- 1Department of Psychology, University of Florida, Gainesville, FL, United States
- 2Kavli Institute for Systems Neuroscience, Norwegian University of Science and Technology, Trondheim, Norway
- 3Queensland Brain Institute, The University of Queensland, Brisbane, QLD, Australia
- 4Department of Clinical and Health Psychology, Center for Cognitive Aging and Memory, University of Florida, Gainesville, FL, United States
- 5Department of Psychiatry, University of California, San Diego, San Diego, CA, United States
- 6Department of Psychology, Stockholm University, Stockholm, Sweden
- 7Stockholm University Brain Imaging Centre (SUBIC), Stockholm, Sweden
- 8Florida Institute for Cybersecurity Research, University of Florida, Gainesville, FL, United States
- 9Department of Aging and Geriatric Research, Institute on Aging, University of Florida, Gainesville, FL, United States
- 10The Evelyn F. and William L. McKnight Brain Institute (MBI), University of Florida, Gainesville, FL, United States
The amygdala has been shown to be responsive to face trustworthiness. While older adults typically give higher face trustworthiness ratings than young adults, a direct link between amygdala response and age-related differences in face trustworthiness evaluation has not yet been confirmed. Additionally, there is a possible modulatory role of the neuropeptide oxytocin in face trustworthiness evaluation, but the results are mixed and effects unexplored in aging. To address these research gaps, young, and older adults were randomly assigned to oxytocin or placebo self-administration via a nasal spray before rating faces on trustworthiness while undergoing functional magnetic resonance imaging. There was no overall age-group difference in face trustworthiness ratings, but older compared to young participants gave higher trustworthiness ratings to ambivalently untrustworthy-looking faces. In both age groups, lower face trustworthiness ratings were associated with higher left amygdala activity. A comparable negative linear association was observed in right amygdala but only among young participants. Also, in the right amygdala, lower and higher, compared to moderate, face trustworthiness ratings were associated with greater right amygdala activity (i.e., positive quadratic (U-shaped) association) for both age groups. Neither the behavioral nor the brain effects were modulated by a single dose of intranasal oxytocin administration, however. These results suggest dampened response to faces with lower trustworthiness among older compared to young adults, supporting the notion of reduced sensitivity to cues of untrustworthiness in aging. The findings also extend evidence of an age-related positivity effect to the evaluation of face trustworthiness.
Introduction
Interpersonal trust is an important feature in human society and serves as a foundation for social relationships as well as economic and political activities (Van Lange, 2015). The trustworthiness that is perceived based on an individuals’ face (i.e., face trustworthiness), while not necessarily informative about the individuals’ actual status of trustworthiness, has been shown to affect interaction with the individual (Van’t Wout and Sanfey, 2008). Face trustworthiness evaluation can take place automatically and implicitly (Winston et al., 2002; Engell et al., 2007), with as minimal an exposure as 100 ms (Willis and Todorov, 2006).
Empirical evidence supports that the amygdala is involved in face trustworthiness evaluation (Bzdok et al., 2011; see Santos et al., 2016 for a meta-analysis). For example, individuals with bilateral amygdala lesions showed impaired evaluation of face trustworthiness (Adolphs et al., 1998). In healthy adults, lower face trustworthiness was associated with greater amygdala response (Winston et al., 2002; Engell et al., 2007). In addition to this negative linear trend, a positive quadratic (U-shaped) association was observed in that high and low (compared to moderate) face trustworthiness was correlated with greater amygdala response (Said et al., 2009; Mattavelli et al., 2012; Freeman et al., 2014), suggesting that amygdala may also generally respond to information with higher emotional salience (Sander et al., 2003; Ziaei et al., 2017).
These previous neuroimaging studies, however, have comprised young adults only, but there is emerging evidence of age-related differences in face trustworthiness evaluation, in that older adults may rate faces overall as more trustworthiness than young adults (Zebrowitz et al., 2013, 2017). This age effect was particularly pronounced for untrustworthy-looking faces, while young and older adults gave comparable ratings for trustworthy-looking faces (Castle et al., 2012; Zebrowitz et al., 2017; Cassidy et al., 2019; see Bailey and Leon, 2019 for a meta-analysis). This observation is consistent with findings that advanced age was associated with decreased sensitivity to deceptive cues (Ruffman et al., 2012; Frazier et al., 2021; see also Ebner et al., 2022, for a summary) and evidence of an age-related positivity effect (i.e., observed in the form of reduced negativity/increased positivity in older vs. young adults’ attention and memory; Carstensen et al., 2011; Pehlivanoglu and Verhaeghen, 2019; Ziaei et al., 2019, 2021). Based on these considerations, in the present study, we hypothesized that older compared to young participants would give higher trustworthiness ratings to faces overall, with this age-related difference particularly pronounced for less trustworthy-looking faces (Hypothesis 1).
Also, previous studies suggest age-related differences in amygdala response associated with face trustworthiness evaluation. Zebrowitz et al. (2018) explored age-related differences in amygdala response and found a trend wise negative linear association between amygdala activity and face trustworthiness in young adults, but older adults showed a trend wise positive quadratic relationship (i.e., high and low compared to moderate face trustworthiness was associated with greater amygdala activity). These trends were, however, not statistically significant when tested in each age group individually. Absence of significant age-related effects in Zebrowitz et al.’s study may have been related to the methodological approach used for defining regions of interest (ROIs). Specifically, Zebrowitz et al. (2018) defined amygdala as ROI based on an anatomical mask (Desikan et al., 2006). Their methodological approach therefore assumed that all voxels in the amygdala respond equally to face trustworthiness. Previous work, however, had demonstrated that only certain regions within the amygdala were responsive to face trustworthiness (Winston et al., 2002; Engell et al., 2007; Todorov, 2008; Said et al., 2009). Thus, analysis based on functional localization to identify regions within the amygdala that respond to face trustworthiness, rather than submitting all amygdala voxels to the analysis, may be more reliable and sensitive to determine age-related differences in the association between face trustworthiness and amygdala activity.
Applying this targeted approach in the present study, we hypothesized that young participants would show greater amygdala activity to untrustworthy- relative to trustworthy-looking faces while this effect would be reduced (or would not be present) in older participants (i.e., reduced (or no) negative linear effect of face trustworthiness on amygdala activity in older participants; Hypothesis 2). At the same time, based on evidence of relatively preserved ability to process emotionally salient information with advanced age (Leclerc and Kensinger, 2008), we predicted that both high and low (relative to moderately) trustworthy-looking faces would be associated with greater amygdala activity in both young and older participants (i.e., positive quadratic (U-shaped) effect of face trustworthiness on amygdala activity in both age groups; Hypothesis 3).
A separate line of work suggests a possible role of the nine amino acid peptide oxytocin on trust-related decision making and behavior, including face trustworthiness evaluation (Van IJzendoorn and Bakermans-Kranenburg, 2012; but see Keech et al., 2018). For example, a single dose of intranasal oxytocin, to trigger central modulation (Born et al., 2002), enhanced trust to others (Baumgartner et al., 2008; Mikolajczak et al., 2010; but see Declerck et al., 2020). Even more directly relevant to the present study, a single dose of intranasal oxytocin resulted in higher trustworthiness (and higher attractiveness) ratings to faces from strangers (Theodoridou et al., 2009, but see Lambert et al., 2014; Grainger et al., 2019).
Oxytocin-related enhancement of trust has been discussed as reflecting increased willingness to accept social risk (Kosfeld et al., 2005); and is in line with the prosocial hypothesis, which proposes that oxytocin increases attention to positive but not negative information, thus promoting affiliative prosocial behaviors (Meyer-Lindenberg, 2008; MacDonald and MacDonald, 2010). Intranasal oxytocin administration furthermore resulted in reduced amygdala activation to fearful faces (Kirsch et al., 2005); possibly reflecting anxiolytic properties of oxytocin (see also Labuschagne et al., 2010 for oxytocin’s anxiolytic properties in study on individuals with generalized social anxiety disorder). In line with both these accounts, oxytocin may reduce amygdala response associated with untrustworthy-looking faces.
Intranasal oxytocin has also been shown to promote altruistic behavior to in-group members but results in defensive aggression to members of the out-group (De Dreu et al., 2010). This social salience hypothesis proposes that oxytocin modulates the salience of social cues, regardless of their valence (Olff et al., 2013; Shamay-Tsoory and Abu-Akel, 2016). Further supporting this account is evidence of an oxytocin-related memory enhancement for faces (i.e., social stimuli) relative to objects (i.e., non-social stimuli; Rimmele et al., 2009). This oxytocin-related memory advantage for faces was accompanied by elevated attention to eyes (Guastella et al., 2008) and eye gaze direction (Tollenaar et al., 2013), suggesting that oxytocin facilitates attention to socially meaningful information.
Of note, this prior work has been done in young adults. Knowledge on age moderation of oxytocin effects, while limited, point to possible age-differential effects of intranasal oxytocin on social-cognitive processes (Campbell-Smith et al., 2015; Horta et al., 2019; Frazier et al., 2021; but see Grainger et al., 2018; also see Horta et al., 2020 for a recent overview). However, given the currently still small knowledge base, we refrained from formulating specific directional hypotheses pertaining to age-related differences in intranasal oxytocin modulation on face trustworthiness ratings and associated amygdala activity. In line with the social salience hypothesis that oxytocin draws attention to important social cues, one could propose that elevated oxytocin levels may increase older adults’ attention to cues of untrustworthiness in faces (and thus enhance their amygdala response to these faces), as negative facial signals that, while highly diagnostic, older adults may ignore otherwise given an age-related positivity bias.
Materials and Methods
Participants
This analysis was part of a larger project (see Ebner et al., 2015, 2016; Lin et al., 2018; Horta et al., 2019; Plasencia et al., 2019; Frazier et al., 2021). The current analysis sample comprised 98 of the originally recruited 105 participants,1 with 48 young (M = 22.43 years, SD = 2.97, 18–31 years, 48% females) and 50 older (M = 71.08 years, SD = 4.98, 63–81 years, 58% females) adults. Using G*Power, sensitivity analyses showed that with the current sample size and p = 0.05 as type I error threshold, we had 80% power to detect a small effect (Cohen’s f = 0.18; Cohen, 2013) for the interaction between age group and face trustworthiness level on trustworthiness ratings (Hypothesis 1); and 80% power to detect small effects (Cohen’s f2 = 0.13; Cohen, 2013) for the linear and quadratic trends of face trustworthiness and their interactions with age group on amygdala activity (Hypothesis 2 and 3). In addition, we had 80% power to detect a small effect (Cohen’s f = 0.22; Cohen, 2013) for the interaction between age group, treatment group, and face trustworthiness level on amygdala activity (i.e., exploratory analysis for oxytocin modulation).
