- 1Department of Neurobiology and Biophysics, Life Sciences Center, Vilnius University, Vilnius, Lithuania
- 2Department of Physiotherapy and Rehabilitation, Xiyuan Hospital, Chinese Academy of Chinese Medical Sciences, Beijing, China
- 3Department of Physical Education, Beijing Language and Culture University, Beijing, China
- 4Department of Traditional Chinese Exercises, College of Physical Education, Minzu University of China, Beijing, China
- 5Department of Acupuncture, College of Acupuncture and Moxibustion, Liaoning University of Traditional Chinese Medicine, Shenyang, China
- 6Faculty of Health, Slovak Medical University, Banská Bystrica, Slovakia
- 7Department of Sports Rehabilitation, School of Sports Medicine and Rehabilitation, Beijing Sport University, Beijing, China
Introduction: Intensive and long-lasting office work is a common cause of muscular and mental disorders due to workplace stressors. Mindful and slow breathing exercises decrease psychological stress and improve mental health, whereas fast breathing increases neuronal excitability. This study aimed to explore the influence of 5 min of mindful breathing (MINDFUL), slow breathing (SLOW), fast breathing (FAST), and listening to music (MUSIC) on muscle tension and executive function during an intensive psychological task.
Methods: Forty-eight participants (24 men and 24 women) were enrolled. Muscle tension was recorded using surface electromyography, and executive function was assessed using the Stroop Color and Word Test (Stroop Test). The respiration rate (RR), oxygen saturation (SpO2), end-tidal carbon dioxide (EtCO2), and the subjects' preferred method were also recorded. During the experiment, participants performed a one-time baseline test (watching a neutral video for 5 min) and then completed 5 min of MUSIC, MINDFUL, SLOW, and FAST in a random sequence. The Stroop Test was performed after each intervention, including the baseline test, and was followed by a 5 min rest before performing the next intervention.
Results: None of the methods significantly influenced muscular activity and performance of the Stroop Test in both men and women, based on the average 5 min values. However, at the fifth minute, men's accuracy rate in the Stroop Test was significantly higher after SLOW than after MUSIC and FAST, and the reaction time after the SLOW was the shortest. SpO2 was significantly higher during SLOW than during MUSIC, and RR was relatively lower after SLOW than after MUSIC. Most men preferred SLOW, and most women preferred MUSIC, whereas FAST was the most unfavorable method for both men and women.
Conclusion: Brief breathing exercises did not substantially affect muscle tension under psychological stress. SLOW demonstrated greater potential for sustaining executive function in men, possibly via its superior respiration efficiency on SpO2 and inhibition of RR.
1. Introduction
Intensive and long-lasting office work is a common cause of muscular and mental disorders due to workplace stressors (Janwantanakul et al., 2008). Many workplace stressors, such as high work and memory demands, mental load, and time pressure are risk factors for shoulder and arm pain (Bongers et al., 2002; Goh et al., 2015). One of the proposed mechanisms is that these stressors increase sustained low-level muscle activity (Bongers et al., 2006; Roman-Liu et al., 2013), which chronically increases muscle tension, impairs body alignment, causes muscular pain, and even leads to headaches (Lundberg et al., 1999; Chowdhury, 2012; Sambataro et al., 2019). In addition to increased muscle tension, chronic stress impairs cognitive function (Marin et al., 2011). Executive functions encompass cognitive processes that work for purposeful and goal-directed behavior (Banich, 2009), and perceived stress has a profound negative impact on executive functions (Kleen et al., 2006; Ohman et al., 2007). Executive function is critical for high work efficiency (Balconi et al., 2020), and high efficacy is always expected to save time. Therefore, an ideal working state should be physically relaxed and mentally effective.
It has been shown that microbreaks at work (i.e., short breaks of < 10–15 min) can benefit physical and psychological wellbeing (Henning et al., 1997; Mclean et al., 2001). Workplace physical activity significantly reduced general musculoskeletal pain, including neck and shoulder pain (Moreira-Silva et al., 2016). Three months of workplace exercise had a moderate effect on executive function compared to the control group (da Silva et al., 2021). This points to the necessity of having work breaks for physical exercise. Baduanjin exercise is one of the most common styles of traditional exercise in China. It is also a popular exercise during work breaks (Zheng et al., 2013) since it takes a short time and requires only a small space. Studies found that Baduanjin improved cognitive function and muscular performance (Zou et al., 2017; Wang et al., 2021; Yang et al., 2023). Breathing techniques are a main component of the Baduanjin exercise. In physiological studies, even a single breathing practice significantly reduced blood pressure, increased heart rate variability, oxygenated blood, and enhanced pulmonary function (Ma et al., 2017). Therefore, in order to optimize the efficacy of workplace exercises, breathing methods alone should be investigated. Slow breathing (SLOW, with a speed of 6 reps/min) and mindful breathing (MINDFUL, merely being aware of breath) are the two main breathing methods used in Baduanjin. These two breathing methods are also commonly used in yoga and psychological treatments.
Mindful breathing and SLOW can decrease psychological stress and improve mental health, while fast breathing (FAST) can increase neuronal excitability, as presented below. MINDFUL reduced emotional volatility in response to negative stimuli, reduced test anxiety (Cho et al., 2016), and even 5 min of breathing could reduce distress (Beng et al., 2016). MINDFUL increased alpha power and enhanced error-related alpha suppression during the subsequent Stroop task, indicating enhanced error monitoring (Bing-Canar et al., 2016). A study using intracranial electroencephalogram demonstrated respiration-locked oscillations during attentive breathing (mindful breathing) with stronger power in the anterior cingulate cortex, premotor cortex, insula, and hippocampus (Herrero et al., 2018), which are regions supposed to be involved in executive function (Carter et al., 1999; Rizzolatti et al., 2002; Eichenbaum, 2004). SLOW (device-guided, 5–6 breaths/min) is currently a Federal Drug Administration-approved treatment indicated by the American Heart Association for relaxation (Larson et al., 2020). Generally, for a healthy person, increased heart rate variability (HRV) indicates a relaxed state. HRV was found at the highest level when the breathing rate was 6 reps/min compared to that with spontaneous breathing and other breathing rates (Bernardi et al., 2001; Radaelli et al., 2004). Although high-frequency power in HRV (reflect vagal tone) is influenced by respiration rate (Laborde et al., 2017), many studies have found that SLOW increased ease, comfort, relaxation, and positive energy and reduced anxiety, dejection, anger, hostility, and confusion (Zaccaro et al., 2018). The executive function was improved after slow-paced breathing (4.5-s inhalation and 5.5-s exhalation) compared to after natural breathing, with higher scores observed for Stroop interference accuracy (Laborde et al., 2022). Meanwhile, the authors found that the improved executive function after slow breathing was not mediated by RMSSD in HRV using bootstrapped mediation analyses (Laborde et al., 2022). Thus, other mediating factors, such as oxygenation and ventilation volume, should be tested. In addition to MINDFUL and SLOW, FAST can increase neuronal excitability that facilitates muscle contraction and may be beneficial for cognitive functions. FAST increased ventilation and raised pH (Barrett et al., 2019). Higher pH increased Ca2+ and Na+ currents, lowered the threshold of the action potential, and shortened the refractory periods of action potentials, which facilitated muscle contraction (Tombaugh and Somjen, 1996; Lu et al., 2012). Enhanced cognitive performance was associated with increased cortical excitability, which could be related to higher glutamatergic and lower GABAergic activation (Salehinejad et al., 2021). One study compared the effect of 12 weeks of practice of slow and rapid types of pranayama breathing and found that both types of breathing were beneficial for cognitive functions, with fast pranayama having additional effects on executive function (Sharma et al., 2014).
In addition to breathing exercises, listening to music (MUSIC) is also a common way to relax during work breaks. In a survey conducted by a large North American recruitment firm, 79% of the respondents felt that MUSIC improved their work satisfaction and productivity (Haake, 2011).
To the best of our knowledge, the acute effects of different breathing techniques on muscle tension have not been investigated, and few studies have elucidated the efficacy of different breathing exercises and MUSIC on executive function. Therefore, this study aimed to explore the influence of a single 5-min session of MINDFUL, SLOW, FAST, and MUSIC on muscle tension and executive function to determine which method is better for body relaxation and mental work under psychological stress. Furthermore, the study aimed to examine physiological responses such as respiration rate, oxygen saturation level, and end-tidal carbon dioxide partial pressure.
2. Materials and methods
2.1. Participants
Forty-eight participants (men: n = 24, age = 30 ± 6, BMI = 23.2 ± 1.7; women: n = 24, age = 29 ± 6, BMI = 21 ± 1.3) were enrolled according to the estimations of sample size using G Power 3.1 (Heinrich Heine University Düsseldorf, Düsseldorf, Germany). Referring to prior studies (Springer et al., 2018; Howard et al., 2020; Redlich Bossy et al., 2020) and assuming an effect size of 0.25, alpha of 0.05, power of 0.8, and correlation coefficient of 0.6, 20 participants were required for each sex. Allowing for a 20% dropout rate, a total of 48 participants were required.
Inclusion criteria were as follows: (1) age 20–39 years; (2) BMI 18.9–24.9; (3) students or office workers; (4) ability to perform MINDFUL and SLOW (with obvious abdominal movement); (5) willingness to test at 3–10 days after the end of menstruation for women; (6) perceived stress scale ≤ 42 (Cohen et al., 1983); and (7) written informed consent. Exclusion criteria were as follows: (1) mental or cardiorespiratory diseases; (2) skeletal muscle disorders in the lower back, shoulder, or arm with a score of pain (visual analog scale) higher than 3; (3) color vision disorder; and (4) unwillingness to complete the experiment. In addition, participants were asked not to consume caffeinated drinks for 2 h before the experiment.
The study was approved by the Medical Ethics Committee of Xiyuan Hospital, China Academy of Chinese Medical Sciences (approval number: 2022XLA013-2).
