- 1Faculty of Health Sciences, School of Kinesiology, University of Western Ontario, London, ON, Canada
- 2Department of Psychiatry, University of Oxford, Oxford, United Kingdom
- 3Canadian Centre for Activity and Aging, University of Western Ontario, London, ON, Canada
- 4Graduate Program in Neuroscience, University of Western Ontario, London, ON, Canada
Introduction: Cognitive flexibility represents a core component of executive function that promotes the ability to efficiently alternate—or “switch”—between different tasks. Literature suggests that acute stress negatively impacts cognitive flexibility, whereas a single bout of aerobic exercise supports a postexercise improvement in cognitive flexibility. Here, we examined whether a single bout of aerobic exercise attenuates a stress-induced decrement in task-switching.
Materials and Methods: Forty participants (age range = 19–30) completed the Trier Social Stress Test (TSST) and were randomized into separate Exercise or Rest groups entailing 20-min sessions of heavy intensity exercise (80% of heart rate maximum via cycle ergometer) or rest, respectively. Stress induction was confirmed via state anxiety and heart rate. Task-switching was assessed prior to the TSST (i.e., pre-TSST), following the TSST (i.e., post-TSST), and following Exercise and Rest interventions (i.e., post-intervention) via pro- (i.e., saccade to veridical target location) and antisaccades (i.e., saccade mirror-symmetrical to target location) arranged in an AABB task-switching paradigm. The underlying principle of the AABB paradigm suggests that when prosaccades are preceded by antisaccades (i.e., task-switch trials), the reaction times are longer compared to their task-repeat counterparts (i.e., unidirectional prosaccade switch-cost).
Results: As expected, the pre-TSST assessment yielded a prosaccade switch cost. Notably, post-TSST physiological measures indicated a reliable stress response and at this assessment a null prosaccade switch-cost was observed. In turn, post-intervention assessments revealed a switch-cost independent of Exercise and Rest groups.
Conclusion: Accordingly, the immediate effects of acute stress supported improved task-switching in young adults; however, these benefits were not modulated by a single bout of aerobic exercise.
Introduction
Executive function represents a constellation of higher-order processes including the core components of inhibitory control, working memory, and cognitive flexibility (Diamond, 2013). Executive function and health are interrelated such that executive function behaves as an antecedent and consequence of health behaviors (for reviews, see Allan et al., 2016; Williams et al., 2017). Indeed, an extensive literature has shown that executive function is modulated via a number of external factors including stress and exercise (Chang et al., 2012; Plieger and Reuter, 2020). In the first case, stress represents a state of threatened homeostasis (Chrousos and Gold, 1992) or an experience wherein an individual appraises a situation as exceeding their resources and endangering their wellbeing (Lazarus and Folkman, 1984). Shields et al.’s (2016) meta-analysis concluded that an acute bout of stress provides a transient disruption to the components of executive function. In particular, the authors reported stress impaired performance on tasks assessing cognitive flexibility and working memory. In contrast, effects of acute stress on inhibitory control were mixed. Importantly, cognitive flexibility requires the efficient ability to inhibit extraneous stimuli and hold a currently relevant task set in mind. The stress-induced reduction in executive performance has been attributed to the reallocation of “finite” executive function resources in conjunction with neurobiological alterations associated with activation of the hypothalamic-pituitary-adrenal (HPA) and sympathetic-adrenal-medullary (SAM) axes (Mather and Sutherland, 2011; Plessow et al., 2011; Shansky and Lipps, 2013). In the second case, meta-analytic evidence suggests single bouts of aerobic and/or resistance exercise provide a brief (i.e., <60-min) benefit to executive function (Lambourne and Tomporowski, 2010; Chang et al., 2012; Ludyga et al., 2016). This effect has been attributed to exercise-based increases in cerebral blood flow (Tari et al., 2020), biomolecule concentrations (Zouhal et al., 2008; Knaepen et al., 2010) and resting state functional connectivity (Schmitt et al., 2019) that support improved efficiency of local neural circuits (Moore and Cao, 2008). Notably, a narrative review by Sandi (2013) highlighted stress response systems such as the HPA and SAM axes are heavily integrated with regions supporting executive functions (Joëls and Baram, 2009). A single bout of exercise has also been demonstrated to buffer the effects of stress and promote stress-related recovery as determined by biological (e.g., heart rate, blood pressure, cortisol levels) and perceptual (e.g., State Trait Anxiety Inventory) measures of stress (LaManca et al., 2001; Brownley et al., 2003; Alderman et al., 2007; Wunsch et al., 2019). Taken together, this underscores the use of a single bout of exercise in attenuating the deleterious effects of acute stress.
To our knowledge, however, no research has directly examined the potential by which a single bout of exercise may blunt a stress-induced decrement in executive function. In particular, the current work explored whether a 20-min heavy intensity (80% HR maximum) aerobic exercise bout via cycle ergometer attenuates stress-induced impairments in the cognitive flexibility component of executive function via task-switching. For example, a number of studies by our group have examined cognitive flexibility via a paradigm wherein individuals alternate between pro- and anti-saccades in predictable (i.e., AABB) or unpredictable (i.e., AABABB…) task-switching schedules (Weiler and Heath, 2012a,b, 2014a; Heath et al., 2015; Weiler et al., 2015). Prosaccades are a goal-directed eye movement (i.e., saccade) directed to a veridical target location, whereas antisaccades entail a response mirror-symmetrical to a target. Antisaccades produce longer reaction times (RTs; Hallett, 1978) and “less accurate and more variable endpoints than prosaccades (Gillen and Heath, 2014) and these behavioral ‘costs’ have been linked to the top-down executive processes of response suppression (i.e., inhibiting a reflexive prosaccade) and vector inversion (i.e., 180° spatial transformation)” (for review see Munoz and Everling, 2004). Further, when a prosaccade is preceded by an antisaccade (i.e., task-switch trial) RTs are “longer than when preceded by its same task-type (i.e., task-repeat trial), whereas antisaccade RTs for task-switch and task-repeat do not reliably differ” (Weiler and Heath, 2012a,b). The basis for the asymmetrical switch-cost is that the executive demands of antisaccades result in a task-set inertia that proactively delays the planning of a subsequent prosaccade (i.e., the unidirectional switch-cost) (Weiler and Heath, 2012a). Notably, Shukla and Heath (Shukla et al., 2020; Shukla and Heath, 2021) demonstrated that a single bout of aerobic exercise provides an immediate and sustained (~47-min) benefit to task-switching performance as evidenced by a decreased magnitude unidirectional prosaccade switch-cost. In particular, Shukla and Heath (2021) had participants complete 20-min of heavy intensity (i.e., 80% of heart rate maximum) aerobic exercise via cycle ergometer and reported a 14 ms decrease in the unidirectional prosaccade switch-cost from a pre- to postexercise assessment and further observed that this benefit was sustained for up to 47-min postexercise (see also Heath and Shukla, 2020).
In the present work, participants (N = 40) completed baseline pro- and antisaccades arranged in an AABB task-switching schedule (i.e., A = prosaccade, B = antisaccade) and were then exposed to a validated acute stressor (i.e., Trier Social Stress Test: TSST). Subsequently, participants completed a second set of pro- and antisaccade task-switching trials after which they were assigned to an Exercise or Rest group. The Exercise group (n = 20) completed 20-min of the aforementioned exercise protocol, whereas the Rest group (n = 20) sat on the cycle ergometer without exercising. Following the Exercise and Rest intervention, participants completed a final set of pro- and antisaccade task-switching trials. Hence, the current protocol provides a basis to quantify whether a stress-induced impairment in task-switching is ameliorated via a 20-min bout of aerobic exercise. As for research predictions, it is anticipated that exposure to the TSST will result in a reliable increase in the magnitude of the unidirectional prosaccade switch-cost; that is, acute stress-induction is predicted to impair cognitive flexibility. In turn, it is anticipated that the unidirectional prosaccade switch-cost magnitude will return to baseline following the Exercise—but not Rest—intervention. In other words, we predict that the exercise intervention will attenuate the residual effects of stress induction on the cognitive flexibility component of executive function.
Materials and methods
Participants
Forty participants (21 female and 19 male, mean age = 22, SD = 3, age range = 19–30) volunteered from the Western University community. An a priori sample size was generated based on the effect size derived from an independent-samples t-test contrasting pre- and postexercise antisaccade RTs (α = 0.05, power = 0.95, dz. = 1.19; Petrella et al., 2019). All participants had normal or corrected-to-normal vision, self-declared being right-hand dominant, reported no history of neurological impairment (including concussion) or eye injury, and reported not taking medication for anxiety, depression, or a related mental health condition, nor did they take any medication that may impact their response to exercise. All participants attained a complete score on the Physical Activity Readiness Questionnaire for Everyone (PAR-Q+ 2021) and completed the Godin Leisure-Time Exercise Questionnaire (Godin, 2011) (Mean = 52, SD =24, Range = 28–99). All participants abstained from engaging in strenuous exercise, caffeine, and alcohol use 12 h prior to the experimental protocol. Participants were also instructed to obtain 8 h of sleep the night prior to the experimental protocol. All study procedures were conducted between 12:00 p.m. and 3:00 p.m. Participants provided informed written consent of a protocol approved by the Health Sciences Research Ethics Board (#118590), Western University. This study was conducted in line with the updated version of the Declaration of Helsinki except for participant registration in a public database.
