- 1CNS Pharmacology and Drug Discovery, Idorsia Pharmaceuticals Ltd., Allschwil, Switzerland
- 2Clinical Science, Global Clinical Development, Idorsia Pharmaceuticals Ltd., Allschwil, Switzerland
Neuropsychiatric symptoms (NPS) affect people with dementia (PwD) almost universally across all stages of the disease, and regardless of its exact etiology. NPS lead to disability and reduced quality of life of PwD and their caregivers. NPS include hyperactivity (agitation and irritability), affective problems (anxiety and depression), psychosis (delusions and hallucinations), apathy, and sleep disturbances. Preclinical studies have shown that the orexin neuropeptide system modulates arousal and a wide range of behaviors via a network of axons projecting from the hypothalamus throughout almost the entire brain to multiple, even distant, regions. Orexin neurons integrate different types of incoming information (e.g., metabolic, circadian, sensory, emotional) and convert them into the required behavioral output coupled to the necessary arousal status. Here we present an overview of the behavioral domains influenced by the orexin system that may be relevant for the expression of some critical NPS in PwD. We also hypothesize on the potential effects of pharmacological interference with the orexin system in the context of NPS in PwD.
Introduction
Dementia is characterized by a progressive decline in cognitive function and is a major cause of disability in the elderly. There are multiple underlying pathologic processes in dementia, including neurodegenerative and vascular diseases. Dementia affects different cognitive domains including memory, language, attention, and executive functions (1). Although cognitive impairment is the hallmark of dementia, behavioral problems, or neuropsychiatric symptoms (NPS), are commonly found across the entire severity spectrum of dementia. They include hyperactivity (agitation, irritability, disinhibition, aberrant motor behavior), psychosis (delusions and hallucinations), affective symptoms (depression and anxiety), apathy, euphoria, sleep disturbances and eating abnormalities. NPS are associated with accelerated disease progression, early institutionalization, and increased mortality. They reduce the quality of life of both PwD and their caregivers (2).
Treatment options for NPS consist of pharmacological and non-pharmacological interventions, with the latter being regarded as first-line option. There is a general shortage of approved drugs for the treatment of NPS in dementia (3), with so far only pimavanserin being approved for the treatment of psychosis in Parkinson Disease (4), and risperidone for the short-term treatment of aggression in Alzheimer Disease (in Canada and Europe) (2). The unmet need is huge, as shown by the number of drugs in clinical development for NPS in dementia (3). In clinical practice, the use of non-pharmacological interventions is restricted by the limited knowledge about their effectiveness and by the lack of specialized training for caregivers (5, 6). Due to these limitations and because severe NPS require quick resolution, NPS are often managed with off-label prescription of drugs (mostly antipsychotics, antidepressants, or anxiolytics) (6). This occurs despite the worrying adverse effects associated with most of these drugs (5).
The orexin system modulates a wide range of behaviors by integrating different types of information (e.g., metabolic, circadian, sensory, emotional) received from afferent neurons and by transforming these inputs into respective output signals distributed via a network of efferent axons projecting throughout the neuraxis. The orexin system comprises orexin (OX)-producing neurons that are located in a specific area of the dorso-lateral hypothalamus. OX neurons synthetize the peptide prepro-orexin, which is then cleaved to produce the two orexin peptides OX-A and OX-B (also named hypocretin-1 and hypocretin-2) (7). Axons of OX neurons project to a wide range of brain regions where OX-A and OX-B are then released (8) and bind to OX1 and OX2 receptors (OX1R, OX2R) to affect neuronal signaling (7). As such, the OX system modulates several homeostatic functions and behaviors.
Orexins were initially described to regulate feeding (9), but genetic and pharmacological studies later revealed that their primary role rather resided in the regulation of arousal and the sleep-wake cycle (10, 11). Ever since, understanding of the OX system's contribution to behavior, including many emotional and cognitive domains, has been expanding (8). The relative contribution of either OX1R or OX2R to the different functions of orexins is not fully elucidated, but pharmacological studies showed a more important role of OX2R signaling in wake promotion relative to OX1R (12). Drugs antagonizing orexin receptors have recently been developed and tested in clinical trials for various neuropsychiatric conditions. Dual orexin receptor antagonists (DORAs) are already approved for the treatment of insomnia (13).
We present here our perspective on which behavioral domains that are influenced by the OX system may be relevant for the expression of NPS in PwD. In this context we hypothesize that pharmacological modulation of orexin receptor signaling may ameliorate or aggravate selected NPS in PwD.
Behaviors that are modulated by the orexin system
Arousal and wakefulness
The most well-characterized function of the OX system is the regulation of sleep-wake transitions (14). Preclinical studies showed that experimental activation of OX neurons promotes and stabilizes wakefulness while their inhibition promotes non-rapid eye movement (non-REM) sleep (15–18) and that progressive loss of OX neurons in mouse models leads to fragmented wake (19). OX peptides exert their wake-stabilizing effects by interacting with the brain arousal network, which includes histaminergic, noradrenergic, and cholinergic neurons (14). Additionally, OX neurons support the wake state through promotion of muscle and motor tone (14, 20). Interestingly, the SAMP8 mouse model of accelerated aging show less non-REM and REM sleep and higher locomotor activity during the inactive phase of the light-dark cycle, which co-occur with higher cerebrospinal fluid (CSF) OX levels (21).
Motor behavior
The orexin system contributes to the hypothalamic control of movement: brain injection of OX-A induces hyperlocomotion, stereotypies and grooming behavior in rats (22, 23); conversely, relative to wild-type mice, orexin-ablated mice show reduced exploratory activity under normal feeding conditions (24, 25). In addition, recent studies have shown that orexin cells are active during motion initiation (26). Their activation stimulates GAD65-expressing neurons in the lateral hypothalamus, which are then responsible for promoting normal locomotion and whose hyperactivity leads to hyperlocomotion (27).
