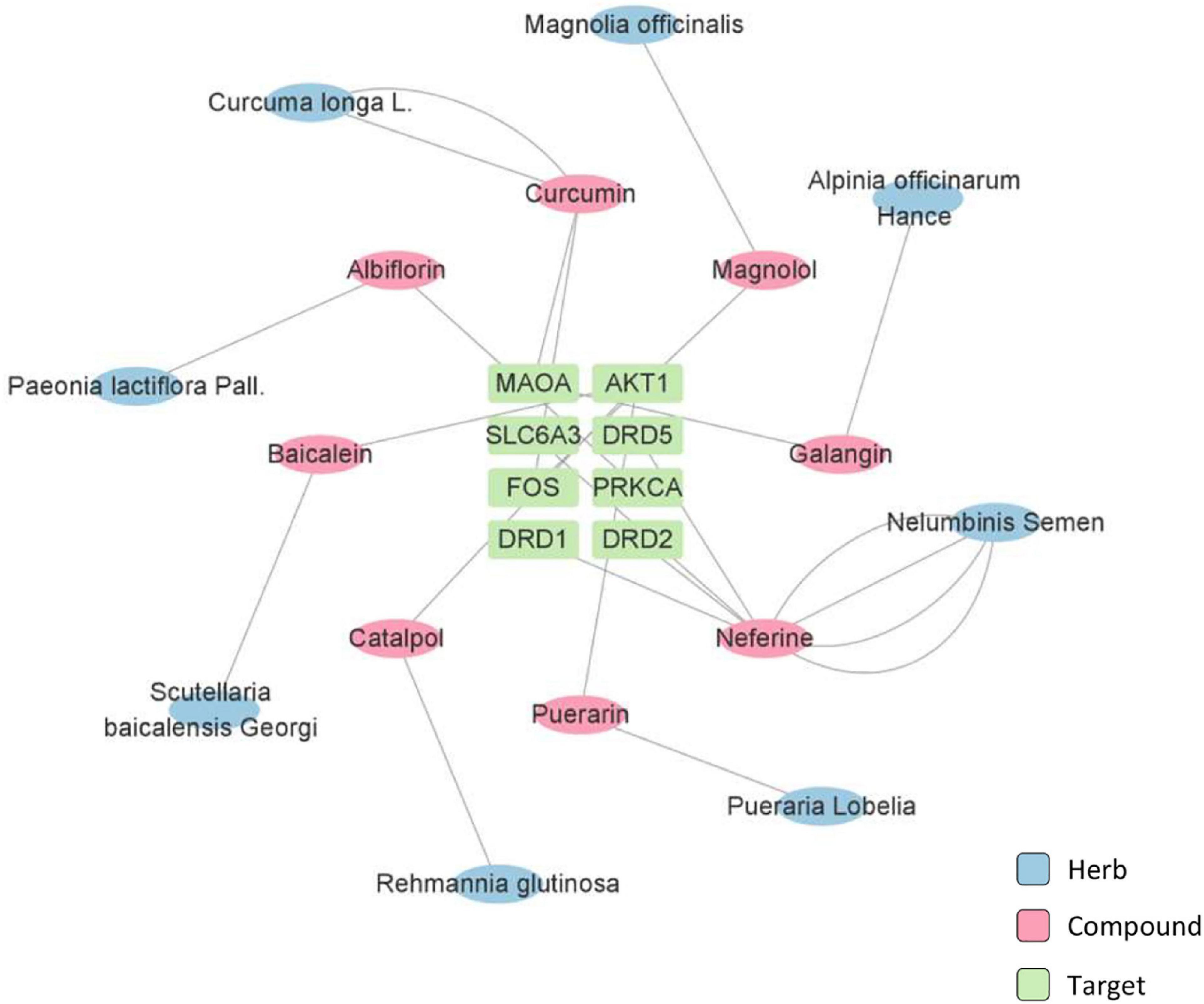
Figure 4. Network pharmacology analysis of herbs and the active compounds for targeting dopaminergic synapse pathway.
4 Conclusion
In this review, we initially discussed the current understanding on the pathology of depression and the molecular targets for different classes of synthetic drugs. Subsequently, we performed comprehensive review and network pharmacology analysis to understand the antidepressant activities of herbal medicines and reveal the underlying mechanisms. Herbal medicines appear to be effective for the treatment of depression without causing undesirable side-effects. As such, the present review may pave a new avenue for the development of novel antidepressant strategies.
Author contributions
YS prepared the original draft. JR and JZ designed, reviewed, and revised the manuscript. JR supervised the work. All authors contributed to the article and approved the submitted version.
Funding
This research and open access publication fees were funded by the Research and Cultivation Plan of High-Level Hospital Construction (HKUSZH201902040), Health and Medical Research Fund (16171751 and 17181231), General Research Fund (17119619), and Science, Technology and Innovation Commission of Shenzhen Municipality (JCYJ20180306173835901).
Conflict of interest
The authors declare that the research was conducted in the absence of any commercial or financial relationships that could be construed as a potential conflict of interest.
Publisher’s note
All claims expressed in this article are solely those of the authors and do not necessarily represent those of their affiliated organizations, or those of the publisher, the editors and the reviewers. Any product that may be evaluated in this article, or claim that may be made by its manufacturer, is not guaranteed or endorsed by the publisher.
References
1. Bauman B, Ko J, Cox S, Mph D, Warner L, Folger S, et al. Vital signs: postpartum depressive symptoms and provider discussions about perinatal depression - United States, 2018. MMWR Morb Mortal Weekly Rep. (2020). 69:575–81. doi: 10.15585/mmwr.mm6919a2
2. World Health Organization [WHO]. Depression. (2021). Available online at: https://www.who.int/en/news-room/fact-sheets/detail/depression (accessed December 22, 2021).
3. Halaris A, Sohl E, Whitham EA. Treatment-resistant depression revisited: a glimmer of hope. J Pers Med. (2021) 11:155. doi: 10.3390/jpm11020155
4. Jennings KD, Ross S, Popper S, Elmore M. Thoughts of harming infants in depressed and nondepressed mothers. J Affect Disord. (1999) 54:21–8. doi: 10.1016/s0165-0327(98)00185-2
5. Amos TB, Tandon N, Lefebvre P, Pilon D, Kamstra RL, Pivneva I, et al. Direct and indirect cost burden and change of employment status in treatment-resistant depression: a matched-cohort study using a US commercial claims database. J Clin Psychiatry. (2018) 79:5360. doi: 10.4088/JCP.17m11725
6. Hillhouse TM, Porter JH. A brief history of the development of antidepressant drugs: from monoamines to glutamate. Exp Clin Psychopharmacol. (2015) 23:1–21.
7. Read J, Gee A, Diggle J, Butler H. The interpersonal adverse effects reported by 1008 users of antidepressants; and the incremental impact of polypharmacy. Psychiatry Res. (2017) 256:423–7. doi: 10.1016/j.psychres.2017.07.003
8. Davies J, Read J. A systematic review into the incidence, severity and duration of antidepressant withdrawal effects: Are guidelines evidence-based? Addict Behav. (2019) 97:111–21.
