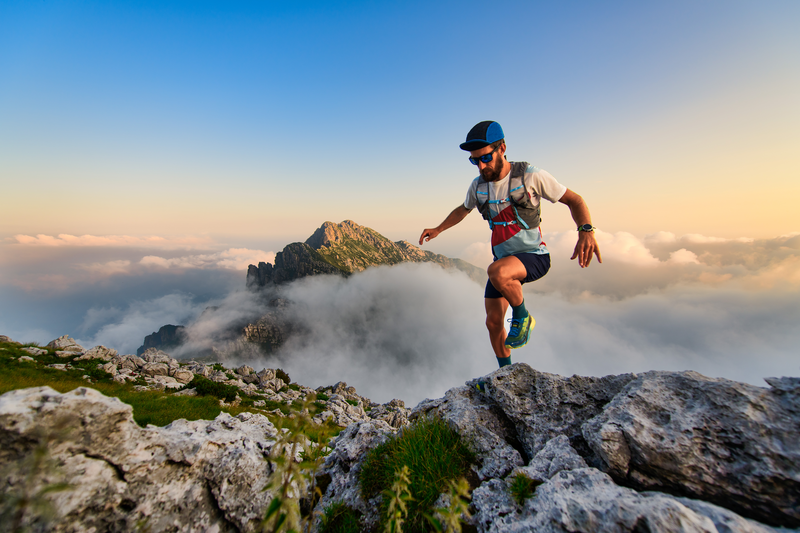
94% of researchers rate our articles as excellent or good
Learn more about the work of our research integrity team to safeguard the quality of each article we publish.
Find out more
REVIEW article
Front. Psychol. , 02 December 2022
Sec. Cognition
Volume 13 - 2022 | https://doi.org/10.3389/fpsyg.2022.950935
This article is part of the Research Topic Using Gaze to study Social Knowledge: Current Challenges and Future Directions View all 7 articles
Social gaze has received much attention in social cognition research in both human and non-human animals. Gaze following appears to be a central skill for acquiring social information, such as the location of food and predators, but can also draw attention to important social interactions, which in turn promotes the evolution of more complex socio-cognitive processes such as theory of mind and social learning. In the past decades, a large number of studies has been conducted in this field introducing differing methodologies. Thereby, various factors influencing the results of gaze following experiments have been identified. This review provides an overview of the advances in the study of gaze following, but also highlights some limitations within the research area. The majority of gaze following studies on animals have focused on primates and canids, which limits evolutionary interpretations to only a few and closely related evolutionary lineages. This review incorporates new insights gained from previously understudied taxa, such as fishes, reptiles, and birds, but it will also provide a brief outline of mammal studies. We propose that the foundations of gaze following emerged early in evolutionary history. Basic, reflexive co-orienting responses might have already evolved in fishes, which would explain the ubiquity of gaze following seen in the amniotes. More complex skills, such as geometrical gaze following and the ability to form social predictions based on gaze, seem to have evolved separately at least two times and appear to be correlated with growing complexity in brain anatomy such as increased numbers of brain neurons. However, more studies on different taxa in key phylogenetic positions are needed to better understand the evolutionary history of this fundamental socio-cognitive skill.
– Studies on gaze following and object choice have traditionally lacked a phylogenetic focus.
– Comparisons between species are especially difficult due to different methodologies used over time.
– A variety of often disregarded factors can potentially impact the results of gaze following experiments.
– Hypotheses about the evolutionary roots of gaze following are possible through comparing this skill in distantly related species.
– Gaze following into the distance appears to be a conserved cognitive trait shared among at least all amniotes, possibly even all vertebrates.
– Geometrical gaze following seems to have evolved convergently in mammals and birds.
– More sophisticated gaze following skills appear to be the result of increased neuronal numbers.
– Non-avian dinosaurs likely already followed others’ gazes.
Sociality in non-human animals takes many forms, ranging from solitary species that meet only to mate, to species living in complex societies. Truly solitary species are rare, as a minimal degree of sociality can be found in many species, at least in the sexually reproductive ones. Species with varying degrees of sociality face different challenges. Consequently, species vary in their socio-cognitive repertoires.
Social interactions rely on the transfer and use of social information. Such information can either be conveyed intentionally through communicative signals or be produced inadvertently. For example, an individual’s presence in a certain location can inform others about food sources and risk of predation. Social information enables the receiver to optimize decisions, and therefore the ability to use such information is adaptive (Morand-Ferron et al., 2010).
Due to the value of social information, various functions have evolved to facilitate its use. One way of acquiring social information is to observe what others are looking at. In this way, one can use others’ visual attention to gather information about the surroundings that would have otherwise remained elusive. The ability to co-orient with others’ gaze directions is called gaze following (Butterworth and Jarrett, 1991).
The advantages of following others’ gazes are numerous and range from gathering information about the location of predators or food sources, to drawing attention to important social interactions (Tomasello et al., 1998). Eavesdropping on social interactions promotes knowledge of third-party relationships and can be used in tactical deception or observational learning (Emery, 2000). Arguably, observational learning would be difficult without gaze following, as the gaze draws attention to central affordances in a task.
Gaze following is an important fundamental skill to study, when uncovering some of the evolutionary roots of social cognition. The presence of gaze following in various distantly related species implies an origin in deep evolutionary time. This quick and easy way of gathering social information might have been the starting point for the evolution of more complex socio-cognitive skills such as empathy and theory of mind (Emery, 2000; Shepherd and Platt, 2008).
Despite gaze following appearing to be a widespread skill, the majority of animal studies have focused on primates and canids. This limits evolutionary interpretations. To better understand the evolution of social cognition in general, and of gaze following in particular, it is necessary to expand research efforts to more distantly related lineages. In recent years, the interest in such taxa has increased and a number of new studies, especially on reptiles and birds, provide insights into the evolution of gaze following.
This review describes general ideas and methodologies of the research field. It also discusses limitations and describes various factors potentially impacting the results of gaze following studies in non-human animals with the aim of improving future experimental designs.
With respect to the evolution of gaze following, this review highlights recent advances in the research on the Sauropsida lineage (reptiles and birds). Though still scarce, new evidence from this lineage is important for understanding deep evolution. We also provide an outline of studies on mammals, including human and non-human primates.
Co-orienting with others’ gazes is a fundamental part of human social cognition. A classic example is when someone in a crowd suddenly looks up and scans the sky. People around will shortly start looking up as well, seeking for the object of interest.
Humans are skilled gaze followers from an early age. In fact, gaze following has first been studied within the discipline of developmental psychology. The earliest insights stem from a study by Scaife and Bruner (1975) that found that human infants start following head directions at 2–4 months, and that all children have developed this ability by 11 months.
It was later shown that already newborns are sensitive to others’ gazes (Batki et al., 2000; Farroni et al., 2002) and have a preference for direct gaze (Farroni et al., 2002). Gaze cueing, where observers orient towards an object in the direction of another’s gaze, also appears to be present in newborns (Farroni et al., 2004).
The ontogenetic onset of gaze following has later been pushed back to 3–6 months (e.g., Butterworth and Jarrett, 1991; Perra and Gattis, 2010). The extent to which infants can modulate their early gaze following responses remains unclear. Senju and Csibra (2008) showed that gaze following of 6-month-old infants depends on ostentive signals such as eye contact or addressing the child. Gredebäck et al. (2018), however, found that infants of the same age responded to gaze cues with and without ostentive signals. More recently, Ishikawa and Itakura (2019) demonstrated that arousal facilitates infants’ gaze following responses, which can in turn be heightened through ostentive signals. It should, however, be noted that the children in this study were between 9 and 10 months old. The ability to modulate gaze following responses based on arousal might thus develop later than spontaneous co-orientations.
These early gaze following responses are, however, not very precise. Infants can only reliably identify the target of observed attention at 12 months (Farroni et al., 2004; Moore, 2008). Moreover, children younger than 10 months follow the head direction of a demonstrator with open and closed eyes without distinction, implying a developmental shift in understanding referentiality of looking around that time (Woodward, 2003; Brooks and Meltzoff, 2005; Csibra and Volein, 2008; Senju et al., 2008). Gaze following abilities are fully developed at 18 months, when the infants follow gazes outside their immediate visual field and behind themselves (Butterworth and Jarrett, 1991; for a recent review see Del Bianco et al., 2019).
The development of gaze following in human infants plays a central role in the development of other socio-cognitive skills, such as theory of mind (Brooks and Meltzoff, 2015), joint attention (Carpenter et al., 1998), and language acquisition (Baldwin, 1991; Schafer and Plunkett, 1998; Houston-Price et al., 2006), illustrating its fundamental role in human social cognition.
The neurocognitive mechanisms underlying gaze following are not fully understood. The consensus is, however, that two distinct pathways guide co-orientations with observed gaze directions. Fast, reflexive co-orientations (Deaner and Platt, 2003) are mediated by an evolutionary conserved subcortical pathway (Sewards and Sewards, 2002; Johnson, 2005) providing fast, yet crude co-orienting responses to gaze. In mammals, the subcortical pathway is proposed to run from the retina to the superior colliculus, the pulvinar, and finally to the amygdala (Morris et al., 1999; Johnson, 2005; Jiang and He, 2006). In fishes, amphibians, reptiles, and birds, the optic tectum represents the homologue of the superior colliculus, while the rest of the pathway remains the same.
This subcortical pathway is, however, likely insufficient to mediate spatially sophisticated gaze following, such as tracking others’ gazes around barriers. Thus, the subcortical pathway is most likely interconnected with more nuanced cortical networks in mammals, such as the fusiform gyrus (face perception and recognition: Johnson, 2005), the exastriate body area (visual processing of the body: Downing et al., 2001) and the superior temporal sulcus (functions explained below; Shepherd, 2010). The cortical homologues responsible for more complex gaze following skills in other vertebrates remain unknown.
The superior temporal sulcus (STS) of humans and non-human primates has been found to contain cells reacting to different facial orientations (e.g., Perrett et al., 1982) and some are specifically sensitive to the direction of eye gaze (Perrett et al., 1985; Yamane et al., 1988). Hence, this structure might be important in the detection of others’ visual attention.
The STS projects onto the parietal intraparietal cortex in macaques (Harries and Perrett, 1991). The lateral part of this structure (lateral parietal area, LIP) houses “gaze mirror neurons,” i.e., neurons that fire both, when looking at a specific location and when watching someone else gazing toward the same location (Shepherd et al., 2009). Such neurons might cause attentional shifts through matching the observed gaze direction with one’s own visual attention, similar to the functioning of mirror neurons of the motor system (Rizzolatti et al., 2009). Visuo-social areas of the fusiform gyrus and STS are moreover interconnected with an extended face processing network that might further modulate gaze following responses (Ishai et al., 2005; Vuilleumier and Pourtois, 2007).
Data on cortical pathways of gaze following stem exclusively from humans and macaques. Nevertheless, it has been proposed that social processing areas might be homologous in all primate (Tootell et al., 2003; Rosa and Tweedale, 2005) and possibly other mammalian species (Kendrick et al., 2001). How other taxa, such as birds, achieve spatially sophisticated gaze following skills remains unclear and will need to be addressed in future studies.
A variety of animal species have been tested for their capacity to follow others’ gaze direction. While in human studies, a distinction is often made between following the direction of the head and shifts of eye gaze alone, most studies on animals use a head directional cue in their gaze following experiments. For that reason, in this review, when speaking of gaze following, we refer to co-orientations with head directions. For a more detailed discussion of this topic, see “Eye morphology”.
Within the great number of animal gaze following studies, many factors and inconsistent methodologies can significantly impact subjects’ performances. Two experimental paradigms are used when studying how animals use social information conveyed through gaze: (1) visual co-orientation, and (2) object choice. In the former, the animal is presented with a demonstrator looking toward a specific location in the environment and the observer’s co-orientation with the line of gaze is recorded. In object choice, subjects are tested for their capacity to use gaze cues to identify the location of food. For an overview of species tested in different experimental paradigms, see Table 1.
Table 1. Overview of species tested in different gaze following paradigms and corresponding references.
Many different factors can influence a species’ performance in gaze following experiments. Realistically, it is difficult to take all of the following issues into account. Nevertheless, this summary should provoke researchers to closely familiarize themselves with the species and subjects they are testing. These considerations should further be taken into account when designing gaze following experiments.
Visual co-orientation is commonly tested in two ways, in line with Povinelli and Eddy’s low-and high-level model of gaze following (Povinelli and Eddy, 1996a). According to this first account of gaze following in chimpanzees (Pan troglodytes), low-level gaze following is a simple co-orienting reflex that does not require any mentalistic attributions. High-level gaze following, on the other hand, requires a representation of others’ visual perspectives.
To test these models, two different experimental setups are used: gaze following into the distance (from here on GFD) and geometrical gaze following (from here on GGF). In the former, a demonstrator is gazing either up or to the side while the reaction of the observer is recorded. This is considered to require only low-level cognitive abilities without an attribution of mental states. GGF refers to tracking gazes around barriers. It is elicited by a demonstrator gazing toward a location behind a barrier that is not seen from the subject’s location. If an observer were to simply co-orient with the demonstrator, one would expect it to look at the barrier. An observer capable of GGF, though, moves around the barrier to inspect the target of the demonstrator’s gaze. This is regarded as high-level gaze following as it is believed to require an understanding of visual perspectives (Bräuer et al., 2005).
Most studies on gaze following in animals have focused on GFD. GGF has been tested in primates (Bräuer et al., 2005; Amici et al., 2009; Bettle and Rosati, 2019), canids (wolves: Range and Virányi, 2011; domestic dogs: Met et al., 2014), birds (Corvids: Bugnyar et al., 2004; Schloegl et al., 2007, 2008b; Northern bald ibises: Loretto et al., 2009; European starlings: Butler and Fernández-Juricic, 2014), and two reptile species (Bearded dragons: Siviter et al., 2017; American alligators: Zeiträg et al., 2022).