All participants were English-speaking, White, with no history of neurological or psychiatric disorder, and with normal or corrected-to-normal vision. All older participants completed the Telephone Interview for Cognitive Status (TICS; Brandt et al., 1988; M = 35.47, SD = 2.42, Min = 30, Max = 42; cut off < 30) as screening for cognitive impairment. Twenty-six young (46% females) and 26 older (54% females) participants were randomly assigned to self-administer 24 international units (IUs) oxytocin via nasal spray and 22 young (50% females) and 24 older (63% females) participants to self-administer 24 IUs of a placebo nasal spray.
As shown in Table 1, overall, young participants performed better in the cognitive tasks (i.e., higher processing speed measured by the WAIS-R Digital Symbol Substitution Test (Wechsler, 1981) and better short-term memory measured by the Rey Auditory Verbal Learning Test (Rey, 1964) than older participants. The age groups also differed in positive affect with higher positive affect scores in older than young participants measured by the Positive Affect Negative Affect Scale (Watson et al., 1988; Röcke et al., 2009).
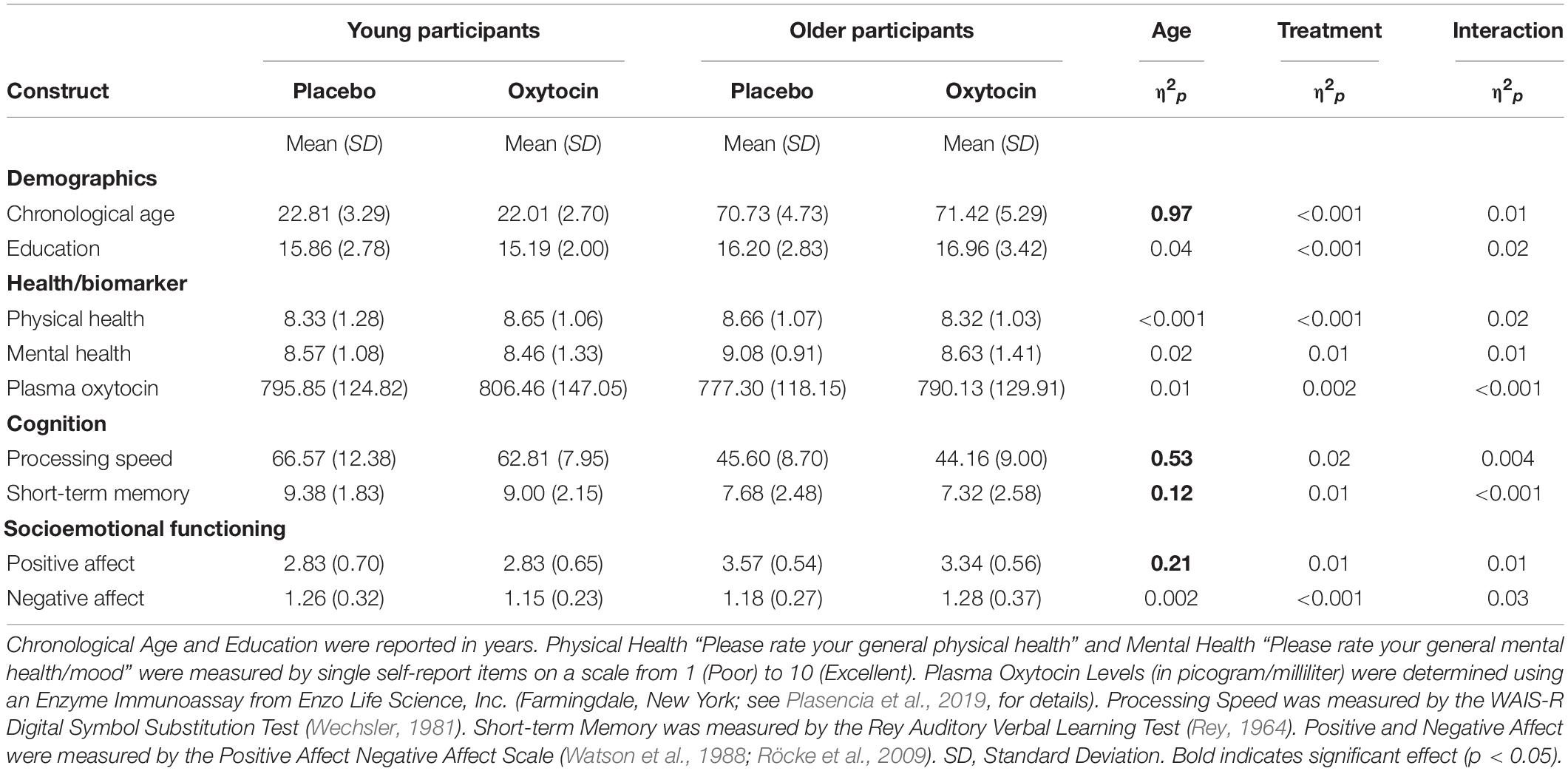
Table 1. Sample description: Mean (standard deviation) and age group as well as treatment group comparisons in demographic, health/biomarker, cognition, personality, and socioemotional measures.
Procedure
The present study used data from a larger project that comprised three test sessions: (1) a phone screen (about 30 min) to determine study eligibility (for drug administration as well as MRI) and to collect background demographic and health information; (2) an in-person screen visit (about 45 min), in which participants gave written informed consent, provided blood and urine samples, completed short questionnaires, and underwent a brief general health evaluation with the study clinician; and (3) an in-person full study visit lasting about 3 h, approximately 2–10 days after the in-person screen visit, during which participants self-administered the study drug and completed the fMRI task reported in this paper (as well as other (f)MRI and questionnaire measures reported elsewhere; Ebner et al., 2015, 2016; Horta et al., 2019; Frazier et al., 2021).
All study sessions were conducted by trained research staff and were completed at the Department of Psychology, the Institute on Aging, and the McKnight Brain Institute at the University of Florida. In-person visits started at about 9 a.m., given the fasting blood draw and diurnal hormone cycles. Participants were instructed to stay hydrated and to avoid caffeine and substance use 24 h before the in-person visits and abstain from food, exercise, and sexual activity at least 2 h before the visits. The study protocol was approved by the University of Florida Institutional Review Board (IRB#39-2013) and registered as a clinical trial with ClinicalTrials.gov (NCT01823146). Only measures considered in the present data analysis are described in detail below.
Short cognitive tasks as well as brief personality and socioemotional questionnaires were completed during the in-person screen visit and are presented in Table 1. Positive and negative affect was assessed at the start of the in-person full study visit (see Table 1 for details). This was followed by saliva sampling and self-administration of 24 IUs (one puff per nostril; IND #100,860) of either oxytocin or a placebo, which contained the same ingredients as the oxytocin spray except for the oxytocin. Treatment assignment was randomized and double-blinded, monitored by the dispensing pharmacy, and followed recommendations for standardized intranasal oxytocin administration (Guastella et al., 2013). Before self-administration, all pre-menopausal women completed a pregnancy test to assure safety.
Before entering the MRI scanner, participants underwent another MRI safety screening, received instructions on the scanning procedure, and practiced the tasks they were working on inside the scanner. The scanning session started about 45 min after drug self-administration, with brief anatomical scans and a 15-min decision-making paradigm (Frazier et al., 2021), before participants completed the Face Trustworthiness Rating Task analyzed for this study (see detailed description below). This was followed by a facial expression identification task (Horta et al., 2019), an 8-min resting state scan (Ebner et al., 2016), and a series of socioemotional questionnaires (Ebner et al., 2015), post-scan, debriefing, and compensation procedures outside the scanner.
Face Trustworthiness Rating Task
As illustrated in Figure 1, each trial started with a jittered fixation cross (range: 2–12 s, mean = 4 s), followed by an image (either a face or a scrambled face) presented in the center of the screen for 4 s, with the rating scale and respective finger use for the response presented below the image. Participants were asked to rate the trustworthiness of each face on a four-point scale (1 = Not at all trustworthy to 4 = Very trustworthy) using an MRI compatible 4-button box, while the face was on the screen, or press any of the four buttons while the scramble was on the screen. Participants’ response and reaction time for each trial were recorded. The task was presented on Experiment Builder (SR Research), separated into two functional runs (about 7 min/run) to reduce participant burden/fatigue and to account for scanner drift. A total of 202 brain images were acquired on the 3T scanner (see details below) in each run.
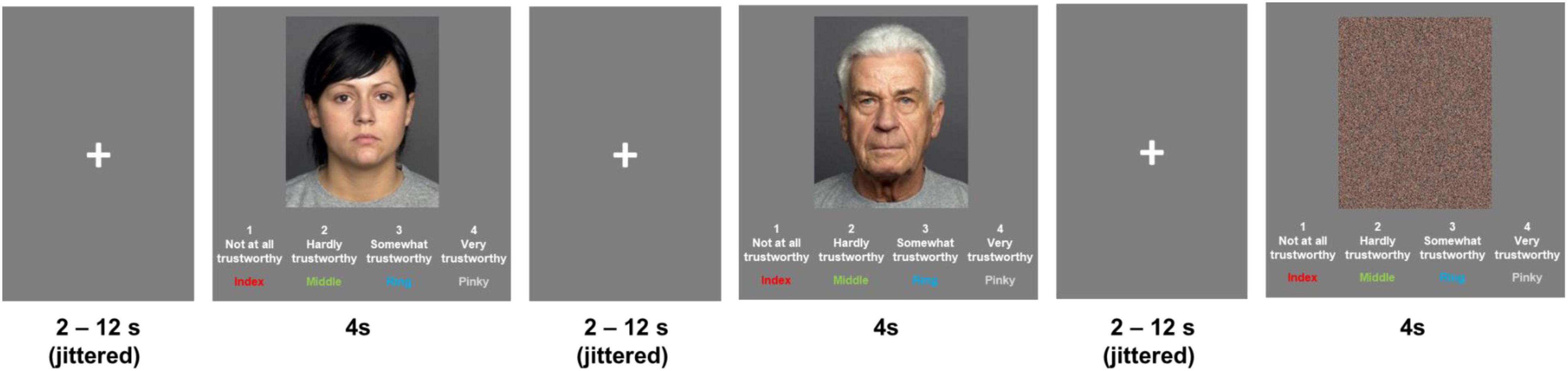
Figure 1. Face trustworthiness rating task: sample stimuli and trial timing. Facial image sources: FACES database (Ebner et al., 2010). Reproduced with permission.