10. McIntyre E, Saliba AJ, Wiener KK, Sarris J. Herbal medicine use behaviour in Australian adults who experience anxiety: a descriptive study. BMC Complement Altern Med. (2016) 16:60. doi: 10.1186/s12906-016-1022-3
11. McIntyre E, Saliba AJ, Wiener KKK, Sarris J. Prevalence and predictors of herbal medicine use in adults experiencing anxiety: a critical review of the literature. Adv Integr Med. (2015) 2:38–48. doi: 10.1186/s12913-016-1423-5
12. Pan YJ, Cheng IC, Yeh LL, Cho YM, Feng J. Utilization of traditional Chinese medicine in patients treated for depression: a population-based study in Taiwan. Complement Ther Med. (2013) 21:215–23.
13. Ashraf H, Salehi A, Sousani M, Sharifi MH. Use of complementary alternative medicine and the associated factors among patients with depression. Evid Based Complement Alternat Med. (2021) 2021:6626394.
14. Kwon CY, Lee B, Chung SY, Kim JW, Shin A, Choi YY, et al. Efficacy and safety of Sihogayonggolmoryeo-tang (Saikokaryukotsuboreito, Chai-Hu-Jia-Long-Gu-Mu-Li-Tang) for post-stroke depression: a systematic review and meta-analysis. Sci Rep. (2019) 9:14536. doi: 10.1038/s41598-019-51055-6
16. Sarris J, Thomson R, Hargraves F, Eaton M, de Manincor M, Veronese N, et al. Multiple lifestyle factors and depressed mood: a cross-sectional and longitudinal analysis of the UK Biobank (N = 84,860). BMC Med. (2020) 18:354. doi: 10.1186/s12916-020-01813-5
17. Yang L, Zhao Y, Wang Y, Liu L, Zhang X, Li B, et al. The effects of psychological stress on depression. Curr Neuropharmacol. (2015) 13:494–504.
18. Brigitta B. Pathophysiology of depression and mechanisms of treatment. Dialogues Clin Neurosci. (2002) 4:7–20.
19. Delgado PL. Depression: the case for a monoamine deficiency. J Clin Psychiatry. (2000) 61(Suppl 6):7–11.
20. U.S. Food and Drug Administration [U.S. FDA]. Depression Medicines. (2019). Available online at: https://www.fda.gov/consumers/free-publications-women/depression-medicines (accessed November 18, 2019).
22. Kadriu B, Musazzi L, Henter ID, Graves M, Popoli M, Zarate CA Jr. Glutamatergic neurotransmission: pathway to developing novel rapid-acting antidepressant treatments. Int J Neuropsychopharmacol. (2018) 22:119–35.
23. Moriguchi S, Takamiya A, Noda Y, Horita N, Wada M, Tsugawa S, et al. Glutamatergic neurometabolite levels in major depressive disorder: a systematic review and meta-analysis of proton magnetic resonance spectroscopy studies. Mol Psychiatry. (2019) 24:952–64.
24. Losenkov IS, Boiko AS, Levchuk LA, Simutkin GG, Bokhan NA, Ivanova SA. Blood-serum glutamate in patients with depressive disorders as a potential peripheral marker of the prognosis of the effectiveness of therapy. Neurochem J. (2018) 12:366–72.
25. Son H, Baek JH, Go BS, Jung DH, Sontakke SB, Chung HJ, et al. Glutamine has antidepressive effects through increments of glutamate and glutamine levels and glutamatergic activity in the medial prefrontal cortex. Neuropharmacology. (2018) 143:143–52. doi: 10.1016/j.neuropharm.2018.09.040
26. Fogaça MV, Duman RS. Cortical GABAergic dysfunction in stress and depression: new insights for therapeutic interventions. Front Cell Neurosci. (2019) 13:87. doi: 10.3389/fncel.2019.00087
27. Lüscher B, Möhler H. Brexanolone, a neurosteroid antidepressant, vindicates the GABAergic deficit hypothesis of depression and may foster resilience. F1000Research. (2019) 8:F1000FacultyRev–751. doi: 10.12688/f1000research.18758.1
28. Draganov M, Vives-Gilabert Y, de Diego-Adeliño J, Vicent-Gil M, Puigdemont D, Portella MJ. Glutamatergic and GABA-ergic abnormalities in First-episode depression. A 1-year follow-up 1H-MR spectroscopic study. J Affect Disord. (2020) 266:572–7. doi: 10.1016/j.jad.2020.01.138
29. Sroykham W, Wongsawat Y. Effects of brain activity, morning salivary cortisol, and emotion regulation on cognitive impairment in elderly people. Medicine. (2019) 98:e16114. doi: 10.1097/MD.0000000000016114
30. Zhao L, Han G, Zhao Y, Jin Y, Ge T, Yang W, et al. Gender differences in depression: evidence from genetics. Front Genet. (2020) 11:562316. doi: 10.3389/fgene.2020.562316
31. Morsink L, Vogelzangs N, Nicklas B, Beekman A, Satterfield S, Rubin S, et al. Associations between sex steroid hormone levels and depressive symptoms in elderly men and women: results from the Health ABC Study. Psychoneuroendocrinology. (2007) 32:874–83. doi: 10.1016/j.psyneuen.2007.06.009
32. Guo L, Chen Y-X, Hu Y-T, Wu X-Y, He Y, Wu J-L, et al. Sex hormones affect acute and chronic stress responses in sexually dimorphic patterns: consequences for depression models. Psychoneuroendocrinology. (2018) 95:34–42. doi: 10.1016/j.psyneuen.2018.05.016
33. Albert KM, Newhouse PA. Estrogen, stress, and depression: cognitive and biological interactions. Annu Rev Clin Psychol. (2019) 15:399–423.
34. Saldanha CJ. Estrogen as a neuroprotectant in both sexes: stories from the bird brain. Front Neurol. (2020) 11:497. doi: 10.3389/fneur.2020.00497
35. Stanikova D, Luck T, Bae YJ, Thiery J, Ceglarek U, Engel C, et al. Increased estrogen level can be associated with depression in males. Psychoneuroendocrinology. (2018) 87:196–203.
36. Hirtz R, Libuda L, Hinney A, Föcker M, Bühlmeier J, Antel J, et al. Lack of evidence for a relationship between the hypothalamus-pituitary-adrenal and the hypothalamus-pituitary-thyroid axis in adolescent depression. Front Endocrinol. (2021) 12:662243. doi: 10.3389/fendo.2021.662243
37. Fischer S, Ehlert U. Hypothalamic–pituitary–thyroid (HPT) axis functioning in anxiety disorders. A systematic review. Depress Anxiety. (2018) 35:98–110. doi: 10.1002/da.22692
38. Zhong S, Chen G, Zhao L, Jia Y, Chen F, Qi Z, et al. Correlation between intrinsic brain activity and thyroid-stimulating hormone level in unmedicated bipolar II depression. Neuroendocrinology. (2019) 108:232–43. doi: 10.1159/000497182
39. Jabeen R, Ahmed N, Tariq MT, Khan KS, Perveen B, Khan A, et al. Psychosocial mood symptoms; a common presentation in thyroid dysfunction. Int J Curr Med Pharm Res. (2020) 6:4977–80.
