- Department of Psychiatry and Behavioral Sciences, McGovern Medical School at UTHealth, Houston, TX, United States
Evolutionary biology provides a unifying theory for testing hypotheses about the relationship between hormones and person perception. Person perception usually receives attention from the perspective of sexual selection. However, because person perception is one trait in a suite regulated by hormones, univariate approaches are insufficient. In this Perspectives article, quantitative genetics is presented as an important but underutilized framework for testing evolutionary hypotheses within this literature. We note tacit assumptions within the current literature on psychiatric genetics, which imperil the interpretation of findings thus far. As regulators of a diverse manifold of traits, hormones mediate tradeoffs among an array of functions. Hormonal pleiotropy also provides the basis of correlational selection, a process whereby selection on one trait in a hormone-mediated suite generates selection on the others. This architecture provides the basis for conflicts between sexual and natural selection within hormone-mediated suites. Due to its role in person perception, psychiatric disorders, and reproductive physiology, the sex hormone estrogen is highlighted as an exemplar here. The implications of this framework for the evolution of person perception are discussed. Empirical quantification of selection on traits within hormone-mediated suites remains an important gap in this literature with great potential to illuminate the fundamental nature of psychiatric disorders.
1 Introduction
Hormones integrate traits into adaptive suites (McGlothlin and Ketterson, 2008). When several traits are regulated by one hormone, their response to evolutionary processes will be linked. Under these circumstances, hormonal mediation may facilitate or restrain phenotypic responses to selection, depending on the form of selection and underlying genetic architecture. Though hormones have adaptive functions, their actions can also predispose individuals to certain conditions, such as cancers and infections (Klein, 2000; Chuffa et al., 2017). Many psychiatric disorders are also linked with variation in hormone expression (Jacobson, 2014; Naughton et al., 2014; Iovino et al., 2018; Gogos et al., 2019). The evolution of hormone-mediated traits involves tradeoffs between their beneficial effects (e.g., maintaining reproduction) and deleterious effects (e.g., susceptibility to cancer).
As an example, estrogen regulates reproductive physiology in women, but a rapid drop in estrogen following parturition leaves women susceptible to depressive episodes (Schiller et al., 2015; Yim et al., 2015). Estrogen’s involvement in both phenotypes provides the basis for a tradeoff between the two. In the presence of tradeoffs, predicting the evolutionary response of traits can be complex and requires a rigorous framework for analysis. The direction of selection on traits involved must be measured empirically because it may differ between traits within the same hormone-mediated suite (McGlothlin and Ketterson, 2008).
Prior work also implicates hormones in the modulation of person perception (Gangestad and Thornhill, 2008; Romero-Martinez et al., 2021). As part of hormone-mediated suites, person perception is unlikely to evolve independently of other traits regulated by the same hormones. In this Perspectives article, we focus on estrogen because it directly influences fitness components and is a well-studied influence on person perception and psychiatric disorders. We introduce evolutionary quantitative genetics to provide a framework for discussion. We then review known and emerging functions of estrogen. Finally, we discuss the evolutionary dynamics of sex hormone-mediated suites and implications for the evolution of psychiatric disorders and person perception. Our hope is that the framework discussed here could similarly be applied to other traits of interest to evolutionary psychology as has been accomplished robustly in behavioral quantitative genetics (Boake, 1994).
1.1 Quantitative genetics – a framework for testing evolutionary hypotheses
Darwin’s theory of evolution by natural selection can be distilled to the following syllogism: for a given population, if more offspring are produced than survive to reproduce, and if individuals vary in their traits (including fitness), and if some of that variation is heritable, then that population will evolve (Darwin, 1859). Darwin recognized a second mechanism, sexual selection, which hinges on variation in the ability to obtain mates (via intrasexual competition or mate choice) rather than variation in survival. This theory was formalized beginning in the 20th century by Ronald Fisher and Sewell Wright, marking the birth of evolutionary quantitative genetics (Lynch and Walsh, 1998; Mayr and Provine, 1998). This approach focuses on phenotypic variation associated with multiple genetic loci, which is the case for most behaviors, psychological traits, and psychiatric disorders (Geschwind and Flint, 2015). A deceptively simple set of equations may be used to predict how variation in quantitative traits is expected to respond to natural or sexual selection. Quantitative genetics thus provides an analytically rigorous framework for applying evolutionary theory to and empirically testing hypotheses posited by evolutionary psychology.
First, phenotypic variance can be decomposed into multiple sources. Twin studies are the most commonly used quantitative genetic study design for psychiatric disorders and psychological traits (Kendler, 1993; Kendler, 2001; Vukasovic and Bratko, 2015). Modern quantitative genetics benefits from pedigree-based studies, including the use of the “animal model,” which permits the use of pedigrees that are missing measurement of traits in some individuals and allows for more precise estimation of variance components (Kruuk, 2004; Kruuk and Hadfield, 2007). When combined with adoption studies, common environmental effects can be estimated (Lynch and Walsh, 1998). If pedigrees are sufficiently large and complex (e.g., contain mothers who have offspring from different fathers) or contain repeated measurements, other parameters such as maternal effects and permanent environmental effects may be estimated. The basic equation describing decomposition of phenotypic variance is given as:
In this equation, P refers to phenotypic variance, G to genetic variance, E to environmental variance, GxE to variance from gene x environment effects, and R to residual variance (Falconer and Mackay, 1996). Genetic variance may arise from additive genetic variation, dominance, or epistasis. The proportion of phenotypic variance accounted for by genetic variance is called broad-sense heritability. Sources of environmental variance include parental effects, common environmental effects, or permanent environmental effects. GxE effects occur when different genotypes respond to environmental change in nonparallel ways. For all psychiatric disorders studied, phenotypic variance results from both environmental and genetic sources (Sullivan and Geschwind, 2019). Face perception likewise has both environmental and heritable sources of variance (Zhu et al., 2010).
For predicting the response to selection, two key parameters are important: narrow-sense heritability and the strength of selection (Lush, 1937; Walsh and Lynch, 2018). Narrow-sense heritability (h2) is defined as the proportion of phenotypic variance (P) due to additive genetic effects (A) (Falconer and Mackay, 1996; Lynch and Walsh, 1998):
Of note, narrow-sense heritability describes only part of the resemblance between relatives’ phenotypes. Other sources of genetic variation will also cause resemblance among relatives. Quantitative genetics focuses on narrow-sense heritability, however, because this component responds to selection. Common environmental and parental effects may also cause resemblance between siblings and can inflate estimates of heritability if not measured (Lynch and Walsh, 1998). Some variance due to epigenetics may be heritable; recent extensions of quantitative genetic models incorporate this (Jablonka and Raz, 2009; Franklin et al., 2010; Stopher et al., 2012; Thomson et al., 2018). In humans, cultural inheritance is especially important and has also recently received attention within quantitative genetics (Danchin et al., 2011, 2013). Any trait with non-zero heritability has the potential to respond to selection.