Aggressive behavior
Reactive (defensive) aggression in rodents is largely controlled by hypothalamic circuitry (28). Activity of the ventrolateral portion of the ventromedial hypothalamus (VMHvl) is particularly associated with an aggression-related arousal state and execution of aggressive actions (29). OX neurons receive projections from the VMH via the dorsomedial hypothalamus (DMH) (30) and recruit GAD2-expressing neurons in the lateral habenula, whose activation promotes reactive aggressive behavior; this suggests that OX cells integrate information regarding aggression-related arousal (OX neurons become activated following the expression of aggressive behavior, see Figure 1A.1, A.2) and influence the activity of areas involved in the expression of reactive aggression. Drugs that antagonize both OX1/2Rs, or selectively OX2Rs, reduce reactive aggression in rodents (31–33) (Figure 1B). As mentioned above, SAMP8 mice have higher inactive phase CSF OX levels (21), and this strain shows higher reactive aggression relative to control animals (34, 35).
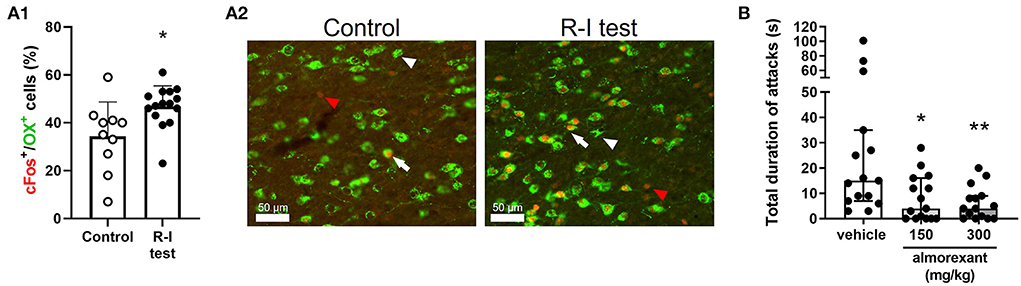
Figure 1. Implication of the OX system in reactive aggression in mice. (A.1,A.2) CD-1 mice were exposed to the resident-intruder (R-I) test or to control condition (i.e., staying in the home-cage, without any manipulation). The effect of aggressive behavior on activation of OX neurons was assessed using immunostaining. (A.1) Percentage of OX neurons expressing the immediate early gene and cellular activation marker c-Fos over the total number of OX neurons. Data are presented individually (scatter) and as mean (bars) + standard deviation (SD); *p < 0.05 vs. control, Student's t-test. (A.2) Representative image of OX+ cells (green) and c-Fos+ cells (red) in the lateral hypothalamus. Scale bar = 50 μm; white arrowhead: OX+ cell; red arrowhead: c-Fos+ cell; white arrow: OX+/c-Fos+ cell. (B) CD-1 mice were exposed to the R-I test after having been treated with vehicle or the dual orexin receptor antagonist almorexant, using a cross-over design. Data are presented individually (scatter) and as median with interquartile range. *p < 0.05, **p < 0.01 vs. vehicle, Dunn's multiple comparisons test (following repeated measure Friedman test). The methods used in these experiments are described in the supplementary information.
Stress-reactivity and anxiety
The physiological and behavioral response to stressful environmental events allows animals to cope with situations relevant for survival. The hypothalamus is an integral part of the neurocircuitry coordinating the body's response to stress, and the contribution of the OX system has been largely investigated. Brain injection of OX peptides increases behavioral (e.g., grooming) and physiological (e.g., plasma levels of corticosterone) correlates of stress exposure (36, 37) and make mice agitated and hyper-responsive to sensory stimulation (38). Acute stressors recruit OX neurons (39, 40), and DORAs reduce stress-induced behavioral changes (41). In addition, brain infusion of OX peptides produces anxiogenic-like effects (42, 43), while OXR antagonists attenuate the expression of anxiety-like behaviors (44).
Reward processing
Rodent studies indicate that the orexin system plays a role in the regulation of reward processing (7, 45, 46). OX neurons project to and modulate the activity of brain areas involved in reward-related behaviors including those of the mesocorticolimbic pathway and the brain's “hedonic hotspots” (8, 47). For example, optogenetic stimulation of OX-neurons-to-VTA projections increase the preference for and seeking of food rewards (48), and OXR antagonists reduce seeking and motivation for drugs of abuse (49, 50). In addition, OX signaling has been proposed to promote the motivational activation necessary for interaction with the environment and adaptive behaviors (51).
Predicted effects of pharmacological orexin receptor modulation on NPS in PwD
Background
The first drugs targeting orexin receptors demonstrating pharmacodynamic effects were two DORAs [namely, almorexant (ACT-078573) (11) and SB-649868 (52)]. Three DORAs are currently approved for the treatment of insomnia (i.e., suvorexant, lemborexant, daridorexant); furthermore, other psychiatric indications are also being investigated using DORAs or selective OX1R or OX2R antagonists (i.e., SO1RA or SO2RA) (13). OX2R agonists (SO2RAgs) with wake-promoting effects are in development for the treatment of narcolepsy (53, 54).
Different treatment regimens were tested in trials with OXR antagonists, depending on the targeted symptoms and the drug used. For example, in trials with DORAs/SO2RAs for insomnia and depression (55, 56) the drugs were administered at bedtime, and in a trial with SO1RA for binge eating disorder the drug was given during morning and evening meals (NCT04753164) (57). In the context of the possible use of OXR antagonists for the treatment of NPS in PwD, bedtime administration has the advantages of maximizing the drug's efficacy on nighttime problems and reducing the risk of daytime somnolence; however, little effect may be expected on disturbances that mostly manifest during the day [except those instances where amelioration of daytime symptoms is achieved through improved nighttime problems, as in the case of depression (56)]. Conversely, daytime dosing may have a greater impact on symptoms, which are expressed during the day, but (depending on the dose) it may be associated with a higher risk of somnolence. SO2RAgs, given in the morning to stabilize daytime wakefulness, may potentially also improve sleep at night by secondary means and thus normalize the disturbed wake-sleep rhythms observed in PwD.
Sleep problems (insomnia, excessive daytime sleepiness)
PwD frequently present with difficulties in falling asleep (58), fragmented sleep (59), and excessive sleepiness during daytime (60). The sleep-wake disturbance in PwD leads to a progressive deterioration of circadian rhythm (61) and, in advanced stages of dementia, even to a reversal of the day-night sleep pattern (58). Sleep disturbance in PwD has been associated with the presence of other NPS such as anxiety and hyperactivity (62).