In the object choice paradigm, an experimenter indicates the location of a hidden piece of food through a cue such as pointing, tapping, or gazing. In this review, we focus exclusively on gaze cues and their use in locating food. Several variations of the object choice paradigm exist: classic object choice, competitive object choice, food theft competition, and the guesser-knower task, which will be explained below.
In the classic object choice paradigm, an experimenter is cueing the subject towards one of two objects that is baited with food. These objects are usually small containers such as cups. When the animal has chosen, the remaining object is removed from access of the subject.
In experiments where gazes are used as cues, a distinction is made between gaze—referring to a shift of both, head and eye direction—and glance—referring to a shift of the eyes alone (e.g., Neiworth et al., 2002; Scheumann and Call, 2004; Burkart and Heschl, 2006).
Surprisingly, most tested species struggled with this paradigm and failed to use gaze to locate food (Anderson et al., 1995; Schloegl et al., 2008a; Giret et al., 2009). The disparity between the seemingly ubiquitous gaze following, but poor results in object choice experiments, suggests a difference in the mental processing of gaze direction and its use in finding food.
Call et al. (2000) argued that there is a functional disparity between following gaze and foraging, and that chimpanzees do not seem to apply their elsewhere proven gaze following skills in foraging contexts. In the same study the authors found that vocalizations and other behaviors, such as approaching the baited object, significantly increased performance. The authors argued that these additional behaviors drew the subjects’ attention towards the demonstrator’s head direction which was then used to locate the hidden food. They called this the attention boosting hypothesis.
However, this hypothesis does not explain why many species follow gaze, as they seemingly cannot use it for locating food. Therefore, one must disentangle whether gaze following takes place in foraging contexts at all, or whether many species are unable to infer the location of food from observed gaze directions.
One study on common marmosets (Callithrix jacchus) showed that the monkeys followed the experimenter’s gaze to the correct container, but were unable to find the hidden food (Burkart and Heschl, 2007). Call et al. (2000) argued in their study that chimpanzees did not understand the communicative and informative intent of the human experimenter. That would also explain why adding vocalizations can enhance object choice performance, as it might convey and emphasize the communicative intent of the situation to the subject. Similarly, Hauser and Wood (2011) found improved performances of rhesus macaques (Macaca mulatta) in an object choice task when using “communicative gaze gestures”, i.e., wide open eyes and jerking the head several times towards the target object. However, Burkart and Heschl (2007) provide an alternative explanation for their marmosets’ behavior: looking at an object might be interpreted as an indication of ownership and lead to avoidance of that object. While this explanation seems reasonable for the cooperatively breeding marmosets, it does not explain the behavior of competitive species such as chimpanzees.
In fact, two lemur species, black lemurs (Eulemur macaco) and brown lemurs (Eulemur fulvus), have been reported to react contrary to common marmosets (Ruiz et al., 2009). In an object choice experiment, the lemurs were more likely to locate hidden food after they had successfully followed the gaze direction from a conspecific’s photograph. The poor performance of many species in object choice experiments is according to this study caused by low gaze following rates. The authors called this effect gaze priming, i.e., an increase of saliency of an object or location through following gaze direction. However, it should be noted that this is, to our knowledge, one of only two studies using conspecific demonstrators (even though just photos) in an object choice experiment, which might have significant impacts on subjects’ behaviors (for a study on ravens, see: Schloegl et al., 2008a).
Unfortunately, object choice studies rarely report whether subjects had followed the demonstrator’s gaze toward the correct object or not. More of these reports are needed to understand the reasons behind the poor performance of many species in the object choice paradigm. Nevertheless, two factors have been identified to improve subjects’ performance. Chimpanzees have been found to perform better when leaving the experimental area after each trial, and having to approach the experimenter at the beginning of each new trial (Barth et al., 2005). This indicates an attentional issue in “classic” object choice setups, where subjects stay in the same place between trials.
Secondly, chimpanzees perform better when the food is hidden in, or behind, an object that allows visual access to the experimenter, but blocks the subject’s view (Call et al., 1998). This is the case with, for example, tubes or barriers, where the experimenter can see the hidden food while cueing its location to the subject. It could be argued that animals do not perceive an experimenter as knowledgeable of the food’s location when they cannot see the hidden food at the time they are giving cues to the subject (for alternative explanations see Call et al., 1998).
Though many species struggle with object choice experiments, it seems as though changes to the setup can significantly improve subjects’ performances. For that reason, alternative versions of the classic object choice paradigm have been introduced.
One alternative explanation for the poor performance of many species in object choice experiments could be that informing others about the location of hidden foods does not come naturally to many animals. Several authors have argued that especially competitive species, such as chimpanzees, would hardly ever inform conspecifics about hidden food, making the experimental setup much less relevant to them. For that reason, Hare et al. (2000) invented the competitive object choice paradigm. This experiment has, to our knowledge, only been conducted on primates. In the competitive object choice setup, two individuals, one dominant and one subordinate, are observing an experimenter placing two food items in an experimental room. One of the food items is visible to both participants, while another is only visible to the subordinate. Once the food is placed, both individuals are released into the experimental room. If the subordinate understood the visual perspective of the dominant, it would be expected to first go and collect the hidden piece of food to avoid competition with the dominant. A variety of primate species appeared to demonstrate an understanding of others’ visual “perspectives” in the competitive object choice paradigm (see below).
In an additional condition in this paradigm, Hare et al. (2000) introduced a delay to the release of the dominant in relation to the subordinate in order to rule out that the choice made by the subordinate would be influenced by the approach and choice of the dominant competitor, i.e., that the subjects were simply choosing the food item that the dominant was not choosing.
Chimpanzees appeared to take visual access of the dominant into account by choosing the food item only visible to them over the one visible to both. Subordinates were still choosing the food item only visible to them when being released with a head start. The same behavior was found in common marmosets (Burkart and Heschl, 2007) and long-tailed macaques (Macaca fascicularis; Overduin-de Vries et al., 2014). Subordinate capuchin monkeys (Cebus apella) also displayed a preference for the hidden food (Hare et al., 2003). This preference, however, broke down when granting the subordinate a head start into the arena.
Using cross-correlations of subordinates’ and dominants’ behaviors, Hall et al. (2014) showed that subordinate chimpanzees rarely follow the dominant’s gaze in a competitive object choice setup. This supports the hypothesis that subordinates are not simply choosing based on the other’s behavior. Dominants, on the other hand, were following subordinates’ gazes and adapting their foraging technique to that of the conspecific with privileged knowledge about the location of food.
To further control for behavior-reading, Overduin-de Vries et al. (2014) introduced a one-way mirror in their study on long-tailed macaques that allowed the subordinate to see the dominant, creating the illusion of a competitive situation. The dominant, however, could not actually see the subordinate or the food, excluding the possibility of giving behavioral cues about which food they will be choosing to the subordinate. Interestingly, in this setup fast subordinates were often observed collecting both food items. In those instances, subjects were going for the visible food item first, before collecting the food only visible to them. Slower individuals more often only chose the food item that was not visible to their competitor.
In the food theft competition, the subject’s choice of object is mediated by its gaze sensitivity in a competitive setting. Two food items are placed in the experimental room, with an experimenter monopolizing one of the items through closer proximity or visual orientation to the item. If the subject chooses the object by the experimenter, the object is immediately removed from access and the trial ends. If the subject chooses the object not contested by the experimenter, it may eat the food item.
A variety of primate species have been successful in this paradigm, including gibbons (Sánchez-Amaro et al., 2020), Old world monkeys (Vick and Anderson, 2003; Flombaum and Santos, 2005), and one species of lemur (ringtailed lemurs, Lemur catta), while three other lemur species failed in this study (mongoose lemurs, Eulemur mongoz; black lemurs, Eulemur macaco; ruffed lemurs,Varecia variegata rubra; Sandel et al., 2011).
In a more complex setup, chimpanzees could reach for a banana either through an opaque or a transparent tunnel (Melis et al., 2006). Chimpanzees preferred to conceal their actions through choosing the opaque tunnel. Orangutans did not show a preference in a replication of this experiment, failing to show an understanding of visual access (Gretscher et al., 2012).
In this object choice paradigm, the subject’s understanding of a demonstrator’s visual access is tested. The standard setup involves a subject and two demonstrators. Food is then hidden outside the visual access of the subject, while one demonstrator has visual access to the baiting process (the knower), and the other demonstrator does not (the guesser). This can be achieved through the guesser being absent (e.g., Maginnity and Grace, 2014), turned away (e.g., Call et al., 2000), or having their eyes closed (Proops and McComb, 2010) during baiting. Both demonstrators (or one demonstrator in different trials) subsequently perform a cue towards one of two objects. A subject with an understanding of visual access should prefer the object indicated by the informed demonstrator.
Two primate species have been successful in this paradigm: chimpanzees (Call et al., 2000) and capuchin monkeys (Defolie et al., 2015). Moreover, some domesticated species could infer visual access of humans: domestic dogs (Canis familiaris; Virányi et al., 2004; Maginnity and Grace, 2014; Catala et al., 2017), domestic horses (Equus caballus; Proops and McComb, 2010; Ringhofer et al., 2021), and domestic pigs (Sus scrofa domesticus; Byrne et al., 2001).
Only one bird species has been subject to the guesser-knower task: common ravens (Corvus corax; Bugnyar, 2011). Ravens were watching a human experimenter cache food in the presence of two conspecifics: a guesser, whose view was obstructed during caching, and a knower, that could witness the caching process. After releasing the subject and either of the two conspecifics into the arena, subjects were pilfering the caches quicker when in competition with an informed conspecific, showing that they were taking others’ visual access into account.
The experimental study of gaze following requires a gaze cue by a demonstrator. Such a demonstrator should on command gaze towards a specific location. To ensure controlled testing conditions, the majority of studies, especially on primates—have used human demonstrators (for an overview see: Rosati and Hare, 2009). This allows for controlling parameters such as looking time, specific target of gaze and the disentanglement of head direction and eye-gaze alone. While it is doubtless beneficial to keep testing conditions as controlled as possible, the relevance of human gaze cues to animals, especially non-primates, has been debated.
The large body of literature on gaze following in a variety of species indicates that many animals can indeed follow the gaze of a human demonstrator—even around barriers. That, however, does not mean that the frequency and sophistication are representative of the species’ socio-cognitive potential. Though commonly brought up in discussions, few studies have directly addressed this topic.
One study on cotton-top tamarins (Saguinus oedipus oedipus) for example showed that the monkeys did not follow the gaze of humans, but only of conspecifics (Neiworth et al., 2002). Contrarily, other primate studies have reported comparable responses to human and conspecific demonstrators (Tomasello et al., 1998; Hare and Tomasello, 2004; Herrmann et al., 2007). However, Byrnit (2004) described that non-enculturated orangutans, i.e., parent-raised, failed to use human gaze cues to identify a target object. Similarly, only enculturated chimpanzees have been found to be sensitive to visual attentive states of human experimenters in a guesser-knower task (Call et al., 2000).
If previous exposure to humans indeed impacts animals’ sensitivity to human gaze cues, the ability to follow human’s gaze might vary significantly depending on age and experience. To our knowledge, only one observational account of such a developmental effect exists from common ravens (Schloegl et al., 2007). The authors observed the first spontaneous co-orientations of raven nestlings to their conspecifics approximately 7 weeks before they started to react to the gaze of a human experimenter.
Previous exposure to humans could also explain the excellent performance of domestic dogs using human gaze cues in object choice tasks (e.g., Miklósi et al., 1998; Hare and Tomasello, 1999; Agnetta et al., 2000; Soproni et al., 2001). It has been argued that this is the result of their long history of domestication and exposure to humans. A direct comparison of dogs and chimpanzees in an object choice task revealed that dogs indeed outperform chimpanzees when using communicative cues given by human experimenters, such as pointing and gazing. Chimpanzees, on the other hand, were better at inferring the location of the hidden food from causal cues such as the sound produced when shaking the baited container or the shape of a board that is slanted because of food being hidden under it (Bräuer et al., 2006). This further supports the hypothesis that the poor performance of chimpanzees in object choice tasks is not caused by a lack of understanding, but rather because they do not recognize the communicative intent of the human experimenter and therefore fail to use it as a cue.
Hattori et al. (2010) compared the responses of humans and chimpanzees to con-and allospecific gaze cues and found that chimpanzees follow human gaze significantly less compared to conspecific gaze and moreover look longer at faces of other chimpanzees than of humans. Interestingly, humans were equally sensitive to gazes of both demonstrator species.
Taken together, these findings indicate that some enculturated individuals and domesticated species might respond stronger to human compared to conspecific gaze cues. However, a recent study on gaze following in domesticated ungulates found that even domesticated species preferred to follow conspecific gazes (Schaffer et al., 2020). This suggests that for the majority of species, the use of conspecific demonstrators would be beneficial and that many studies might not have revealed the full gaze following potential of their tested species.
To keep experimental conditions controlled while using conspecific demonstrators, some studies have used photographs and even videos of conspecifics to induce gaze following responses. This seems to work surprisingly well. Primates such as rhesus macaques (Lorincz et al., 2000), Diana monkeys (Cercopithecus diana diana; Scerif et al., 2004), and two lemur species (Ruiz et al., 2009) followed the gaze direction of a photograph of a conspecific to an object. Several primate species have also been found to co-orient to videos of conspecifics, such as chimpanzees, bonobos, orangutans (Kano and Call, 2014) and rhesus macaques (Emery et al., 1997).