When examining suites of traits, however, heritability must be extended to account for genetic correlations between those traits. The multivariate extension of heritability is defined by a matrix of additive genetic variances and covariances among traits called the G-matrix. From the G-matrix, one can calculate genetic correlations (rG):
The genetic correlation is simply the Pearson correlation between the additive genetic components of two traits (A1 and A2). Recent studies suggest genetic correlations among many psychiatric disorders (Brainstorm et al., 2018; Grotzinger et al., 2022). Interestingly, another recent study suggested that psychiatric disorders are genetically correlated most strongly with pulmonary, gastrointestinal, and neurological disorders (Athanasiadis et al., 2022). Collectively, these studies imply that evolution of traits involved in one psychiatric disorder will depend on evolution of traits involved not only in other psychiatric disorders, but also in disorders involving organs beyond the brain. Similarly, genetic correlations among scores in the Minnesota Multiphasic Personality Inventory would suggest that personality traits will not evolve entirely independently of each other – a pattern of great import for the evolutionary psychology of personality (Viken and Rose, 2007).
The second key parameter, the strength of selection, was formalized by George Price (Price, 1970, 1972). The “Price Equation” defines selection on a phenotype as the covariance between that phenotype (P) and fitness (ω):
Fitness in evolutionary genetics is defined as differential reproductive success—an individual’s lifetime number of pregnancies relative to the population mean. The average number of children varies widely among human populations (United Nations, 2022). Because usually S < 1, variance in relative reproductive success sets the maximum potential response of a trait to selection, known as the “opportunity for selection” (Crow, 1958). According to Fisher, the strength of selection is more accurately defined by the genetic correlation between a phenotype and fitness, though this is rarely empirically measured (Fisher, 1958). Of note for studies of sexual selection, three covariances are important: between a trait and relative mating success, between a trait and relative reproductive success, and between relative mating success and relative reproductive success (Arnold and Wade, 1984). When there is no relationship between relative mating success and relative reproductive success, no sexual selection can occur. Sexual and natural selection may oppose each other in direction.
A few studies have examined fitness consequences of psychiatric disorders. Bipolar disorder appears to reduce fecundity, yet may be associated with increased fertility at younger ages (Power et al., 2013; Jacobson, 2016; Grover et al., 2019; Hope et al., 2020). Compared to women without psychiatric disorders, affected women are at risk for several negative fitness outcomes, including recurrent miscarriage, sexually transmitted infections, and reproductive cancer (Hope et al., 2022). Higher than average anxiety, on the other hand, was associated with quadratic (U-shaped) increases in fitness with individuals showing lower than average or higher than average anxiety having more children (Jacobson and Roche, 2018). For major depressive disorder, one study showed affected individuals do not have decreased fecundity when compared to their siblings, but another showed that affected individuals have lower fecundity compared with the general population (Tondo et al., 2011; Power et al., 2013). Although the relationship between psychiatric disorders and fitness outcomes has been preliminarily examined, the association between other psychological traits and fitness outcomes warrants further study, as this relationship is key to understanding their contemporary evolution.
The “Breeder’s Equation” describes the expected response to selection (Lush, 1937):
The response to selection for any given trait (R) is that trait’s heritability multiplied by the strength of selection. Whenever h2 < 1, the effect of selection on a trait is proportionally diminished.
The multivariate extension of the Breeder’s Equation allows for estimation of response of a suite of traits to selection (Lande, 1979). This equation is especially important for hormone-mediated suites of traits:
Here, Δz̄ is the vector of responses in a suite of phenotypes, G is the G-matrix, P-1 is the phenotypic variance–covariance matrix for the traits, and S is the vector of selection differentials on those traits. The pertinent consequence of this equation for hormone-mediated suites is that the response of one trait to selection depends on selection directly on that trait in addition to selection on every other trait with which it is genetically correlated (Lande and Arnold, 1983). When one trait responds to selection on another trait with which it is genetically correlated, the process is called correlational selection. Within a suite of genetically correlated traits, direct selection on each trait may differ in strength, form, or direction. The overall direction of change for a trait in response to selection, thus, does not only depend on selection directly on that trait, but on correlational selection through other traits as well (Lande and Arnold, 1983; Arnold, 1992). To our knowledge, no studies have used the quantitative genetic framework to predict response to selection for psychiatric disorders or other psychological traits.
Hormonal pleiotropy is an important source of genetic covariance (Wittman et al., 2021). For traits involved in hormone-mediated suites, evolution of each of those traits will potentially be dependent on selection acting on multiple others. This may cause the trait of interest to respond to selection in ways not predicted by univariate models (Arnold, 1992; McGlothlin and Ketterson, 2008).
Some properties of quantitative genetic parameters are largely overlooked, but have important implications for evolutionary psychology. First, these parameters are properties of populations, not individuals. Second, all quantitative genetic parameters are specific to the age during which they are measured and can change over the life of an organism. Prior empirical work demonstrates that heritability and other quantitative genetic parameters can change significantly with age (Wilson et al., 2005). Selection may favor increases in a phenotype in young individuals and decreases in that phenotype in older individuals (Roff, 1992; Stearns, 1992). Third, these parameters are specific to the population in which they are measured. One ought not assume that heritability measured in one population will be the same as heritability measured in another. Finally, quantitative genetic parameters are specific to the generation in which they are measured and may change in response to different processes, including selection (Athanasiadis et al., 2022). Selection may change strength, form, or direction from one generation to the next and in turn alter trait heritability. For these reasons, the adaptive value of a trait in the past or future should be distinguished from current selection on that trait. This discordance is called “mismatch” (Corbett et al., 2018). For example, the neurobiological systems regulating response to reward are not adapted to stimuli from recreational drugs, and those with substance use disorders may have lower lifetime reproductive success (Nesse and Berridge, 1997; Troisi, 2001; Jacobson, 2016). Other examples of quantitative genetic predictions of hypotheses from the evolutionary psychology literature are given in Table 1. These examples illustrate how quantitative genetics may analytically bridge evolutionary theory with evolutionary psychology by allowing for empirical tests of hypotheses about how evolutionary processes shape variation in psychological traits.