OXR antagonists administered at bedtime might have beneficial effects on sleep-related NPS in PwD. Indeed, two recent studies showed that sleep problems in AD can be ameliorated by DORAs (i.e., suvorexant:(63); lemborexant:(64)). Both trials showed improved sleep during the night. Actigraphy measurements in the lemborexant study demonstrated reduced sleep bouts during daytime, indicating a less disturbed sleep-wake rhythm as consequence of the nighttime treatment. Possibly due to the relatively long half-life of the drug, daytime somnolence was reported in the suvorexant trial, although this was not considered severe (65). Despite these beneficial effects on sleep parameters, effects of DORA on other nighttime NPS, including nighttime agitation, have not yet been assessed.
Given the wake-promoting effects of SO2RAgs, morning dosing may reduce the excessive daytime sleepiness observed in PwD and in addition help restore a normal sleep-wake rhythm.
Hyperactivity (agitation/aggression, irritability, disinhibition, aberrant motor behavior) and anxiety
Agitation is one of the most frequent and pervasive NPS in PwD. Agitated behaviors are accompanied by signs of emotional distress and may include excessive motor activity (e.g., pacing, rocking, restlessness), verbal aggression (e.g., yelling, screaming), and/or physical aggression (e.g., grabbing, scratching, slamming doors) (66). Agitated behaviors can occur both during the day and the night (67), with nighttime agitation posing a huge burden for caregivers (68). Most aggressive behaviors shown by PwD can be interpreted as being reactive to environmental circumstances (e.g., lack of understanding of the caregivers' intentions, leading to rejection of care) (69). Other hyperactive behaviors include irritability and aberrant motor behavior, which may present as wandering, purposeless activity (e.g., insistently repeating demands or questions) and inappropriate activities (e.g., hiding objects in inappropriate places) (70). Anxiety is also highly prevalent in PwD (71), and it is expressed with both psychological and somatic manifestations (e.g., worry, palpitations, shortness of breath) (72).
From a neuropsychological perspective, hyperactive NPS and agitation, in particular, have been proposed to stem from an aberrant regulation of emotional salience, which may lead to overestimation of threat, hypervigilance and lowered stress thresholds (2).
As described above, the OX system regulates several behaviors of relevance for hyperactive NPS and anxiety. Specifically, OX system activation increases the motor output and stress reactivity, and OXR antagonists reduce reactive aggression, stress-induced behavioral changes, and anxiety in animals. Initial evidence in acute challenge tests in humans also indicate anxiolytic or stress-reducing capacity of SO1RAs or suvorexant (73–75). These laboratory findings suggest that an OXR antagonist might reduce hyperactive NPS and anxiety. One behavior that could benefit the most from bedtime administration would be nighttime agitation, because of the direct impact of the drug on both sleep and emotional reactivity.
Interestingly, the first beneficial effects of DORAs on hyperactive behaviors have been recently demonstrated in the case of nocturnal delirium. Delirium is a common condition in elderly and critically ill patients (76), characterized by a disturbance of consciousness which can be accompanied by hyperactive behaviors (77). Administration of suvorexant has been shown to improve nocturnal delirium in PwD and critically ill patients (76) (studies conducted only in Japan for the moment). These findings suggest that hyperactive behaviors may benefit from administration of DORAs; however, properly designed, controlled studies are needed to assess whether DORAs/SORAs improve hyperactive NPS in PwD. A clinical trial (NCT05307692) currently investigates the effects of seltorexant (a SO2RA) specifically on agitation as a primary outcome in patients with AD (78). Nobody has yet explored the effect of DORAs/SORAs on hyperactive NPS in the context of neuropsychiatric conditions other than PwD.
To the best of our knowledge, no trial has yet studied the effect of OXR antagonists on hyperactivity, when given during the day. Such timing of administration might attenuate hyperactive daytime symptoms but, depending on the level on OX2R antagonism exerted by the drug, could simultaneously also increase the daytime sleepiness.
Apathy
Apathy is another NPS prevalent in PwD, which often presents itself already in the pre-dementia phase. Apathy is characterized by a lack of motivation, reduced interest in rewarding situations, and akinesia and has major impact on patients' quality of life (2). As mentioned above, preclinical studies showed that activation of the OX system positively regulates reward processing and motivational activation, and OXR antagonism reduces seeking of and motivation for rewards.
Given the blunting of reward perception by antagonism of orexin signaling, one can hypothesize that OXR antagonists may worsen the apathetic phenotype shown by PwD, especially if the drugs are administered at daytime, when patients engage and interact with their environment. However, in several neurodegenerative disorders, apathy is positively correlated with sleep problems and with low levels of activity during the day (79–81), raising the question of whether restoring the sleep-wake rhythm [i.e., by reducing sleep fragmentation and increasing daytime activity as shown by the lemborexant trial (64)] may have beneficial effects on apathy. Given that orexins promote motivational activation, daytime treatment with SO2RAgs could also alleviate apathetic behaviors.
Discussion
Based on the preclinical knowledge on the function of the OX system, we believe that selected NPS experienced by PwD, including agitation/aggression, irritability, aberrant motor behavior, anxiety and sleep problems may be ameliorated by the pharmacological modulation of OXRs. At the same time, some NPS could also be negatively impacted by treatment with OXR modulators (e.g., daytime sleepiness, disturbed sleep-wake rhythm and apathy). Therefore, it is critical to choose the treatment regimen (i.e., bedtime vs. daytime), duration (i.e., acute vs. chronic) and the dose of the OX modulator wisely. Administration of OXR antagonists at bedtime, for instance, would have the clear advantage of ameliorating both sleep-wake disturbances and nighttime agitation. Secondary beneficial effects may then manifest on other NPS during the day as a consequence of a restored sleep-wake cycle: apathy and lack of engagement in daily activities, for example, may improve. Conversely, OXR antagonist administration during the day may have a more direct effect on reducing hyperactive NPS and anxiety. Although there is a risk of worsening sleep-wake problems and apathy, such risk could be mitigated by adopting an “as needed” (i.e., pro re nata, PRN) administration: for example, patients may be given an OXR antagonist only when they present with a presumably OXR modulator-responsive NPS (e.g., agitation). Different types of NPS may also require different degrees of OXR blockade or activation to be modulated. While studies suggested that at least 65% blockade of OX2R is necessary to promote sleep (12, 82), the level of OX1R/OX2R blockade/activation needed to modulate NPS-relevant behaviors is not known. Gathering more knowledge on this aspect may allow further fine-tuning of the treatment for a better efficacy-safety balance.