Procedure
Stress induction
The Trier Social Stress Test (TSST) is an ecologically valid stressor that has been used in hundreds of studies to explore the impact of acute stress of psychological and physiological processes (Kirschbaum et al., 1993; Dickerson and Kemeny, 2004). For the TSST participants were informed that they would perform a filmed task where they would present a job interview-style speech to a panel of two judges trained in public speaking. Notably, participants were not familiar with the individuals who served on the mock judging panel. A camera was visible during the filmed speech to induce a state of social evaluation; however, the camera did not record participants’ performance, and following the completion of the study participants were debriefed that filming did not take place. The participant was then left alone in the examination room for 10-min for speech preparation before the judges returned. Participants then delivered their 5-min speech after which they were instructed to perform serial subtractions of 13 from 1,022 (i.e., 1,022 minus 13; 996 minus 13; etc.) in front of the same panel of judges. The serial subtractions lasted for 5-min or until subjects reached 0 (see Birkett, 2011 for further details on the TSST protocol). The nomenclatures TSST-Speech and TSST-Math are subsequently used below to describe the TSST speech and serial subtraction components, respectively. Prior to and immediately following the TSST, participants completed a measure of state anxiety. Throughout all data collection participants wore a heart rate monitor (Polar H10 Wearlink + Coded Transmitter, Polar Electro Inc., Lake Success, NY, United States).
Exercise intervention
Participants (n = 20; 11 females, mean age: 20, range: 19–24) assigned to the Exercise Group sat on a cycle ergometer (Monark 818E Ergometer, Monark Exercise AB, Vansbro, Sweden) and completed a single bout of exercise that entailed: (1) a 2.5-min warm-up at an intensity less than 50% of their predicted maximum heart rate (i.e., HRmax; 220- age), (2) a step-transition to a 20-min session of heavy intensity exercise (i.e., 80% of HRmax), (3) a 2.5 min cool-down matching the intensity associated with the warm-up. The 80% of HRmax intensity was used based on previous work by our group demonstrating that a 20-min bout of exercise at the same intensity improves task-switching (Heath and Shukla, 2020; Shukla et al., 2020; Shukla and Heath, 2021). To maintain participants’ desired heart rate, the resistance on the cycle ergometer was adjusted as necessary. The postexercise oculomotor assessment (see below) was completed once heart rate returned to below 100 beats per minute (bpm).
Rest intervention
Participants (n = 20; 10 females, mean age: 23, range: 18–30) sat on the same ergometer used by the Exercise Group. Here, participants sat for an equivalent time period (i.e., 25-min) as the exercise intervention without cycling and heart rate was measured as per the Exercise Group. Participants chatted with the experimenter during this time. The post-rest intervention oculomotor assessment was completed following the same timeline as per the Exercise Group.
Stress measures
The State Trait Anxiety Inventory (STAI; Spielberger et al., 1983) was used to assess state and trait anxiety for all participants independent of group assignment. Twenty items assessed state anxiety and 20 items assessed trait anxiety with a higher score indicating increased anxiety. Trait anxiety was assessed upon entering the lab, whereas state anxiety was assessed at four time points: (1) upon entering the lab, (2) pre-TSST, (3) post-TSST, and (4) following either the Exercise or Rest intervention (i.e., post-intervention) (for timeline of events see Figure 1). Reliability estimates were computed via Cronbach’s alpha (α) and McDonald’s omega (ω)for the STAI State (Baseline) and Trait subscales. STAI State Baseline (α = 0.894, ω = 0.896) and Trait (α = 0.944, ω = 0.946).
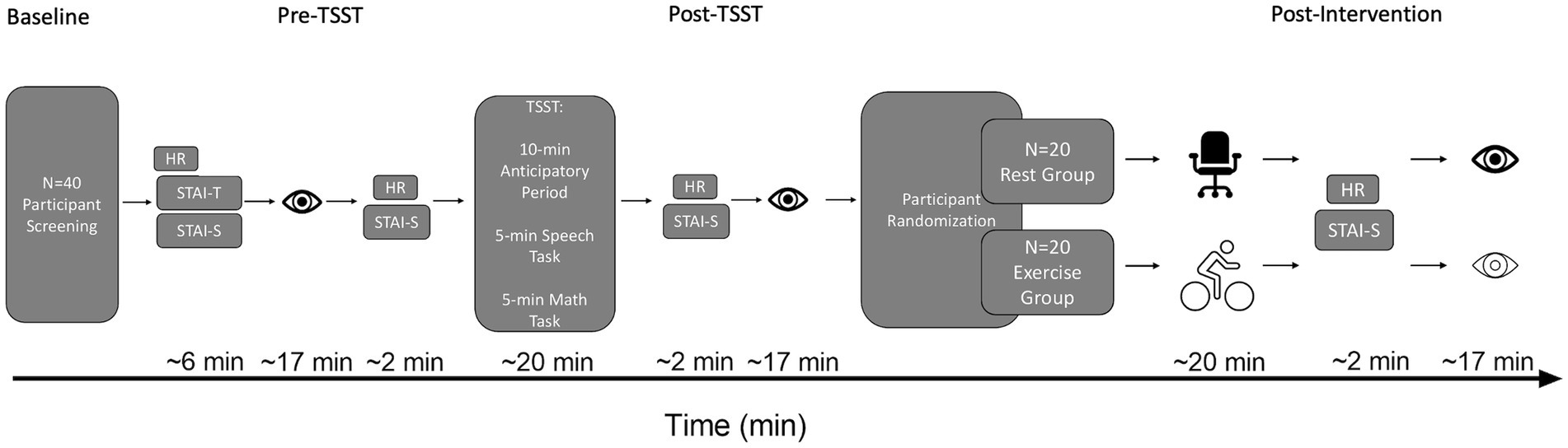
Figure 1. Schematic of the timeline of HR, STAI, and oculomotor assessments. The eye icon represents an oculomotor assessment. The desk chair icon represents the Rest group. The cyclist icon represents the Exercise group. HR, Heart rate; STAI-T, Trait Anxiety; STAI-S, State Anxiety; TSST, Trier Social Stress Test.
Oculomotor task
Prior to the TSST (i.e., pre-TSST), immediately following the TSST (i.e., post-TSST), and after Exercise and Rest interventions (i.e., post-intervention), participants completed an oculomotor task-switching paradigm (see Figure 1). For this assessment, participants sat on a height adjustable chair in front of a table (760 mm in height) with their head placed in a head/chin rest. Visual stimuli were presented on a 30-inch LCD monitor (60 Hz, 8 ms response rate, 1,280 × 960 pixels, Dell 3007WFP, Round Rock, TX, United States) placed 550 mm from the front edge of the tabletop and centered on participants’ midline and consisted of 1° luminance matched (40 cd/m2) red and green fixation crosses, as well as white target circles (2.5° diameter, 127 cd/m2) located 13° (i.e., proximal target) and 17° (i.e., distal target) to the left and right of fixation. The gaze location of participants’ left eye was measured via a video-based eye-tracking system (EyeLink 1000 Plus, SR Research, Ottawa, ON, Canada) sampling at 1,000 Hz. Prior to data collection a nine-point calibration of the viewing space was performed and confirmed via an immediate validation (<1° of error for each of the nine points in the calibration grid). Computer events were controlled via MATLAB (R2018b, The MathWorks, Natick, MA, United States) and the Psychophysics Toolbox extensions (v 3.0; Brainard, 1997; Kleiner et al., 2007) including the EyeLink Toolbox (Cornelissen et al., 2002). The lights in the experimental suite were extinguished during data collection.
A trial began with the presentation of a green or a red fixation cross which instructed participants to direct their gaze to its location. The color of the fixation cross indicated the nature of the upcoming trial. A green fixation cross indicated a prosaccade (i.e., saccade to veridical target location), whereas a red fixation cross indicated an antisaccade (i.e., saccade mirror-symmetrical to target location; see Figure 2).
