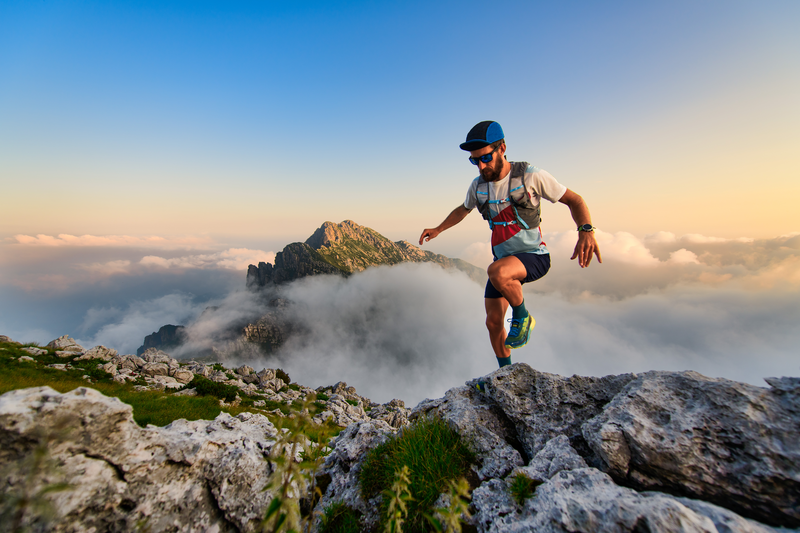
95% of researchers rate our articles as excellent or good
Learn more about the work of our research integrity team to safeguard the quality of each article we publish.
Find out more
ORIGINAL RESEARCH article
Front. Psychol. , 12 June 2024
Sec. Emotion Science
Volume 15 - 2024 | https://doi.org/10.3389/fpsyg.2024.1391723
This article is part of the Research Topic How Do Affective Stimuli Impact Actions? Unveiling the Relationship Between Emotional Stimuli and Motor Behavior View all 10 articles
Efficient inhibitory control in the context of prepotent actions is vital. However, such action inhibition may be profoundly influenced by affective states. Interestingly, research indicates that action control can be either impaired or improved by emotional stimuli. Thus, a great deal of confusion surrounds our knowledge of the complex dynamics subtending emotions and action control. Here, we aimed to investigate whether negative stimuli, even when non-consciously presented and task-irrelevant, can affect action control relative to neutral stimuli. Additionally, we tested whether individual differences in intracortical excitability may predict action control capabilities. To address these issues, we asked participants to complete a modified version of the Stop Signal Task (SST) in which fearful or neutral stimuli were subliminally presented before the go signals as primes. Moreover, we assessed participants’ resting-state corticospinal excitability, short intracortical inhibition (SICI), and intracortical facilitation (ICF). Results demonstrated better action control capabilities when fearful stimuli were subliminally presented and interindividual SICI predicted stronger action inhibition capabilities. Taken together, these results shed new light on the intricate dynamics between action, consciousness, and motor control, suggesting that intracortical measures can be used as potential biomarkers of reduced motor inhibition in research and clinical settings.
Cognitive neuroscience has long been fascinated by the intricate ways in which emotions influence complex cognitive functions, including action inhibition. From an operational point of view, the examination of action inhibition typically involves the utilization of the stop-signal task (SST), which is specifically devised to offer a sensitive assessment of the duration it takes for the brain to inhibit or suppress inappropriate motor responses (Logan, 1994; Verbruggen et al., 2019). In an SST, participants are requested to respond to a “go” stimulus. Nevertheless, on certain occasions, the go stimulus is succeeded by a “stop” signal, necessitating participants to refrain from continuing the ongoing action. In assessing the participant’s performance on the SST, the stop signal reaction time (SSRT), an indicator of inhibition, is calculated according to the concept proposed by Logan et al. (1984). Several studies using SST have aimed to uncover the influence of emotional stimuli on action control capabilities. However, findings are inconsistent, as these investigations collectively reveal that emotion can either impair, facilitate, or have no effect on action control (for a review see Battaglia et al., 2021). A factor that might have influenced the divergent outcomes is the varied functions of the emotional stimulus, such as being presented as a stop signal, go signal, or as a prime before the go signal. Additionally, the significance of the emotional stimulus for the SST plays a role. Emotional stimuli can be either task-relevant, necessitating explicit discrimination of emotional stimuli (e.g., Ding et al., 2021) or task-irrelevant (i.e., not requiring emotion discrimination; e.g., Sagaspe et al., 2011). Experiments employing SSTs that required emotion discrimination found that emotional stimuli produce worse inhibition capacity compared to neutral stimuli (i.e., longer SSRT; Song et al., 2016; Ding et al., 2020), while an SST with task-irrelevant emotional stimuli yielded varied outcomes with respect to inhibitory performance (Sagaspe et al., 2011; Pawliczek et al., 2013; Rebetez et al., 2015; Derntl and Habel, 2017). Emotional stimuli presented as primes have been reported to interfere with the action control capabilities, lengthening the SSRT (Verbruggen and Houwer, 2007; Krypotos et al., 2011; Kalanthroff et al., 2013). On the other hand, emotional stimuli presented as stop signals were found to facilitate action control (Pessoa et al., 2012; Senderecka, 2016, 2018). Similarly, in our recent works (Battaglia et al., 2022a,b), we demonstrated that emotional stimuli presented as stop signals can increase action inhibition capabilities (i.e., shorter SSRT) relative to neutral control stimuli. Overall, it seems that the detrimental effect obtained from presenting task-relevant emotional stimuli (Calbi et al., 2022; Mancini et al., 2022) or task-irrelevant emotional stimuli as primes can be elucidated through the attentional account proposed by Schimmack (2005), suggesting that emotional stimuli attract attention and consequently disrupt the execution of the ongoing task (Pessoa, 2009; Pessoa et al., 2012). A way to test the attentional account is to investigate whether presenting subliminal task-irrelevant emotional stimuli as a prime is still able to impact high cognitive processes, such as action control. A significant portion of cognitive processes can transpire non-consciously and influence behavior (Merikle et al., 2001; Eimer and Schlaghecken, 2003; Kunde, 2003; Lau and Passingham, 2007). In particular, there is now considerable evidence that the processing of potentially dangerous stimuli can occur even outside conscious awareness (Morris et al., 1998; Whalen et al., 1998; Diano et al., 2017). Behavioral studies have provided evidence that undetected fearful faces and bodies can influence the assessment of a subsequent visible probe stimulus (Yang et al., 2011). This influence extends to various cognitive processes, such as, among others, the orienting of covert spatial attention (Carlson and Reinke, 2008) as well as the recognition of happy faces (Tamietto and de Gelder, 2008). Interestingly, the influence of non-conscious stimuli on motor responses has already been investigated (Engelen et al., 2018) whereas the role of emotion awareness in the control of action remains unresolved. Here, we investigated this issue by presenting our participants with a modified version of our SST (Battaglia et al., 2022b) in which the same emotional (i.e., fearful) and neutral body postures previously presented as stop signals were now briefly flashed (~17 ms) and sandwich-masked before the go signals. If emotionally salient negative stimuli, even though non-consciously presented, are able to impede action control, we should expect longer SSRT in trials with negative stimuli compared to neutral stimuli presented as primes. Contrary to this idea, the fact that the negative stimuli are presented subliminally may enhance action control (i.e., faster SSRT), is consistent with the freezing account, which suggests that emotional stimuli might lead to a temporary suspension of all task-unrelated ongoing activities, thereby enhancing action control (Ohman et al., 2001; Flykt, 2006).
Moreover, prior studies employing Transcranial Magnetic Stimulation (TMS) during the SST indicate that the suppression of a motor response relies on contextual modulation of corticospinal excitability and intracortical inhibition within the primary motor cortex (M1; Duque et al., 2017). While motor-evoked potentials (MEPs) provide a reliable measure of corticospinal excitability, paired-pulse TMS is administered to directly assess modulations of intracortical excitability within M1. To do this, a conditioning TMS pulse below the threshold intensity needed to elicit an MEP is followed at short interstimulus intervals (ISIs) by a suprathreshold test TMS pulse eliciting a MEP. Certainly, at ISIs of 1–5 ms, the conditioning pulse induces a reduction in the MEP elicited by the test pulse, denoted as short intracortical inhibition (SICI). Conversely, longer ISIs in the range of 7–20 milliseconds lead to MEP facilitation, recognized as intracortical facilitation (ICF). Specifically, it is theorized that SICI and ICF primarily represent the activation of low-threshold inhibitory interneurons mediated by gamma-aminobutyric acid (GABA) (Ziemann et al., 1996; Di Lazzaro et al., 2000; Ilic et al., 2002) and glutamatergic interneurons (Nakamura et al., 1997; Ziemann, 2003), respectively. Notably, SICI and ICF are modulated by the observation of emotionally relevant stimuli (Borgomaneri et al., 2015b,c, 2017) and interindividual differences in SICI predict better action suppression (He et al., 2019; Chowdhury et al., 2019a,b; Tran et al., 2020; Ding et al., 2021; Loomes et al., 2023). Therefore, here we additionally tested whether action control in the context of subliminally presented emotional stimuli can be influenced by individual differences in resting-state intracortical measures of motor excitability. Our investigation aims to provide new insights into the intricate relationship between emotions, consciousness, and action control, suggesting potentially useful biomarkers for assessing deficits in action control in the psychiatric domain, in which several pathologies suffer deficits in action control in an emotional context (Battaglia et al., 2021; Di Gregorio and Battaglia, 2024).