However, not only primates have been found to co-orient with artificial stimuli. Bearded dragons (Pogona vitticeps) followed the video of a conspecific gazing up and to the side (Siviter et al., 2017), and even archerfish co-oriented themselves with a photograph of a conspecific (Leadner et al., 2021). Butler and Fernández-Juricic (2014) have gone as far as creating a robot version of a European starling (Sturnus vulgaris) to test for GGF skills in this species. The subjects followed the robot’s gaze behind barriers, suggesting that artificial demonstrators can be used in GGF studies.
One problem when interpreting gaze following is knowing exactly where an animal is looking. Birds, for example, can switch from lateral to binocular vision, therefore the target of their gaze cannot be deduced from their eye orientation (Dawkins, 2002). In animal studies, the target of gaze can only be inferred. This is usually done by extrapolating postural indicators such as beak or snout direction.
Similarly, when using conspecifics as demonstrators, they usually orient their bodies or at least the head toward that location. In the presence of a co-orienting response, it is therefore difficult to discern which cue the observer has been following, the direction of the body, the head, or the eyes alone. Some studies on primates have started to disentangle these cues, but again with human demonstrators to specifically control the body parts orienting toward the target (e.g., Anderson et al., 1996; Neiworth et al., 2002; Burkart and Heschl, 2006).
Differences in the accuracy of gaze tracking might be caused by varying eye morphologies. It has long been believed that human eyes are unique in their salience through the contrast between the white sclera and the dark central iris. This eye morphology has been thought to allow for accurate identification of the target of the conspecifics’ gaze, and has been an explanation for the ability of humans to use eye gaze alone, in comparison to non-human primates that rely on head direction to track gaze (Tomasello et al., 2007). This has been called the cooperative eye hypothesis. However, a recent study found that despite the differences in scleral pigmentation, the contrast between sclera and iris is comparable for great apes and humans (Perea-García et al., 2019). Thus, the contrast of the eyes alone is not sufficient to explain the differences in the sophistication of gaze following between humans and non-human primates.
Kano et al. (2022) recently shed new light on this question. Humans and chimpanzees were asked to choose one out of three pictures of faces of humans and chimpanzees. The task was to pick the face with averted eyes. In different conditions, parameters of the eyes, such as brightness and size were manipulated. Humans and chimpanzees were both better at discriminating the human’s eye direction and this effect was most prevalent in visually challenging conditions, i.e., when the pictures were shaded or small. The authors argued that the uniformly white sclera of humans, rather than the contrast of sclera and iris, is responsible for human gaze following skills as it makes it easier to follow eye movement alone rather than head direction.
Other animals, however, do not possess such an eye morphology and need to rely on other directional cues, such as head directions. While it seems likely that the evolution of a conspicuous eye morphology in humans has refined the ability to extract social information from the eyes alone, it does not mean that other animals lacking this morphology cannot follow others’ gaze directions. They, however, need to rely on different directional cues. In ravens, for example, the direction that the beak is pointing towards is a clearer indicator of others’ visual attention than a shift of the small, dark, and evenly colored eyes.
The phylogenetic ubiquity of co-orientations in combination with evidence for an evolutionary conserved subcortical pathway guiding gaze following responses moreover indicate that the evolutionary roots of this skill run deeper than the evolution of human eye morphologies. It is, hence, more likely that uniquely human abilities’ in extracting directional cues from shifts of eye directions alone are a species-specific extension of the gaze following capacities of other animals rather than a separate, more sophisticated way of extracting directional information.
Another factor influencing gaze following are the emotions associated with attentional shifts. Goossens et al. (2008) have studied this effect in long-tailed macaques through a human experimenter accompanying their gaze shifts with mimicked facial expressions representing different emotional states for the monkeys, namely aggression, submission and affiliation. The authors reported that long-tailed macaques were more likely to follow gaze when the attentional shift was accompanied by facial expressions of fear and submission. This could be an indication that gaze following can be employed flexibly in socially meaningful situations.
Teufel et al. (2010) found that facial expressions generally facilitated gaze following in Barbary macaques (Macaca sylvanus), resulting in a higher gaze following frequency in trials where the gaze cue was accompanied by a facial expression. This effect was present in all age classes but was reduced in older individuals, indicating an impact of experience on modulating gaze following responses. While it might be beneficial for developing individuals to pay attention to as much detail in their social and physical environment as possible, more experienced individuals might only attend to events that are critical to them. In this study, the strongest effect on gaze following was observed when gaze cues were accompanied by “commenting” facial expressions. These are facial expressions that Barbary macaques make when observing third-party interactions. The authors therefore argued that the augmenting effect of facial expressions on gaze following responses might have evolved to facilitate the acquisition of social knowledge through drawing attention to social interactions. In humans, negative facial expressions, such as disgust and fear, have been shown to cause quicker gaze following responses compared to happy or neutral faces (e.g., Pecchinenda et al., 2008; Matsunaka and Hiraki, 2014). In contrast, a similar study on pigtail macaques (Macaca nemestrina) did not find evidence for an effect of facial expressions on gaze following performances (Paukner et al., 2007).
These experimental setups are limited firstly to emotions that are visible in facial expressions, and secondly to species exhibiting facial expressions, which is mainly the case in primates. This however does not mean that other animals are not affected by observing facial expressions. Horses have, for example, been shown to follow human gaze less frequently when the experimenter expressed disgust, indicating interspecies interpretation of facial expressions (Baba et al., 2019).
Not only does the emotional state of the demonstrator impact gaze following performances, but also the emotional state of the subject. Putnam et al. (2016), for example, found that rhesus macaques were more likely to follow gaze in response to videos of a conspecific after inhaling intranasal oxytocin. The oxytocin might enhance the motivation for social interactions through receptors projecting onto social cortical regions, such as the STS.
Social dynamics, i.e., the relationship between the demonstrator and the observer, can affect the likelihood of gaze following. For example, in rhesus macaques, social status impacts gaze following (Shepherd et al., 2006). While low-status males followed the gaze of all familiar conspecifics, high-status males exclusively followed the gaze of other high-status individuals. For low-ranking individuals, it might be crucial to monitor the behavior of their conspecifics to avoid aggressive encounters, while high-ranking individuals are only threatened by other high-ranking monkeys. This shows that gaze following responses may be modulated by social context to optimize gathering of relevant social information. Contrarily, in Barabary macaques, the social status of the demonstrator does not influence gaze following responses (Teufel et al., 2010).
But status, or even kinship, is not always the most important social factor in gaze following. In crested macaques (Macaca nigra), such factors had no influence, but instead strong positive bonds between individuals facilitated quicker responses in gaze following (Micheletta and Waller, 2012). The authors argued that social information from friends might be more relevant due to shared interests and motivations. Also, when locating resources such as food, the competition might be reduced between affiliates. A different motivation to follow a friend’s gaze could be to monitor social interactions involving affiliates to support in conflicts or provide post-conflict affiliation.
Social dynamics also play a role when using human demonstrators. This effect was reported in a study on object choice in jackdaws (von Bayern and Emery, 2009). The birds only responded to gaze cues by a familiar experimenter. Similar to the crested macaques, familiarity could make it easier for jackdaws to predict the other’s actions, especially in a competitive situation.
Lastly, an effect that has rarely been tested in animals, is the impact of sex on gaze following. In humans, women show a stronger gaze cueing effect, i.e., they are more likely to follow other’s gaze (Bayliss et al., 2005). They moreover react quicker to gaze than men. Interestingly, this sex-difference was correlated with self-reported empathy levels (Alwall et al., 2010).
The only reports of such an effect in animals stem from rhesus and Barbary macaques. Both studies found that females were more likely to follow gaze (Paukner et al., 2007; Rosati et al., 2016). In contrast, a different study on Barbary macaques found that sex had no effect on gaze following (Teufel et al., 2010). More studies are needed to understand sex differences in gaze following and their connections to empathy in animals.
Primates play a central role in the study of gaze following as most experimental methods have been developed in primate studies (see sections above), with chimpanzees being the first animal species to be tested in this paradigm. Here, we provide a brief overview of the current state of knowledge on gaze following in primates (for more detailed reviews see: Emery, 2000; Zuberbühler, 2008; Rosati and Hare, 2009; Shepherd, 2010).
To date, reports of GFD of at least some members from all major primate radiation exist: apes, including great apes (e.g., Bräuer et al., 2005) and gibbons (Horton and Caldwell, 2006), Old World monkeys (e.g., Emery et al., 1997), New World monkeys (e.g., Neiworth et al., 2002), and lemurs (e.g., Shepherd and Platt, 2008; but see Ruiz et al., 2009).
The number of GGF studies is lower. Evidence for gaze following around barriers exists from all major primate radiations besides the lemurs: great apes (e.g., Bräuer et al., 2005), Old World monkeys (e.g., Goossens et al., 2012) and one species of New World monkey (common marmosets: Burkart and Heschl, 2006).
Many primates have been found to struggle with using gaze cues to find food in the classic object choice task (e.g., Anderson et al., 1995; Neiworth et al., 2002; Burkart and Heschl, 2006). It seems unlikely that primates are incapable of locating food based on experimental cues. It rather appears that they fail to understand the communicative intent of a human experimenter. A competitive version of this experiment improved the performance of some species in that they avoided food items that dominant conspecifics were looking at (chimpanzees: Hare et al., 2000; capuchin monkeys: Hare et al., 2003; common marmosets: Burkart and Heschl, 2007; long-tailed macaques: Overduin-de Vries et al., 2014). Successful performances in this task are usually interpreted as an understanding of visual access in the tested species, though close attention needs to be paid to exclude the possibility of solving the task through behavior-reading.
Few more primate species have been tested in other variations of the object choice paradigm: food theft competition and guesser-knower task. Many primates seem successful in a food theft competition task (olive baboons, Papio anubis: Vick and Anderson, 2003; rhesus macaques: Flombaum and Santos, 2005; chimpanzees: Melis et al., 2006; ringtailed lemurs: Sandel et al., 2011; gibbons: Sánchez-Amaro et al., 2020), which supports the hypothesis that a competitive setting is more relevant to a variety of species. Two species have moreover been found to infer visual access of human experimenters in a guesser-knower task, namely chimpanzees (Call et al., 2000) and capuchin monkeys (Defolie et al., 2015).
Other than primates, the largest body of literature on the use of gaze cues in mammals stems from domestic dogs. Dogs successfully use variations of gaze to locate hidden food, making them significantly better at this task than any tested primate species (Hare et al., 1998; Miklósi et al., 1998; Agnetta et al., 2000; McKinley and Sambrook, 2000; Soproni et al., 2001; Bräuer et al., 2006). The cues in these studies include gazing, gaze alternations, glancing, different numbers of objects to choose from, and different distances between experimenter and object, i.e., the cue being performed by an experimenter standing close to or far away from the baited container. In a guesser-knower task, dogs demonstrated an understanding of visual access by preferring the cue of the knower over the one of the guesser (Maginnity and Grace, 2014; Catala et al., 2017) and by begging more from a human facing the dog compared to one with their back turned (Virányi et al., 2004).
Interestingly, the results are less clear when it comes to GFD. It has been reported that dogs do not spontaneously follow human gaze into distant space (Agnetta et al., 2000). Their performance improved, though, when the target of gaze was clearly defined, or when the communicative intent was made clear through ostensive cues, such as calling the dogs’ name (Téglás et al., 2012; Werhahn et al., 2016; Duranton et al., 2017). In a comparison with pack-living domestic dogs and wolves (Canis lupus), wolves actually followed human gaze more frequently, while both species followed their packmates’ gaze at comparable rates (Werhahn et al., 2016).
An explanation for this phenomenon could be that dogs are overly focused on humans and struggle with directing their attention away from them to a location in the environment. Wallis et al. (2015), for example, reported that even a short training of dogs to seek eye contact with humans disrupted their gaze following responses. With respect to GGF, dogs seemed capable of tracking humans’ gazes around visual barriers, and even more so in a foraging context, i.e., when they were aware of food being hidden (Met et al., 2014). This suggests that domestication might favor the use of gaze cues to locate food but might simultaneously hinder spontaneous gaze following responses.
Other canids tested are wolves, dingoes (Canis lupus dingo) and silver foxes (Vulpes vulpes). Hand-raised wolves followed both, human and conspecific gaze into the distance and around barriers. They were found to follow humans’ gaze into distant space at 14 weeks, while GGF only developed after 6 months (Range and Virányi, 2011). The difference in ontogeny supports the hypothesis of two different cognitive processes developing at different times. The comparably later onset of GGF indicates a more complex underlying mechanism that takes longer to develop. Dingoes failed to locate hidden food using gaze cues given by a human experimenter (Smith and Litchfield, 2010). Domesticated silver fox kits performed comparable to dogs in an object choice tests with gaze cues and significantly better than their feral counterparts (Hare et al., 2005). Only one study on domestic cats (Felis silvestris catus) in an object choice paradigm exists. Cats were able to use human gaze to locate hidden food even without ostensive cues (Pongrácz et al., 2019). These studies support the hypothesis that previous exposure to humans and domestication drastically improve animals’ interpretation of experimenter-given cues.
A variety of farm animals have been subject to gaze following studies. Visual co-orientation has been tested in a number of ungulates: domestic goats (Capra hircus), llamas (Lama glama), guanacos (Lama guanicoe), and mouflons (Ovis orientalis orientalis; Kaminski et al., 2005; Schaffer et al., 2020). All these species, except for the guanacos, followed humans’ gazes into distant space. In an object choice task, however, goats failed to use experimenter-given gaze cues to find hidden food (Kaminski et al., 2005). In a follow-up study, Nawroth et al. (2015) showed that dwarf goats (Capra aegagrus hircus) have an understanding of visual attention of humans through increased anticipatory behaviors when a human was facing them compared to when they were not. However, they did not—just as many primate species—apply these skills in an object choice task.
