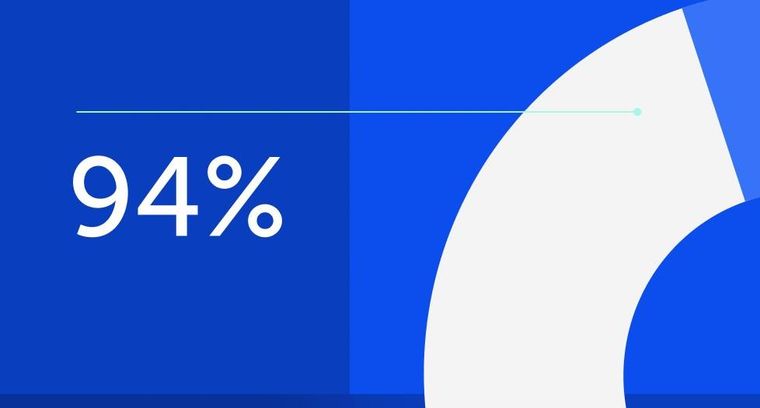
94% of researchers rate our articles as excellent or good
Learn more about the work of our research integrity team to safeguard the quality of each article we publish.
Find out more
REVIEW article
Front. Psychol., 11 December 2024
Sec. Developmental Psychology
Volume 15 - 2024 | https://doi.org/10.3389/fpsyg.2024.1474961
Infants are exposed to a myriad of sounds early in life, including caregivers' speech, songs, human-made and natural (non-anthropogenic) environmental sounds. While decades of research have established that infants have sophisticated perceptual abilities to process speech, less is known about how they perceive natural environmental sounds. This review synthesizes current findings about the perception of natural environmental sounds in the first years of life, emphasizing their role in auditory development and describing how these studies contribute to the emerging field of human auditory ecology. Some of the existing studies explore infants' responses to animal vocalizations and water sounds. Infants demonstrate an initial broad sensitivity to primate vocalizations, which narrows to human speech through experience. They also show early recognition of water sounds, with preferences for natural over artificial water sounds already at birth, indicating an evolutionary ancient sensitivity. However, this ability undergoes refinement with age and experience. The few studies available suggest that infants' auditory processing of natural sounds is complex and influenced by both genetic predispositions and exposure. Building on these existing results, this review highlights the need for ecologically valid experimental paradigms that better represent the natural auditory environments humans evolved in. Understanding how children process natural soundscapes not only deepens our understanding of auditory development but also offers practical insights for advancing environmental awareness, improving auditory interventions for children with hearing loss, and promoting wellbeing through exposure to natural sounds.
Infants encounter a myriad of sounds early in life. Their caregivers' speech, songs heard at daycare, the family dog's barking, leaves rattling in the park, and birds singing are all part of the earliest human experiences. Decades of research have shown that young infants have sophisticated perceptual abilities to process speech, laying the foundations for language acquisition (Nallet and Gervain, 2021; Werker, 2018). Children's sensitivity to music is also beginning to be understood (Trehub and Hannon, 2006; Winkler et al., 2009; Trainor and Unrau, 2011). Much less is known about how infants perceive sounds that are not generated by humans, in particular how they perceive natural environmental sounds and soundscapes. Yet, understanding how infants process natural auditory signals is fundamental to the study of auditory development, and human development more generally (Cummings et al., 2009).
Further, this endeavor is central to the development of a new field called “human auditory ecology,” the scientific study of human beings' ability to perceive the ecological processes at work in natural habitats (Lorenzi et al., 2023). For many non-human species, being able to detect, discriminate, identify, and orient toward natural sounds such as animal vocalizations, and geophysical sounds (i.e., wind, rain or a stream of water) determines survival and reproduction through the ability to represent and monitor the immediate acoustic environment. A fundamental question of this novel field of research is, therefore, whether these auditory abilities and underlying mechanisms operate throughout the life span or whether they emerge through exposure, learning and cultural transmission. Urban habitats and spoken language are relatively recent in humanity's history and evolution. By contrast, natural soundscapes—the complex arrangements of animal vocalizations and geophysical sounds as shaped by sound propagation characteristics of natural settings such as forests or savannahs (Grinfeder et al., 2022)—have preceded the appearance of Homo sapiens 300,000 years ago (Senter, 2008).
Natural and urban soundscapes differ in many ways. Figure 1 illustrates some of the spectro-temporal differences between natural soundscapes recorded in protected nature reserves (specifically, forests, savannah and desert) and common urban soundscapes (specifically, street traffic and crowd in a restaurant). Figure 1 shows the modulation power spectra of single acoustic samples of natural vs. urban soundscapes selected from our database (Singh and Theunissen, 2003, see also the Supplementary Appendix). Additional analyses (average modulation power spectra calculated over a larger corpus of acoustic samples) are presented in Supplementary Figure 6 of the Appendix. For instance, Figure 1 reveals that unlike urban soundscapes, the soundscapes recorded in the desert, tropical forest and savannah show greater modulation power for relatively fast temporal modulation and for relatively high spectral modulation, reflecting the rapid, periodic trills and harmonic structure of insect stridulations and bird vocalizations, respectively.
Figure 1. Modulation power spectra (MPS) of natural versus urban soundscapes. MPS shows how modulation power varies as a function of spectral-modulation (ordinate) and temporal-modulation (abscissa) rate (see Singh and Theunissen, 2003 for more information about MPS analysis). These representations highlight the spectral and temporal structure in the spectrogram of sounds (Theunissen and Elie, 2014). MPS were computed on single acoustic recordings conducted in closed and open terrestrial natural habitats (boreal, temperate and tropical forests, a savannah and a desert), and in two typical indoor and outdoor urban settings (street traffic and crowd). Each MPS is normalized by its own maximum modulation power. Sources: B. Krause, Wild Sanctuary (natural soundscapes); S. Meunier, LMA, CNRS, and royalty free sound library SoundBible (urban soundscapes). See Supplementary Appendix for additional information about the stimuli.