Face stimuli were selected from the FACES database (Ebner et al., 2010).2 We chose 32 young (age range: 18–31 years) and 32 older (age range: 69–80 years) neutrally expressive faces with equal numbers of male and female faces per age group. Faces were evenly split into two sets based on their age and gender, with eight faces per age-by-gender group in each run. Among each set of 32 face images, 16 faces (four from each age-by-gender group) were selected to create the scrambled face images (Figure 1; also see text footnote 2). In each run, the presentation order of the 48 face images (32 face and 16 scrambled face images) was pseudorandomized so that no more than three faces of the same age-by-gender group or three scrambled face images repeated in a row.
Image Acquisition and Preprocessing
Brain images were acquired on a 3T Philips Achieva MR Scanner (Philips Medical Systems, Best, The Netherlands) with a 32-channel head coil. Whole-brain high-resolution three-dimensional T1-weighted anatomical reference images were acquired using an MP-RAGE sequence (sagittal plane, FOV = 240 × 240 × 170 mm; 1 mm3 isotropic voxels). Functional images were acquired using whole-head gradient-echo-planar imaging and single-shot gradient echo (38 interleaved slices, TR = 2 s, TE = 30 ms, FOV = 252 × 252 × 133 mm, flip angle = 90°, in-plane resolution = 80 × 80, no skip).
We applied standard preprocessing procedures using Statistical Parametric Mapping 12 (SPM 12; Welcome Department of Imaging Neuroscience). This included segmentation and spatial normalization into MNI space for structural images and slice time correction, realignment and unwarp, coregistration with structural data, spatial normalization into MNI space, resampled voxel size of 2 mm3, and smoothing with an 8 mm Gaussian kernel for functional images.
Data Analysis
Categorization of Face Stimuli
Previous work had demonstrated that amygdala response was better predicted by consensus ratings (e.g., averaged ratings across a group of individuals) than by individual ratings (Engell et al., 2007). We therefore based the levels of face trustworthiness in our analyses on norm ratings (from an independent study by Pehlivanoglu et al., 2022)3 rather than on our study participants’ individual ratings.4 In particular, Pehlivanoglu et al. (2022) comprised 87 young (M = 30.98 years, SD = 4.46, 25–39 years, 28% females), 59 middle-aged (M = 51.91 years, SD = 6.09, 44–59 years, 64% females), and 47 older (M = 65.64 years, SD = 4.22, 60–78 years, 70% females) adults who rated the trustworthiness of each face in the FACES database (“How trustworthy is this face?”) on a scale ranging from 0 (“Not at all trustworthy”) to 100 (“Extremely trustworthy”). Based on that data, we categorized the 64 face images used in the present study into five face trustworthiness levels.5
Behavioral Data
To test for age-related differences in face trustworthiness ratings (Hypothesis 1) and explore a potential oxytocin moderation on this effect, we conducted a repeated analysis of variance (ANOVA) on face trustworthiness ratings, with face trustworthiness level (categorical: 1 = least trustworthy to 5 = most trustworthy) as within-subject variable and age group (dichotomous: young vs. older) as well as treatment group (dichotomous: oxytocin vs. placebo) as between-subject variables.
Functional Magnetic Resonance Imaging Data
The functional brain data analysis comprised two steps. In the first step, we conducted a parametric analysis (Büchel et al., 1998) in SPM12 to determine voxels in the amygdala for which blood-oxygen-level-dependent (BOLD) signal was associated with face trustworthiness ratings. In particular, we created a first-level model for each participant. This model included zero-order regressors for the time series of face trials for each run. The model also included two first-order regressors (i.e., one regressor for each run) to capture the linear trend of face trustworthiness. We furthermore added 2 s-order regressors, orthogonal to the first-order regressors, to capture the quadratic (U-shaped) trend of face trustworthiness. In addition, the first-level model contained 16 regressors of no-interest: representing the time series of scramble face trials and the fixation for each run as well as six head movement parameters for each run. All regressors were convolved with the canonical hemodynamic response function (HRF). We used the general linear model approach (GLM; Friston et al., 1994) to calculate participant-specific parameter estimates pertaining to each regressor. The regressor estimates of the linear and the quadratic effects of face trustworthiness were used to create two corresponding second-level random effect models, respectively. We created two t-contrasts to identify brain activity associated with a significant negative linear and a significant positive quadratic effect, respectively.
Based on the literature, our hypotheses specifically focused on amygdala response associated with face trustworthiness. Therefore, we used small-volume correction of the p-values for the clusters in left and right amygdala that showed linear and/or quadratic effects of face trustworthiness. The amygdala mask we used for this small-volume correction was defined by the Automated Anatomical Labeling atlas (Tzourio-Mazoyer et al., 2002). For broader exploration of our dataset, we also conducted whole-brain analyses with a voxel-wise threshold of p < 0.05, FWE-corrected.
In the second step, we aimed to determine age-related differences in the associations between face trustworthiness level and BOLD activity (Hypothesis 2 and 3); as well as oxytocin moderation of these age-related differences. Thus, we created a first-level model, in which we defined regressors to represent the time series at each level of face trustworthiness per run (i.e., ten regressors in total for five levels of face trustworthiness and two runs). In addition, the model also consisted of 16 regressors of no-interest, including two for scramble faces, two for fixation, and twelve for head movement parameters. We then computed five t-contrasts to estimate BOLD signal change associated with each level of face trustworthiness. Using the Marsbar toolbox (Brett et al., 2002), we extracted parameter estimation of these t-contrasts, which represented the relative brain activation associated with each level of face trustworthiness, for each participant from clusters within the left and right amygdala that showed significant linear and/or quadratic effects of face trustworthiness. We conducted two multilevel regression models on these extracted parameter estimates, one for left and one for right amygdala, to determine age-related differences in the associations between face trustworthiness level and BOLD activity (Hypothesis 2 and 3); as well as oxytocin moderation of these age-related differences.
In each model, the face trustworthiness level and its quadratic transformation (which was orthogonal to the face trustworthiness level) served as within-subject predictors to model the linear and quadratic effects of face trustworthiness, respectively. Age group and treatment group, as well as their interaction, served as between-subject predictors in these models. We also considered the interaction between the linear and quadratic trends of face trustworthiness with age group and treatment group in the models, to assess the extent to which age group and/or treatment interacted with the linear and/or quadratic effects of face trustworthiness on amygdala activity. With reference to literature supporting sex-dimorphic effects of oxytocin (Lischke et al., 2012; Luo et al., 2017; Lieberz et al., 2020), including in aging (Ebner et al., 2015, 2016), in all analyses, participants sex (0 = male, 1 = female) served as a covariate.6
Results
Behavioral Results
The Mauchly’s test for face trustworthiness level was significant, indicating that the assumption of sphericity was violated. Therefore, we reported any effects involving this variable with Greenhouse-Geisser corrected degrees of freedom. The main effect of age group was not significant [F(1, 93) = 1.03, p = 0.31, ηp2 = 0.01], suggesting no age-related differences in overall face trustworthiness ratings. The main effect of face trustworthiness level was significant [F(3.01, 280.25) = 70.98, p < 0.001, ηp2 = 0.43], in that both young and older participants gave higher trustworthiness ratings to faces with higher levels of trustworthiness, indicating high consistency in trustworthiness ratings between the current sample and the independent norm sample (Pehlivanoglu et al., 2022). This main effect of face trustworthiness level was further qualified by a significant age moderation [F(3.01, 280.25) = 3.68, p = 0.013, ηp2 = 0.038]. In particular, older (M = 2.66, SE = 0.05) compared to young (M = 2.47, SE = 0.05) participants gave higher trustworthiness ratings to faces from the second level of face trustworthiness [F(1, 93) = 6.46, p = 0.01, ηp2 = 0.065], while the age groups did not differ in their ratings of the other four levels of trustworthiness (all ps > 0.10, Figure 2). This result partially supported Hypothesis 1 in that older participants rated somewhat untrustworthy-looking faces as relatively more trustworthy than did young participants. No other main or interaction effects were significant for face trustworthiness ratings (all ps > 0.05; see Supplementary Material for parameter estimates of all variables).
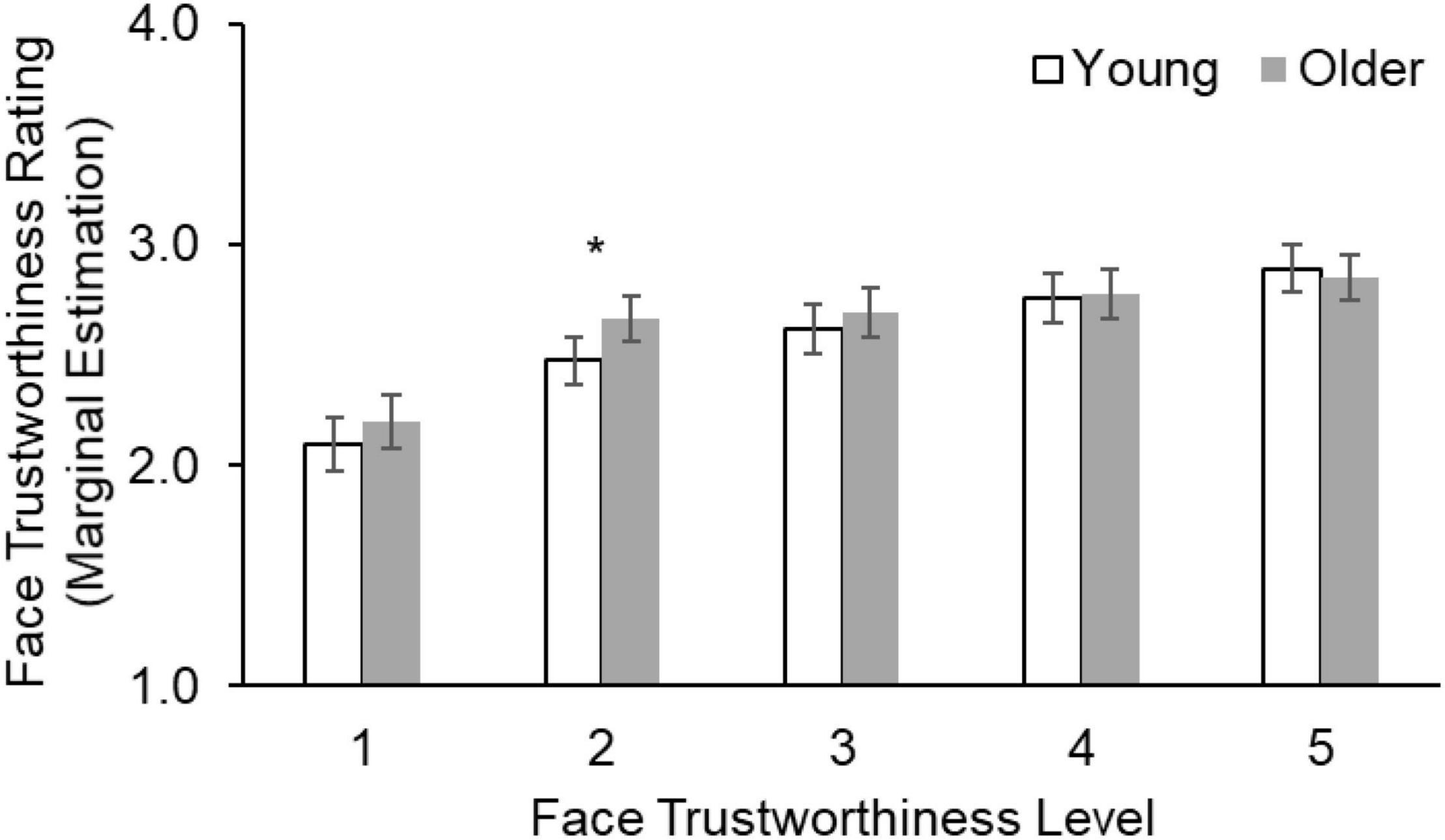
Figure 2. Face trustworthiness ratings (with higher scores indicating higher trustworthiness ratings) as a function of face trustworthiness level in young and older participants. Older compared to young participants gave higher trustworthiness ratings for “somewhat untrustworthy-looking” faces (face trustworthiness level 2). Error bars indicate 95% confidence intervals. *p < 0.05.