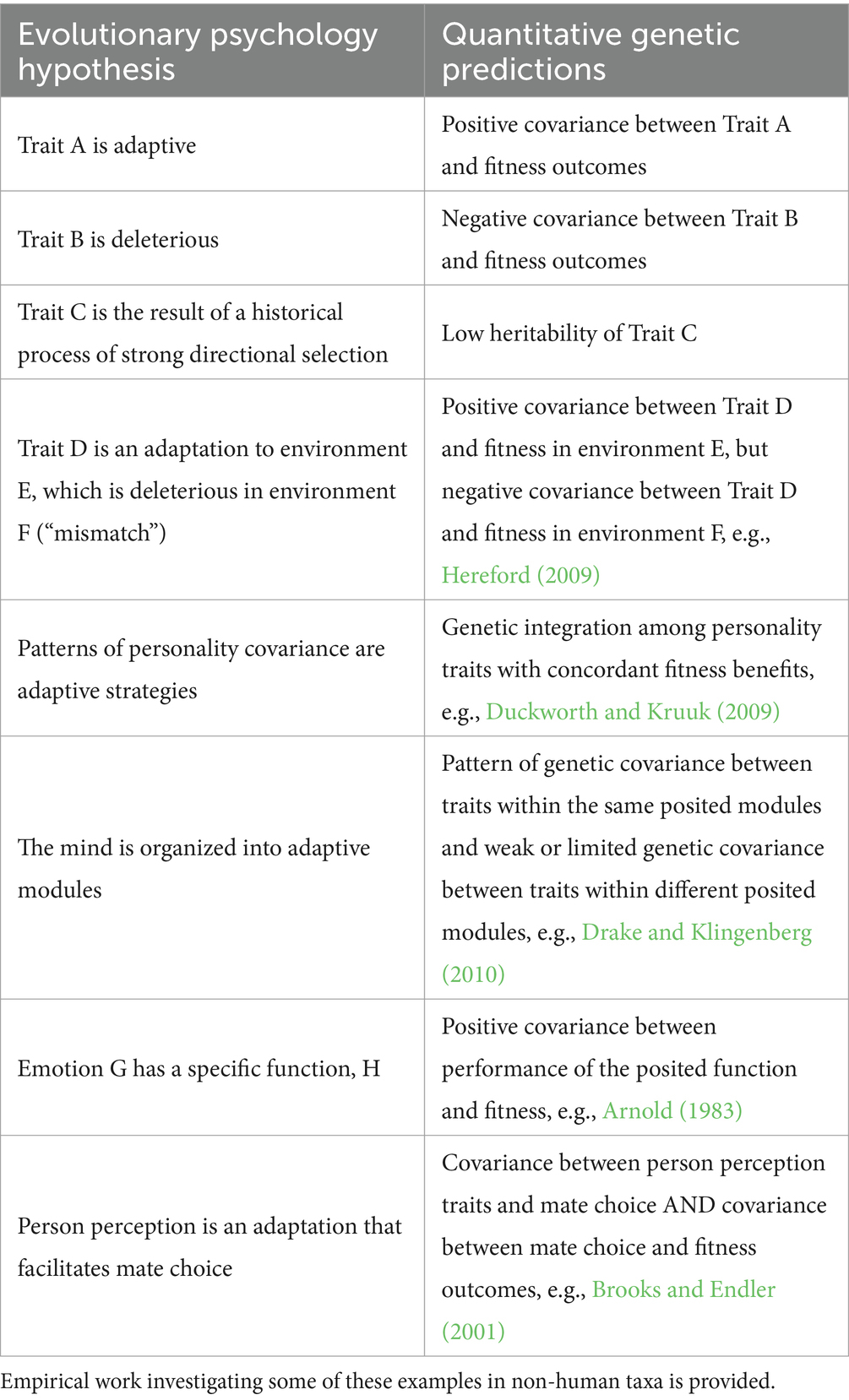
Table 1. Examples of hypotheses from evolutionary psychology and their concordant quantitative genetic predictions.
1.2 The estrogen-mediated suite of traits
Sex hormones are privileged with regard to selection because they regulate reproductive traits and thus generate strong potential for correlational selection among other traits in their suite (McGlothlin and Ketterson, 2008). In humans, sex hormones are well-studied for their effects on the development of sexual characteristics. Here, we focus on estrogen, but the principles we elucidate should be considered for other sex hormones.
Estrogen signaling, in addition to regulating growth, development, and physiology of female reproduction, is crucial for the timing of life history transitions (e.g., menarche and menopause), metabolism, immune function, adipogenesis, skeletal modeling, cardiovascular system functioning, and mood regulation (Dluzen, 2005; Kovats, 2015). Estrogen may also regulate mate preference (Gangestad and Thornhill, 2008; Jünger et al., 2018). Additionally, estrogen is a major factor in carcinogenesis, especially in cancers of the breast and female reproductive tract (Grady et al., 1995; Clemons and Goss, 2001; Kaaks et al., 2002; Rossouw et al., 2002; Yager and Davidson, 2006; Reid et al., 2017). This network of traits influenced by estrogen provides the architecture for correlational selection (Figure 1).
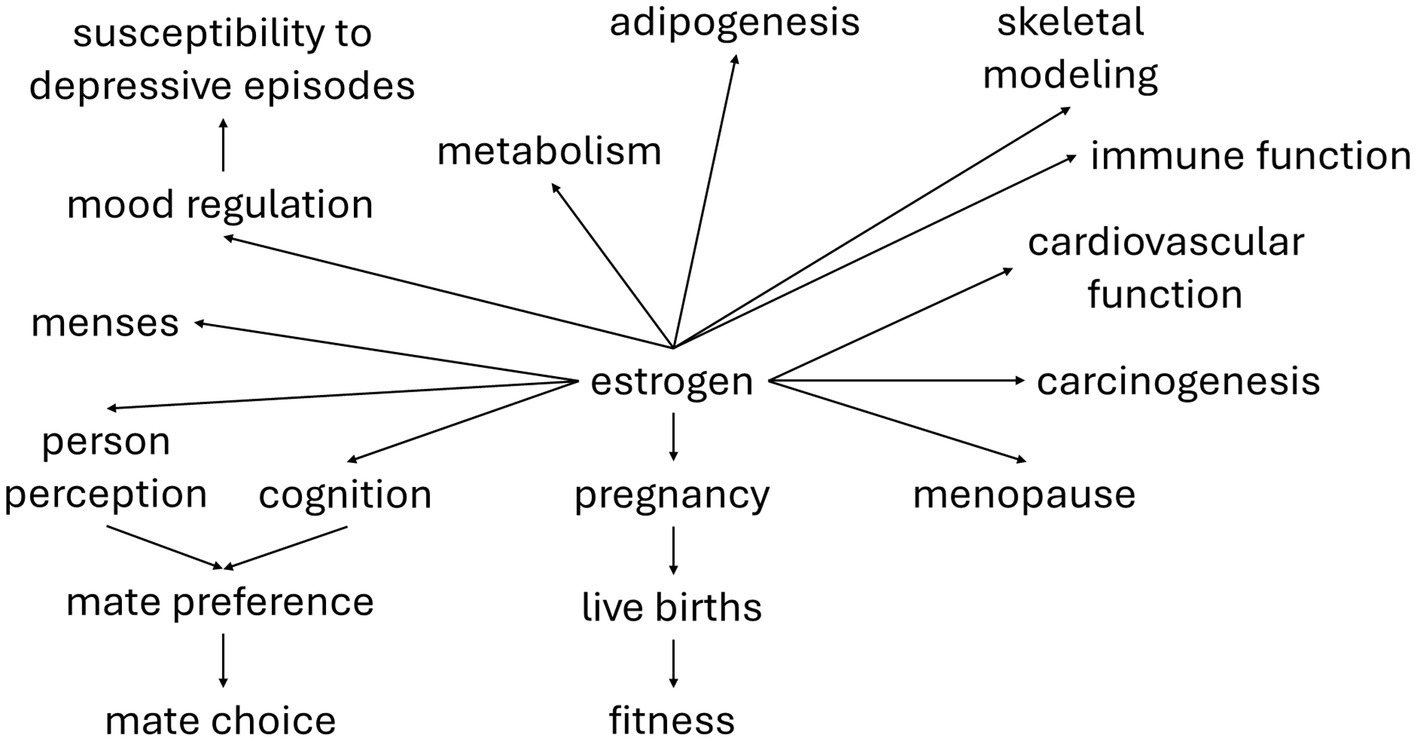
Figure 1. The pleiotropic effects of estrogen. Due to the reproductive effects of estrogen, its correlation with fitness should be quite strong, providing the basis for correlational selection on other traits that it regulates. The strength and direction of genetic correlations between traits within this estrogen-mediated suite partially determines whether the evolutionary response of individual traits is facilitated or constrained. A thorough understanding of the function of hormone-regulated traits must include consideration of this underlying genetic architecture.