40. Kafle B, Khadka B, Tiwari ML. Prevalence of thyroid dysfunction among depression patients in a tertiary care centre. JNMA J Nepal Med Assoc. (2020) 58:654–8. doi: 10.31729/jnma.5296
41. Duval F. Thyroid hormone treatment of mood disorders. Curr Treat Options Psychiatry. (2018) 5:363–76.
42. Boku S, Nakagawa S, Toda H, Hishimoto A. Neural basis of major depressive disorder: beyond monoamine hypothesis. Psychiatry Clin Neurosci. (2018) 72:3–12. doi: 10.1111/pcn.12604
43. Matsuo K, Harada K, Fujita Y, Okamoto Y, Ota M, Narita H, et al. Distinctive neuroanatomical substrates for depression in bipolar disorder versus major depressive disorder. Cereb Cortex. (2018) 29:202–14. doi: 10.1093/cercor/bhx319
44. Bliźniewska-Kowalska K, Gałecki P, Szemraj J, Talarowska M. Expression of selected genes involved in neurogenesis in the etiopathogenesis of depressive disorders. J Personal Med. (2021) 11:168. doi: 10.3390/jpm11030168
45. Grosso C. Herbal Medicine in Depression: Traditional Medicine to Innovative Drug Delivery. Cham: Springer International Publishing AG (2016).
46. Silvestrini B. Trazodone: from the mental pain to the “dys-stress” hypothesis of depression. Clin Neuropharmacol. (1989) 12(Suppl 1):S4–10. doi: 10.1097/00002826-198901001-00002
47. O’Connor E, Rossom RC, Henninger M, Groom HC, Burda BU, Henderson JT, et al. U.S. Preventive Services Task Force Evidence Syntheses, formerly Systematic Evidence Reviews. Screening for Depression in Adults: An Updated Systematic Evidence Review for the US Preventive Services Task Force. Rockville, MD: Agency for Healthcare Research and Quality (US) (2016).
48. Anderson HD, Pace WD, Libby AM, West DR, Valuck RJ. Rates of 5 common antidepressant side effects among new adult and adolescent cases of depression: a retrospective US claims study. Clin Ther. (2012) 34:113–23. doi: 10.1016/j.clinthera.2011.11.024
49. Machado-Vieira R, Baumann J, Wheeler-Castillo C, Latov D, Henter ID, Salvadore G, et al. The timing of antidepressant effects: a comparison of diverse pharmacological and somatic treatments. Pharmaceuticals. (2010) 3:19–41. doi: 10.3390/ph3010019
51. Sansone RA, Sansone LA. Antidepressant adherence: are patients taking their medications? Innov Clin Neurosci. (2012) 9:41–6.
53. Buckman JEJ, Underwood A, Clarke K, Saunders R, Hollon SD, Fearon P, et al. Risk factors for relapse and recurrence of depression in adults and how they operate: a four-phase systematic review and meta-synthesis. Clin Psychol Rev. (2018) 64:13–38. doi: 10.1016/j.cpr.2018.07.005
54. Müller WE. Current St John’s wort research from mode of action to clinical efficacy. Pharmacol Res. (2003) 47:101–9.
55. Zirak N, Shafiee M, Soltani G, Mirzaei M, Sahebkar A. Hypericum perforatum in the treatment of psychiatric and neurodegenerative disorders: current evidence and potential mechanisms of action. J Cell Physiol. (2019) 234:8496–508. doi: 10.1002/jcp.27781
56. Szewczyk B, Pochwat B, Muszyńska B, Opoka W, Krakowska A, Rafało-Ulińska A, et al. Antidepressant-like activity of hyperforin and changes in BDNF and zinc levels in mice exposed to chronic unpredictable mild stress. Behav Brain Res. (2019) 372:112045. doi: 10.1016/j.bbr.2019.112045
57. Lou H, Jing X, Ren D, Wei X, Zhang X. Eriodictyol protects against H(2)O(2)-induced neuron-like PC12 cell death through activation of Nrf2/ARE signaling pathway. Neurochem Int. (2012) 61:251–7. doi: 10.1016/j.neuint.2012.05.013
58. Overstreet DH, Kralic JE, Morrow AL, Ma ZZ, Zhang Y, Lee DY. NPI-031G (puerarin) reduces anxiogenic effects of alcohol withdrawal or benzodiazepine inverse or 5-HT2C agonists. Pharmacol Biochem Behav. (2003) 75:619–25.
59. Chueh F-S, Chang C-P, Chio C-C, Lin M-T. Puerarin acts through brain serotonergic mechanisms to induce thermal effects. J Pharmacol Sci. (2004) 96:420–7. doi: 10.1254/jphs.fp0040424
60. Qiu Z-K, Zhang G-H, Zhong D-S, He J-L, Liu X, Chen J-S, et al. Puerarin ameliorated the behavioral deficits induced by chronic stress in rats. Sci Rep. (2017) 7:1–13. doi: 10.1038/s41598-017-06552-x
61. Zhang C, Hu CX, Li SH, Zhang X, Lu HQ, Lin ZM. Anti-depressant effects of puerarin on depression induced by chronic unpredicted mild stress in rats. Acta Pharm Sin. (2018) 12:220–6.
62. Liu R, Li Y, Wang Z, Li J, Liang Y, Li M. Puerarin mitigates symptoms of depression in ovariectomized female rats by regulating hippocampal cAMP-CREB-BDNF signaling pathway. Trop J Pharmaceut Res. (2021) 20:1403–9.
63. Zhao J, Jia Y, Zhao W, Chen H, Zhang X, Ngo FY, et al. Botanical drug puerarin ameliorates liposaccharide-induced depressive behaviors in mice via inhibiting RagA/mTOR/p70S6K pathways. Oxid Med Cell Longev. (2021) 2021:7716201. doi: 10.1155/2021/7716201
64. Gao L-N, Yan M, Zhou L, Wang J, Sai C, Fu Y, et al. Puerarin alleviates depression-like behavior induced by high-fat diet combined with chronic unpredictable mild stress via repairing TLR4-induced inflammatory damages and phospholipid metabolism disorders. Front Pharmacol. (2021) 12:767333. doi: 10.3389/fphar.2021.767333
65. Endocrine Society. Hormone Health Network.”Serotonin | Hormone Health Network.”. (2022). Available online at: https://www.hormone.org/your-health-and-hormones/glands-and-hormones-a-to-z/hormones/serotonin (accessed January 23, 2022).