In contrast, juvenile domestic pigs could find hidden food using a human’s head and body orientation, but interestingly did not follow head direction into distant space (Nawroth et al., 2014). In a guesser-knower task, pigs could choose to follow an informed or uninformed conspecific into one of two corridors, of which one has been baited with food before (Byrne et al., 2001). One pig successfully solved this task. These findings indicate that negative results from one testing paradigm do not necessarily predict a species’ performance in another paradigm.
Domestic horses identified a visually attentive experimenter over an inattentive one to approach for food, using body and head orientation as well as open or closed eyes as cues for visual attention. However, when the attentional cues were mixed, the horses’ performance broke down (Proops and McComb, 2010). They moreover passed a guesser-knower task and thus demonstrated sensitivity to visual attention of human experimenters (Ringhofer et al., 2021). In an object choice task, however, horses could not use alternating gaze as a cue to find hidden food (Proops et al., 2010).
Finally, some other mammalian species have been tested in object choice experiments. Asian elephants (Elephas maximus) did not use any human-given cues to locate food (Ketchaisri et al., 2019). Bottlenose dolphins (Tursiops truncatus) were able to use static and dynamic gaze to identify the correct object but failed with eye gaze alone (Tschudin et al., 2001; Pack and Herman, 2004). South African fur seals (Arctocephalus pusillus) used human gaze with head direction to identify the correct location of food (Scheumann and Call, 2004), while Gray seals (Halichoerus grypus) failed to do so (Shapiro et al., 2003).
The above-presented studies show a large variety in gaze following and the use of gaze in locating food within mammals. The majority of studies have focused on object choice paradigms, which appears inherently difficult to many animals. Gaze following studies in mammals are rare, limiting inferences of the status of this socio-cognitive skill within the mammalian clade.
After mammals, birds have been subject to most gaze following studies, especially corvids. This group is generally regarded among the most cognitively complex birds. Within the corvids, the common raven has been most extensively studied. Ravens follow the gaze of a human experimenter into distant space and geometrically around barriers (Bugnyar et al., 2004). While ravens already followed the experimenter’s look-ups as fledglings, GGF occurred only after 6 months. The same developmental trajectory was found in another corvid species, the rook (Corvus frugilegus; Schloegl et al., 2008b).
Both studies provided similar explanations for the development of gaze following skills, namely different ecological valences of the two modes of gaze following. Scanning the sky might serve as anti-predatory response and would therefore be a crucial cue already for fledglings. Looking around barriers, on the other hand, might serve as a cue to food sources, which is not important to fledglings due to parental care. Moreover, the emergence of GGF coincides with the time when ravens first start hiding behind barriers to conceal their caching (Bugnyar et al., 2007), indicating a developmental milestone in the understanding of visual perspectives.
The same developmental pattern has been found in primates and other mammals, where GFD emerges early in the development (e.g., rhesus macaques: Ferrari et al., 2000; chimpanzees: Tomasello et al., 2001; wolves: Range and Virányi, 2011), and GGF develops significantly later (e.g., human infants: Scaife and Bruner, 1975; chimpanzees: Okamoto et al., 2004; wolves: Range and Virányi, 2011). A more likely explanation, thus, is that the two modes of gaze following require different cognitive processes that develop at different times. The gap in the development of the two modes indicates more complex cognitive processes involved in GGF that the early developing brain is not yet capable of. Gaze following skills appear to develop at comparable rates and in the same pattern in birds and mammals, even though their brain morphologies differ drastically.
Ravens were moreover found to habituate quickly to look-ups, but not to geometrical visual cues of an experimenter (Bugnyar et al., 2004; Schloegl et al., 2007). To solve the habituation problem, Schloegl et al. (2007) introduced a new experimenter when the ravens stopped reacting to gaze cues of the familiar experimenter. This increased gaze following responses, though the subjects did not respond as strongly as in initial demonstrations. This increase, however, subsided quickly, indicating a rapid generalization between experimenters. The authors explained the lack of habituation in the geometrical experiment by the natural tendency of ravens to cache food. When tracking gaze behind a barrier and not finding an interesting object there, ravens might expect the object to be hidden and that a continuous search could be advantageous. As a comparison, chimpanzees do not habituate to gaze cues without an interesting target until adulthood (Tomasello et al., 2001).
Ravens have also been tested in object choice. Schloegl et al. (2008a) investigated whether ravens can locate a hidden piece of food through a variety of experimenter-given cues. The ravens did not seem to use gaze cues, not even when the experimenter was kneeling closely to the target object while gazing at it. Interestingly, the ravens also did not respond to a conspecific giving gazing cues towards one of two locations. The authors argued that the functional value of GGF is to use visual barriers to cache food outside of view from competitors rather than to locate the caches of conspecifics. While this seems to be a reasonable explanation for the evolution of GGF in ravens, it does not explain its presence in other non-caching birds and mammals.
In a comparative study on caching rooks and non-caching jackdaws (Corvus monedula; Schloegl et al., 2008b), only the rooks followed the gaze of a human experimenter into distant space as well as geometrically. The authors found only weak evidence for gaze following in jackdaws even when using a conspecific demonstrator perhaps due to a higher vigilance in these birds that makes the detection of gaze follows very difficult.
In an object-choice situation, the jackdaws identified the correct food location using cross-distal pointing and alternating gazes of their caretaker. They did not respond to static cues such as static gaze or head direction and did not respond to cues from an unfamiliar human (von Bayern and Emery, 2009). The authors argued that the dynamic nature of the used cues—in contrast to the static cues—conveyed the communicative intent of the gaze. A similar effect has been found in primates when accompanying experimenter-given cues with gestures and behaviors stressing the communicative intent (Call et al., 2000; Hauser and Wood, 2011). Furthermore, these findings suggest that the negative results on gaze following in jackdaws in the above-mentioned study (Schloegl et al., 2008b) were likely due to methodological artefacts.
The ability to use experimenter-given cues in an object-choice task of a fourth species of the corvid family—Clark’s nutcracker (Nucifraga columbiana)—has been examined (Tornick et al., 2011). These birds are non-social, in contrast to the other tested corvid species. Most subjects immediately used a touch gesture to identify the location of hidden food, which can be explained by local enhancement. Additionally, the birds successfully learned to use point and gaze cues. The gaze cue consisted of both head and eye direction and was dynamic, i.e., the gaze was alternated between the subject and the goal location. Despite methodological differences in studies, it seems that Clark’s nutcrackers perform comparably to social corvid species. This indicates that the social-nonsocial dichotomy is not sufficient to explain the presence of socio-cognitive skills, as they might either be derived from a social ancestor, or might be advantageous without social living (for a more detailed discussion see: Wilkinson et al., 2010a).
Outside of the corvid family, GFD with a conspecific demonstrator has been found in Greylag geese (Anser anser; Kehmeier et al., 2011), African penguins (Spheniscus demersus; Nawroth et al., 2017) and Northern bald ibises (Geronticus eremita; Loretto et al., 2009). Only three non-corvid bird species have been tested in GGF. European starlings (Butler and Fernández-Juricic, 2014) and red junglefowl (Gallus gallus; Zeiträg et al., 2022) successfully tracked the gaze of a conspecific around a barrier, while Northern bald ibises (Loretto et al., 2009) failed to follow a conspecific’s gaze geometrically. However, due to the many positive accounts of GGF in other bird species, it is possible that this negative account of GGF is caused by experimental artefacts.
A recent study (Zeiträg et al., 2022) reported the first accounts of gaze following in palaeognath birds. These birds are the less neurocognitively derived of the two major bird clades—palaeognaths and neognaths. They have retained many ancestral features from the non-avian dinosaurs (for a more detailed discussion see below). In this study, the authors found that three palaeognath species—greater rheas (Rhea americana), emus (Dromaius novaehollandiae), and elegant crested tinamous (Eudromia elegans) were capable of GFD– both up and to the side—as well as GGF.
The only non-corvid bird species tested in an object-choice experiment is the African gray parrot (Psittacus erithacus; Giret et al., 2009). The experimenter-given cues in this study included different pointing cues and distal and proximal gaze cues. Only one parrot spontaneously used a combination of proximal sustained pointing and gazing, a second one was able to learn to use the same gesture. Gaze cues alone were insufficient for any subject to locate the food.
There are very few studies on gaze following in reptiles, likely because they are considered non-social and thus unsuitable subjects to study social cognition. However, two studies have shown that non-social reptile species have socio-cognitive skills such as social learning (Wilkinson et al., 2010a; Kis et al., 2015). Studying reptiles is crucial for understanding the evolution of social cognition in Sauropsida, the clade containing reptiles and birds. Many studies have focused on the cognitive skills of mammals and birds. However, when trying to make inferences about the emergence of cognitive traits—in particular in birds, data from reptiles is needed (Matsubara et al., 2017).
Wilkinson et al. (2010b) conducted the first study on gaze following in a reptile—the red-footed tortoise (Geochelone carbonaria). They showed that this species co-orients with a conspecific’s upward gaze. Since then, three more reptilian species have been found capable of GFD: bearded dragons (Pogona vitticeps; Siviter et al., 2017), leopard geckos (Eublepharis macularius; Simpson and O’Hara, 2019), and American alligators (Alligator mississippiensis; Zeiträg et al., 2022).
Only two of these studies have additionally investigated GGF. Evidence for GGF was neither found in bearded dragons (Siviter et al., 2017) nor American alligators (Zeiträg et al., 2022). Though only few studies on reptiles exist, the large phylogenetic difference between the tested species indicates that GFD is present in distantly related reptilian radiations. The absence of GGF could be a result of the limited number of studies, or of an actual absence of this skill in reptiles. The brains of mammals and birds have, compared to reptiles, substantially more neurons in their telencephalon and cerebellum—regions commonly associated with higher cognitive capacities (Kverková et al., 2022). This neuroanatomical difference could explain the absence of GGF in reptiles. However, more studies are needed to verify the absence of this skill in reptiles.
To understand the evolutionary roots of gaze following, data from distantly related animal taxa capable of using gazes of others is needed. However, several taxa are either understudied or have not been investigated at all. No studies on amphibians exist, and research on reptilians has just started to gain more attention.
One recent study investigated the use of attentional cues in archerfish (Leadner et al., 2021). These fish spit water jets at insects above the surface. The subjects in the study were trained to spit water at a target on a computer monitor above their tank. In the test, the fish were confronted with the picture of a conspecific on the screen, oriented toward the right or left. After that, a target appeared on the left or right of the screen, half of the time congruent with the side indicated by the fish on screen. Archerfish were quicker to spit water at the target when it aligned with the demonstrator’s orientation. However, fish cannot turn their heads independently of their body. Therefore, the cue was a full-body orientation, so it is not possible to discern which part of the cue conveyed the direction of attention.
Interestingly, the authors reported an absence of inhibition of return (IOR) in archerfish. IOR describes the inhibition of returning attention toward a location that has already been observed after shifting attention elsewhere as a result of peripheral cues (McKee et al., 2007). When following gaze cues though, the IOR is absent in humans as well as the studied archerfish. The authors argued that archerfish, just like humans, might possess neural substrates specialized in processing social cues.
Whether the described co-orienting behavior is a special adaptation of archerfish and their hunting style, or a skill shared among fish species in unknown. As described above, all vertebrates share an evolutionary old subcortical pathway that mediates fast, reflexive shifts in visual attention. More studies on fish and amphibian species are needed to verify whether the presence of this pathway is sufficient for the presence of gaze following in all vertebrates.
What animals actually understand about the gaze of others has been debated since Povinelli and Eddy (1996a) first introduced the low-and high-level explanation of gaze following (see above). While GGF can be interpreted as an understanding of the referential nature of gaze, very few studies have looked closer into social predictions that animals form based on observed gaze.
In this context, checking back (also called double looks) is of special interest. Checking back was first described when human children were found to look back to an experimenter in the absence of a target in their line of sight (e.g., Scaife and Bruner, 1975; Butterworth and Cochran, 1980). Children start this gaze alternation at 8 months, comparably late in contrast to the early onset of visual co-orientation at 2 months (Scaife and Bruner, 1975). Developmental psychologists have interpreted this behavior as a sign of an understanding of the mental aspects of gaze, i.e., that gazing refers to a target in the environment. Through alternating gazes between the gazer and the location they have oriented their gaze towards, infants try to identify the correct gaze target. It has been reported that babies point at a target and then turn back to the gazer as if to confirm its correctness (Butterworth and Cochran, 1980).
In animals, this behavior has first been described in chimpanzees by Call et al. (1998) as the animal looking back to the experimenter in the absence of interesting objects in their line of sight. Since the first description of checking back in chimpanzees, it has been reported in other great ape species like bonobos (Pan paniscus), gorillas (Gorilla gorilla) and orangutans (Pongo pygmaeus; Bräuer et al., 2005; Okamoto-Barth et al., 2007), pileated gibbons (Hylobates pileatus; Horton and Caldwell, 2006) and Old World monkeys—Diana monkeys (Scerif et al., 2004), and long-tailed macaques (Goossens et al., 2008). Interestingly, though specifically studied, no evidence for checking back was found in two species of New World monkey—spider monkeys (Ateles geoffroyi) and capuchin monkeys (Amici et al., 2009).
Bräuer et al. (2005) found a comparable ontogenetic trajectory of checking back in non-human primates and human infants. All five species of great ape checked back at comparable rates, but age had a significant effect on the number of checking back instances. The behavior was absent in infants, was first observed in juveniles, and occurred most often in adults.