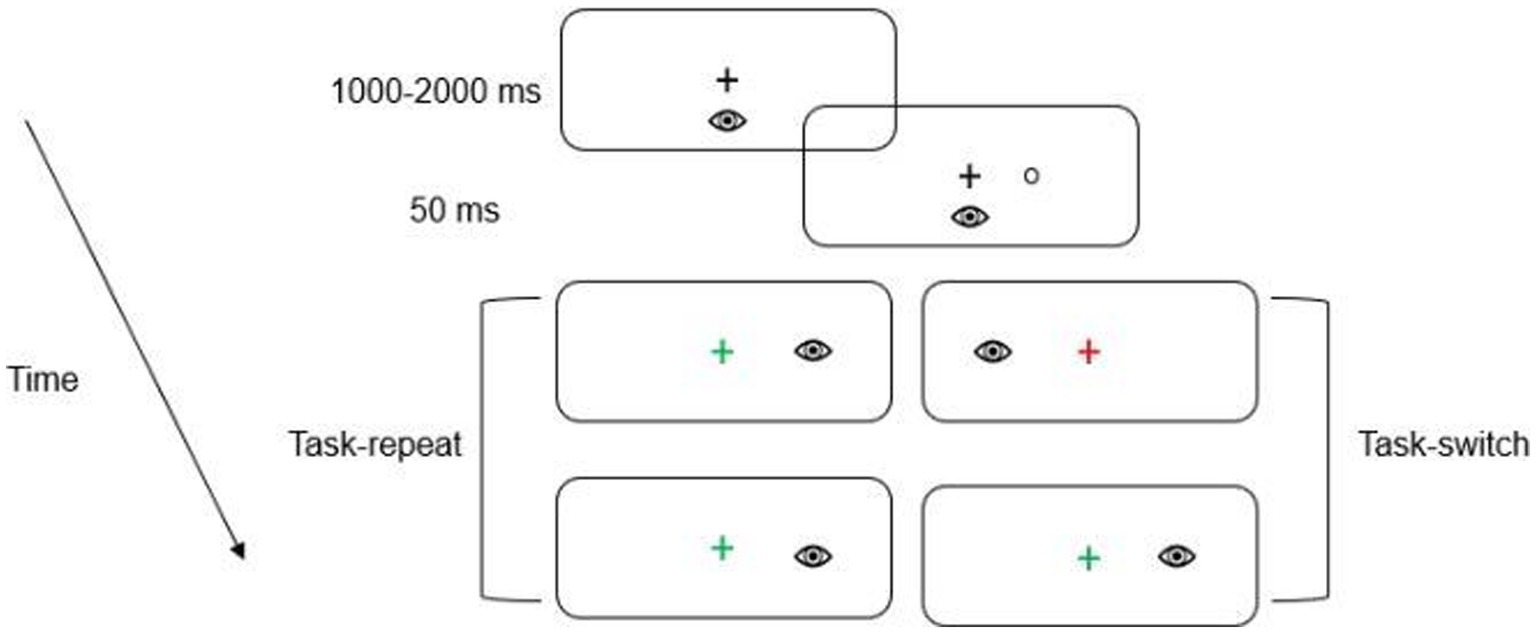
Figure 2. Schematic of the timeline of visual events. The first two panels depict the fixation cross and the presentation of the target, respectively. In this schematic, the green fixation cross denotes a saccade to the target’s veridical location (i.e., prosaccade), whereas the red fixation cross indicates a saccade to the target’s mirror-symmetrical (i.e., antisaccade) location. The subsequent panels depict an example of a task-repeat (i.e., prosaccade followed by a prosaccade) and task-switch (i.e., antisaccade followed by a prosaccade). Participants alternated between pro- and antisaccades after every second trial (i.e., AABB). For this schematic only a single target eccentricity presented in the right visual field is depicted.
Once a stable gaze was achieved (i.e., ±1.5° for 450 ms), a uniformly distributed randomized foreperiod between 1,000–2000 ms was initiated during which time the fixation cross remained visible. Following the foreperiod, a target was presented for 50 ms after which the target and fixation cross were extinguished (i.e., overlap paradigm). An overlap paradigm was used to minimize the frequency of directional errors (i.e., a prosaccade instead of an instructed antisaccade or vice versa) given that the primary metric for this study was RT for directionally correct responses. The onset of the target cued participants to pro- or antisaccade “as quickly and accurately as possible.” Pro- and antisaccades were arranged in a predictable AABB task-switching paradigm such that 40 prosaccade task-switch (i.e., prosaccade on trial N and an antisaccade on trial N-1) and 40 prosaccade task-repeat (i.e., prosaccade on trial N and N-1) trials were completed with an equivalent number of antisaccade task-switch and task-repeat trials (i.e., 160 trials). An AABB paradigm involves the conscious switching between distinct task sets (i.e., from a standard non-executive prosaccade task set to a non-standard, executive antisaccade task set and vice versa). Accordingly, the AABB task arrangement allows for the precise assessment of the cognitive flexibility domain of executive function (Diamond, 2013; see also above in more detail). For each oculomotor assessment an equal number of trials were pseudo-randomly presented to each target location (i.e., left proximal, left distal, right proximal, right distal). As shown in Figure 1, the oculomotor assessment of task-switching occurred pre-TSST, post-TSST and post-intervention. Each oculomotor assessment required ~17 min to complete (including calibration time) and is a time frame known to elicit a positive postexercise executive function benefit (Chang et al., 2012).
Data reduction, dependent variables, and statistical analyses
Stress variables
Mean heart rate (HR) was calculated by averaging data pre-TSST, 5-min TSST (Speech), 5-min TSST (Math) and post-TSST. STAI scores were tabulated for trait and state subscales, and the latter were summed at baseline, pre-TSST, post-TSST, and post-intervention.
Oculomotor variables
Point of gaze data were filtered offline using a dual-pass Butterworth filter employing a low-pass cut-off frequency of 15 Hz. A five-point central-finite difference algorithm was used to compute instantaneous velocities and accelerations. Saccade onset was determined via velocity and acceleration values that exceeded 30°/s and 8,000°/s2, respectively. Saccade offset was determined when velocity fell below 30°/s for 40 ms. Trials with missing data (e.g., blinks, signal loss) were excluded as were trials with: (1) an anticipatory response (RTs < 50 ms; Wenban-Smith and Findlay, 1991), (2) RTs > 2.5 standard deviations of a participant- and task-specific mean, and (3) trials with amplitudes <2° or >2.5 standard deviations of a participant- and task-specific mean (Gillen and Heath, 2014). Trials involving a directional error were excluded from subsequent analyses because such responses engage planning mechanisms distinct from their directionally correct counterparts (DeSimone et al., 2014). Less than 15% of trials were removed and only 2 and 4% of pro- and antisaccades entailed a directional error. The low error rate is attributed to the use of an overlap paradigm and the predictable nature of the AABB paradigm used here.
Statistical analyses
Stress variables included HR and STAI scores. Mean HR values were analyzed via 4 (time: pre-TSST, concurrent TSST-Speech and TSST-Math, post-TSST) by 2 (group: Rest vs. Exercise) mixed-model ANOVA with group serving as the between-groups factor. STAI values were analyzed via 4 (time: baseline, pre-TSST, post-TSST, and post-intervention) by 2 (group: Rest vs. Exercise) with the former serving as the between-groups factor. This procedure is in keeping with previous studies examining HR and STAI scores during the TSST (Hellhammer and Schubert, 2012). Oculomotor dependent variables included RT (i.e., time from response cueing to saccade onset), saccade duration (i.e., time from saccade onset to saccade offset), and saccade gain variability (i.e., within-participant standard deviation of saccade amplitude/veridical target location). Oculomotor dependent variables were examined via 3 (time: pre-TSST, post-TSST, post-intervention) by 2 (task: prosaccade, antisaccade) by 2 (task transition: task-switch, task-repeat) by 2 group (i.e., Rest vs. Exercise) mixed-groups ANOVA. Huynh-Feldt corrections for violations for sphericity are reported where appropriate (corrected degrees of freedom reported to one decimal place) and an alpha level of 0.05 was used for all ANOVA models. Interactions and appropriate main effects were decomposed via simple effects (i.e., paired-samples t-tests). Where appropriate, Bayesian statistics were employed to confirm null results and the two one-sided test (TOST) statistic is reported to determine whether results were within an equivalence boundary (Lakens, 2017). The effect size used to compute the TOST statistic (dz = 0.54) was derived from paired-samples t-tests comparing prosaccade task-switch and task-repeat trials following Exercise and Rest interventions.
Results
Stress measures
Heart rate
Results produced a main effect of time, F(3, 114) = 96.67, p < 0.001, ηp2 = 0.72. Figure 3 shows an increase in HR during the TSST-Speech and TSST-Math portions in relation to the pre-TSST [all t(39) = −11.25, and − 9.28, ps < 0.001, dz = −1.78 and − 1.47] and that values for the 10-min post-TSST did not reliably differ from the pre-TSST assessment [t(39) = 0.42, p = 0.67, dz = 0.07]. Notably, a null time by group interaction, F(3, 114) = 1.32, p = 0.27, ηp2 = 0.03, indicated that HR values did not reliably differ between Exercise and Rest groups.