Estrogen signaling accomplishes this diversity of functions by acting through several molecular mechanisms, including binding to receptors or directly to DNA to alter transcription (Bjornstrom and Sjoberg, 2005). Serum estradiol concentration is regulated by sex hormone binding globulin (Siiteri et al., 1982; Arathimos et al., 2020). Free estradiol may be important for some phenotypes, such as carcinogenesis, while fluctuations in hormone levels are important for others, such as menstrual cycles, maintenance and progression of pregnancies, and mood regulation (Deroo and Korach, 2006; Burns and Korach, 2012; Christensen et al., 2012; Hamilton et al., 2017; Gordon et al., 2019). Nuances in the mechanisms by which a suite of traits is regulated allow for partial independence in the evolution of those traits when rG < 1 (Wagner and Lynch, 2008).
Though some of the functions of estrogen are limited to women, estrogen is important in regulating several functions in men as well (Kousteni et al., 2001). This scenario is the basis for cross-sex genetic correlations (rMF), whereby evolution of trait expression in men will be linked to the evolution of those same traits in women. Cross-sex genetic correlations have been the target of intensive work in evolutionary biology due to the role that this genetic architecture can play in sexual selection (Badyaev, 2002). The major consequence of rMF is that selection on one sex will generate selection in the other. If rMF between the sexes is negative, and selection on the sexes is in opposite directions, the evolution of sex differences is expected (Lande, 1980). On the other hand, if rMF is positive and selection on the sexes is in opposite directions, the evolution of sex differences will be constrained. The long-term outcomes of sex differences depend on the strength, direction, and consistency of selection and the strength and direction of rMF (Badyaev, 2002; Walsh and Lynch, 2018).
Estrogen is implicated in several psychiatric disorders and may be partially responsible for observed sex differences. Sex differences in risk of depression, for example, emerge in puberty and continue throughout life (Kessler, 2003). This risk is mechanistically linked with fluctuations in estrogen levels, for example during pregnancy, peripartum periods, and menopause (Payne, 2003; Bennett et al., 2004; Freeman et al., 2006; O'Hara and Swain, 2009; O'Hara and McCabe, 2013). Estrogen, by regulating mood, is associated with both major depressive disorder and bipolar disorder (Halbreich and Kahn, 2001; Borrow and Cameron, 2014; Frey and Dias, 2014). Cycle-related changes in mood symptoms in both these disorders have also been reported (Payne et al., 2007). Estrogen and progesterone jointly increase vulnerability for developing anxiety disorders and influence the presentation, course, and treatment response of anxiety disorders, especially in women (Pigott et al., 2019). In schizophrenia, several possible mechanisms also associate estrogen signaling with cognitive function (McGregor et al., 2017). Estrogen receptor expression in the frontal cortex and hippocampus shows sex differences in major depressive disorder, but not in schizophrenia and bipolar disorder (Perlman et al., 2005). Sex differences in susceptibility to neurodegenerative diseases may also be related to neuroprotective effects of estrogen (Vegeto et al., 2020).
2 Discussion
When an array of phenotypes is regulated by one hormone, predicting the response to selection of a single phenotype is difficult without large sampling and detailed phenotyping efforts. Within the field of hormones and person perception, most efforts have focused on relating estrogen function individually to various phenotypes, such as mate choice or preference (Feinberg et al., 2006; Garver-Apgar et al., 2008; Lukaszewski and Roney, 2009). Large quantitative genetic studies within these fields could provide important empirical tests of theory, especially considering existing methods to construct pedigrees from already collected genetic data (Staples et al., 2014). For clinical fields, evolutionary theory can shed light on unresolved fundamental questions about the nature of psychiatric disorders (Nesse, 2023).
Hormonal regulation of person perception is a key area for understanding how psychiatric disorders evolve due to its role in sexual selection. Sex hormones are associated with variation in perception of faces, morphology, and emotions (Gangestad and Thornhill, 2008; Romero-Martinez et al., 2021). Person perception varies between populations and biological sexes, is influenced by psychiatric disorders, and changes with reproductive physiology, season, and age (Gangestad and Thornhill, 2008; Pawlowski and Sorokowski, 2008; Kohler et al., 2010, 2011; Boothroyd and Vukovic, 2019; Olderbak et al., 2019). This abundant phenotypic variation suggests ample opportunity for evolutionary processes to change the distribution of these traits from one generation to the next.
Crucially, hormonal regulation of person perception modifies the sensory processes necessary for expressing mating preference and choice, but is not equivalent to these. Mating preference and mate choice must be measured empirically, a problem which presents unique methodological challenges (Andersson and Simmons, 2006; Dougherty, 2020; Clancey et al., 2022). Variation in the perception of mates over time (e.g., across the menstrual cycle) allows for changes in mate preference, but these changes do not necessarily entail changes in mate choice, especially given variation in human mating systems (Todd et al., 2007; Schacht and Kramer, 2019). In turn, changes variation in mate choice may or may not be under natural or sexual selection depending on the relationship between mate choice and fitness (Shuster and Wade, 2003; Walsh and Lynch, 2018). For example, in many human societies, marriage is primarily an economic transaction involving the influence of an individual’s parents or family (Ingoldsby, 2006; Buunk et al., 2009). In these societies, the expression of individual preference may be facilitated or opposed by familial influence. The fitness consequences (e.g., frequency of extrapair mating) can depend on alignment of individual and familial preferences (Scelza, 2011). Quantitative genetics provides a robust framework for studying the role of hormonal regulation of person perception in sexual selection as well as the evolution of psychiatric disorders in the setting of hormone-mediated suites of traits.
More empirical work is needed to clarify several evolutionary quantitative genetic questions about hormones and person perception, such as quantification of univariate and multivariate heritability of hormone-mediated suites. For reasons outlined above, this work must include studies of different populations, ages, and generations. Most studies of heritability of clinical phenotypes do not examine these differences. The unwarranted, tacit assumption is that risky genetic loci are stable across these contexts or change on longer timescales than are clinically relevant. Given that sex-hormone mediated traits undergo large regulatory changes during puberty, these knowledge gaps are especially important targets for empirical work. Few studies have quantified contemporary selection on hormones and person perception or examined mechanisms of selection [though (see Hill et al., 2013)], which means the processes possibly driving changes in heritability are almost completely overlooked.