In line with the hypothesis that checking back shows an expectancy violation when the demonstrator’s gaze is not referring to a target in the environment, pileated gibbons were found to check back more when a target appeared in a location that was incongruent with the location indicated through the gaze direction of a human experimenter or the photograph of a conspecific (Horton and Caldwell, 2006). The same was found in Diana monkeys (Scerif et al., 2004). Long-tailed macaques checked back more often in gaze shifts accompanied by social facial expressions, indicating an overall heightened attention in socially relevant situations (Goossens et al., 2008).
However, the mentalistic interpretation of checking back in infants has received criticism. Corkum and Moore (1995) for example argued that young children only look back at adults to confirm their attention or because they have expectations of the gazer’s behavior in the current situation. In an experimental set-up such an expectation could be that the experimenter will orient their gaze towards a new location after a brief break. Looking back at the experimenter could therefore be a sign of expecting a new gaze cue.
Call et al. (1998) argued in their study on chimpanzees that their subjects might have just returned to their neutral forward orientation. Finding the experimenter still gazing towards a location might have triggered a second, independent co-orientation. However, Bräuer et al. (2005) ruled this alternative explanation out by observing that checking back increased with age, indicating a learning process over time, from a simple co-orienting reflex in infants and juveniles to a perspective-taking model in adults.
To experimentally test the function of checking back, Okamoto-Barth et al. (2007) investigated great apes’ checking back behaviors in a “meaningful” and a “meaningless” condition. In both conditions, the experimenter was looking towards a target. In the meaningful condition, the experimenter’s line of sight was blocked by an opaque barrier. As there was nothing of interest to be seen when following the experimenter’s gaze, the authors hypothesized that apes will be more likely to look back at the experimenter in this condition. In the meaningless condition, the barrier between experimenter and target had a window, so that the experimenter and the subject could see the target. In this condition, the authors expected less checking back behavior as following the experimenter’s gaze would lead the apes to discover the target. The hypotheses were confirmed, as the chimpanzees and bonobos checked back more often in the meaningful condition. Orangutans and gorillas on the other hand seemed insensitive to the differences in the barrier conditions, producing checking back behaviors in both. This insensitivity indicates that the occurrence of checking back alone might not be sufficient to show understanding of visual perspectives and the referential nature of gaze.
Perhaps surprisingly, a recent study discovered checking back in three species of palaeognath and one species of neognath birds (Zeiträg et al., 2022). This was the first-ever description of checking back in any bird species, while no such behavior was found in alligators. The discrepancy between the two is likely caused by differences in their neuroanatomy. Birds have significantly more neurons in their brains than crocodylians and non-avian reptiles in general. However, proportionally, the biggest increase of neuronal numbers is accounted for by the cerebellum (Kverková et al., 2022). The higher neuronal numbers in the cerebellum of birds could explain the presence of checking back behaviors as this structure is believed to be involved in the formation of so-called internal forward models. These models are top-down processes using prior information to predict actions and others’ behaviors (Wolpert et al., 1998; Wolpert and Flanagan, 2001; Bastian, 2006; Roth et al., 2013). The model is updated in case of a mismatch between the prediction and sensorimotor feedback. Checking back could thus firstly be diagnostic of the violation of a social prediction, and secondly represent an attempt to update the model by retracking the gaze direction. These novel results indicate that the increased number of cerebellar neurons of birds likely allow for the formation of more stable internal forward models and the connected social predictions compared to other reptiles.
The ability to visually co-orient with the gaze direction of others has been found in distantly related taxa, suggesting roots in deep evolutionary time. The origin of this skill, however, remains elusive. Perhaps, GFD evolved before vertebrates became land dwellers, or maybe shortly after. The lack of studies on non-amniotes, such as amphibians and fishes, makes it difficult to pinpoint the emergence of this skill. What speaks for a very old origin, is the conserved subcortical pathway in the vertebrate brain, involved in fast, reflexive co-orientation responses to the gaze of others. At least one fish species appears sensitive to body orientations of others, though this could be an adaptation to the species’ hunting style (see above). Based on evidence for GFD from all tested amniotes (mammals, reptiles, and birds), it is likely that this skill was present in the stem amniote, about 325 million years ago.
The ability for GGF, on the other hand, appears to have evolved in parallel, or convergently, in Synapsida (the lineage including the mammals) and Sauropsida (the lineage including the reptiles and birds), as this skill has to date only been found in mammals and birds. Decades of research in this area seem to have confirmed that GFD and GGF represent two distinct skills, as already suggested by Povinelli and Eddy (1996a). This implies that GGF relies on more complex, and hence later evolved, neurocognitive structures.
Two lines of evidence support this assumption. Firstly, in all species where the ontogeny of GGF has been studied, its onset clearly succeeds the development of GFD. Secondly, and perhaps more importantly, the two lineages exhibiting GGF skills, mammals and birds, have over time drastically increased their total and relative brain sizes, as well as their neuronal numbers. This disproportionally large increase left them with significantly more neurons relative to body size than reptiles. The heightened computational power connected to more neurons in the brain might equip mammals and birds with the capacity for visual perspective taking, while the lower neuronal numbers of reptiles do not allow for sophisticated visual socio-cognitive skills. However, more studies are needed to verify the absence of GGF in reptiles and to better understand the correlational relationship between neuronal numbers and GGF.
It is still unclear when GGF arose in the two different lineages. Mammals are the last extant representatives of the Synapsida. Thus, any comparisons with animal groups outside mammals, but within the synapsids, are not possible. However, within mammals, monotremes and marsupials have to our knowledge not been tested in gaze following. Monotremes are egg-laying mammals that diverged long before marsupial and placental mammals and are as close as we can get to the earliest mammals today. Marsupials are more derived, but their brains have retained more ancestral features compared to placental mammals (Ashwell, 2010; Álvarez-Carretero et al., 2022; Flannery et al., 2022; Kverková et al., 2022). Studies on these neurocognitively distinct groups would substantially support our understanding of the timing of the emergence of GGF in mammals, or to show that it might have even evolved before mammals.
In Sauropsida the picture is somewhat clearer. As GGF is not found in reptiles, it likely evolved in the dinosaur lineage. At least it seems to have existed in the first birds around 150 million years ago. But it is not unlikely that GGF existed in non-avian dinosaur taxa. The Maniraptora is the group of theropod dinosaurs from which the birds derived, and its members show overlapping traits with birds, in particular with palaeognaths. Their brains had morphologies comparable to modern palaeognaths (Balanoff et al., 2014). Moreover, they had comparable scaling relationships of body and brain size (Ksepka et al., 2020). Even some social behaviors connected to reproductive strategies were similar, such as the parental care system, where the male incubates the eggs from several females and provides care of the chicks (Varricchio et al., 2008; Varricchio and Jackson, 2016). That said, GGF might have been present even deeper into the non-avian dinosaurs. However, to better understand its origin, more studies on the neurocognition of GGF in birds are needed. Finally, more palaeontological neuroanatomy studies will help shed light on the evolution of GGF.
Gaze following—with its different levels—appears to be an important foundation for social cognition. This can, for example, be seen in the crucial role it plays for a developing human mind. Without gaze following a wealth of information is lost, and the opportunities to evolve essential skills, such as perspective taking and social predictions, are hampered. Considering the likely cardinal function this fundamental and underlying social behavior has, it is surprisingly understudied from an evolutionary perspective.
CZ and TJ collected publications relevant for this review. CZ wrote the manuscript with extensive help of TJ and MO. All authors contributed to the article and approved the submitted version.
This work was supported by Swedish Research Council grants 2021-02973 and 2019-03265, and the LMK Foundation. Open Access publishing fees have been covered by Lund University's APC fund.
We thank Steve Brusatte for his comments on the manuscript.
The authors declare that the research was conducted in the absence of any commercial or financial relationships that could be construed as a potential conflict of interest.
All claims expressed in this article are solely those of the authors and do not necessarily represent those of their affiliated organizations, or those of the publisher, the editors and the reviewers. Any product that may be evaluated in this article, or claim that may be made by its manufacturer, is not guaranteed or endorsed by the publisher.
Agnetta, B., Hare, B., and Tomasello, M. (2000). Cues to food location that domestic dogs (Canis familiaris) of different ages do and do not use. Anim. Cogn. 3, 107–112. doi: 10.1007/s100710000070
Álvarez-Carretero, S., Tamuri, A. U., Battini, M., Nascimento, F. F., Carlisle, E., Asher, R. J., et al. (2022). A species-level timeline of mammal evolution integrating phylogenomic data. Nature 602, 263–267. doi: 10.1038/s41586-021-04341-1
Alwall, N., Johansson, D., and Hansen, S. (2010). The gender difference in gaze-cueing: associations with empathizing and systemizing. Personal. Individ. Differ. 49, 729–732. doi: 10.1016/j.paid.2010.06.016
Amici, F., Aureli, F., Visalberghi, E., and Call, J. (2009). Spider monkeys (Ateles geoffroyi) and capuchin monkeys (Cebus apella) follow gaze around barriers: evidence for perspective taking? J. Comp. Psychol. 123, 368–374. doi: 10.1037/a0017079
Anderson, J. R., Kuroshima, H., Hattori, Y., and Fujita, K. (2005). Attention to combined attention in new world monkeys (Cebus apella, Saimiri sciureus). J. Comp. Psychol. 119, 461–464. doi: 10.1037/0735-7036.119.4.461
Anderson, J. R., and Mitchell, R. W. (1999). Macaques but not lemurs co-orient visually with humans. Folia Primatol. 70, 17–22. doi: 10.1159/000021670
Anderson, J. R., Montant, M., and Schmitt, D. (1996). Rhesus monkeys fail to use gaze direction as an experimenter-given cue in an object-choice task. Behav. Process. 37, 47–55. doi: 10.1016/0376-6357(95)00074-7
Anderson, J. R., Sallaberry, P., and Barbier, H. (1995). Use of experimenter-given cues during object-choice tasks by capuchin monkeys. Anim. Behav. 49, 201–208. doi: 10.1016/0003-3472(95)80168-5
Ashwell, K. (2010). The Neurobiology of Australian Marsupials: Brain Evolution in the Other Mammalian Radiation. Cambridge: Cambridge University Press.
Baba, C., Kawai, M., and Takimoto-Inose, A. (2019). Are horses (Equus caballus) sensitive to human emotional cues? Animals 9:630. doi: 10.3390/ani9090630
Balanoff, A. M., Bever, G. S., and Norell, M. A. (2014). Reconsidering the avian nature of the oviraptorosaur brain (Dinosauria: Theropoda). PLoS One 9:e113559. doi: 10.1371/journal.pone.0113559
Baldwin, D. A. (1991). Infants' contribution to the achievement of joint reference. Child Dev. 62, 875–890. doi: 10.2307/1131140
Barth, J., Reaux, J. E., and Povinelli, D. J. (2005). Chimpanzees’ (pan troglodytes) use of gaze cues in object-choice tasks: different methods yield different results. Anim. Cogn. 8, 84–92. doi: 10.1007/s10071-004-0235-x
Bastian, A. J. (2006). Learning to predict the future: the cerebellum adapts feedforward movement control. Curr. Opin. Neurobiol. 16, 645–649. doi: 10.1016/j.conb.2006.08.016
Batki, A., Baron-Cohen, S., Wheelwright, S., Connellan, J., and Ahluwalia, J. (2000). Is there an innate gaze module? Evidence from human neonates. Infant Behav. Dev. 23, 223–229. doi: 10.1016/S0163-6383(01)00037-6
Bayliss, A. P., Di Pellegrino, G., and Tipper, S. P. (2005). Sex differences in eye gaze and symbolic cueing of attention. Q. J. Exp. Psychol. A 58, 631–650. doi: 10.1080/02724980443000124
Bettle, R., and Rosati, A. G. (2019). Flexible gaze-following in rhesus monkeys. Anim. Cogn. 22, 673–686. doi: 10.1007/s10071-019-01263-4
Bräuer, J., Call, J., and Tomasello, M. (2005). All great ape species follow gaze to distant locations and around barriers. J. Comp. Psychol. 119, 145–154. doi: 10.1037/0735-7036.119.2.145
Bräuer, J., Kaminski, J., Riedel, J., Call, J., and Tomasello, M. (2006). Making inferences about the location of hidden food: social dog, causal ape. J. Comp. Psychol. 120, 38–47. doi: 10.1037/0735-7036.120.1.38
Brooks, R., and Meltzoff, A. N. (2005). The development of gaze following and its relation to language. Dev. Sci. 8, 535–543. doi: 10.1111/j.1467-7687.2005.00445.x
Brooks, R., and Meltzoff, A. N. (2015). Connecting the dots from infancy to childhood: a longitudinal study connecting gaze following, language, and explicit theory of mind. J. Exp. Child Psychol. 130, 67–78. doi: 10.1016/j.jecp.2014.09.010
Bugnyar, T. (2011). Knower-guesser differentiation in ravens: others' viewpoints matter. Proc. R. Soc. B Biol. Sci. 278, 634–640. doi: 10.1098/rspb.2010.1514
Bugnyar, T., Reber, S. A., and Buckner, C. (2016). Ravens attribute visual access to unseen competitors. Nat. Commun. 7. doi: 10.1038/ncomms1050
Bugnyar, T., Stoewe, M., and Heinrich, B. (2007). The ontogeny of caching in ravens, Corvus corax. Animal Behav. 74, 757–767. doi: 10.1016/j.anbehav.2006.08.019
Bugnyar, T., Stöwe, M., and Heinrich, B. (2004). Ravens, Corvus corax, follow gaze direction of humans around obstacles. Proc. R. Soc. B Biol. Sci. 271, 1331–1336. doi: 10.1098/rspb.2004.2738
Burkart, J., and Heschl, A. (2006). Geometrical gaze following in common marmosets (Callithrix jacchus). J. Comp. Psychol. 120, 120–130. doi: 10.1037/0735-7036.120.2.120
Burkart, J. M., and Heschl, A. (2007). Understanding visual access in common marmosets, Callithrix jacchus: perspective taking or behaviour reading? Anim. Behav. 73, 457–469. doi: 10.1016/j.anbehav.2006.05.019
Butler, S. R., and Fernández-Juricic, E. (2014). European starlings recognize the location of robotic conspecific attention. Biol. Lett. 10:20140665. doi: 10.1098/rsbl.2014.0665
Butterworth, G., and Cochran, E. (1980). Towards a mechanism of joint visual attention in human infancy. Int. J. Behav. Dev. 3, 253–272. doi: 10.1177/016502548000300303
Butterworth, G., and Jarrett, N. (1991). What minds have in common is space: spatial mechanisms serving joint visual attention in infancy. Br. J. Dev. Psychol. 9, 55–72. doi: 10.1111/j.2044-835X.1991.tb00862.x
Byrne, R., Mendl, M., Devereux, C., and Held, S. (2001). Behaviour of domestic pigs in a visual perspective taking task. Behaviour 138, 1337–1354. doi: 10.1163/156853901317367627
Byrnit, J. T. (2004). Nonenculturated orangutans' (Pongo pygmaeus) use of experimenter-given manual and facial cues in an object-choice task. J. Comp. Psychol. 118, 309–315. doi: 10.1037/0735-7036.118.3.309
Byrnit, J. T. (2009). Gorillas' (Gorilla gorilla) use of experimenter-given manual and facial cues in an object-choice task. Animal Cognit 12, 401–404. doi: 10.1007/s10071-008-9600200-1
Call, J., Agnetta, B., and Tomasello, M. (2000). Cues that chimpanzees do and do not use to find hidden objects. Anim. Cogn. 3, 23–34. doi: 10.1007/s100710050047
Call, J., Hare, B. A., and Tomasello, M. (1998). Chimpanzee gaze following in an object-choice task. Anim. Cogn. 1, 89–99. doi: 10.1007/s100710050013
Carpenter, M., Nagell, K., Tomasello, M., Butterworth, G., and Moore, C. (1998). Social cognition, joint attention, and communicative competence from 9 to 15 months of age. Monogr. Soc. Res. Child Dev. 63:i. doi: 10.2307/1166214
Catala, A., Mang, B., Wallis, L., and Huber, L. (2017). Dogs demonstrate perspective taking based on geometrical gaze following in a guesser-knower task. Anim. Cogn. 20, 581–589. doi: 10.1007/s10071-017-1082-x
Chen, T., Gao, J., Tan, J., Tao, R., and Su, Y. (2017). Variation in gaze-following between two Asian 970 colobine monkeys. Primates 58, 525–534. doi: 10.1007/s10329-017-0612-0
Clark, H., and Leavens, D. A. (2021). The performance of domestic dogs (Canis lupus familiaris) on 975 two versions of the object choice task. Animal Cognit. 24, 1087–1098. doi: 10.1007/s10071-021-01500-9
Corkum, V., and Moore, C. (1995). “The development of joint attention in infants” in Joint Attention: Its Origins and Role in Development. eds. C. Moore and P. J. Dunham (Hillsdale, NJ: Lawrence Erlbraum Associates), 61–83.
