- 1Gdansk University of Physical Education and Sport, Gdańsk, Poland
- 2Fujian Normal University, Fuzhou, China
- 3Escola Superior Desporto e Lazer, Instituto Politécnico de Viana do Castelo, Rua Escola Industrial e Comercial de Nun’Álvares, Viana do Castelo, Portugal
- 4Sport Physical Activity and Health Research and Innovation Center, Viana do Castelo, Portugal
Introduction: This study aimed to compare the effects of structured sports games (SG) and psychomotricity activities (PCM) on the locomotor, stability, and manipulative motor competencies of preschool children.
Methods: A randomized controlled trial was conducted over an 8-week period, involving two experimental groups (SG, n = 30 and PCM, n = 30) and one control group (CG, n = 28), with participants attending two intervention sessions per week. A total of 88 5-year-old children participated in the experiment (boys n = 48; girls n = 40). They were evaluated three times (at baseline, after 4 weeks, and after 8 weeks) using the Motor Competence Assessment test to measure their locomotor, stability, and manipulative motor competencies.
Results: The scores were standardized to percentiles based on sex and age. However, significant differences were observed between groups post-intervention in the locomotor domain (p = 0.003;
Discussion: Our study highlights the significant benefits of increased practice in developing motor competence, particularly in children’s locomotor and manipulative skills. Additionally, at this age, fun, competition, and social interaction seem to play a crucial role, as the SG group demonstrated greater improvements compared to the PCM group.
Introduction
Motor competence (MC) refers to an individual’s ability to perform a wide range of motor skills, including both fine and gross motor activities (Stodden et al., 2008; Feitoza et al., 2022). Although it has been given different expressions to MC, such as fundamental movement skills, motor development, motor efficiency, motor coordination, motor ability or motor fitness (Cattuzzo et al., 2016), there is a broad consensus that this concept imply proficiency on fundamental movements skills (Stodden et al., 2008), which are based on locomotor, stability and manipulative abilities (Gallahue and Ozmun, 2006). Thus, MC is the overall ability to perform various motor skills, while motor efficiency is the ability to perform tasks with minimal effort, motor coordination is the smooth execution of movements, motor ability reflects innate movement potential, and motor fitness involves the physical attributes that support skilled movement (Cattuzzo et al., 2016).
The MC is fundamental to a child’s overall development, particularly during the preschool years, a critical period (between 2–3 and 6–7 years of age) for acquiring and refining these skills (Gallahue and Ozmun, 2006; True et al., 2017). MC can be linked to neural maturation and the development of motor pathways in the brain, which can be essential for coordinate movements (Gerván et al., 2017; Thomason et al., 2018). Thus, neural maturation and the development of motor pathways in the brain, particularly in areas such as the motor cortex, cerebellum, and basal ganglia, may play a critical role in MC (Doyon et al., 2009). Through motor experiences, the refinement of synaptic connections, the myelination of motor neurons, and the strengthening of corticospinal and sensorimotor pathways may contribute to enable improved coordination, precision, and motor learning (Ruddy and Carson, 2013).
High levels of MC in early childhood are associated with better physical health (Sigmundsson and Haga, 2016), cognitive function, and social–emotional well-being (Robinson et al., 2015). MC may influence cognitive skills such as memory and executive control by engaging neural networks involved in motor planning, coordination, and cognitive regulation (Fernandes et al., 2016), with evidence showing that activities requiring fine and gross motor skills enhance connectivity between the cerebellum and prefrontal cortex, thereby improving working memory, inhibitory control, and cognitive flexibility (Biino et al., 2023). This is because engaging in diverse physical activities may promote muscle strength, coordination, and motor planning (Myer et al., 2015). Furthermore, children with advanced motor skills are more likely to participate in physical activities (Wrotniak et al., 2006; Williams et al., 2008), fostering healthy lifestyle habits and enhancing their self-confidence and social interactions. This is in line with the concept of physical literacy, which highlight the need of developing motivation, confidence, physical competence knowledge, and understanding to value and engage in physical activity for life (Whitehead, 2001). Physical literacy complements motor competence by providing a broader framework that includes not only the ability to perform basic motor skills, but also the knowledge, motivation, and confidence necessary to engage in physical activities throughout life, fostering a holistic development of movement capabilities (Whitehead, 2001).
The rise in sedentary behavior among preschool children poses significant risks to their MC and overall development (Sedlak et al., 2015). A recent study found that 7-year-old children with high sedentary time spent an average of 83.8 ± 55.0 min (27.4% of their sedentary time) on screen-based activities (Hoffmann et al., 2019). Those with medium sedentary time spent 82.8 ± 50.5 min (39.8% of their sedentary time), while children with low sedentary time spent 77.2 ± 59.4 min (71.3% of their sedentary time) on screens (Hoffmann et al., 2019). Studies suggest that excessive sedentary behavior delays the development of fundamental motor skills (Zacks et al., 2021). These delays can lead to poorer MC, affecting a child’s ability to engage in and enjoy physical activities (Cantell et al., 2008). Additionally, sedentary behavior can reduce muscle strength and endurance, limiting opportunities for children to develop and refine their motor skills through active play (Kolehmainen et al., 2015). In fact, it has traditionally been assumed that the development of fundamental movement skills is guaranteed and occurs naturally. However, the literature has shown that this is not the case, with the environment (especially the practice) in which the child develops playing a significant role (Goodway and Branta, 2003; Goodway and Branta, 2003; Saraiva et al., 2024). For instance, children from lower socioeconomic backgrounds or with limited access to stimulating play environments often face reduced opportunities to engage in physical activities (Ferguson et al., 2013). The lack of physical activity not only hinders motor development but also might contribute to potential long-term health issues (Vlahov et al., 2014; Musálek et al., 2021). MC acquired in childhood may lay the foundation for lifelong physical activity patterns by fostering confidence and proficiency in movement, which promotes sustained engagement in physical activity into adulthood, ultimately contributing to better health outcomes by reducing risks of obesity, cardiovascular disease, and other chronic conditions (Petrusevski et al., 2022). Therefore, reducing sedentary behavior and promoting active play are essential for fostering optimal motor development and overall health in preschool children (Bai et al., 2024).
Introducing structured practices, even when guided and supported by feedback-driven instruction, can positively impact motor competence, especially in situations where opportunities for spontaneous practice are limited (Sutapa et al., 2021). Engaging in structured physical exercise programs can significantly impact the development of MC across locomotor, stability, and manipulative domains in preschool children (Sutapa et al., 2021). For instance, a meta-analysis has showed that physical education can significantly enhance overall MC in children and adolescents (Lorås, 2020). Some studies (van et al., 2017) suggest that regular participation in structured physical activities can enhance motor skills within these domains. The literature is unclear on how to effectively improve MC, however, exposure to (quality) practice may be a key factor, with a certain degree of variability in practice being essential to expand the child’s motor repertoire (Saraiva et al., 2024). Variability refers to the dynamic process of diversifying movement experiences to prevent repetition of fixed actions, allowing participants to engage in different ways and patterns of the same type of movement (Pesce et al., 2019). This variability may enhance learning by promoting adaptability and supporting the development of motor competence through exposure to a range of movement scenarios (Sternad, 2018). Structured programs can vary widely in content and approach. For instance, sports games and general psychomotor individual activities can influence the development of MC domains. On one hand, sports games promote team interaction, and the inherent competition serves as a stimulus to challenge and develop skills. On the other hand, general psychomotor individual activities are more individualized and do not require constant interaction with a team, allowing the individual to focus solely on the task at hand. Sports games may enhance locomotor skills through dynamic movements like running, jumping, and agility actions required during gameplay, which are engaging due to the collaborative dynamics inherent in team sports (Konoh, 2013). Manipulative skills such as throwing, catching, and kicking can be particularly promoted through strategic interactions with objects in sports games settings (Hashemi et al., 2015). In contrast, general psychomotor individual activities focus on generalized body movements, emphasizing foundational aspects of MC, can be particularly interesting for targeting specific skills such as balance exercises, fine motor tasks, and basic locomotor movements, without the competitive or cooperative demands of sports games (Teixeira Costa et al., 2015; Ene et al., 2016).
Despite the potential benefits, research on how enrollment in different physical exercise programs impacts children’s MC is limited (e.g., Stodden et al., 2008; Barnett et al., 2016). Particularly lacking are experimental studies testing how structured physical exercise programs with varying content affect MC variables in children. Conducting such experimental studies could significantly advance our understanding and guide educators in directing interventions to ensure adequate development in children’s key MC areas. Thus, this study aimed to compare the effects of structured sports games (SG) and psychomotor activities (PCM) on the locomotor, stability, and manipulative motor competencies of preschool children. It was hypothesized that locomotor and manipulative abilities will be greater in SG, while stability levels will be higher in PCM. This hypothesis is based on the expectation that the specificity of the practice plays a key role. Since SG often require multidirectional movements and coordination between hands, feet and the ball, they may have a greater impact on developing competences related to these movements. In contrast, PMC typically focuses on single-body movements, which may be more relevant for tasks that require stability, potentially offering a better transfer to stability-related tests.