Neuroimaging Results
Regions of Interest Analyses
As shown in Table 2, one cluster in left amygdala showed a significant negative linear effect of face trustworthiness level, in that lower face trustworthiness ratings were associated with higher left amygdala activity. In addition, two clusters in the right amygdala showed significant positive quadratic effects of face trustworthiness level: that is, lower and higher compared to moderate face trustworthiness ratings were associated with greater right amygdala activity.
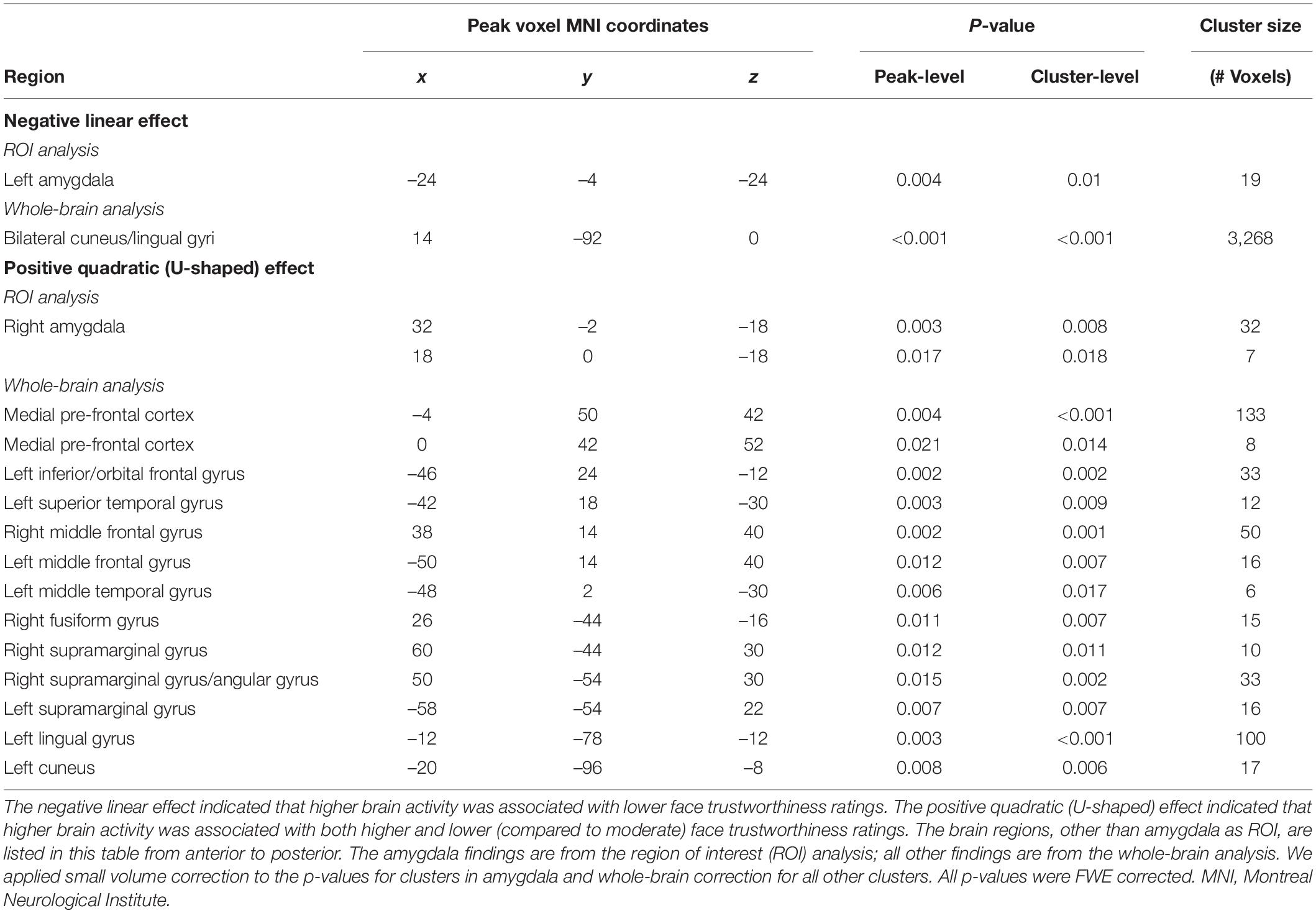
Table 2. Brain regions showing associations with face trustworthiness ratings across young and older participants.
Left Amygdala
Multilevel modeling analysis on the extracted beta values confirmed the significant negative linear effect of face trustworthiness level on left amygdala activity (B = –0.13, z = –3.55, p < 0.001, Cohen’s f2 = 0.04; Figure 3A). This negative linear effect in the left amygdala was not moderated by age group (B = 0.03, z = 0.63, p = 0.53, Cohen’s f2 = 0.001), thus not supporting Hypothesis 2 in the left amygdala. No other main or interaction effects were significant for the left amygdala (all ps > 0.05; see Supplementary Material for parameter estimates of all variables).
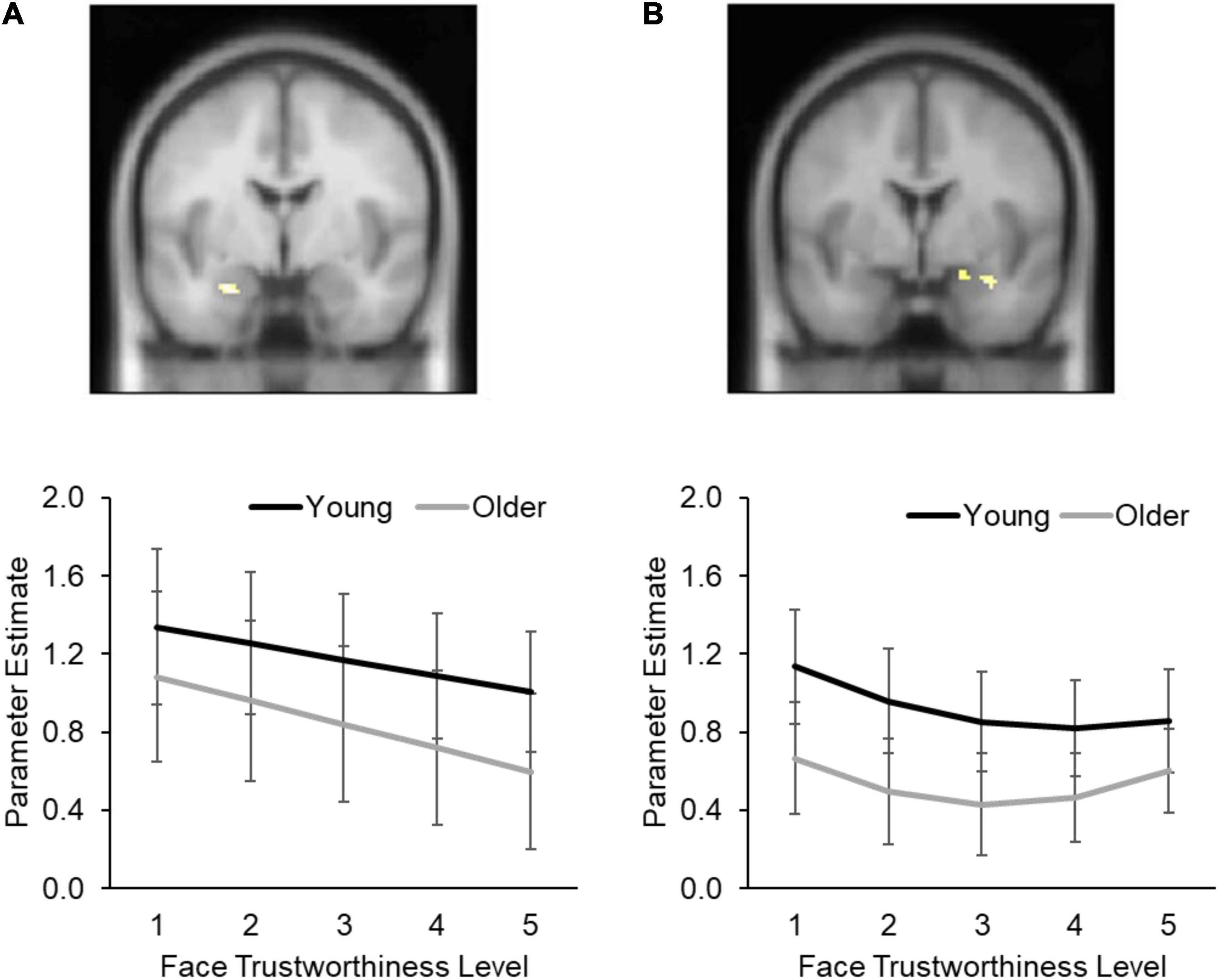
Figure 3. Amygdala response as a function of face trustworthiness in young and older participants for left (A) and right (B) amygdala. (A) In both age groups, left amygdala activity decreased with increasing face trustworthiness level (i.e., lower face trustworthiness ratings were associated with higher left amygdala activity; negative linear effect). (B) Also, for both age groups lower and higher compared to moderate face trustworthiness ratings were associated with greater right amygdala activity (positive quadratic (U-shaped) association); that is, lower and higher compared to moderate face trustworthiness were associated with greater right amygdala activity. In addition, only young participants also showed a negative linear effect of face trustworthiness level in right amygdala activity. Error bars indicate 95% confidence intervals.
