- 1Escola Superior Desporto e Lazer, Instituto Politécnico de Viana do Castelo, Viana do Castelo, Portugal
- 2The Research Centre in Sports Sciences, Health Sciences and Human Development (CIDESD), Vila Real, Portugal
- 3Sport Performance, Recreation, Innovation and Technology (SPRINT), Melgaço, Portugal
- 4Faculty of Sport, Centre of Research, Education, Innovation, and Intervention in Sport (CIFI2D), University of Porto, Porto, Portugal
- 5N2i, Polytechnic Institute of Maia, Maia, Portugal
- 6Physical Education Department, Universidade Federal de Mato Grosso, Cuiabá, MT, Brazil
- 7Department of Physical Activity Sciences, Universidad de Los Lagos, Santiago, Chile
- 8Exercise and Rehabilitation Sciences Institute, School of Physical Therapy, Faculty of Rehabilitation Sciences, Universidad Andres Bello, Santiago, Chile
- 9Centre of Research and Studies in Soccer (NUPEF), Universidade Federal de Viçosa, Viçosa, Brazil
- 10University of Coimbra, Research Unit for Sport and Physical Activity (CIDAF), Faculty of Sport Sciences and Physical Education, Coimbra, Portugal
- 11Department of Physical Education and Sport Sciences, University of Granada, Melilla, Spain
- 12Department of Physiology and Biochemistry, University School of Physical Education, Wrocław, Poland
- 13Wrocław University of Health and Sport Sciences, Wrocław, Poland
Background: For a long time, in sports, researchers have tried to understand an expert by comparing them with novices, raising the doubts if the visual search characteristics distinguish experts from novices. Therefore, the aim of the present study was to review and conduct a meta-analysis to evaluate the differences in visual search behavior between experts and novices in team sports athletes.
Methods: This systematic review with meta-analysis followed the PRISMA 2020 and Cochrane's guidelines. Healthy team athletes were included, which engaged in regular practice, from any sex or competitive level, specifically classified a priori as expert or novice in the original research (i.e., if they were classified after the experiment, based on one of the tests, the study would be excluded). We considered only research published in peer-reviewed journals, with no limitations regarding date or language. It was considered healthy team sport athletes engaged in regular practice. The scenarios could be in situ or film-based. The databases of EBSCO (Academic Search Complete, Academic Search Ultimate, APA PsycArticles, and APA PsycINFO), PubMed, Scopus, SPORTDiscus, and Web of Science were used to perform the searches. The risk of bias was calculated through the RoBANS tool.
Results: From a total of 6,257 records, of which 985 were duplicates, titles and abstracts of 5,272 were screened, and 45 required full-text analysis. Of those, 23 were excluded due to not fulfilling the eligibility criteria regarding participants. In the end, 22 studies were selected, however, as two studies were part of the same trial and were analyzed conjointly.
Discussion: Experts showed to be older and with more years of practice. The ability to distinguish experts from novices was not so clear regarding the variables analyzed. This could be due to the strategies chosen in each study, which were specific to each scenario, and when grouping all together, it was lost information within non-representative averages. The distinction between experts and novices was not clear, showing a lot of heterogeneity in the included studies. The expert classification itself may have been the conditioning aspect for these results, retaining the doubt and the need for more studies in the field.
Systematic review registration: The protocol was pre-registered in OSF (project https://osf.io/3j4qv/, register https://osf.io/dvk2n).
Introduction
Decision-making, which is characterized as the players' ability to choose the most appropriate action from a vast number of possibilities to achieve a specific goal (Hastie, 2001), has been shown to play an important role in athletes to enable them to attain the highest performance in sports (Gréhaigne et al., 2001; Forsman et al., 2016). During a game, the athlete has to continuously analyze the environmental information and adjust their behavior by considering his/her teammate's and opponent's actions and contextual factors (Araujo and Davids, 2016). In fact, Newell (1986) noticed that the three interacting categories of constraints, organismic (i.e., performer), environmental, and task constraints, determine the optimal coordination and control of any activity.
The continuous perceptual-cognitive skill required for better decision-making refers to the ability to identify and attain environmental information, integrate with the existing knowledge, and select and execute an appropriate response (Marteniuk, 1976). That capacity relies strongly on visual search strategies, which differentiate experts from non-experts across a wide range of sports (Le Runigo et al., 2005). Indeed, knowing where and when to look is crucial for successful sports performance, since the visual display is immense and often saturated with information both relevant and irrelevant to the task (Mann et al., 2007). In fact, studies have shown that expert players (e.g., Vaeyens et al., 2007a; Roca et al., 2011; Ericsson et al., 2018), with higher skills in decision-making (Vickers, 1996b; Roca et al., 2012) and higher tactical behaviors (Williams and Davids, 1995; Cardoso et al., 2019), present superior ability to use perceptual-cognitive processes. Notably, more skilled players are able to adapt their visual search behaviors, according to the specificity of the situation (e.g., 1 vs. 1, 2 vs. 2, or 11 vs. 11), by utilizing more effective and assertive information search strategies (Vaeyens et al., 2007a). Besides, these players are able to better manage their cognitive effort when making decisions (Cardoso et al., 2019).