Identifying specific groups of PwD whose NPS are likely to respond to OXR modulation could be an elegant approach for a targeted treatment of NPS. This is challenging as NPS are not stable over time (83, 84) and several types of NPS often co-occur in PwD (85–87) [e.g., patients might present both apathy and agitation (88)].
SO2RAgs, although still very early in clinical development, should also be kept in mind as potential treatment that might become available in the future. A combination of OXR agonists, for instance, during the day, with OXR antagonists given during the night, might allow the successful treatment of the NPS spectra experienced by certain patients.
In the above considerations, one must consider the expected changes in the OX system itself that may occur in the context of neurodegeneration. Several studies have investigated OX levels in the cerebrospinal fluid (CSF) of PwD (especially in AD patients). Despite some evidence indicating higher OX levels in the cerebrospinal fluid (CSF) (89) and a positive correlation with the presence of NPS found in one study (90), a recent meta-analysis did not support the notion that CSF OX levels in AD patients differ from those of healthy controls (91). In apparent discrepancy to the lack of change in CSF OX levels, AD brains present with a 40-70% reduction in the number of OX neurons (73, 92, 93). These findings might be explained by a hyperactive phenotype of the remaining OX cells, induced by either cell-autonomous or circuit-related mechanisms, which may lead to higher OX production and release. Of note, a recent study showed that aged mice with more fragmented sleep, have a reduced number of OX neurons that are hyperexcitable due to impaired potassium channel currents (94), thus providing a mechanistic basis for the observed sleep disturbances. However, human evidence for a direct contribution of OX system dysregulation to NPS in PwD, is yet to be demonstrated.
In summary, there is increasing preclinical and emerging clinical evidence suggesting that the OX system is likely involved in the expression of several NPS in PwD. Pharmacological modulation of OXR signaling has the potential to improve certain NPS. The type of intervention (dual or selective OXR antagonism or agonism) and treatment regimen (daytime vs. nighttime administration; chronic vs. pro re nata) must be carefully chosen and adapted to the individual patient and symptomatology so as not to cause unwanted worsening of other NPS. We hope that future clinical studies will explore the efficacy and safety of OXR modulators in PwD.
Data availability statement
The original contributions presented in the study are included in the article/Supplementary material, further inquiries can be directed to the corresponding author.
Ethics statement
Experimental procedures were approved by the Basel-Landschaft Veterinary Office and adhered to Swiss federal regulations on animal experimentation.
Author contributions
Conceptualization: GB, PC, and MS. Writing—original draft preparation: GB. Writing—review and editing: GB, PC, HM, and MS. Investigation and formal analysis: GB and HM. Supervision: MS. All authors contributed to the article and approved the submitted version.
Acknowledgments
We thank Catherine Roch and Philippe Panchaud for critical discussions while formulating the hypotheses presented in this Perspective.
Conflict of interest
Authors GB, PC, HM, and MS are full-time employees of Idorsia Pharmaceuticals Ltd.
The authors declare that this study received funding from Idorsia Pharmaceuticals Ltd., Allschwil, Switzerland. The funder had the following involvement with the study: conceptualization and investigation.
Publisher's note
All claims expressed in this article are solely those of the authors and do not necessarily represent those of their affiliated organizations, or those of the publisher, the editors and the reviewers. Any product that may be evaluated in this article, or claim that may be made by its manufacturer, is not guaranteed or endorsed by the publisher.
Supplementary material
The Supplementary Material for this article can be found online at: https://www.frontiersin.org/articles/10.3389/fpsyt.2022.1052233/full#supplementary-material
References
2. Lanctot KL, Amatniek J, Ancoli-Israel S, Arnold SE, Ballard C, Cohen-Mansfield J, et al. Neuropsychiatric signs and symptoms of Alzheimer's disease: new treatment paradigms. Alzheimers Dement (N Y). (2017) 3:440–9. doi: 10.1016/j.trci.2017.07.001
3. Cummings J. New approaches to symptomatic treatments for Alzheimer's disease. Mol Neurodegener. (2021) 16:2. doi: 10.1186/s13024-021-00424-9
4. Lee G, Cummings J, Decourt B, Leverenz JB, Sabbagh MN. Clinical drug development for dementia with Lewy bodies: past and present. Expert Opin Investig Drugs. (2019) 28:951–65. doi: 10.1080/13543784.2019.1681398
5. Magierski R, Sobow T, Schwertner E, Religa D. Pharmacotherapy of behavioral and psychological symptoms of dementia: state of the art and future progress. Front Pharmacol. (2020) 11:1168. doi: 10.3389/fphar.2020.01168
6. Koch J, Amos JG, Beattie E, Lautenschlager NT, Doyle C, Anstey KJ, et al. Non-pharmacological interventions for neuropsychiatric symptoms of dementia in residential aged care settings: An umbrella review. Int J Nurs Stud. (2022) 128:104187. doi: 10.1016/j.ijnurstu.2022.104187
7. Dale NC, Hoyer D, Jacobson LH, Pfleger KDG, Johnstone EKM. Orexin signaling: a complex, multifaceted process. Front Cell Neurosci. (2022) 16:812359. doi: 10.3389/fncel.2022.812359
8. Katzman MA, Katzman MP. Neurobiology of the orexin system and its potential role in the regulation of hedonic tone. Brain Sci. (2022) 12:150. doi: 10.3390/brainsci12020150