Right Amygdala
Multilevel modeling analysis on the extracted beta values confirmed the significant positive quadratic effect of face trustworthiness level on right amygdala activity (B = 0.50, z = 1.98, p = 0.047, Cohen’s f2 = 0.02; Figure 3B). This positive quadratic effect in the right amygdala was not moderated by age group (B = 0.04, z = 1.09, p = 0.28, Cohen’s f2 < 0.001). This finding supported Hypothesis 3 in the right amygdala, in that both young and older participants showed greater right amygdala activity for higher and lower face trustworthiness compared to moderate face trustworthiness (i.e., positive quadratic effect; Figure 3B).
There also was a significant negative linear effect of face trustworthiness level on right amygdala activity (B = –0.10, z = –3.26, p = 0.001, Cohen’s f2 = 0.01). This negative linear effect was further qualified by an age-group moderation (B = 0.09, z = 2.17, p = 0.03, Cohen’s f2 = 0.01), confirming Hypothesis 2 for right amygdala. That is, young but not older participants showed greater right amygdala activity to faces for lower face trustworthiness (i.e., Figure 3B).
The interaction between age group, treatment group, and the linear effect of face trustworthiness was not significant (B = –0.08, z = –1.09, p = 0.28, Cohen’s f2 = 0.003), thus not supporting an oxytocin moderation on age-related differences in the negative linear association between face trustworthiness and right amygdala activity. No other main or interaction effects were significant for right amygdala (all ps > 0.05; see Supplementary Material for parameter estimates of all variables).
Whole-Brain Exploratory Analysis
An exploratory whole-brain analysis showed a negative linear effect of face trustworthiness level on activity in a cluster encompassing bilateral lingual gyrus and cuneus in both age groups. In addition, a positive quadratic effect of face trustworthiness level on brain activity was observed in various regions including the medial prefrontal cortex, bilateral middle frontal gyri, left superior temporal sulcus (e.g., superior and middle temporal gyrus), bilateral supramarginal gyrus, left lingual gyrus, left cuneus, and right fusiform gyrus (Table 2).
Discussion
This study examined age-related differences in face trustworthiness evaluation and associated amygdala activity and probed the moderating role of a single dose of intranasal oxytocin on these effects. The study generated three main findings. First, older compared to young participants rated somewhat untrustworthy-looking faces as more trustworthy (while the age groups gave comparable face trustworthiness ratings overall). Second, young participants showed greater bilateral amygdala activity to untrustworthy- relative to trustworthy-looking faces, but this effect was only present in left but not in right amygdala in older participants. Furthermore, both age groups showed a positive quadratic (U-shaped) association between right amygdala activity and face trustworthiness ratings, in that both high and low (relative to moderately) trustworthy-looking faces were associated with greater right amygdala activity. Third, in this study, intranasal oxytocin did not moderate face trustworthiness ratings or associated amygdala activity in either of the age groups. Next, we discuss empirical and theoretical implications of these novel findings.
Higher Trustworthiness Ratings for Ambiguously Untrustworthy-Looking Faces in Older Than Young Adults
Our finding that older compared to young adults evaluated somewhat untrustworthy-looking faces as more trustworthy is in line with emerging evidence of decreased sensitivity to deceptive cues in aging (Ruffman et al., 2012; Frazier et al., 2021; see Ebner et al., 2022, for an overview). This finding also suggests that the age-related positivity effect (Reed et al., 2014) applies to face trustworthiness evaluation (see also Zebrowitz et al., 2013, 2017), in that older adults rated untrustworthy-looking faces, as faces depicting negative facial cues, as less negative/more positive than young adults did.
Previous studies have reported comparable findings (i.e., relatively more favorable ratings for untrustworthy-looking faces from older than young adults; Castle et al., 2012; Zebrowitz et al., 2017; Cassidy et al., 2019; see also Bailey et al., 2016). Our study importantly qualifies this previous work by demonstrating that higher trustworthiness ratings were only given for somewhat untrustworthy-looking faces, but not for very untrustworthy-looking faces. While both signal negative facial cues, somewhat untrustworthy-looking faces display ambiguous (negative) facial cues. Thus, it is possible that the positivity effect in aging for face trustworthiness is selective; that is, older adults may be as sensitive as young adults to negative/deceptive cues in non-ambivalent faces, but not in ambivalent faces.
In fact, supporting this interpretation, ambivalent information requires rather complex cognitive operations such as interference resolution (e.g., attending to specific aspects of a stimulus while ignoring (salient) other aspects; Stanley and Blanchard-Fields, 2008; O’Connor et al., 2019). Given age-related decline in working memory (Pehlivanoglu et al., 2014; for a meta-analysis see, Bopp and Verhaeghen, 2007) combined with age-related reduction in sensitivity to deceptive cues (Castle et al., 2012) and an increased positivity effect with age (Carstensen and Mikels, 2005), older participants may have had difficulty in focusing on cues of untrustworthiness while filtering out cues of trustworthiness in ambiguously untrustworthy-looking faces. In line with this explanation, other studies showed that older compared to young adults used fewer negative words to describe ambiguous scenarios (Juang and Knight, 2016; Mikels and Shuster, 2016) and gave more positive evaluations to ambiguous facial expressions (i.e., surprise; Neta and Tong, 2016; Neta et al., 2018).
This age-related reduced differentiation when confronted with ambiguously negative (untrustworthy) cues may be reflective of older adults’ perceptual dedifferentiation (Park et al., 2012) and/or may reflect a shift in decision criteria in trust-related decision making. That is, when cues of untrustworthiness are not distinct, older adults may not pick up on them, and perhaps particularly so for negative cues. In support of this interpretation, age-related dedifferentiation in face emotion recognition is greater/or only occurs for negative (e.g., anger, disgust) but not positive or neutral expressions (Franklin and Zebrowitz, 2017).
Lateralization and Age Effects in the Association Between Amygdala Response and Face Trustworthiness
Consistent with previous findings in young adults (Said et al., 2009; Freeman et al., 2014; see Santos et al., 2016, for a meta-analysis), we identified a complex pattern of amygdala response to face trustworthiness in our age-heterogeneous sample, which qualifies prior work (Said et al., 2009). In particular, we observed a lateralization in amygdala response to facial trustworthiness that was further moderated by age, as discussed next.
Comparable across both age groups, right amygdala response was greater to more and less trustworthy-looking than to moderately trustworthy-looking faces (i.e., U-shaped association). In left amygdala, in contrast, we observed a negative linear association; in that left amygdala activity decreased with increasing face trustworthiness. Right amygdala has been shown to mediate unconscious processing of emotional information, while left amygdala has been associated with conscious processing of emotional stimuli (Morris et al., 1998, 1999; Allen et al., 2021). Also, a U-shaped amygdala response to face trustworthiness has been observed in implicit tasks (Mattavelli et al., 2012; Rule et al., 2013) or when stimuli were presented so briefly (e.g., 200 ms) that elaborate processing was not possible (Freeman et al., 2014). Mapping onto these previous findings, the U-shaped association between right amygdala response and face trustworthiness ratings in our study may reflect more implicit processing, while the negative linear association between left amygdala response and face trustworthiness ratings may reflect more explicit processing.
Further, supporting an age-differential response pattern in right amygdala, we found that young but not older participants showed a negative linear trend in addition to the U-shaped association. As a result, young but not older participants showed enhanced right amygdala activity to less untrustworthy-looking faces. As amygdala response toward face trustworthiness may reflect the level of social salience (Sander et al., 2003; Vuilleumier, 2009), which further signal approach/avoidance behavior (Todorov, 2008; Todorov and Engell, 2008), age-differential linear response in right amygdala could be interpreted as suggesting greater salience of untrustworthy-looking faces among young but not older adults (or at least, that salience of untrustworthy-looking faces is reduced among older relative to young adults). Thus, our amygdala findings are in accord with brain data on the positivity effect suggesting reduced response to negative but not positive information (Mather et al., 2004; see also Reed and Carstensen, 2012, for an overview).
Visual inspection of the two clusters identified in left and right amygdala suggested their location in the lateral nuclei, known as the gateway of amygdala, that receive perceptual input (LeDoux, 2007). Stronger magnetic field imaging such as with a 7T scanner in future studies will allow enhanced localization (Vu et al., 2017) and facilitate interpretation of the processes underlying face trustworthiness evaluation in young and older adults.
Oxytocin Did Not Modulate Face Trustworthiness Evaluation in Brain or Behavior
In this study we found no evidence of a moderation of face trustworthiness evaluation by intranasal oxytocin, neither in behavior nor in brain response. Oxytocin has been promoted as crucial modulator of social cognition and behavior (Harari-Dahan and Bernstein, 2014; Quintana et al., 2018), including in aging (Horta et al., 2020), and has been discussed in the context of trustworthiness perception (Van IJzendoorn and Bakermans-Kranenburg, 2012). However, some empirical work does not support such effects (Lambert et al., 2014; Grainger et al., 2018; see also Declerck et al., 2020). The present study findings align with studies that do not support an effect of a single dose of intranasal oxytocin on face trustworthiness evaluation and extend this null effect to older adults (see Grainger et al., 2019).
There are various possible explanations for the observed non-significant effects of oxytocin on face trustworthiness in brain and behavior. The present study used faces with neutral expressions, which may have reduced emotional reaction/approach-avoidance tendencies to the images. Some of the previous studies that found social-cognitive effects of oxytocin used strong social (e.g., in-group vs. out-group; De Dreu et al., 2010) and emotional (e.g., emotional expression; Horta et al., 2019) manipulations in their experimental designs. It is possible that stimuli with affluent social and emotional content facilitate and/or enhance oxytocin’s social-cognitive effects.