Methods
Trial design
The objective and hypothesis of our study are directly aligned with the study design, as they provide a framework for the comparison of the effects of SG and PCM on preschool children’s motor competencies. Our hypothesis suggests that locomotor abilities will be enhanced by SG, while PCM will have a greater impact on stability. To test this hypothesis, the study utilized a randomized controlled design, assigning preschool children to three groups: SG, PCM, and a control group (CG). By measuring MC before and after the intervention using MC tests, the study design allows for a clear comparison of the effects of SG and PCM on locomotor, stability, and manipulative motor skills, thus providing evidence to support or refute the hypothesized differences in outcomes between the groups.
The study followed the CONSORT guidelines to ensure a comprehensive reporting of information (Merkow et al., 2021). The research began after approval from Yibin Third People’s Hospital under code YBSDSRMYY-2024-01. Children and their legal guardians were briefed on the study’s design, associated risks, and potential benefits. Upon their voluntary agreement to participate, legal guardians signed informed consent forms. The study adhered to ethical standards outlined in the Declaration of Helsinki.
Participants
The study included participants who met the following criteria: (i) healthy 5-year-old children, with no sex restrictions; (ii) not currently participating in structured sports or physical exercise programs at the time of the initial evaluation; (iii) not enrolled in other intervention programs during the study period; (iv) attending all scheduled evaluations; and (v) maintaining an adherence level of 90% or higher during the intervention. The recruitment process involved directly contacting kindergartens in the region, opting for a convenience sampling strategy because it provided easy access to the population. Through kindergarten directors, parents were invited to participate in the study, and those who expressed interest in volunteering were included in the list of potential participants (see Figure 1).
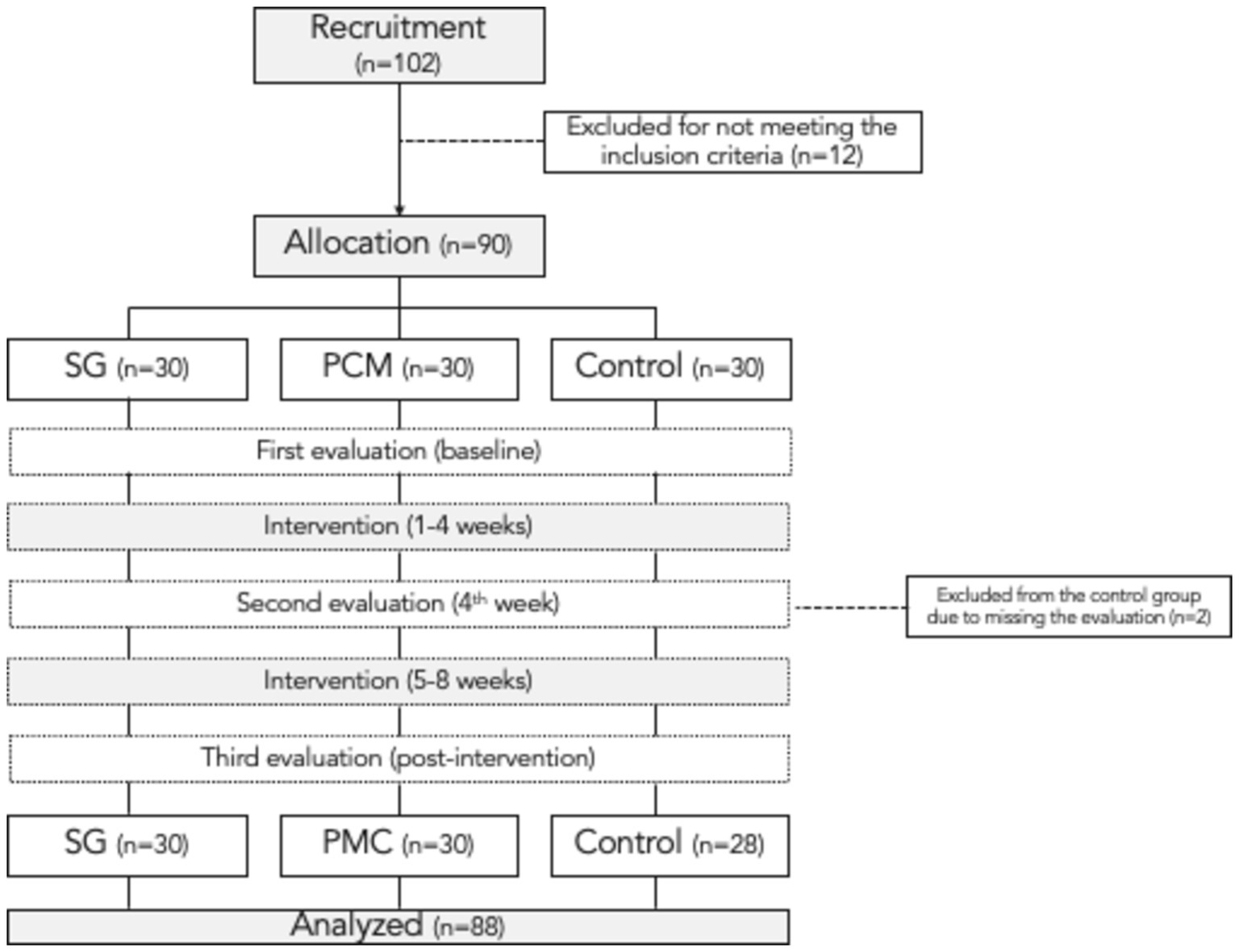
Figure 1. Reporting of the participants number over the phases of the experiment. SG, sports games groups; PCM, psychomotricity activities group.
From an initial pool of 102 potential volunteers, 88 were considered eligible (Figure 2). The remaining 14 were excluded: 9 because they were enrolled in structured physical exercise programs at the time of the first evaluation, 3 were unavailable for measurements during the initial, and the remaining 2 were not available in the second evaluation period. The sample was composed by 48 boys 308 (110.2 ± 3.6 cm, 18.8 ± 1.7 kg) and 40 girls (108.8 ± 2.9 cm, 17.1 ± 1.7 kg).
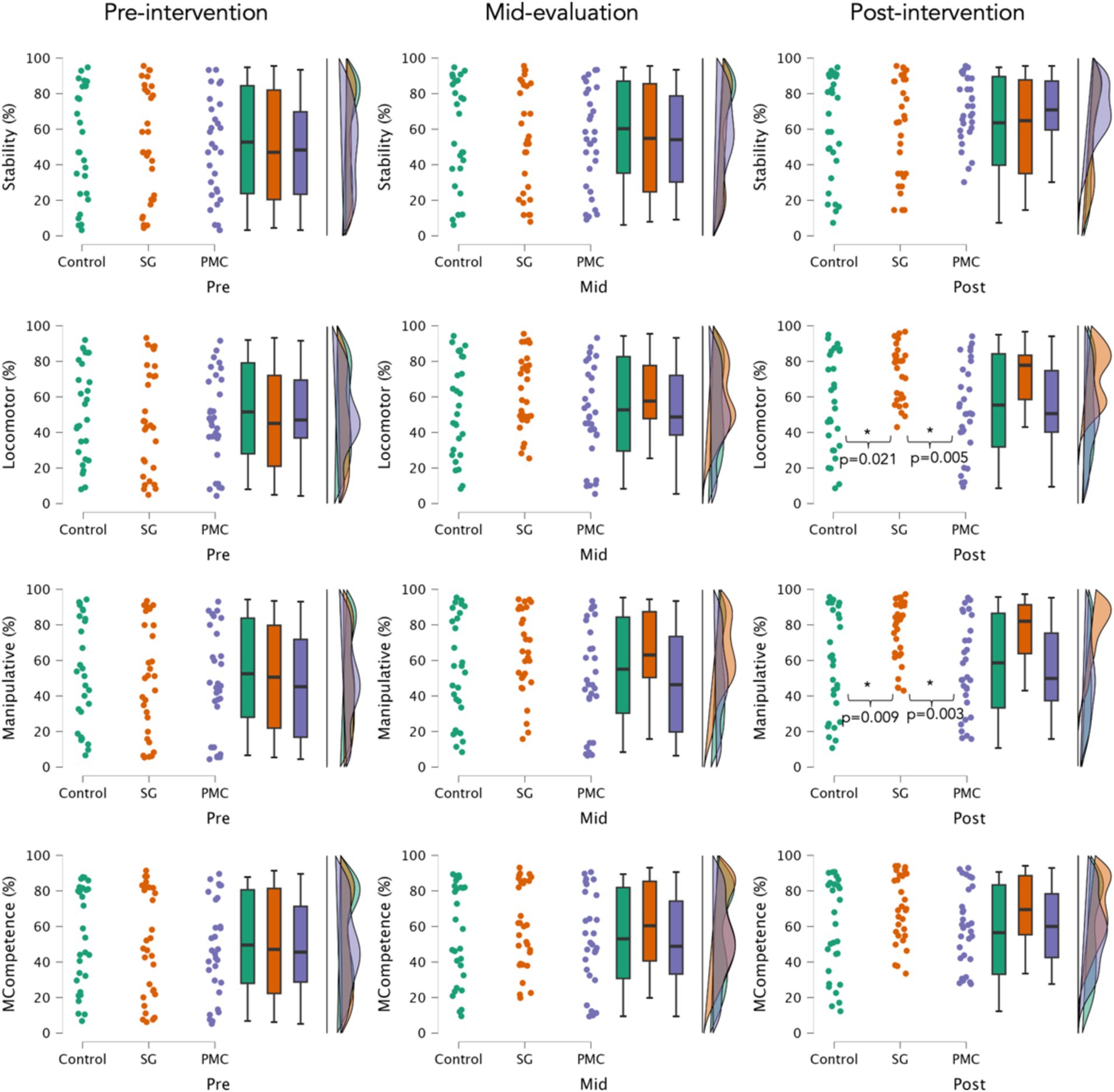
Figure 2. Percentiles (%) of motor competence across various domains over three evaluation periods. MC: overall motor competence score; *: statistically significant (p < 0.05). SG, sports games groups; PCM, psychomotricity activities group.