Overall, we have discussed beneficial and adverse consequences of sex hormone signaling, highlighting the fact that tradeoffs among various functions of hormonal suites are common. This discussion emphasizes the importance of caution in drawing conclusions about the evolution of psychological traits and psychiatric disorders from single phenotype studies. This caveat is especially important for the field of hormones and person perception due to the possibility of conflicts between sexual and natural selection. Our hope is that future work will incorporate evolutionary quantitative genetic approaches to studying adaptive hormone-mediated suites of traits.
Data availability statement
The original contributions presented in the study are included in the article/supplementary material, further inquiries can be directed to the corresponding author.
Author contributions
CG: Writing – review & editing, Writing – original draft, Visualization, Conceptualization. TK: Writing – review & editing, Supervision, Conceptualization. TP: Writing – review & editing, Supervision, Conceptualization.
Funding
The author(s) declare that no financial support was received for the research, authorship, and/or publication of this article.
Acknowledgments
The authors would like to thank J. M. West for fruitful discussion during the writing and revision of this manuscript.
Conflict of interest
The authors declare that the research was conducted in the absence of any commercial or financial relationships that could be construed as a potential conflict of interest.
Publisher’s note
All claims expressed in this article are solely those of the authors and do not necessarily represent those of their affiliated organizations, or those of the publisher, the editors and the reviewers. Any product that may be evaluated in this article, or claim that may be made by its manufacturer, is not guaranteed or endorsed by the publisher.
References
Andersson, M., and Simmons, L. W. (2006). Sexual selection and mate choice. Trends Ecol. Evol. 21, 296–302. doi: 10.1016/j.tree.2006.03.015
Arathimos, R., Millard, L. A. C., Bell, J. A., Relton, C. L., and Suderman, M. (2020). Impact of sex hormone-binding globulin on the human phenome. Hum. Mol. Genet. 29, 1824–1832. doi: 10.1093/hmg/ddz269
Arnold, S. J. (1983). Morphology, performance and fitness. Am. Zool. 23, 347–361. doi: 10.1093/icb/23.2.347
Arnold, S. J. (1992). Constraints on phenotypic evolution. Am. Nat. 140, S85–S107. doi: 10.1086/285398
Arnold, S. J., and Wade, M. J. (1984). On the measurement of natural and sexual selection: theory. Evolution 38, 709–719. doi: 10.2307/2408383
Athanasiadis, G., Meijsen, J. J., Helenius, D., Schork, A. J., Ingason, A., Thompson, W. K., et al. (2022). A comprehensive map of genetic relationships among diagnostic categories based on 48.6 million relative pairs from the Danish genealogy. Proc. Natl. Acad. Sci. USA 119, 1–9.
Badyaev, A. V. (2002). Growing apart: an ontogenetic perspective on the evolution of sexual size dimorphism. Trends Ecol. Evol. 17, 369–378. doi: 10.1016/S0169-5347(02)02569-7
Bennett, H. A., Einarson, A., Taddio, A., Koren, G., and Einarson, T. R. (2004). Prevalence of depression during pregnancy: systematic review. Obstet. Gynecol. 103, 698–709. doi: 10.1097/01.AOG.0000116689.75396.5f
Bjornstrom, L., and Sjoberg, M. (2005). Mechanisms of estrogen receptor signaling: convergence of genomic and nongenomic actions on target genes. Mol. Endocrinol. 19, 833–842. doi: 10.1210/me.2004-0486
Boake, C. R. B. (1994). Quantitative Genetic Studies of Behavioral Evolution. Chicago: University of Chicago Press.
Boothroyd, L. G., and Vukovic, J. (2019). “Mate preferences across the lifespan” in The Oxford handbook of evolutionary psychology and behavioral endocrinology. eds. L. L. M. Welling and T. K. Shackelford (New York, NY, USA: Oxford University Press).
Borrow, A. P., and Cameron, N. M. (2014). Estrogenic mediation of serotonergic and neurotrophic systems: implications for female mood disorders. Prog. Neuro-Psychopharmacol. Biol. Psychiatry 54, 13–25. doi: 10.1016/j.pnpbp.2014.05.009
Brainstorm, C., Anttila, V., Bulik-Sullivan, B., Finucane, H. K., Walters, R. K., Bras, J., et al. (2018). Analysis of shared heritability in common disorders of the brain. Science 360, 1–12.
Brooks, R., and Endler, J. A. (2001). Female guppies agree to differ: phenotypic and genetic variation in mate-choice behavior and the consequences for sexual selection. Evolution 55, 1644–1655
Burns, K. A., and Korach, K. S. (2012). Estrogen receptors and human disease: an update. Arch. Toxicol. 86, 1491–1504. doi: 10.1007/s00204-012-0868-5
Buunk, A. P., Park, J. H., and Duncan, L. A. (2009). Cultural variation in parental influence on mate choice. Cross-Cult. Res. 44, 23–40.
Christensen, A., Bentley, G. E., Cabrera, R., Ortega, H. H., Perfito, N., Wu, T. J., et al. (2012). Hormone regulation of female reproduction. Horm. Metab. Res. 44, 587–591. doi: 10.1055/s-0032-1306301
Chuffa, L. G., Lupi-Junior, L. A., Costa, A. B., Amorim, J. P., and Seiva, F. R. (2017). The role of sex hormones and steroid receptors on female reproductive cancers. Steroids 118, 93–108. doi: 10.1016/j.steroids.2016.12.011
Clancey, E., Johnson, T. R., Harmon, L. J., and Hohenlohe, P. A. (2022). Estimation of the strength of mate preference from mated pairs observed in the wild. Evolution 76, 29–41. doi: 10.1111/evo.14397
Clemons, M., and Goss, P. (2001). Estrogen and the risk of breast cancer. N. Engl. J. Med. 344, 276–285. doi: 10.1056/NEJM200101253440407
Corbett, S., Courtiol, A., Lummaa, V., Moorad, J., and Stearns, S. (2018). The transition to modernity and chronic disease: mismatch and natural selection. Nat. Rev. Genet. 19, 419–430. doi: 10.1038/s41576-018-0012-3
Crow, J. F. (1958). Some possibilities for measuring selection intensities in man. Hum. Biol. 61, 763–775.
Danchin, E., Charmantier, A., Champagne, F. A., Mesoudi, A., Pujol, B., and Blanchet, S. (2011). Beyond DNA: integrating inclusive inheritance into an extended theory of evolution. Nat. Rev. Genet. 12, 475–486. doi: 10.1038/nrg3028
Danchin, E., Pujol, B., and Wagner, R. H. (2013). The double pedigree: a method for studying culturally and genetically inherited behavior in tandem. PLoS One 8:e61254. doi: 10.1371/journal.pone.0061254
Darwin, C. (1859). On the origin of species by means of natural selection or the preservation of favoured races in the struggle for life. Edison, NJ, USA: Castle Books.