9. Sakurai T, Amemiya A, Ishii M, Matsuzaki I, Chemelli RM, Tanaka H, et al. Orexins and orexin receptors: a family of hypothalamic neuropeptides and G protein-coupled receptors that regulate feeding behavior. Cell. (1998) 92:573–85. doi: 10.1016/S0092-8674(00)80949-6
10. Chemelli RM, Willie JT, Sinton CM, Elmquist JK, Scammell T, Lee C, et al. Narcolepsy in orexin knockout mice: molecular genetics of sleep regulation. Cell. (1999) 98:437–51. doi: 10.1016/S0092-8674(00)81973-X
11. Brisbare-Roch C, Dingemanse J, Koberstein R, Hoever P, Aissaoui H, Flores S, et al. Promotion of sleep by targeting the orexin system in rats, dogs and humans. Nat Med. (2007) 13:150–5. doi: 10.1038/nm1544
12. Treiber A, de Kanter R, Roch C, Gatfield J, Boss C, von Raumer M, et al. The use of physiology-based pharmacokinetic and pharmacodynamic modeling in the discovery of the dual orexin receptor antagonist ACT-541468. J Pharmacol Exp Ther. (2017) 362:489–503. doi: 10.1124/jpet.117.241596
13. Jacobson LH, Hoyer D, de Lecea L. Hypocretins (orexins): The ultimate translational neuropeptides. J Intern Med. (2022) 291:533–56. doi: 10.1111/joim.13406
14. Nevarez N, de Lecea L. Recent advances in understanding the roles of hypocretin/orexin in arousal, affect, and motivation. F1000Res. (2018) 7:F1000. doi: 10.12688/f1000research.15097.1
15. Tsunematsu T, Tabuchi S, Tanaka KF, Boyden ES, Tominaga M, Yamanaka A. Long-lasting silencing of orexin/hypocretin neurons using archaerhodopsin induces slow-wave sleep in mice. Behav Brain Res. (2013) 255:64–74. doi: 10.1016/j.bbr.2013.05.021
16. Sasaki K, Suzuki M, Mieda M, Tsujino N, Roth B, Sakurai T. Pharmacogenetic modulation of orexin neurons alters sleep/wakefulness states in mice. PLoS ONE. (2011) 6:e20360. doi: 10.1371/journal.pone.0020360
17. De Luca R, Nardone S, Grace KP, Venner A, Cristofolini M, Bandaru SS, et al. Orexin neurons inhibit sleep to promote arousal. Nat Commun. (2022) 13:4163. doi: 10.1038/s41467-022-31591-y
18. Adamantidis AR, Zhang F, Aravanis AM, Deisseroth K, de Lecea L. Neural substrates of awakening probed with optogenetic control of hypocretin neurons. Nature. (2007) 450:420–4. doi: 10.1038/nature06310
19. Branch AF, Navidi W, Tabuchi S, Terao A, Yamanaka A, Scammell TE, et al. Progressive loss of the orexin neurons reveals dual effects on wakefulness. Sleep. (2016) 39:369–77. doi: 10.5665/sleep.5446
20. Hasegawa E, Maejima T, Yoshida T, Masseck OA, Herlitze S, Yoshioka M, et al. Serotonin neurons in the dorsal raphe mediate the anticataplectic action of orexin neurons by reducing amygdala activity. Proc Natl Acad Sci USA. (2017) 114:E3526–E35. doi: 10.1073/pnas.1614552114
21. Beuckmann CT, Suzuki H, Musiek ES, Ueno T, Sato T, Bando M, et al. Evaluation of SAMP8 mice as a model for sleep-wake and rhythm disturbances associated with alzheimer's disease: impact of treatment with the dual orexin (hypocretin) receptor antagonist lemborexant. J Alzheimers Dis. (2021) 81:1151–67. doi: 10.3233/JAD-201054
22. Thorpe AJ, Kotz CM. Orexin A in the nucleus accumbens stimulates feeding and locomotor activity. Brain Res. (2005) 1050:156–62. doi: 10.1016/j.brainres.2005.05.045
23. Nakamura T, Uramura K, Nambu T, Yada T, Goto K, Yanagisawa M, et al. Orexin-induced hyperlocomotion and stereotypy are mediated by the dopaminergic system. Brain Res. (2000) 873:181–7. doi: 10.1016/S0006-8993(00)02555-5
24. Hara J, Beuckmann CT, Nambu T, Willie JT, Chemelli RM, Sinton CM, et al. Genetic ablation of orexin neurons in mice results in narcolepsy, hypophagia, and obesity. Neuron. (2001) 30:345–54. doi: 10.1016/S0896-6273(01)00293-8
25. Yamanaka A, Beuckmann CT, Willie JT, Hara J, Tsujino N, Mieda M, et al. Hypothalamic orexin neurons regulate arousal according to energy balance in mice. Neuron. (2003) 38:701–13. doi: 10.1016/S0896-6273(03)00331-3
26. Karnani MM, Schone C, Bracey EF, Gonzalez JA, Viskaitis P, Li HT, et al. Role of spontaneous and sensory orexin network dynamics in rapid locomotion initiation. Prog Neurobiol. (2020) 187:101771. doi: 10.1016/j.pneurobio.2020.101771
27. Kosse C, Schone C, Bracey E, Burdakov D. Orexin-driven GAD65 network of the lateral hypothalamus sets physical activity in mice. Proc Natl Acad Sci U S A. (2017) 114:4525–30. doi: 10.1073/pnas.1619700114
28. Falkner AL, Grosenick L, Davidson TJ, Deisseroth K, Lin D. Hypothalamic control of male aggression-seeking behavior. Nat Neurosci. (2016) 19:596–604. doi: 10.1038/nn.4264
29. Lischinsky JE, Lin D. Neural mechanisms of aggression across species. Nat Neurosci. (2020) 23:1317–28. doi: 10.1038/s41593-020-00715-2
30. Todd WD, Machado NL, A. time to fight: Circadian control of aggression and associated autonomic support. Auton Neurosci. (2019) 217:35–40. doi: 10.1016/j.autneu.2018.12.008
31. Nollet M, Gaillard P, Tanti A, Girault V, Belzung C, Leman S. Neurogenesis-independent antidepressant-like effects on behavior and stress axis response of a dual orexin receptor antagonist in a rodent model of depression. Neuropsychopharmacology. (2012) 37:2210–21. doi: 10.1038/npp.2012.70
32. Flanigan ME, Aleyasin H, Li L, Burnett CJ, Chan KL, LeClair KB, et al. Orexin signaling in GABAergic lateral habenula neurons modulates aggressive behavior in male mice. Nat Neurosci. (2020) 23:638–50. doi: 10.1038/s41593-020-0617-7
33. Katahira H, Sunagawa M, Watanabe D, Kanada Y, Katayama A, Yamauchi R, et al. Antistress effects of Kampo medicine “Yokukansan” via regulation of orexin secretion. Neuropsychiatr Dis Treat. (2017) 13:863–72. doi: 10.2147/NDT.S129418
34. Maes T, Mascaro C, Rotllant D, Lufino MMP, Estiarte A, Guibourt N, et al. Modulation of KDM1A with vafidemstat rescues memory deficit and behavioral alterations. PLoS ONE. (2020) 15:e0233468. doi: 10.1371/journal.pone.0233468