The study lasted 10 weeks. Eight weeks were dedicated to the intervention, while the initial week was for baseline assessments, and the final week (the 10th) was for post-intervention evaluations. The second evaluation (intermediate) occurred in the middle of the 4th week of the intervention.
Interventions
The interventions were conducted over 8 consecutive weeks, consisting of 30-min sessions twice a week, spaced 48 h apart. The participants had not been previously exposed to these interventions or any structured psychomotor programs. During the intervention period, the children did not participate in any additional physical education or structured physical programs. The sessions took place in kindergarten facilities and were led by the research team, who planned and prescribed the interventions. Each class (SG and PCM) was taught by two groups, each consisting of one lead teacher and two assistants, with each group specializing in only one of the approaches. The lead teachers had over 10 years of experience in children’s physical education, while the assistants had a minimum of 2 years of experience in the field. Supervision was provided in all sessions by the designated teacher and a team of monitors, who were trained in the methodological approaches for the classes and closely assisted the children in performing the activities. The contents and games of the interventions are presented in Table 1.
During the initial week of assessments, the research team met with the participants and conducted an unregistered pilot class to ensure the children became familiar with the teachers. The pilot session involved introductions and some ice-breaker recreational activities, aiming to establish a social foundation for the upcoming intervention phase. The session was the same for both experimental groups, ensuring similar conditions.
Outcomes
In each of the evaluation weeks, morning sessions were dedicated to conducting data assessments consistently across three evaluations. The evaluations were conducted in one-hour blocks, starting at 9 a.m., followed by 10 a.m. and 11 a.m. Participants were grouped together to facilitate efficient assessment in a circuit format. The evaluations at each time point were standardized for all participants, ensuring consistency in the timing and conditions, as well as the same day of the week for each session. This approach helped to ensure the replicability of the evaluations. These assessments were conducted indoors in a controlled environment, specifically at a temperature of 21.5°C and a relative humidity of 55%. A team of six evaluators administered the Motor Competence Assessment tests. Each evaluator received 2 weeks of training on the specific tests they were responsible for, and a pre- and post-test for accuracy was conducted in a pilot phase to ensure the quality of the observations. Children were assessed in groups, following a structured sequence that included demographic information, anthropometric measurements, and a standardized warm-up routine. This warm-up involved 5 min of jogging followed by 3 min each of upper and lower limb dynamic stretching. The assessment battery then included tests for jumping sideways, shifting platforms, ball throwing velocity, ball kicking velocity, standing long jump, and shuttle run change of direction. This approach ensured that all evaluations were conducted under consistent conditions, providing a reliable basis for data collection and analysis. The motor competence assessment battery was chosen because it has been validated (Luz et al., 2016) and showed reliable for evaluating MC in children (Silva et al., 2022). The tests were followed by 2-min rest periods, during which the children had the chance to relax and interact with their peers. Teachers offered verbal encouragement to maintain their motivation. Additionally, motivation was further enhanced by presenting the tests as challenges, designed to encourage their commitment to the evaluations.
Shifting platforms
Participants in this test started by standing on one of two wooden platforms, each measuring 25 cm by 25 cm by 2 cm, and supported by four 3.7 cm feet at the corners (Rodrigues et al., 2019). The second platform was positioned adjacent to them on the floor, either to their right or left, depending on convenience. Upon hearing the command “Ready and Go,” participants swiftly moved the adjacent platform to the opposite side and stepped onto it. This sequence was repeated as quickly as possible for 20 s. Each successful transition earned participants two points—one for moving the platform and another for stepping onto it. The test demonstrated excellent reliability with an intra-class correlation score of 0.99 (Silva et al., 2022). Each participant was allowed two attempts, with a 3-min rest period between them, and only the highest score from the two trials was recorded. The average within-participant coefficient of variability for the completed trials was 1.4%.
Jumping sideways
Participants were tasked with performing sideways jumps using both feet simultaneously for a duration of 15 s on a rectangular surface measuring 100 cm long and 60 cm wide, divided by a small wooden beam (60 cm long, 4 cm high, and 2 cm wide) (Rodrigues et al., 2019). The test began with the prompt “Ready and Go.” Points were awarded for each successful jump where both feet landed within the designated area without touching the boundaries or stepping on the wooden beam. Each participant completed two trials, with only the highest score recorded. The assessment demonstrated strong reliability, achieving an intra-class correlation coefficient of 0.84 (Silva et al., 2022). A preliminary familiarization trial was provided for practice purposes but did not count towards the final score. To ensure participants were adequately rested between attempts, a 3-min rest period separated each trial.
Ball throwing velocity
Participants stood behind a designated line positioned 1 meter above the floor, facing a wall located at least 6 meters away in a designated area measuring at least 5 meters by 5 meters (Rodrigues et al., 2019). The objective was to throw a ball against the wall using an overarm motion at maximum speed. Before each throw, participants were allowed one or two preparatory steps. A cross measuring 40 cm by 40 cm was placed on the wall at a height of 170 cm to assist with alignment. The type of ball used was a tennis ball (6.5 cm diameter, 57 g weight). Peak ball velocity was measured in meters per second using a shoulder-level radar gun (Pocket radar, Model PR1000-BC, Inc. Santa Rosa, California) positioned near the throwing line facing the wall. This radar gun had previously demonstrated concurrent validity against the Stalker Radar (reference criterion), establishing its reliability and sensitivity for measuring ball velocity during both throwing and kicking activities (Hernández-Belmonte and Sánchez-Pay, 2021). The test achieved a high level of reliability, indicated by an intra-class correlation score of 0.86 (Silva et al., 2022). Participants were permitted two attempts, and the highest score was recorded. A preliminary trial was provided for familiarization purposes but did not contribute to the final score. Between each trial, participants were given a 1-min rest period to minimize fatigue and ensure consistent performance. The average within-participant coefficient of variability for the completed trials was 3.2%.
Ball kicking velocity
Participants positioned themselves beneath a 1-meter line in an area with dimensions of at least 5 meters by 5 meters, ensuring a minimum distance of 6 meters from a wall (Rodrigues et al., 2019). The task involved kicking a soccer ball at maximum velocity against the wall. Before each kick, participants were allowed one or two preparatory balancing steps. The size of the soccer ball was 62 cm circumference and 350 g weight.
Ball velocity was measured in meters per second using a radar gun (Pocket radar, Model PR1000-BC, Inc. Santa Rosa, California) positioned beside the participant’s dominant foot, near the 1-meter line on the floor, and facing the target wall. The test demonstrated very good reliability with an intra-class correlation score of 0.86 (Silva et al., 2022). Participants were allowed two attempts, with only the best score recorded. A preliminary familiarization trial was provided but not included in the final scoring. Between trials, participants had a 1-min rest period to minimize fatigue and ensure consistent performance. The average within-participant coefficient of variability for the completed trials was 4.7%.