In this study, 46 right-handed, healthy individuals with normal or corrected-to-normal vision participated. All participants completed the SST. We organized the experiment into two distinct groups. The first group, comprising 30 participants [Behavioral Group; mean age = 23.43, standard deviation (S.D.) = 3.32, 16 females] completed our previously published modified version of the SST (Battaglia et al., 2022b). The second group (Neurophysiological Group; mean age = 23.60, S.D. = 2.10, 12 females) included 20 participants. Four participants in the Neurophysiological group were excluded because of technical failures either in the SST or in the neurophysiological recording. Participants in this group performed the same SST as the Behavioral Group and additionally underwent neurophysiological measurements, such as SICI and ICF. All participants were confirmed as right-handed using the Edinburgh Handedness Inventory (Oldfield, 1971). Participants were uninformed about the objectives of the experiment and reported no history of neurological or psychiatric disorders, visual impairments, medication usage, or any contraindications to TMS (Rossi et al., 2021). The sample size for the Behavioral group was determined through power analysis, revealing that a total of 30 participants is required to achieve a statistical power (1−β) of 0.99 (two-tailed α = 0.01; effect size f = 0.40) (similar effect sizes were reported in studies such as Verbruggen and Houwer, 2007; Krypotos et al., 2011) number of measurements = 2; correlation = 0.5, analysis performed with G*Power software (Faul et al., 2007). Another power analysis determined that a sample size of 20 participants is required to attain a statistical power (1−β) of 0.90 (two-tailed α = 0.05; r = 0.65) in the Neurophysiological group (see for example Chowdhury et al., 2018; Loomes et al., 2023). The two groups were matched for age [t(42) = −0.08, p = 0.93; d = 0.03] and gender [χ2 (1, N = 46) = 0.03, p = 0.86]. Furthermore, considering the observed impact of personality traits such as trait anxiety (Avila and Parcet, 2001; Neo et al., 2011; Toh and Yang, 2020; Hsieh et al., 2022) and impulsivity (Logan et al., 1997; Avila and Parcet, 2001; Bari and Robbins, 2013; Pawliczek et al., 2013) on the inhibitory control, we conducted an additional examination. Specifically, we investigated whether these personality traits play a role in influencing action control when emotionally negative stimuli are presented as primes (i.e., before the Go stimulus). Subjective anxiety levels were assessed using the State–Trait Anxiety Inventory (STAI; Trait-scale-Y2) (Spielberger et al., 1970) while subjective impulsivity levels were measured with the Barratt Impulsiveness Scale-11 (BIS-11) (Patton et al., 1995). The STAI-Y2 comprises a 20-item self-report questionnaire that gages anxiety frequency. The BIS-11 is a self-report questionnaire with 30 items, evaluating both impulsive and non-impulsive behaviors. The two groups did not show any significant difference in terms of anxiety [STAI-Y2: t(44) = 0.09, p = 0.92, d = 0.03] but a difference was found in terms of impulsivity scores [BIS-11: t(44) = 3.57, p < 0.01, d = 1.08] in which the Behavioral Group showed a higher score than the Neurophysiological Group (Behavioral Group: mean = 70.20, S.D. = 7.20; Neurophysiological Group: mean = 62.60, S.D. = 6.19). However, such a difference did not affect our findings (see the Results section).
Data collection was conducted anonymously, with all participants providing their informed consent before engaging in the task. The study was conducted in accordance with the ethical principles of the World Medical Association Declaration of Helsinki and was approved by the Bioethical Committee of the University of Bologna.
In this study, all participants engaged in the same behavioral task followed by the awareness questionnaires and the assessment of personality traits. Only the Neurophysiological Group underwent an additional TMS session (at the beginning of the experimental session), in which neurophysiological parameters such as single-pulse MEPs, SICI, and ICF were measured at rest, to assess corticospinal excitability, short-interval intracortical inhibition, and intracortical facilitation, respectively.
The SST required participants to execute a basic reaction time (RT) task, incorporating both Go and Stop-trials, a methodology grounded in foundational work (Logan, 1994; Verbruggen and Logan, 2008). Typically, in SSTs, participants respond to Go stimuli (e.g., pressing left for a left-pointing arrow, right for a right-pointing arrow) but must inhibit their response when a Stop signal, indicated here as “XX,” appears following a variable delay (see Figure 1).
The task commenced with a preliminary practice block of 32 trials, succeeded by four experimental blocks. Each block consisted of 64 trials, with a distribution of 75% Go-trials (48 trials) and 25% Stop-trials (16 trials), cumulatively amounting to 256 trials. Every trial began with a black dot centered on a white screen, serving as a fixation point for 250 ms. This was followed by a noise-like pattern mask (see Figure 2), displayed for 70 ms, created using custom image segmentation software. After this mask, an image of a body (either expressing fear or neutral emotion) was flashed briefly (~17 ms) and was immediately replaced by the same mask stimulus (200 ms). The body postures, sourced from a validated database ensured that arousal, valence, and implied motion were matched (Borgomaneri et al., 2012, 2015a,b, 2017, 2020a,c, 2024), were presented in both Go and Stop-trials as prime stimuli. After the second mask, a blank screen appeared for 500 ms before the presentation of the Go signal (i.e., the arrow). In Go-trials, participants responded quickly and accurately to the arrow direction, visible for 70 ms. After the Go signal, a blank screen appeared until the end of the trial. The trial ended at the participant’s response or 1,500 ms after the Go signal. Conversely, in Stop-trials, participants were instructed to withhold their response upon the appearance of the Stop signal, displayed until the participant’s response or 1,500 ms after the Go signal, following a variable Stop-signal delay (SSD; i.e., the time between the go and the stop signals presentation) with respect to the Go signal onset. The initial SSD was set at 250 ms, and was dynamically adjusted for each trial using a staircase procedure (Band et al., 2003; Matzke et al., 2018; Verbruggen et al., 2019), targeting a 50% success rate in Stop-trials. Importantly, separate staircases were computed for each specific condition (fearful and neutral prime stimuli). The staircase was independent within-subject, as the SSD was adjusted individually based on performance in 50 ms increments (ranging from 50 to 650 ms), separately for emotional and neutral prime trials. In particular, if participants successfully inhibited their response on a Stop-trial, the SSD was increased by 50 ms on a subsequent Stop-trial, while if they failed to withhold their motor response, the SSD was reduced by 50 ms on a subsequent Stop-trial. Finally, the next trial was presented after a 500 ms interval. Participants were instructed to respond as quickly and accurately as possible to the arrow and were asked to inhibit their response upon viewing a stimulus that followed the initial Go-signal that appeared on the screen. However, they were also instructed that sometimes it might not be possible to successfully inhibit their response and, in such cases, they should continue to perform the task irrespective of having made an error (Pessoa et al., 2012; Verbruggen et al., 2019). Moreover, participants were instructed not to hesitate or decelerate in order to minimize the likelihood of stopping. In general, our SST was crafted in accordance with the guidelines provided by Verbruggen et al. (2019). After the task, we assessed participants’ abilities to process the 17 ms prime bodies. We evaluated subjective awareness of the prime bodies using targeted questions of the priming phase (Sweeny et al., 2009). The participants were asked to respond to the following questions: (1) “Did you see anything other than the arrow and the crosses?” (2) “Was there a stimulus just before the arrow, and if so, could you identify what it was?” (3) “Did you see a body?” (4) “What posture did it have?” A report acknowledging awareness of the presence of a body in a neutral (i.e., running) and a negative (i.e., fearful) posture was considered as an indication that the prime presence was perceived. Upon completing the subjective awareness questionnaire, participants were then asked to fill out the personality traits questionnaires.