Also, the majority of faces used in the present study fell somewhere in the middle of the trustworthiness spectrum, as they were retrieved from a naturalistic set of images taken from real people (see details about the FACES database in Ebner et al., 2010). The present study’s 4-point rating scale with two extreme, opposite ends (i.e., very untrustworthy to very trustworthy) may have had limited sensitivity to differentiate along trustworthiness among this set of naturalistic images and thus also limited our ability to model the BOLD signal along this dimension. For example, with a comparable sample size of 96 participants who underwent a single dose of either intranasal oxytocin or placebo and using neutral faces as stimuli but a 7-point trustworthiness rating scale, Theodoridou et al. (2009) found higher face trustworthiness ratings in the oxytocin than the placebo group. Future studies will be able to test if such methodological differences underlie variability in detecting oxytocin effects on face trustworthiness evaluation.
Moving forward, studies with a larger sample size are warranted to follow up on the oxytocin null effects observed in the present study. In fact, a recent meta-analysis (Leppanen et al., 2017), which was published after completion of the present data collection and suggested that for a study with oxytocin vs. placebo administration as between-subject factor a sample size of 64 participants per treatment group is necessary to obtain sufficient power (80%) to detect a moderate effect of oxytocin on social cognition (see Supplementary Material for details regarding the interaction we observed between treatment group and face trustworthiness level, which were not statistical significant as per our alpha level cut off, but approached significance). While randomized for treatment group assignment, the present study used a between-subject design and all measures were collected after treatment administration. Future extension of this work would benefit from a cross-over design to account for possible individual differences at baseline. In fact, following recent recommendations (Naselaris et al., 2021), within-subject designs are better suited as their higher sampling of a more limited number of subjects compared to the smaller sampling of a larger number of individuals in a between-subject design are more powerful to detect significant effects. This emphasis on depth of assessment in individual brains over breadth of assessment among multiple brains is also beneficial for enhancing resolution and the signal-to-noise ratio in neuroimaging analysis, and thus a fruitful path forward.
Conclusion
The present study extends previous work by demonstrating age-related differences in face trustworthiness ratings for ambiguously untrustworthy-looking faces. The study also demonstrates lateralization as well as age effects in amygdala response underlying face trustworthiness evaluation. In sum, this work supports the notion of reduced sensitivity/dampened response to cues of untrustworthiness in aging and supports an extension of the age-related positivity effect to the domain of face trustworthiness evaluation. Finally, the paper adds to a growing literature of possible null effects of single-dose intranasal oxytocin administration on face trustworthiness perception.
Data Availability Statement
The raw data supporting the conclusions of this article will be made available by the authors, without undue reservation.
Ethics Statement
The studies involving human participants were reviewed and approved by the University of Florida Institutional Review Board. The patients/participants provided their written informed consent to participate in this study.
Author Contributions
TL: conceptualization, methodology, data collection, formal analysis, writing original draft, and revising. DP: conceptualization, data collection, writing original draft, and revising. MZ: methodology and revising. PL: methodology, writing original draft, and revising. AW, DF, and HF: conceptualization, methodology, and revising. NE: conceptualization, methodology, formal analysis, writing original draft, and revising. All authors contributed to the article and approved the submitted version.
Funding
This work was supported by the University of Florida Clinical and Translational Science pilot award (NIH/NCATS, UL1 TR000064), the Scientific Research Network on Decision Neuroscience and Aging pilot award (NIH/NIA, R24 AG039350), the National Institute on Aging grants R01AG059809, R01AG057764, and R01AG072658, the Florida Department of Health Ed and Ethel Moore Alzheimer’s Disease Research Program grant 22A12, as well as the College of Liberal Arts and Sciences, the Department of Psychology, the Center for Cognitive Aging and Memory, and the Claude D. Pepper Older Americans Independence Center at the University of Florida. A portion of this work was performed in the McKnight Brain Institute at the National High Magnetic Field Laboratory’s AMRIS Facility, supported by the National Science Foundation Cooperative Agreement No. DMR-1157490 and the State of Florida.
Conflict of Interest
The authors declare that the research was conducted in the absence of any commercial or financial relationships that could be construed as a potential conflict of interest.
Publisher’s Note
All claims expressed in this article are solely those of the authors and do not necessarily represent those of their affiliated organizations, or those of the publisher, the editors and the reviewers. Any product that may be evaluated in this article, or claim that may be made by its manufacturer, is not guaranteed or endorsed by the publisher.
Acknowledgments
We would like to thank the members of the Social-Cognitive and Affective Developmental Lab, the staff at the McKnight Brain Institute at the National High Magnetic Field Laboratory’s AMRIS Facility, and the faculty and staff at the Institute on Aging at University of Florida for support in study logistics and data collection. We would also like to thank the study pharmacist Susan E. Beltz and the study MD Bhanuprasad Sandesara for their roles in drug-related study logistics.
Supplementary Material
The Supplementary Material for this article can be found online at: https://www.frontiersin.org/articles/10.3389/fpsyg.2022.838642/full#supplementary-material
Footnotes
- ^ A total of 7 participants (4 young; 3 older) did not complete the functional magnetic resonance imaging (fMRI) task due to technical issues, time limitations, or drop out after the screening session.
- ^ https://faces.mpdl.mpg.de/imeji/
- ^ https://psyarxiv.com/rknu2/
- ^ The correlation between the present study’s subjective face trustworthiness ratings and the face trustworthiness ratings (norm ratings) from Pehlivanoglu et al. (2022) was high (r = 0.72), supporting strong convergence across the two studies.
- ^ This approach allowed us to model both linear and quadratic effects of face trustworthiness on brain activity, while maintaining sufficient number of trials within each face trustworthiness level for robust estimation.
- ^ We conducted parallel analyses with sex as a moderator. None of the interactions with sex were significant, thus not supporting a moderation effect of sex on the results reported here.
References
Adolphs, R., Tranel, D., and Damasio, A. R. (1998). The human amygdala in social judgment. Nature 393, 470–474. doi: 10.1038/30982
Allen, H. N., Bobnar, H. J., and Kolber, B. J. (2021). Left and right hemispheric lateralization of the amygdala in pain. Prog. Neurobiol. 196:101891. doi: 10.1016/j.pneurobio.2020.101891
Bailey, P. E., and Leon, T. (2019). A systematic review and meta-analysis of age-related differences in trust. Psychol. Aging 34, 674–680. doi: 10.1037/pag0000368
Bailey, P. E., Szczap, P., McLennan, S. N., Slessor, G., Ruffman, T., and Rendell, P. G. (2016). Age-related similarities and differences in first impressions of trustworthiness. Cogn. Emot. 30, 1017–1026. doi: 10.1080/02699931.2015.1039493
Baumgartner, T., Heinrichs, M., Vonlanthen, A., Fischbacher, U., and Fehr, E. (2008). Oxytocin shapes the neural circuitry of trust and trust adaptation in humans. Neuron 58, 639–650. doi: 10.1016/j.neuron.2008.04.009
Bopp, K. L., and Verhaeghen, P. (2007). Age-related differences in control processes in verbal and visuospatial working memory: storage, transformation, supervision, and coordination. J. Gerontol. B Psychol. Sci. Soc. Sci. 62, 239–246. doi: 10.1093/geronb/62.5.p239
Born, J., Lange, T., Kern, W., McGregor, G. P., Bickel, U., and Fehm, H. L. (2002). Sniffing neuropeptides: a transnasal approach to the human brain. Nat. Neurosci. 5, 514–516. doi: 10.1038/nn849
Brandt, J., Spencer, M., and Folstein, M. (1988). The telephone interview for cognitive status. Neuropsychiatry Neuropsychol. Behav. Neurol. 1, 111–117. doi: 10.3171/2020.6.JNS20638
Brett, M., Anton, J. L., Valabregue, R., and Poline, J. B. (2002). “Region of interest analysis using an SPM toolbox [abstract],” in Proceedings of the 8th International Conference on Functional Mapping of the Human Brain, Sendai.
Büchel, C., Holmes, A. P., Rees, G., and Friston, K. J. (1998). Characterizing stimulus–response functions using nonlinear regressors in parametric fMRI experiments. Neuroimage 8, 140–148. doi: 10.1006/nimg.1998.0351
Bzdok, D., Langner, R., Caspers, S., Kurth, F., Habel, U., Zilles, K., et al. (2011). ALE meta-analysis on facial judgments of trustworthiness and attractiveness. Brain Struct. Funct. 215, 209–223. doi: 10.1007/s00429-010-0287-4
Campbell-Smith, E. J., Holmes, N. M., Lingawi, N. W., Panayi, M. C., and Westbrook, R. F. (2015). Oxytocin signaling in basolateral and central amygdala nuclei differentially regulates the acquisition, expression, and extinction of context-conditioned fear in rats. Learn. Mem. 22, 247–257. doi: 10.1101/lm.036962.114
Carstensen, L. L., and Mikels, J. A. (2005). At the intersection of emotion and cognition: aging and the positivity effect. Curr. Dir. Psychol. Sci. 14, 117–121. doi: 10.1038/s41598-021-81728-0
Carstensen, L. L., Turan, B., Scheibe, S., Ram, N., Ersner-Hershfield, H., Samanez-Larkin, G. R., et al. (2011). Emotional experience improves with age: evidence based on over 10 years of experience sampling. Psychol. Aging 26, 21–33. doi: 10.1037/a0021285
Cassidy, B. S., Boucher, K. L., Lanie, S. T., and Krendl, A. C. (2019). Age effects on trustworthiness activation and trust biases in face perception. J. Gerontol. B 74, 87–92. doi: 10.1093/geronb/gby062
Castle, E., Eisenberger, N. I., Seeman, T. E., Moons, W. G., Boggero, I. A., Grinblatt, M. S., et al. (2012). Neural and behavioral bases of age differences in perceptions of trust. Proc. Natl. Acad. Sci. U.S.A. 109, 20848–20852. doi: 10.1073/pnas.1218518109
Cohen, J. (2013). Statistical Power Analysis for the Behavioral Sciences. Cambridge, MA: Academic press.