2.2. Interventions
Mindful breathing, SLOW, FAST, and MUSIC were the four interventions. For MINDFUL, participants were required to be aware of the breath, without controlling the speed and depth, and without judgment of the quality (Burg and Michalak, 2011). The breath counting method was allowed for participants who felt it challenging to focus on their breath. SLOW was set at a speed of six breaths per minute, and obvious abdominal movement was required. An audio metronome was designed with a rising tone for inhalation and a falling tone for exhalation, setting the rate at 4 s for inhalation and 6 s for exhalation. Participants were instructed to gently expand their abdomen in addition to the natural expansion of the ribcage during inhaling, and relax their abdomen with attention on the area around the navel during exhaling. They were allowed to hold their breath naturally after exhalation to mimic natural breathing. The assessment of SLOW with obvious abdominal movement was conducted using two respiration belts. To determine the FAST rate, a respiration belt was used to measure RR during spontaneous breathing. FAST was then set at a speed that was 30% higher than an individual's regular breathing rate. During FAST, participants were also guided by an audio metronome. Regarding MUSIC, participants chose the relaxing music they preferred. Classical music (piano, Kiss the Rain; guzheng, Yun Shui Chan Xin) was provided, although participants could also listen to their own relaxing music.
2.3. Experimental protocol
The same participants were tested in all conditions to limit inter-individual differences and minimize random noise (Laborde et al., 2017). The duration of the interventions, the Stroop test, and the rest between interventions were set at 5 min according to the previous studies (Endo et al., 2013; Beng et al., 2016; Kluger and Gross, 2020). To ensure that the participants could perform breathing exercises properly, they received one-time online training 1 month before the testing day and were requested to practice for 1 month. All participants were invited to a social platform group where they could ask questions and exchange training videos, and instructors checked and guided participants' movement of SLOW during the 1-month training period through video recordings and/or online meetings. Prior to the experiment, participants underwent two training sessions on the Stroop test to reduce the learning effect. Then, participants sat on an adjustable chair in a standardized posture, with ~90 degrees flexion of the knees and hips, and a straight upper body (Figure 1); the computer screen was set at 50–80 cm in front of the face. The angle between the arm and the trunk was about 20 degrees, and the angle between the forearm and the arm was about 110 degrees, the junction of the front one-third of the forearm and the back two-thirds (the tester made marks on participants' arms) was above the edge of the table. Two markers were put on the table beside the participants' forearms to eliminate the movement. During the experiment, participants performed a one-time baseline test (watching a neutral video for 5 min) and then completed 5 min of MUSIC, MINDFUL, SLOW, and FAST in a random sequence. The Stroop test was performed after each intervention, including the baseline test, and was followed by a 5 min rest before performing the next intervention. While watching the video, listening to music, and performing breathing methods, participants overlapped their hands and rested the ulnar side on the table with palms toward the body and eyes closed. Before the Stroop test, participants opened their eyes and waited for 10 s to “wake up.” At the resting time, participants were allowed to stand up, walk, and drink water. Sequence randomization used the Latin Square Williams design, as shown in Figure 2.
2.4. Data collection
2.4.1. Muscle tension
Muscle tension/activity was measured using sEMG (Delsys Trigno wireless system, Natick, MA, USA). The skin was gently abraded and cleaned with an alcohol wipe, and the hair was removed when necessary. Two rectangular Delsys Trigno EMG sensors (27 mm × 37 mm × 15 mm) with 99% silver electrode contacts adhered to the upper trapezius (halfway along the line from the acromion to the spine on vertebra C7 on both left and right sides). We chose the upper trapezius muscle because its activity is more evident than that of most other body sites during mental tasks (Schleifer et al., 2002). The wireless electrodes were suitable for our experiments since participants reported that the electrodes did not cause distraction. The sampling frequency was 2148 Hz, with an amplification gain of 1000. Raw sEMG data were band-pass filtered between 20 and 450 Hz using a fourth-order Butterworth filter, and band-stop filtered at 50 Hz with aliases with sidebands of 0.25 Hz. The RMS was used to represent muscle tension.
To verify and consolidate the results, we used two methods to normalize the RMS values. The first used the mean value (average of four interventions for each participant) as a reference. Halaki and Ginn (2012) summarized that using the mean activation level of EMG could decrease the variability between individuals. The second used the baseline (Figure 2) as the reference. Roman-Liu et al. (2013) conducted a study that tested muscle tension (using sEMG) under mental load with repeated measures and claimed that taking the baseline as the reference value was the most appropriate reference. Normalization was performed by dividing muscle tension during the baseline condition by muscle tension during different breathing methods or the Stroop test and multiplied by 100.
2.4.2. Executive function
Executive function was determined from the accuracy and reaction time of the Stroop test and tested on a computer using E-prime 3.0 (Psychology Software Tools, Pittsburgh, PA, USA). The test consisted of four words (“red,” “blue,” “green,” and “yellow”), which were randomly displayed with a color that was the same as or different from the word's meaning. A series of color words were presented consecutively during the Stroop test. The participants were instructed to name the color of the displayed word as accurately and quickly as possible. A new color word was shown for 21 ms after one response or for 2000 ms without a response (no response within 2000 ms was recorded as an incorrect answer). There were 24 samples in each cycle, and the system randomly generated the sequence for every cycle. The accuracy was calculated by dividing the number of correct answers by the total number of color words and then multiplying the result by 100.
2.4.3. Respiration rate
A respiration belt (Vernier, Beaverton, OR, USA) was secured around the chest, level with the xiphoid, to record the respiration rate. This belt has been used in previous studies to provide ground truth data (Jakkaew and Onoye, 2020). The signals were presented as a force with a sampling frequency of 10 Hz. There was a channel detecting inhalations and calculating the number of breaths per minute. The sample window for the calculation was 30 s, the advance interval was 10 s, and the value updates every 10 s.
2.4.4. Oxygen saturation and end-tidal carbon dioxide partial pressure
A health monitor (Contec-CMS8000, Qinhuangdao, China) was used to record SpO2 and EtCO2. The SpO2 measuring range was 0–100%, resolution 1%, and actualization interval 1 s. The flexible pad was worn on the right thumb since the participants needed the index and middle fingers to press the keys on the keyboard. The expired air was continuously collected using a disposable nasal cannula for measuring EtCO2. The sampling gas flow rate was 50 ml/min ± 10 ml/min, resolution 0.1 mmHg (0–69 mmHg), actualization interval 1 s, and the delay time 2–3 s. Atmospheric pressure was set at a default value of 760 mmHg. Routine calibration was not required, but 1–2 min of warm-up was implemented prior to each participant. Participants could opt out of the EtCO2 test if the cannula was uncomfortable.
2.4.5. Preferred intervention
At the end of the test, participants selected their preferred intervention according to their general subjective feelings of relaxation and working efficiency.
2.5. Outcomes
The primary outcomes were as follows: muscle tension, represented by root mean square (RMS) values from surface electromyography (sEMG) recording; and executive function, represented by accuracy and reaction time from the Stroop test. The secondary outcomes were RR from the respiration belt, SpO2 and EtCO2 from a health monitor, and the participants' preferred method.
2.6. Statistical analysis
The Shapiro–Wilk test was performed to assess data distribution. The percentage of missing data was calculated, and Little's MCAR test was used to determine the missingness model. The effect of interventions from the average 5 min values and every minute's values was tested using generalized estimating equations (GEEs) on SPSS (IBM Corp., Armonk, NY, USA) since normal and skewed distributions were mixed throughout the data, and some could not be converted to a normal distribution using log transform. The exchangeable correlation structure was chosen as the present study had a balanced design, and the value of goodness-of-fit was the highest. Significance was set at an alpha level of P < 0.05 and a highly significant level of P < 0.01, with the Holm correction, applied for the post-hoc test. The Wilcoxon paired test was used to evaluate the difference between the parameters when performing interventions and those while taking the Stroop test, and the significance was set at P < 0.05. Effect sizes were calculated using Hedges' g (g) method. Participants' preference (percentage of favorable intervention) was analyzed using the chi-squared test on MedCalc (MedCalc Inc., Mariakerke, Belgium), and the significance was set at P < 0.05 and P < 0.01 with Holm correction.
3. Results
According to the inclusion criteria, 48 participants were enrolled in this study. Three male participants were excluded: one fell asleep during SLOW and MINDFUL, one was in bad condition (the error rate was over 40% in the Stroop test), and one did not perform MINDFUL and SLOW as required. Two women were excluded: one reported a bad condition during the testing and another did not perform MINDFUL and SLOW as required. Therefore, 21 men and 22 women were included in the analysis. All missing data were missed completely at random (Little's MCAR test, P > 0.05).
According to the Latin Square Williams design (Figure 2), the participant performed each method according to the horizontal sequence as the first participant performed ABCD, the second performed BCAD, the third performed CDBA, the fourth DACB, and the fifth restarted from ABCD. In order to test for the effect of fatigue and/or learning, we compared each vertical sequence (ABCD, BCDA, DABC, and CDAB) using the averaged 5 min values. Every post-hoc comparison's result was not significant as the lowest p-value was 0.127 from men's data and 0.074 from women's data. Therefore, we concluded that fatigue and/or learning effects were non-significant.
3.1. Muscle tension
Among the included participants, the sEMG signals of one man and two women were not recorded successfully. Thus, 20 men and 20 women were included in the analysis. Men's data were skewed after SLOW and FAST, while women's were normally distributed. Men had no missing data from the right upper trapezius and 2.5% from the left side; women had 2.5% missing data from the right side and no missing data from the left side. Outliers were defined as outside of median – (3 × median absolute deviation) and median + (3 × median absolute deviation) (Trevino et al., 2015). Outliers were not removed because they were genuine observations. To verify the results, we analyzed the data after removing the outliers and found no significant differences from the results that retained outliers.
As depicted in Table 1, for both men and women, different interventions did not significantly affect muscle tension during the interventions and the Stroop test. However, there was a clear trend for men to have the lowest muscle tension during MINDFUL, and the tension decreased minute-by-minute. The muscle tension was relatively high after SLOW. The muscle tension in women was lower after MUSIC and FAST than that after MINDFUL and SLOW, and it was the lowest after FAST. In addition, the results were inconsistent between the left and right sides in both men and women.