Following the completion of the behavioral tasks, the Neurophysiological Group underwent the electrode montage setup, detection of optimal scalp position, and measurement of resting motor threshold (rMT). To investigate motor excitability, MEPs generated by TMS applied to the left M1 were captured from the right first dorsal interosseus (FDI) muscle. A Biopac MP-35 (Biopac, United States) electromyograph, was utilized for recording. The electromyogram (EMG) signals underwent band-pass filtering (30–500 Hz), were sampled at a rate of 5 kHz, digitized, and then stored on a computer for subsequent offline analysis. A belly-tendon montage was used, and pairs of silver-chloride surface electrodes were positioned, with ground electrodes on the wrist. A figure-of-eight coil, connected to a Magstim Bistim2 stimulator (Magstim, Whitland, Dyfed, United Kingdom), was then positioned over the M1. The intersection of the coil was placed tangentially to the scalp with the handle pointing backward and laterally at a 45° angle away from the midline. In this way, the current induced in the neural tissue was directed approximately perpendicular to the line of the central sulcus, optimal for trans-synaptic activation of the corticospinal pathways (Brasil-Neto et al., 1992; Mills et al., 1992). Employing a slightly suprathreshold stimulus intensity, the coil was moved over the left hemisphere to identify the optimal position that elicited maximal MEPs in the contralateral FDI muscle. Subsequently, the identified optimal position of the coil was marked on the scalp with a pen to maintain accurate coil placement throughout the experiment. The rMT was defined as the minimal intensity of stimulator output that generated MEPs with an amplitude of at least 50 μV with 50% probability, determined by approximately 20 pulses (Rossini et al., 1994). The absence of voluntary contraction was visually confirmed throughout the experiment. If muscle tension was observed, the experiment was momentarily paused, and the subject was instructed to relax.
Motor-evoked potentials were recorded in three sessions: Single pulse (SP), SICI, ICF. During the SP session, intensity was set to evoke MEPs with a peak-to-peak amplitude of ~1.0 mV. During the paired-pulse TMS paradigm, SICI and ICF were measured using an established protocol (Kujirai et al., 1993; Ziemann et al., 1996). The conditioning (CS) and test (TS) stimuli were administered using the same coil. The intensity of the CS was set at 80% of the rMT, a level at which MEPs were consistently not induced. The TS intensity matched that used in the SP session. Two ISIs, specifically 3 and 12 ms, were chosen, as these are commonly employed for investigating SICI and ICF circuits, respectively (Kujirai et al., 1993; Ziemann et al., 1996; Borgomaneri et al., 2015a,b, 2017).
In this investigation, reactive inhibition indices were computed for each specific condition (fearful and neutral prime stimuli) within the SST, employing the SSRT measurement, as delineated in prior work by Battaglia et al. (2022b), and in alignment with the race model concept proposed by Logan et al. (1984). Before delving into SSRT analysis, we confirmed the reliability of participant performance on the SST by assessing the inhibition rate, which is expected to hover around the 50% mark, as per the guidelines of Band et al. (2003), Logan et al. (2014), Matzke et al. (2018), and Verbruggen et al. (2019). Adhering to the methodology advocated by Verbruggen et al. (2013), our SSRT estimation employed the integration method, incorporating Go-trial omissions into the calculations. This method involves integrating the distribution of RTs from Go-trials to pinpoint the moment when the cumulative distribution matches the probability of responding post-stop-signal presentation, denoted as “p(respond| signal).” The conclusion of the stopping process is indicated at the point on the RT distribution curve where the integral equals this probability. Specifically, the ending time of the stop process corresponds to the nth RT, where n equals the total number of RTs in the Go-trial RT distribution multiplied by “p(respond|signal).” For determining the nth RT, all responses in Go-trials are considered, including those with choice errors and premature responses. It is crucial to note that omissions (i.e., Go-trials where participants did not respond before the trial’s end) are assigned the maximum RT to account for the absence of a response. Furthermore, premature responses in unsuccessful Stop-trials (responses executed before the presentation of the Stop-signal) are included in the calculation of “p(respond| signal)” and the mean SSD. This approach, known as the integration method, is recognized for producing the most reliable and least biased estimation of the SSRT (for a comprehensive review and detailed explanation of this methodology, see Verbruggen et al., 2019). For the analysis, we utilized custom scripts developed in MATLAB (The MathWorks, Inc., Natick, MA, United States) to estimate SSRT, and conducted the statistical analyses using R software (R Foundation for Statistical Computing, Vienna, Austria). Our approach included ANOVAs to investigate the effect of the Prime stimulus (Fearful/Neutral) as a within-subject factor and the Group (Behavioral/Neurophysiological) as a between-subject factor. Post hoc analyses were conducted with Bonferroni test and the significance threshold was set at p < 0.05. Mean MEP amplitudes were measured peak-to-peak (in mV). Since background EMG is known to affect motor excitability (Devanne et al., 1997), MEPs were visually inspected and the ones preceded by background EMG were removed from further analysis. To measure the effects of ICF and SICI, we normalized MEPs in the paired-pulse sessions by comparing them to the SP session. This involved estimating the impact of the subthreshold CS on the MEP elicited by the suprathreshold TS. The ratio was then calculated by dividing the mean conditioned MEP by the mean unconditioned test MEP (Kujirai et al., 1993; Ziemann et al., 1996).
We commenced our analysis by examining the foundational assumptions of the independent race model as outlined by Verbruggen et al. (2019). Our primary focus was to compare whether the mean RT during Unsuccessful Stop-trials (instances where participants failed to halt their action despite a Stop-signal) was shorter than the mean RT in Go-trials. In our analysis of RTs, we conducted a 2 × 2 ANOVA with Trial type (Go/Unsuccessful Stop) as a within-subject factor and Group (Behavioral/Neurophysiological) as a between-subject factor, aimed at exploring the processing differences between these trial types. The analysis revealed a main effect of Trial type [F(1,44) = 47.87, p < 0.01, ηp2 = 0.96], with significantly longer RTs for Go-trials (mean = 549 ms, S.D. = 18.2 ms) compared to Unsuccessful Stop-trials (mean = 482 ms, S.D. = 13.4 ms). The consistency of longer RTs for Go-trials aligns with the theoretical expectations of the SST, where Go-trials typically require more cognitive processing (see Table 1 for details). Next, we validated the efficacy of the staircase procedure. Our goal was to confirm that the inhibition rate (the proportion of successful stops when a Stop-signal is presented) hovered around the 50% mark across all priming, as detailed in Table 1. We then proceeded to conduct a 2 × 2 ANOVA on the inhibition rate, considering the Prime (Fearful/Neutral) as a within-subject factor and the Group (Behavioral/Neurophysiological) as a between-subject factor. The findings indicated no significant differences in inhibition rate across Groups [F(1,44) = 0.054, p = 0.81, ηp2 = 0.016] or Primes [F(1,44) = 0.08, p = 0.77, ηp2 = 0.025]. Furthermore, the Group × Prime interaction was also non-significant [F(1,44) = 3.05, p = 0.08, ηp2 = 0.95], suggesting a uniform stop performance percentage when a Stop-signal is presented, irrespective of prime stimuli and participant groups.
We extended our investigation to the proportion of correct responses in Go-trials across groups, using a similar 2 × 2 ANOVA with Prime (Fearful/Neutral) and Group (Behavioral/Neurophysiological) factors. Results showed no significant Group differences [F(1,44) = 0.92, p = 0.34, ηp2 = 0.15], nor any influence of the factor Prime [F(1,44) = 3.38, p = 0.07, ηp2 = 0.57]. The interaction effect was also not significant [F(1,44) = 1.57, p = 0.21, ηp2 = 0.26], indicating consistent performance accuracy across all groups and prime stimuli. Additionally, we analyzed the Go-trial RTs with a 2 × 2 ANOVA with Prime (Fearful/Neutral) and Group (Behavioral/Neurophysiological) as factors. The analysis did not reveal any significant differences in reaction times between Groups [F(1,44) = 0.18, p = 0.28, ηp2 = 0.08] nor were they influenced by the factor Prime [F(1,44) = 0.54, p = 0.96, ηp2 = 0.23]. A significant Prime × Group interaction was observed [F(1,44) = 1.57, p = 0.03, ηp2 = 0.68]. However, Bonferroni post hoc comparisons revealed no significant results (all p > 0.5; RT Behavioral: mean Fear = 564 ms, S.D. = 22 ms, mean Neutral = 572 ms, S.D. = 21 ms; RT Neurophysiological: mean Fear = 533 ms, S.D. = 30 ms, mean Neutral = 525 ms, S.D. = 28 ms). Results suggest homogeneous reaction times across different conditions. Lastly, we analyzed the SSD data through a 2 × 2 ANOVA with Prime (Fearful/Neutral) and Group (Behavioral/Neurophysiological) factors. The results highlighted no significant Group differences [F(1,44) = 2.79, p = 0.1, ηp2 = 0.29]. Interestingly, a significant impact of the factor Prime [F(1,44) = 6.58, p = 0.01, ηp2 = 0.69] was found with a 10 ms shorter SSD for Neutral primes (mean = 228, S.D. = 13.5) than for Fearful primes (mean = 240, S.D. = 13.8). As expected, the emotional content of the Prime stimuli impacted the execution of participants’ actions, resulting in a distinct differentiation of SSD that was appropriately adjusted through successful staircase procedures. Additionally, the interaction among these factors was not significant [F(1,44) = 0.107, p = 0.74, ηp2 = 0.01], indicating a uniform SSD across all participant groups and primes.
