- College of Sport and Exercise Science, Institute for Health and Sport, Victoria University, Melbourne, VIC, Australia
Introduction
There are several situations that can cause an athlete's regular training volume to be reduced, such as injury, traveling overseas for competition, or highly relevant to the current world climate, inability to attend a training venue due to infection risk (i.e., COVID-19). Subsequently, practitioners have sought supplementary training methods that can complement or be added to physical training to meet the needs of their athletes. One such training alternative is motor imagery (MI), defined as a simulation state that involves covertly rehearsing a motor action mentally by incorporating visual and kinesthetic aspects of movement, without actually executing movement (Frank et al., 2014; Eaves et al., 2016). MI is most effective when combined with physical training, providing an additive effective to performance (Lindsay et al., 2021). Considerable evidence exists attesting to the beneficial effects of MI for performance and skill development (e.g., Simonsmeier et al., 2020; Lindsay et al., 2021). Motor Simulation Theory (MST) proposes that the positive effects of MI can in part be attributed to shared neural mechanisms with physical practice, termed functional equivalence (Jeannerod, 1994, 1995; Debarnot et al., 2014; Moran and O'Shea, 2020). Consistent with MST, Hétu et al. (2013) found that MI activated areas of the brain similar to that observed during motor execution including premotor and parietal cortices, and fronto-parietal regions. Further evidence indicates that MI may also be capable of producing similar training-related adaptations in central neural structures as physical training. For example, Leung et al. (2013) showed that increases in corticospinal excitability were equivalent following MI training relative to physical training of a bicep-curl, suggesting similar changes in motor execution processes.
More recently, researchers have advocated for combining techniques such as action observation (AO) with MI to facilitate greater improvements in performance relative to MI or AO delivered as separate interventions (Eaves et al., 2016; Wright et al., 2021). AO involves observing actions displayed via video or physical demonstration of another individual or self with the intention of replicating the observed movement (Wright et al., 2021). The effectiveness of AO for improving motor skill performance and learning has commonly been contextualized through the notion that observation of motor actions activates an action observation network (AON), comprising of similar motor areas of the brain (e.g., premotor cortex, inferior parietal lobule and supplementary motor area) utilized during physical action (Cross et al., 2009).
Combining Action Observation and Motor Imagery
Previous research has often treated MI and AO as separate interventions (Jeunet et al., 2020), however, there is an emerging body of evidence to suggest that both techniques can be combined or structured so that AO precedes MI to act as a visual primer of the movement being practiced (Kim et al., 2017; Romano-Smith et al., 2018). Treated as a single technique, AOMI interventions have been shown to be more effective for enhancing learning and performance relative to AO or MI alone (Eaves et al., 2016). For example, Romano-Smith et al. (2018) found that participants practicing a manual aiming task with either simultaneous or alternate AOMI improved performance significantly more than MI or AO alone. The success of AOMI has largely been attributed increases in neural activity in motor regions of the brain, which exceeds levels produced in MI or AO alone (Romano-Smith et al., 2018). This is supported by neurophysiological research suggesting that AOMI produces more significant cortico-motor activity compared to AO alone (Wright et al., 2014). Wright et al. (2018) found that AOMI facilitated significantly greater corticospinal excitability relative to AO or MI alone when practicing a basketball free-throw. Overall, the emerging body of evidence provides some encouraging findings to suggest that AOMI could facilitate greater neurophysiological activity in motor-related brain areas, potentially contributing to improved motor skill and learning outcomes.
Though evidence indicates clear benefits of AOMI, one element that deserves further consideration is the type of video footage used during AOMI. Presently, AOMI interventions have primarily utilized 2-D video footage from the first-person perspective (Romano-Smith et al., 2018; Scott et al., 2018). An issue related to the type of video footage is its influence on the sense of agency (SoA) for the participant. SoA is described as an individual's experience of the level of control and initiation they have over an action (Braun et al., 2018). Further, SoA and the degree of immersion an individual experiences within virtual environments have been proposed to be important factors in the efficacy of virtual training (Rose et al., 2018). Immersion is described as the degree of which simulated environments produce experiences that accurately replicate the multimodal sensory nature of the real-world (Rose et al., 2018). Recent research suggests that the degree of immersion in virtual environments can influence an individual's perception of whether they really have control over their own movements (i.e., SoA; Kong et al., 2017). However, further research is needed to understand the relationship between immersion and SoA.