Standing long jump
Participants began the test by positioning themselves on a designated starting line and were instructed to perform a maximal bilateral jump, executing a simultaneous take-off and landing with both feet (Rodrigues et al., 2019). The jump spanned over a marked surface, or a measuring tape placed perpendicular to the starting line alongside the jumping area. To aid in accuracy, a flexible scale tape was placed on the floor to mark the landing spot where the back of the heel closest to the starting line touched down. Children were instructed to propel themselves forward, ensuring that any imbalance that occurred would be in the forward direction while keeping their feet planted on the ground. If they lost balance backward, the jump was repeated. The distance in centimeters from the starting line to this point was then recorded as the measurement of the jump. The test demonstrated excellent reliability with an intra-class correlation score of 0.97 (Silva et al., 2022). Participants were given two attempts, and the best result was considered for scoring purposes. A 1-min rest interval was allocated between each trial to ensure optimal performance and minimize any potential fatigue effects. The average within-participant coefficient of variability for the completed trials was 3.8%.
Shuttle run change-of-direction test
Participants began positioned on a 100 cm long and 5 cm wide start line (Rodrigues et al., 2019). Upon hearing the command “Ready and Go,” they sprinted at maximum speed towards another 100 cm long and 5 cm wide line located 10 meters away. The starting position was standardized, with participants consistently using their preferred leg for both starting and changing direction. Initially, participants started in a staggered stance with their preferred leg forward, ensuring this positioning was recorded to maintain consistency across all evaluations. Immediately past this line, two rounded blocks (each 10 cm high and 5 cm in diameter) were placed, spaced 25 cm apart. Participants retrieved one block, sprinted back to the starting line, and placed it beyond the line, disregarding its exact position. They then returned to collect the second block. The test concluded once participants crossed the start/finish line while carrying the second block. Timing was measured using two pairs of photocells positioned at hip height (Wichro, Wireless Race, Chronojump Boscosystem, Spain). Each participant completed two trials, with a 2-min rest interval between them. The test exhibited very good reliability, boasting an intra-class correlation score of 0.86 (Silva et al., 2022). The final score was determined by the best time achieved from the two trials. The average within-participant coefficient of variability for the completed trials was 4.1%.
Secondary outcomes
To mitigate potential influences of physical activity and screen time on the outcomes, we employed a custom survey adapted from the Surveillance of Digital-Media Habits in Early Childhood Questionnaire, focusing on screen media use (Susilowati et al., 2021). Additionally, parents completed a validated physical activity questionnaire specifically designed for preschool children, comprising six items. These surveys were administered to parents during the initial, intermediate, and final evaluations to ensure comprehensive monitoring throughout the study period (Gascón et al., 2013).
Sample size
The initial exploration of the recommended sample size involved examining an effect size (f) of 0.3, aiming for a power of 0.95 with 3 groups and 3 measurements. Using G*Power software (version 3.1.9.6, Universität Düsseldorf, Psychologie – HHU, Düsseldorf, Germany), an estimation for a repeated measures ANOVA with within-between interaction suggested a recommended total sample size of 39 participants.
Randomization
Randomization was achieved by assigning numbers to participants and then randomly allocating them to one of the groups using opaque envelopes, ensuring an equal chance of enrollment in each group. A 1:1 allocation ratio was ensured. The randomization process was conducted manually by a researcher who had no further interaction with the participants during subsequent stages of the study. Group allocation occurred prior to the initial assessment of MC, and no participants changed groups throughout the study. Neither the evaluators nor the children were blinded to the study.
Statistical procedures
Transforming participants’ results in each MCA test into age- and sex-specific normative values (percentiles) was crucial for calculating MCA sub-scales and total scores, as detailed in previous studies (Rodrigues et al., 2021). These studies identified significant developmental changes in the relationships among model components, consistently representing three domains: locomotor, stability, and manipulative sub-scales. Furthermore, recent research (Rodrigues et al., 2022) emphasized the averaging of normative values across age and sex categories for each test to compute MCA sub-scales and total scores. The formula applied for calculating each sub-scale (e.g., locomotor, stability, manipulative) was ((LC test 1 / (LC test 1 + LC test 2)) * P test 1) + ((LC test 2 / (LC test 1 + LC test 2)) * P test 2), where LC denotes loading coefficient and P denotes percentile value. This approach ensured robust evaluation and interpretation of MC across diverse demographic groups.
Descriptive statistics, including mean and standard deviation, were computed initially. The outliers were initially assessed using a Q-Q plot, and no significant deviations were observed. Normality as well as homogeneity were confirmed using Kolmogorov–Smirnov and Levene’s tests, respectively, indicating normality (p > 0.05) and homogeneity (p > 0.05) within the sample. A mixed ANOVA was subsequently conducted, incorporating time (baseline, 3-months, and 6-months) and groups (target games, striking/fielding sports, net/wall games, invasion games, and a CG) to explore potential significant interactions. Effect size was assessed using partial eta squared (), and post-hoc analysis was performed using the Bonferroni test. The standardized effect size for pairwise comparisons was calculated using Cohen’s d, with the magnitude of differences interpreted as follows (Hopkins et al., 2009): 0.0–0.2 indicating trivial effects, 0.2–0.6 indicating small effects, 0.6–1.2 indicating moderate effects, and 1.2–2.0 indicating large effects. Statistical analyses were carried out using SPSS (IBM Corp. Released 2021. IBM SPSS Statistics for Windows, Version 28.0. Armonk, NY: IBM Corp.), with statistical significance set at p < 0.05.
Results
Table 2 presents the anthropometric characteristics (body mass and height) of boys and girls from each sub-group.

Table 2. Descriptive statistics (mean ± standard deviation) of the body mass and height of boys and girls from each sub-group.
Figure 2 shows percentiles (%) of MC across various domains over three evaluation periods. Significant interactions between time and groups were observed in the stability (F = 23.797; p < 0.001; =0.359), locomotor (F = 28.820; p < 0.001; =0.404), manipulative (F = 26.206; p < 0.001; =0.381), and overall MC score (F = 20.012; p < 0.001; =0.320).
No significant differences were found between groups in the baseline in stability (F = 0.244; p = 0.784; =0.006), locomotor (F = 0.289; p = 0.750; =0.007), manipulative (F = 0.319; p = 0.728; =0.007), and MC overall score (F = 0.284; p = 0.754; =0.007). Additionally, no significant differences were found between groups in the intermediate evaluation in stability (F = 0.095; p = 0.910; =0.002), locomotor (F = 1.747; p = 0.180; =0.039), manipulative (F = 2.201; p = 0.117; =0.049), and MC overall score (F = 1.011; p = 0.368; =0.023).
No significant differences were found between groups post-intervention in stability (F = 1.552; p = 0.218; =0.035), and overall MC score (F = 2.474; p = 0.090; =0.055). However, significant differences were observed between groups post-intervention in the locomotor domain (F = 6.220; p = 0.003; =0.128), with the SG showing significantly higher values compared to the CG (mean difference: 17.0%; p = 0.021; d = 0.783), and PCM (mean difference: 19.8%; p = 0.005; d = 0.947). Additionally, significant differences were found between groups post-intervention in the manipulative domain (F = 7.059; p = 0.001; =0.142), with the SG showing significantly higher values compared to the CG (mean difference: 19.3%; p = 0.009; d = 0.845) and PCM (mean difference: 21.4%; p = 0.003; d = 0.998).
The Table 2 shows the descriptive statistics (mean ± standard deviation) of the scores standardized to percentiles obtained by participants in the three groups across the three evaluation periods. Significant interactions between groups and time were observed in jumping sideways (F = 19.478; p < 0.001; =0.314), shifting platforms (F = 14.497; p < 0.001; =0.254), standing long jump (F = 30.522; p < 0.001; =0.418), shuttle run (F = 18.520; p < 0.001; =0.304), throwing velocity (F = 14.255; p < 0.001; =0.251), and kicking velocity (F = 25.714; p < 0.001; =0.377). No significant differences were found between groups in post-intervention performance for jumping sideways (F = 0.797; p = 0.454; =0.018), shifting platforms (F = 2.595; p = 0.081; =0.058), and shuttle run (F = 2.807; p = 0.066; =0.062). However, significant differences were observed in the standing long jump (F = 8.504; p < 0.001; =0.167), throwing velocity (F = 3.731; p = 0.028; =0.081) and kicking velocity (F = 9.205; p < 0.001; =0.178).