The two normalization methods yielded similar results, as both had non-significant p-values and similar trends.
3.2. Executive function
Data from 21 men and 22 women were analyzed for executive function, with no missing data. The accuracy data for men and women were skewed, while reaction times were normally distributed.
Based on the average 5 min values, different interventions did not significantly affect mental activity in terms of accuracy and reaction time in both men and women, as shown in Table 2 and Figure 3. However, men's accuracy rate in the Stroop test was highest after SLOW among all methods, and it was significantly higher after SLOW than after MUSIC and FAST at the 5th min (SLOW vs. MUSIC, Wald χ2 = 9.44, P = 0.011, g = 0.622; SLOW vs. FAST, Wald χ2 = 16.9, P < 0.001, g = 0.863). This indicates that the SLOW intervention has a better potential to sustain the ability of inhibition control in men. Additionally, men's reaction time after the SLOW was stable with a slight decrease through 5 min task, and became the shortest at the 5th min, which indicates participants' working speed after SLOW was sustained well relative to after other three methods.
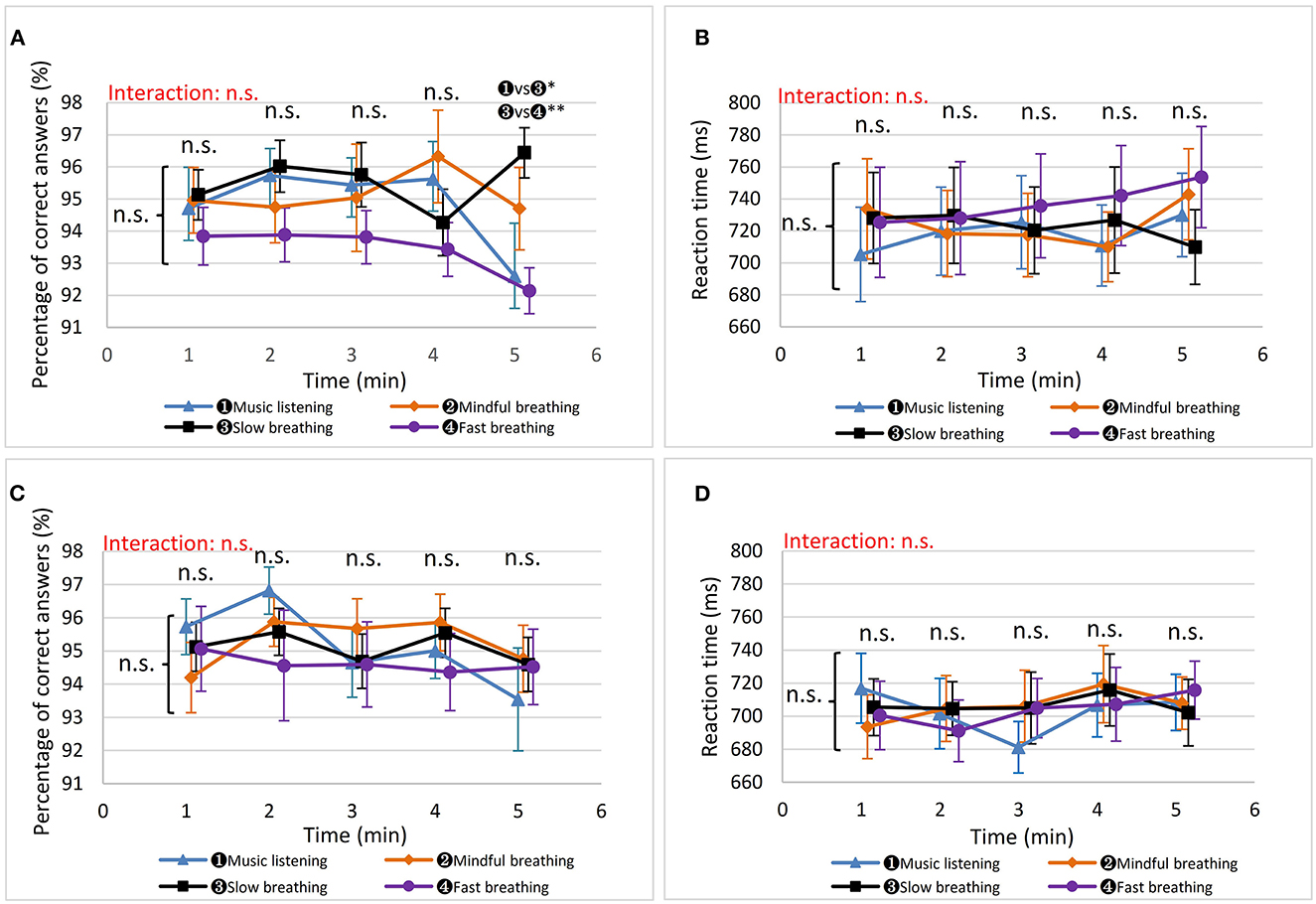
Figure 3. The results of executive function. “n. s.” beside the curly brackets denotes that no significant difference was found between each intervention based on the average 5 min values. “n. s.” or “ vs
” above each minute presents the results of the comparisons based on the values in that minute. The results of interactive effects were colored in red. *P < 0.05, **P < 0.01. (A) Men's accuracy in the Stroop test. (B) Men's reaction time in the Stroop test. (C) Women's accuracy in the Stroop test. (D) Women's reaction time in the Stroop test.
MINDFUL and MUSIC show comparable results in executive function in both women and men. Regarding FAST, clear trends were showing a gradual decrease in accuracy rate and a gradual increase in reaction time in both women and men, with sharper changes in men. This suggests that FAST did not boost executive function but had an adverse impact on it.
In Table 2, we used the mean and standard deviation instead of the median and interquartile ranges because the accuracy was skewed, not due to outliers, but because participants had higher accuracy. Only the mean and SD could clearly and consistently show the analytic results from GEE and the non-parametric Wilcoxon paired-rank test (we conducted the Wilcoxon paired-rank test to confirm the results from GEE).
3.3. Respiration rate
One woman's respiration rate was not recorded successfully; therefore, data from 21 men and 21 women were analyzed. All data were normally distributed. Men did not have missing data; women had 1.1%.
As depicted in Figure 4A, men's RR was significantly different during different interventions (Wald χ2 = 559.3, P < 0.001). It was not surprising that the RR of FAST was faster than that of the other methods and SLOW was the slowest. Interestingly, the RR during MINDFUL was significantly lower than that during MUSIC (Wald χ2 = 7.168, P = 0.007, g = 0.499). During the Stroop test, based on the average 5 min values, RR after SLOW was significantly lower than after MINDFUL (Wald χ2 = 6.982, P = 0.049, g = 0.382). There was a significant interaction effect on RR during the Stroop test after SLOW and after MUSIC (Wald χ2 = 15.9, P = 0.019). RR at the 1st min after SLOW was significantly lower than after MUSIC (P = 0.010, g = 0.544) and MINDFUL (P = 0.001, g = 0.609), and the 2nd min after SLOW was also lower than MINDFUL at the 2nd min (P = 0.043, g = 0.542). There were no significant differences at and after the 3rd min. According to Figure 4A and the results obtained, it is evident that 5 min of SLOW reduced RR during stressful conditions for a longer duration than MUSIC and MINDFUL, although not beyond 5 min. From performing intervention to taking the Stroop test, the average 5 min values for RR increased significantly in MUSIC (13.5 ± 3.62 vs. 18.8 ± 3.03, t = −6.68, P < 0.001, g = 0.542), in MINDFUL (11.6 ± 3.98 vs. 18.7 ± 2.60, t = −6.80, P < 0.001, g = 2.105), and in SLOW (6.02 ± 0.09 vs. 17.5 ± 3.57, t = −14.7, P < 0.001, g = 4.516). However, RR after FAST did not change significantly (17.5 ± 4.00 vs. 18.2 ± 2.96, t = −0.70, P = 0.490, g = 0.198).