66. Wang F, Yang J, Pan F, Ho RC, Huang JH. Editorial: neurotransmitters and emotions. Front Psychol. (2020) 11:21. doi: 10.3389/fpsyg.2020.00021
67. Xu LF, Chu WJ, Qing XY, Li S, Wang XS, Qing GW, et al. Protopine inhibits serotonin transporter and noradrenaline transporter and has the antidepressant-like effect in mice models. Neuropharmacology. (2006) 50:934–40. doi: 10.1016/j.neuropharm.2006.01.003
68. Han XH, Hong SS, Hwang JS, Lee MK, Hwang BY, Ro JS. Monoamine oxidase inhibitory components from Cayratia japonica. Arch Pharm Res. (2007) 30:13–7. doi: 10.1007/BF02977772
69. Fuchs T, Jefferson SJ, Hooper A, Yee PH, Maguire J, Luscher B. Disinhibition of somatostatin-positive GABAergic interneurons results in an anxiolytic and antidepressant-like brain state. Mol Psychiatry. (2017) 22:920–30. doi: 10.1038/mp.2016.188
71. Powell JG, Garland S, Preston K, Piszczatoski C. Brexanolone (Zulresso): finally, an FDA-approved treatment for postpartum depression. Ann Pharmacother. (2020) 54:157–63. doi: 10.1177/1060028019873320
72. Li LF, Yang J, Ma SP, Qu R. Magnolol treatment reversed the glial pathology in an unpredictable chronic mild stress-induced rat model of depression. Eur J Pharmacol. (2013) 711:42–9. doi: 10.1016/j.ejphar.2013.04.008
73. Alexeev M, Grosenbaugh DK, Mott DD, Fisher JL. The natural products magnolol and honokiol are positive allosteric modulators of both synaptic and extra-synaptic GABA(A) receptors. Neuropharmacology. (2012) 62:2507–14. doi: 10.1016/j.neuropharm.2012.03.002
74. Möhler H. The GABA system in anxiety and depression and its therapeutic potential. Neuropharmacology. (2012) 62:42–53.
75. Mombereau C, Kaupmann K, Froestl W, Sansig G, van der Putten H, Cryan JF. Genetic and pharmacological evidence of a role for GABA(B) receptors in the modulation of anxiety- and antidepressant-like behavior. Neuropsychopharmacology. (2004) 29:1050–62. doi: 10.1038/sj.npp.1300413
76. Frankowska M, Filip M, Przegaliński E. Effects of GABAB receptor ligands in animal tests of depression and anxiety. Pharmacol Rep. (2007) 59:645–55.
77. Crupi R, Impellizzeri D, Cuzzocrea S. Role of metabotropic glutamate receptors in neurological disorders. Front Mol Neurosci. (2019) 12:20. doi: 10.3389/fnmol.2019.00020
78. Paul IA, Skolnick P. Glutamate and depression: clinical and preclinical studies. Ann N Y Acad Sci. (2003) 1003:250–72.
79. Adhikary R, Mandal V. l-theanine: a potential multifaceted natural bioactive amide as health supplement. Asian Pac J Trop Biomed. (2017) 7:842–8.
80. Hidese S, Ota M, Wakabayashi C, Noda T, Ozawa H, Okubo T, et al. Effects of chronic l-theanine administration in patients with major depressive disorder: an open-label study. Acta Neuropsychiatr. (2017) 29:72–9. doi: 10.1017/neu.2016.33
81. Gudmand-Hoeyer J, Timmermann S, Ottesen JT. Patient-specific modeling of the neuroendocrine HPA-axis and its relation to depression: ultradian and circadian oscillations. Math Biosci. (2014) 257:23–32. doi: 10.1016/j.mbs.2014.07.013
82. Reus VI, Wolkowitz OM. Antiglucocorticoid drugs in the treatment of depression. Expert Opin Investig Drugs. (2001) 10:1789–96.
83. Pao LH, Lu SW, Sun GG, Chiou SH, Ma KH. Three Chinese herbal medicines promote neuroproliferation in vitro, and reverse the effects of chronic mild stress on behavior, the HPA axis, and proliferation of hippocampal precursor cell in vivo. J Ethnopharmacol. (2012) 144:261–9. doi: 10.1016/j.jep.2012.09.002
84. Wu J, Du J, Xu C, Le J, Xu Y, Liu B, et al. Icariin attenuates social defeat-induced down-regulation of glucocorticoid receptor in mice. Pharmacol Biochem Behav. (2011) 98:273–8. doi: 10.1016/j.pbb.2011.01.008
85. Butterweck V, Hegger M, Winterhoff H. Flavonoids of St. John’s wort reduce HPA axis function in the rat. Planta Med. (2004) 70:1008–11.
86. Miranda M, Morici JF, Zanoni MB, Bekinschtein P. Brain-derived neurotrophic factor: a key molecule for memory in the healthy and the pathological brain. Front Cell Neurosci. (2019) 13:363. doi: 10.3389/fncel.2019.00363
87. Mondal AC, Fatima M. Direct and indirect evidences of BDNF and NGF as key modulators in depression: role of antidepressants treatment. Int J Neurosci. (2019) 129:283–96. doi: 10.1080/00207454.2018.1527328
88. Mosiołek A, Mosiołek J, Jakima S, Piêta A, Szulc A. Effects of antidepressant treatment on neurotrophic factors (BDNF and IGF-1) in patients with major depressive disorder (MDD). J Clin Med. (2021) 10:3377.
89. Mao QQ, Ip SP, Ko KM, Tsai SH, Che CT. Peony glycosides produce antidepressant-like action in mice exposed to chronic unpredictable mild stress: effects on hypothalamic-pituitary-adrenal function and brain-derived neurotrophic factor. Prog Neuropsychopharmacol Biol Psychiatry. (2009) 33:1211–6. doi: 10.1016/j.pnpbp.2009.07.002
90. Chen H, Huang Q, Zhang S, Hu K, Xiong W, Xiao L, et al. The Chinese herbal formula PAPZ ameliorates behavioral abnormalities in depressive mice. Nutrients. (2019) 11:859. doi: 10.3390/nu11040859
91. Zhu L, Nang C, Luo F, Pan H, Zhang K, Liu J, et al. Esculetin attenuates lipopolysaccharide (LPS)-induced neuroinflammatory processes and depressive-like behavior in mice. Physiol Behav. (2016) 163:184–92. doi: 10.1016/j.physbeh.2016.04.051
92. Benmansour S, Deltheil T, Piotrowski J, Nicolas L, Reperant C, Gardier AM, et al. Influence of brain-derived neurotrophic factor (BDNF) on serotonin neurotransmission in the hippocampus of adult rodents. Eur J Pharmacol. (2008) 587:90–8. doi: 10.1016/j.ejphar.2008.03.048
93. Osimo EF, Pillinger T, Rodriguez IM, Khandaker GM, Pariante CM, Howes OD. Inflammatory markers in depression: a meta-analysis of mean differences and variability in 5,166 patients and 5,083 controls. Brain Behav Immunity. (2020) 87:901–9. doi: 10.1016/j.bbi.2020.02.010
94. Köhler O, Benros ME, Nordentoft M, Farkouh ME, Iyengar RL, Mors O, et al. Effect of anti-inflammatory treatment on depression, depressive symptoms, and adverse effects: a systematic review and meta-analysis of randomized clinical trials. JAMA Psychiatry. (2014) 71:1381–91.