It is reasonable to assume that auditory mechanisms involved in the passive and active monitoring of natural sounds and soundscapes have an ancestral origin shared with many non-human species equipped with tympanic ears, predating the occurrence of spoken language and “cocktail party” situations. These ancestral mechanisms may be optimized through evolution for spectro-temporal cues quite different from those typically found in more recent urban settings. Furthermore, it is also reasonable to assume that exposure, learning and expertise shape our ability to monitor natural sounds and soundscapes. Consistent with this idea, expert listeners have been found to adopt a more analytical listening strategy prioritizing precision, whereas non experts attend to soundscapes in a more holistic way (Guastavino, 2003). It follows that developmental studies exploring infants and children's ability to perceive animal vocalizations and geophysical sounds should help build theoretically more solid foundations for human auditory ecology by clarifying the factors responsible for our ability to build a clear sense of place and time through our ears and auditory brain (Gervain and Mehler, 2010; Lickliter and Witherington, 2017; Oyama, 1979; Reh et al., 2020; Werker and Hensch, 2015).
From birth, infants are exposed to many different natural sounds such as rain, thunder, rustling leaves, streams of water and birds chirping. What are the acoustic properties of these sounds? Figure 2 illustrates the spectro-temporal similarities and differences between natural sounds such as bird vocalizations, insect stridulations, primate vocalizations and streams and speech sounds from a variety of languages. Similarly to Figure 1, Figure 2 shows modulation power spectra of single acoustic samples of natural vs. speech sounds selected from our database. Additional analyses (average modulation power spectra and modulation statistics calculated over a larger corpus of acoustic samples) are presented in Supplementary Figure 7 of the Appendix. Consistent with Singh and Theunissen's (2003) canonical study, natural sounds are lowpass in shape: they show most of their modulation power for low temporal and spectral modulations. Speech sounds and to a some extent primate vocalizations and some bird songs show more spectral modulation power at relatively high spectral modulations, indicating the presence of fine-grained harmonic structure. Insect sounds do not show this spectral feature, but have more modulation power at relatively high temporal modulations due to fast, periodic stridulations/timbalations. Some bird songs also show this temporal feature, presumably caused by fast trills. Streams of water show none of these spectro-temporal features, they are more similar to broadband noise.
Figure 2. Modulation power spectra (MPS) of natural sounds (bird vocalizations, primate vocalization, insect stridulation and water sounds) and speech sounds. Natural sounds: (i) Bird songs: single recordings from eight bird species selected from a protected European cold forest in the East of France (the Risoux forest in France). Sources: J.-C. Roché & MNHN; (ii) Primate vocalization: single recording of a baboon “wahoo” vocalization. Source: Gemignani and Gervain (2024); (iii) Insect stridulation: single recording of Tettigonia viridissima, the great green bush-cricket inhabiting the Risoux forest. Source: J. Sueur, MNHN; (iv) Water sounds: single recordings of a single headwater forest stream with distinct water temperatures and discharge rates (here, a slow versus a fast discharge). Source: Klaus et al. (2019). Speech sounds: single sentences recorded in ten different languages from a female speaker: Basque, Dutch, English, French, Japanese, Marathi, Polish, Spanish, Turkish, and Zulu (1 recording per language). Source: Ramus et al. (1999). Each MPS is normalized by its own maximum modulation power. See Supplementary Appendix for additional information about the stimuli.
Does the perception of natural sounds—especially (non-human) animal sounds—rely on neural mechanisms shared with speech processing (Gervain et al., 2014; Vouloumanos et al., 2010) or are they distinct? The efficient neural coding hypothesis suggests that the mammalian sensory system evolved to encode sensory information optimally (Simoncelli and Olshausen, 2001). Thus, our perceptual systems are optimized for natural stimuli (Gervain et al., 2014), and language evolved leveraging the capabilities of these systems (Lewicki, 2002; Smith and Lewicki, 2006). This account implies that speech perception and the perception of natural sounds have shared underlying neural representations. By contrast, speech has been argued to be “special,” since it is our species-specific communicative signal, and as such a sound that our vocal tract can produce. This link with the motor system and the auditory feedback loop distinguishes speech from other sounds, which we can only perceive, but not produce (Liberman and Mattingly, 1985). Despite these theoretical debates, studies are only now starting to explore how we perceive natural sounds, in particular in their full ecological complexity, e.g. natural soundscapes (Lorenzi et al., 2023).
Investigating development is highly relevant to these theoretical questions, as the similarities and differences between the developmental trajectories of speech perception and natural sound perception abilities can shed light on whether or to what extent they share underlying mechanisms. Further, infants and young children often have limited experience of some of these sounds categories. It is thus easier to determine what auditory sensitivities are biologically endowed, possibly shaped by our evolutionary history, and which ones require experience to emerge. To date, however, only a few studies have tested the perception of natural soundscapes in developmental research, each with distinct research objectives.
Many animal species communicate with auditory signals. Of these, two groups have received particular attention in the study of children's perceptual sensitivities: primates, our closest phylogenetic relatives, who have vocal tracts at least somewhat comparable to our, and birds, some of which produce particularly complex, elaborate, acoustically rich and at least to some extent combinatorially productive vocalizations.
Many studies have focused on how at birth and in the first months of life infants process the vocalizations of primates. Vocalizations are a salient signal from birth onward and share evolutionary significance across species (Cristia et al., 2014). Interestingly, a significant number of studies reported no selective processing for human speech compared to primate vocalizations from birth up to 3–4 months of life, despite maintaining behavioral listening preferences for vocalizations of biological origin over artificial sounds (Cristia et al., 2014; Ferry et al., 2013; Perszyk and Waxman, 2016; Vouloumanos et al., 2010; Shultz and Vouloumanos, 2010). This broad preference suggests that similar neural processes might be involved in the early perception of both human and non-human vocalizations, at least within the more general primate category (Perszyk and Waxman, 2016). Indeed, since speech and non-human primate vocalizations share certain acoustic properties such as a harmonic structure (Figure 2), given the similarities between humans and other primates' vocal tracts (Altmann et al., 2007; Smith and Lewicki, 2006), newborns may be inherently drawn to harmonically rich sounds with spectral and temporal irregularities (Belin, 2006). The same reasoning applies to the temporal structure of speech and primate vocalizations, which are both characterized by relatively slow amplitude-modulation, i.e., temporal envelope patterns reflecting neural and motor constraints on articulatory processes (Figure 2 and Supplementary Figure 7).
This broad initial sensitivity to primate vocalizations (Vouloumanos et al., 2010) may then be sharpened into more specific preferences for speech by early experience between 4 and 6 months (Scott et al., 2007; Vouloumanos et al., 2010; Perszyk and Waxman, 2016). Indeed, one study examining neural responses in 4-month-old infants found that primate vocalizations and speech activated similar brain regions. However, speech triggered stronger activity in the left hemisphere, while monkey vocalizations elicited greater activity in the right hemisphere (Minagawa-Kawai et al., 2011). This aligns with the maturation of auditory cortices in the first months of life (Polver et al., 2023). However, further studies are necessary to understand the neural underpinnings of these processes.