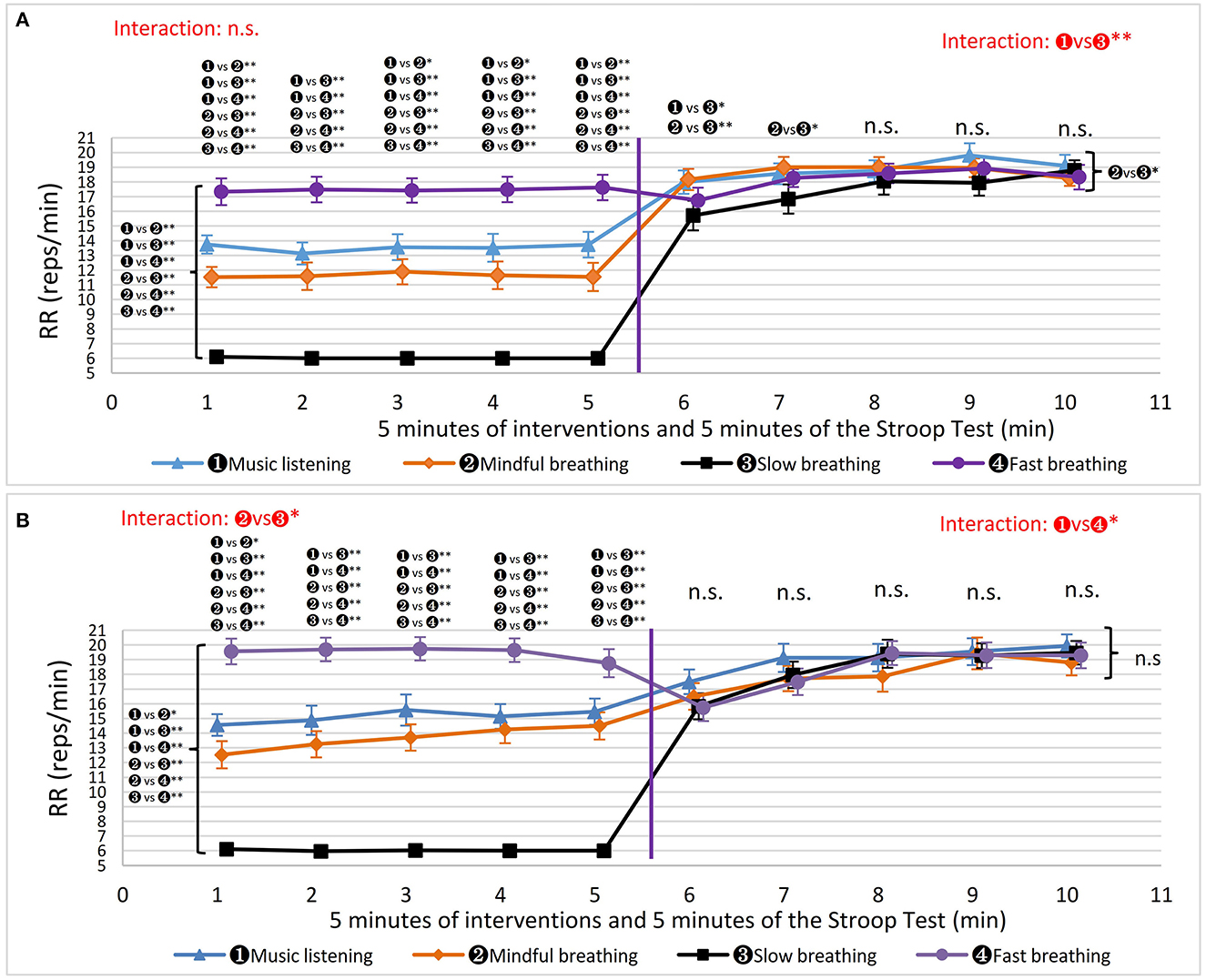
Figure 4. The results of respiration rate (RR). The markers beside the curly brackets, such as “n. s.” or “ vs
*” etc., denote the results of comparison based on the average 5 min values. The markers above each minute present the results of the comparisons based on the values in that minute. The results of interactive effects were colored in red. *P < 0.05, **P < 0.01. (A) Men's RR. (B) Women's RR.
The changes in women's RR are shown in Figure 4B. During the intervention periods, the RR was significantly different between interventions using the average 5 min values (Wald χ2 = 841.6, P < 0.001). Similar to men's results, RR during MINDFUL was also significantly lower than during MUSIC (Wald χ2 = 6.509, P = 0.011) in women. Furthermore, there are significant interactions between MINDFUL and SLOW (Wald χ2 = 16.8, P = 0.013) due to the increase of RR during MINDFUL. During the Stroop test, the results were different from those of men, as RR after various interventions were not different in average 5 min values, nor at each minute, except for the interactive effect after MUSIC and after FAST (Wald χ2 = 16.8, P = 020). From performing intervention to taking the Stroop test, RR increased significantly in MUSIC (14.9 ± 3.8 vs. 19.0 ± 3.9, z = −3.32, P < 0.001, g = 1.065), in MINDFUL (13.6 ± 4.1 vs. 18.1 ± 3.8, z = −3.56, P = 0.001, g =1.138), and in SLOW (6.00 ± 0.1 vs. 18.4 ± 3.5, z = −4.02, P = 0.001, g = 5.008). Similar to men, RR during FAST did not change significantly (19.6 ± 3.9 vs. 18.3 ± 3.5, z = −1.91, P = 0.0559, g = 0.351).
3.4. Oxygen saturation
SpO2 was not recorded successfully for one man and one woman. Therefore, 20 men and 21 women were included in this analysis. The data from both men and women were normally distributed. Men had 5.8% missing values, whereas women had 3.7%.
For men, during the interventions, the SpO2 was significantly different between MUSIC and SLOW (96.6 ± 0.9 vs. 97.4 ± 1.3, Wald χ2 = 9.53, P = 0.012, g = 0.716), MUSIC and FAST (96.6 ± 0.9 vs. 97.4 ± 1.0, Wald χ2 = 8.79, P = 0.015, g = 0.841), and MINDFUL and FAST (97.0 ± 1.1 vs. 97.4 ± 1.0, Wald χ2 = 7.64, P = 0.023, g = 0.381) (Figure 5A). In addition, there was an interactive effect between MUSIC and SLOW (Wald χ2 = 21.2, P = 0.002). In contrast, there was no significant difference between MUSIC and MINDFUL (96.6 ± 0.9 vs. 97.0 ± 1.1, Wald χ2 = 0.31, P = 0.502, g = 0.398). During the Stroop test, different interventions did not influence SpO2 significantly for the average 5 min values and at each minute. SpO2 decreased significantly from performing FAST to taking the Stroop test (97.4 ± 1.03 vs. 96.7 ± 0.99, t = 2.72, P = 0.014, g = 0.693). The effect of the interventions lasted for 1 min during the Stroop test, as SpO2 dropped significantly from the 2nd minute after MUSIC (P < 0.001, MINDFUL (P < 0.001), SLOW (P = 0.016), and FAST (P < 0.001).
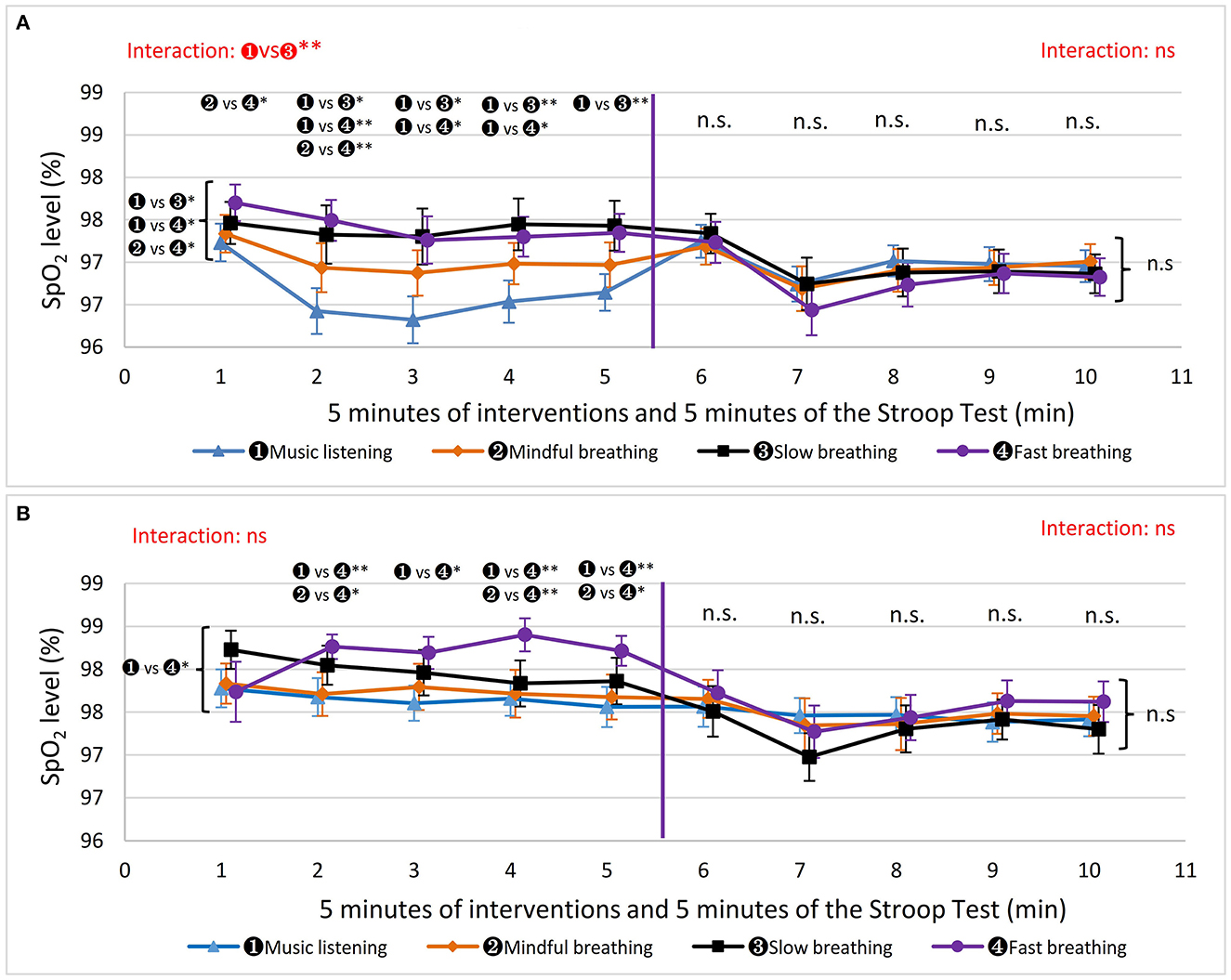
Figure 5. The results of oxygen saturation (SpO2). The markers beside the curly brackets, such as “n. s.” or “ vs
*” etc., denote the results of comparison based on the average 5 min values. The markers above each minute present the results of the comparisons based on the values in that minute. The results of interactive effects were colored in red. *P < 0.05, **P < 0.01. (A) Men's SpO2. (B) Women's SpO2.
For women, during the different interventions, only MUSIC and FAST influenced SpO2 differently (97.7 ± 0.95 vs. 98.2 ± 0.73, Wald χ2 = 9.46, P = 0.012, g = 0.590) (Figure 5B). During the Stroop test, different interventions did not influence SpO2 significantly. From performing intervention to taking the Stroop test, SpO2 decreased significantly after SLOW (97.9 ± 1.07 vs. 97.3 ± 1.17, t = 3.17, P = 0.005, g = 0.535) and FAST (98.2 ± 0.73 vs. 97.5 ± 1.15, t = 3.00, P = 0.007, g = 0.727). During the Stroop test, SpO2 decreased significantly from the 1st min to the 2nd min only after SLOW (P = 0.008).