35. Vasilopoulou F, Bellver-Sanchis A, Companys-Alemany J, Jarne-Ferrer J, Irisarri A, Palomera-Avalos V, et al. Cognitive decline and BPSD are concomitant with autophagic and synaptic deficits associated with G9a alterations in aged SAMP8 mice. Cells. (2022) 11:2603. doi: 10.3390/cells11162603
36. Espana RA, Plahn S, Berridge CW. Circadian-dependent and circadian-independent behavioral actions of hypocretin/orexin. Brain Res. (2002) 943:224–36. doi: 10.1016/S0006-8993(02)02653-7
37. Kuru M, Ueta Y, Serino R, Nakazato M, Yamamoto Y, Shibuya I, et al. Centrally administered orexin/hypocretin activates HPA axis in rats. Neuroreport. (2000) 11:1977–80. doi: 10.1097/00001756-200006260-00034
38. Deutschman CS, Raj NR, McGuire EO, Kelz MB. Orexinergic activity modulates altered vital signs and pituitary hormone secretion in experimental sepsis. Crit Care Med. (2013) 41:e368–75. doi: 10.1097/CCM.0b013e31828e9843
39. Sakamoto F, Yamada S, Ueta Y. Centrally administered orexin-A activates corticotropin-releasing factor-containing neurons in the hypothalamic paraventricular nucleus and central amygdaloid nucleus of rats: possible involvement of central orexins on stress-activated central CRF neurons. Regul Pept. (2004) 118:183–91. doi: 10.1016/j.regpep.2003.12.014
40. Reyes TM, Walker JR, DeCino C, Hogenesch JB, Sawchenko PE. Categorically distinct acute stressors elicit dissimilar transcriptional profiles in the paraventricular nucleus of the hypothalamus. J Neurosci. (2003) 23:5607–16. doi: 10.1523/JNEUROSCI.23-13-05607.2003
41. Sargin D. The role of the orexin system in stress response. Neuropharmacology. (2019) 154:68–78. doi: 10.1016/j.neuropharm.2018.09.034
42. Li Y, Li S, Wei C, Wang H, Sui N, Kirouac GJ. Orexins in the paraventricular nucleus of the thalamus mediate anxiety-like responses in rats. Psychopharmacology (Berl). (2010) 212:251–65. doi: 10.1007/s00213-010-1948-y
43. Lungwitz EA, Molosh A, Johnson PL, Harvey BP, Dirks RC, Dietrich A, et al. Orexin-A induces anxiety-like behavior through interactions with glutamatergic receptors in the bed nucleus of the stria terminalis of rats. Physiol Behav. (2012) 107:726–32. doi: 10.1016/j.physbeh.2012.05.019
44. Bahaaddini M, Khatamsaz S, Esmaeili-Mahani S, Abbasnejad M, Raoof M. The role of trigeminal nucleus caudalis orexin 1 receptor in orofacial pain-induced anxiety in rat. Neuroreport. (2016) 27:1107–13. doi: 10.1097/WNR.0000000000000660
45. Brown RM, Dayas CV, James MH, Smith RJ. New directions in modelling dysregulated reward seeking for food and drugs. Neurosci Biobehav Rev. (2022) 132:1037–48. doi: 10.1016/j.neubiorev.2021.10.043
46. Peleg-Raibstein D, Burdakov D. Do orexin/hypocretin neurons signal stress or reward? Peptides. (2021) 145:170629. doi: 10.1016/j.peptides.2021.170629
47. Vaseghi S, Zarrabian S, Haghparast A. Reviewing the role of the orexinergic system and stressors in modulating mood and reward-related behaviors. Neurosci Biobehav Rev. (2022) 133:104516. doi: 10.1016/j.neubiorev.2021.104516
48. Thomas CS, Mohammadkhani A, Rana M, Qiao M, Baimel C, Borgland SL. Optogenetic stimulation of lateral hypothalamic orexin/dynorphin inputs in the ventral tegmental area potentiates mesolimbic dopamine neurotransmission and promotes reward-seeking behaviours. Neuropsychopharmacology. (2022) 47:728–40. doi: 10.1038/s41386-021-01196-y
49. James MH, Charnley JL, Levi EM, Jones E, Yeoh JW, Smith DW, et al. Orexin-1 receptor signalling within the ventral tegmental area, but not the paraventricular thalamus, is critical to regulating cue-induced reinstatement of cocaine-seeking. Int J Neuropsychopharmacol. (2011) 14:684–90. doi: 10.1017/S1461145711000423
50. Fragale JE, Pantazis CB, James MH, Aston-Jones G. The role of orexin-1 receptor signaling in demand for the opioid fentanyl. Neuropsychopharmacology. (2019) 44:1690–7. doi: 10.1038/s41386-019-0420-x
51. Mahler SV, Moorman DE, Smith RJ, James MH, Aston-Jones G. Motivational activation: a unifying hypothesis of orexin/hypocretin function. Nat Neurosci. (2014) 17:1298–303. doi: 10.1038/nn.3810
52. Bettica P, Nucci G, Pyke C, Squassante L, Zamuner S, Ratti E, et al. Phase I studies on the safety, tolerability, pharmacokinetics and pharmacodynamics of SB-649868, a novel dual orexin receptor antagonist. J Psychopharmacol. (2012) 26:1058–70. doi: 10.1177/0269881111408954
53. Fujimoto T, Rikimaru K, Fukuda K, Sugimoto H, Masuda K, Ohyabu N, et al. Discovery of TAK-925 as a potent, selective, and brain-penetrant orexin 2 receptor agonist. ACS Med Chem Lett. (2022) 13:457–62. doi: 10.1021/acsmedchemlett.1c00626
54. Yukitake H, Fujimoto T, Ishikawa T, Suzuki A, Shimizu Y, Rikimaru K, et al. TAK-925, an orexin 2 receptor-selective agonist, shows robust wake-promoting effects in mice. Pharmacol Biochem Behav. (2019) 187:172794. doi: 10.1016/j.pbb.2019.172794
55. Mignot E, Mayleben D, Fietze I, Leger D, Zammit G, Bassetti CLA, et al. Safety and efficacy of daridorexant in patients with insomnia disorder: results from two multicentre, randomised, double-blind, placebo-controlled, phase 3 trials. Lancet Neurol. (2022) 21:125–39. doi: 10.1016/S1474-4422(21)00436-1
56. Savitz A, Wajs E, Zhang Y, Xu H, Etropolski M, Thase ME, et al. Efficacy and safety of seltorexant as adjunctive therapy in major depressive disorder: a phase 2b, randomized, placebo-controlled, adaptive dose-finding study. Int J Neuropsychopharmacol. (2021) 24:965–76. doi: 10.1093/ijnp/pyab050
57. Clinical Trials.gov. Multicenter Study to Evaluate the Efficacy and Safety of Oral ACT-539313 in the Treatment of Adults With Moderate to Severe Binge Eating Disorder. Available online at: https://clinicaltrials.gov/ct2/show/NCT04753164 (accessed September 20, 2022).