In conclusion, these analyses confirm the reliability of the SST data collected during our experimental phases. The foundational assumption of an appropriate inhibition rate stands validated, paving the way for a reliable estimation of the SSRT, in line with Verbruggen et al. (2019).
The analysis of the SSRT was complemented by a 2 × 2 ANOVA with Prime (Fearful/Neutral) as a within-subject factor and Group (Behavioral/Neurophysiological) as a between-subject factor. The ANOVA results indicated a significant main effect of the factor Prime on SSRT [F(1, 44) = 5.56, p = 0.02, ηp2 = 0.81] in which SSRT related to Fear prime stimuli are shorter than those for Neutral prime stimuli (Fearful: mean = 322, S.D. = 5.91; Neutral: mean = 338, S.D. = 5.91) (see Figure 3). As expected, no main effect for the Group factor was found [F(1, 44) = 0.01, p = 0.9, ηp2 = 0.002]. This suggests a similar response inhibition ability across groups. The Prime × Group interaction was also found to be non-significant [F(1, 44) = 1.25, p = 0.27, ηp2 = 0.18]. Out of 46 participants, two explicitly reported the presence of the neutral and fearful body postures as primes. Thus, we carried out the same Prime × Group ANOVA removing these two participants to ensure that the results were not influenced by this factor. The ANOVA results confirmed the main effect of the Prime factor [F(1, 42) = 4.78, p = 0.03 ηp2 = 0.79], while the main effect of the factor Group was not found to be significant [F(1, 42) = 0.07, p = 0.79, ηp2 = 0.01], nor was the Prime × Group interaction [F(1, 42) = 1.13, p = 0.29, ηp2 = 0.18]. These results are in line with previous findings, which demonstrate that action control is influenced by the presence of emotional stimuli (see Battaglia et al., 2021 for a review on the topic) but, crucially, our findings demonstrated that such effects are detectable even when the negative arousing stimuli are not consciously perceived, corroborating to the idea that consciousness of the emotional stimuli is not a prerequisite to observe an influence on behavior. To assess whether differences in participants’ impulsivity may have an impact on the results, we conducted an analysis using a Generalized Linear Mixed Model with Prime, BIS-11, and their interaction as fixed effects and subject as a random effect. Model comparisons revealed a significant improvement in fit when the factor Prime was included as a predictor [AIC = 924.39, BIC = 934.48, χ2(4) = 7.51, p < 0.01]. However, the further inclusion of BIS-11 scores was also significant [AIC = 925.86, BIC = 938.47, χ2(6) = 7.51, p < 0.01]. Therefore, considering both statistical significance and goodness-of-fit measures, a model including only the Prime factor is the best balance between explanatory power and simplicity.
Figure 3. Post hoc comparison of the SSRT between prime conditions. The violin plot illustrates the distribution of the SSRT across the two prime conditions: Fearful and Neutral body postures presentation. Each violin shape represents the density distribution of the SSRT values, with wider sections indicating a higher concentration of data points. Boxplots summarize the median (central line), interquartile range (box edges), and range excluding outliers (whiskers) of SSRTs within each prime condition.
To investigate the relationship between the SSRT and neurophysiological measures, regression analyses were conducted. In a stepwise regression model, with SSRT as the dependent variable, SICI and ICF were introduced as predictors. Initially, we examined SSRT as an index calculated as the difference between the two prime stimuli (neutral minus fearful). This regression model was significant [R2 = 0.27; F(1,14) = 5.19, p = 0.04]. This finding is in line with previous results (He et al., 2019; Loomes et al., 2023) suggesting that lower levels of SICI correspond to an inhibition advantage. Additionally, here we have demonstrated that such behavioral advantage is unrelated to the prime stimulus that was (subliminally) presented. Moreover, we decided to investigate whether individual SICI and ICF may predict action control performance regardless of the type of prime presented. To do so, we evaluated SSRT by averaging the SSRTs related to the observation of the neutral and the fearful prime stimuli. In this case, the regression model including SICI as a predictor did not yield any significant results [R2 = 0.01; F(1,14) = 0.14, p = 0.71]. No predictor showed a significant positive correlation with SSRT in this context (Figure 4).
Figure 4. Correlation matrix of Stop Signal Reaction Times (SSRT) with neurophysiological indices (Short Intracortical Inhibition—SICI and Intracortical Facilitation—ICF). The off-diagonal scatter plots with red trend lines depict the pairwise correlations. Significance levels are indicated as follows:. denotes p < 0.1; *p < 0.05; **p < 0.01; and ***p < 0.001.
In exploring the relationship between SSRT and personality traits, correlation and regression analyses were carried out using the full participant sample. An SSRT index calculated as the difference between the two prime stimuli (neutral minus fearful), was entered as the dependent variable in a stepwise regression model, with the STAI-Y2 and BIS-11 subscales (i.e., MI: motor impulsivity; AI: attentional impulsivity; nPI: non-planning impulsivity) entered as predictors (Battaglia et al., 2022b). This analysis results in a significant regression model [R2 = 0.11; F(1,43) = 4.56, p = 0.04]. However, after removing two statistical outliers with a residual greater than 2 sigma, the model was non-significant [R2 = 0.02; F(1,41) = 2.02, p = 0.16], and no predictor showed a significant correlation with SSRT in this context. Next, an SSRT index calculated as the mean between the two prime stimuli (averaging neutral and fearful) was used as the dependent variable. Again, the regression model was non-significant [R2 = 0.0003; F(1,44) = 0.016, p = 0.9], even after the removal of three statistical outliers with residuals greater than 2 sigma [R2 = 0.0002; F(1,44) = 0.01, p = 0.92], with no predictor showing a significant correlation with SSRT (see Figure 5). These findings demonstrated that personality traits such as impulsivity and anxiety did not influence our findings.
Figure 5. Correlation matrix of SSRT with personality traits questionnaires. The off-diagonal scatter plots with red trend lines depict the pairwise correlations. In particular, Stop Signal Reaction Times (SSRT), State-Trait Anxiety Inventory (STAI), and all the Barratt Impulsiveness Scale-11 subscale: MI, Motor impulsivity; AI, Attentional impulsivity; and nPI, Non-planning impulsivity. Significance levels are indicated as follows:. denotes p < 0.1; *p < 0.05; **p < 0.01; and ***p < 0.001.
Perceiving negative salient cues will likely trigger an adequate motor response in the observer, aligning with the idea that emotion and action readiness are closely interconnected (Frijda, 2010; Borgomaneri et al., 2014, 2021). Overall, akin to consciously perceived threats, it appears that non-consciously perceived threats may also be linked to action preparation, as indicated, for instance, by changes in heart rate (Ruiz-Padial et al., 2005, 2011). However, it was still unclear whether non-consciously perceived threats may influence action control capabilities, measured using the SST, which can offer a reliable measure of the time taken by the brain to cancel an ongoing action (i.e., SSRT). By presenting subliminal task-irrelevant negative or neutral prime stimuli before the go signal, we investigated their influence on the ability to stop the participants’ ongoing action (i.e., discriminating the orientation of the go arrow signal) when a neutral stop stimulus (i.e., a series of crosses) was presented. Additionally, we aimed to test whether individual measures of SICI and ICF can be used as neurophysiological markers to predict action cancelation performance when subliminal emotional stimuli are presented. Results demonstrated that participants showed better action control in trials in which fearful task-irrelevant body expressions were presented compared to neutral body posture presentation. The current findings reinforce the idea that the perception of emotion is inherently connected to action systems, and provide additional evidence for the existence of a “negative bias” also for non-consciously presented threatening stimuli, shedding new light on the way non-conscious negative stimuli impact higher cognitive functions, such as action control.
The majority of current studies utilizing the SST with emotional stimuli have consistently shown that presenting an emotional image before the go stimulus tends to hinder the ability to inhibit an action (Verbruggen and Houwer, 2007; Kalanthroff et al., 2013; Rebetez et al., 2015). Conversely, when the emotional stimulus serves as the stop signal, a facilitatory effect is generally observed (Pessoa et al., 2012; Senderecka, 2016, 2018). Moreover, some studies using go/no-go tasks found that emotional stimuli impacted action control only when task-relevant, but not when task-irrelevant (Calbi et al., 2022; Mancini et al., 2022). However, go/no-go tasks and SST recruit widely different neural dynamics (Raud et al., 2020), and the abovementioned studies did not employ subliminal stimuli, but rather the emotion conveyed by the stimulus is relevant to the task.