3.5. End-tidal carbon dioxide partial pressure
Data were not recorded successfully for one man and two women. Therefore, data from 20 men and 20 women were analyzed. All data had a normal distribution except during the Stroop test after FAST in women. There were 6% missing data from men and 2.5% from women.
The results for men are shown in Figure 6A. During the different interventions, EtCO2 was significantly different, using the average 5 min values, between FAST and MUSIC (35.3 ± 5.29 vs. 39.9 ± 4.82, Wald χ2 =18.19, P < 0.001, g = 0.929), FAST and MINDFUL (35.3 ± 5.29 vs. 40.0 ± 5.80, Wald χ2 =19.22, P < 0.001, g = 0.865), and FAST and SLOW (35.3 ± 5.29 vs. 38.5 ± 6.36, Wald χ2 = 7.02, P = 0.032, g = 0.547). In addition, there were significant interactive effects between SLOW and MUSIC (Wald χ2 = 48.8, P < 0.001), between SLOW and MINDFUL (Wald χ2 = 26.4, P < 0.001), and between SLOW and FAST (Wald χ2 = 26.2, P < 0.001). Notably, EtCO2 after SLOW started to reduce from the 2nd min; it was significantly lower than that of MUSIC and MINDFUL at the 4th min (37.0 ± 6.70 vs. 40.6 ± 5.12, P = 0.004, g = 0.547; 37.0 ± 6.70 vs. 39.8 ± 7.01, P = 0.036, g = 0.408), and at the 5th min (37.0 ± 6.70 vs. 40.2 ± 6.07, P = 0.005, g = 0.472; 37.0 ± 6.70 vs. 40.2 ± 6.88, P = 0.003, g = 0.447). During the Stroop test, different interventions did not influence EtCO2 significantly based on the average 5 min values. Only at the 1st min, EtCO2 after FAST was significantly lower than that after MUSIC (39.0 ± 3.76 vs. 36.2 ± 4.88, P = 0.046, g = 0.642). From performing intervention to taking the Stroop test, EtCO2 increased significantly only in FAST (35.3 ± 5.29 vs. 37.9 ± 4.68, t = −3.30, P = 0.023, g = 0.521).
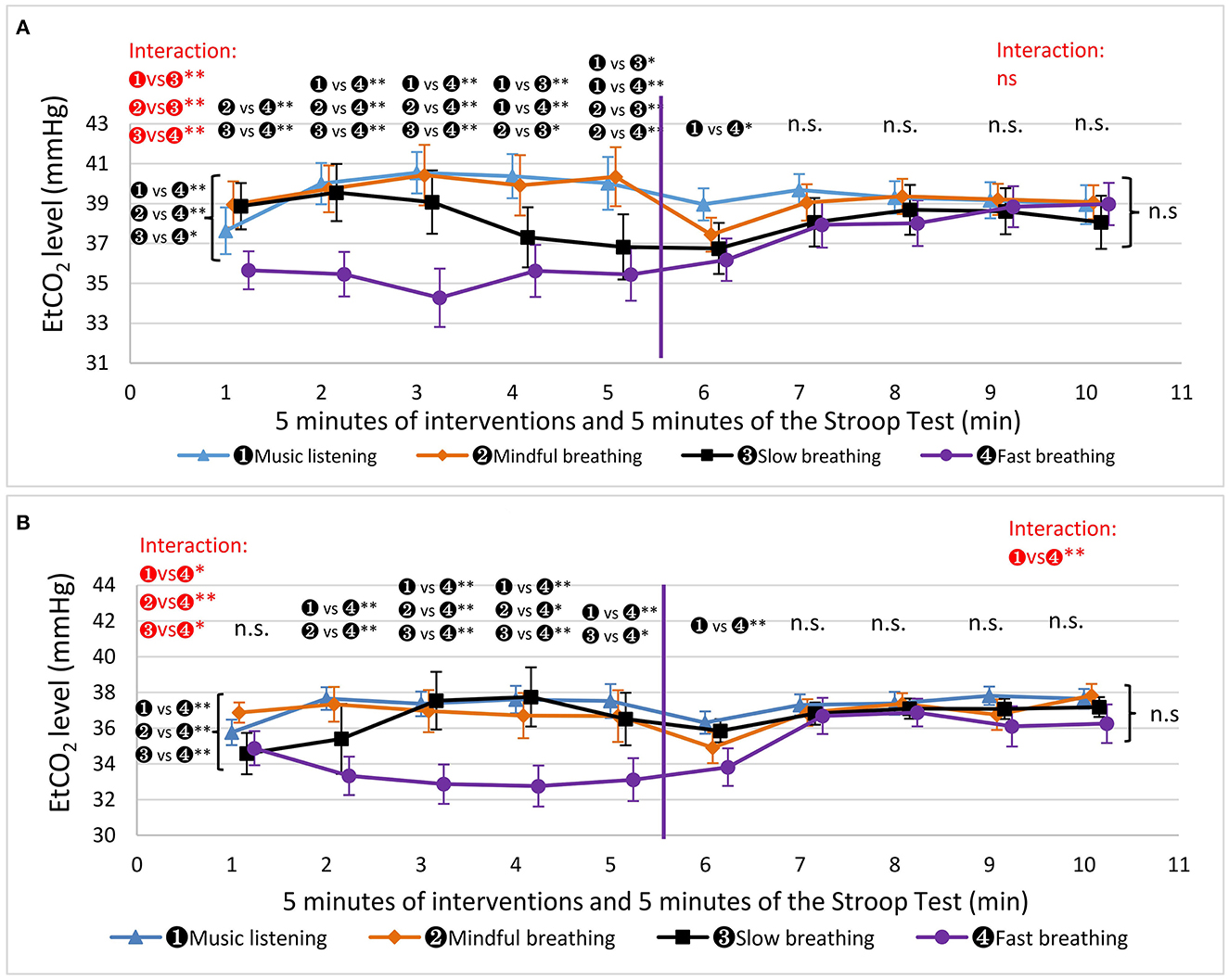
Figure 6. The results of end-tidal carbon dioxide partial pressure (EtCO2). The markers beside the curly brackets, such as “n. s.” or “ vs
*” etc., denote the results of comparison based on the average 5 min values. The markers above each minute present the results of the comparisons based on the values in that minute. The results of interactive effects were colored in red. *P < 0.05, **P < 0.01. (A) Men's EtCo2. (B) Women's EtCo2.
The results for women are shown in Figure 6B. EtCO2 during FAST (33.2 ± 4.18) was significantly lower than that during the other three interventions (MUSIC: 37.1 ± 2.87, Wald χ2 = 18.66, P < 0.001, g = 1.088; MINDFUL: 36.7 ± 4.43, Wald χ2 = 10.74, P = 0.004, g = 0.813; SLOW: 35.9 ± 5.70, Wald χ2 = 12.32, P = 0.002, g = 0.540), and there were no significant differences between MUSIC, MINDFUL and SLOW. Furthermore, there were significant interactive effects between FAST and MUSIC (Wald χ2 = 17.0, P = 0.011), between FAST and MINDFUL (Wald χ2 = 25.2, P < 0.001), and between FAST and SLOW (Wald χ2 = 16.4, P = 0.013). During the Stroop test, different interventions did not influence EtCO2 significantly for the average 5 min values. However, there was an interactive effect after MUSIC and after FAST (Wald χ2 = 30.0, P < 0.001), and EtCO2 after FAST was significantly lower than that after MUSIC (33.8 ± 5.25 vs. 36.3 ± 2.95, P = 0.002, g = 0.587) only in the 1st min. From performing the intervention to the Stroop test, comparing the average 5 min values, EtCO2 increased significantly only for FAST (33.2 ± 4.18 vs. 35.8 ± 5.00, t = −3.49, P = 0.003, g = 0.564).
3.6. Preferred intervention
As shown in Figure 7A, 52% (n = 11) of the male participants preferred SLOW, 24% (n = 5) MUSIC, 19% (n = 4) MINDFUL, and 5% (n = 1) FAST. Statistically, the percentage of participants who preferred SLOW was significantly higher than that of participants who preferred FAST (χ2 = 11.37, P = 0.004). For the female participants, 48% (n = 10) preferred MUSIC, 24% (n = 5) MINDFUL, 14% (n = 3) SLOW, and 10% (n = 2) FAST (Figure 7B). One woman could not firmly choose a favorite, so she did not respond. The percentage of female participants who preferred MUSIC was significantly higher than those who preferred FAST (χ2 = 7.19, P = 0.004).
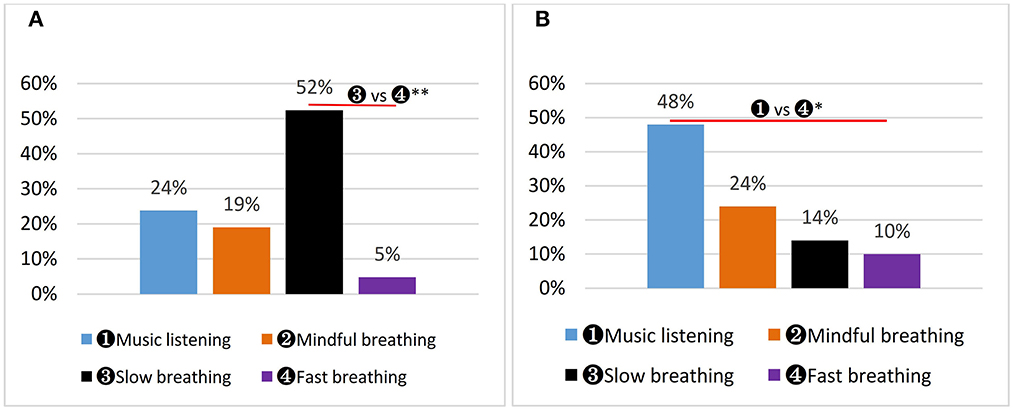
Figure 7. Participants' preferred intervention. *P < 0.05, **P < 0.01. (A) Men's preferred intervention. (B) Women's preferred intervention.