Csibra, G., and Volein, A. (2008). Infants can infer the presence of hidden objects from referential gaze information. Br. J. Dev. Psychol. 26, 1–11. doi: 10.1348/026151007X185987
Dawkins, M. S. (2002). What are birds looking at? Head movements and eye use in chickens. Anim. Behav. 63, 991–998. doi: 10.1006/anbe.2002.1999
Deaner, R. O., and Platt, M. L. (2003). Reflexive social attention in monkeys and humans. Curr. Biol. 13, 1609–1613. doi: 10.1016/j.cub.2003.08.025
Defolie, C., Malassis, R., Serre, M., and Meunier, H. (2015). Tufted capuchins (Cebus apella) adapt their communicative behaviour to human’s attentional states. Anim. Cogn. 18, 747–755. doi: 10.1007/s10071-015-0841-9
Del Bianco, T., Falck-Ytter, T., Thorup, E., and Gredebäck, G. (2019). The developmental origins of gaze-following in human infants. Infancy 24, 433–454. doi: 10.1111/infa.12276
Downing, P. E., Jiang, Y., Shuman, M., and Kanwisher, N. (2001). A cortical area selective for visual processing of the human body. Science 293, 2470–2473. doi: 10.1126/science.1063414
Duranton, C., Range, F., and Virányi, Z. (2017). Do pet dogs (Canis familiaris) follow ostensive and non-ostensive human gaze to distant space and to objects? R. Soc. Open Sci. 4:170349. doi: 10.1098/rsos.170349
Emery, N. J. (2000). The eyes have it: the neuroethology, function and evolution of social gaze. Neurosci. Biobehav. Rev. 24, 581–604. doi: 10.1016/S0149-7634(00)00025-7
Emery, N. J., Lorincz, E. N., Perrett, D. I., Oram, M. W., and Baker, C. I. (1997). Gaze following and joint attention in rhesus monkeys (Macaca mulatta). J. Comp. Psychol. 111, 286–293. doi: 10.1037/0735-7036.111.3.286
Farroni, T., Csibra, G., Simion, F., and Johnson, M. H. (2002). Eye contact detection in humans from birth. Proc. Natl. Acad. Sci. 99, 9602–9605. doi: 10.1073/pnas.152159999
Farroni, T., Massaccesi, S., Pividori, D., and Johnson, M. H. (2004). Gaze following in newborns. Infancy 5, 39–60. doi: 10.1207/s15327078in0501_2
Ferrari, P., Kohler, E., Fogassi, L., and Gallese, V. (2000). The ability to follow eye gaze and its emergence during development in macaque monkeys. Proc. Natl. Acad. Sci. 97, 13997–14002. doi: 10.1073/pnas.250241197
Ferrari, P. F., Coudé, G., Gallese, V., and Fogassi, L. (2008). Having access to others’ mind through gaze: the role of ontogenetic and learning processes in gaze-following behavior of macaques. Soc. Neurosci. 3, 239–249. doi: 10.1080/17470910701429065
Flannery, T. F., Rich, T. H., Vickers-Rich, P., Ziegler, T., Veatch, E. G., and Helgen, K. M. (2022). A review of monotreme (Monotremata) evolution. Alcheringa Aust. J. Palaeontol. 46, 3–20. doi: 10.1080/03115518.2022.2025900
Flombaum, J. I., and Santos, L. R. (2005). Rhesus monkeys attribute perceptions to others. Curr. Biol. 15, 447–452. doi: 10.1016/j.cub.2004.12.076
Giret, N., Miklósi, Á., Kreutzer, M., and Bovet, D. (2009). Use of experimenter-given cues by African gray parrots (Psittacus erithacus). Anim. Cogn. 12, 1–10. doi: 10.1007/s10071-008-0163-2
Goossens, B. M., Dekleva, M., Reader, S. M., Sterck, E. H., and Bolhuis, J. J. (2008). Gaze following in monkeys is modulated by observed facial expressions. Anim. Behav. 75, 1673–1681. doi: 10.1016/j.anbehav.2007.10.020
Goossens, B. M., van den Berg, L. M., Reader, S. M., and Sterck, E. H. (2012). An analysis of gaze following to a hidden location in long-tailed macaques (Macaca fascicularis). Behaviour 149, 1319–1337. doi: 10.1163/1568539X-00003017
Gredebäck, G., Astor, K., and Fawcett, C. (2018). Gaze following is not dependent on ostensive cues: a critical test of natural pedagogy. Child Dev. 89, 2091–2098. doi: 10.1111/cdev.13026
Gretscher, H., Haun, D. B., Liebal, K., and Kaminski, J. (2012). Orang-utans rely on orientation cues and egocentric rules when judging others' perspectives in a competitive food task. Anim. Behav. 84, 323–331. doi: 10.1016/j.anbehav.2012.04.021
Hall, K., Oram, M. W., Campbell, M. W., Eppley, T. M., Byrne, R. W., and De Waal, F. B. (2014). Using cross correlations to investigate how chimpanzees (pan troglodytes) use conspecific gaze cues to extract and exploit information in a foraging competition. Am. J. Primatol. 76, 932–941. doi: 10.1002/ajp.22279
Hare, B., Addessi, E., Call, J., Tomasello, M., and Visalberghi, E. (2003). Do capuchin monkeys, Cebus apella, know what conspecifics do and do not see? Anim. Behav. 65, 131–142. doi: 10.1006/anbe.2002.2017
Hare, B., Call, J., Agnetta, B., and Tomasello, M. (2000). Chimpanzees know what conspecifics do and do not see. Anim. Behav. 59, 771–785. doi: 10.1006/anbe.1999.1377
Hare, B., Call, J., and Tomasello, M. (1998). Communication of food location between human and dog (Canis familiaris). Evol. Commun. 2, 137–159. doi: 10.1075/eoc.2.1.06har
Hare, B., Call, J., and Tomasello, M. (2001). Do chimpanzees know what conspecifics know? Anim. Behav. 61, 139–151. doi: 10.1006/anbe.2000.1518
Hare, B., Plyusnina, I., Ignacio, N., Schepina, O., Stepika, A., Wrangham, R., et al. (2005). Social cognitive evolution in captive foxes is a correlated by-product of experimental domestication. Curr. Biol. 15, 226–230. doi: 10.1016/j.cub.2005.01.040
Hare, B., and Tomasello, M. (1999). Domestic dogs (Canis familiaris) use human and conspecific social cues to locate hidden food. J. Comp. Psychol. 113, 173–177. doi: 10.1037/0735-7036.113.2.173
Hare, B., and Tomasello, M. (2004). Chimpanzees are more skilful in competitive than in cooperative cognitive tasks. Anim. Behav. 68, 571–581. doi: 10.1016/j.anbehav.2003.11.011
Harries, M., and Perrett, D. (1991). Visual processing of faces in temporal cortex: physiological evidence for a modular organization and possible anatomical correlates. J. Cogn. Neurosci. 3, 9–24. doi: 10.1162/jocn.1991.3.1.9
Hattori, Y., Kano, F., and Tomonaga, M. (2010). Differential sensitivity to conspecific and allospecific cues in chimpanzees and humans: a comparative eye-tracking study. Biol. Lett. 6, 610–613. doi: 10.1098/rsbl.2010.0120
Hauser, M. D., and Wood, J. N. (2011). Replication of ‘rhesus monkeys correctly read the goal-relevant gestures of a human agent’. Proc. R. Soc. B Biol. Sci. 278, 158–159. doi: 10.1098/rspb.2010.1441
Herrmann, E., Call, J., Hernández-Lloreda, M. V., Hare, B., and Tomasello, M. (2007). Humans have evolved specialized skills of social cognition: the cultural intelligence hypothesis. Science 317, 1360–1366. doi: 10.1126/science.1146282
Herrmann, E., Hare, B., Call, J., and Tomasello, M. (2010). Differences in the cognitive skills of bonobos and chimpanzees. PLOS One 5:e12438. doi: 10.1371/journal.pone.0012438
Horton, K. E., and Caldwell, C. A. (2006). Visual co-orientation and expectations about attentional orientation in pileated gibbons (Hylobates pileatus). Behav. Process. 72, 65–73. doi: 10.1016/j.beproc.2005.12.004
Houston-Price, C., Plunkett, K., and Duffy, H. (2006). The use of social and salience cues in early word learning. J. Exp. Child Psychol. 95, 27–55. doi: 10.1016/j.jecp.2006.03.006
Inoue, Y., Inoue, E., and Itakura, S. (2004). Use of experimenter-given directional cues by a young white-handed gibbon (Hylobates lar). Jpn. Psychol. Res. 46, 262–267. doi: 10.1111/j.1468-5584.2004.00258.x
Ishai, A., Schmidt, C. F., and Boesiger, P. (2005). Face perception is mediated by a distributed cortical network. Brain Res. Bull. 67, 87–93. doi: 10.1016/j.brainresbull.2005.05.027
Ishikawa, M., and Itakura, S. (2019). Physiological arousal predicts gaze following in infants. Proc. R. Soc. B 286:20182746. doi: 10.1098/rspb.2018.2746
Itakura, S. (1996). An exploratory study of gaze-monitoring in nonhuman primates. Japanese Psychol. Res. 38, 174–180. doi: 10.1111/j.1468-5884.1996.tb00022.x
Itakura, S., Agnetta, B., Hare, B., and Tomasello, M. (1999). Chimpanzee use of human and conspecific social cues to locate hidden food. Dev. Sci. 2, 448–456. doi: 10.1111/1467-7687.00089
Itakura, S., and Anderson, J. R. (1996). Learning to use experimenter-given cues during an object choice task by a capuchin monkey. Cogn. Psychol. 15, 103–112.
Itakura, S., and Tanaka, M. (1998). Use of experimenter-given cues during object-choice tasks by chimpanzees (Pan troglodytes), an orangutan (Pongo pygmaeus), and human infants (Homo sapiens). J. Comp. Psychol. 112:119. doi: 10.1037/0735-10997036.112.2.119
Jiang, Y., and He, S. (2006). Cortical responses to invisible faces: dissociating subsystems for facial-information processing. Curr. Biol. 16, 2023–2029. doi: 10.1016/j.cub.2006.08.084
Johnson, M. H. (2005). Subcortical face processing. Nat. Rev. Neurosci. 6, 766–774. doi: 10.1038/nrn1766
Kaminski, J., Riedel, J., Call, J., and Tomasello, M. (2005). Domestic goats, Capra hircus, follow gaze direction and use social cues in an object choice task. Anim. Behav. 69, 11–18. doi: 10.1016/j.anbehav.2004.05.008
Kano, F., and Call, J. (2014). Cross-species variation in gaze following and conspecific preference among great apes, human infants and adults. Anim. Behav. 91, 137–150. doi: 10.1016/j.anbehav.2014.03.011
Kano, F., Kawaguchi, Y., and Yeow, H. (2022). Experimental evidence that uniformly white sclera enhances the visibility of eye-gaze direction in humans and chimpanzees. elife 11:e74086. doi: 10.7554/eLife.74086
Kaplan, G., and Rogers, L. J. (2002). Patterns of gazing in orangutans (Pongo pygmaeus). Int. J. Primatol. 23, 501–526. doi: 10.1023/A:1014913532057
Karin-D'Arcy, R. M., and Povinelli, D. J. (2002). Do chimpanzees know what each other see? A closer look. Int. J. Comp. Psychol. 15.