Generally, researchers conducting an AOMI intervention will ask participants to imagine themselves executing the motor skill; whereas, the video is usually a recording of another person performing the skill. According to the comparator model of motor control (Frith, 2005; David et al., 2008), the level of control of a movement is governed by an internal prediction model within the brain (David et al., 2008). When a new motor command is produced, this causes creation of an efference copy. If the efference copy is the same as the actual sensory input (e.g., AO video footage) the motor action is interpreted as being self-caused, leading to SoA (Braun et al., 2018). Captured this way, it is possible that using video footage during AO of another individual may result in a mismatch between the efference copy and the sensory input (AO of another individual), culminating in a lack of perceived self-caused movement (Braun et al., 2018). Previous MI research seems consistent with this idea, suggesting that kinesthetic MI may be inhibited when viewing another person (Callow and Hardy, 2004). In order to create a representative AOMI experience for the individual understanding how to create a SoA is an important consideration for the practitioner to ensure that the simulated movement is perceived as self-caused.
Virtual Reality for Motor Imagery
Alternative technologies, such as virtual reality (VR), present a viable solution for improving SoA of AOMI interventions by generating a more interactive and immersive practice environment. Given the increased neurophysiological activity reported during AOMI, authors support this combined approach as being the optimal method for mental simulation interventions in sport (McNeil et al., 2021; Wright et al., 2021). For example, Im et al. (2016) reported that virtual-reality-guided MI of a wrist extension task increased cortical excitability in both stroke patients and healthy participants more than MI alone. Similarly, Bedir and Erhan (2021) reported significant improvements in shot accuracy in curling, bowling and archery athletes following VR-based imagery relative to Visual Motor Behavior Rehearsal and Video Modeling. In addition, it was noted that the VR component may have contributed to participants adapting to imagery training earlier due to the enhanced simulation of kinesthetic, visual and auditory senses. VR can improve the level of “presence,” which is defined as how much individuals feel immersed within an environment (Slater, 2018; Bird, 2020). VR typically presents virtual scenarios enabling the participant to interact (Düking et al., 2018). These VR scenarios, however, may limit ecological validity, which refers to similarity of perceptual information in the simulation to the real-world (i.e., competitive environment) (Araujo et al., 2007).
360°VR as an Alternative
360°VR overcomes potential ecological validity limitations of VR, by presenting real-world immersive video, while also using a head-mounted display (HMD) similar to VR [see Kittel et al. (2020a) for a SWOT analysis of this technology]. Harris et al. (2020) explain that physical fidelity and realism are vital for the “VR equivalent of mental imagery” (p. 4), however, this suggests that VR and MI are always separate. We suggest that 360°VR deserves consideration as the AO component of combined AOMI training as it provides realistic simulations that can be created in an easy cost-effective manner. Realism is a vital component of presence (Schubert et al., 2001), suggesting the vision provided through 360°VR would lead to greater presence than virtual environments (i.e., VR). 360°VR presented using a HMD allows participants to scan the environment using head movements, which in turn increases the visual flow perceived (Craig, 2013; Pagé et al., 2019). Participants have the ability to attune to cues they perceive to be relevant, rather than flat-screen video providing a limited number of cues, given the less interactive nature of the presentation. This is pertinent when considering the importance of SoA. Flat screen video does not allow participants to scan, potentially diminishing the SoA in AOMI. Incorporating 360°VR as the AO component is likely to increase the perception of SoA. This would allow a more personalized and exploratory approach, as research has indicated that personalized MI can provide benefits over generic MI (Wilson et al., 2010). An important consideration is that individuals may not be able to generate and control MI as prescribed by the coach or sport psychologist (Wright et al., 2021). Therefore, 360°VR presents a viable addition to facilitate MI generation that could be the missing link for providing the initial vivid imagery required. Wright et al. (2021) explain that when using flat-screen video as the AO component, the practitioner can control the viewing perspective. However, 360°VR enables the performer to go one step further by allowing them the opportunity to scan through the environment, as they would in competition. Figure 1 provides an example of how 360°VR and MI could be implemented for practicing skills in Australian Rules Football adapted from recommendations provided by Wright et al. (2021).