58. Dauvilliers Y. Hypocretin/orexin, sleep and Alzheimer's disease. Front Neurol Neurosci. (2021) 45:139–49. doi: 10.1159/000514967
59. Brzecka A, Leszek J, Ashraf GM, Ejma M, Avila-Rodriguez MF, Yarla NS, et al. Sleep disorders associated with Alzheimer's disease: a perspective. Front Neurosci. (2018) 12:330. doi: 10.3389/fnins.2018.00330
60. Boeve A, Ferman TJ, Aakre J, St Louis E, Silber M, Machulda M, et al. Excessive daytime sleepiness in major dementia syndromes. Am J Alzheimers Dis Other Demen. (2019) 34:261–4. doi: 10.1177/1533317519828046
61. Benca R, Herring WJ, Khandker R, Qureshi ZP. Burden of insomnia and sleep disturbances and the impact of sleep treatments in patients with probable or possible Alzheimer's disease: a structured literature review. J Alzheimers Dis. (2022) 86:83–109. doi: 10.3233/JAD-215324
62. Kabeshita Y, Adachi H, Matsushita M, Kanemoto H, Sato S, Suzuki Y, et al. Sleep disturbances are key symptoms of very early stage Alzheimer disease with behavioral and psychological symptoms: a Japan multi-center cross-sectional study (J-BIRD). Int J Geriatr Psychiatry. (2017) 32:222–30. doi: 10.1002/gps.4470
63. Herring WJ, Ceesay P, Snyder E, Bliwise D, Budd K, Hutzelmann J, et al. Polysomnographic assessment of suvorexant in patients with probable Alzheimer's disease dementia and insomnia: a randomized trial. Alzheimers Dement. (2020) 16:541–51. doi: 10.1002/alz.12035
64. Moline M, Thein S, Bsharat M, Rabbee N, Kemethofer-Waliczky M, Filippov G, et al. Safety and efficacy of lemborexant in patients with irregular sleep-wake rhythm disorder and Alzheimer's disease dementia: results from a phase 2 randomized clinical trial. J Prev Alzheimers Dis. (2021) 8:7–18. doi: 10.14283/jpad.2020.69
65. Voysey ZJ, Barker RA, Lazar AS. The treatment of sleep dysfunction in neurodegenerative disorders. Neurotherapeutics. (2021) 18:202–16. doi: 10.1007/s13311-020-00959-7
66. Deardorff WJ, Grossberg GT. Behavioral and psychological symptoms in Alzheimer's dementia and vascular dementia. Handb Clin Neurol. (2019) 165:5–32. doi: 10.1016/B978-0-444-64012-3.00002-2
67. Cohen-Mansfield J, Marx MS, Rosenthal AS, A. description of agitation in a nursing home. J Gerontol. (1989) 44:M77–84. doi: 10.1093/geronj/44.3.M77
68. Walther S, Mahlberg R, Eichmann U, Kunz D. Delta-9-tetrahydrocannabinol for nighttime agitation in severe dementia. Psychopharmacology (Berl). (2006) 185:524–8. doi: 10.1007/s00213-006-0343-1
69. Volicer L. Importance of distinguishing reactive and proactive aggression in dementia care. J Geriatr Psychiatry Neurol. (2021) 34:243–7. doi: 10.1177/0891988720924706
70. Nagata T, Shinagawa S, Ochiai Y, Kada H, Kasahara H, Nukariya K, et al. Relationship of frontal lobe dysfunction and aberrant motor behaviors in patients with Alzheimer's disease. Int Psychogeriatr. (2010) 22:463–9. doi: 10.1017/S1041610209991323
71. Leung DKY, Chan WC, Spector A, Wong GHY. Prevalence of depression, anxiety, and apathy symptoms across dementia stages: a systematic review and meta-analysis. Int J Geriatr Psychiatry. (2021) 36:1330–44. doi: 10.1002/gps.5556
72. Seignourel PJ, Kunik ME, Snow L, Wilson N, Stanley M. Anxiety in dementia: a critical review. Clin Psychol Rev. (2008) 28:1071–82. doi: 10.1016/j.cpr.2008.02.008
73. Fronczek R, van Geest S, Frolich M, Overeem S, Roelandse FW, Lammers GJ, et al. Hypocretin (orexin) loss in Alzheimer's disease. Neurobiol Aging. (2012) 33:1642–50. doi: 10.1016/j.neurobiolaging.2011.03.014
74. Kaufmann P, Ort M, Golor G, Kornberger R, Dingemanse J. Multiple-dose clinical pharmacology of the selective orexin-1 receptor antagonist ACT-539313. Prog Neuropsychopharmacol Biol Psychiatry. (2021) 108:110166. doi: 10.1016/j.pnpbp.2020.110166
75. Gorka SM, Khorrami KJ, Manzler CA, Phan KL. Acute orexin antagonism selectively modulates anticipatory anxiety in humans: implications for addiction and anxiety. Transl Psychiatry. (2022) 12:308. doi: 10.1038/s41398-022-02090-x
76. Xu S, Cui Y, Shen J, Wang P. Suvorexant for the prevention of delirium: a meta-analysis. Medicine (Baltimore). (2020) 99:e21043. doi: 10.1097/MD.0000000000021043
77. O'Keeffe ST. Clinical subtypes of delirium in the elderly. Dement Geriatr Cogn Disord. (1999) 10:380–5. doi: 10.1159/000017174
78. Clinical Trials.gov. A Study of Seltorexant in Participants With Probable Alzheimer's Disease. Available online at: https://clinicaltrials.gov/ct2/show/NCT05307692.