Specifically, in the standing long jump, the SG group showed significantly higher values compared to the CG (mean difference: 39.4%; p = 0.006; d = 0.070), although no significant differences were found when comparing SG to PCM (mean difference: 49.5%; p > 0.999; d = 0.060). In throwing velocity, the SG group exhibited significantly higher values compared to the PCM group (mean difference: 33.4%; p = 0.036; d = 0.718). Finally, in kicking velocity, the SG group showed significantly higher values than the CG (mean difference: 41.9%; p = 0.002; d = 1.044) (see Table 3).
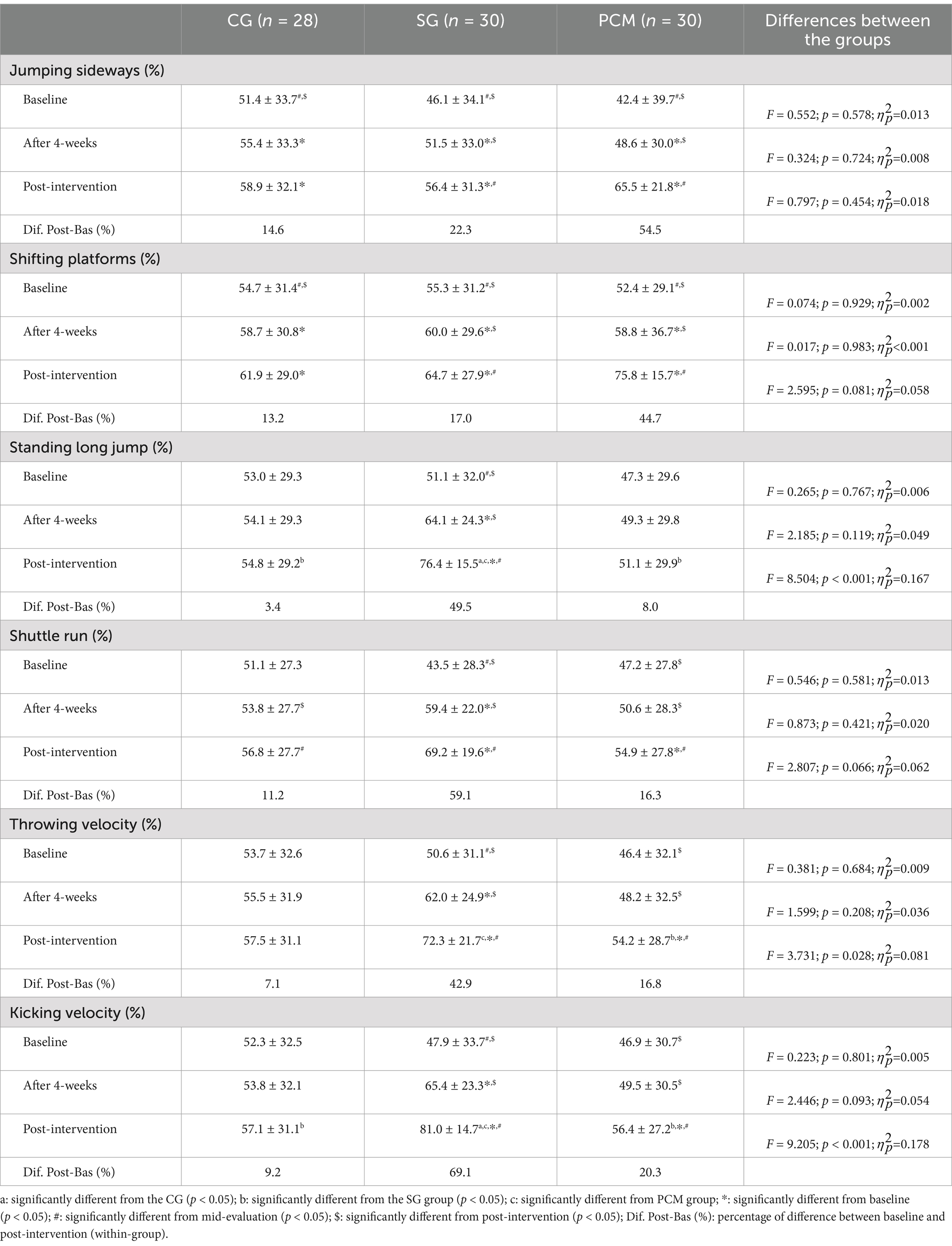
Table 3. Descriptive statistics (mean ± standard deviation) of the motor competence percentiles obtained by participants in the three groups across the three evaluation periods.
Secondary outcomes
Table 4 presents the time spent on digital media during weekdays and the result of the parent questionnaire on physical activity levels in baseline and post-intervention in the CG, SG and PCM groups.
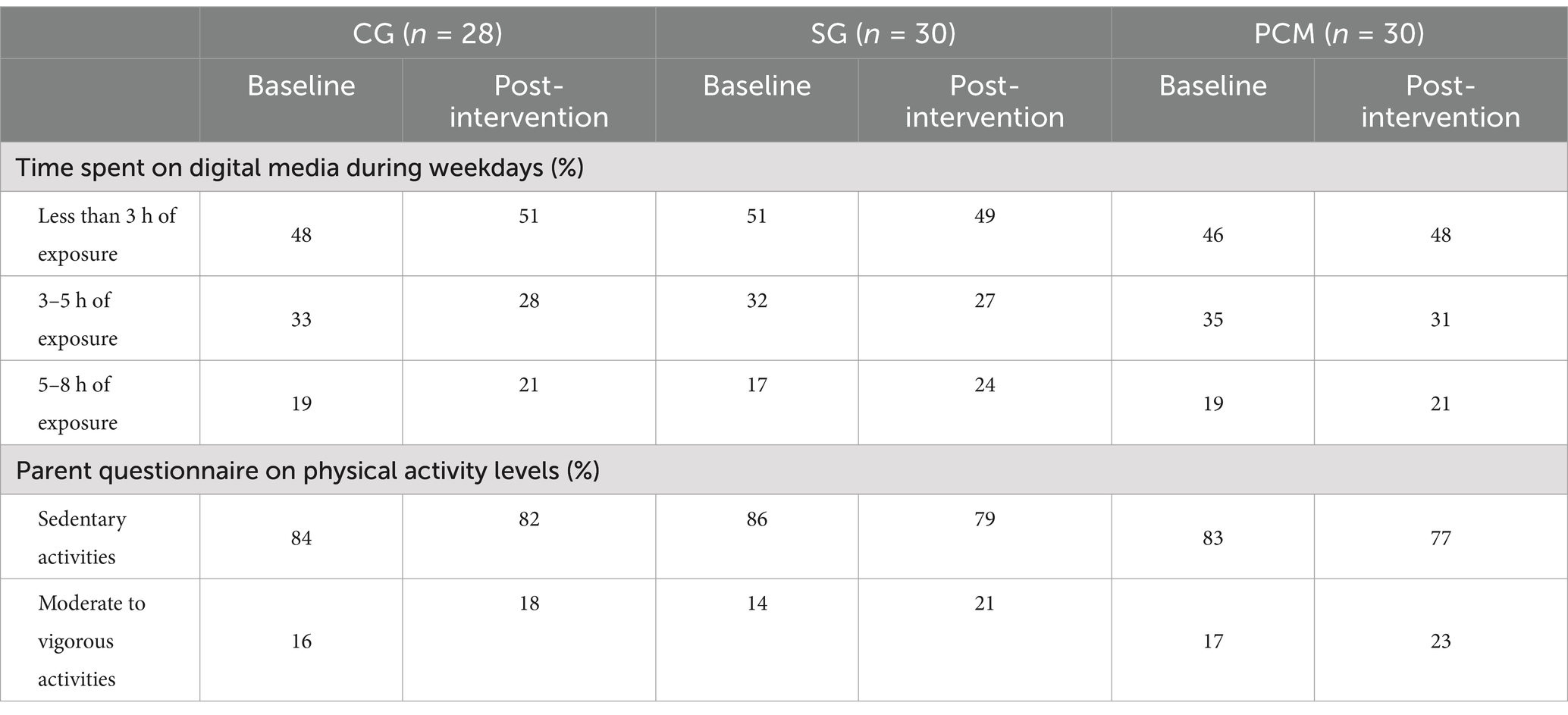
Table 4. Percentage of the time spent on digital media during weekdays and on sedentary and moderate to vigorous activities resulting from the parent questionnaire on physical activity levels in the baseline and post-intervention in each sub-group.
Discussion
The aim of the present study was to evaluate the impacts of structured SG and PCM on the locomotor, stability, and manipulative motor skills of preschool-aged children. Our study’s findings suggest that interventions based on sports games significantly enhanced the main MC domains of locomotor and manipulative skills, resulting in significantly improved scores among children. These interventions were also found to be significantly more effective compared to both the PCM group and the CG. Additionally, it was revealed that neither experimental group (SG and PCM) differed significantly from the CG in terms of stability and overall MC scores after the intervention period.