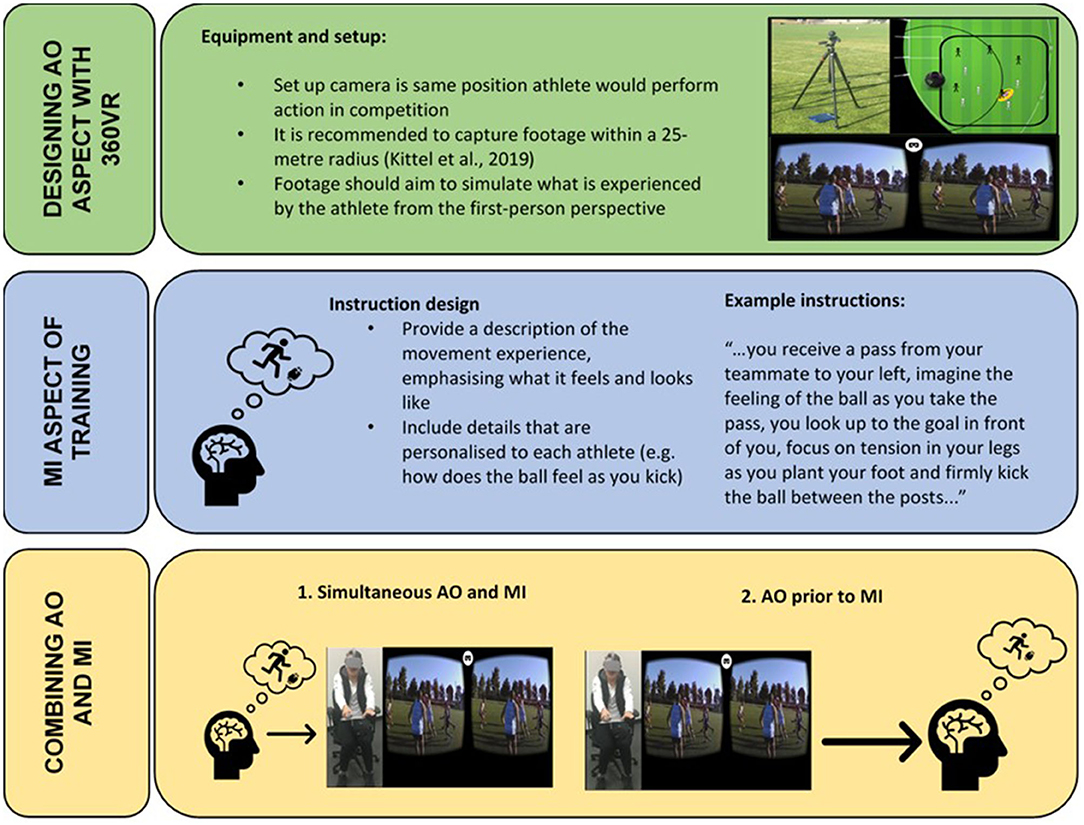
Figure 1. Example of how 360°VR and MI can be implemented for practicing skills in Australian Rules Football. Adapted from recommendations provided by Wright et al. (2021).