4. Discussion
The purpose of the current study was to test which method (within breathing techniques and music listening) is better for body relaxation and work efficacy such that it could be used alone or in combination with physical activity during work breaks. To the best of our knowledge, this is unprecedented research to investigate the effect of breathing techniques on muscle tension, even though we found different breathing methods did not influence muscle tension significantly. However, MINDFUL tended to be better at muscle tension reduction under stressful conditions for men, and SLOW showed superior potential over other methods on executive function. In addition, the results of RR, SpO2, and EtCO2 provided many interesting and meaningful physiological findings.
4.1. Muscle tension
One explanation for increased muscle tension under stress is that the elevated sympathetic activity initiates the release of catecholamines, which further increases muscle tension (Melin and Lundberg, 1997). Another study suggested that stress-induced hyperventilation causes excessive exhalation of CO2, which increases pH and facilitates muscle contraction (Schleifer et al., 2002). Mental stress in computer work increased muscle tension and brief MINDFUL or SLOW was able to reduce stress (Lundberg et al., 1999; Karthikeyan et al., 2012; Beng et al., 2016). Therefore, we investigated the influence of different breathing methods and MUSIC on muscle tension.
Unexpectedly, but meaningfully, we found that 5 min of MINDFUL, SLOW, FAST, and MUSIC did not influence upper trapezius activity when taking the stressful psychological task, the Stroop test. To verify the results, successful stress induction was confirmed, as RR, a stress indicator, increased when performing the Stroop test. Second, two sEMG normalization methods (normalized from the mean and baseline) were performed, and there were no significant differences between each intervention.
According to the mean values, men's muscle tension was the lowest after MINDFUL from both the right and left sides of the upper trapezius, and the muscle tension was higher after SLOW than after MINDFUL and MUSIC. This implies that it might be difficult to relax physically while being energized mentally. The muscle tension in women was lower after MUSIC and FAST than that after SLOW and MINDFUL, while the lowest was after FAST. Oddly, FAST was the least favorable method. In addition, the muscle tension was inconsistent between the left and right sides. For example, men's muscle tension was the highest after FAST from the right side and the highest after SLOW from the left side. These odd and inconsistent results might be due to the influence of posture. We noticed that some participants' muscle tension changed substantially when their posture was altered. We strictly specified where the participants' arms were to rest. However, there were movements of the upper body during the Stroop test. Cohen et al. (1983) also stressed “EMG is influenced by many other factors, apart from stress. Static factors like body morphology and dynamic factors like posture influence the recorded EMG signals possibly even more than stress.”
4.2. Executive function
Slow breathing has been shown to have better accuracy, but not reaction time, on the Stroop test compared to that of watching a neutral TV program (Laborde et al., 2022). In the present study, SLOW showed greater potential for sustaining executive function in men, as the accuracy rate during the average 5 min values was highest after slow breathing, with the accuracy rate in the 5th min being significantly higher after slow breathing compared to music listening and fast breathing, and the reaction time being shortest after slow breathing. A resonance model proposed 6 reps/min for SLOW as being the most beneficial. In this model, SLOW was related to heart rate, blood pressure, baroreflex modulation, and resonance characteristics of the cardiovascular system (Lehrer and Gevirtz, 2014). Laborde et al. (2019) summarized that these processes could strengthen homeostasis, improve gas exchange, and increase vagal afferents. Our findings support this model as SpO2 during SLOW was higher than during MUSIC.
Respiration interacts with the autonomic nervous system, as inhalation is associated with sympathetic activity and exhalation with parasympathetic activity (Jerath et al., 2006). SLOW increases parasympathetic outflow (Kromenacker et al., 2018), while decreased sympathetic activity and increased parasympathetic activity is likely associated with better cognitive performance (Forte et al., 2019). In the present study, SLOW was set as 4 s of inhalation and 6-s exhalations, which was meant to increase parasympathetic activity, and the improved cognitive function might be caused by the activation of the parasympathetic outflow. As mentioned in the introduction, Laborde et al. (2022) observed an increased RMSSD in HRV (represents cardiac vagal activity/pare-sympathetic activity) during slow breathing (4.5-s inhalation and 5.5-s exhalation) and improved executive function after slow breathing. However, they found that improved executive function (compared to watching TV) was not mediated by RMSSD using bootstrapped mediation analyses (Laborde et al., 2022). Nevertheless, while slow breathing reduces stress (Zaccaro et al., 2018), the Stroop test was stressful, and even mild acute uncontrollable stress can cause a rapid and dramatic loss of prefrontal cognitive abilities (Arnsten, xbib2009). SLOW may improve executive function by alleviating the effects of stress and maintaining prefrontal neuronal function during stressful psychological tasks. The human body is a complex system. Oxygenation and respiration rate might also be the medicative factors, which will be discussed in the next part, “cardiorespiratory Activity.”
Regarding the results in women, the measured interventions did not significantly affect their executive function. Women have shorter diaphragms, higher positions of the sternum, a greater inclination of the ribs, and more chest movement than men during regular breathing (García-Martínez et al., 2016; LoMauro and Aliverti, 2018). Additionally, cultural influences may lead women to contract their abdominal muscles in order to appear more slender. Therefore, we suggest that women might need a longer time than men to practice SLOW (with obvious abdominal movement) to gain the same effect as men.
The effect of 5 min of MINDFUL on the performance of the executive test was not significantly different from MUSIC, even though the RR during MINDFUL was significantly lower than during MUSIC, and the SpO2 was relatively higher than that of MUSIC.
Fast breathing was the least effective intervention according to the Stroop test results. We did not find a boost in mental ability, as we hypothesized, due to increased pH and neuronal excitability. The acute effects we found were not consistent with chronic effects, as a previous study showed that 12 weeks of fast yoga breathing significantly improved healthy young adults' executive function (Sharma et al., 2014). Fast breathing often accompanies high-anxiety states, and it is possible that voluntarily increasing the breathing rate can trigger mechanisms similar to those triggered by anxiety (Masaoka and Homma, 1997; Homma and Masaoka, 2008). In our study, some men felt nervous after FAST, which might be due to mild hyperventilation and that decreased performance in the Stroop test.
Additionally, as inhalation and exhalation trigger different autonomic nervous activities, we suggest conducting a study to compare the different effects of inhalation/exhalation ratios on executive function.
4.3. Cardiorespiratory activity
The respiration rate, SpO2, and EtCO2 were evaluated to monitor physiological responses and explain the results. Although not the primary focus, there were new findings, and some could meaningfully strengthen the results of previous studies.
Both men's and women's RR during MINDFUL were lower than during MUSIC, which is in line with the finding that MINDFUL tended to result in a lower rate of respiration (Hunt et al., 2021). Paying attention to breathing strengthens breathing activity, even though we do not intend to influence it. In the Stroop test, men's RR after SLOW was the lowest when compared to that of the other interventions. We propose two physiological mechanisms to explain these results. First, SLOW increased ventilation volume, as shown in Figure 6A, which activated the regulation center in the medulla oblongata and inhibited the respiration rate (Kandel et al., 2013). Second, SLOW activated the parasympathetic nervous system (increased vagal tone) (Radaelli et al., 2004). The effect after SLOW decreased stress during the Stroop test, so the respiration rate did not increase as much as it did after MINDFUL and MUSIC. The second proposed mechanism was strengthened by the results of FAST. FAST increased ventilation more than SLOW, and theoretically, it should inhibit RR more than SLOW during the Stroop test, but it did not. Therefore, the reduction in RR after SLOW during the Stroop test was more likely due to the increased parasympathetic activity. Additionally, since RR is an indicator of stress, our findings are consistent with a previous report (Hunt et al., 2021) where SLOW was more effective than MINDFUL in stress reduction.
Oxygen saturation was higher during SLOW than during MUSIC and higher during FAST than during MUSIC and MINDFUL. There were slight differences in the mean and standard deviation between the SLOW and FAST groups. However, EtCO2, directly influenced by ventilation volume, was significantly different between the SLOW and FAST groups. These results confirmed SLOW's superior respiration efficiency compared to FAST, as hemoglobin oxygenation during SLOW can reach levels as high as those during FAST, but with less ventilation.
Consistently, the SpO2 alterations by different interventions did not last longer than 1 min during the Stroop test. A similar result was also found in EtCO2, where only FAST maintained EtCO2 higher than MUSIC in the 1st min. These two findings indicate that the carryover effect of breathing on SpO2 and EtCO2 was short.
4.4. Breathing method preference
Subjective preference should be a serious consideration when choosing a breathing technique because it directly reflects participants' physiological state. In this study, neither men nor women liked FAST, most men liked SLOW, and most women liked MUSIC. Participants chose according to their general subjective feelings of relaxation and working efficiency. The results mean most women felt better after MUSIC, whereas most men felt better after SLOW. Although 52% of men preferred SLOW, there were still 24% who preferred MINDFUL. Similarly, although 48% of women preferred MUSIC, there were still 24% who preferred MINDFUL. These findings demonstrate the diversity of participants' physiological responses after using different methods. Our findings can be used as a reference, but people should choose a breathing method (or music) according to their mental and physical states.
4.5. Limitations
This study has at least four limitations. First, we noticed that some participants' muscle tension changed substantially when their posture was altered. This phenomenon was difficult to control because some participants unconsciously adjusted their posture during the Stroop test. Second, 5 min of MINDFUL might be too short to generate enough of an effect to influence cognitive function and muscle tension, although a previous study found that it significantly reduced distress scores. Third, we did not ask participants the specific reasons for their preference ratings. Fourth, although we used the Latin Square Williams design to balance the influence of the carryover effect, there should be still a carryover effect between each intervention. Future studies might consider testing interventions on different days.