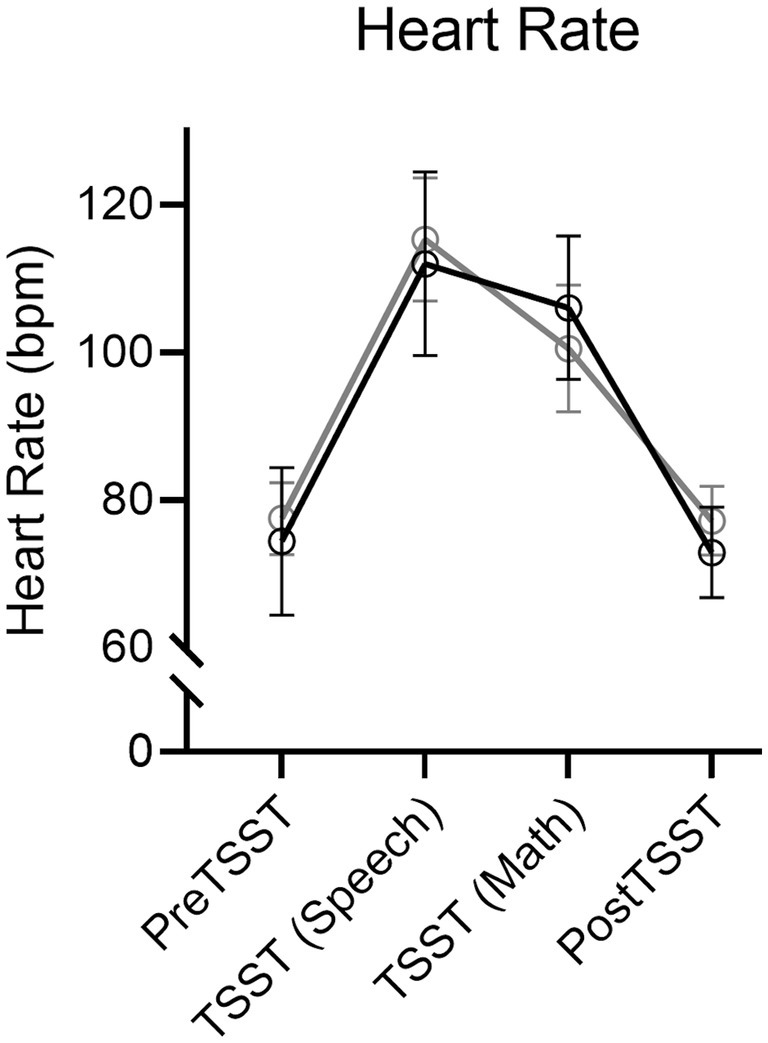
Figure 3. Mean heart rate (in beats/min: bpm) and associated 95% confidence intervals for Exercise and Rest groups at baseline, TSST-Speech, TSST-Math, and Post-TSST.
State trait anxiety inventory
Results produced a main effect of time, F(2.2, 83.9) = 108.03, p < 0.001, ηp2 = 0.74. STAI scores increased from pre-TSST to post-TSST [t(39) = 6.12, p = <0.001, dz = 0.97] (see Figure 4) however, a null time by group interaction, F(2.2, 83.9) = 1.03, p = 0.37, ηp2 = 0.03, indicated that STAI scores did not reliably differ between Exercise and Rest groups.
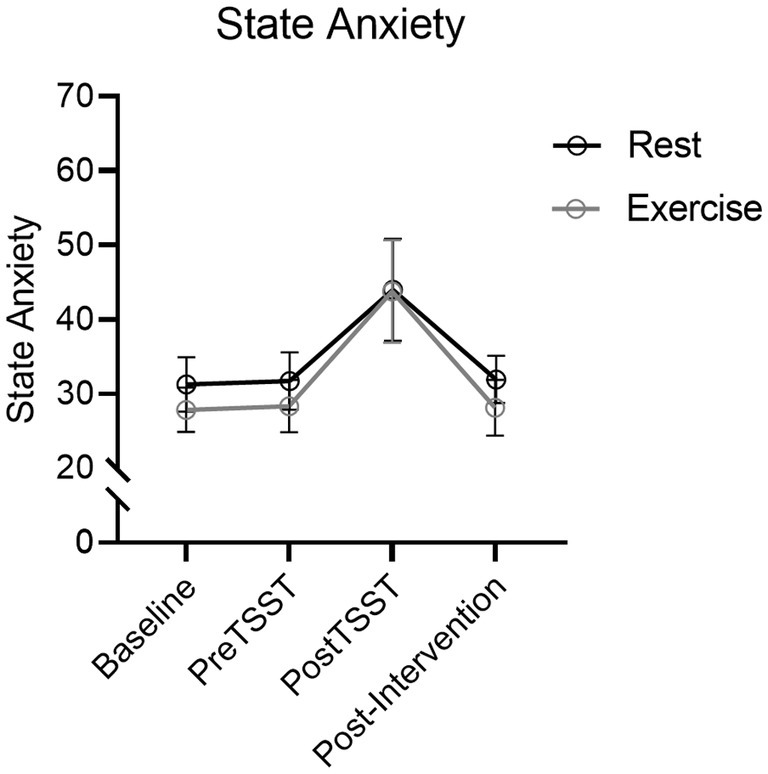
Figure 4. STAI mean values for Exercise and Rest groups at baseline, TSST-Speech, TSST-Math and Post-TSST. Error bars represent 95% within-participant confidence intervals.
Oculomotor measures
Reaction time
Results produced main effects of time, F(2, 76) = 16.91, p < 0.001, ηp2 = 0.31, task, F(1, 38) = 162.24, p < 0.001, ηp2 = 0.81, and task transition, F(1, 38) = 8.48, p = 0.006, ηp2 = 0.18, as well as interactions involving task by task transition, F(1, 38) = 12.54, p = 0.001, ηp2 = 0.25, and time by task by task transition, F(2, 76) = 9.69, p < 0.001, ηp2 = 0.20. As expected, RTs for prosaccades (201 ms, SD = 36) were shorter than antisaccades (254 ms, SD = 43)—a finding consistent across task-switch and task-repeat trials. In decomposing the three-way interaction, we computed task by task transition ANOVAs separately for the Pre-TSST, Post-TSST, and Post-intervention oculomotor assessments. Figures 5, 6 demonstrate that pre-TSST and post-intervention assessments produced task by task transition interactions, all F(1, 38) = 27.22 and 7.70, ps < 0.001 and 0.009, all ηp2 = 0.42 and 0.17, indicating that prosaccade task-switch RTs were longer than their task-repeat counterparts [all t(39) = 4.76 and 3.39 for pre-TSST and post-intervention, respectively, ps < 0.001 and = 0.02, dz = 0.75 and 0.54], whereas antisaccade task-switch and task-repeat trials did not reliably differ [all t(39) < −1.78, ps > 0.08, dz < −0.28]. In turn, the post-TSST assessment did not yield a task by task-transition interaction, F(1, 38) = 0.49, p = 0.49, all ηp2 = 0.01. In other words, the unidirectional prosaccade switch-cost was observed pre-TSST and following Exercise and Rest interventions but not following TSST administration. Further, and given the nature of our research question, we note that results did not yield a main effect of group, F(1, 38) = 0.05, p = 0.82, ηp2 = 0.001, nor any higher-order interaction involving group, all Fs(2, 76) < 1.47, ps > 0.24, all ηp2 < 0.04. As well, we note that TOST statistics indicated that post-TSST switch-costs for the Exercise (12 ms, SD = 24) and Rest (12 ms, SD = 22) groups were within an equivalence boundary, t(37.7) = 1.71, p = 0.048 (dz = 0.54).