95. Li C, Huang B, Zhang Y-W. Chinese herbal medicine for the treatment of depression: effects on the neuroendocrine-immune network. Pharmaceuticals. (2021) 14:65. doi: 10.3390/ph14010065
96. Zhang L, Previn R, Lu L, Liao R-F, Jin Y, Wang R-K. Crocin, a natural product attenuates lipopolysaccharide-induced anxiety and depressive-like behaviors through suppressing NF-kB and NLRP3 signaling pathway. Brain Res Bull. (2018) 142:352–9. doi: 10.1016/j.brainresbull.2018.08.021
97. Tan X, Du X, Jiang Y, Botchway BOA, Hu Z, Fang M. Inhibition of autophagy in microglia alters depressive-like behavior via BDNF pathway in postpartum depression. Front Psychiatry. (2018) 9:434. doi: 10.3389/fpsyt.2018.00434
98. Konovalova J, Gerasymchuk D, Parkkinen I, Chmielarz P, Domanskyi A. Interplay between MicroRNAs and oxidative stress in neurodegenerative diseases. Int J Mol Sci. (2019) 20:6055.
99. Michel TM, Pülschen D, Thome J. The role of oxidative stress in depressive disorders. Curr Pharm Des. (2012) 18:5890–9.
100. Khanzode SD, Dakhale GN, Khanzode SS, Saoji A, Palasodkar R. Oxidative damage and major depression: the potential antioxidant action of selective serotonin re-uptake inhibitors. Redox Rep. (2003) 8:365–70. doi: 10.1179/135100003225003393
101. Fanibunda SE, Deb S, Maniyadath B, Tiwari P, Ghai U, Gupta S, et al. Serotonin regulates mitochondrial biogenesis and function in rodent cortical neurons via the 5-HT(2A) receptor and SIRT1-PGC-1α axis. Proc Natl Acad Sci USA. (2019) 116:11028–37. doi: 10.1073/pnas.1821332116
102. Zhang L, Liu C, Yuan M. Eriodictyol produces antidepressant-like effects and ameliorates cognitive impairments induced by chronic stress. Neuroreport. (2020) 31:1111–20. doi: 10.1097/WNR.0000000000001525
103. Liao D, Lv C, Cao L, Yao D, Wu Y, Long M, et al. Curcumin attenuates chronic unpredictable mild stress-induced depressive-like behaviors via restoring changes in oxidative stress and the activation of Nrf2 signaling pathway in rats. Oxid Med Cell Longev. (2020) 2020:9268083. doi: 10.1155/2020/9268083
104. Naqvi F, Saleem S, Naqvi F, Batool Z, Sadir S, Tabassum S, et al. Curcumin lessens unpredictable chronic mild stress-induced depression and memory deficits by modulating oxidative stress and cholinergic activity. Pak J Pharm Sci. (2019) 32(4Supplementary):1893–900.
105. Bouayed J. Polyphenols: a potential new strategy for the prevention and treatment of anxiety and depression. Curr Nutr Food Sci. (2010) 6:13–8. doi: 10.1080/1028415X.2015.1103461
106. Rao M, Gershon MD. The bowel and beyond: the enteric nervous system in neurological disorders. Nat Rev Gastroenterol Hepatol. (2016) 13:517–28.
107. Mazzoli R, Pessione E. The neuro-endocrinological role of microbial glutamate and GABA signaling. Front Microbiol. (2016) 7:1934. doi: 10.3389/fmicb.2016.01934
108. Yano JM, Yu K, Donaldson GP, Shastri GG, Ann P, Ma L, et al. Indigenous bacteria from the gut microbiota regulate host serotonin biosynthesis. Cell. (2015) 161:264–76.
109. Logsdon AF, Erickson MA, Rhea EM, Salameh TS, Banks WA. Gut reactions: how the blood-brain barrier connects the microbiome and the brain. Exp Biol Med. (2018) 243:159–65.
110. Silva YP, Bernardi A, Frozza RL. The role of short-chain fatty acids from gut microbiota in gut-brain communication. Front Endocrinol. (2020) 11:25. doi: 10.3389/fendo.2020.00025
111. Wong ML, Inserra A, Lewis MD, Mastronardi CA, Leong L, Choo J, et al. Inflammasome signaling affects anxiety- and depressive-like behavior and gut microbiome composition. Mol Psychiatry. (2016) 21:797–805. doi: 10.1038/mp.2016.46
112. Cao C, Liu M, Qu S, Huang R, Qi M, Zhu Z, et al. Chinese medicine formula Kai-Xin-San ameliorates depression-like behaviours in chronic unpredictable mild stressed mice by regulating gut microbiota-inflammation-stress system. J Ethnopharmacol. (2020) 261:113055. doi: 10.1016/j.jep.2020.113055
113. Song X, Wang W, Ding S, Liu X, Wang Y, Ma H. Puerarin ameliorates depression-like behaviors of with chronic unpredictable mild stress mice by remodeling their gut microbiota. J Affect Disord. (2021) 290:353–63. doi: 10.1016/j.jad.2021.04.037
114. Aron AT, Loehr MO, Bogena J, Chang CJ. An endoperoxide reactivity-based FRET probe for ratiometric fluorescence imaging of labile iron pools in living cells. J Am Chem Soc. (2016) 138:14338–46. doi: 10.1021/jacs.6b08016
115. Dixon SJ, Lemberg KM, Lamprecht MR, Skouta R, Zaitsev EM, Gleason CE, et al. Ferroptosis: an iron-dependent form of nonapoptotic cell death. Cell. (2012) 149:1060–72.
116. Yager S, Forlenza MJ, Miller GE. Depression and oxidative damage to lipids. Psychoneuroendocrinology. (2010) 35:1356–62.
117. Cao H, Zuo C, Huang Y, Zhu L, Zhao J, Yang Y, et al. Hippocampal proteomic analysis reveals activation of necroptosis and ferroptosis in a mouse model of chronic unpredictable mild stress-induced depression. Behav Brain Res. (2021) 407:113261. doi: 10.1016/j.bbr.2021.113261
118. Jiao H, Yang H, Yan Z, Chen J, Xu M, Jiang Y, et al. Traditional Chinese formula xiaoyaosan alleviates depressive-like behavior in CUMS mice by regulating PEBP1-GPX4-mediated ferroptosis in the hippocampus. Neuropsychiatr Dis Treat. (2021) 17:1001–19. doi: 10.2147/NDT.S302443
119. Guan X, Li Z, Zhu S, Cheng M, Ju Y, Ren L, et al. Galangin attenuated cerebral ischemia-reperfusion injury by inhibition of ferroptosis through activating the SLC7A11/GPX4 axis in gerbils. Life Sci. (2021) 264:118660. doi: 10.1016/j.lfs.2020.118660
120. Lei P, Bai T, Sun Y. Mechanisms of ferroptosis and relations with regulated cell death: a review. Front Physiol. (2019) 10:139. doi: 10.3389/fphys.2019.00139
121. Lam KY, Wu Q-Y, Hu W-H, Yao P, Wang H-Y, Dong TT, et al. Asarones from Acori Tatarinowii Rhizoma stimulate expression and secretion of neurotrophic factors in cultured astrocytes. Neurosci Lett. (2019) 707:134308. doi: 10.1016/j.neulet.2019.134308
122. Chellian R, Pandy V, Mohamed Z. Biphasic effects of α-asarone on immobility in the tail suspension test: evidence for the involvement of the noradrenergic and serotonergic systems in its antidepressant-like activity. Front Pharmacol. (2016) 7:72. doi: 10.3389/fphar.2016.00072
123. Huang B, Liu H, Wu Y, Li C, Tang Q, Zhang Y-W. Two lignan glycosides from Albizia julibrissin durazz. noncompetitively inhibit serotonin transporter. Pharmaceuticals. (2022) 15:344. doi: 10.3390/ph15030344
124. Liu J, Lv Y-W, Shi J-L, Ma X-J, Chen Y, Zheng Z-Q, et al. Anti-Anxiety Effect of (-)-Syringaresnol-4-O-β-d-apiofuranosyl-(1→ 2)-β-d-glucopyranoside from Albizzia julibrissin Durazz (Leguminosae). Molecules. (2017) 22:1331. doi: 10.3390/molecules22081331
125. Salehi A, Rabiei Z, Setorki M. Antidepressant effects of hydroalcoholic extract of Alpinia officinarum rhizome on chronic unpredictable stress induced depression in BALB/c mice. J Med Plants. (2020) 1:170–9.