Importantly, while impulsivity is found to impact the ability to halt an action in response to negative stimuli acting as a stop signal (Battaglia et al., 2022b), this is no longer applicable in the context of subliminal emotional priming. However, building upon our prior research findings (Battaglia et al., 2022a,b), our current study reveals that negative stimuli can enhance action control both when presented as stop signals and when primed before the go signals. Notably, the non-conscious presentation of the emotional prime stimuli ensures that they do not detrimentally capture attention, which would interfere with action control. Contrarily, we demonstrate that the subliminal presentation of negative stimuli enhances task performance in line with several evidences (de Gelder et al., 2005; Yang et al., 2011; Bertini et al., 2013; Zhan and de Gelder, 2019). The effect of the subliminal presentation of a fearful body image on the motor system is not surprising considering that functional magnetic resonance imaging (fMRI) studies have reported non-consciously perceived negative bodies, displayed in the blind field of a cortically blind patient, stimuli managed to elicit extensive cortical activity, encompassing motor and premotor cortices (Van den Stock et al., 2011). We also found that SICI, but not ICF, can predict action control abilities, with participants better in action control demonstrating higher levels of SICI, in line with recent research (He et al., 2019; Chowdhury et al., 2019a,b; Tran et al., 2020; Ding et al., 2021; Loomes et al., 2023). These intriguing findings suggest that the tonic inhibition observed when an individual is not actively engaged in intentional response control (specifically measured at rest before the SST) still serves as a predictor for stopping efficiency in subsequent tasks. Furthermore, they also imply that while action control involves various brain regions (i.e., the Action Inhibition Network; Borgomaneri et al., 2020b), the variability in the SSRT is partially influenced by the variations in local intracortical inhibitory mechanisms within the motor system. This mechanism is believed to be modulated by GABAA neurotransmission (Ziemann et al., 1996). Interestingly, only two previous studies have investigated the potential contribution of glutamatergic projection, mediated by ICF, in action control (Chowdhury et al., 2019a,b; Ding et al., 2021). In line with our results, both investigations found no correlation between the ICF and the SSRT, suggesting that intracortical glutamatergic interneurons may not be involved in the process of response inhibition, but rather during action preparation (Bundt and Huster, 2023). An important limitation of our study is that we did not collect objective measures of awareness. However, the fast presentation of the stimuli (~17 ms), together with the use of the sandwich mask procedures, is generally considered a subliminal presentation (Bar and Biederman, 1998; Harris et al., 2011; Breitmeyer, 2015). Moreover, the subjective measures confirmed that out of 46 participants, only two were aware of the presence of an emotional stimulus. Another issue is the absence of a positive stimulus or another negative control stimulus (e.g., angry body posture) as additional prime stimuli to test possible valence or arousal-related effects. However, previous data (Battaglia et al., 2022a,b) suggest no difference between positive and negative stimuli in influencing the SSRT when used as stop stimuli (but see Mancini et al., 2022 for different results using a go/no-go task). Similarly, we may expect a similar effect using angry bodies as prime stimuli, in line with findings suggesting that high arousal negative emotional states are capable of inhibiting the processing of nontarget information and enhancing selective attention (Finucane, 2011).
Taken together, our data add important information in the framework of the Cognitive vs. Affective Primacy debate (Storbeck and Clore, 2007, for review), supporting the Affective Primacy Hypothesis (Zajonc, 1980, 2000; LeDoux, 1996), based on which emotional information is processed quickly and automatically, before information about ontological kinds. Moreover, our results suggest that SICI can be considered as a potentially useful biomarker for inhibitory control deficit in the clinical setting. Indeed, resting-state intracortical inhibition has been found to be reduced in many disorders with inhibitory control deficits (Greenberg et al., 1998, 2000; Hoegl et al., 2012; Wu et al., 2012). For example, reduced SICI and increased ICF were observed in individuals with attention-deficit/hyperactivity disorder (Hoegl et al., 2012; Wu et al., 2012) and individuals with obsessive-compulsive disorder (Greenberg et al., 1998, 2000). Importantly, most of these deficits are accompanied by problems in emotion perception. Thus, investigations taking into account action control embedding emotional stimuli in clinical populations are highly desirable (Battaglia et al., 2023, 2024; Tanaka et al., 2024).
The raw data supporting the conclusions of this article will be made available by the authors, without undue reservation.
The studies involving humans were approved by Bioethical Committee of the University of Bologna. The studies were conducted in accordance with the local legislation and institutional requirements. The participants provided their written informed consent to participate in this study.
TQ: Data curation, Formal analysis, Visualization, Writing – original draft. GI: Investigation, Writing – review & editing. LP: Data curation, Writing – review & editing. PC: Data curation, Formal analysis, Software, Writing – review & editing. SBa: Writing – review & editing. SBo: Conceptualization, Funding acquisition, Investigation, Methodology, Project administration, Resources, Supervision, Writing – original draft, Writing – review & editing.
The author(s) declare financial support was received for the research, authorship, and/or publication of this article. SBo was supported by Next Generation EU (NGEU) and funded by the Ministry of University and Research (MUR), National Recovery and Resilience Plan (NRRP) PRIN 2022 (grant no. 2022XKZBFC—CUP J53D23008340001): the influence of emotions on action control: brain network plasticity and potential trans-diagnostic applications (D DN. 104 02.02.2022) and Bial Foundation, Portugal (033/22). SBa was supported by #NEXTGENERATIONEU (NGEU) and funded by the Ministry of University and Research (MUR), National Recovery and Resilience Plan (NRRP), and project MNESYS (PE0000006)—a multiscale integrated approach to the study of the nervous system in health and disease (DN. 1553 11.10.2022).
The authors declare that the research was conducted in the absence of any commercial or financial relationships that could be construed as a potential conflict of interest.
All claims expressed in this article are solely those of the authors and do not necessarily represent those of their affiliated organizations, or those of the publisher, the editors and the reviewers. Any product that may be evaluated in this article, or claim that may be made by its manufacturer, is not guaranteed or endorsed by the publisher.