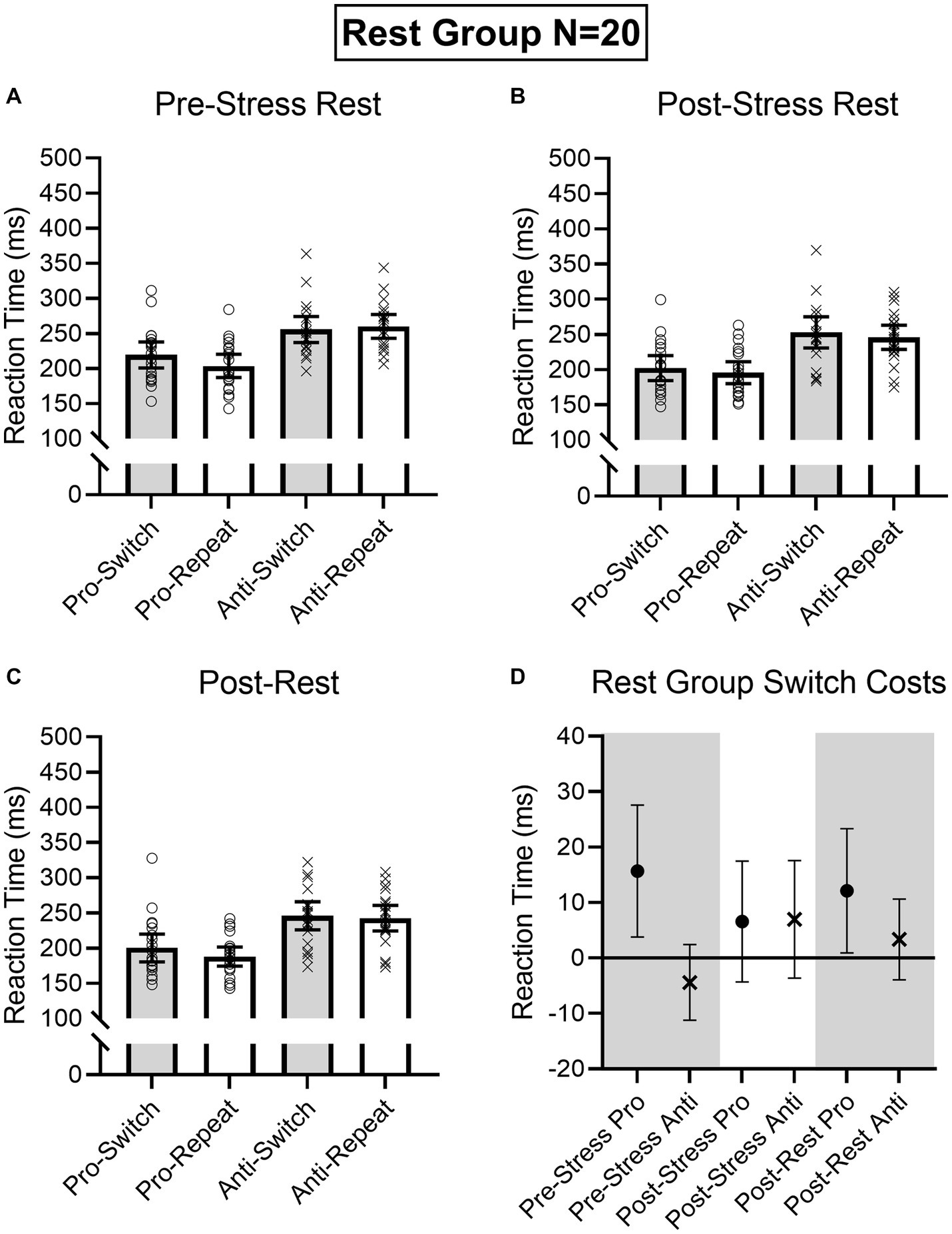
Figure 5. Rest group participant-specific pro- and antisaccade task-switch and task-repeat reaction times (i.e., open symbols) and associated group means at baseline (A), post-TSST (B), and post-Rest (C). Error bars represent 95% within-participant confidence intervals. As well, the lower right panel (D) shows difference scores and 95% between-participant confidence intervals relating baseline reaction times to each subsequent oculomotor assessment. For this panel, the absence of overlap between the error bar and zero (i.e., the horizontal line) represents a reliable effect inclusive to a test of the null hypothesis.
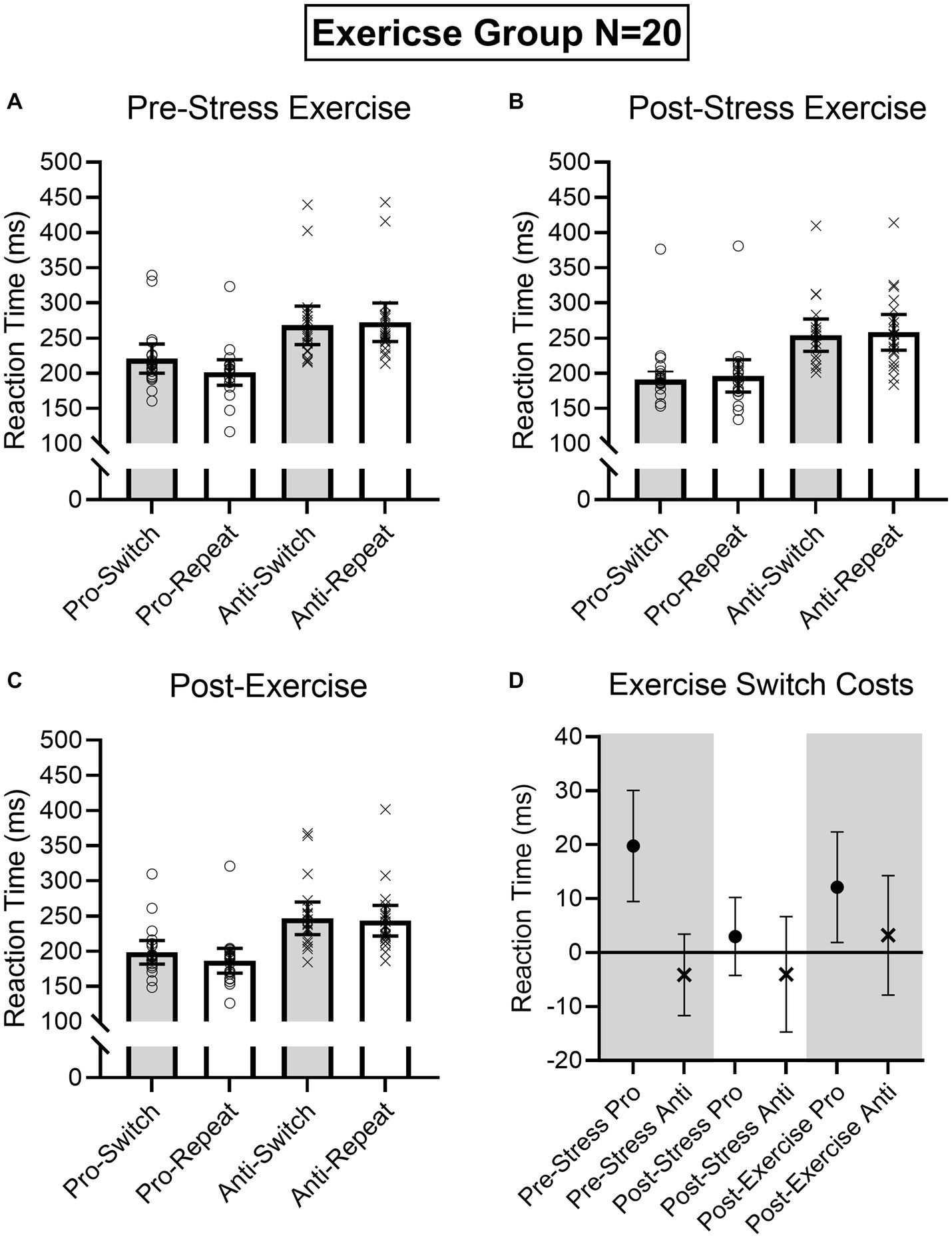
Figure 6. Exercise group participant-specific pro- and antisaccade task-switch and task-repeat reaction times (i.e., open symbols) and associated group means at baseline (A), post-TSST (B), and post-Exercise (C). Error bars represent 95% within-participant confidence intervals. As well, the lower right panel (D) shows difference scores and 95% between-participant confidence intervals relating baseline reaction times to each subsequent oculomotor assessment. For this panel, the absence of overlap between the error bar and zero (i.e., the horizontal line) represents a reliable effect inclusive to a test of the null hypothesis.
Given the importance of evaluating the null findings, in addition to the frequentist statistics provided above, we conducted single-sample Bayesian statistics comparing prosaccade switch-costs (i.e., task-switch minus task-repeat trial) separately for each assessment interval (i.e., pre-TSST, post-TSS and post-intervention). Bayes factors for the alternative hypotheses (i.e., BF10) at pre-TSST, post-TSST and post-intervention were 803.36, 0.507, and 19.94, respectively. In line with van Doorn et al.’s (2021) nomenclature such findings evidence “very strong” and “strong” evidence for the alternative hypothesis, respectively, for the pre-TSST and post-intervention assessments. In turn, the value for the post-TSST provided less than ‘anecdotal’ evidence for the alternative hypothesis. Moreover, evaluation of the post-TSST Bayes factor for the null hypothesis (i.e., BF01 = 4.24) indicated that the null hypothesis was more likely than the alternative. In other words, our exercise manipulation did not modulate the unidirectional prosaccade switch-cost.
Saccade duration and gain variability
Results yielded main effects of task, all Fs(1, 38) = 20.91 and 84.36, ps < 0.001, all ηp2 = 0.36 and 0.69. Antisaccade durations were longer (75 ms, SD = 14) and endpoints were more variable (0.22, SD = 0.08) than prosaccades (saccade duration: 68 ms, SD = 8; saccade gain: 0.12, SD = 0.08).
Discussion
We examined the effects of acute stress and a single bout of aerobic exercise on cognitive flexibility via the AABB pro- and antisaccade task-switching paradigm. In addressing our findings, commentary is first provided regarding the effects of acute stress on cognitive flexibility before discussing whether our exercise manipulation modified a stress-induced disruption to cognitive flexibility.
The TSST produces a reliable and transient stress response
HR was elevated from pre-TSST to TSST-Speech (38 bpm) and TSST-Math (27 bpm) and are values on par to the respective 31 bpm and 27 bpm HR increases in TSST-Speech and TSST-Math reported by Rosenbaum et al. (2018). HR values returned to pre-TSST values approximately 15 min after the TSST. In terms of STAI results, values increased from pre- to post-TSST and the magnitude of this increase is on par with previous work (e.g., Birkett, 2011). Hence, the HR and STAI data demonstrate the TSST elicited a reliable stress response and thus serves as a framework by which to assess stress-induced changes in cognitive flexibility.