Our research has showed that children participating in SG, particularly those focusing on mastering ball control and learning team dynamics, as well as targeting through kicking and throwing at specific goals, experienced significant benefits in improving motor skills related to locomotion. These improvements significantly enhanced abilities such as standing long jump and shuttle run. The statistical significance of these improvements was observed after 8 weeks of training in comparison to PCM and CG. Interestingly, no differences were found in the 4th week. Skill acquisition is a dynamic process influenced by the frequency, intensity, and specificity of practice (Smith et al., 1999). By employing various SG drills and drawing on Newell’s constraints model (Newell, 1986), the interaction among individual constraints (e.g., physical capabilities like strength and coordination), task constraints (e.g., specific movement demands of team sports), and environmental constraints (e.g., dynamic and unpredictable game scenarios) likely created opportunities for learners to enhance locomotor capacities such as explosive running power, directly contributing to improvements in tasks like the standing long jump. Additionally, the variability inherent in open drills promoted a broader range of movement patterns and responsiveness, potentially translating into better performance in the shuttle run test.
Our study substantiates specificity principles by indicating that targeted training over an 8-week period led to statistically significant improvements in motor skills, contrasting with the absence of such improvements observed in the initial 4-week phase. Possibly, the initial practice stages focus on cognitive processing and perceptual-motor mapping before transitioning to automaticity through continued training may justify the findings (Ackerman and Cianciolo, 2000). Furthermore, the observed benefits can be attributed to neuroplastic changes within the motor cortex, as repeated engagement in specific motor tasks refines neural pathways responsible for movement coordination and efficiency (Calmels, 2020). Considering the improvements observed in the SG group, they can possibly be attributed to the integration of complex movements such as running, jumping, and directional changes in dynamic, real-time scenarios. These activities emphasize specific motor skills essential for locomotion. This practice may translate into enhanced performance in locomotor assessments, whereas general psychomotor interventions might not offer the necessary task-specific focus required for improving locomotor skills (Jiménez-Díaz et al., 2019). Furthermore, the competition and cooperation promoted by SG may have provided a greater stimulus for improvement, as well as the constant manipulation of objects, such as the ball.
In our experimental study, significant improvements in manipulative MC were observed in the SG experimental group, particularly in ball kicking and throwing velocities. Post-intervention scores showed a significant enhancement compared to both the PCM and control groups. These differences became statistically significant only after 8 weeks, with no differences at the 4-week. This outcome highlights the specific advantages of SG training interventions in developing precise motor skills necessary for manipulating objects in dynamic environments, such as throwing and kicking towards targets (McNeill et al., 2020). SG require participants to engage in specific motor tasks such as kicking and throwing with precision and timing, demanding the integration of sensory feedback and motor planning in dynamic settings (Davids et al., 2000). Moreover, these games can provide children with immediate feedback, allowing them to adapt their behavior and coordination in dynamic settings. Skills learned in contexts closely resembling actual performance conditions (i.e., SG) are more likely to transfer effectively to similar tasks compared to skills acquired through general psychomotor exercises which can explain the improvements in the tests (Soderstrom and Bjork, 2015). Thus, the refinement of motor programs involved in precise manipulative tasks like ball kicking and throwing, potentially explaining the observed improvements in the SG group (Mukherjee et al., 2017). In contrast, general PCM interventions may lack the specificity and intensity needed to elicit similar enhancements in manipulative MC, focusing instead on broader aspects of sensory integration and coordination.
Our study did not find any significant effects of either experimental group (SG and PCM) in enhancing stability MC compared to the CG. SG often prioritize dynamic movements actions such as running, jumping, and rapid changes in direction, which may not directly translate to improvements in stability tasks. In contrast, general PCM interventions typically encompass activities aimed at enhancing overall body awareness and sensorimotor integration, although potentially lacking the specificity required to improve static balance skills specifically (Moschos and Pollatou, 2022). Improvements in stability MC are closely linked to adaptations in proprioceptive feedback mechanisms and neuromuscular coordination (Wong et al., 2012), which may require targeted exercises focusing explicitly on balance and postural control. Indeed, it should be noted that the regulatory mechanisms of postural balance depend on the interaction between the visual, vestibular and proprioceptive systems, which depend on the maturational process (Paillard and Noé, 2015). Nevertheless, from the age of 4, children undergo rapid development related to the maturation of the central nervous system involved in integrating sensory inputs to maintain balance (Venetsanou and Kambas, 2011), for instance, the strengthening of corticospinal connections enhances motor coordination domains such as precision and timing in voluntary movements, critical for tasks requiring dynamic balance and stability. Therefore, the observed lack of significant effects in our study could be a result of a maturated stability system, and/or could also reflect the complexity of assessing stability MC, which may necessitate longer intervention periods or more focused training protocols than those provided in the current study duration. On the other hand, future studies could consider incorporating different exercises, such as children’s yoga or more targeted balance exercises.
While our study suggests the beneficial impact of SG interventions on enhancing locomotor and manipulative MC among children, several limitations should be noted. One limitation is the lack of monitoring the specific dose of exposure to drills and exercises for each participant during practices, making it impossible to quantify the necessary dose magnitude for observing improvements. Additionally, heterogeneity within the group, such as differences in sex or competence levels, can also influence the magnitude of adaptations. Also, MC is a multifaceted concept that can vary depending on the observed abilities, potentially yielding different effects with alternative battery tests. A consensus must be established in the future to define appropriate assessment batteries and standardize key definitions, which will help strengthen and clarify the findings in the field. Therefore, future research should employ multiple assessment batteries and increase sample size and diversity to ensure the generalizability of findings. Finally, an 8-week period may not be sufficient to gain a comprehensive understanding of the long-term effects or to identify potential sensitive periods or plateaus resulting from the programs; therefore, longer intervention durations are necessary.
Despite its limitations, our study is among the few that explore the effects of different intervention approaches on preschool children aimed at enhancing their MC. Experimental studies are crucial, particularly because a significant portion of research in this field consists of observational studies, primarily cross-sectional. Furthermore, it is recommended that interventions of varying durations be implemented to better understand how prolonged exposure may influence group differences. Our results highlight a key message: activities such as SG can significantly enhance locomotor and manipulative competencies. Moreover, they can be particularly beneficial for preschoolers as they may promote the development of social skills through teamwork and communication, enhance emotional regulation by teaching children to manage frustration and celebrate collective successes, and stimulate cognitive growth by encouraging problem-solving and strategic thinking, making them a valuable complement to regular psychomotor teaching classes. However, a more comprehensive range of interventions should include PCM and others that improve stability (e.g., balance training, coordination). Therefore, interventions should always be fitted to the individual needs of children and encompass a wide array of activities to target all dimensions of MC effectively. Moreover, it is important to note that the interventions used are flexible in terms of the equipment required for implementation, making them applicable in a variety of settings. However, all interventions, including SG, must be properly supervised by teachers to ensure that competitiveness does not disrupt the learning environment. Additionally, incorporating more cooperative games can help children enjoy the experience.
Conclusion
In conclusion, our study emphasizes the significant benefits of SG interventions in enhancing children’s MC, particularly in locomotor and manipulative domains. Over an 8-week period, participants engaging in SG activities showed significant improvements in skills such as shuttle run, throwing, and kicking, which translated into enhanced performance in specific motor assessments compared to psychomotor and control groups. These findings highlight the effectiveness of targeted, task-specific training in refining motor skills. However, enhancing stability remains a different challenge, as none of the experimental interventions showed effective in improving this competency.
Data availability statement
The raw data supporting the conclusions of this article will be made available by the authors, without undue reservation.
Ethics statement
The research began after approval from Yibin Third People’s Hospital under code YBSDSRMYY-2024-01. The studies were conducted in accordance with the local legislation and institutional requirements. Written informed consent for participation in this study was provided by the participants’ legal guardians/next of kin.
Author contributions
XG: Data curation, Formal analysis, Investigation, Methodology, Validation, Writing – original draft, Writing – review & editing. CL: Investigation, Writing – original draft, Writing – review & editing. ZZ: Investigation, Writing – original draft, Writing – review & editing. AS: Writing – original draft, Writing – review & editing. FC: Conceptualization, Supervision, Validation, Writing – original draft, Writing – review & editing.
Funding
The author(s) declare that no financial support was received for the research, authorship, and/or publication of this article.
Conflict of interest
The authors declare that the research was conducted in the absence of any commercial or financial relationships that could be construed as a potential conflict of interest.
The author(s) declared that they were an editorial board member of Frontiers, at the time of submission. This had no impact on the peer review process and the final decision.