126. Chaurasiya ND, Ibrahim MA, Muhammad I, Walker LA, Tekwani BL. Monoamine oxidase inhibitory constituents of propolis: kinetics and mechanism of inhibition of recombinant human MAO-A and MAO-B. Molecules. (2014) 19:18936–52. doi: 10.3390/molecules191118936
127. Gong W, Zhu S, Chen C, Yin Q, Li X, Du G, et al. The anti-depression effect of angelicae sinensis radix is related to the pharmacological activity of modulating the hematological anomalies. Front Pharmacol. (2019) 10:192. doi: 10.3389/fphar.2019.00192
128. Shen J, Zhang J, Deng M, Liu Y, Hu Y, Zhang L. The antidepressant effect of Angelica sinensis extracts on chronic unpredictable mild stress-induced depression is mediated via the upregulation of the BDNF signaling pathway in rats. Evid Based Complement Alternat Med. (2016) 2016:7434692. doi: 10.1155/2016/7434692
129. Ma J-C, Zhang H-L, Huang H-P, Ma Z-L, Chen S-F, Qiu Z-K, et al. Antidepressant-like effects of Z-ligustilide on chronic unpredictable mild stress-induced depression in rats. Exp Therapeut Med. (2021) 22:1–7. doi: 10.3892/etm.2021.10109
130. Li X, Wu T, Yu Z, Li T, Zhang J, Zhang Z, et al. Apocynum venetum leaf extract reverses depressive-like behaviors in chronically stressed rats by inhibiting oxidative stress and apoptosis. Biomed Pharmacother. (2018) 100:394–406. doi: 10.1016/j.biopha.2018.01.137
131. Song M-T, Ruan J, Zhang R-Y, Deng J, Ma Z-Q, Ma S-P. Astragaloside IV ameliorates neuroinflammation-induced depressive-like behaviors in mice via the PPARγ/NF-κB/NLRP3 inflammasome axis. Acta Pharmacol Sin. (2018) 39:1559–70.
132. Zhou Y, Huang S, Wu F, Zheng Q, Zhang F, Luo Y, et al. Atractylenolide III reduces depressive-and anxiogenic-like behaviors in rat depression models. Neurosc Lett. (2021) 759:136050. doi: 10.1016/j.neulet.2021.136050
133. Xia J, Gu L, Guo Y, Feng H, Chen S, Jurat J, et al. Gut microbiota mediates the preventive effects of dietary capsaicin against depression-like behavior induced by lipopolysaccharide in mice. Front Cell Infect Microbiol. (2021) 11:627608. doi: 10.3389/fcimb.2021.627608
134. Jagadeesan S, Chiroma SM, Baharuldin MTH, Taib CNM, Amom Z, Adenan MI, et al. Centella asiatica prevents chronic unpredictable mild stress-induced behavioral changes in rats. Biomed Res Therapy. (2019) 6:3233–43.
135. Jeong HJ, Yang SY, Kim HY, Kim NR, Jang JB, Kim HM. Chelidonic acid evokes antidepressant-like effect through the up-regulation of BDNF in forced swimming test. Exp Biol Med. (2016) 241:1559–67. doi: 10.1177/1535370216642044
136. Rajabi M, Mohaddes G, Farajdokht F, Nayebi Rad S, Mesgari M, Babri S. Impact of loganin on pro-inflammatory cytokines and depression-and anxiety-like behaviors in male diabetic rats. Physiol Int. (2018) 105:199–209.
137. Ji L-I, Wang X, Li J-J, Zhong X-J, Zhang B, Juan J, et al. New iridoid derivatives from the fruits of Cornus officinalis and their neuroprotective activities. Molecules. (2019) 24(3):625. doi: 10.3390/molecules24030625
138. Tian W, Zhao J, Lee J-H, Akanda MR, Cho J-H, Kim S-K, et al. Neuroprotective effects of Cornus officinalis on stress-induced hippocampal deficits in rats and H2O2-induced neurotoxicity in SH-SY5Y neuroblastoma cells. Antioxidants. (2019) 9:27. doi: 10.3390/antiox9010027
139. Lu L, Wu D, Wang K, Tang J, Chen G. Beneficial effects of crocin against depression via pituitary adenylate cyclase-activating polypeptide. Biomed Res Int. (2020) 2020:3903125. doi: 10.1155/2020/3903125
140. Xiao Q, Shu R, Wu C, Tong Y, Xiong Z, Zhou J, et al. Crocin-I alleviates the depression-like behaviors probably via modulating “microbiota-gut-brain” axis in mice exposed to chronic restraint stress. J Affect Disord. (2020) 276:476–86. doi: 10.1016/j.jad.2020.07.041
141. Xiao Q, Xiong Z, Yu C, Zhou J, Shen Q, Wang L, et al. Antidepressant activity of crocin-I is associated with amelioration of neuroinflammation and attenuates oxidative damage induced by corticosterone in mice. Physiol Behav. (2019) 212:112699. doi: 10.1016/j.physbeh.2019.112699
142. Wu Y, Sun F, Guo Y, Zhang Y, Li L, Dang R, et al. Curcumin relieves chronic unpredictable mild stress-induced depression-like behavior through the PGC-1α/FNDC5/BDNF Pathway. Behav Neurol. (2021) 2021:2630445. doi: 10.1155/2021/2630445
143. Xia B, Tong Y, Xia C, Chen C, Shan X. α-cyperone confers antidepressant-like effects in mice via neuroplasticity enhancement by SIRT3/ROS mediated NLRP3 inflammasome deactivation. Front Pharmacol. (2020) 11:577062. doi: 10.3389/fphar.2020.577062
144. Liu L, Zhao Z, Lu L, Liu J, Sun J, Dong J. Icariin and icaritin ameliorated hippocampus neuroinflammation via mediating HMGB1 expression in social defeat model in mice. Int Immunopharmacol. (2019) 75:105799. doi: 10.1016/j.intimp.2019.105799
145. Kim YR, Park BK, Seo CS, Kim NS, Lee MY. Antidepressant and anxiolytic-like effects of the stem bark extract of fraxinus rhynchophylla hance and its components in a mouse model of depressive-like disorder induced by reserpine administration. Front Behav Neurosci. (2021) 15:650833. doi: 10.3389/fnbeh.2021.650833
146. Xu X, Piao HN, Aosai F, Zeng XY, Cheng JH, Cui YX, et al. Arctigenin protects against depression by inhibiting microglial activation and neuroinflammation via HMGB1/TLR4/NF−κB and TNF−α/TNFR1/NF−κB pathways. Br J Pharmacol. (2020) 177:5224–45.