When developing 360°VR, there are several technical considerations for presenting this innovative footage. For example, there are several different 360°VR cameras available at different costs. These can range from several hundred dollars (2022 retail price) such as the 360fly4k (single lens) used by Kittel et al. (2019) and the Ricoh Theta S 360° camera (dual lens) used by Pagé et al. (2019). There are options that are more expensive available such as six Go-Pros in a cube (Panchuk et al., 2018). There is a trade-off between better quality cameras that use multiple lenses, but require significantly more post-production stitching the footage together. Although HMDs present stereoscopic vision that allows the viewer to perceive the depth of objects and increase representativeness (Farley et al., 2020), the headsets can differentiate the quality of the video. For example, there are lower costing (and lower resolution) such as the Utopia 360 HMD (Pagé et al., 2019) to tethered HMDs with in-built screens that allows other individuals to see the 360°VR through an attached display (Oculus Rift; Panchuk et al., 2018). The Oculus Go may be an appropriate middle ground, given it is affordable and uses an in-built screen, but is lower in price and not tethered to a display. While VR may cause issues with latency between their perception and the virtual avatar (for a more technical overview of 360°VR, please see Farley et al., 2020), this is not an issue with 360°VR as the participant is not interacting with a virtual avatar. Future research is required to understand these technical elements of 360°VR, such as utilizing monoscopic versus stereoscopic views.
As highlighted earlier, presence is vital for simulated environments, whether that be MI or AO in a virtual environment. To be immersed and present, there needs to be a strong sense of plausibility, namely the feeling that the situation is actually occurring (Slater and Sanchez-Vives, 2016; Harris et al., 2020). Given the high levels of ecological validity that 360°VR provides (Kittel et al., 2019), this can offer increased plausibility for MI for the individual to feel the situation is real. A reported limitation of using 360°VR is the difficulty in combining perception and action, given participants are observing a real environment, rather than interacting within a virtual environment (Kittel et al., 2020a). Incorporating MI with 360°VR technology as the AO component could help mitigate this, as MI can include the kinesthetic (i.e., moving component). Theoretically, this may increase the perception-action coupling that is not always present when using 360°VR technology (Fadde and Zaichkowsky, 2018). 360°VR has demonstrated positive long-term behavioral changes in perceptual-cognitive skills such as decision-making (Kittel et al., 2020b), which could be attributed to greater embodiment, allowing greater sensorimotor engagement for behavioral adaptations (Bohil et al., 2011; Kilteni et al., 2012). The implementation of this technology as the AO component could enhance the optimized long-term memory changes gained with the combination of AOMI (Kim et al., 2017; Wright et al., 2021). From a practical standpoint, 360°VR presents an affordable option for practitioners to present AO in a more immersive way. 360°VR is significantly financially more affordable and accessible than VR (Düking et al., 2018; Kittel et al., 2020a). 360 cameras are relatively comparable in price to standard cameras, and when presented through a HMD, this is significantly less expensive than the associated costs of developing and presenting a virtual environment in VR. Importantly, 360°VR presents a more novel presentation method for AO, with this technology rated as more enjoyable and relevant as a perceptual-cognitive tool than screen-based video (Kittel et al., 2020b).
Conclusion
In conclusion, 360°VR provides an innovative tool to be used in conjunction with MI in sport. Given the current climate, in which athletes may be spending long periods in quarantine situations before competitions, simulated training techniques such as AOMI may prove an invaluable technique in the athletes' toolbox. The enhanced realism of 360°VR lends a greater sense of presence and embodiment for the individual to be immersed in the imagined environment. Furthermore, the SoA is improved by the individual's ability to scan within a real-world environment. As a result, 360°VR allows MI to provide consistent simulations for athletes, with AO and MI combined for greater neurophysiological stimulation. With the long-term behavioral changes afforded by AOMI and 360°VR, the integration of this technology as the AO component would theoretically enhance SoA and should be investigated further from a theoretical and applied perspective in the literature.
Author Contributions
RL, AK, and MS contributed to conception and structure of this article and writing the first draft of the manuscript. All authors were involved in manuscript revision, reading, and approval of the submitted version.
Funding
This work was supported by the Institute for Health and Sport, Victoria University.