An acute stressor boosts task- switching performance
Pre-TSST prosaccade task-switch RTs were longer than their task-repeat counterparts, whereas antisaccade task-switch and task-repeat RTs did not differ. In other words, a unidirectional prosaccade switch-cost was present pre-stress and is in line with prior work by our group (Weiler and Heath, 2012a, 2014a; Weiler et al., 2015; Heath et al., 2016; Tari and Heath, 2019 Tari et al., 2019 Heath and Shukla, 2020; Shukla et al., 2020; Shukla and Heath, 2021) and others (Manoach et al., 2007; Chan and DeSouza, 2013). When considering this finding, it is important to note that some have argued that the RT difference between prosaccade task-switch and task-repeat trials may reflect a repetition; that is, the second of two consecutively performed prosaccades is associated with an RT reduction. Notably, however, a purpose-designed study by our group showed that prosaccade task-repetition trials yield RTs on par to prosaccades executed in a separate block of trials (Weiler and Heath, 2014a). In other words, results support the assertion that the RT difference between prosaccade task-switch and task-repeat trials reflect a switch-cost wherein the planning times for the former trial-type are delayed due to a task-set inertia from a previously completed antisaccade trial (Weiler and Heath, 2014a; Weiler et al., 2015; see also Tari et al., 2019).
Although a unidirectional prosaccade switch-cost was observed pre-stress, it was not present post-stress. The absence of a switch-cost post-stress cannot be attributed to an “implicit – or explicit – control strategy” (Heath and Shukla, 2020) designed to increase prosaccade task-switch trial planning times (i.e., speed-accuracy trade-off) given that saccade durations and endpoint accuracy for all trial-types did not vary across the different assessment intervals. Moreover, and as will be discussed in more detail below, the absence of a prosaccade switch-cost cannot be attributed to a practice-effect given that a switch-cost was observed at the Exercise and Rest group post-intervention assessments. Indeed, at the outset we were surprised by the absence of a post-TSST switch-cost given that a meta-analysis of the extant (Shields et al., 2016) concluded that cognitive flexibility is adversely impacted by an acute stressor. However, it is important to acknowledge that prior work has reported findings similar to those observed here. For example, Gabrys et al. (2019) investigated the effects of the TSST on cognitive flexibility via the computer-based Berg Card Sorting Task (BCST). The BCST comprises of an electronic card deck wherein each card contains a different assortment of one of four colors, shapes, and quantities. Target cards are presented and the participants is required to sort response cards according to different sorting rules (which are unknown to the participant). The primary outcome for the BCST was perseverative errors (i.e., sorting cards in accordance with the previous sorting rule) and results showed that participants exposed to the TSST exhibited reduced perseverative errors compared to a control group. Similarly, Goldfarb et al. (2017) examined the effects of the cold pressor task on cognitive flexibility via the modified delayed match-to-sample task. In the first phase of the delayed match-to-sample task, participants learned two colored stimuli, whereas in the second phase participants were required to either remember the colored stimuli or to forget the stimuli and learn two novel stimuli (i.e., a task requiring cognitive flexibility). Participants exposed to the cold pressor task demonstrated improved cognitive flexibility which the authors asserted may be due to the fact that the ability to incorporate newly relevant information relies on the dorsal striatum, which has been documented to show enhanced functioning following acute stress (Packard, 2009; Delgadoy Palacios et al., 2014). Hence, there is evidence indicating that a stressor may serve to focus or limit attentional resources and support disengagement from task-irrelevant information. In other words, an acute stressor may provide a level of task-engagement and attentional selectively supporting a transient benefit to task-switching.
Exercise does not modulate task-switching following stress
A single bout of aerobic exercise did not modulate post-stress task-switching performance. Instead, our post-stress assessment revealed an equivalent magnitude unidirectional prosaccade switch-cost for Exercise and Rest groups. This represents an unexpected result given a myriad of previous studies showing that a single bout of aerobic exercise provides a positive benefit to each core component of executive function (for reviews see, Lambourne and Tomporowski, 2010; Chang et al., 2012; Morris et al., 2020). Indeed, a previous investigation in our lab showed a 14 ms decrease (dz = 0.72) to the magnitude of the unidirectional switch-cost when completed immediately and up to 47-min following a 20-min single bout of heavy intensity aerobic exercise (i.e., the same exercise protocol as used here; see Heath and Shukla, 2020; Shukla et al., 2020; Shukla and Heath, 2021). In contextualizing the present work with earlier work, the prior studies did not employ an acute stress manipulation. Thus, in the subsequent section we address why an acute stressor may “blunt” a postexercise benefit to executive function.
Neurobiological mechanisms
The interaction of several neurobiological systems likely influences the relationship between stress, exercise and cognitive flexibility (for reviews of stress on neural systems and the prefrontal cortex see, Arnsten, 2009; Joëls and Baram, 2009). It is well-documented that immediately after a stressor there is a rapid increase in the concentration of monoamines and neuropeptides (e.g., dopamine, serotonin, norepinephrine, and corticotropin-releasing hormone) in frontoparietal regions (Joëls and Baram, 2009), and that such a change may alter executive function. For example, increases in dopamine and norepinephrine alter neuronal firing patterns in the prefrontal cortex (Joëls et al., 2006; McMorris, 2016) and this state change in animal models has been shown to facilitate high-level movement planning and decision-making (Barbas, 2000) and in humans has been shown to elicit improved executive function via increased task-based attentional focus (McMorris, 2016). A second mechanism may reflect the release of cortisol from the HPA axis (Joëls and Baram, 2009). Cortisol exerts both fast-acting (i.e., non-genomic) and slow-acting (i.e., genomic) effects on neurobiological systems (Joëls et al., 2011) and is documented to affect the activity of local neural networks supporting executive function (Reul and Kloet, 1985). For example, Schwabe et al. (2013) administered to participants a mineralocorticoid antagonist (i.e., an agent that blocks the stimulating effects of cortisol) or a placebo prior to a stressor (i.e., cold pressor with element of social evaluation) or a non-stressor. After the stressor, participants performed the stop-signal task (i.e., task of inhibitory control) and results indicated that exposure to a stressor enhanced inhibitory control; however, this result was not present following when the stressor was preceded by a mineralocorticoid antagonism. Put another way, acute stress may modulate executive function, in part, via cortisol.
In addressing the absence of a postexercise benefit to task-switching, we note the time-dependent release of cortisol exerts fast (i.e., non-genomic) and slow (i.e., genomic) effects. As the final oculomotor assessment was administered approximately 55 min following completion of the stress-inducing event (i.e., the TSST), this likely coincided with the beginning of the “slow, genomic effects” of cortisol (Joëls and Baram, 2009). The genomic effects of cortisol have been documented to impede components of executive function (Shields et al., 2015) and may blunt the putative neuroprotective benefit that a single bout of exercise provides to task-switching.
Study limitations
First, cortisol was not measured and therefore precludes direct interpretation of how it may have influenced task-switching efficiency. Second, the present work employed only a 20-min bout of high-intensity aerobic exercise and evaluated cognitive flexibility via task-switching at a single postexercise timepoint (i.e., immediately after exercise). Thus, it is unclear whether different exercise durations across the continuum of exercise intensities would induce a similar result and it is unclear whether exercise may produce a delayed improvement in executive function. Third, our results are limited to healthy and recreationally active young adults. As the current work does not include a pre-registered replication, we would strongly encourage future works to consider a replication of this study in similarly aged, middle-aged, and old-aged healthy, cognitively intact individuals. Indeed, given that older adults show differing levels of reactivity to stress-inducing events (Uchino et al., 2005) and to exercise (Ludyga et al., 2016) it remains unknown whether our findings extend to broader populations. Last, including another measure of arousal such as pupillometry (see Ayala and Heath, 2021) would afford insight into the mechanisms underlying changes to cognitive flexibility as a result of acute stress.
Conclusion
The current findings provide insight into the complex dynamics between acute stress and cognitive flexibility. Namely, acute stress reduces the magnitude of a unidirectional prosaccade switch-cost and that a single bout of heavy intensity exercise did not differentially modulate these effects compared to rest.
Data availability statement
The raw data supporting the conclusions of this article will be made available by the authors, without undue reservation.
Ethics statement
The studies involving human participants were reviewed and approved by Western University Research Ethics Board. The patients/participants provided their written informed consent to participate in this study.
Author contributions
AM, BT, HP, and MH conceptualized the study, contributed to the methodology, and data analysis. AM, JA, and MS collected the data. AM wrote the initial manuscript draft. HP and MH reviewed and edited the manuscript, supervised the study. MH acquired grant funding. All authors contributed to the article and approved the submitted version.
Funding
This work was supported by the Natural Sciences and Engineering Research Council Discovery Grant (MH).
Conflict of interest
The authors declare that the research was conducted in the absence of any commercial or financial relationships that could be construed as a potential conflict of interest.
Publisher’s note
All claims expressed in this article are solely those of the authors and do not necessarily represent those of their affiliated organizations, or those of the publisher, the editors and the reviewers. Any product that may be evaluated in this article, or claim that may be made by its manufacturer, is not guaranteed or endorsed by the publisher.