Some studies also looked at infants' sensitivity to bird songs. Bird vocalizations are the most frequent biotic components of natural soundscapes (Lorenzi et al., 2023) and convey salient spectro-temporal cues that make them easy to distinguish from other animal acoustic productions such as insect stridulations or primate vocalizations (Figure 2 and Supplementary Figure 7; see also Catchpole and Slater, 2008; Fay and Popper, 1994; Hoy et al., 1998 for reviews). One study investigated whether infants could behaviorally distinguish between repetitive, low-frequency sounds made by sea birds and melodious, high-frequency songs of garden birds (Lange-Küttner, 2010). The study hypothesized that infants might be more responsive to the low-frequency sea bird sounds, which fall within the frequency range of the human voice, possibly due to the relative immaturity of their auditory systems (Lange-Küttner, 2010). Infants were recruited from Aberdeen, Scotland, a harbor town where sea birds are common. The participants included 5- to 7-month-old infants, 10- to 12-month-old infants, and Scottish undergraduate students (Lange-Küttner, 2010). Infants showed a preference for sea-bird sounds, whereas adults preferred garden-bird songs. Older infants (10- to 12-month-olds) were in between, as they began to show increased preferential looking times to garden-bird songs, though they still preferred sea-bird sounds (Lange-Küttner, 2010). To determine if familiarity with sea-bird sounds influenced these results, a follow-up experiment was conducted in central Europe (Leipzig, Germany) with 4–5- and 6–8-month-old infants as well as in London with adults from diverse ethnic backgrounds. In these locations, sea birds are not part of the natural habitat. Infants still preferred sea-bird sounds, while adults preferred garden-bird songs, suggesting that the preference for sea-bird sounds in infants might be a universal disposition rather than a result of local exposure (Lange-Küttner, 2010). In this study, it was also tested whether individual bird calls influence preference within bird categories by investigating if certain exemplars have a greater impact on looking behavior. If individual exemplars strongly drive preference due to their specific acoustic characteristics, stronger within-category preferences would be observed. Conversely, if attention is evenly distributed among exemplars, indicating a representation of the category, evenly distributed preferences between seabird and garden bird categories would be expected. German infants showed fewer within-category preferences for both sea-bird sounds and garden-bird songs and more between-category preferences than Scottish infants. This suggests that the local environment may still shape universal biases, as greater exposure to sea-bird sounds might have facilitated early perceptual categorization in the Scottish infants (Lange-Küttner, 2010). These findings highlight the need for studies conducted in different settings, e.g. rural, wild or urban, to explore the effects of exposure and experience (Lorenzi et al., 2023).
Another study compared infants' responses to bird songs and speech in an unfamiliar language (Santolin et al., 2019). The study examined 4-month-olds' looking preferences for bird song (sung by a European starling) compared to sentences in Mandarin Chinese that either maintained normal prosodic features (Forward condition) or violated them (Backward condition), using an infant-controlled looking time preference procedure (Santolin et al., 2019). The findings showed that infants preferred bird songs over backward speech but did not exhibit a preference between forward speech and bird songs. This suggests that infants are drawn to naturally produced sounds, whether human or non-human, as reliable sources for learning (Santolin et al., 2019; Ravignani et al., 2019).
Currently, there are no studies, to our knowledge, that have examined the neural mechanisms of children's perception of bird song and other non-mammalian vocalizations. This underscores the need for further research in this area.
Studies investigating how children process non-biological natural sounds are particularly limited. Of this sound category, essentially only water sounds have received any attention so far. This is not surprising as water holds a unique significance within natural environments due to its fundamental role for survival (Lorenzi et al., 2023). Water also has unique acoustic properties (Geffen et al., 2011; Guyot et al., 2017; McDermott et al., 2009). Water sounds belong to the broad category of “textures,” that is quasi-stationary sounds resulting from the superimposition of many independent sound sources (i.e. bubbles). Sound textures are assumed to be perceived through a temporal integration process discarding acoustic details and keeping only summary statistics (McDermott et al., 2009).
Water sounds are among the first auditory stimuli encountered by infants, making them inherently familiar (Gervain et al., 2014). Two studies (Gervain et al., 2014, 2016) thus investigated how water sounds are processed across development. In both studies, a generative model with a small set of parameters was used to generate sounds of running water (Geffen et al., 2011). Specifically, the parameters of the model were set in such a way that the resulting sounds were either scale-invariant, i.e. did not have any privileged temporal scale, characteristic of many natural sounds, or they were variable scale (Figure 3; Geffen et al., 2011). Figure 4 shows that texture statistics computed by a model of the human auditory system differ—sometimes substantially as in the case of statistics estimating temporal envelope sparsity—across the scale-invariant and variable-scale synthetic water sounds used by Gervain et al. (2014, 2016).
Figure 3. The generative model of water sounds used in Geffen et al. (2011) and Gervain et al. (2014). The model generates sounds using a population of gammatone chirps, each defined by its frequency, amplitude and cycle constant of decay. These parameters can be set such that the chirps are (i) scale invariant (upper inset), i.e. the cycle constant of decay is fixed and therefore the shape of the chirp is constant, frequency is inversely proportional to duration, or (ii) variable scale (lower inset), i.e. duration is held constant, and independent of frequency, therefore the shape of the chirp changes.
Figure 4. Summary statistics computed by a model of auditory texture perception (McWalter and Dau, 2017) in response to the scale-invariant (“natural,” top panels) and scale-variable (“not-natural,” bottom panels) synthetic water sounds used by Geffen et al. (2011) and Gervain et al. (2014)). Summary statistics mean, variance, skew and kurtosis (ordinate) are shown as a function of cochlear channel (abscissa). Cross-band correlations (right-most panels) are shown for each pair of cochlear channels (the hue value from green to yellow covers the 0–1 range of cross-band correlations). The two synthesized sounds differ substantially in terms of their excitation pattern (the internal power spectrum of sounds) and sparsity in each cochlear channel, with scale-invariant (natural) sounds being sparser than scale-variable (not-natural) sounds. The coordination of the temporal envelopes at the output of the cochlear channels also appears to be somewhat different between the two sounds. See Supplementary Appendix for additional information about the computational auditory model.