Conflict of Interest
The authors declare that the research was conducted in the absence of any commercial or financial relationships that could be construed as a potential conflict of interest.
Publisher's Note
All claims expressed in this article are solely those of the authors and do not necessarily represent those of their affiliated organizations, or those of the publisher, the editors and the reviewers. Any product that may be evaluated in this article, or claim that may be made by its manufacturer, is not guaranteed or endorsed by the publisher.
References
Araujo, D., Davids, K., and Passos, P. (2007). Ecological validity, representative design, and correspondence between experimental task constraints and behavioral setting: Comment on Rogers, Kadar, and Costall (2005). Ecol. Psychol. 19, 69–78. doi: 10.1080/10407410709336951
Bedir, D., and Erhan, S. E. (2021). The effect of virtual reality technology on the imagery skills and performance of target-based sports athletes. Front. Psychol. 11, 2073. doi: 10.3389/fpsyg.2020.02073
Bird, J. M. (2020). The use of virtual reality head-mounted displays within applied sport psychology. J. Sport Psychol. Action 11, 115–128. doi: 10.1080/21520704.2018.1563573
Bohil, C. J., Alicea, B., and Biocca, F. A. (2011). Virtual reality in neuroscience research and therapy. Nat. Rev. Neurosci. 12, 752–762.doi: 10.1038/nrn3122
Braun, N., Debener, S., Spychala, N., Bongartz, E., Sörös, P., Müller, H. H. O., et al. (2018). The senses of agency and ownership: a review. Front. Psychol. 9, 535. doi: 10.3389/fpsyg.2018.00535
Callow, N., and Hardy, L. (2004). The relationship between the use of kinaesthetic imagery and different visual imagery perspectives. J. Sports. Sci. 22, 167–177. doi: 10.1080/02640410310001641449
Craig, C. (2013). Understanding perception and action in sport: how can virtual reality technology help? Sports Technol. 6, 161–169. doi: 10.1080/19346182.2013.855224
Cross, E. S., Hamilton, A. F. D. C., Kraemer, D. J., Kelley, W. M., and Grafton, S. T. (2009). Dissociable substrates for body motion and physical experience in the human action observation network. Eur. J. Neurosci. 30, 1383–1392. doi: 10.1111/j.1460-9568.2009.06941.x
David, N., Newen, A., and Vogeley, K. (2008). The “sense of agency” and its underlying cognitive and neural mechanisms. Conscious. Cogn. 17, 523–534. doi: 10.1016/j.concog.2008.03.004
Debarnot, U., Sperduti, M., Di Rienzo, F., and Guillot, A. (2014). Experts bodies, experts minds: how physical and mental training shape the brain. Front. Human Neurosci. 8, 280. doi: 10.3389/fnhum.2014.00280
Düking, P., Holmberg, H.-C., and Sperlich, B. (2018). The potential usefulness of virtual reality systems for athletes: a short SWOT analysis. Front. Physiol. 9, 128. doi: 10.3389/fphys.2018.00128
Eaves, D. L., Riach, M., Holmes, P. S., and Wright, D. J. (2016). Motor imagery during action observation: a brief review of evidence, theory and future research opportunities. Front. Neurosci. 10, 514. doi: 10.3389/fnins.2016.00514
Fadde, P. J., and Zaichkowsky, L. (2018). Training perceptual-cognitive skills in sports using technology. J. Sport Psychol. Action 9, 239–248. doi: 10.1080/21520704.2018.1509162
Farley, O. R., Spencer, K., and Baudinet, L. (2020). Virtual reality in sports coaching, skill acquisition and application to surfing: a review. J. Human Sport Exerc. 15, 535–548. doi: 10.14198/jhse.2020.153.06