Avila, C., and Parcet, M. A. (2001). Personality and inhibitory deficits in the stop-signal task: the mediating role of Gray’s anxiety and impulsivity. Personal. Individ. Differ. 31, 975–986. doi: 10.1016/S0191-8869(00)00199-9
Band, G. P. H., van der Molen, M. W., and Logan, G. D. (2003). Horse-race model simulations of the stop-signal procedure. Acta Psychol. 112, 105–142. doi: 10.1016/S0001-6918(02)00079-3
Bar, M., and Biederman, I. (1998). Subliminal Visual Priming. Psychol. Sci. 9, 464–468. doi: 10.1111/1467-9280.00086
Bari, A., and Robbins, T. W. (2013). Inhibition and impulsivity: behavioral and neural basis of response control. Prog. Neurobiol. 108, 44–79. doi: 10.1016/J.PNEUROBIO.2013.06.005
Battaglia, S., Avenanti, A., Vécsei, L., and Tanaka, M. (2024, 2024). Neurodegeneration in cognitive impairment and mood disorders for experimental, clinical and translational neuropsychiatry. Biomedicine 12:574. doi: 10.3390/BIOMEDICINES12030574
Battaglia, S., Cardellicchio, P., Di Fazio, C., Nazzi, C., Fracasso, A., and Borgomaneri, S. (2022a). Stopping in (e)motion: reactive action inhibition when facing valence-independent emotional stimuli. Front. Behav. Neurosci. 16:998714. doi: 10.3389/fnbeh.2022.998714
Battaglia, S., Cardellicchio, P., Di Fazio, C., Nazzi, C., Fracasso, A., and Borgomaneri, S. (2022b). The influence of vicarious fear-learning in “infecting” reactive action inhibition. Front. Behav. Neurosci. 16:946263. doi: 10.3389/fnbeh.2022.946263
Battaglia, S., Schmidt, A., Hassel, S., and Tanaka, M. (2023). Editorial: case reports in neuroimaging and stimulation. Front. Psychol. 14:1264669. doi: 10.3389/fpsyt.2023.1264669
Battaglia, S., Serio, G., Scarpazza, C., D’Ausilio, A., and Borgomaneri, S. (2021). Frozen in (e)motion: how reactive motor inhibition is influenced by the emotional content of stimuli in healthy and psychiatric populations. Behav. Res. Ther. 146:103963. doi: 10.1016/j.brat.2021.103963
Bertini, C., Cecere, R., and Làdavas, E. (2013). I am blind, but I “see” fear. Cortex 49, 985–993. doi: 10.1016/j.cortex.2012.02.006
Borgomaneri, S., Bolloni, C., Sessa, P., and Avenanti, A. (2020a). Blocking facial mimicry affects recognition of facial and body expressions. PLoS One 15:e0229364. doi: 10.1371/journal.pone.0229364
Borgomaneri, S., Gazzola, V., and Avenanti, A. (2012). Motor mapping of implied actions during perception of emotional body language. Brain Stimul. 5, 70–76. doi: 10.1016/j.brs.2012.03.011
Borgomaneri, S., Gazzola, V., and Avenanti, A. (2014). Temporal dynamics of motor cortex excitability during perception of natural emotional scenes. Soc. Cogn. Affect. Neurosci. 9, 1451–1457. doi: 10.1093/scan/nst139
Borgomaneri, S., Gazzola, V., and Avenanti, A. (2015a). Transcranial magnetic stimulation reveals two functionally distinct stages of motor cortex involvement during perception of emotional body language. Brain Struct. Funct. 220, 2765–2781. doi: 10.1007/s00429-014-0825-6
Borgomaneri, S., Serio, G., and Battaglia, S. (2020b). Please, don’t do it! Fifteen years of progress of non-invasive brain stimulation in action inhibition. Cortex 132, 404–422. doi: 10.1016/j.cortex.2020.09.002
Borgomaneri, S., Vitale, F., and Avenanti, A. (2015b). Early changes in corticospinal excitability when seeing fearful body expressions. Sci. Rep. 5, 1–9. doi: 10.1038/srep14122
Borgomaneri, S., Vitale, F., and Avenanti, A. (2017). Behavioral inhibition system sensitivity enhances motor cortex suppression when watching fearful body expressions. Brain Struct. Funct. 222, 3267–3282. doi: 10.1007/S00429-017-1403-5
Borgomaneri, S., Vitale, F., and Avenanti, A. (2020c). Early motor reactivity to observed human body postures is affected by body expression, not gender. Neuropsychologia 146:107541. doi: 10.1016/j.neuropsychologia.2020.107541
Borgomaneri, S., Vitale, F., Battaglia, S., and Avenanti, A. (2021). Early right motor cortex response to happy and fearful facial expressions: a TMS motor-evoked potential study. Brain Sci. 11:1203. doi: 10.3390/brainsci11091203
Borgomaneri, S., Vitale, F., Battaglia, S., de Vega, M., and Avenanti, A. (2024). Task-related modulation of motor response to emotional bodies: a TMS motor-evoked potential study. Cortex 171, 235–246. doi: 10.1016/j.cortex.2023.10.013
Borgomaneri, S., Vitale, F., Gazzola, V., and Avenanti, A. (2015c). Seeing fearful body language rapidly freezes the observer’s motor cortex. Cortex 65, 232–245. doi: 10.1016/J.CORTEX.2015.01.014
Brasil-Neto, J. P., Cohen, L. G., Panizza, M., Nilsson, J., Roth, B. J., and Hallett, M. (1992). Optimal focal transcranial magnetic activation of the human motor cortex: effects of coil orientation, shape of the induced current pulse, and stimulus intensity. J. Clin. Neurophysiol. 9, 132–136. doi: 10.1097/00004691-199201000-00014
Breitmeyer, B. G. (2015). Psychophysical “blinding” methods reveal a functional hierarchy of unconscious visual processing. Conscious. Cogn. 35, 234–250. doi: 10.1016/J.CONCOG.2015.01.012
Bundt, C., and Huster, R. J. (2023). Corticospinal excitability reductions during action preparation and action stopping: different sides of the same inhibitory coin? Neuropsychologia 195, 1–18. doi: 10.1016/j.neuropsychologia.2024.108799
Calbi, M., Montalti, M., Pederzani, C., Arcuri, E., Umiltà, M. A., Gallese, V., et al. (2022). Emotional body postures affect inhibitory control only when task-relevant. Front. Psychol. 13:1035328. doi: 10.3389/fpsyg.2022.1035328
Carlson, J. M., and Reinke, K. S. (2008). Masked fearful faces modulate the orienting of covert spatial attention. Emotion 8, 522–529. doi: 10.1037/a0012653
Chowdhury, N. S., Livesey, E. J., Blaszczynski, A., and Harris, J. A. (2018). Variations in response control within at-risk gamblers and non-gambling controls explained by GABAergic inhibition in the motor cortex. Cortex 103, 153–163. doi: 10.1016/j.cortex.2018.03.004
Chowdhury, N. S., Livesey, E. J., and Harris, J. A. (2019a). Contralateral and ipsilateral relationships between Intracortical inhibition and stopping efficiency. Neuroscience 415, 10–17. doi: 10.1016/j.neuroscience.2019.07.013
Chowdhury, N. S., Livesey, E. J., and Harris, J. A. (2019b). Individual differences in intracortical inhibition during behavioural inhibition. Neuropsychologia 124, 55–65. doi: 10.1016/j.neuropsychologia.2019.01.008
de Gelder, B., Morris, J. S., and Dolan, R. J. (2005). Unconscious fear influences emotional awareness of faces and voices. Proc. Natl. Acad. Sci. USA 102, 18682–18687. doi: 10.1073/pnas.0509179102
Derntl, B., and Habel, U. (2017). Angry but not neutral faces facilitate response inhibition in schizophrenia patients. Eur. Arch. Psychiatry Clin. Neurosci. 267, 621–627. doi: 10.1007/s00406-016-0748-8
Devanne, H., Lavoie, B. A., and Capaday, C. (1997). Input-output properties and gain changes in the human corticospinal pathway. Exp. Brain Res. 114, 329–338. doi: 10.1007/PL00005641
Di Gregorio, F., and Battaglia, S. (2024). The intricate brain–body interaction in psychiatric and neurological diseases. Adv. Clin. Exp. Med. 33, 321–326. doi: 10.17219/ACEM/185689
Di Lazzaro, V., Oliviero, A., Meglio, M., Cioni, B., Tamburrini, G., Tonali, P., et al. (2000). Direct demonstration of the effect of lorazepam on the excitability of the human motor cortex. Clin. Neurophysiol. 111, 794–799. doi: 10.1016/s1388-2457(99)00314-4
Diano, M., Celeghin, A., Bagnis, A., and Tamietto, M. (2017). Amygdala response to emotional stimuli without awareness: facts and interpretations. Front. Psychol. 7:2029. doi: 10.3389/fpsyg.2016.02029
Ding, Q., Cai, H., Wu, M., Cai, G., Chen, H., Li, W., et al. (2021). Short intracortical facilitation associates with motor-inhibitory control. Behav. Brain Res. 407:113266. doi: 10.1016/j.bbr.2021.113266
Ding, J., Wang, Y., Wang, C., d’Oleire Uquillas, F., He, Q., Cheng, L., et al. (2020). Negative impact of sadness on response inhibition in females: an explicit emotional stop signal task fMRI study. Front. Behav. Neurosci. 14:119. doi: 10.3389/fnbeh.2020.00119
Duque, J., Greenhouse, I., Labruna, L., and Ivry, R. B. (2017). Physiological markers of motor inhibition during human behavior. Trends Neurosci. 40, 219–236. doi: 10.1016/j.tins.2017.02.006
Eimer, M., and Schlaghecken, F. (2003). Response facilitation and inhibition in subliminal priming. Biol. Psychol. 64, 7–26. doi: 10.1016/S0301-0511(03)00100-5
Engelen, T., Zhan, M., Sack, A. T., and de Gelder, B. (2018). The influence of conscious and unconscious body threat expressions on motor evoked potentials studied with continuous flash suppression. Front. Neurosci. 12:480. doi: 10.3389/fnins.2018.00480
Faul, F., Erdfelder, E., Lang, A. G., and Buchner, A. (2007). G*power 3: a flexible statistical power analysis program for the social, behavioral, and biomedical sciences. Behav. Res. Methods 39, 175–191. doi: 10.3758/BF03193146
Finucane, A. M. (2011). The effect of fear and anger on selective attention. Emotion 11, 970–974. doi: 10.1037/A0022574
Flykt, A. (2006). Preparedness for action: responding to the snake in the grass. Am. J. Psychol. 119, 29–43. doi: 10.2307/20445317
Frijda, N. H. (2010). Impulsive action and motivation. Biol. Psychol. 84, 570–579. doi: 10.1016/j.biopsycho.2010.01.005
Greenberg, B. D., Ziemann, U., Corá-Locatelli, G., Harmon, A., Murphy, D. L., Keel, J. C., et al. (2000). Altered cortical excitability in obsessive-compulsive disorder. Neurology 54, 142–147. doi: 10.1212/wnl.54.1.142