Scale-invariant sounds generated by the model were rated by human adults as natural, and described verbally as different instances of water sounds (e.g. rain, running tap etc.). When scale-invariance across spectral bands was violated, adults did not perceive the sounds as natural. Gervain et al. (2014, 2016) investigated whether very young infants were also sensitive to scale-invariance in water sounds.
The first study (Gervain et al., 2014), which focused on 5-month-old infants, habituated infants to either scale-invariant or variable-scale sounds. When habituated to scale-invariant sounds, infants looked significantly longer to a change to variable-scale sounds, whereas infants habituated to variable-scale sounds showed no such difference. These results suggest that infants were able to form a perceptual category of the scale-invariant, i.e. natural water sounds, but not of variable-scale sounds, which indeed are not perceived as natural sounds by adults either. Further, infants showed no preference between those scale-variant water sounds that adults judged more typical (e.g. rain) and those that they judged less typical, suggesting that scale-variance is possibly a more important feature of water sounds for infants than familiarity.
One aspect not investigated in these studies, as noted by the authors, is the influence of experience and initial exposure. In the second study, therefore, the same stimuli were presented to newborn infants between 0–3 days old, and fNIRS was utilized to uncover the neural mechanisms involved in processing these sounds (Gervain et al., 2016). The results revealed that newborns are able to process the statistical properties of scale-invariant natural stimuli, successfully discriminating variable-scale and scale-invariant stimuli, similarly to 5-month-olds. The localization of the differential response in the left frontal and temporal areas aligns with adult studies, which demonstrate that rapidly changing auditory events preferentially engage the left temporal areas (Hickok and Poeppel, 2007; Zatorre et al., 2002; Gervain et al., 2016). These findings indicate that the human brain is ready from early life to process natural sounds as distinctive signals (Gervain et al., 2016).
Interestingly, however, a recent study by Agrawal and Schachner (2023) suggests that children's sensitivity to a specific attribute of water sounds, temperature undergoes developmental refinement. Figure 5 shows how texture statistics, especially cross-band temporal-envelope correlations, differ between the sounds of cold and hot water, used by Agrawal and Schachner (2023). The authors found that children's ability to estimate the temperature of water from its sound, robust in adults, is not yet present in children between 3–6 years of age. This skill appears only in middle childhood at ages 7–11 years, and develops gradually over the first decade of life. These age-related differences in children may be partially driven by varying amounts of relevant experience and changes in auditory sensitivity over the course of childhood.
Figure 5. Summary statistics computed by a model of auditory texture perception (McWalter and Dau, 2017) in response to the hot (top panels) and cold (bottom panels) water sounds used by Agrawal and Schachner (2023). The two sounds show comparable power spectra and envelope sparsity in each cochlear channel. However, the coordination of the temporal envelopes at the output of the cochlear channels appears larger for the sound of hot water. See Supplementary Appendix for additional information about the stimuli and computational auditory model.
The perception of water sounds has also been explored in atypically developing children. Testing anecdotal reports suggesting that children with Williams syndrome have exceptional skills for recognizing environmental sounds by timbre, one study presented water sounds (e.g., sea, shower, fountain, waterfall, river) and sounds produced by walking (e.g., running downstairs, walking on shingle, walking on pavement, walking on rubble, running on pavement) to children with Williams syndrome, Down syndrome, and typically developing children in an identification task. Results showed that children with Williams syndrome performed lower than their typically developing peers and similarly to those with Down syndrome for both types of sounds. This indicates that Williams syndrome children do not have increased auditory sensitivity, challenging previous claims (Martínez-Castilla et al., 2015). Rather, both groups of atypically developing children showed poorer identification than typically developing peers.
Some studies approached the question of the specificity of children's auditory perception by comparing their perception of a wide variety of different sound categories. One of the first such studies, Shultz and Vouloumanos (2010) compared 3-month-old infants' listening patterns to speech in unfamiliar languages, rhesus macaque vocalizations, human non-communicative vocalizations, human non-speech communicative vocalizations, and environmental sound stimuli. Environmental sounds comprised mechanical sounds (e.g., bells, hammers) and natural geophysical sounds (e.g., wind, rain) commonly found in infants' surroundings (Shultz and Vouloumanos, 2010). The study found that 3-month-old infants listened longer to speech than to any other sound category. However, the difference was less pronounced when speech was compared to environmental sounds (Shultz and Vouloumanos, 2010). The authors suggest that infants may not perceive mixed environmental sounds as a coherent category because they originate from diverse sources (Shultz and Vouloumanos, 2010). This strongly implies a necessity for more controlled experiments in this area.
Another study focused on the development of sound-object associations using environmental sounds (e.g. animal cries, human nonverbal vocalizations, vehicle noises, alarms, water sounds, and music) and their matched verbal descriptions in 15–20-month-old infants (Cummings et al., 2009). The authors observed that toddlers were better able to learn associations for both types of sounds with age, but there was no difference between the two sound types (Cummings et al., 2009).
A more recent study investigated 6–12-year-old-children's attitudes to different soundscapes, encompassing adult conversation, children's play, nature sounds (such as leaves rustling and water sounds), animal sounds (like barking and bird songs), motorized and electromechanical sounds (such as traffic and construction), and classical music (Su et al., 2023). The findings revealed that children anticipated more social interaction when exposed to children's sounds and nature sounds, possibly due to social cues and interactive activities like water play. In contrast, they associated animal sounds and classical music with solitary activities, owing to their perceived relaxing and restorative qualities. Intriguingly, these findings resonate with reports of the restorative effects of environmental sounds observed in adults (Su et al., 2023; Lorenzi et al., 2023). Conversely, motorized and electromechanical sounds were generally avoided by children (Su et al., 2023).