Frank, C., Land, W. M., Popp, C., and Schack, T. (2014). Mental representation and mental practice: experimental investigation on the functional links between motor memory and motor imagery. PLOS ONE 9, e95175. doi: 10.1371/journal.pone.0095175
Frith, C. (2005). The self in action: lessons from delusions of control. Conscious. Cogn. 14, 752–770. doi: 10.1016/j.concog.2005.04.002
Harris, D. J., Bird, J. M., Smart, P. A., Wilson, M. R., and Vine, S. J. (2020). A framework for the testing and validation of simulated environments in experimentation and training. Front. Psychol. 11, 605. doi: 10.3389/fpsyg.2020.00605
Hétu, S., Grégoire, M., Saimpont, A., Coll, M.-P., Eugène, F., Michon, P.-E., et al. (2013). The neural network of motor imagery: an ALE meta-analysis. Neurosci. Biobehav. Rev. 37, 930–949. doi: 10.1016/j.neubiorev.2013.03.017
Im, H., Ku, J., Kim, H. J., and Kang, Y. J. (2016). Virtual reality-guided motor imagery increases corticomotor excitability in healthy volunteers and stroke patients. Ann. Rehabil. Med. 40, 420–431. doi: 10.5535/arm.2016.40.3.420
Jeannerod, M. (1994). The representing brain: neural correlates of motor intention and imagery. Behav. Brain Sci. 17, 187–202. doi: 10.1017/S0140525X00034026
Jeannerod, M. (1995). Mental imagery in the motor context. Neuropsychologia 33, 1419–1432. doi: 10.1016/0028-3932(95)00073-C
Jeunet, C., Hauw, D., and Millán, J. R. (2020). Sport psychology: technologies ahead. Front. Sports Active Liv. 2, 10. doi: 10.3389/fspor.2020.00010
Kilteni, K., Groten, R., and Slater, M. (2012). The sense of embodiment in virtual reality. Presence Teleoper. Virtual Environ. 21, 373–387. doi: 10.1162/PRES_a_00124
Kim, T., Frank, C., and Schack, T. (2017). A systematic investigation of the effect of action observation training and motor imagery training on the development of mental representation structure and skill performance. Front. Human Neurosci. 11, 499. doi: 10.3389/fnhum.2017.00499
Kittel, A., Larkin, P., Cunningham, I., and Spittle, M. (2020a). 360° VR: a SWOT analysis. Front. Psychol. 11, 2525. doi: 10.3389/fpsyg.2020.563474
Kittel, A., Larkin, P., Elsworthy, N., Lindsay, R., and Spittle, M. (2020b). Effectiveness of 360° virtual reality and match broadcast video to improve decision-making skill. Sci. Med. Football 5, 1–8. doi: 10.1080/24733938.2020.1802506
Kittel, A., Larkin, P., Elsworthy, N., and Spittle, M. (2019). Using 360° virtual reality as a decision-making assessment tool in sport. J. Sci. Med. Sport 22, 1049–1053. doi: 10.1016/j.jsams.2019.03.012
Kong, G., He, K., and Wei, K. (2017). Sensorimotor experience in virtual reality enhances sense of agency associated with an avatar. Conscious. Cogn. 52, 115–124. doi: 10.1016/j.concog.2017.04.018
Leung, M. C., Spittle, M., and Kidgell, D. J. (2013). Corticospinal excitability following short-term motor imagery training of a strength task. J. Imag. Res. Sport Phys. Activity 8, 35–44. doi: 10.1515/jirspa-2012-0006
Lindsay, R. S., Larkin, P., Kittel, A., and Spittle, M. (2021). Mental imagery training programs for developing sport-specific motor skills: a systematic review and meta-analysis. Phys. Educ. Sport Pedag. 2021, 1–22. doi: 10.1080/17408989.2021.1991297
McNeil, D. G., Spittle, M., and Mesagno, C. (2021). Imagery training for reactive agility: Performance improvements for decision time but not overall reactive agility. Int. J. Sport Exerc. Psychol. 19, 429–445. doi: 10.1080/1612197X.2019.1696866
Moran, A., and O'Shea, H. (2020). Motor imagery practice and cognitive processes. Front. Psychol. 11, 394. doi: 10.3389/fpsyg.2020.00394