79. Mulin E, Zeitzer JM, Friedman L, Le Duff F, Yesavage J, Robert PH, et al. Relationship between apathy and sleep disturbance in mild and moderate Alzheimer's disease: an actigraphic study. J Alzheimers Dis. (2011) 25:85–91. doi: 10.3233/JAD-2011-101701
80. Zhou G, Liu S, Yu X, Zhao X, Ma L, Shan P. High prevalence of sleep disorders and behavioral and psychological symptoms of dementia in late-onset Alzheimer disease: A study in Eastern China. Medicine (Baltimore). (2019) 98:e18405. doi: 10.1097/MD.0000000000018405
81. Cosin C, Sibon I, Poli M, Allard M, Debruxelles S, Renou P, et al. Circadian sleep/wake rhythm abnormalities as a risk factor of a poststroke apathy. Int J Stroke. (2015) 10:710–5. doi: 10.1111/ijs.12433
82. Gotter AL, Winrow CJ, Brunner J, Garson SL, Fox SV, Binns J, et al. The duration of sleep promoting efficacy by dual orexin receptor antagonists is dependent upon receptor occupancy threshold. BMC Neurosci. (2013) 14:90. doi: 10.1186/1471-2202-14-90
83. Eikelboom WS, den Teuling A, Pol DE, Coesmans M, Franzen S, Jiskoot LC, et al. Biweekly fluctuations of neuropsychiatric symptoms according to the Neuropsychiatric Inventory: Erratic symptoms or scores? Int J Geriatr Psychiatry. (2022) 37:10.1002/gps.5770. doi: 10.1002/gps.5770
84. Connors MH, Seeher KM, Crawford J, Ames D, Woodward M, Brodaty H. The stability of neuropsychiatric subsyndromes in Alzheimer's disease. Alzheimers Dement. (2018) 14:880–8. doi: 10.1016/j.jalz.2018.02.006
85. Regier NG, Hodgson NA, Gitlin LN. Neuropsychiatric symptom profiles of community-dwelling persons living with dementia: Factor structures revisited. Int J Geriatr Psychiatry. (2020) 35:1009–20. doi: 10.1002/gps.5323
86. Krell-Roesch J, Syrjanen JA, Vassilaki M, Lowe VJ, Vemuri P, Mielke MM, et al. Brain regional glucose metabolism, neuropsychiatric symptoms, and the risk of incident mild cognitive impairment: the mayo clinic study of aging. Am J Geriatr Psychiatry. (2021) 29:179–91. doi: 10.1016/j.jagp.2020.06.006
87. Lyketsos CG, Sheppard JM, Steinberg M, Tschanz JA, Norton MC, Steffens DC, et al. Neuropsychiatric disturbance in Alzheimer's disease clusters into three groups: the cache county study. Int J Geriatr Psychiatry. (2001) 16:1043–53. doi: 10.1002/gps.448
88. Chow TW, Binns MA, Cummings JL, Lam I, Black SE, Miller BL, et al. Apathy symptom profile and behavioral associations in frontotemporal dementia vs dementia of Alzheimer type. Arch Neurol. (2009) 66:888–93. doi: 10.1001/archneurol.2009.92
89. Gabelle A, Jaussent I, Hirtz C, Vialaret J, Navucet S, Grasselli C, et al. Cerebrospinal fluid levels of orexin-A and histamine, and sleep profile within the Alzheimer process. Neurobiol Aging. (2017) 53:59–66. doi: 10.1016/j.neurobiolaging.2017.01.011
90. Liguori C, Mercuri NB, Nuccetelli M, Izzi F, Bernardini S, Placidi F. Cerebrospinal fluid orexin levels and nocturnal sleep disruption in Alzheimer's disease patients showing neuropsychiatric symptoms. J Alzheimers Dis. (2018) 66:993–9. doi: 10.3233/JAD-180769
91. Treu SP, Plante DT. Cerebrospinal fluid orexin in Alzheimer's disease: a systematic review and meta-analysis. Sleep Med. (2021) 85:230–8. doi: 10.1016/j.sleep.2021.07.007
92. Oh J, Eser RA, Ehrenberg AJ, Morales D, Petersen C, Kudlacek J, et al. Profound degeneration of wake-promoting neurons in Alzheimer's disease. Alzheimers Dement. (2019) 15:1253–63. doi: 10.1016/j.jalz.2019.06.3916
93. Kasanuki K, Iseki E, Kondo D, Fujishiro H, Minegishi M, Sato K, et al. Neuropathological investigation of hypocretin expression in brains of dementia with Lewy bodies. Neurosci Lett. (2014) 569:68–73. doi: 10.1016/j.neulet.2014.03.020
Keywords: dementia, neuropsychiatric symptoms, behavioral symptoms, orexin, orexin receptor modulators
Citation: Bergamini G, Coloma P, Massinet H and Steiner MA (2022) What evidence is there for implicating the brain orexin system in neuropsychiatric symptoms in dementia? Front. Psychiatry 13:1052233. doi: 10.3389/fpsyt.2022.1052233
Received: 23 September 2022; Accepted: 07 November 2022;
Published: 25 November 2022.
Edited by:
Debora Cutuli, Sapienza University of Rome, ItalyReviewed by:
Audun Vik-mo, University of Bergen, NorwayCopyright © 2022 Bergamini, Coloma, Massinet and Steiner. This is an open-access article distributed under the terms of the Creative Commons Attribution License (CC BY). The use, distribution or reproduction in other forums is permitted, provided the original author(s) and the copyright owner(s) are credited and that the original publication in this journal is cited, in accordance with accepted academic practice. No use, distribution or reproduction is permitted which does not comply with these terms.
*Correspondence: Giorgio Bergamini, Z2lvcmdpby5iZXJnYW1pbmlAaWRvcnNpYS5jb20=