The use of acoustic databases collected by soundscape ecologists and eco-acousticians (Sueur and Farina, 2015) and implementation of novel behavioral paradigms inspired by cognitive ethologists offer developmental psychologists and neuroscientists unique opportunities to set up experimental paradigms with enhanced ecological validity. In that respect, future work should consider testing infant and children with acoustic stimuli and behavioral tasks targeting the repertoire of natural auditory behaviors in humans (Kingstone et al., 2008; Miller et al., 2022), that is sounds and behaviors involved in environmental monitoring (Keidser et al., 2022) by contrast with communication behaviors. The objective would be to study the human capacity to process natural soundscape information in (truly) ecologically-valid situations (Lewkowicz, 2001; Schmuckler, 2001; Holleman et al., 2020), that is for stimuli and tasks representative of those experienced in everyday life and relevant to the psychological process being investigated. This requires replacing laboratory stimuli by biotic and abiotic sounds recorded in situ—that is natural sounds shaped by the specific propagation characteristic of natural habitats (Mouterde et al., 2014)—and the identification of the repertoire of natural behaviors for humans via ethnographic studies aiming at characterizing “ordinary listening behaviors” in rural or wild settings. Evaluating the strength of rain or wind, composition and speed of running waters, discriminating dusk from night or more simply assessing changes in biodiversity in the surrounding acoustic environment may be important behaviors for people living in such places as they probably were for our ancestors. This enterprise belongs to cognitive ethology (Kingstone et al., 2008). Unfortunately, such studies are clearly lacking. To the best of our knowledge, at least two cognitive psychology studies suggest that sensory processing and attention may differ between rural and urban elderly people (Hirst et al., 2022). These studies indicate that rural environments are less complex than urban ones and situations typical of urban life such as road crossing require divided attention more than focused attention (Cassarino and Setti, 2016). More work is clearly warranted to characterize differences between urban, rural and wild settings, not only in terms of soundscape features (e.g., De Coensel et al., 2003) but also in terms of listening behaviors.
Taken together, these studies, summarized in Table 1, paint a complex picture and the available developmental data do not yet allow definitive conclusions to be drawn regarding the following competing hypotheses formulated to explain human ability to process natural sounds: (1) early sensitivity to water sounds suggests that infants and children perceive natural sounds through general auditory mechanisms distinct from those involved in speech processing and presumably shaped by ancestral selective pressures (Chen and Wiens, 2020; Lorenzi et al., 2023); (2) alternatively, newborns' similar preferences for monkey vocalizations and speech suggest that mechanisms involved in environmental sound and speech processing may initially develop together and then undergo specialization due to subsequent exposure (Perszyk and Waxman, 2016); (3) it is also possible that different classes of natural sounds are processed differently, depending on their acoustic characteristics or their survival value. Thus, primate or other animal vocalizations, which share some of their acoustic features with speech, such as harmonicity or slow temporal modulations, may be perceived differently from natural sounds and other texture-like sounds. Any attempt to test further these competing hypotheses should adopt an ecological perspective by capitalizing on the available databases of soundscapes collected in natural settings acoustically similar to human ancestral habitats and test basic auditory capacities presumably engaged in ordinary listening behaviors, some of which are currently being investigated in adults (Lorenzi et al., 2023).
As we look ahead, the next crucial steps in the research agenda of the field of human auditory ecology involve a principled investigation of how natural soundscapes are perceived and processed across development into adulthood.
First, we need to explore children's basic perceptual sensitivities when processing natural sounds and soundscapes. Natural soundscapes show strong periodicity due to the day-night cycle, with distinct choruses at dawn and dusk forming a double-peaked circadian pattern of biological activity (Lorenzi et al., 2023), as well as due to the change of seasons. The biodiversity of a habitat also has its signature in its soundscape. Birds, insects, and amphibians, as primary contributors, produce vocalizations with faster temporal modulations, creating unique acoustic regularities that mammals, including human ancestors, have been exposed to for millions of years (Lorenzi et al., 2023). Exploring whether children, like adults, can discriminate between different habitats (e.g. savannah vs. rainforest), the same habitat at different times of the day or in different seasons—that is global attributes of natural auditory scenes (McMullin et al., 2024)—will provide comprehensive insights into how auditory discrimination develops and the role of experience in shaping this skill. Behavioral studies with adults (Apoux et al., 2023) suggest that exposure may not fundamentally impact discrimination of global attributes of natural soundscapes such as geolocation (habitat) or moment of the day. Several hours of training does not change adults' discrimination performance. May there be a critical period for attuning to the specifics of one's auditory environment just like there is a critical period for speech and music perception? Do young infants show greater or lesser sensitivity to natural sounds and soundscapes than adults? Can they discriminate all their relevant features? Do rural and urban children show similar sensitivities? Answering these questions is fundamental for a better understanding of human auditory ecology. Behavioral measures can be complemented by brain imaging techniques, now also readily applicable to even the youngest infants, to explore the neural correlates of these abilities. Indeed, integrating behavioral and neural measures could offer a more nuanced understanding. For example, infants might show neural signatures of being able to auditorily perceive the temperature of water, even if this sensitivity is not apparent behaviorally (Agrawal and Schachner, 2023).
Children's sensitivity to the characteristics of natural sounds and soundscapes can be built on in education and in raising awareness of ecology and biodiversity. By understanding children's sensitivity to biodiversity in soundscapes, we can develop educational strategies that nurture environmental consciousness in children. Indeed, an exciting aspect of this research is the potential to cultivate ecological awareness from an early age, ultimately fostering a deeper connection to and responsibility for the natural world.
Beyond basic auditory sensitivities, research shows that natural sounds, and in particular biodiversity (species richness and species abundance) and water sounds in natural soundscapes enhance wellbeing and exert restorative effects on their listeners (for a recent review, see Ratcliffe, 2021). Most of this research, however, have investigated restorative effects in adults. Much less is known about how natural sounds impact children's moods. Yet, these restorative effects may be useful in family, educational and even clinical contexts to improve children's moods, reduce stress and anxiety and promote wellbeing. More research is thus needed to understand how natural sounds or the lack of them impact children's psychological and mental health. This research could involve not only behavioral, but also physiological measures of mood such as using infant / child heart rate monitors to measure heart rate variability (HRV). Understanding these developmental trajectories will highlight the significance of natural soundscapes in enhancing cognitive and emotional health from infancy through adulthood.
This is also crucial for deaf and hard-of-hearing children. Currently, most intervention and rehabilitation programs (hearing aids, cochlear implants etc.) are optimized for speech perception and urban environments (Lorenzi et al., 2023). It is thus little known to what extent these programs and devices restore the objective and subjective percepts of natural sounds. It may thus be the case that deaf and hard-of-hearing people benefit less from natural sounds than their hearing peers because the hearing support or intervention they receive does not restore auditory experiences with natural sounds sufficiently well (Lorenzi et al., 2023; Miller-Viacava et al., 2023). More research is needed to improve natural soundscape perception and emotional responses through these devices, especially for early interventions.
In conclusion, understanding how children perceive natural sounds is crucial for unraveling the complexities of auditory development and its evolutionary underpinnings. Infants and young children are exposed to a rich tapestry of natural sounds from birth, and their perceptual abilities in this domain are only beginning to be understood. Current research highlights the importance of both biologically predisposed and experiential factors in shaping these auditory sensitivities. As we advance, adopting ecological approaches and utilizing natural soundscapes in experimental paradigms will provide deeper insights into the development of human auditory ecology. This will not only enhance our understanding of sensory processing but also inform strategies to harness the restorative and educational potential of natural sounds, ultimately promoting wellbeing and environmental awareness from an early age.