Pagé, C., Bernier, P.-M., and Trempe, M. (2019). Using video simulations and virtual reality to improve decision-making skills in basketball. J. Sports Sci. 37, 2403–2410. doi: 10.1080/02640414.2019.1638193
Panchuk, D., Klusemann, M. J., and Hadlow, S. M. (2018). Exploring the effectiveness of immersive video for training decision-making capability in elite, youth basketball players. Front. Psychol. 9, 2315. doi: 10.3389/fpsyg.2018.02315
Romano-Smith, S., Wood, G., Wright, D. J., and Wakefield, C. J. (2018). Simultaneous and alternate action observation and motor imagery combinations improve aiming performance. Psychol. Sport Exerc. 38, 100–106. doi: 10.1016/j.psychsport.2018.06.003
Rose, T., Nam, C. S., and Chen, K. B. (2018). Immersion of virtual reality for rehabilitation-review. Appl. Ergon. 69, 153–161. doi: 10.1016/j.apergo.2018.01.009
Schubert, T., Friedmann, F., and Regenbrecht, H. (2001). The experience of presence: Factor analytic insights. Presence Teleoper. Virtual Environ. 10, 266–281. doi: 10.1162/105474601300343603
Scott, M., Taylor, S., Chesterton, P., Vogt, S., and Eaves, D. L. (2018). Motor imagery during action observation increases eccentric hamstring force: an acute non-physical intervention. Disabil. Rehab. 40, 1443–1451. doi: 10.1080/09638288.2017.1300333
Simonsmeier, B., Andronie, M., Buecker, S., and Frank, C. (2020). The effects of imagery interventions in sports: a meta-analysis. Rev. Sport Exerc. Psychol. 14, 186–207. doi: 10.31234/osf.io/g5tp2
Slater, M. (2018). Immersion and the illusion of presence in virtual reality. Br. J. Psychol. 109, 431–433. doi: 10.1111/bjop.12305
Slater, M., and Sanchez-Vives, M. V. (2016). Enhancing our lives with immersive virtual reality. Front. Robot AI 3, 74. doi: 10.3389/frobt.2016.00074
Wilson, C., Smith, D., Burden, A., and Holmes, P. (2010). Participant-generated imagery scripts produce greater EMG activity and imagery ability. Eur. J. Sport Sci. 10, 417–425. doi: 10.1080/17461391003770491
Wright, D. J., Frank, C., and Bruton, A. M. (2021). Recommendations for combining action observation and motor imagery interventions in sport. J. Sport Psychol. Action 2021, 1–13. doi: 10.1080/21520704.2021.1971810
Wright, D. J., Williams, J., and Holmes, P. S. (2014). Combined action observation and imagery facilitates corticospinal excitability. Front. Human Neurosci. 8, 951–951. doi: 10.3389/fnhum.2014.00951
Keywords: mental imagery, immersive video, sport psychology, virtual reality, neuroscience
Citation: Lindsay R, Kittel A and Spittle M (2022) Motor Imagery and Action Observation: A Case for the Integration of 360°VR. Front. Psychol. 13:880185. doi: 10.3389/fpsyg.2022.880185
Received: 21 February 2022; Accepted: 07 April 2022;
Published: 26 April 2022.
Edited by:
Karen Zentgraf, Goethe University Frankfurt, GermanyReviewed by:
Jorn Munzert, University of Giessen, GermanyCarolina Cruz-Neira, University of Central Florida, United States
Copyright © 2022 Lindsay, Kittel and Spittle. This is an open-access article distributed under the terms of the Creative Commons Attribution License (CC BY). The use, distribution or reproduction in other forums is permitted, provided the original author(s) and the copyright owner(s) are credited and that the original publication in this journal is cited, in accordance with accepted academic practice. No use, distribution or reproduction is permitted which does not comply with these terms.
*Correspondence: Riki Lindsay, UmlraS5MaW5kc2F5QHZ1LmVkdS5hdQ==