Publisher’s note
All claims expressed in this article are solely those of the authors and do not necessarily represent those of their affiliated organizations, or those of the publisher, the editors and the reviewers. Any product that may be evaluated in this article, or claim that may be made by its manufacturer, is not guaranteed or endorsed by the publisher.
Abbreviations
MCA, motor competence assessment; MC, motor competence; PCM, psychomotor activities; SG, structured sports games.
References
Ackerman, P. L., and Cianciolo, A. T. (2000). Cognitive, perceptual-speed, and psychomotor determinants of individual differences during skill acquisition. J. Exp. Psychol. Appl. 6, 259–290. doi: 10.1037/1076-898X.6.4.259
Bai, M., Lin, N., Yu, J. J., Teng, Z., and Xu, M. (2024). The effect of planned active play on the fundamental movement skills of preschool children. Hum. Mov. Sci. 96:103241. doi: 10.1016/j.humov.2024.103241
Barnett, L. M., Stodden, D., Cohen, K. E., Smith, J. J., Lubans, D. R., Lenoir, M., et al. (2016). Fundamental movement skills: an important focus. J. Teach. Phys. Educ. 35, 219–225. doi: 10.1123/jtpe.2014-0209
Biino, V., Tinagli, V., Borioni, F., and Pesce, C. (2023). Cognitively enriched physical activity may foster motor competence and executive function as early as preschool age: a pilot trial. Phys. Educ. Sport Pedagog. 28, 425–443. doi: 10.1080/17408989.2021.1990249
Calmels, C. (2020). Neural correlates of motor expertise: extensive motor training and cortical changes. Brain Res. 1739:146323. doi: 10.1016/j.brainres.2019.146323
Cantell, M., Crawford, S. G., and (Tish), P. K. (2008). Physical fitness and health indices in children, adolescents and adults with high or low motor competence. Hum. Mov. Sci. 27, 344–362. doi: 10.1016/j.humov.2008.02.007
Cattuzzo, M. T., dos, R., Ré, A. H., de, I., Melo, B., de, M., et al. (2016). Motor competence and health related physical fitness in youth: a systematic review. J. Sci. Med. Sport 19, 123–129. doi: 10.1016/j.jsams.2014.12.004
Davids, K., Lees, A., and Burwitz, L. (2000). Understanding and measuring coordination and control in kicking skills in soccer: implications for talent identification and skill acquisition. J. Sports Sci. 18, 703–714. doi: 10.1080/02640410050120087
Doyon, J., Bellec, P., Amsel, R., Penhune, V., Monchi, O., Carrier, J., et al. (2009). Contributions of the basal ganglia and functionally related brain structures to motor learning. Behav. Brain Res. 199, 61–75. doi: 10.1016/j.bbr.2008.11.012
Ene, M. I., Iconomescu, T.-M., Talaghir, L.-G., and Neofit, A. (2016). Developing spatial and body Schema orientation in preschoolers and primary school through physical activities. Int. J. Educ. Sci. 15, 27–33. doi: 10.1080/09751122.2016.11890510
Feitoza, A. H. P., Santos, A. B. D., Barnett, L. M., and Cattuzzo, M. T. (2022). Motor competence, physical activity, and perceived motor competence: a relational systems approach. J. Sports Sci. 40, 2371–2383. doi: 10.1080/02640414.2022.2158268
Ferguson, K. T., Cassells, R. C., MacAllister, J. W., and Evans, G. W. (2013). The physical environment and child development: an international review. Int. J. Psychol. 48, 437–468. doi: 10.1080/00207594.2013.804190
Fernandes, V. R., Ribeiro, M. L. S., Melo, T., de Tarso Maciel-Pinheiro, P., Guimarães, T. T., Araújo, N. B., et al. (2016). Motor coordination correlates with academic achievement and cognitive function in children. Front. Psychol. 7:318. doi: 10.3389/fpsyg.2016.00318
Gallahue, D., and Ozmun, J. (2006). Understanding motor development: Infants, children, adolescents, adults. 6th Edn. Boston: McGraw-Hill.
Gascón, M. B., Rojas, C. R., Lopez, G. W., Crawford, P., and Cruz, A. J. (2013). Assessing the validity of a physical activity questionnaire developed for parents of preschool children in Mexico. J. Health Popul. Nutr. 30, 439–446. doi: 10.3329/jhpn.v30i4.13327
Gerván, P., Soltész, P., Filep, O., Berencsi, A., and Kovács, I. (2017). Posterior-anterior brain maturation reflected in perceptual, motor and cognitive performance. Front. Psychol. 8:674. doi: 10.3389/fpsyg.2017.00674
Goodway, J. D., and Branta, C. F. (2003). Influence of a motor skill intervention on fundamental motor skill development of disadvantaged preschool children. Res. Q. Exerc. Sport 74, 36–46. doi: 10.1080/02701367.2003.10609062
Hashemi, M., Khameneh, N. N., and Salehian, M. H. (2015). Effect of selected games on the development of manipulative skills in 4-6 year-old preschool girls. Med. Sport 68, 49–55.
Hernández-Belmonte, A., and Sánchez-Pay, A. (2021). Concurrent validity, inter-unit reliability and biological variability of a low-cost pocket radar for ball velocity measurement in soccer and tennis. J. Sports Sci. 39, 1312–1319. doi: 10.1080/02640414.2020.1868090
Hoffmann, B., Kobel, S., Wartha, O., Kettner, S., Dreyhaupt, J., and Steinacker, J. M. (2019). High sedentary time in children is not only due to screen media use: a cross-sectional study. BMC Pediatr. 19:154. doi: 10.1186/s12887-019-1521-8
Hopkins, W. G., Marshall, S. W., Batterham, A. M., and Hanin, J. (2009). Progressive statistics for studies in sports medicine and exercise science. Med. Sci. Sports Exerc. 41, 3–12. doi: 10.1249/MSS.0b013e31818cb278
Jiménez-Díaz, J., Chaves-Castro, K., and Salazar, W. (2019). Effects of different movement programs on motor competence: a systematic review with Meta-analysis. J. Phys. Act. Health 16, 657–666. doi: 10.1123/jpah.2018-0179
Kolehmainen, N., Ramsay, C., McKee, L., Missiuna, C., Owen, C., and Francis, J. (2015). Participation in physical play and leisure in children with motor impairments: mixed-methods study to generate evidence for developing an intervention. Phys. Ther. 95, 1374–1386. doi: 10.2522/ptj.20140404
Konoh, O. E. (2013). Integrated use of sports games in physical education of preschool children. Pedag. Psychol. Med. Biol. Probl. Phys. Train. Sports 17, 27–30.
Lorås, H. (2020). The effects of physical education on motor competence in children and adolescents: a systematic review and Meta-analysis. Sports 8:88. doi: 10.3390/sports8060088
Luz, C., Rodrigues, L. P., Almeida, G., and Cordovil, R. (2016). Development and validation of a model of motor competence in children and adolescents. J. Sci. Med. Sport 19, 568–572. doi: 10.1016/j.jsams.2015.07.005
McNeill, E., Toth, A. J., Harrison, A. J., and Campbell, M. J. (2020). Cognitive to physical performance: a conceptual model for the role of motor simulation in performance. Int. Rev. Sport Exerc. Psychol. 13, 205–230. doi: 10.1080/1750984X.2019.1689573
Merkow, R. P., Kaji, A. H., and Itani, K. M. F. (2021). The CONSORT framework. JAMA Surg. 156, 877–878. doi: 10.1001/jamasurg.2021.0549
Moschos, G., and Pollatou, E. (2022). The effect of a psychomotor intervention program in children 3–10 years of age: a systematic review. Body Mov. Dance Psychother. 17, 294–309. doi: 10.1080/17432979.2022.2078406
Mukherjee, S., Ting Jamie, L. C., and Fong, L. H. (2017). Fundamental motor skill proficiency of 6- to 9-year-old Singaporean children. Percept. Mot. Skills 124, 584–600. doi: 10.1177/0031512517703005
Musálek, M., Sedlak, P., Dvořáková, H., Vážná, A., Novák, J., Kokštejn, J., et al. (2021). Insufficient physical fitness and deficits in basic eating habits in Normal-weight obese children are apparent from pre-school age or sooner. Nutrients 13:3464. doi: 10.3390/nu13103464
Myer, G. D., Faigenbaum, A. D., Edwards, N. M., Clark, J. F., Best, T. M., and Sallis, R. E. (2015). Sixty minutes of what? A developing brain perspective for activating children with an integrative exercise approach. Br. J. Sports Med. 49, 1510–1516. doi: 10.1136/bjsports-2014-093661
Newell, K. M. (1986). “Constraints on the development of coordination” in Motor development in children: Aspects of coordination and control. ed. M. G. Wade (Boston: Martinus Nijhoff), 341–360.