SP: Writing – original draft, Conceptualization. NM-V: Data curation, Formal analysis, Methodology, Visualization, Writing – review & editing. MF: Data curation, Formal analysis, Methodology, Visualization, Writing – review & editing. JG: Writing – review & editing, Conceptualization. CL: Writing – review & editing, Conceptualization.
The author(s) declare financial support was received for the research, authorship, and/or publication of this article. This work was supported by ANR-17-EURE-0017, ANR-20-CE28 Hearbiodiv and ANR-20-CE28 Audieco to CL, as well as the ERC Consolidator Grant “BabyRhythm 773202,” a FARE grant nr. R204MPRHKE from the Italian Ministry for Universities and Research, a PNRR-MAD-2022–12376739 Grant (“SYNPHONIA” Next Generation EU – PNRR M6C2 - Investimento 2.1 Valorizzazione e potenziamento della ricerca biomedica del SSN CUP C93C22009100007) and a MUR 2022WX3FM5 PRIN grant [Bando PRIN 2022 CUP C53D23004290006, Piano Nazionale di Ripresa e Resilienza (PNRR), Missione 4, Componente 2 – Investimento 1.1. “Progetti di ricerca di Rilevante Interesse Nazionale” – PRIN] awarded to JG. Open Access funding provided by Università degli Studi di Padova | University of Padua, Open Science Committee.
Natural soundscape recordings were made available by the Bernie Krause Natural Sound Archive (BKNSA) currently licensed exclusively to Wild Sanctuary, Inc. Urban soundscapes recorded in Marseille (France) were made available by Sabine Meunier (LMA, CNRS). Bird and insect recordings were made available by Jérôme Sueur and Sylvain Haupert (Museum national d'Histoire naturelle). The running water and speech database were made available by Klaus et al. (2019) and Ramus et al. (1999), respectively. The authors wish to thank Marcus Klaus, Bernie Krause, Elie Grinfeder, Jérôme Sueur, Léo Varnet, Sabine Meunier, and Richard McWalter for helping us to build up the sound database used in the present study.
The authors declare that the research was conducted in the absence of any commercial or financial relationships that could be construed as a potential conflict of interest.
All claims expressed in this article are solely those of the authors and do not necessarily represent those of their affiliated organizations, or those of the publisher, the editors and the reviewers. Any product that may be evaluated in this article, or claim that may be made by its manufacturer, is not guaranteed or endorsed by the publisher.
The Supplementary Material for this article can be found online at: https://www.frontiersin.org/articles/10.3389/fpsyg.2024.1474961/full#supplementary-material
Agrawal, T., and Schachner, A. (2023). Hearing water temperature: characterizing the development of nuanced perception of sound sources. Dev. Sci. 26:e13321. doi: 10.1111/desc.13321
Altmann, C. F., Doehrmann, O., and Kaiser, J. (2007). Selectivity for animal vocalizations in the human auditory cortex. Cereb. Cortex 17, 2601–2608. doi: 10.1093/cercor/bhl167
Apoux, F., Miller-Viacava, N., Ferrière, R., Dai, H., Krause, B., Sueur, J., et al. (2023). Auditory discrimination of natural soundscapes. J. Acoust. Soc. Am. 153, 2706–2706. doi: 10.1121/10.0017972
Belin, P. (2006). Voice processing in human and non-human primates. Philos. Trans. R. Soc. B Biol. Sci. 361, 2091–2107. doi: 10.1098/rstb.2006.1933
Cassarino, M., and Setti, A. (2016). Complexity as key to designing cognitive-friendly environments for older people. Front. Psychol. 7:1329. doi: 10.3389/fpsyg.2016.01329
Catchpole, C. K., and Slater, P. J. B. (2008). Bird Song: Biological Themes and Variations, 2nd ed. Cambridge: Cambridge University Press. doi: 10.1017/CBO9780511754791
Chen, Z., and Wiens, J. J. (2020). The origins of acoustic communication in vertebrates. Nat. Commun. 11 :369. doi: 10.1016/j.ecolind.2021.107942
Cristia, A., Minagawa, Y., and Dupoux, E. (2014). Responses to vocalizations and auditory controls in the human newborn brain. PLoS ONE 9:e115162. doi: 10.1371/journal.pone.0115162
Cummings, A., Saygin, A. P., Bates, E., and Dick, F. (2009). Infants' recognition of meaningful verbal and nonverbal sounds. Lang. Learn. Dev. 5, 172–190. doi: 10.1080/15475440902754086
De Coensel, B., Botteldooren, D., and De Muer, T. (2003). 1/f noise in rural and urban soundscapes. Acta acustica united with acustica, 89, 287–295.
Fay, R. R., and Popper, A. N. (1994). Comparative Hearing: Mammals. Springer Handbook of Auditory Research. New York, NY: Springer. doi: 10.1007/978-1-4612-2700-7_1
Ferry, A. L., Hespos, S. J., and Waxman, S. R. (2013). Nonhuman primate vocalizations support categorization in very young human infants. Proc. Nat. Acad. Sci. 110, 15231–15235. doi: 10.1073/pnas.122116611
Geffen, M. N., Gervain, J., Werker, J. F., and Magnasco, M. O. (2011). Auditory perception of self-similarity in water sounds. Front. Integr. Neurosci. 5:15. doi: 10.3389/fnint.2011.00015
Gemignani, J., and Gervain, J. (2024). A within-subject multimodal NIRS-EEG classifier for infant data. Sensors 24:4161. doi: 10.3390/s24134161
Gervain, J., and Mehler, J. (2010). Speech perception and language acquisition in the first year of life. Annu. Rev. Psychol. 61, 191–218. doi: 10.1146/annurev.psych.093008.100408
Gervain, J., Werker, J. F., Black, A., and Geffen, M. N. (2016). The neural correlates of processing scale-invariant environmental sounds at birth. Neuroimage 133, 144–150. doi: 10.1016/j.neuroimage.2016.03.001
Gervain, J., Werker, J. F., and Geffen, M. N. (2014). Category-specific processing of scale-invariant sounds in infancy. PLoS ONE 9:e96278. doi: 10.1371/journal.pone.0096278
Grinfeder, E., Lorenzi, C., Haupert, S., and Sueur, J. (2022). What do we mean by “soundscape”? A functional description. Front. Ecol. Evol. 10:894232 doi: 10.3389/fevo.2022.894232
Guastavino, C. (2003). Etude sémantique et acoustique de la perception des basses fréquences dans l'environnement sonore urbain (Doctoral dissertation). Paris, p. 6.