147. Xu X, Zeng X-Y, Cui Y-X, Li Y-B, Cheng J-H, Zhao X-D, et al. Antidepressive effect of arctiin by attenuating neuroinflammation via HMGB1/TLR4-and TNF-α/TNFR1-mediated NF-κB activation. ACS Chem Neurosci. (2020) 11:2214–30. doi: 10.1021/acschemneuro.0c00120
148. Hamdan DI, Hafez SS, Hassan WH, Morsi MM, Khalil HM, Ahmed YH, et al. Chemical profiles with cardioprotective and anti-depressive effects of Morus macroura Miq. leaves and stem branches dichloromethane fractions on isoprenaline induced post-MI depression. RSC Adv. (2022) 12:3476–93. doi: 10.1039/d1ra08320a
149. Li H, Xiao Y, Han L, Jia Y, Luo S, Zhang D, et al. Ganoderma lucidum polysaccharides ameliorated depression-like behaviors in the chronic social defeat stress depression model via modulation of Dectin-1 and the innate immune system. Brain Res Bull. (2021) 171:16–24. doi: 10.1016/j.brainresbull.2021.03.002
150. Zhang L, Zhang L, Sui R. Ganoderic acid A-mediated modulation of microglial polarization is involved in depressive-like behaviors and neuroinflammation in a rat model of post-stroke depression. Neuropsychiatr Dis Treat. (2021) 17:2671. doi: 10.2147/NDT.S317207
151. Pawar AT, Gaikwad GD, Kuchekar BS. Antidepressant effect of Hedyotis corymbosa extract in olfactory bulbectomy rats. Pharm Res. (2018) 10:213–7.
152. Chiu CH, Chyau CC, Chen CC, Lee LY, Chen WP, Liu JL, et al. Erinacine A-enriched Hericium erinaceus mycelium produces antidepressant-like effects through modulating BDNF/PI3K/Akt/GSK-3β signaling in Mice. Int J Mol Sci. (2018) 19:341. doi: 10.3390/ijms19020341
153. Lew SY, Lim SH, Lim LW, Wong KH. Neuroprotective effects of Hericium erinaceus (Bull.: Fr.) Pers. against high-dose corticosterone-induced oxidative stress in PC-12 cells. BMC Complement Med Ther. (2020) 20:340. doi: 10.1186/s12906-020-03132-x
154. Zhai X, Chen Y, Han X, Zhu Y, Li X, Zhang Y, et al. The protective effect of hypericin on postpartum depression rat model by inhibiting the NLRP3 inflammasome activation and regulating glucocorticoid metabolism. Int Immunopharmacol. (2022) 105:108560. doi: 10.1016/j.intimp.2022.108560
155. Sánchez-Vidaña DI, Po KK-T, Fung TK-H, Chow JK-W, Lau WK-W, So P-K, et al. Lavender essential oil ameliorates depression-like behavior and increases neurogenesis and dendritic complexity in rats. Neurosci Lett. (2019) 701:180–92. doi: 10.1016/j.neulet.2019.02.042
156. Jia M, Li C, Zheng Y, Ding X, Chen M, Ding J, et al. Leonurine exerts antidepressant-like effects in the chronic mild stress-induced depression model in mice by inhibiting neuroinflammation. Int J Neuropsychopharmacol. (2017) 20:886–95. doi: 10.1093/ijnp/pyx062
157. Meng P, Zhu Q, Yang H, Liu D, Lin X, Liu J, et al. Leonurine promotes neurite outgrowth and neurotrophic activity by modulating the GR/SGK1 signaling pathway in cultured PC12 cells. Neuroreport. (2019) 30:247. doi: 10.1097/WNR.0000000000001180
158. Fan X-X, Sun W-Y, Li Y, Tang Q, Li L-N, Yu X, et al. Honokiol improves depression-like behaviors in rats by HIF-1α-VEGF signaling pathway activation. Front Pharmacol. (2022) 13:968124. doi: 10.3389/fphar.2022.968124
159. Tao W, Hu Y, Chen Z, Dai Y, Hu Y, Qi M. Magnolol attenuates depressive-like behaviors by polarizing microglia towards the M2 phenotype through the regulation of Nrf2/HO-1/NLRP3 signaling pathway. Phytomedicine. (2021) 91:153692. doi: 10.1016/j.phymed.2021.153692
160. Chi L, Khan I, Lin Z, Zhang J, Lee MYS, Leong W, et al. Fructo-oligosaccharides from Morinda officinalis remodeled gut microbiota and alleviated depression features in a stress rat model. Phytomedicine. (2020) 67:153157. doi: 10.1016/j.phymed.2019.153157
161. Ekeanyanwu RC, Nkwocha CC, Ekeanyanwu CL. Behavioural and biochemical indications of the antidepressant activities of essential oils from Monodora myristica (Gaertn) seed and Xylopia aethiopica (Dunal) fruit in rats. IBRO Neurosci Rep. (2021) 10:66–74. doi: 10.1016/j.ibneur.2021.01.001
162. Dong Z, Xie Q, Xu F, Shen X, Hao Y, Li J, et al. Neferine alleviates chronic stress-induced depression by regulating monoamine neurotransmitter secretion and gut microbiota structure. Front Pharmacol. (2022) 13:974949. doi: 10.3389/fphar.2022.974949
163. Zhao ZX, Fu J, Ma SR, Peng R, Yu JB, Cong L, et al. Gut-brain axis metabolic pathway regulates antidepressant efficacy of albiflorin. Theranostics. (2018) 8:5945–59. doi: 10.7150/thno.28068
164. Tang M, Chen M, Li Q. Paeoniflorin ameliorates chronic stress-induced depression-like behavior in mice model by affecting ERK1/2 pathway. Bioengineered. (2021) 12:11329–41. doi: 10.1080/21655979.2021.2003676
165. Li J, Gao W, Zhao Z, Li Y, Yang L, Wei W, et al. Ginsenoside Rg1 reduced microglial activation and mitochondrial dysfunction to alleviate depression-like behaviour via the GAS5/EZH2/SOCS3/NRF2 Axis. Mol Neurobiol. (2022) 59:2855–73. doi: 10.1007/s12035-022-02740-7
166. Jiang N, Huang H, Zhang Y, Lv J, Wang Q, He Q, et al. Ginsenoside Rb1 produces antidepressant-like effects in a chronic social defeat stress model of depression through the BDNF-Trkb signaling pathway. Front Pharmacol. (2021) 12:680903. doi: 10.3389/fphar.2021.680903
167. Li Y, Yang X, Chen S, Wu L, Zhou J, Jia K, et al. Integrated network pharmacology and GC-MS-based metabolomics to investigate the effect of xiang-su volatile oil against menopausal depression. Front Pharmacol. (2021) 12:765638. doi: 10.3389/fphar.2021.765638