Kehmeier, S., Schloegl, C., Scheiber, I. B., and Weiß, B. M. (2011). Early development of gaze following into distant space in juvenile Greylag geese (Anser anser). Anim. Cogn. 14, 477–485. doi: 10.1007/s10071-011-0381-x
Kendrick, K. M., da Costa, A. P., Leigh, A. E., Hinton, M. R., and Peirce, J. W. (2001). Sheep don't forget a face. Nature 414, 165–166. doi: 10.1038/35102669
Ketchaisri, O., Siripunkaw, C., and Plotnik, J. M. (2019). The use of a human’s location and social cues by Asian elephants in an object-choice task. Anim. Cogn. 22, 907–915. doi: 10.1007/s10071-019-01283-0
Kis, A., Huber, L., and Wilkinson, A. (2015). Social learning by imitation in a reptile (Pogona vitticeps). Anim. Cogn. 18, 325–331. doi: 10.1007/s10071-014-0803-7
Ksepka, D. T., Balanoff, A. M., Smith, N. A., Bever, G. S., Bhullar, B.-A. S., Bourdon, E., et al. (2020). Tempo and pattern of avian brain size evolution. Curr. Biol. 30:e2023, 2026–2036.e3. doi: 10.1016/j.cub.2020.03.060
Kverková, K., Marhounová, L., Polonyiová, A., Kocourek, M., Zhang, Y., Olkowicz, S., et al. (2022). The evolution of brain neuron numbers in amniotes. Proc. Natl. Acad. Sci. 119:e2121624119. doi: 10.1073/pnas.2121624119
Leadner, K., Sekely, L., Klein, R. M., and Gabay, S. (2021). Evolution of social attentional cues: evidence from the archerfish. Cognition 207:104511. doi: 10.1016/j.cognition.2020.104511
Liebal, K., and Kaminski, J. (2012). Gibbons (Hylobates pileatus, H. moloch, H. lar, Symphalangus syndactylus) follow human gaze, but do not take the visual perspective of others. Anim. Cogn. 15, 1211–1216. doi: 10.1007/s10071-012-0543-5
Loretto, M.-C., Schloegl, C., and Bugnyar, T. (2009). Northern bald ibises follow others’ gaze into distant space but not behind barriers. Biol. Lett. 6, 14–17. doi: 10.1098/rsbl.2009.0510
Lorincz, E. N., Baker, C. I., and Perrett, D. I. (2000). “Visual cues for attention following in rhesus monkeys” in Picture Perception in Animals. ed. J. Fagot (Cambridge: Cambridge University Press), 343–372.
Maginnity, M. E., and Grace, R. C. (2014). Visual perspective taking by dogs (Canis familiaris) in a guesser-knower task: evidence for a canine theory of mind? Anim. Cogn. 17, 1375–1392. doi: 10.1007/s10071-014-0773-9
Matsubara, S., Deeming, D. C., and Wilkinson, A. (2017). Cold-blooded cognition: new directions in reptile cognition. Curr. Opin. Behav. Sci. 16, 126–130. doi: 10.1016/j.cobeha.2017.06.006
Matsunaka, R., and Hiraki, K. (2014). Fearful gaze cueing: gaze direction and facial expression independently influence overt orienting responses in 12-month-olds. PLoS One 9:e89567. doi: 10.1371/journal.pone.0089567
McKee, D., Christie, J., and Klein, R. (2007). On the uniqueness of attentional capture by uninformative gaze cues: facilitation interacts with the Simon effect and is rarely followed by IOR. Can. J. Exp. Psychol./Revue canadienne de psychologie expérimentale 61, 293–303. doi: 10.1037/cjep2007029
McKinley, J., and Sambrook, T. D. (2000). Use of human-given cues by domestic dogs (Canis familiaris) and horses (Equus caballus). Anim. Cogn. 3, 13–22. doi: 10.1007/s100710050046
Melis, A. P., Call, J., and Tomasello, M. (2006). Chimpanzees (pan troglodytes) conceal visual and auditory information from others. J. Comp. Psychol. 120, 154–162. doi: 10.1037/0735-7036.120.2.154
Met, A., Miklosi, A., and Lakatos, G. (2014). Gaze-following behind barriers in domestic dogs. Anim. Cogn. 17, 1401–1405. doi: 10.1007/s10071-014-0754-z
Micheletta, J., and Waller, B. M. (2012). Friendship affects gaze following in a tolerant species of macaque, Macaca nigra. Anim. Behav. 83, 459–467. doi: 10.1016/j.anbehav.2011.11.018
Miklósi, Á., Polgárdi, R., Topál, J., and Csányi, V. (1998). Use of experimenter-given cues in dogs. Anim. Cogn. 1, 113–121. doi: 10.1007/s100710050016
Moore, C. (2008). The development of gaze following. Child Dev. Perspect. 2, 66–70. doi: 10.1111/j.1750-8606.2008.00052.x
Morand-Ferron, J., Doligez, B., Dall, S., and Reader, S. (2010). Social information use. Encyclopedia Anim. Behav. 3, 242–250. doi: 10.1016/B978-0-08-045337-8.00281-3
Morris, J. S., Öhman, A., and Dolan, R. J. (1999). A subcortical pathway to the right amygdala mediating “unseen” fear. Proc. Natl. Acad. Sci. 96, 1680–1685. doi: 10.1073/pnas.96.4.1680
Mulcahy, N. J., and Call, J. (2009). The performance of bonobos (Pan paniscus), chimpanzees (Pan troglodytes), and orangutans (Pongo pygmaeus) in two versions of an object-choice task. J. Comparat. Psychol. 123, 304–309. doi: 10.1037/a0016222
Nawroth, C., Ebersbach, M., and von Borell, E. (2014). Juvenile domestic pigs (Sus scrofa domestica) use human-given cues in an object choice task. Anim. Cogn. 17, 701–713. doi: 10.1007/s10071-013-0702-3
Nawroth, C., Trincas, E., and Favaro, L. (2017). African penguins follow the gaze direction of conspecifics. PeerJ 5:e3459. doi: 10.7717/peerj.3459
Nawroth, C., von Borell, E., and Langbein, J. (2015). ‘Goats that stare at men’: dwarf goats alter their behaviour in response to human head orientation, but do not spontaneously use head direction as a cue in a food-related context. Anim. Cogn. 18, 65–73. doi: 10.1007/s10071-014-0777-5
Neiworth, J. J., Burman, M. A., Basile, B. M., and Lickteig, M. T. (2002). Use of experimenter-given cues in visual co-orienting and in an object-choice task by a New World monkey species, cotton top tamarins (Saguinus oedipus). J. Comp. Psychol. 116, 3–11. doi: 10.1037/0735-7036.116.1.3
Okamoto, S., Tanaka, M., and Tomonaga, M. (2004). Looking back: the “representational mechanism” of joint attention in an infant chimpanzee (pan troglodytes). Jpn. Psychol. Res. 46, 236–245. doi: 10.1111/j.1468-5584.2004.00255.x
Okamoto, S., Tomonaga, M., Ishii, K., Kawai, N., Tanaka, M., and Matsuzawa, T. (2002). An infant chimpanzee (Pan troglodytes) follows human gaze. Animal Cognition 5, 107–114. doi: 10.1007/s10071-002-0133-z
Okamoto-Barth, S., Call, J., and Tomasello, M. (2007). Great apes' understanding of other individuals' line of sight. Psychol. Sci. 18, 462–468. doi: 10.1111/j.1467-9280.2007.01922.x
Overduin-de Vries, A., Spruijt, B., and Sterck, E. (2014). Long-tailed macaques (Macaca fascicularis) understand what conspecifics can see in a competitive situation. Anim. Cogn. 17, 77–84. doi: 10.1007/s10071-013-0639-6
Pack, A. A., and Herman, L. M. (2004). Bottlenosed dolphins (Tursiops truncatus) comprehend the referent of both static and dynamic human gazing and pointing in an object-choice task. J. Comp. Psychol. 118, 160–171. doi: 10.1037/0735-7036.118.2.160
Parron, C., and Meguerditchian, A. (2016). Gaze following in baboons (Papio anubis): juveniles adjust their gaze and body position to human's head redirections. Am. J. Primatol. 78, 1265–1271. doi: 10.1002/ajp.22580
Paukner, A., Anderson, J. R., Fogassi, L., and Ferrari, P. F. (2007). Do facial gestures, visibility or speed of movement influence gaze following responses in pigtail macaques? Primates 48, 241–244. doi: 10.1007/s10329-006-0024-z
Pecchinenda, A., Pes, M., Ferlazzo, F., and Zoccolotti, P. (2008). The combined effect of gaze direction and facial expression on cueing spatial attention. Emotion 8, 628–634. doi: 10.1037/a0013437
Peignot, P., and Anderson, J. R. (1999). Use of experimenter-given manual and facial cues by gorillas (Gorilla gorilla) in an object-choice task. J. Comp. Psychol. 113, 253–260. doi: 10.1037/0735-7036.113.3.253
Perea-García, J. O., Kret, M. E., Monteiro, A., and Hobaiter, C. (2019). Scleral pigmentation leads to conspicuous, not cryptic, eye morphology in chimpanzees. Proc. Natl. Acad. Sci. 116, 19248–19250. doi: 10.1073/pnas.1911410116
Perra, O., and Gattis, M. (2010). The control of social attention from 1 to 4 months. Br. J. Dev. Psychol. 28, 891–908. doi: 10.1348/026151010X487014
Perrett, D. I., Rolls, E. T., and Caan, W. (1982). Visual neurones responsive to faces in the monkey temporal cortex. Exp. Brain Res. 47, 329–342. doi: 10.1007/BF00239352
Perrett, D. I., Smith, P., Potter, D., Mistlin, A., Head, A., Milner, A. D., et al. (1985). Visual cells in the temporal cortex sensitive to face view and gaze direction. Proc. Royal Soc. London Ser. B. Biol. Sci. 223, 293–317. doi: 10.1098/rspb.1985.0003
Pongrácz, P., Szapu, J. S., and Faragó, T. (2019). Cats (Felis silvestris catus) read human gaze for referential information. Intelligence 74, 43–52. doi: 10.1016/j.intell.2018.11.001
Povinelli, D. J., and Eddy, T. J. (1996a). Chimpanzees: joint visual attention. Psychol. Sci. 7, 129–135. doi: 10.1111/j.1467-9280.1996.tb00345.x
Povinelli, D. J., and Eddy, T. J. (1996b). Factors influencing young chimpanzees' (Pan troglodytes) recognition of attention. J. Comparat. Psychol. 110, 336–345. doi: 10.1037/0735-7036.110.4.336
Povinelli, D. J., and Eddy, T. J. (1997). Specificity of gaze‐following in young chimpanzees. Br J Dev Psychol 15, 213–222. doi: 10.1111/j.2044-1245835X.1997.tb00735.x
Proops, L., and McComb, K. (2010). Attributing attention: the use of human-given cues by domestic horses (Equus caballus). Anim. Cogn. 13, 197–205. doi: 10.1007/s10071-009-0257-5
Proops, L., Walton, M., and McComb, K. (2010). The use of human-given cues by domestic horses, Equus caballus, during an object choice task. Anim. Behav. 79, 1205–1209. doi: 10.1007/s10071-009-0257-5
Putnam, P., Roman, J., Zimmerman, P., and Gothard, K. M. (2016). Oxytocin enhances gaze-following responses to videos of natural social behavior in adult male rhesus monkeys. Psychoneuroendocrinology 72, 47–53. doi: 10.1016/j.psyneuen.2016.05.016
Range, F., and Virányi, Z. (2011). Development of gaze following abilities in wolves (Canis lupus). PLoS One 6:e16888. doi: 10.1371/journal.pone.0016888
Ringhofer, M., Trösch, M., Lansade, L., and Yamamoto, S. (2021). Horses with sustained attention follow the pointing of a human who knows where food is hidden. Sci. Rep. 11:16184. doi: 10.1038/s41598-021-95727-8
Rizzolatti, G., Fabbri-Destro, M., and Cattaneo, L. (2009). Mirror neurons and their clinical relevance. Nat. Clin. Pract. Neurol. 5, 24–34. doi: 10.1038/ncpneuro0990
Rosa, M. G., and Tweedale, R. (2005). Brain maps, great and small: lessons from comparative studies of primate visual cortical organization. Phil. Trans. R. Soc B Biol. Sci. 360, 665–691. doi: 10.1098/rstb.2005.1626
Rosati, A. G., Arre, A. M., Platt, M. L., and Santos, L. R. (2016). Rhesus monkeys show human-like changes in gaze following across the lifespan. Proc. R. Soc. B Biol. Sci. 283:20160376. doi: 10.1098/rspb.2016.0376
Rosati, A. G., and Hare, B. (2009). Looking past the model species: diversity in gaze-following skills across primates. Curr. Opin. Neurobiol. 19, 45–51. doi: 10.1016/j.conb.2009.03.002
Rosati, A. G., and Santos, L. R. (2017). Tolerant Barbary macaques maintain juvenile levels of social attention in old age, but despotic rhesus macaques do not. Anim. Behav. 130, 199–207. doi: 10.1016/j.anbehav.2017.06.019
Roth, M. J., Synofzik, M., and Lindner, A. (2013). The cerebellum optimizes perceptual predictions about external sensory events. Curr. Biol. 23, 930–935. doi: 10.1016/j.cub.2013.04.027
Roy, A., Shepherd, S. V., and Platt, M. L. (2014). Reversible inactivation of pSTS suppresses social gaze following in the macaque (Macaca mulatta). Soc. Cogn. Affect Neurosci. 9, 209–217. doi: 10.1093/scan/nss123
Ruiz, A., Gómez, J. C., Roeder, J. J., and Byrne, R. W. (2009). Gaze following and gaze priming in lemurs. Anim. Cogn. 12, 427–434. doi: 10.1007/s10071-008-0202-z
Sánchez-Amaro, A., Tan, J., Kaufhold, S. P., and Rossano, F. (2020). Gibbons exploit information about what a competitor can see. Anim. Cogn. 23, 289–299. doi: 10.1007/s10071-019-01333-7
Sandel, A. A., MacLean, E. L., and Hare, B. (2011). Evidence from four lemur species that ringtailed lemur social cognition converges with that of haplorhine primates. Anim. Behav. 81, 925–931. doi: 10.1016/j.anbehav.2011.01.020
Santos, L. R., and Hauser, M. D. (1999). How monkeys see the eyes: Cotton-top tamarins’ reaction to changes in visual attention and action. Anim. Cogn. 2, 131–139. doi: 10.1007/s100710050033
Scaife, M., and Bruner, J. S. (1975). The capacity for joint visual attention in the infant. Nature 253, 265–266. doi: 10.1038/253265a0
Scerif, G., Gomez, J.-C., and Byrne, R. W. (2004). What do Diana monkeys know about the focus of attention of a conspecific? Anim. Behav. 68, 1239–1247. doi: 10.1016/j.anbehav.2004.01.011
Schafer, G., and Plunkett, K. (1998). Rapid word learning by fifteen-month-olds under tightly controlled conditions. Child Dev. 69, 309–320. doi: 10.2307/1132166
Schaffer, A., Caicoya, A. L., Colell, M., Holland, R., Ensenyat, C., and Amici, F. (2020). Gaze following in ungulates: domesticated and non-domesticated species follow the gaze of both humans and conspecifics in an experimental context. Front. Psychol. 11:604904. doi: 10.3389/fpsyg.2020.604904
Scheumann, M., and Call, J. (2004). The use of experimenter-given cues by south African fur seals (Arctocephalus pusillus). Anim. Cogn. 7, 224–230. doi: 10.1007/s10071-004-0216-0
Schloegl, C., Kotrschal, K., and Bugnyar, T. (2007). Gaze following in common ravens, Corvus corax: ontogeny and habituation. Anim. Behav. 74, 769–778. doi: 10.1016/j.anbehav.2006.08.017
Schloegl, C., Kotrschal, K., and Bugnyar, T. (2008a). Do common ravens (Corvus corax) rely on human or conspecific gaze cues to detect hidden food? Anim. Cogn. 11, 231–241. doi: 10.1007/s10071-007-0105-4
Schloegl, C., Schmidt, J., Scheid, C., Kotrschal, K., and Bugnyar, T. (2008b). “Gaze following in non-human animals: the corvid example” in Animal Behaviour: New Research. ed. F. Columbus (New York, NY: Nova Science Publishers), 73–92.