5. Conclusion
Different breathing methods and MUSIC did not significantly influence muscle tension. Men's executive function tended to be better after SLOW than after MUSIC and FAST, whereas women's executive function did not depend on the intervention. SLOW's superior respiration efficiency on SpO2 and inhibition of RR might be the causes of the men's results. Most women preferred MUSIC, and most men preferred SLOW, while FAST was least favorable.
Data availability statement
The data that support the findings of this study are available upon request from the corresponding author JX.
Author contributions
OR, JX, and W-ML contributed to the conceptualization, methodology, and formal analysis. W-ML, JX, F-FR, Z-SC, and Z-MB contributed to data collection and/or processing. W-ML wrote the original draft. OR, JX, F-FR, Z-SC, C-RL, and Z-MB reviewed and edited the manuscript. OR and JX supervised the study, provided equipment, and acquired funds. All authors have read and agreed to the published version of the manuscript.
Funding
JX was funded by the Scientific and Technological Innovation Project of China Academy of Chinese Medical Sciences (No. CI2021A02010). W-ML was funded by Vilnius University, Lithuania.
Acknowledgments
The authors sincerely thank all the participants. The authors are grateful to Zeng Xie for the ethics approval application; to Maiwulamu Aihemaiti, Li Wang, Yiting Sun, and Mengdan Li for data processing; and to all the dear colleagues who provided support and suggestions for this study.
Conflict of interest
The authors declare that the research was conducted in the absence of any commercial or financial relationships that could be construed as a potential conflict of interest.
Publisher's note
All claims expressed in this article are solely those of the authors and do not necessarily represent those of their affiliated organizations, or those of the publisher, the editors and the reviewers. Any product that may be evaluated in this article, or claim that may be made by its manufacturer, is not guaranteed or endorsed by the publisher.
References
Arnsten, A. F. (2009). Stress signalling pathways that impair prefrontal cortex structure and function. Nat. Rev. Neurosci. 10, 410–422. doi: 10.1038/nrn2648
Balconi, M., Angioletti, L., and Crivelli, D. (2020). Neuro-empowerment of executive functions in the workplace: the reason why. Front. Psychol. 11, 1519. doi: 10.3389/fpsyg.2020.01519
Banich, M. T. (2009). Executive function: the search for an integrated account. Curr. Dir. Psychol. Sci. 18, 89–94. doi: 10.1111/j.1467-8721.2009.01615.x
Barrett, K. E., Boitano, S., Barman, S. M., and Brooks, H. L. (2019). Ganong's Review of Medical Physiology. 26th edition. New York: McGraw Hill Medical.
Beng, T. S., Ahmad, F., Loong, L. C., Chin, L. E., Zainal, N. Z., Guan, N. C., et al. (2016). Distress reduction for palliative care patients and families with 5-minute mindful breathing: a pilot study. Am. J. Hospice Palliative Care 33, 555–560. doi: 10.1177/1049909115569048
Bernardi, L., Porta, C., Gabutti, A., Spicuzza, L., and Sleight, P. (2001). Modulatory effects of respiration. Autonomic. Neurosci. Basic Clin. 90, 47–56. doi: 10.1016/S1566-0702(01)00267-3
Bing-Canar, H., Pizzuto, J., and Compton, R. J. (2016). Mindfulness-of-breathing exercise modulates EEG alpha activity during cognitive performance. Psychophysiology. 53, 1366–1376. doi: 10.1111/psyp.12678
Bongers, P. M., Ijmker, S., van den Heuvel, S., and Blatter, B. M. (2006). Epidemiology of work related neck and upper limb problems: psychosocial and personal risk factors (part I) and effective interventions from a bio behavioural perspective (part II). J. Occup. Rehabil. 16, 279–302. doi: 10.1007/s10926-006-9044-1
Bongers, P. M., Kremer, A. M., and Laak, J. ter (2002). Are psychosocial factors, risk factors for symptoms and signs of the shoulder, elbow, or hand/wrist?: A review of the epidemiological literature. Am. J. Ind. Med. 41, 315–342. doi: 10.1002/ajim.10050
Burg, J. M., and Michalak, J. (2011). The healthy quality of mindful breathing: Associations with rumination and depression. Cogn. Therap. Res. 35, 179–185. doi: 10.1007/s10608-010-9343-x
Carter, C., Botvinick, M., and Cohen, J. (1999). The contribution of the anterior cingulate cortex to executive processes in cognition. Rev. Neurosci. 10, 49–58. doi: 10.1515/REVNEURO.1999.10.1.49
Cho, H., Ryu, S., Noh, J., and Lee, J. (2016). The effectiveness of daily mindful breathing practices on test anxiety of students. PLoS ONE 11, e0164822. doi: 10.1371/journal.pone.0164822
Chowdhury, D. (2012). Tension type headache. Ann. Indian Acad. Neurol. 15, S83–88. doi: 10.4103/0972-2327.100023
Cohen, S., Kamarck, T., and Mermelstein, R. (1983). A global measure of perceived stress. J. Health Soc. Behav. 24, 385–396. doi: 10.2307/2136404
da Silva, D. N. F. B., Faro, H. K. C., Tavares, M. P. M., do Nascimento Neto, L. I., Agrícola, P. M. D., da Silva Machado, D. G., et al. (2021). Influence of workplace exercise on workers' cognitive performance. Revista Brasileira de Medicina do Trabalho 19, 157–164. doi: 10.47626/1679-4435-2021-573
Eichenbaum, H. (2004). Hippocampus: cognitive processes and neural representations that underlie declarative memory. Neuron. 44, 109–120. doi: 10.1016/j.neuron.2004.08.028
Endo, K., Matsukawa, K., Liang, N., Nakatsuka, C., Tsuchimochi, H., Okamura, H., et al. (2013). Dynamic exercise improves cognitive function in association with increased prefrontal oxygenation. J. Physiol. Sci. JPS. 63, 287–298. doi: 10.1007/s12576-013-0267-6
Forte, G., Favieri, F., and Casagrande, M. (2019). Heart rate variability and cognitive function: A systematic review. Front. Neurosci. 13, 710. doi: 10.3389/fnins.2019.00710
García-Martínez, D., Torres-Tamayo, N., Torres-Sanchez, I., García-Río, F., and Bastir, M. (2016). Morphological and functional implications of sexual dimorphism in the human skeletal thorax. Am. J. Phys. Anthropol. 161, 467–477. doi: 10.1002/ajpa.23051
Goh, J., Pfeffer, J., Zenios, S. A., Rajpal, S., et al. (2015). Workplace stressors and health outcomes: Health policy for the workplace. Behav. Sci. Policy 1, 43–52. doi: 10.1353/bsp.2015.0001
Haake, A. B. (2011). Individual music listening in workplace settings: an exploratory survey of offices in the UK. Musicae Sci. 15, 107–129. doi: 10.1177/1029864911398065
Halaki, M., and Ginn, K. (2012). Normalization of EMG Signals: To Normalize or Not to Normalize and What to Normalize to? Computational Intelligence in Electromyography Analysis—A Perspective on Current Applications and Future Challenges. IntechOpen. doi: 10.5772/49957
Henning, R. A., Jacques, P., Kissel, G. V., Sullivan, A. B., and Alteras-Webb, S. M. (1997). Frequent short rest breaks from computer work: effects on productivity and well-being at two field sites. Ergonomics. 40, 78–91. doi: 10.1080/001401397188396
Herrero, J. L., Khuvis, S., Yeagle, E., Cerf, M., and Mehta, A. D. (2018). Breathing above the brain stem: volitional control and attentional modulation in humans. J. Neurophysiol. 119, 145–159. doi: 10.1152/jn.00551.2017
Homma, I., and Masaoka, Y. (2008). Breathing rhythms and emotions. Exp. Physiol. 93, 1011–1021. doi: 10.1113/expphysiol.2008.042424
Howard, M., Roiser, J. P., Gilbert, S. J., Burgess, P. W., Dayan, P., and Serpell, L. (2020). Short-term fasting selectively influences impulsivity in healthy individuals. Front. Psychol. 11, 1644. doi: 10.3389/fpsyg.2020.01644
Hunt, M., Rajagopal, T., Cerecino, F., and O'Neil, M. (2021). Mindful versus diaphragmatic breathing: spirituality moderates the impact on heart rate variability. Mindfulness. 12, 2743–2753. doi: 10.1007/s12671-021-01738-x
Jakkaew, P., and Onoye, T. (2020). Non-contact respiration monitoring and body movements detection for sleep using thermal imaging. Sensors (Basel, Switzerland). 20, 6307. doi: 10.3390/s20216307
Janwantanakul, P., Pensri, P., Jiamjarasrangsri, V., and Sinsongsook, T. (2008). Prevalence of self-reported musculoskeletal symptoms among office workers. Occupat. Med. 58, 436–438. doi: 10.1093/occmed/kqn072
Jerath, R., Edry, J. W., Barnes, V. A., and Jerath, V. (2006). Physiology of long pranayamic breathing: neural respiratory elements may provide a mechanism that explains how slow deep breathing shifts the autonomic nervous system. Med. Hypoth. 67, 566–571. doi: 10.1016/j.mehy.2006.02.042
Kandel, E. R., Schwartz, J. H., Jessell, T. M., Siegelbaum, S., Hudspeth, A. J., Mack, S., et al. (2013). Principles of Neural Science, Fifth Edition. New York: McGraw Hill Professional.