168. Wang C, Lin H, Yang N, Wang H, Zhao Y, Li P, et al. Effects of platycodins folium on depression in mice based on a UPLC-Q/TOF-MS serum assay and hippocampus metabolomics. Molecules. (2019) 24:1712. doi: 10.3390/molecules24091712
169. Shen F, Song Z, Xie P, Li L, Wang B, Peng D, et al. Polygonatum sibiricum polysaccharide prevents depression-like behaviors by reducing oxidative stress, inflammation, and cellular and synaptic damage. J Ethnopharmacol. (2021) 275:114164. doi: 10.1016/j.jep.2021.114164
170. Chen X-Q, Chen S-J, Liang W-N, Wang M, Li C-F, Wang S-S, et al. Saikosaponin A attenuates perimenopausal depression-like symptoms by chronic unpredictable mild stress. Neurosci Lett. (2018) 662:283–9. doi: 10.1016/j.neulet.2017.09.046
171. Xu L, Su J, Guo L, Wang S, Deng X, Ma S. Modulation of LPA1 receptor-mediated neuronal apoptosis by Saikosaponin-d: a target involved in depression. Neuropharmacology. (2019) 155:150–61. doi: 10.1016/j.neuropharm.2019.05.027
172. Wu X, Wang J, Song L, Guan Y, Cao C, Cui Y, et al. Catalpol weakens depressive-like behavior in mice with streptozotocin-induced hyperglycemia via PI3K/AKT/Nrf2/HO-1 signaling pathway. Neuroscience. (2021) 473:102–18. doi: 10.1016/j.neuroscience.2021.07.029
173. Zhang T, Yang C, Chu J, Ning L-N, Zeng P, Wang X-M, et al. Emodin prevented depression in chronic unpredicted mild stress-exposed rats by targeting miR-139-5p/5-lipoxygenase. Front Cell Dev Biol. (2021) 9:696619. doi: 10.3389/fcell.2021.696619
174. Wang K, Zhai Q, Wang S, Li Q, Liu J, Meng F, et al. Cryptotanshinone ameliorates CUS-induced depressive-like behaviors in mice. Transl Neurosci. (2021) 12:469–81. doi: 10.1515/tnsci-2020-0198
175. Asmita O, Meenu J, Kumar SS. Neuroprotective activity of santalum album seeds in sepsis encephalopathy. Adv Pharm Res. (2019) 2019:9636944.
176. Wang M, Liu J, Sun W, Li N, Yu Z, Zhu K, et al. Schisantherin A exerts sedative and hypnotic effects through regulating GABA and its receptor in mice and rats. Nat Product Commun. (2019) 14:1934578X19858165.
177. Xu M, Xiao F, Wang M, Yan T, Yang H, Wu B, et al. Schisantherin B improves the pathological manifestations of mice caused by behavior desperation in different ages-depression with cognitive impairment. Biomol Therapeut. (2019) 27:160. doi: 10.4062/biomolther.2018.074
178. Wang X, Hu D, Zhang L, Lian G, Zhao S, Wang C, et al. Gomisin A inhibits lipopolysaccharide-induced inflammatory responses in N9 microglia via blocking the NF-κB/MAPKs pathway. Food Chem Toxicol. (2014) 63:119–27. doi: 10.1016/j.fct.2013.10.048
179. Araki R, Hiraki Y, Nishida S, Inatomi Y, Yabe T. Gomisin N ameliorates lipopolysaccharide-induced depressive-like behaviors by attenuating inflammation in the hypothalamic paraventricular nucleus and central nucleus of the amygdala in mice. J Pharmacol Sci. (2016) 132:138–44. doi: 10.1016/j.jphs.2016.09.004
180. Zhang R, Guo L, Ji Z, Li X, Zhang C, Ma Z, et al. Radix scutellariae attenuates CUMS-induced depressive-like behavior by promoting neurogenesis via cAMP/PKA Pathway. Neurochem Res. (2018) 43:2111–20. doi: 10.1007/s11064-018-2635-3
181. Lee HW, Ryu HW, Kang MG, Park D, Lee H, Shin HM, et al. Potent inhibition of monoamine oxidase A by decursin from Angelica gigas Nakai and by wogonin from Scutellaria baicalensis Georgi. Int J Biol Macromol. (2017) 97:598–605. doi: 10.1016/j.ijbiomac.2017.01.080
182. Xiao Z, Cao Z, Yang J, Jia Z, Du Y, Sun G, et al. Baicalin promotes hippocampal neurogenesis via the Wnt/β-catenin pathway in a chronic unpredictable mild stress-induced mouse model of depression. Biochem Pharmacol. (2021) 190:114594. doi: 10.1016/j.bcp.2021.114594
183. Lu L, Yang LK, Yue J, Wang XS, Qi JY, Yang F, et al. Scutellarin alleviates depression-like behaviors induced by LPS in mice partially through inhibition of astrocyte-mediated neuroinflammation. Neurosci Lett. (2021) 765:136284. doi: 10.1016/j.neulet.2021.136284
184. Lee B, Choi GM, Sur B. Silibinin prevents depression-like behaviors in a single prolonged stress rat model: the possible role of serotonin. BMC Complement Med Ther. (2020) 20:70. doi: 10.1186/s12906-020-2868-y
Keywords: depression, molecular mechanisms, herbal medicines, active constituents, antidepressant
Citation: Sun Y, Zhao J and Rong J (2022) Dissecting the molecular mechanisms underlying the antidepressant activities of herbal medicines through the comprehensive review of the recent literatures. Front. Psychiatry 13:1054726. doi: 10.3389/fpsyt.2022.1054726
Received: 27 September 2022; Accepted: 07 December 2022;
Published: 22 December 2022.
Edited by:
Xinjing Yang, South China Hospital of Shenzhen University, ChinaReviewed by:
Magdalena Sowa-Kucma, University of Rzeszów, PolandYue Li, Tianjin University of Traditional Chinese Medicine, China
Copyright © 2022 Sun, Zhao and Rong. This is an open-access article distributed under the terms of the Creative Commons Attribution License (CC BY). The use, distribution or reproduction in other forums is permitted, provided the original author(s) and the copyright owner(s) are credited and that the original publication in this journal is cited, in accordance with accepted academic practice. No use, distribution or reproduction is permitted which does not comply with these terms.
*Correspondence: Jia Zhao, ✉ emhhb2ppYTdAaGt1Lmhr; Jianhui Rong, ✉ anJvbmdAaGt1Lmhr