Guyot, P., Houix, O., Misdariis, N., Susini, P., Pinquier, J., and André-Obrecht, R. (2017). Identification of categories of liquid sounds. J. Acoust. Soc. Am. 142, 878–889. doi: 10.1121/1.4996124
Hickok, G., and Poeppel, D. (2007). The cortical organization of speech processing. Nat. Rev. Neurosci. 8, 393–402. doi: 10.1038/nrn2113
Hirst, R. J., Cassarino, M., Kenny, R. A., Newell, F. N., and Setti, A. (2022). Urban and rural environments differentially shape multisensory perception in ageing. Aging Neuropsychol. Cogn. 29, 197–212. doi: 10.1080/13825585.2020.1859084
Holleman, G. A., Hooge, I. T., Kemner, C., and Hessels, R. S. (2020). The ‘real-world approach'and its problems: a critique of the term ecological validity. Front. Psychol. 11:721. doi: 10.3389/fpsyg.2020.00721
Hoy, R. R., Popper, A. N, and Fay, R. R. (1998). Comparative Hearing: Insects. Springer Handbook of Auditory Research. New York, NY: Springer.
Keidser, G., Naylor, G., Brungart, D. S., Caduff, A., Campos, J., Carlile, S., et al. (2022). Comment on the point of view “ecological validity, external validity and mundane realism in hearing science”. Ear Hear. 43, 1601–1602. doi: 10.1097/AUD.0000000000001241
Kingstone, A., Smilek, D., and Eastwood, J. D. (2008). Cognitive ethology: a new approach for studying human cognition. Br. J. Psychol. 99, 317–340. doi: 10.1348/000712607X251243
Klaus, M., Geibrink, E., Hotchkiss, E.R., and Karlsson, J. (2019). Listening to air–water gas exchange in running waters. Limnol. Oceanogr. Methods 17, 395–414. doi: 10.1002/lom3.10321
Lange-Küttner, C. (2010). Discrimination of sea-bird sounds vs. garden-bird songs: do Scottish and German-Saxon infants show the same preferential looking behaviour as adults? Eur. J. Dev Psychol. 7, 578–602. doi: 10.1080/17405620902937531
Lewicki, M. S. (2002). Efficient coding of natural sounds. Nat. Neurosci. 5, 356–363. doi: 10.1038/nn831
Lewkowicz, D. J. (2001). The concept of ecological validity: what are its limitations and is it bad to be invalid? Infancy 2, 437–450. doi: 10.1207/S15327078IN0204_03
Liberman, A. M., and Mattingly, I. G. (1985). The motor theory of speech perception revised. Cognition 21, 1–36. doi: 10.1016/0010-0277(85)90021-6
Lickliter, R., and Witherington, D. C. (2017). Towards a truly developmental epigenetics. Hum. Dev. 60, 124–138. doi: 10.1159/000477996
Lorenzi, C., Apoux, F., Grinfeder, E., Krause, B., Miller-Viacava, N., and Sueur, J. (2023). Human auditory ecology: extending hearing research to the perception of natural soundscapes by humans in rapidly changing environments. Trends Hear. 27:23312165231212032. doi: 10.1177/23312165231212032
Martínez-Castilla, P., García-Nogales, M. Á., Campos, R., and Rodríguez, M. (2015). Environmental sound recognition by timbre in children with Williams syndrome. Child Neuropsychol. 21, 90–105. doi: 10.1080/09297049.2013.876492
McDermott, J. H., Oxenham, A. J., and Simoncelli, E. P. (2009). “Sound texture synthesis via filter statistics,” in 2009 IEEE Workshop on Applications of Signal Processing to Audio and Acoustics (New Paltz, NY: IEEE), 297–300.
McMullin, M.A., Kumar, R., Higgins, N.C., Gygi, B., Elhilali, M., and Snyder, J.S. (2024). Preliminary evidence for global properties in human listeners during natural auditory scene perception. Open Mind 8, 333–365. doi: 10.1162/opmi_a_00131
McWalter, R., and Dau, T. (2017). Cascaded amplitude modulations in sound texture perception. Front. Neurosci. 11:485. doi: 10.3389/fnins.2017.00485
Miller, C. T., Gire, D., Hoke, K., Huk, A. C., Kelley, D., Leopold, D. A., et al. (2022). Natural behavior is the language of the brain. Curr. Biol. 32, R482–R493. doi: 10.1016/j.cub.2022.03.031
Miller-Viacava, N., Lazard, D., Delmas, T., Krause, B., Apoux, F., and Lorenzi, C. (2023). Sensorineural hearing loss alters auditory discrimination of natural soundscapes. Int. J. Audiol. 63, 1–10. doi: 10.1080/14992027.2023.2272559
Minagawa-Kawai, Y., Van Der Lely, H., Ramus, F., Sato, Y., Mazuka, R., and Dupoux, E. (2011). Optical brain imaging reveals general auditory and language-specific processing in early infant development. Cereb. Cortex 21, 254–261. doi: 10.1093/cercor/bhq082
Mouterde, S. C., Theunissen, F. E., Elie, J. E., Vignal, C., and Mathevon, N. (2014). Acoustic communication and sound degradation: how do the individual signatures of male and female zebra finch calls transmit over distance?. PLoS ONE 9:e102842. doi: 10.1371/journal.pone.0102842
Nallet, C., and Gervain, J. (2021). Neurodevelopmental preparedness for language in the neonatal brain. Ann. Rev. Dev. Psychol. 3, 41–58. doi: 10.1146/annurev-devpsych-050620-025732
Oyama, S. (1979). The concept of the sensitive period in developmental studies. Merrill Palmer Q. Behav. Dev. 25, 83–103.