Deroo, B. J., and Korach, K. S. (2006). Estrogen receptors and human disease. J. Clin. Invest. 116, 561–570. doi: 10.1172/JCI27987
Dluzen, D. E. (2005). Unconventional effects of estrogen uncovered. Trends Pharmacol. Sci. 26, 485–487. doi: 10.1016/j.tips.2005.08.001
Dougherty, L. R. (2020). Designing mate choice experiments. Biol. Rev. Camb. Philos. Soc. 95, 759–781. doi: 10.1111/brv.12586
Drake, A. G., and Klingenberg, C. P. (2010). Large-scale diversification of skull shape in domestic dogs: disparity and modularity. Am. Nat. 175, 289–301. doi: 10.1086/650372
Duckworth, R. A., and Kruuk, L. E. (2009). Evolution of genetic integration between dispersal and colonization ability in a bird. Evolution 63, 968–977. doi: 10.1111/j.1558-5646.2009.00625.x
Falconer, D. S., and Mackay, T. F. C. (1996). Introduction to quantitative genetics. Essex, U.K.: Longman.
Feinberg, D. R., Jones, B. C., Law Smith, M. J., Moore, F. R., DeBruine, L. M., Cornwell, R. E., et al. (2006). Menstrual cycle, trait estrogen level, and masculinity preferences in the human voice. Horm. Behav. 49, 215–222. doi: 10.1016/j.yhbeh.2005.07.004
Franklin, T. B., Russig, H., Weiss, I. C., Graff, J., Linder, N., Michalon, A., et al. (2010). Epigenetic transmission of the impact of early stress across generations. Biol. Psychiatry 68, 408–415. doi: 10.1016/j.biopsych.2010.05.036
Freeman, E. W., Sammel, M. D., Lin, H., and Nelson, D. B. (2006). Associations of hormones and menopausal status with depressed mood in women with no history of depression. Arch. Gen. Psychiatry 63, 375–382. doi: 10.1001/archpsyc.63.4.375
Frey, B. N., and Dias, R. S. (2014). Sex hormones and biomarkers of neuroprotection and neurodegeneration: implications for female reproductive events in bipolar disorder. Bipolar Disord. 16, 48–57. doi: 10.1111/bdi.12151
Garver-Apgar, C. E., Gangestad, S. W., and Thornhill, R. (2008). Hormonal correlates of women's mid-cycle preference for the scent of symmetry. Evol. Hum. Behav. 29, 223–232. doi: 10.1016/j.evolhumbehav.2007.12.007
Geschwind, D. H., and Flint, J. (2015). Genetics and genomics of psychiatric disease. Science 349, 1489–1494. doi: 10.1126/science.aaa8954
Gogos, A., Ney, L. J., Seymour, N., Van Rheenen, T. E., and Felmingham, K. L. (2019). Sex differences in schizophrenia, bipolar disorder, and post-traumatic stress disorder: are gonadal hormones the link? Br. J. Pharmacol. 176, 4119–4135. doi: 10.1111/bph.14584
Gordon, J. L., Peltier, A., Grummisch, J. A., and Sykes Tottenham, L. (2019). Estradiol fluctuation, sensitivity to stress, and depressive symptoms in the menopause transition: a pilot study. Front. Psychol. 10:1319. doi: 10.3389/fpsyg.2019.01319
Grady, D., Gebretsadik, T., Kerlikowske, K., Ernster, V., and Petitti, D. (1995). Hormone replacement therapy and endometrial cancer risk: a Meta-analysis. Obstet. Gynecol. 85, 304–313. doi: 10.1016/0029-7844(94)00383-O
Grotzinger, A. D., Mallard, T. T., Akingbuwa, W. A., Ip, H. F., Adams, M. J., Lewis, C. M., et al. (2022). Genetic architecture of 11 major psychiatric disorders at biobehavioral, functional genomic and molecular genetic levels of analysis. Nat. Genet. 54, 548–559.
Grover, S., Sahoo, S., Chaudhary, S., Chakrabarti, S., Nehra, R., and Avasthi, A. (2019). Gender differences, family size and fertility rate among patients with bipolar disorder: a study from India. Psychiatry Res. 272, 562–568. doi: 10.1016/j.psychres.2018.12.156
Halbreich, U., and Kahn, L. S. (2001). Role of estrogen in the Aetiology and treatment of mood disorders. CNS Drugs 15, 797–817. doi: 10.2165/00023210-200115100-00005
Hamilton, K. J., Hewitt, S. C., Arao, Y., and Korach, K. S. (2017). Estrogen Hormone Biology. Curr. Top. Dev. Biol. 125, 109–146. doi: 10.1016/bs.ctdb.2016.12.005
Hereford, J. (2009). A quantitative survey of local adaptation and fitness trade-offs. Am. Nat. 173, 579–588. doi: 10.1086/597611
Hill, A. K., Hunt, J., Welling, L. L. M., Cárdenas, R. A., Rotella, M. A., Wheatley, J. R., et al. (2013). Quantifying the strength and form of sexual selection on men's traits. Evol. Hum. Behav. 34, 334–341. doi: 10.1016/j.evolhumbehav.2013.05.004
Hope, H., Parisi, R., Ashcroft, D. M., Williams, R., Coton, S., Kosidou, K., et al. (2020). Fertility trends of women with serious mental illness in the United Kingdom 1992-2017: a primary care cohort study using the clinical practice research datalink. J. Affect. Disord. 269, 141–147. doi: 10.1016/j.jad.2020.03.037
Hope, H., Pierce, M., Johnstone, E. D., Myers, J., and Abel, K. M. (2022). The sexual and reproductive health of women with mental illness: a primary care registry study. Arch. Womens Ment. Health 25, 585–593. doi: 10.1007/s00737-022-01214-y
Ingoldsby, B. B. (2006). “Mate selection and marriage” in Families in global and multicultural perspective. eds. B. B. Ingoldsby and S. D. Smith (Thousand Oaks, CA, USA: Sage Publications).