De Dreu, C. K., Greer, L. L., Handgraaf, M. J., Shalvi, S., Van Kleef, G. A., Baas, M., et al. (2010). The neuropeptide oxytocin regulates parochial altruism in intergroup conflict among humans. Science 328, 1408–1411. doi: 10.1126/science.1189047
Declerck, C. H., Boone, C., Pauwels, L., Vogt, B., and Fehr, E. (2020). A registered replication study on oxytocin and trust. Nat. Hum. Behav. 4, 646–655. doi: 10.1038/s41562-020-0878-x
Desikan, R. S., Ségonne, F., Fischl, B., Quinn, B. T., Dickerson, B. C., Blacker, D., et al. (2006). An automated labeling system for subdividing the human cerebral cortex on MRI scans into gyral based regions of interest. Neuroimage 31, 968–980. doi: 10.1016/j.neuroimage.2006.01.021
Ebner, N., Horta, M., Lin, T., Fischer, H., Cohen, R., and Feifel, D. (2015). Oxytocin modulates meta-mood as a function of age and sex. Front. Aging Neurosci. 7:175. doi: 10.3389/fnagi.2015.00175
Ebner, N. C., Chen, H., Porges, E., Lin, T., Fischer, H., Feifel, D., et al. (2016). Oxytocin’s effect on resting-state functional connectivity varies by age and sex. Psychoneuroendocrinology 69, 50–59. doi: 10.1016/j.psyneuen.2016.03.013
Ebner, N. C., Pehlivanoglu, D., Polk, R., Turner, G. R., and Spreng, R. N. (2022). “Aging online: rethinking the aging decision maker in a digital era,” in A Fresh look at Fraud: Theoretical and Applied Approaches (Behavioral Economics and Healthy Behaviors), eds Y. Hanoch and S. Wood (Milton Park: Routledge Taylor Francis).
Ebner, N. C., Riediger, M., and Lindenberger, U. (2010). FACES—A database of facial expressions in young, middle-aged, and older women and men: development and validation. Behav. Res. Methods 42, 351–362. doi: 10.3758/BRM.42.1.351
Engell, A. D., Haxby, J. V., and Todorov, A. (2007). Implicit trustworthiness decisions: automatic coding of face properties in the human amygdala. J. Cogn. Neurosci. 19, 1508–1519. doi: 10.1162/jocn.2007.19.9.1508
Franklin, R. G. Jr., and Zebrowitz, L. A. (2017). Age differences in emotion recognition: task demands or perceptual dedifferentiation? Exp. Aging Res. 43, 453–466. doi: 10.1080/0361073X.2017.1369628
Frazier, I., Lin, T., Liu, P., Skarsten, S., Feifel, D., and Ebner, N. C. (2021). Age and intranasal oxytocin effects on trust-related decisions after breach of trust: behavioral and brain evidence. Psychol. Aging 36, 10–21. doi: 10.1037/pag0000545
Freeman, J. B., Stolier, R. M., Ingbretsen, Z. A., and Hehman, E. A. (2014). Amygdala responsivity to high-level social information from unseen faces. J. Neurosci. 34, 10573–10581. doi: 10.1523/JNEUROSCI.5063-13.2014
Friston, K. J., Holmes, A. P., Worsley, K. J., Poline, J. P., Frith, C. D., and Frackowiak, R. S. (1994). Statistical parametric maps in functional imaging: a general linear approach. Hum. Brain Mapping 2, 189–210. doi: 10.1016/j.neuroimage.2007.01.029
Grainger, S. A., Henry, J. D., Steinvik, H. R., and Vanman, E. J. (2019). Intranasal oxytocin does not alter initial perceptions of facial trustworthiness in younger or older adults. J. Psychopharmacol. 33, 250–254. doi: 10.1177/0269881118806303
Grainger, S. A., Henry, J. D., Steinvik, H. R., Vanman, E. J., Rendell, P. G., and Labuschagne, I. (2018). Intranasal oxytocin does not reduce age-related difficulties in social cognition. Horm. Behav. 99, 25–34. doi: 10.1016/j.yhbeh.2018.01.009
Guastella, A. J., Hickie, I. B., McGuinness, M. M., Otis, M., Woods, E. A., Disinger, H. M., et al. (2013). Recommendations for the standardisation of oxytocin nasal administration and guidelines for its reporting in human research. Psychoneuroendocrinology 38, 612–625. doi: 10.1016/j.psyneuen.2012.11.019
Guastella, A. J., Mitchell, P. B., and Dadds, M. R. (2008). Oxytocin increases gaze to the eye region of human faces. Biol. Psychiatry 63, 3–5.
Harari-Dahan, O., and Bernstein, A. (2014). A general approach-avoidance hypothesis of oxytocin: accounting for social and non-social effects of oxytocin. Neurosci. Biobehav. Rev. 47, 506–519. doi: 10.1016/j.neubiorev.2014.10.007
Horta, M., Pehlivanoglu, D., and Ebner, N. C. (2020). The role of intranasal oxytocin on social cognition: an integrative human lifespan approach. Curr. Behav. Neurosci. Rep. 7, 175–192. doi: 10.1007/s40473-020-00214-5
Horta, M., Ziaei, M., Lin, T., Porges, E. C., Fischer, H., Feifel, D., et al. (2019). Oxytocin alters patterns of brain activity and amygdalar connectivity by age during dynamic facial emotion identification. Neurobiol. Aging 78, 42–51. doi: 10.1016/j.neurobiolaging.2019.01.016
Juang, C., and Knight, B. G. (2016). Age differences in interpreting ambiguous situations: the effects of content themes and depressed mood. J. Gerontol. B Psychol. Sci. Soc. Sci. 71, 1024–1033. doi: 10.1093/geronb/gbv037
Keech, B., Crowe, S., and Hocking, D. R. (2018). Intranasal oxytocin, social cognition and neurodevelopmental disorders: a meta-analysis. Psychoneuroendocrinology 87, 9–19. doi: 10.1016/j.psyneuen.2017.09.022
Kirsch, P., Esslinger, C., Chen, Q., Mier, D., Lis, S., Siddhanti, S., et al. (2005). Oxytocin modulates neural circuitry for social cognition and fear in humans. J. Neurosci. 25, 11489–11493. doi: 10.1523/JNEUROSCI.3984-05.2005
Kosfeld, M., Heinrichs, M., Zak, P. J., Fischbacher, U., and Fehr, E. (2005). Oxytocin increases trust in humans. Nature 435, 673–676.
Labuschagne, I., Phan, K. L., Wood, A., Angstadt, M., Chua, P., Heinrichs, M., et al. (2010). Oxytocin attenuates amygdala reactivity to fear in generalized social anxiety disorder. Neuropsychopharmacology 35, 2403–2413. doi: 10.1038/npp.2010.123
Lambert, B., Declerck, C. H., and Boone, C. (2014). Oxytocin does not make a face appear more trustworthy but improves the accuracy of trustworthiness judgments. Psychoneuroendocrinology 40, 60–68. doi: 10.1016/j.psyneuen.2013.10.015
Leclerc, C. M., and Kensinger, E. A. (2008). Effects of age on detection of emotional information. Psychol. Aging 23, 209–215. doi: 10.1037/0882-7974.23.1.209
Leppanen, J., Ng, K. W., Tchanturia, K., and Treasure, J. (2017). Meta-analysis of the effects of intranasal oxytocin on interpretation and expression of emotions. Neurosci. Biobehav. Rev. 78, 125–144. doi: 10.1016/j.neubiorev.2017.04.010
Lieberz, J., Scheele, D., Spengler, F. B., Matheisen, T., Schneider, L., Stoffel-Wagner, B., et al. (2020). Kinetics of oxytocin effects on amygdala and striatal reactivity vary between women and men. Neuropsychopharmacology 45, 1134–1140. doi: 10.1038/s41386-019-0582-6
Lin, T., Liu, G. A., Perez, E., Rainer, R. D., Febo, M., Cruz-Almeida, Y., et al. (2018). Systemic inflammation mediates age-related cognitive deficits. Front. Aging Neurosci. 10:236. doi: 10.3389/fnagi.2018.00236
Lischke, A., Gamer, M., Berger, C., Grossmann, A., Hauenstein, K., Heinrichs, M., et al. (2012). Oxytocin increases amygdala reactivity to threatening scenes in females. Psychoneuroendocrinology 37, 1431–1438. doi: 10.1016/j.psyneuen.2012.01.011
Luo, L., Becker, B., Geng, Y., Zhao, Z., Gao, S., Zhao, W., et al. (2017). Sex-dependent neural effect of oxytocin during subliminal processing of negative emotion faces. Neuroimage 162, 127–137. doi: 10.1016/j.neuroimage.2017.08.079
MacDonald, K., and MacDonald, T. M. (2010). The peptide that binds: a systematic review of oxytocin and its prosocial effects in humans. Harv. Rev. Psychiatry 18, 1–21. doi: 10.3109/10673220903523615
Mather, M., Canli, T., English, T., Whitfield, S., Wais, P., Ochsner, K., et al. (2004). Amygdala responses to emotionally valenced stimuli in older and younger adults. Psychol. Sci. 15, 259–263. doi: 10.1111/j.0956-7976.2004.00662.x
Mattavelli, G., Andrews, T. J., Asghar, A. U., Towler, J. R., and Young, A. W. (2012). Response of face-selective brain regions to trustworthiness and gender of faces. Neuropsychologia 50, 2205–2211. doi: 10.1016/j.neuropsychologia.2012.05.024
Meyer-Lindenberg, A. (2008). Impact of prosocial neuropeptides on human brain function. Prog. Brain Res. 170, 463–470. doi: 10.1016/S0079-6123(08)00436-6
Mikels, J. A., and Shuster, M. M. (2016). The interpretative lenses of older adults are not rose-colored—just less dark: aging and the interpretation of ambiguous scenarios. Emotion 16, 94–100. doi: 10.1037/emo0000104
Mikolajczak, M., Pinon, N., Lane, A., de Timary, P., and Luminet, O. (2010). Oxytocin not only increases trust when money is at stake, but also when confidential information is in the balance. Biol. Psychol. 85, 182–184. doi: 10.1016/j.biopsycho.2010.05.010
Morris, J. S., Öhman, A., and Dolan, R. J. (1998). Conscious and unconscious emotional learning in the human amygdala. Nature 393, 467–470. doi: 10.1038/30976
Morris, J. S., Öhman, A., and Dolan, R. J. (1999). A subcortical pathway to the right amygdala mediating “unseen” fear. Proc. Natl. Acad. Sci. U.S.A. 96, 1680–1685. doi: 10.1073/pnas.96.4.1680
Naselaris, T., Allen, E., and Kay, K. (2021). Extensive sampling for complete models of individual brains. Curr. Opin. Behav. Sci. 40, 45–51.
Neta, M., Tong, T. T., and Henley, D. J. (2018). It’s a matter of time (perspectives): shifting valence responses to emotional ambiguity. Motiv. Emot. 42, 258–266.