Perszyk, D. R., and Waxman, S. R. (2016). Listening to the calls of the wild: the role of experience in linking language and cognition in young infants. Cognition 153, 175–181. doi: 10.1016/j.cognition.2016.05.004
Polver, S., Háden, G. P., Bulf, H., Winkler, I., and Tóth, B. (2023). Early maturation of sound duration processing in the infant's brain. Sci. Rep. 13:10287. doi: 10.1038/s41598-023-36794-x
Ramus, F., Nespor, M., and Mehler, J. (1999). Correlates of linguistic rhythm in the speech signal. Cognition 73, 265–292. doi: 10.1016/S0010-0277(00)00101-3
Ratcliffe, E. (2021). Sound and soundscape in restorative natural environments: a narrative literature review. Front. Psychol. 12:570563. doi: 10.3389/fpsyg.2021.570563
Ravignani, A., Dalla Bella, S., Falk, S., Kello, C. T., Noriega, F., and Kotz, S. A. (2019). Rhythm in speech and animal vocalizations: a cross-species perspective. Ann. N. Y. Acad. Sci. 1453, 79–98. doi: 10.1111/nyas.14166
Reh, R. K., Dias, B. G., Nelson III, C. A., Kaufer, D., Werker, J. F., Kolb, B., et al. (2020). Critical period regulation across multiple timescales. Proc. Nat. Acad. Sci. 117, 23242–23251. doi: 10.1073/pnas.1820836117
Santolin, C., Russo, S., Calignano, G., Saffran, J. R., and Valenza, E. (2019). The role of prosody in infants' preference for speech: a comparison between speech and birdsong. Infancy 24, 827–833. doi: 10.1111/infa.12295
Schmuckler, M. A. (2001). What is ecological validity? A dimensional analysis. Infancy 2, 419–436. doi: 10.1207/S15327078IN0204_02
Scott, L. S., Pascalis, O., and Nelson, C. A. (2007). A domain-general theory of the development of perceptual discrimination. Curr. Dir. Psychol. Sci. 16, 197–201. doi: 10.1111/j.1467-8721.2007.00503.x
Senter, P. (2008). Voices of the past: a review of Paleozoic and Mesozoic animal sounds. Hist. Biol.
Shultz, S., and Vouloumanos, A. (2010). Three-month-olds prefer speech to other naturally occurring signals. Lang. Learn. Dev. 6, 241–257. doi: 10.1080/15475440903507830
Simoncelli, E. P., and Olshausen, B. A. (2001). Natural image statistics and neural representation. Annu. Rev. Neurosci. 24, 1193–1216. doi: 10.1146/annurev.neuro.24.1.1193
Singh, N.C., and Theunissen, F.E. (2003). Modulation spectra of natural sounds and ethological theories of auditory processing. J. Acoust. Soc. Am. 114, 3394–3411. doi: 10.1121/1.1624067
Smith, E. C., and Lewicki, M. S. (2006). Efficient auditory coding. Nature 439, 978–982. doi: 10.1038/nature04485
Su, H., Ma, H., and Wang, C. (2023). Effects of soundscape on Chinese children's social interaction measured by self-reported behavioral expectations. Appl. Acoust. 207:109350. doi: 10.1016/j.apacoust.2023.109350
Sueur, J., and Farina, A. (2015). Ecoacoustics: the ecological investigation and interpretation of environmental sound. Biosemiotics 8, 493–502. doi: 10.1007/s12304-015-9248-x
Theunissen, F. E., and Elie, J. E. (2014). Neural processing of natural sounds. Nat. Rev. Neurosci. 15, 355–366. doi: 10.1038/nrn3731
Trainor, L. J., and Unrau, A. (2011). “Development of pitch and music perception,” in Human Auditory Development, eds. L. Werner, R. R. Fay, and A. N. Popper (New York, NY: Springer New York), 223–254.
Trehub, S. E., and Hannon, E. E. (2006). Infant music perception: domain-general or domain-specific mechanisms? Cognition 100, 73–99. doi: 10.1016/j.cognition.2005.11.006
Varnet, L., Ortiz-Barajas, M.C., Guevara Erra, R., Gervain, J., and Lorenzi, C. (2017). A cross-linguistic study of speech modulation spectra. J. Acoust. Soc. Am. 142, 1976–1989. doi: 10.1121/1.5006179
Velasco, C., Jones, R., King, S., and Spence, C. (2013). The sound of temperature: what information do pouring sounds convey concerning the temperature of a beverage. J. Sens. Stud. 28, 335–345. doi: 10.1111/joss.12052
Vouloumanos, A., Hauser, M. D., Werker, J. F., and Martin, A. (2010). The tuning of human neonates' preference for speech. Child Dev. 81, 517–527. doi: 10.1111/j.1467-8624.2009.01412.x
Werker, J. F. (2018). Perceptual beginnings to language acquisition. Appl. Psycholinguist. 39, 703–728. doi: 10.1017/S0142716418000152
Werker, J. F., and Hensch, T. K. (2015). Critical periods in speech perception: new directions. Annu. Rev. Psychol. 66, 173–196. doi: 10.1146/annurev-psych-010814-015104
Winkler, I., Háden, G. P., Ladinig, O., Sziller, I., and Honing, H. (2009). Newborn infants detect the beat in music. Proc. Nat. Acad. Sci. 106, 2468–2471. doi: 10.1073/pnas.0809035106
Keywords: human auditory ecology, auditory development, infants, children, environmental sounds, natural soundscapes, animal vocalizations, water sounds
Citation: Polver S, Miller-Viacava N, Fraticelli M, Gervain J and Lorenzi C (2024) Developmental origins of natural sound perception. Front. Psychol. 15:1474961. doi: 10.3389/fpsyg.2024.1474961
Received: 02 August 2024; Accepted: 26 November 2024;
Published: 11 December 2024.
Edited by:
Catherine E. Read, Rutgers, The State University of New Jersey, United StatesReviewed by:
Evelyne Mercure, Goldsmiths University of London, United KingdomCopyright © 2024 Polver, Miller-Viacava, Fraticelli, Gervain and Lorenzi. This is an open-access article distributed under the terms of the Creative Commons Attribution License (CC BY). The use, distribution or reproduction in other forums is permitted, provided the original author(s) and the copyright owner(s) are credited and that the original publication in this journal is cited, in accordance with accepted academic practice. No use, distribution or reproduction is permitted which does not comply with these terms.
*Correspondence: Silvia Polver, c2lsdmlhLnBvbHZlckB1bmlwZC5pdA==
Disclaimer: All claims expressed in this article are solely those of the authors and do not necessarily represent those of their affiliated organizations, or those of the publisher, the editors and the reviewers. Any product that may be evaluated in this article or claim that may be made by its manufacturer is not guaranteed or endorsed by the publisher.
Research integrity at Frontiers
Learn more about the work of our research integrity team to safeguard the quality of each article we publish.