Schmid, B., Karg, K., Perner, J., and Tomasello, M. (2017). Great apes are sensitive to prior reliability of an informant in a gaze following task. PLOS One 12:e0187451. doi: 10.1371/journal.pone.0187451
Schmidt, J., Scheid, C., Kotrschal, K., Bugnyar, T., and Schloegl, C. (2011). Gaze direction–A cue for hidden food in rooks (Corvus frugilegus)? Behav Process 88, 88–93. doi: 10.1016/j.beproc.2011.08.002
Senju, A., and Csibra, G. (2008). Gaze following in human infants depends on communicative signals. Curr. Biol. 18, 668–671. doi: 10.1016/j.cub.2008.03.059
Senju, A., Csibra, G., and Johnson, M. H. (2008). Understanding the referential nature of looking: infants’ preference for object-directed gaze. Cognition 108, 303–319. doi: 10.1016/j.cognition.2008.02.009
Sewards, T. V., and Sewards, M. A. (2002). Innate visual object recognition in vertebrates: some proposed pathways and mechanisms. Comp. Biochem. Physiol. A Mol. Integr. Physiol. 132, 861–891. doi: 10.1016/S1095-6433(02)00119-8
Shapiro, A. D., Janik, V. M., and Slater, P. J. (2003). A gray Seal's (Halichoerus grypus) responses to experimenter-given pointing and directional cues. J. Comp. Psychol. 117, 355–362. doi: 10.1037/0735-7036.117.4.355
Shepherd, S. V. (2010). Following gaze: gaze-following behavior as a window into social cognition. Front. Integr. Neurosci. 4:5. doi: 10.3389/fnint.2010.00005
Shepherd, S. V., Deaner, R. O., and Platt, M. L. (2006). Social status gates social attention in monkeys. Curr. Biol. 16, R119–R120. doi: 10.1016/j.cub.2006.02.013
Shepherd, S. V., Klein, J. T., Deaner, R. O., and Platt, M. L. (2009). Mirroring of attention by neurons in macaque parietal cortex. Proc. Natl. Acad. Sci. 106, 9489–9494. doi: 10.1073/pnas.0900419106
Shepherd, S. V., and Platt, M. L. (2008). Spontaneous social orienting and gaze following in ringtailed lemurs (Lemur catta). Anim. Cogn. 11, 13–20. doi: 10.1007/s10071-007-0083-6
Simpson, J., and O’Hara, S. J. (2019). Gaze following in an asocial reptile (Eublepharis macularius). Anim. Cogn. 22, 145–152. doi: 10.1007/s10071-018-1230-y
Siviter, H., Deeming, D. C., van Giezen, M., and Wilkinson, A. (2017). Incubation environment impacts the social cognition of adult lizards. R. Soc. Open Sci. 4:170742. doi: 10.1098/rsos.170742
Smith, B. P., and Litchfield, C. A. (2010). Dingoes (Canis dingo) can use human social cues to locate hidden food. Anim. Cogn. 13, 367–376. doi: 10.1007/s10071-009-0287-z
Soproni, K., Miklosi, A., Topal, J., and Csanyi, V. (2001). Comprehension of human communicative signs in pet dogs (Canis familiaris). J. Comp. Psychol. 115, 122–126. doi: 10.1037//0735-7036.115.2.122
Téglás, E., Gergely, A., Kupán, K., Miklósi, Á., and Topál, J. (2012). Dogs' gaze following is tuned to human communicative signals. Curr. Biol. 22, 209–212. doi: 10.1016/j.cub.2011.12.018
Teufel, C., Gutmann, A., Pirow, R., and Fischer, J. (2010). Facial expressions modulate the ontogenetic trajectory of gaze-following among monkeys. Dev. Sci. 13, 913–922. doi: 10.1111/j.1467-7687.2010.00956.x
Tomasello, M., Call, J., and Hare, B. (1998). Five primate species follow the visual gaze of conspecifics. Anim. Behav. 55, 1063–1069. doi: 10.1006/anbe.1997.0636
Tomasello, M., Hare, B., and Fogleman, T. (2001). The ontogeny of gaze following in chimpanzees, pan troglodytes, and rhesus macaques, Macaca mulatta. Anim. Behav. 61, 335–343. doi: 10.1006/anbe.2000.1598
Tomasello, M., Hare, B., Lehmann, H., and Call, J. (2007). Reliance on head versus eyes in the gaze following of great apes and human infants: the cooperative eye hypothesis. J. Hum. Evol. 52, 314–320. doi: 10.1016/j.jhevol.2006.10.001
Tootell, R. B., Tsao, D., and Vanduffel, W. (2003). Neuroimaging weighs in: humans meet macaques in “primate” visual cortex. J. Neurosci. 23, 3981–3989. doi: 10.1523/JNEUROSCI.23-10-03981.2003
Tornick, J. K., Gibson, B. M., Kispert, D., and Wilkinson, M. (2011). Clark’s nutcrackers (Nucifraga columbiana) use gestures to identify the location of hidden food. Anim. Cogn. 14, 117–125. doi: 10.1007/s10071-010-0349-2
Tschudin, A., Call, J., Dunbar, R. I., Harris, G., and van der Elst, C. (2001). Comprehension of signs by dolphins (Tursiops truncatus). J. Comp. Psychol. 115, 100–105. doi: 10.1037/0735-7036.115.1.100
Varricchio, D. J., and Jackson, F. D. (2016). Reproduction in Mesozoic birds and evolution of the modern avian reproductive mode. Auk Ornithol. Adv. 133, 654–684. doi: 10.1642/AUK-15-216.1
Varricchio, D. J., Moore, J. R., Erickson, G. M., Norell, M. A., Jackson, F. D., and Borkowski, J. J. (2008). Avian paternal care had dinosaur origin. Science 322, 1826–1828. doi: 10.1126/science.1163245
Vick, S.-J., and Anderson, J. R. (2003). Use of human visual attention cues by olive baboons (Papio anubis) in a competitive task. J. Comp. Psychol. 117, 209–216. doi: 10.1037/0735-7036.117.2.209
Virányi, Z., Topál, J., Gácsi, M., Miklósi, Á., and Csányi, V. (2004). Dogs respond appropriately to cues of humans’ attentional focus. Behav. Process. 66, 161–172. doi: 10.1016/j.beproc.2004.01.012
von Bayern, A. M., and Emery, N. J. (2009). Jackdaws respond to human attentional states and communicative cues in different contexts. Curr. Biol. 19, 602–606. doi: 10.1016/j.cub.2009.02.062
Vuilleumier, P., and Pourtois, G. (2007). Distributed and interactive brain mechanisms during emotion face perception: evidence from functional neuroimaging. Neuropsychologia 45, 174–194. doi: 10.1016/j.neuropsychologia.2006.06.003
Wallis, L. J., Range, F., Muller, C. A., Serisier, S., Huber, L., and Viranyi, Z. (2015). Training for eye contact modulates gaze following in dogs. Anim. Behav. 106, 27–35. doi: 10.1016/j.anbehav.2015.04.020
Werhahn, G., Virányi, Z., Barrera, G., Sommese, A., and Range, F. (2016). Wolves (Canis lupus) and dogs (Canis familiaris) differ in following human gaze into distant space but respond similar to their packmates’ gaze. J. Comp. Psychol. 130, 288–298. doi: 10.1037/com0000036
Wilkinson, A., Kuenstner, K., Mueller, J., and Huber, L. (2010a). Social learning in a non-social reptile (Geochelone carbonaria). Biol. Lett. 6, 614–616. doi: 10.1098/rsbl.2010.0092
Wilkinson, A., Mandl, I., Bugnyar, T., and Huber, L. (2010b). Gaze following in the red-footed tortoise (Geochelone carbonaria). Anim. Cogn. 13, 765–769. doi: 10.1007/s10071-010-0320-2
Wolpert, D. M., and Flanagan, J. R. (2001). Motor prediction. Curr. Biol. 11, R729–R732. doi: 10.1016/s0960-9822(01)00432-8
Wolpert, D. M., Miall, R. C., and Kawato, M. (1998). Internal models in the cerebellum. Trends Cogn. Sci. 2, 338–347. doi: 10.1016/S1364-6613(98)01221-2
Woodward, A. L. (2003). Infants’ developing understanding of the link between looker and object. Dev. Sci. 6, 297–311. doi: 10.1111/1467-7687.00286
Yamane, S., Kaji, S., and Kawano, K. (1988). What facial features activate face neurons in the inferotemporal cortex of the monkey? Exp. Brain Res. 73, 209–214. doi: 10.1007/BF00279674
Zeiträg, C., Reber, S. A., and Osvath, M. (2022). Gaze-following in Archosauria: Alligators and palaeognath birds suggest dinosaur origin of visual perspective taking. bioRxiv [Preprint]. doi: 10.1101/2022.09.23.509198
Keywords: gaze, evolution, social cognition, social information, orienting
Citation: Zeiträg C, Jensen TR and Osvath M (2022) Gaze following: A socio-cognitive skill rooted in deep time. Front. Psychol. 13:950935. doi: 10.3389/fpsyg.2022.950935
Received: 23 May 2022; Accepted: 07 October 2022;
Published: 02 December 2022.
Edited by:
Vanessa Wilson, Université de Neuchâtel, SwitzerlandReviewed by:
Alina Schaffer, Leipzig University, GermanyCopyright © 2022 Zeiträg, Jensen and Osvath. This is an open-access article distributed under the terms of the Creative Commons Attribution License (CC BY). The use, distribution or reproduction in other forums is permitted, provided the original author(s) and the copyright owner(s) are credited and that the original publication in this journal is cited, in accordance with accepted academic practice. No use, distribution or reproduction is permitted which does not comply with these terms.
*Correspondence: Claudia Zeiträg, Y2xhdWRpYS56ZWl0cmFnQGx1Y3MubHUuc2U=
Disclaimer: All claims expressed in this article are solely those of the authors and do not necessarily represent those of their affiliated organizations, or those of the publisher, the editors and the reviewers. Any product that may be evaluated in this article or claim that may be made by its manufacturer is not guaranteed or endorsed by the publisher.
Research integrity at Frontiers
Learn more about the work of our research integrity team to safeguard the quality of each article we publish.