O’Connor, A. M., Lyon, T. D., and Evans, A. D. (2019). Younger and older adults’ lie-detection and credibility judgments of children’s coached reports. Psychol. Crime Law 25, 925–944. doi: 10.1080/1068316x.2019.1597092
Olff, M., Frijling, J. L., Kubzansky, L. D., Bradley, B., Ellenbogen, M. A., Cardoso, C., et al. (2013). The role of oxytocin in social bonding, stress regulation and mental health: an update on the moderating effects of context and interindividual differences. Psychoneuroendocrinology 38, 1883–1894.
Park, J., Carp, J., Kennedy, K. M., Rodrigue, K. M., Bischof, G. N., Huang, C. M., et al. (2012). Neural broadening or neural attenuation? Investigating age-related dedifferentiation in the face network in a large lifespan sample. J. Neurosci. 32, 2154–2158. doi: 10.1523/JNEUROSCI.4494-11.2012
Pehlivanoglu, D., Jain, S., Ariel, R., and Verhaeghen, P. (2014). The ties to unbind: age-related differences in feature (un) binding in working memory for emotional faces. Front. Psychol. 5:253. doi: 10.3389/fpsyg.2014.00253
Pehlivanoglu, D., Lin, T., Lighthall, N., Wilson, R., Turner, G. R., Spreng, R. N., et al. (2022). Facial Trustworthiness Perceptions Across the Adult Lifespan. Available online at: https://psyarxiv.com/rknu2/° (accessed May 20, 2022).
Pehlivanoglu, D., and Verhaeghen, P. (2019). Now you feel it, now you don’t: motivated attention to emotional content is modulated by age and task demands. Cogn. Affect. Behav. Neurosci. 19, 1299–1316. doi: 10.3758/s13415-019-00741-z
Plasencia, G., Luedicke, J. M., Nazarloo, H. P., Carter, C. S., and Ebner, N. C. (2019). Plasma oxytocin and vasopressin levels in young and older men and women: functional relationships with attachment and cognition. Psychoneuroendocrinology 110:104419. doi: 10.1016/j.psyneuen.2019.104419
Quintana, D. S., Smerud, K. T., Andreassen, O. A., and Djupesland, P. G. (2018). Evidence for intranasal oxytocin delivery to the brain: recent advances and future perspectives. Ther. Deliv. 9, 515–525. doi: 10.4155/tde-2018-0002
Reed, A. E., and Carstensen, L. L. (2012). The theory behind the age-related positivity effect. Front. Psychol. 3:339. doi: 10.3389/fpsyg.2012.00339
Reed, A. E., Chan, L., and Mikels, J. A. (2014). Meta-analysis of the age-related positivity effect: age differences in preferences for positive over negative information. Psychol. Aging 29, 1–15. doi: 10.1037/a0035194
Rimmele, U., Hediger, K., Heinrichs, M., and Klaver, P. (2009). Oxytocin makes a face in memory familiar. J. Neurosci. 29, 38–42. doi: 10.1523/JNEUROSCI.4260-08.2009
Röcke, C., Li, S. C., and Smith, J. (2009). Intraindividual variability in positive and negative affect over 45 days: do older adults fluctuate less than young adults? Psychol. Aging 24, 863–878. doi: 10.1037/a0016276
Ruffman, T., Murray, J., Halberstadt, J., and Vater, T. (2012). Age-related differences in deception. Psychol. Aging 27, 543–549. doi: 10.1037/a0023380
Rule, N. O., Krendl, A. C., Ivcevic, Z., and Ambady, N. (2013). Accuracy and consensus in judgments of trustworthiness from faces: behavioral and neural correlates. J. Pers. Soc. Psychol. 104, 409–426. doi: 10.1037/a0031050
Said, C. P., Baron, S. G., and Todorov, A. (2009). Nonlinear amygdala response to face trustworthiness: contributions of high and low spatial frequency information. J. Cogn. Neurosci. 21, 519–528. doi: 10.1162/jocn.2009.21041
Sander, D., Grafman, J., and Zalla, T. (2003). The human amygdala: an evolved system for relevance detection. Rev. Neurosci. 14, 303–316.
Santos, S., Almeida, I., Oliveiros, B., and Castelo-Branco, M. (2016). The role of the amygdala in facial trustworthiness processing: a systematic review and meta-analyses of fMRI studies. PLoS One 11:e0167276. doi: 10.1371/journal.pone.0167276
Shamay-Tsoory, S. G., and Abu-Akel, A. (2016). The social salience hypothesis of oxytocin. Biol. Psychiatry 79, 194–202.
Stanley, J. T., and Blanchard-Fields, F. (2008). Challenges older adults face in detecting deceit: the role of emotion recognition. Psychol. Aging 23, 24–32. doi: 10.1037/0882-7974.23.1.24
Theodoridou, A., Rowe, A. C., Penton-Voak, I. S., and Rogers, P. J. (2009). Oxytocin and social perception: oxytocin increases perceived facial trustworthiness and attractiveness. Horm. Behav. 56, 128–132. doi: 10.1016/j.yhbeh.2009.03.019
Todorov, A. (2008). Evaluating faces on trustworthiness: an extension of systems for recognition of emotions signaling approach/avoidance behaviors. Ann. N. Y. Acad. Sci. 1124, 208–224. doi: 10.1196/annals.1440.012
Todorov, A., and Engell, A. D. (2008). The role of the amygdala in implicit evaluation of emotionally neutral faces. Soc. Cogn. Affect. Neurosci. 3, 303–312. doi: 10.1093/scan/nsn033
Tollenaar, M. S., Chatzimanoli, M., van der Wee, N. J., and Putman, P. (2013). Enhanced orienting of attention in response to emotional gaze cues after oxytocin administration in healthy young men. Psychoneuroendocrinology 38, 1797–1802. doi: 10.1016/j.psyneuen.2013.02.018
Tzourio-Mazoyer, N., Landeau, B., Papathanassiou, D., Crivello, F., Etard, O., Delcroix, N., et al. (2002). Automated anatomical labeling of activations in SPM using a macroscopic anatomical parcellation of the MNI MRI single-subject brain. Neuroimage 15, 273–289.
Van IJzendoorn, M. H., and Bakermans-Kranenburg, M. J. (2012). A sniff of trust: meta-analysis of the effects of intranasal oxytocin administration on face recognition, trust to in-group, and trust to out-group. Psychoneuroendocrinology 37, 438–443. doi: 10.1016/j.psyneuen.2011.07.008
Van Lange, P. A. (2015). Generalized trust: four lessons from genetics and culture. Curr. Dir. Psychol. Sci. 24, 71–76.
Van’t Wout, M., and Sanfey, A. G. (2008). Friend or foe: the effect of implicit trustworthiness judgments in social decision-making. Cognition 108, 796–803. doi: 10.1016/j.cognition.2008.07.002
Vu, A. T., Jamison, K., Glasser, M. F., Smith, S. M., Coalson, T., Moeller, S., et al. (2017). Tradeoffs in pushing the spatial resolution of fMRI for the 7T human connectome project. Neuroimage 154, 23–32. doi: 10.1016/j.neuroimage.2016.11.049
Vuilleumier, P. (2009). “The role of the human amygdala in perception and attention,” in The Human Amygdala, eds P. J. Whalen and E. A. Phelps (New York, NY: The Guilford Press).
Watson, D., Clark, L. A., and Tellegen, A. (1988). Development and validation of brief measures of positive and negative affect: the PANAS scales. J. Pers. Soc. Psychol. 54, 1063–1070.
Wechsler, D. (1981). WAIS-R: Manual: Wechsler Adult Intelligence Scale—Revised. New York, NY: Psychological Corporation.
Willis, J., and Todorov, A. (2006). First impressions: making up your mind after a 100-ms exposure to a face. Psychol. Sci. 17, 592–598. doi: 10.1111/j.1467-9280.2006.01750.x
Winston, J. S., Strange, B. A., O’Doherty, J., and Dolan, R. J. (2002). Automatic and intentional brain responses during evaluation of trustworthiness of faces. Nat. Neurosci. 5, 277–283. doi: 10.1038/nn816
Zebrowitz, L. A., Boshyan, J., Ward, N., Gutchess, A., and Hadjikhani, N. (2017). The older adult positivity effect in evaluations of trustworthiness: emotion regulation or cognitive capacity? PLoS One 12:e0169823. doi: 10.1371/journal.pone.0169823
Zebrowitz, L. A., Franklin, R. G. Jr., Hillman, S., and Boc, H. (2013). Older and younger adults’ first impressions from faces: similar in agreement but different in positivity. Psychol. Aging 28, 202–212. doi: 10.1037/a0030927
Zebrowitz, L. A., Ward, N., Boshyan, J., Gutchess, A., and Hadjikhani, N. (2018). Older adults’ neural activation in the reward circuit is sensitive to face trustworthiness. Cogn. Affect. Behav. Neurosci. 18, 21–34. doi: 10.3758/s13415-017-0549-1
Ziaei, M., Ebner, N. C., and Burianová, H. (2017). Functional brain networks involved in gaze and emotional processing. Eur. J. Neurosci. 45, 312–320. doi: 10.1111/ejn.13464
Ziaei, M., Oestreich, L., Reutens, D. C., and Ebner, N. C. (2021). Age-related differences in negative cognitive empathy but similarities in positive affective empathy. Brain Struct. Funct. 226, 1823–1840. doi: 10.1007/s00429-021-02291-y
Keywords: face trustworthiness, aging, amygdala, fMRI, oxytocin
Citation: Lin T, Pehlivanoglu D, Ziaei M, Liu P, Woods AJ, Feifel D, Fischer H and Ebner NC (2022) Age-Related Differences in Amygdala Activation Associated With Face Trustworthiness but No Evidence of Oxytocin Modulation. Front. Psychol. 13:838642. doi: 10.3389/fpsyg.2022.838642
Received: 18 December 2021; Accepted: 31 May 2022;
Published: 23 June 2022.
Edited by:
Lixia Yang, Ryerson University, CanadaReviewed by:
Kaat Alaerts, KU Leuven, BelgiumSalomon Israel, Hebrew University of Jerusalem, Israel
Copyright © 2022 Lin, Pehlivanoglu, Ziaei, Liu, Woods, Feifel, Fischer and Ebner. This is an open-access article distributed under the terms of the Creative Commons Attribution License (CC BY). The use, distribution or reproduction in other forums is permitted, provided the original author(s) and the copyright owner(s) are credited and that the original publication in this journal is cited, in accordance with accepted academic practice. No use, distribution or reproduction is permitted which does not comply with these terms.
*Correspondence: Tian Lin, bGludGlhbjA1MjdAdWZsLmVkdQ==