Greenberg, B. D., Ziemann, U., Harmon, A., Murphy, D. L., and Wassermann, E. M. (1998). Decreased neuronal inhibition in cerebral cortex in obsessive-compulsive disorder on transcranial magnetic stimulation. Lancet 352, 881–882. doi: 10.1016/S0140-6736(05)60009-8
Harris, J. A., Wu, C.-T., and Woldoff, M. G. (2011). Sandwich masking eliminates both visual awareness of faces and face-specific brain activity through a feed-forward mechanism. J. Vis. 11:3. doi: 10.1126/scisignal.2001449
He, J. L., Fuelscher, I., Coxon, J., Chowdhury, N., Teo, W. P., Barhoun, P., et al. (2019). Individual differences in intracortical inhibition predict motor-inhibitory performance. Exp. Brain Res. 237, 2715–2727. doi: 10.1007/s00221-019-05622-y
Hoegl, T., Heinrich, H., Barth, W., Lösel, F., Moll, G. H., and Kratz, O. (2012). Time course analysis of motor excitability in a response inhibition task according to the level of hyperactivity and impulsivity in children with ADHD. PLoS One 7:e46066. doi: 10.1371/journal.pone.0046066
Hsieh, M. T., Lu, H., Chen, L. F., Liu, C. Y., Hsu, S. C., and Cheng, C. H. (2022). Cancellation but not restraint ability is modulated by trait anxiety: an event-related potential and oscillation study using go-Nogo and stop-signal tasks. J. Affect. Disord. 299, 188–195. doi: 10.1016/J.JAD.2021.11.066
Ilic, T. V., Meintzschel, F., Cleff, U., Ruge, D., Kessler, K. R., and Ziemann, U. (2002). Short-interval paired-pulse inhibition and facilitation of human motor cortex: the dimension of stimulus intensity. J. Physiol. 545, 153–167. doi: 10.1113/jphysiol.2002.030122
Kalanthroff, E., Cohen, N., and Henik, A. (2013). Stop feeling: inhibition of emotional interference following stop-signal trials. Front. Hum. Neurosci. 7:78. doi: 10.3389/fnhum.2013.00078
Krypotos, A.-M., and Jahfari, S., Ast, V. A. van, Kindt, M., and Forstmann, B. U. (2011). Individual differences in heart rate variability predict the degree of slowing during response inhibition and initiation in the presence of emotional stimuli. Front. Psychol. 2,:278. doi: 10.3389/fpsyg.2011.00278
Kujirai, T., Caramia, M. D., Rothwell, J. C., Day, B. L., Thompson, P. D., Ferbert, A., et al. (1993). Corticocortical inhibition in human motor cortex. J. Physiol. 471, 501–519. doi: 10.1113/JPHYSIOL.1993.SP019912
Kunde, W. (2003). Sequential modulations of stimulus-response correspondence effects depend on awareness of response conflict. Psychon. Bull. Rev. 10, 198–205. doi: 10.3758/BF03196485
Lau, H. C., and Passingham, R. E. (2007). Unconscious activation of the cognitive control system in the human prefrontal cortex. J. Neurosci. 27, 5805–5811. doi: 10.1523/JNEUROSCI.4335-06.2007
LeDoux, J. (1996). Emotional networks and motor control: a fearful view. Prog. Brain Res. 107, 437–446. doi: 10.1016/S0079-6123(08)61880-4
Logan, G. D. (1994). “On the ability to inhibit thought and action: a users’ guide to the stop signal paradigm” in Inhibitory Processes in Attention, Memory, and Language. eds. D. Dagenbach and T. H. Carr (San Diego: Academic Press), 189–239.
Logan, G. D., Cowan, W. B., and Davis, K. A. (1984). On the ability to inhibit simple and choice reaction time responses: a model and a method. J. Exp. Psychol. Hum. Percept. Perform. 10, 276–291. doi: 10.1037/0096-1523.10.2.276
Logan, G. D., Schachar, R. J., and Tannock, R. (1997). Impulsivity and inhibitory control. Psychol. Sci. 8, 60–64. doi: 10.1111/J.1467-9280.1997.TB00545.X
Logan, G. D., Van Zandt, T., Verbruggen, F., and Wagenmakers, E. J. (2014). On the ability to inhibit thought and action: general and special theories of an act of control. Psychol. Rev. 121, 66–95. doi: 10.1037/A0035230
Loomes, M., Tran, D. M. D., Chowdhury, N. S., Birney, D. P., Harris, J. A., and Livesey, E. J. (2023). Is cortical inhibition in primary motor cortex related to executive control? Cortex 160, 100–114. doi: 10.1016/j.cortex.2022.12.013
Mancini, C., Falciati, L., Maioli, C., and Mirabella, G. (2022). Happy facial expressions impair inhibitory control with respect to fearful facial expressions but only when task-relevant. Emotion 22, 142–152. doi: 10.1037/EMO0001058
Matzke, D., Verbruggen, F., and Logan, G. D. (2018). “The stop-signal paradigm” in Stevens’ Handbook of Experimental Psychology and Cognitive Neuroscience, vol. 1–45. eds. J. T. Wixted and E. J. Wagenmakers (Hoboken, New Jersey: John Wiley & Sons. Inc.).
Merikle, P. M., Smilek, D., and Eastwood, J. D. (2001). Perception without awareness: perspectives from cognitive psychology. Cognition 79, 115–134. doi: 10.1016/S0010-0277(00)00126-8
Mills, K. R., Boniface, S. J., and Schubert, M. (1992). Magnetic brain stimulation with a double coil: the importance of coil orientation. Electroencephal. Clin. Neurophysiol. Evok. Potent. Sect. 85, 17–21. doi: 10.1016/0168-5597(92)90096-T
Morris, J. S., Öhman, A., and Dolan, R. J. (1998). Conscious and unconscious emotional learning in the human amygdala. Nature 393, 467–470. doi: 10.1038/30976
Nakamura, H., Kitagawa, H., Kawaguchi, Y., and Tsuji, H. (1997). Intracortical facilitation and inhibition after transcranial magnetic stimulation in conscious humans. J. Physiol. 498, 817–823. doi: 10.1113/jphysiol.1997.sp021905
Neo, P. S. H., Thurlow, J. K., and McNaughton, N. (2011). Stopping, goal-conflict, trait anxiety and frontal rhythmic power in the stop-signal task. Cogn. Affect. Behav. Neurosci. 11, 485–493. doi: 10.3758/s13415-011-0046-x
Ohman, A., Flykt, A., and Esteves, F. (2001). Emotion drives attention: detecting snakes in the grass. J. Exp. Psychol. Gen. 130, 466–478. doi: 10.1037//0096-3445.130.3.466
Oldfield, R. C. (1971). The assessment and analysis of handedness: the Edinburgh inventory. Neuropsychologia 9, 97–113. doi: 10.1016/0028-3932(71)90067-4
Patton, J. H., Stanford, M. S., and Barratt, E. S. (1995). Factor structure of the Barratt impulsiveness scale. J. Clin. Psychol. 51, 768–774. doi: 10.1002/1097-4679(199511)51:6<768::AID-JCLP2270510607>3.0.CO;2-1
Pawliczek, C. M., Derntl, B., Kellermann, T., Kohn, N., Gur, R. C., and Habel, U. (2013). Inhibitory control and trait aggression: neural and behavioral insights using the emotional stop signal task. NeuroImage 79, 264–274. doi: 10.1016/J.NEUROIMAGE.2013.04.104
Pessoa, L. (2009). How do emotion and motivation direct executive control? Trends Cogn. Sci. 13, 160–166. doi: 10.1016/J.TICS.2009.01.006
Pessoa, L., Padmala, S., Kenzer, A., and Bauer, A. (2012). Interactions between cognition and emotion during response inhibition. Emotion 12, 192–197. doi: 10.1037/a0024109
Raud, L., Westerhausen, R., Dooley, N., and Huster, R. J. (2020). Differences in unity: the go/no-go and stop signal tasks rely on different mechanisms. NeuroImage 210:116582. doi: 10.1016/J.NEUROIMAGE.2020.116582
Rebetez, M. M. L., Rochat, L., Billieux, J., Gay, P., and Van der Linden, M. (2015). Do emotional stimuli interfere with two distinct components of inhibition? Cognit. Emot. 29, 559–567. doi: 10.1080/02699931.2014.922054
Rossi, S., Antal, A., Bestmann, S., Bikson, M., Brewer, C., Brockmöller, J., et al. (2021). Safety and recommendations for TMS use in healthy subjects and patient populations, with updates on training, ethical and regulatory issues: expert guidelines. Clin. Neurophysiol. 132, 269–306. doi: 10.1016/J.CLINPH.2020.10.003
Rossini, P. M., Barker, A. T., Berardelli, A., Caramia, M. D., Caruso, G., Cracco, R. Q., et al. (1994). Non-invasive electrical and magnetic stimulation of the brain, spinal cord and roots: basic principles and procedures for routine clinical application. Report of an IFCN committee. Electroencephalogr. Clin. Neurophysiol. 91, 79–92. doi: 10.1016/0013-4694(94)90029-9
Ruiz-Padial, E., Mata, J. L., Rodríguez, S., Fernández, M. C., and Vila, J. (2005). Non-conscious modulation of cardiac defense by masked phobic pictures. Int. J. Psychophysiol. 56, 271–281. doi: 10.1016/j.ijpsycho.2004.12.010
Ruiz-Padial, E., Vila, J., and Thayer, J. F. (2011). The effect of conscious and non-conscious presentation of biologically relevant emotion pictures on emotion modulated startle and phasic heart rate. Int. J. Psychophysiol. 79, 341–346. doi: 10.1016/j.ijpsycho.2010.12.001
Sagaspe, P., Schwartz, S., and Vuilleumier, P. (2011). Fear and stop: a role for the amygdala in motor inhibition by emotional signals. NeuroImage 55, 1825–1835. doi: 10.1016/j.neuroimage.2011.01.027
Schimmack, U. (2005). Attentional interference effects of emotional pictures: Threat, negativity, or arousal? Emotion, 5, 55–66. doi: 10.1037/15283542.5.1.55
Senderecka, M. (2016). Threatening visual stimuli influence response inhibition and error monitoring: an event-related potential study. Biol. Psychol. 113, 24–36. doi: 10.1016/j.biopsycho.2015.11.003
Senderecka, M. (2018). Emotional enhancement of error detection—the role of perceptual processing and inhibition monitoring in failed auditory stop trials. Cogn. Affect. Behav. Neurosci. 18, 1–20. doi: 10.3758/s13415-017-0546-4
Song, S., Zou, Z., Song, H., Wang, Y., Uquillas, F. D. O., Wang, H., et al. (2016). Romantic love is associated with enhanced inhibitory control in an emotional stop-signal task. Front. Psychol. 7:1574. doi: 10.3389/fpsyg.2016.01574
Spielberger, C. D., Gorsuch, R. L., Lushene, R., Vagg, P., and Jacobs, G. (1970). Manual for the State-Trait Anxiety Inventory (self-evaluation questionnaire). CA: Consulting psychologists press.