Karthikeyan, P., Murugappan, M., and Yaacob, S. (2012). “EMG signal based human stress level classification using wavelet packet transform,” in S. G. Ponnambalam, J. Parkkinen, and K. C. Ramanathan, eds Trends in Intelligent Robotics, Automation, and Manufacturing, Communications in Computer and Information Science. (Berlin, Heidelberg: Springer), p. 236–243. doi: 10.1007/978-3-642-35197-6_26
Kleen, J. K., Sitomer, M. T., Killeen, P. R., and Conrad, C. D. (2006). Chronic stress impairs spatial memory and motivation for reward without disrupting motor ability and motivation to explore. Behav Neurosci. 120, 842–851. doi: 10.1037/0735-7044.120.4.842
Kluger, D. S., and Gross, J. (2020). Depth and phase of respiration modulate cortico-muscular communication. Neuroimage., 222, 117272. doi: 10.1016/j.neuroimage.2020.117272
Kromenacker, B. W., Sanova, A. A., Marcus, F. I., Allen, J. J. B., and Lane, R. D. (2018). Vagal mediation of low-frequency heart rate variability during slow yogic breathing. Psychosom. Med. 80, 581–587. doi: 10.1097/PSY.0000000000000603
Laborde, S., Allen, M. S., Borges, U., Hosang, T. J., Furley, P., Mosley, E., et al. (2022). The influence of slow-paced breathing on executive function. J. Psychophysiol. 36, 13–27. doi: 10.1027/0269-8803/a000279
Laborde, S., Lentes, T., Hosang, T. J., Borges, U., Mosley, E., Dosseville, F., et al. (2019). Influence of slow-paced breathing on inhibition after physical exertion. Front. Psychol. 10, 1923. doi: 10.3389/fpsyg.2019.01923
Laborde, S., Mosley, E., and Thayer, J. F. (2017). Heart rate variability and cardiac vagal tone in psychophysiological research - recommendations for experiment planning, data analysis, and data reporting. Front. Psychol. 8, 213. doi: 10.3389/fpsyg.2017.00213
Larson, M., Chantigian, D. P., Asirvatham-Jeyaraj, N., Van de Winckel, A., and Keller-Ross, M. L. (2020). Slow-paced breathing and autonomic function in people post-stroke. Front Physiol. 11, 573325. doi: 10.3389/fphys.2020.573325
Lehrer, P. M., and Gevirtz, R. (2014). Heart rate variability biofeedback: how and why does it work? Front. Psychol. 5, 756. doi: 10.3389/fpsyg.2014.00756
LoMauro, A., and Aliverti, A. (2018). Sex differences in respiratory function. Breathe 14, 131–140. doi: 10.1183/20734735.000318
Lu, Y., Yi, L., Liu, D., Li, J., Sun, L., Zhang, Z., et al. (2012). Alkalosis leads to the over-activity of cortical principal neurons. Neurosci Lett. 525, 117–122. doi: 10.1016/j.neulet.2012.07.034
Lundberg, U., Dohns, I. E., Melin, B., Sandsjö, L., Palmerud, G., Kadefors, R., et al. (1999). Psychophysiological stress responses, muscle tension, and neck and shoulder pain among supermarket cashiers. J. Occup. Health Psychol. 4, 245–255. doi: 10.1037/1076-8998.4.3.245
Ma, X., Yue, Z-. Q., Gong, Z-. Q., Zhang, H., Duan, N-. Y., Shi, Y-. T., et al. (2017). The effect of diaphragmatic breathing on attention, negative affect and stress in healthy adults. Front. Psychol. 8, 874. doi: 10.3389/fpsyg.2017.00874
Marin, M-. F., Lord, C., Andrews, J., Juster, R-. P., Sindi, S., Arsenault-Lapierre, G., et al. (2011). Chronic stress, cognitive functioning and mental health. Neurobiol. Learn Mem. 96, 583–595. doi: 10.1016/j.nlm.2011.02.016
Masaoka, Y., and Homma, I. (1997). Anxiety and respiratory patterns: their relationship during mental stress and physical load. Int. J. Psychophysiol. 27, 153–159. doi: 10.1016/s0167-8760(97)00052-4
Mclean, L., Tingley, M., Scott, R. N., and Rickards, J. (2001). Computer terminal work and the benefit of microbreaks. Appl Ergon. 32, 225–237. doi: 10.1016/S0003-6870(00)00071-5
Melin, B., and Lundberg, U. (1997). A biopsychosocial approach to work-stress and musculosketal disorders. J. Psychophysiol. 11, 238–247.
Moreira-Silva, I., Teixeira, P. M., Santos, R., Abreu, S., Moreira, C., Mota, J., et al. (2016). The effects of workplace physical activity programs on musculoskeletal pain: a systematic review and meta-analysis. Workplace Health Safety 64, 210–222. doi: 10.1177/2165079916629688
Ohman, L., Nordin, S., Bergdahl, J., Birgander, L. S., and Neely, A. S. (2007). Cognitive function in outpatients with perceived chronic stress. Scandinavian J. Work Environ. Health 33, 223–232. doi: 10.5271/sjweh.1131
Radaelli, A., Raco, R., Perfetti, P., Viola, A., Azzellino, A., Signorini, M. G., et al. (2004). Effects of slow, controlled breathing on baroreceptor control of heart rate and blood pressure in healthy men. J. Hypertens. 22, 1361–1370. doi: 10.1097/01.hjh.0000125446.28861.51
Redlich Bossy, M., Mueller, D., Seifritz, E., Vetter, S., and Egger, S. T. (2020). Feasibility and efficacy of a psychological therapy for patients with a schizophrenic psychosis in an inpatient setting: Study protocol of a randomized switch controlled trial. Front. Public Health. 8, 391. doi: 10.3389/fpubh.2020.00391
Rizzolatti, G., Fogassi, L., and Gallese, V. (2002). Motor and cognitive functions of the ventral premotor cortex. Curr. Opin. Neurobiol. 12, 149–154. doi: 10.1016/s0959-4388(02)00308-2
Roman-Liu, D., Grabarek, I., Bartuzi, P., and Choromański, W. (2013). The influence of mental load on muscle tension. Ergonomics 56, 1125–1133. doi: 10.1080/00140139.2013.798429
Salehinejad, M. A., Wischnewski, M., Ghanavati, E., Mosayebi-Samani, M., Kuo, M-. F., Nitsche, M. A., et al. (2021). Cognitive functions and underlying parameters of human brain physiology are associated with chronotype. Nat. Commun. 12, 4672. doi: 10.1038/s41467-021-24885-0
Sambataro, S., Cervino, G., Bocchieri, S., Bruna, R. L., and Cicciù, M. (2019). TMJ dysfunctions systemic implications and postural assessments: a review of recent literature. J. Funct. Morphol. Kinesiol. 4, 58. doi: 10.3390/jfmk4030058
Schleifer, L. M., Ley, R., and Spalding, T. W. (2002). A hyperventilation theory of job stress and musculoskeletal disorders. Am. J. Ind. Med. 41, 420–432. doi: 10.1002/ajim.10061
Sharma, V. K., Rajajeyakumar, M., Velkumary, S., Subramanian, S. K., Bhavanani, A. B., Sahai, A., et al. (2014). Effect of fast and slow pranayama practice on cognitive functions in healthy volunteers. JCDR 8, 10–13.
Springer, A., Venkatakrishnan, A., Mohan, S., Nelson, L., Silva, M., and Pirolli, P. (2018). Leveraging self-affirmation to improve behavior change: A mobile health app experiment. JMIR mHealth uHealth. 6, e157. doi: 10.2196/mhealth.9151
Tombaugh, G. C., and Somjen, G. G. (1996). Effects of extracellular pH on voltage-gated Na+, K+ and Ca2+ currents in isolated rat CA1 neurons. J. Physiol. 493, 719–732. doi: 10.1113/jphysiol.1996.sp021417
Trevino, M. B., Mazur-Hart, D., Machida, Y., King, T., Nadler, J., Galkina, E. V., et al. (2015). Liver perilipin 5 expression worsens hepatosteatosis but not insulin resistance in high fat-fed mice. Mol. Endocrinol. (Baltimore, Md.). 29, 1414–1425. doi: 10.1210/me.2015-1069
Wang, X., Wu, J., Ye, M., Wang, L., and Zheng, G. (2021). Effect of Baduanjin exercise on the cognitive function of middle-aged and older adults: a systematic review and meta-analysis. Complement Ther. Med. 59, 102727. doi: 10.1016/j.ctim.2021.102727
Yang, W-. Y., Xu, Y., Ye, L., Rong, L-. J., Feng, J., Huang, B-. L., et al. (2023). Effects of Baduanjin exercise on quality-of-life and exercise capacity in patients with heart failure: A systematic review and meta-analysis. Complement Ther. Clin Pract. 50, 101675. doi: 10.1016/j.ctcp.2022.101675
Zaccaro, A., Piarulli, A., Laurino, M., Garbella, E., Menicucci, D., Neri, B., et al. (2018). How breath-control can change your life: a systematic review on psycho-physiological correlates of slow breathing. Front Hum. Neurosci. 12, 353. doi: 10.3389/fnhum.2018.00353
Zheng, G., Li, M., Lan, X., Yan, X., Lin, Q., Chen, L., et al. (2013). The effect of Baduanjin exercise for physical and psychological wellbeing of college students: study protocol for a randomized controlled trial. Trials. 14, 422. doi: 10.1186/1745-6215-14-422
Keywords: slow breathing, mindful breathing, fast breathing, music listening, muscle tension, muscle activity, executive function, cognitive function
Citation: Liang W-M, Xiao J, Ren F-F, Chen Z-S, Li C-R, Bai Z-M and Rukšenas O (2023) Acute effect of breathing exercises on muscle tension and executive function under psychological stress. Front. Psychol. 14:1155134. doi: 10.3389/fpsyg.2023.1155134
Received: 31 January 2023; Accepted: 06 April 2023;
Published: 25 May 2023.
Edited by:
Alice Cancer, Catholic University of the Sacred Heart, ItalyReviewed by:
Andrea Zaccaro, University of Studies G. d'Annunzio Chieti and Pescara, ItalyPatricia Gerbarg, New York Medical College, United States
Copyright © 2023 Liang, Xiao, Ren, Chen, Li, Bai and Rukšenas. This is an open-access article distributed under the terms of the Creative Commons Attribution License (CC BY). The use, distribution or reproduction in other forums is permitted, provided the original author(s) and the copyright owner(s) are credited and that the original publication in this journal is cited, in accordance with accepted academic practice. No use, distribution or reproduction is permitted which does not comply with these terms.
*Correspondence: Jing Xiao, NTgyODAyNDRAc2luYS5jb20=