Iovino, M., Messana, T., De Pergola, G., Iovino, E., Dicuonzo, F., Guastamacchia, E., et al. (2018). The role of Neurohypophyseal hormones vasopressin and oxytocin in neuropsychiatric disorders. Endocr. Metab. Immune Disord. Drug Targets 18, 341–347. doi: 10.2174/1871530318666180220104900
Jablonka, E., and Raz, G. (2009). Transgenerational epigenetic inheritance: prevalence, mechanisms, and implications for the study of heredity and evolution. Q. Rev. Biol. 84, 131–176. doi: 10.1086/598822
Jacobson, L. (2014). Hypothalamic-pituitary-adrenocortical axis: neuropsychiatric aspects. Compr. Physiol. 4, 715–738. doi: 10.1002/cphy.c130036
Jacobson, N. C. (2016). Current evolutionary adaptiveness of psychiatric disorders: fertility rates, parent-child relationship quality, and psychiatric disorders across the lifespan. J. Abnorm. Psychol. 125, 824–839. doi: 10.1037/abn0000185
Jacobson, N. C., and Roche, M. J. (2018). Current evolutionary adaptiveness of anxiety: extreme phenotypes of anxiety predict increased fertility across multiple generations. J. Psychiatr. Res. 106, 82–90. doi: 10.1016/j.jpsychires.2018.10.002
Jünger, J., Kordsmeyer, T. L., Gerlach, T. M., and Penke, L. (2018). Fertile women evaluate male bodies as more attractive, regardless of masculinity. Evol. Hum. Behav. 39, 412–423. doi: 10.1016/j.evolhumbehav.2018.03.007
Kaaks, R., Lukanova, A., and Kurzer, M. S. (2002). Obesity, endogenous hormones, and endometrial cancer risk: a synthetic review. Am. Soc. Prevent. Oncol. 11, 1531–1543
Kendler, K. S. (1993). Twin studies of psychiatric illness: current status and future directions. Arch. Gen. Psychiatry 50, 905–915. doi: 10.1001/archpsyc.1993.01820230075007
Kendler, K. S. (2001). Twin studies of psychiatric illness: an update. Arch. Gen. Psychiatry 58, 1005–1014. doi: 10.1001/archpsyc.58.11.1005
Kessler, R. (2003). Epidemiology of women and depression. J. Affect. Disord. 74, 5–13. doi: 10.1016/S0165-0327(02)00426-3
Klein, S. L. (2000). The effects of hormones on sex differences in infection: from genes to behavior. Neurosci. Biobehav. Rev. 24, 627–638. doi: 10.1016/S0149-7634(00)00027-0
Kohler, C. G., Hoffman, L. J., Eastman, L. B., Healey, K., and Moberg, P. J. (2011). Facial emotion perception in depression and bipolar disorder: a quantitative review. Psychiatry Res. 188, 303–309. doi: 10.1016/j.psychres.2011.04.019
Kohler, C. G., Walker, J. B., Martin, E. A., Healey, K. M., and Moberg, P. J. (2010). Facial emotion perception in schizophrenia: a meta-analytic review. Schizophr. Bull. 36, 1009–1019. doi: 10.1093/schbul/sbn192
Kousteni, S., Bellido, T., Plotkin, L. I., O'Brien, C. A., Bodenner, D. L., Han, L., et al. (2001). Nongenotropic, sex-nonspecific signaling through the estrogen or androgen receptors: dissociation from transcriptional activity. Cell 104, 719–730
Kovats, S. (2015). Estrogen receptors regulate innate immune cells and signaling pathways. Cell. Immunol. 294, 63–69. doi: 10.1016/j.cellimm.2015.01.018
Kruuk, L. E. (2004). Estimating genetic parameters in natural populations using the "animal model". Philos. Trans. R. Soc. Lond. Ser. B Biol. Sci. 359, 873–890. doi: 10.1098/rstb.2003.1437
Kruuk, L. E., and Hadfield, J. D. (2007). How to separate genetic and environmental causes of similarity between relatives. J. Evol. Biol. 20, 1890–1903. doi: 10.1111/j.1420-9101.2007.01377.x
Lande, R. (1979). Quantitative genetic analysis of multivariate evolution, applied to brain: body size allometry. Evolution 33, 402–416. doi: 10.1111/j.1558-5646.1979.tb04694.x
Lande, R. (1980). Sexual dimorphism, sexual selection and adaptation in polygenic characters. Evolution 34, 292–305. doi: 10.1111/j.1558-5646.1980.tb04817.x
Lande, R., and Arnold, S. J. (1983). The measurement of selection on correlated characters. Evolution 37, 1210–1226. doi: 10.2307/2408842
Lukaszewski, A. W., and Roney, J. R. (2009). Estimated hormones predict women’s mate preferences for dominant personality traits. Personal. Individ. Differ. 47, 191–196. doi: 10.1016/j.paid.2009.02.019
Lynch, M., and Walsh, B. (1998). Genetics and analysis of quantitative traits. Sunderland, MA, USA: Sinauer Associates, Inc.
Mayr, E., and Provine, W. (1998). The evolutionary synthesis: Perspectives on the unification of biology. Cambridge, MA, USA: Harvard University Press.
McGlothlin, J. W., and Ketterson, E. D. (2008). Hormone-mediated suites as adaptations and evolutionary constraints. Philos. Trans. R. Soc. Lond. Ser. B Biol. Sci. 363, 1611–1620. doi: 10.1098/rstb.2007.0002
McGregor, C., Riordan, A., and Thornton, J. (2017). Estrogens and the cognitive symptoms of schizophrenia: possible neuroprotective mechanisms. Front. Neuroendocrinol. 47, 19–33. doi: 10.1016/j.yfrne.2017.06.003
Naughton, M., Dinan, T. G., and Scott, L. V. (2014). Corticotropin-releasing hormone and the hypothalamic-pituitary-adrenal axis in psychiatric disease. Handb. Clin. Neurol. 124, 69–91. doi: 10.1016/B978-0-444-59602-4.00005-8
Nesse, R. M. (2023). Evolutionary psychiatry: foundations, progress and challenges. World Psychiatry 22, 177–202. doi: 10.1002/wps.21072
Nesse, R. M., and Berridge, K. C. (1997). Psychoactive drug use in evolutionary perspective. Science 278, 63–66. doi: 10.1126/science.278.5335.63
O'Hara, M. W., and McCabe, J. E. (2013). Postpartum depression: current status and future directions. Annu. Rev. Clin. Psychol. 9, 379–407. doi: 10.1146/annurev-clinpsy-050212-185612
O'Hara, M. W., and Swain, A. M. (2009). Rates and risk of postpartum depression—a meta-analysis. Int. Rev. Psychiatry 8, 37–54.
Olderbak, S., Wilhelm, O., Hildebrandt, A., and Quoidbach, J. (2019). Sex differences in facial emotion perception ability across the lifespan. Cogn. Emot. 33, 579–588. doi: 10.1080/02699931.2018.1454403
Pawlowski, B., and Sorokowski, P. (2008). Men's attraction to women's bodies changes seasonally. Perception 37, 1079–1085. doi: 10.1068/p5715
Payne, J. L. (2003). The role of estrogen in mood disorders in women. Int. Rev. Psychiatry 15, 280–290. doi: 10.1080/0954026031000136893
Payne, J. L., Roy, P. S., Murphy-Eberenz, K., Weismann, M. M., Swartz, K. L., McInnis, M. G., et al. (2007). Reproductive cycle-associated mood symptoms in women with major depression and bipolar disorder. J. Affect. Disord. 99, 221–229. doi: 10.1016/j.jad.2006.08.013
Perlman, W. R., Tomaskovic-Crook, E., Montague, D. M., Webster, M. J., Rubinow, D. R., Kleinman, J. E., et al. (2005). Alteration in estrogen receptor alpha mRNA levels in frontal cortex and hippocampus of patients with major mental illness. Biol. Psychiatry 58, 812–824. doi: 10.1016/j.biopsych.2005.04.047
Pigott, T. A., Duran, A. N., Jalnapurkar, I., Kimm, T., Linscheid, S., and Allen, M. K. (2019). “Sex differences in anxiety disorders” in The Oxford handbook of evolutionary psychology and behavioral endocrinology. eds. L. L. M. Welling and T. K. Shackelford (New York, NY, USA: Oxford University Press).