Paillard, T., and Noé, F. (2015). Techniques and methods for testing the postural function in healthy and pathological subjects. Biomed. Res. Int. 2015, 1–15. doi: 10.1155/2015/891390
Pesce, C., Croce, R., Ben-Soussan, T. D., Vazou, S., McCullick, B., Tomporowski, P. D., et al. (2019). Variability of practice as an interface between motor and cognitive development. Int. J. Sport Exerc. Psychol. 17, 133–152. doi: 10.1080/1612197X.2016.1223421
Petrusevski, C., Morgan, A., MacDermid, J., Wilson, M., and Richardson, J. (2022). Framing physical literacy for aging adults: an integrative review. Disabil. Rehabil. 44, 8149–8160. doi: 10.1080/09638288.2021.2012841
Robinson, L. E., Stodden, D. F., Barnett, L. M., Lopes, V. P., Logan, S. W., Rodrigues, L. P., et al. (2015). Motor competence and its effect on positive developmental trajectories of health. Sports Med. 45, 1273–1284. doi: 10.1007/s40279-015-0351-6
Rodrigues, L. P., Cordovil, R., Luz, C., and Lopes, V. P. (2021). Model invariance of the motor competence assessment (MCA) from early childhood to young adulthood. J. Sports Sci. 39, 2353–2360. doi: 10.1080/02640414.2021.1932290
Rodrigues, L. P., Luz, C., Cordovil, R., Bezerra, P., Silva, B., Camões, M., et al. (2019). Normative values of the motor competence assessment (MCA) from 3 to 23 years of age. J. Sci. Med. Sport 22, 1038–1043. doi: 10.1016/j.jsams.2019.05.009
Rodrigues, L. P., Luz, C., Cordovil, R., Pombo, A., and Lopes, V. P. (2022). Motor competence assessment (MCA) scoring method. Children 9:1769. doi: 10.3390/children9111769
Ruddy, K. L., and Carson, R. G. (2013). Neural pathways mediating cross education of motor function. Front. Hum. Neurosci. 7:397. doi: 10.3389/fnhum.2013.00397
Saraiva, F., Paschoal, D., Willig, R. M., Reyes, A. C., and Silva, A. F. (2024). Mastering movement: a cross-sectional investigation of motor competence in children and adolescents engaged in sports. PLoS One 19:e0304524. doi: 10.1371/journal.pone.0304524
Sedlak, P., Pařízková, J., Daniš, R., Dvořáková, H., and Vignerová, J. (2015). Secular changes of adiposity and motor development in Czech preschool children: lifestyle changes in fifty-five year retrospective study. Biomed. Res. Int. 2015, 1–9. doi: 10.1155/2015/823841
Sigmundsson, H., and Haga, M. (2016). Motor competence is associated with physical fitness in four- to six-year-old preschool children. Eur. Early Child. Educ. Res. J. 24, 477–488. doi: 10.1080/1350293X.2016.1164411
Silva, A. F., Nobari, H., Badicu, G., Ceylan, H. I., Lima, R., Lagoa, M. J., et al. (2022). Reliability levels of motor competence in youth athletes. BMC Pediatr. 22:430. doi: 10.1186/s12887-022-03483-z
Smith, M. E., McEvoy, L. K., and Gevins, A. (1999). Neurophysiological indices of strategy development and skill acquisition. Cogn. Brain Res. 7, 389–404. doi: 10.1016/S0926-6410(98)00043-3
Soderstrom, N. C., and Bjork, R. A. (2015). Learning versus performance. Perspect. Psychol. Sci. 10, 176–199. doi: 10.1177/1745691615569000
Sternad, D. (2018). It’s not (only) the mean that matters: variability, noise and exploration in skill learning. Curr. Opin. Behav. Sci. 20, 183–195. doi: 10.1016/j.cobeha.2018.01.004
Stodden, D. F., Goodway, J. D., Langendorfer, S. J., Roberton, M. A., Rudisill, M. E., Garcia, C., et al. (2008). A developmental perspective on the role of motor skill competence in physical activity: an emergent relationship. Quest 60, 290–306. doi: 10.1080/00336297.2008.10483582
Susilowati, I. H., Nugraha, S., Alimoeso, S., and Hasiholan, B. P. (2021). Screen time for preschool children: learning from home during the COVID-19 pandemic. Glob. Pediatr. Health 8:2333794X2110178. doi: 10.1177/2333794X211017836
Sutapa, P., Pratama, K. W., Rosly, M. M., Ali, S. K. S., and Karakauki, M. (2021). Improving motor skills in early childhood through goal-oriented play activity. Children 8:994. doi: 10.3390/children8110994
Teixeira Costa, H. J., Abelairas-Gomez, C., Arufe-Giráldez, V., Pazos-Couto, J. M., and Barcala-Furelos, R. (2015). Influence of a physical education plan on psychomotor development profiles of preschool children. J. Hum. Sport Exerc. 10:11. doi: 10.14198/jhse.2015.101.11
Thomason, M. E., Hect, J., Waller, R., Manning, J. H., Stacks, A. M., Beeghly, M., et al. (2018). Prenatal neural origins of infant motor development: associations between fetal brain and infant motor development. Dev. Psychopathol. 30, 763–772. doi: 10.1017/S095457941800072X
True, L., Pfeiffer, K. A., Dowda, M., Williams, H. G., Brown, W. H., O’Neill, J. R., et al. (2017). Motor competence and characteristics within the preschool environment. J. Sci. Med. Sport 20, 751–755. doi: 10.1016/j.jsams.2016.11.019
van, A., Broderick, C. R., van, N., Ward, R. E., and Parmenter, B. (2017). Interventions to improve fundamental motor skills in pre-school aged children: a systematic review and meta-analysis. J. Sci. Med. Sport 20, 658–666. doi: 10.1016/j.jsams.2016.11.008
Venetsanou, F., and Kambas, A. (2011). The effects of age and gender on balance skills in preschool children. Facta Univ. Phys. Educ. Sport 9, 81–90.
Vlahov, E., Baghurst, T. M., and Mwavita, M. (2014). Preschool motor development predicting high school health-related physical fitness: a prospective study. Percept. Mot. Skills 119, 279–291. doi: 10.2466/10.25.PMS.119c16z8
Whitehead, M. (2001). The concept of physical literacy. Eur. J. Phys. Educ. 6, 127–138. doi: 10.1080/1740898010060205
Williams, H. G., Pfeiffer, K. A., O’Neill, J. R., Dowda, M., McIver, K. L., Brown, W. H., et al. (2008). Motor skill performance and physical activity in preschool children. Obesity 16, 1421–1426. doi: 10.1038/oby.2008.214
Wong, J. D., Kistemaker, D. A., Chin, A., and Gribble, P. L. (2012). Can proprioceptive training improve motor learning? J. Neurophysiol. 108, 3313–3321. doi: 10.1152/jn.00122.2012
Wrotniak, B. H., Epstein, L. H., Dorn, J. M., Jones, K. E., and Kondilis, V. A. (2006). The relationship between motor proficiency and physical activity in children. Pediatrics 118, e1758–e1765. doi: 10.1542/peds.2006-0742
Keywords: motor competence, motor development, child, physical exercise, motor skills
Citation: Guo X, Li C, Zhang Z, Silva AF and Clemente FM (2024) Can motor competence be influenced by the type of training interventions preschool children are exposed to? A randomized experimental study comparing sports games and psychomotricity activities. Front. Psychol. 15:1476297. doi: 10.3389/fpsyg.2024.1476297
Edited by:
Vânia Loureiro, Polytechnic Institute of Beja, PortugalReviewed by:
María Antonia Parra Rizo, Miguel Hernández University of Elche, SpainEduardo Matta Mello Portugal, Brazilian Institute of Medicine and Rehabilitation (IBMR), Brazil
Agustín Aibar-Almazán, University of Jaén, Spain
Mario Mauro, University of Bologna, Italy
Copyright © 2024 Guo, Li, Zhang, Silva and Clemente. This is an open-access article distributed under the terms of the Creative Commons Attribution License (CC BY). The use, distribution or reproduction in other forums is permitted, provided the original author(s) and the copyright owner(s) are credited and that the original publication in this journal is cited, in accordance with accepted academic practice. No use, distribution or reproduction is permitted which does not comply with these terms.
*Correspondence: Xiaodan Guo, eGlhb2Rhbi5ndW9AYXdmLmdkYS5wbA==Filipe Manuel Clemente, ZmlsaXBlLmNsZW1lbnRlNUBnbWFpbC5jb20=