References
Alderman, B. L., Arent, S. M., Landers, D. M., and Rogers, T. J. (2007). Aerobic exercise intensity and time of stressor administration influence cardiovascular responses to psychological stress. Psychophysiology 44, 759–766. doi: 10.1111/j.1469-8986.2007.00548.x
Allan, J. L., McMinn, D., and Daly, M. (2016). A bidirectional relationship between executive function and health behavior: evidence, implications, and future directions. Front. Neurosci. 64, 135–168. doi: 10.1146/annurev-psych-113011-143750
Arnsten, A. F. (2009). Stress signalling pathways that impair prefrontal cortex structure and function. Nat. Rev. Neurosci. 10, 410–422. doi: 10.1038/nrn2648
Ayala, N., and Heath, M. (2021). Pupillometry reveals the role of arousal in a postexercise benefit to executive function. Brain Sci. 11:1048. doi: 10.3390/brainsci11081048
Barbas, H. (2000). Connections underlying the synthesis of cognition, memory, and emotion in primate prefrontal cortices. Brain Res. Bull. 52, 319–330. doi: 10.1016/S0361-9230(99)00245-2
Birkett, M. A. (2011). The Trier social stress test protocol for inducing psychological stress. J. Vis. Exp. 56:3238. doi: 10.3791/3238
Brainard, D. H. (1997). The psychophysics toolbox. Spat. Vis. 10, 433–436. doi: 10.1163/156856897X00357
Brownley, K. A., Hinderliter, A. L., West, S. G., Girdler, S. S., Sherwood, A., and Light, K. C. (2003). Sympathoadrenergic mechanisms in reduced hemodynamic stress responses after exercise. Med. Sci. Sports Exerc. 35, 978–986. doi: 10.1249/01.MSS.0000069335.12756.1B
Chan, J. L., and DeSouza, J. F. (2013). The effects of attentional load on saccadic task switching. Exp. Brain Res. 227, 301–309. doi: 10.1007/s00221-013-3452-1
Chang, Y. K., Labban, J. D., Gapin, J. I., and Etnier, J. L. (2012). The effects of acute exercise on cognitive performance: a meta-analysis. Brain Res. 1453, 87–101. doi: 10.1123/japa.20.4.497
Chrousos, G. P., and Gold, P. W. (1992). The concepts of stress and stress system disorders: overview of physical and behavioral homeostasis. JAMA 267, 1244–1252. doi: 10.1001/jama.1992.03480090092034
Cornelissen, F. W., Peters, E. M., and Palmer, J. (2002). The Eyelink toolbox: eye tracking with MATLAB and the psychophysics toolbox. Behav. Res. Methods Instrum. Comput. 34, 613–617. doi: 10.3758/bf03195489
Delgadoy Palacios, R., Verhoye, M., Hennigsen, K., Wiborg, O., and Van der Linden, A. (2014). Diffusion kurtosis imaging and high-resolution MRI demonstrate structural aberrations of caudate putamen and amygdala after chronic mild stress. PLoS One 9:e95077. doi: 10.1371/journal.pone.0095077
DeSimone, J. C., Weiler, J., Aber, G. S., and Heath, M. (2014). The unidirectional prosaccade switch-cost: correct and error antisaccades differentially influence the planning times for subsequent prosaccades. Vis. Res. 96, 17–24. doi: 10.1016/j.visres.2013.12.005
Diamond, A. (2013). Executive functions. Annu. Rev. Psychol. 64, 135–168. doi: 10.1146/annurev-psych-113011-143750
Dickerson, S. S., and Kemeny, M. E. (2004). Acute stressors and cortisol responses: a theoretical integration and synthesis of laboratory research. Psychol. Bull. 130, 355–391. doi: 10.1037/0033-2909.130.3.355
Gabrys, R. L., Howell, J. W., Cebulski, S. F., Anisman, H., and Matheson, K. (2019). Acute stressor effects on cognitive flexibility: mediating role of stressor appraisals and cortisol. Stress 22, 182–189. doi: 10.1080/10253890.2018.1494152
Gillen, C., and Heath, M. (2014). Target frequency influences antisaccade endpoint bias: evidence for perceptual averaging. Vis. Res. 105, 151–158. doi: 10.1016/j.visres.2014.10.010
Godin, G. (2011). The Godin-Shephard leisure-time physical activity questionnaire. Health Fit J Canada 4, 18–22. doi: 10.14288/hfjc.v4i1.82
Goldfarb, E. V., Frobose, M. I., Cools, R., and Phelps, E. A. (2017). Stress and cognitive flexibility: cortisol increases are associated with enhanced updating but impaired switching. J. Cogn. Neurosci. 29, 14–24. doi: 10.1162/jocn_a_01029
Hallett, P. E. (1978). Primary and secondary saccades to goals defined by instructions. Vis. Res. 18, 1279–1296. doi: 10.1016/0042-6989(78)90218-3
Heath, M., Gillen, C., and Samani, A. (2016). Alternating between pro-and antisaccades: switch-costs manifest via decoupling the spatial relations between stimulus and response. Exp. Brain Res. 234, 853–865. doi: 10.1007/s00221-015-4510-7
Heath, M., Hassall, C. D., MacLean, S., and Krigolson, O. E. (2015). Event-related brain potentials during the visuomotor mental rotation task: the contingent negative variation scales to angle of rotation. Neuroscience 311, 153–165. doi: 10.1016/j.neuroscience.2015.10.018
Heath, M., and Shukla, D. (2020). A single bout of aerobic exercise provides an immediate “boost” to cognitive flexibility. Front. Psychol. 11:1106. doi: 10.3389/fpsyg.2020.01106
Hellhammer, J., and Schubert, M. (2012). The physiological response to Trier social stress test relates to subjective measures of stress during but not before or after the test. Psychoneuroendocrinology 37, 119–124. doi: 10.1016/j.psyneuen.2011.05.012
Joëls, M., and Baram, T. Z. (2009). The neuro-symphony of stress. Nat. Rev. Neurosci. 10, 459–466. doi: 10.1038/nrn2632
Joëls, M., Fernandez, G., and Roozendaal, B. (2011). Stress and emotional memory: a matter of timing. Trends Cogn. Sci. 15, 280–288. doi: 10.1016/j.tics.2011.04.004
Joëls, M., Pu, Z., Wiegert, O., Oitzl, M. S., and Krugers, H. J. (2006). Learning under stress: how does it work? Trends Cogn. Sci. 10, 152–158. doi: 10.1016/j.tics.2006.02.002
Kirschbaum, C., Pirke, K. M., and Hellhammer, D. H. (1993). The ‘Trier social stress test’–a tool for investigating psychobiological stress responses in a laboratory setting. Neuropsychobiology 28, 76–81. doi: 10.1159/000119004
Kleiner, M., Brainard, D., and Pelli, D. (2007). What's new in Psychtoolbox-3? Perception 36, 1–16. doi: 10.1177/03010066070360S101
Knaepen, K., Goekint, M., Heyman, E. M., and Meeusen, R. (2010). Neuroplasticity - exercise-induced response of peripheral brain-derived neurotrophic factor: a systematic review of experimental studies in human subjects. Sports Med. 40, 765–801. doi: 10.2165/11534530-000000000-00000
Lakens, D. (2017). Equivalence tests: a practical primer for t tests, correlations, and meta-analyses. Soc. Psychol. Personal. Sci. 8, 355–362. doi: 10.1177/1948550617697177
LaManca, J. J., Peckerman, A., Sisto, S. A., DeLuca, J., Cook, S., and Natelson, B. H. (2001). Cardiovascular responses of women with chronic fatigue syndrome to stressful cognitive testing before and after strenuous exercise. Psychosom. Med. 63, 756–764. doi: 10.1097/00006842-200109000-00009
Lambourne, K., and Tomporowski, P. (2010). The effect of exercise-induced arousal on cognitive task performance: a meta-regression analysis. Brain Res. 1341, 12–24. doi: 10.1016/j.brainres.2010.03.091
Ludyga, S., Gerber, M., Brand, S., Holsboer-Trachsler, E., and Pühse, U. (2016). Acute effects of moderate aerobic exercise on specific aspects of executive function in different age and fitness groups: a meta-analysis. Psychophysiology 53, 1611–1626. doi: 10.1111/psyp.12736
Manoach, D. S., Thakkar, K. N., Cain, M. S., Polli, F. E., Edelman, J. A., Fischl, B., et al. (2007). Neural activity is modulated by trial history: a functional magnetic resonance imaging study of the effects of a previous antisaccade. J. Neurosci. 27, 1791–1798. doi: 10.1523/JNEUROSCI.3662-06.2007
Mather, M., and Sutherland, M. R. (2011). Arousal-biased competition in perception and memory. Perspect. Psychol. Sci. 6, 114–133. doi: 10.1177/1745691611400234
McMorris, T. (2016). Developing the catecholamines hypothesis for the acute exercise-cognition interaction in humans: lessons from animal studies. Physiol. Behav. 165, 291–299. doi: 10.1016/j.physbeh.2016.08.011