Power, R. A., Kyaga, S., Uher, R., MacCabe, J. H., Langstrom, N., Landen, M., et al. (2013). Fecundity of patients with schizophrenia, autism, bipolar disorder, depression, anorexia nervosa, or substance abuse vs their unaffected siblings. JAMA Psychiatry 70, 22–30. doi: 10.1001/jamapsychiatry.2013.268
Price, G. R. (1972). Extension of covariance selection mathematics. Ann. Hum. Genet. 35, 485–490. doi: 10.1111/j.1469-1809.1957.tb01874.x
Reid, B. M., Permuth, J. B., and Sellers, T. A. (2017). Epidemiology of ovarian cancer: a review. Cancer Biol. Med. 14, 9–32. doi: 10.20892/j.issn.2095-3941.2016.0084
Romero-Martinez, A., Sarrate-Costa, C., and Moya-Albiol, L. (2021). A systematic review of the role of oxytocin, cortisol, and testosterone in facial emotional processing. Biology (Basel) 10, 1–34. doi: 10.3390/biology10121334
Rossouw, J. E., Anderson, G. L., and Prentice, R. L. (2002). Investing in healthy postmenopausal women: principal results from the Women’s health initiative randomized controlled trial. JAMA 288, 321–333.
Scelza, B. A. (2011). Female choice and extra-pair paternity in a traditional human population. Biol. Lett. 7, 889–891. doi: 10.1098/rsbl.2011.0478
Schacht, R., and Kramer, K. L. (2019). Are we monogamous? A review of the evolution of pair-bonding in humans and its contemporary variation cross-culturally. Front. Ecol. Evol. 7:230. doi: 10.3389/fevo.2019.00230
Schiller, C. E., Meltzer-Brody, S., and Rubinow, D. R. (2015). The role of reproductive hormones in postpartum depression. CNS Spectr. 20, 48–59. doi: 10.1017/S1092852914000480
Shuster, S. M., and Wade, M. J. (2003). Mating systems and strategies. Princeton, NJ, USA: Princeton University Press.
Siiteri, P. K., Murai, J. T., Hammond, G. L., Nisker, J. A., Raymoure, W. J., and Kuhn, R. W. (1982). The serum transport of steroid hormones. Recent Prog. Horm. Res. 38, 457–510
Staples, J., Qiao, D., Cho, M. H., and Silverman, E. K.G. University of Washington Center for Mendelian, D.A. Nickerson, and J.E. Below (2014). PRIMUS: rapid reconstruction of pedigrees from genome-wide estimates of identity by descent. Am. J. Hum. Genet. 95, 553–564.
Stopher, K. V., Walling, C. A., Morris, A., Guinness, F. E., Clutton-Brock, T. H., Pemberton, J. M., et al. (2012). Shared spatial effects on quantitative genetic parameters: accounting for spatial autocorrelation and home range overlap reduces estimates of heritability in wild red deer. Evolution 66, 2411–2426. doi: 10.1111/j.1558-5646.2012.01620.x
Sullivan, P. F., and Geschwind, D. H. (2019). Defining the genetic, genomic, cellular, and diagnostic architectures of psychiatric disorders. Cell 177, 162–183. doi: 10.1016/j.cell.2019.01.015
Thomson, C. E., Winney, I. S., Salles, O. C., and Pujol, B. (2018). A guide to using a multiple-matrix animal model to disentangle genetic and nongenetic causes of phenotypic variance. PLoS One 13:e0197720. doi: 10.1371/journal.pone.0197720
Todd, P. M., Penke, L., Fasolo, B., and Lenton, A. P. (2007). Different cognitive processes underlie human mate choices and mate preferences. Proc. Natl. Acad. Sci. USA 10, 15011–15016.
Tondo, L., Lepri, B., and Baldessarini, R. J. (2011). Reproduction among 1975 Sardinian women and men diagnosed with major mood disorders. Acta Psychiatr. Scand. 123, 283–289.
Troisi, A. (2001). Harmful effects of substance abuse: a Darwinian perspective. Funct. Neurol. 16, 237–243
United Nations (2022). Department of economic and social affairs, world population prospects 2022: Summary of results. New York, United Nations Publication: UN DESA.
Vegeto, E., Villa, A., Della Torre, S., Crippa, V., Rusmini, P., Cristofani, R., et al. (2020). The role of sex and sex hormones in neurodegenerative diseases. Endocr. Rev. 41, 273–319. doi: 10.1210/endrev/bnz005
Viken, R. J., and Rose, R. J. (2007). Genetic variation and covariation in the original and restructured clinical scales of the MMPI. J. Abnorm. Psychol. 116, 842–847. doi: 10.1037/0021-843X.116.4.842
Vukasovic, T., and Bratko, D. (2015). Heritability of personality: a meta-analysis of behavior genetic studies. Psychol. Bull. 141, 769–785. doi: 10.1037/bul0000017
Wagner, G. P., and Lynch, V. J. (2008). The gene regulatory logic of transcription factor evolution. Trends Ecol. Evol. 23, 377–385. doi: 10.1016/j.tree.2008.03.006
Walsh, B., and Lynch, M. (2018). Evolution and selection of quantitative traits. New York: Oxford University Press.
Wilson, A. J., Kruuk, L. E., and Coltman, D. W. (2005). Ontogenetic patterns in heritable variation for Body size: using random regression models in a wild ungulate population. Am. Nat. 166, e177–e192. doi: 10.1086/497441
Wittman, T. N., Robinson, C. D., McGlothlin, J. W., and Cox, R. M. (2021). Hormonal pleiotropy structures genetic covariance. Evol Lett 5, 397–407. doi: 10.1002/evl3.240
Yager, J. D., and Davidson, N. E. (2006). Estrogen carcinogenesis in breast Cancer. N. Engl. J. Med. 354, 270–282. doi: 10.1056/NEJMra050776
Yim, I. S., Tanner Stapleton, L. R., Guardino, C. M., Hahn-Holbrook, J., and Dunkel Schetter, C. (2015). Biological and psychosocial predictors of postpartum depression: systematic review and call for integration. Annu. Rev. Clin. Psychol. 11, 99–137. doi: 10.1146/annurev-clinpsy-101414-020426
Keywords: evolutionary psychiatry, hormones, quantitative genetics, person perception, evolutionary theory
Citation: Gurguis CI, Kimm TS and Pigott TA (2024) Perspective: the evolution of hormones and person perception—a quantitative genetic framework. Front. Psychol. 15:1395974. doi: 10.3389/fpsyg.2024.1395974
Edited by:
Lisa L. M. Welling, Oakland University, United StatesReviewed by:
Joelson Moreno Brito De Moura, Federal Rural University of Pernambuco, BrazilCopyright © 2024 Gurguis, Kimm and Pigott. This is an open-access article distributed under the terms of the Creative Commons Attribution License (CC BY). The use, distribution or reproduction in other forums is permitted, provided the original author(s) and the copyright owner(s) are credited and that the original publication in this journal is cited, in accordance with accepted academic practice. No use, distribution or reproduction is permitted which does not comply with these terms.
*Correspondence: Christopher I. Gurguis, Q2hyaXN0b3BoZXIuR3VyZ3Vpc0B1dGgudG1jLmVkdQ==