Storbeck, J., and Clore, G. L. (2007). On the interdependence of cognition and emotion. Cognit. Emot. 21, 1212–1237. doi: 10.1080/02699930701438020
Sweeny, T. D., Grabowecky, M., Suzuki, S., and Paller, K. A. (2009). Long-lasting effects of subliminal affective priming from facial expressions. Conscious. Cogn. 18, 929–938. doi: 10.1016/j.concog.2009.07.011
Tamietto, M., and de Gelder, B. (2008). Affective blindsight in the intact brain: neural interhemispheric summation for unseen fearful expressions. Neuropsychologia 46, 820–828. doi: 10.1016/j.neuropsychologia.2007.11.002
Tanaka, M., Battaglia, S., Giménez-Llort, L., Chen, C., Hepsomali, P., Avenanti, A., et al. (2024). Innovation at the intersection: emerging translational research in neurology and psychiatry. Cells 13:790. doi: 10.3390/CELLS13100790
Toh, W. X., and Yang, H. (2020). Similar but not quite the same: differential unique associations of trait fear and trait anxiety with inhibitory control. Personal. Individ. Differ. 155:109718. doi: 10.1016/J.PAID.2019.109718
Tran, D. M. D., Chowdhury, N. S., McNair, N. A., Harris, J. A., and Livesey, E. J. (2020). Linking cortical and behavioural inhibition: testing the parameter specificity of a transcranial magnetic stimulation protocol. Brain Stimul. 13, 1381–1383. doi: 10.1016/j.brs.2020.07.010
Van den Stock, J., Tamietto, M., Sorger, B., Pichon, S., Grézes, J., de Gelder, B., et al. (2011). Cortico-subcortical visual, somatosensory, and motor activations for perceiving dynamic whole-body emotional expressions with and without striate cortex (V1). Proc. Natl. Acad. Sci. USA 108, 16188–16193. doi: 10.1073/pnas.1107214108
Verbruggen, F., Aron, A. R., Band, G. P. H., Beste, C., Bissett, P. G., Brockett, A. T., et al. (2019). A consensus guide to capturing the ability to inhibit actions and impulsive behaviors in the stop-signal task. eLife 8:e46323. doi: 10.7554/elife.46323
Verbruggen, F., Chambers, C. D., and Logan, G. D. (2013). Fictitious inhibitory differences: how skewness and slowing distort the estimation of stopping latencies. Psychol. Sci. 24, 352–362. doi: 10.1177/0956797612457390
Verbruggen, F., and Houwer, J. D. (2007). Do emotional stimuli interfere with response inhibition? Evidence from the stop signal paradigm. Cognit. Emot. 21, 391–403. doi: 10.1080/02699930600625081
Verbruggen, F., and Logan, G. D. (2008). Response inhibition in the stop-signal paradigm. Trends Cogn. Sci. 12, 418–424. doi: 10.1016/j.tics.2008.07.005
Whalen, P. J., Rauch, S. L., Etcoff, N. L., McInerney, S. C., Lee, M. B., and Jenike, M. A. (1998). Masked presentations of emotional facial expressions modulate amygdala activity without explicit knowledge. J. Neurosci. 18, 411–418. doi: 10.1523/JNEUROSCI.18-01-00411.1998
Wu, S. W., Gilbert, D. L., Shahana, N., Huddleston, D. A., and Mostofsky, S. H. (2012). Transcranial magnetic stimulation measures in attention-deficit/ hyperactivity disorder. Pediatr. Neurol. 47, 177–185. doi: 10.1016/j.pediatrneurol.2012.06.003
Yang, J., Xu, X., Du, X., Shi, C., and Fang, F. (2011). Effects of unconscious processing on implicit memory for fearful faces. PLoS One 6:e14641. doi: 10.1371/journal.pone.0014641
Zajonc, R. B. (1980). Feeling and thinking: preferences need no inferences. Am. Psychol. 35, 151–175. doi: 10.1037/0003-066X.35.2.151
Zajonc, R. B. (2000). “Feeling and thinking: closing the debate over the independence of affect” in Feeling and Thinking: The Role of Affect in Social Cognition. ed. E. P. Forgas (Cambridge, United Kingdom: Cambridge University Press), 31–58.
Zhan, M., and de Gelder, B. (2019). Unconscious fearful body perception enhances discrimination of conscious anger expressions under continuous flash suppression. Neuropsychologia 128, 325–331. doi: 10.1016/j.neuropsychologia.2018.04.019
Ziemann, U. (2003). Pharmacology of TMS. Suppl. Clin. Neurophysiol. 56, 226–231. doi: 10.1016/S1567-424X(09)70226-0
Keywords: action control, stop signal task, fearful body postures, transcranial magnetic stimulation, intracortical inhibition and facilitation
Citation: Quettier T, Ippolito G, Però L, Cardellicchio P, Battaglia S and Borgomaneri S (2024) Individual differences in intracortical inhibition predict action control when facing emotional stimuli. Front. Psychol. 15:1391723. doi: 10.3389/fpsyg.2024.1391723
Received: 26 February 2024; Accepted: 23 May 2024;
Published: 12 June 2024.
Edited by:
Tjeerd Jellema, University of Hull, United KingdomReviewed by:
Wei Xing Toh, Nanyang Technological University, SingaporeCopyright © 2024 Quettier, Ippolito, Però, Cardellicchio, Battaglia and Borgomaneri. This is an open-access article distributed under the terms of the Creative Commons Attribution License (CC BY). The use, distribution or reproduction in other forums is permitted, provided the original author(s) and the copyright owner(s) are credited and that the original publication in this journal is cited, in accordance with accepted academic practice. No use, distribution or reproduction is permitted which does not comply with these terms.
*Correspondence: Sara Borgomaneri, c2FyYS5ib3Jnb21hbmVyaUB1bmliby5pdA==; Thomas Quettier, dGhvbWFzLnF1ZXR0aWVyMkB1bmliby5pdA==
Disclaimer: All claims expressed in this article are solely those of the authors and do not necessarily represent those of their affiliated organizations, or those of the publisher, the editors and the reviewers. Any product that may be evaluated in this article or claim that may be made by its manufacturer is not guaranteed or endorsed by the publisher.
Research integrity at Frontiers
Learn more about the work of our research integrity team to safeguard the quality of each article we publish.