Moore, C. I., and Cao, R. (2008). The hemo-neural hypothesis: on the role of blood flow in information processing. J. Neurophysiol. 99, 2035–2047. doi: 10.1152/jn.01366.2006
Morris, T. P., Fried, P. J., Macone, J., Stillman, A., Gomes-Osman, J., Costa-Miserachs, D., et al. (2020). Light aerobic exercise modulates executive function and cortical excitability. Eur. J. Neurosci. 51, 1723–1734. doi: 10.1111/ejn.14593
Munoz, D. P., and Everling, S. (2004). Look away: the anti-saccade task and the voluntary control of eye movement. Nat. Rev. Neurosci. 5, 218–228. doi: 10.1038/nrn1345
Packard, M. G. (2009). Anxiety, cognition, and habit: a multiple memory systems perspective. Brain Res. 1293, 121–128. doi: 10.1016/j.brainres.2009.03.029
Petrella, A. F., Belfry, G., and Heath, M. (2019). Older adults elicit a single-bout post-exercise executive benefit across a continuum of aerobically supported metabolic intensities. Brain Res. 1712, 197–206. doi: 10.1016/j.brainres.2019.02.009
Plessow, F., Fischer, R., Kirschbaum, C., and Goschke, T. (2011). Inflexibly focused under stress: acute psychosocial stress increases shielding of action goals at the expense of reduced cognitive flexibility with increasing time lag to the stressor. J. Cogn. Neurosci. 23, 3218–3227. doi: 10.1162/jocn_a_00024
Plieger, T., and Reuter, M. (2020). Stress and executive functioning: a review considering moderating factors. Neurobiol. Learn. Mem. 173:107254. doi: 10.1016/j.nlm.2020.107254
Reul, J. M. H. M., and Kloet, E. D. (1985). Two receptor systems for corticosterone in rat brain: microdistribution and differential occupation. Endocrinology 117, 2505–2511. doi: 10.1210/endo-117-6-2505
Rosenbaum, D., Hilsendegen, P., Thomas, M., Haeussinger, F. B., Metzger, F. G., Nuerk, H. C., et al. (2018). Cortical hemodynamic changes during the trier social stress test: an fNIRS study. NeuroImage 171, 107–115. doi: 10.1016/j.neuroimage.2017.12.061
Sandi, C. (2013). Stress and cognition. Wiley interdisciplinary reviews. Cognitive science 4, 245–2619. doi: 10.1002/wcs.1222
Schmitt, A., Upadhyay, N., Martin, J. A., Rojas, S., Strüder, H. K., and Boecker, H. (2019). Modulation of distinct intrinsic resting state brain networks by acute exercise bouts of differing intensity. Brain Plast. 5, 39–55. doi: 10.3233/BPL-190081
Schwabe, L., Höffken, O., Tegenthoff, M., and Wolf, O. T. (2013). Stress-induced enhancement of response inhibition depends on mineralocorticoid receptor activation. Psychoneuroendocrinology 38, 2319–2326. doi: 10.1016/j.psyneuen.2013.05.001
Shansky, R. M., and Lipps, J. (2013). Stress-induced cognitive dysfunction: hormone-neurotransmitter interactions in the prefrontal cortex. Front. Hum. Neurosci. 7:123. doi: 10.3389/fnhum.2013.00123
Shields, G. S., Bonner, J. C., and Moons, W. G. (2015). Does cortisol influence core executive functions? A meta-analysis of acute cortisol administration effects on working memory, inhibition, and set-shifting. Psychoneuroendocrinology 58, 91–103. doi: 10.1016/j.psyneuen.2015.04.017
Shields, G. S., Sazma, M. A., and Yonelinas, A. P. (2016). The effects of acute stress on core executive functions: a meta-analysis and comparison with cortisol. Neurosci. Biobehav. Rev. 68, 651–668. doi: 10.1016/j.neubiorev.2016.06.038
Shukla, D., Al-Shamil, Z., Belfry, G., and Heath, M. (2020). A single bout of moderate intensity exercise improves cognitive fexibility: evidence from task-switching. Exp. Brain Res. 238, 2333–2346. doi: 10.1007/s00221-020-05885-w
Shukla, D., and Heath, M. (2021). A single bout of exercise provides a persistent benefit to cognitive flexibility. Res. Q. Exerc. Sport 93, 516–527. doi: 10.1080/02701367.2021.1873902
Spielberger, C. D., Gorsuch, R. L., Lushene, R., Vagg, P. R., and Jacobs, G. A. (1983). Manual for the State-Trait Anxiety Inventory. Palo Alto, CA: Consulting Psychologists Press.
Tari, B., and Heath, M. (2019). Pro-and antisaccade task-switching: response suppression—and not vector inversion—contributes to a task-set inertia. Exp. Brain Res. 237, 3475–3484. doi: 10.1007/s00221-019-05686-w
Tari, B., Fadel, M. A., and Heath, M. (2019). Response suppression produces a switch-cost for spatially compatible saccades. Exp. Brain. Res. 237, 1195–1203. doi: 10.1007/s00221-019-05497-z
Tari, B., Vanhie, J. J., Belfry, G. R., Shoemaker, J. K., and Heath, M. (2020). Increased cerebral blood flow supports a single-bout postexercise benefit to executive function: evidence from hypercapnia. J. Neurophysiol. 124, 930–940. doi: 10.3758/s13428-021-01735-x
Uchino, B. N., Holt-Lunstad, J., Bloor, L. E., and Campo, R. A. (2005). Aging and cardiovascular reactivity to stress: longitudinal evidence for changes in stress reactivity. Psychol. Aging 20, 134–143. doi: 10.1037/0882-7974.20.1.134
van Doorn, J., van den Bergh, D., Böhm, U., Dablander, F., Derks, K., Draws, T., et al. (2021). The JASP guidelines for conducting and reporting a Bayesian analysis. Psychon. J. Neurosci. 28, 813–826. doi: 10.3758/s13423-020-01798-5
Weiler, J., Hassall, C. D., Krigolson, O. E., and Heath, M. (2015). The unidirectional prosaccade switch-cost: electroencephalographic evidence of task-set inertia in oculomotor control. Behav. Brain Res. 278, 323–329. doi: 10.1016/j.bbr.2014.10.012
Weiler, J., and Heath, M. (2012a). The prior-antisaccade effect influences the planning and online control of prosaccades. Exp. Brain Res. 216, 545–552. doi: 10.1007/s00221-011-2958-7
Weiler, J., and Heath, M. (2012b). Task-switching in oculomotor control: unidirectional switch-cost when alternating between pro-and antisaccades. Neurosci. Lett. 530, 150–154. doi: 10.1016/j.neulet.2012.10.007
Weiler, J., and Heath, M. (2014a). Oculomotor task switching: alternating from a nonstandard to a standard response yields the unidirectional prosaccade switch-cost. J. Neurophysiol. 112, 2176–2184. doi: 10.1152/jn.00352.2014
Weiler, J., and Heath, M. (2014b). Repetitive antisaccade execution does not increase the unidirectional prosaccade switch-cost. Acta Psychol. 146, 67–72. doi: 10.1016/j.actpsy.2013.12.005
Wenban-Smith, M. G., and Findlay, J. M. (1991). Express saccades: is there a separate population in humans? Exp. Brain Res. 87, 218–222. doi: 10.1007/BF00228523
Williams, P. G., Tinajero, R., and Suchy, Y. (2017). Executive functioning and health. Oxford Handbooks Online, 1–53. doi: 10.1093/oxfordhb/9780199935291.013.75
Wunsch, K., Wurst, R., von Dawans, B., Strahler, J., Kasten, N., and Fuchs, R. (2019). Habitual and acute exercise effects on salivary biomarkers in response to psychosocial stress. Psychoneuroendocrinology 106, 216–225. doi: 10.1016/j.psyneuen.2019.03.015
Keywords: antisaccades, cognitive flexibility, executive function, oculomotor, psychosocial stressor
Citation: Morava A, Tari B, Ahn J, Shirzad M, Heath M and Prapavessis H (2023) Acute stress imparts a transient benefit to task-switching that is not modulated following a single bout of exercise. Front. Psychol. 14:1157644. doi: 10.3389/fpsyg.2023.1157644
Edited by:
Chong Chen, Yamaguchi University Graduate School of Medicine, JapanReviewed by:
Yujiro Kawata, Juntendo University, JapanLeor Zmigrod, University of Cambridge, United Kingdom
Copyright © 2023 Morava, Tari, Ahn, Shirzad, Heath and Prapavessis. This is an open-access article distributed under the terms of the Creative Commons Attribution License (CC BY). The use, distribution or reproduction in other forums is permitted, provided the original author(s) and the copyright owner(s) are credited and that the original publication in this journal is cited, in accordance with accepted academic practice. No use, distribution or reproduction is permitted which does not comply with these terms.
*Correspondence: Anisa Morava, YW1vcmF2YUB1d28uY2E=