Studies showed that under certain conditions, a shift in the gaze is invariably preceded by a shift in attention (Shepherd et al., 1986; Kowler et al., 1995; Henderson, 2003). However, for many years, it was difficult to link attention with shifts in gaze (e.g., Posner, 1980). The literature now has strong evidence to confirm that when a saccade is made to a new location, there is a corresponding shift in attention in the direction of the saccade, meaning that when athletes shift their gaze to a new location, they also shift their attention to that location at least for a brief period (Vickers, 2009). This could be influenced by the visual field, i.e., normally what is measured is the central, or foveal vision, neglecting the peripheral vision. However, the central vision only represents 5 degrees of the visual field, but has the highest visual acuity (Millodot, 2017), allowing one to clearly see the visual stimuli. On the other hand, peripheral vision supports visual processing (Rosenholtz, 2016).
To analyze eye movement, the main variables analyzed are as follows: (i) fixations, (ii) saccades, and (iii) smooth pursuits (Bojko, 2013). When exploring fixations, studies have calculated their location (the relevant cues to which the subject is directing his/her gaze), their duration (the time spent on those cues), and the timing of fixations (the moment when the subject looks at those cues). There is a general acceptance that a fixation measures attention (Discombe and Cotterill, 2015). Saccades are characterized as a rapid movement from one fixation to another (Kowler, 2011). Those movements, which usually only take 30–80 ms, are useful for the brain to sample the visual environment (Discombe and Cotterill, 2015). Finally, smooth pursuits occur when we slowly track an object, but they are not voluntary movements (Kowler, 2011). Other less commonly recorded eye movements, which can only be detected by some high-end eye trackers, include microsaccades (actions to bring the drift back to the center of the fixation), tremors (very small eye movements during the fixation), drifts (automatically slow movements away from the center of the fixation), and glissades (a movement that the eye produces to correct for an overshot saccade) (Discombe and Cotterill, 2015). Another well-studied vision strategy variable in sport is the quiet eye, which represents the final fixation or tracking gaze made before the initiation of the action of importance in a motor coordination task (Vickers, 2007, 2009; Vickers et al., 2019). This occurs within 1° to 3° of visual angle (or less) for a minimum of 100 ms (Vickers, 2009; Dalton, 2021), allowing to perceive the task-relevant environmental cues and to master the motor plan for a successful upcoming task (Dalton, 2021).
The curiosity about what makes an expert athlete occupy a higher level when compared to other subjects started in the early 21st century (Dalton, 2021). In fact, the question begins by defining what really an expert athlete is, since the idea of the 10,000 h of deliberate practice of Ericsson (1996) has been shown to fail, persisting a lack of a clear definition of expert. In addition, a superior athletic performance could be readily apparent observed; however, perceptual-cognitive mechanisms that contribute to the experts' advantage are much less evident (Mann et al., 2007). Nevertheless, studies have been conducted comparing experts with non-expert athletes, mainly based on their performance level. In general, studies have shown that experts are better at detecting perceptual cues, make more efficient eye movements, and have better attentional processing compared with less accomplished athletes or nonathletes (Mann et al., 2007; Voss et al., 2010). This was reflected in a superior visual acuity (Laby et al., 1996; Uchida et al., 2013), better visual-perceptual and visual-cognitive abilities (Starkes and Ericsson, 2003; Williams et al., 2011), sensitivity (Hoffman et al., 1984), and better visual tracking abilities (Vickers and Adolphe, 1997) in expert athletes. In addition, elite athletes also exhibited fewer fixations of longer duration (Vaeyens et al., 2007b; Dalton, 2021) and spent more time fixating on key athletes and areas of space that could be exploited or exposed (Vaeyens et al., 2007b). The quiet eye has also been revealed to be longer by experts, and its onset is invariably earlier (Vickers, 2009). However, despite the fact that a considerable number of studies addressed visual assessment and training in athletes, relatively few have attempted to directly link these capabilities to on-field production statistics from competitive matches (Laby and Appelbaum, 2021). Moreover, it is hypothesized that the quality and accuracy of decisions can be influenced by different covariables, such as age, the relative age effect, or expertise (Sierra-Díaz et al., 2017; Araújo et al., 2019), as well as acute factors, such as fatigue (Russell et al., 2019).
The majority of the published work in this field has conducted laboratory-based simulations (e.g., Rivilla-García et al., 2013; Krzepota et al., 2016; Castro et al., 2017). However, this strategy could not be enough to fully understand the processes underlying decision-making (Dicks et al., 2010; Mann et al., 2010), since the perception-action couplings supporting decision-making are context-specific (Passos et al., 2008). Moreover, the small size of the scenario visualized (even large-screen projections are no match for the size of real-life events) and the analysis in 2D instead of 3D as in real life also limit the perception-action couplings. Therefore, evaluation in ecological contexts seems to play an important role to reveal the full nature of the expert advantage (Mann et al., 2010). Indeed, although greater performances are typically reported in the film-based simulations conducted, it could be possible that different processes may be used when viewing film simulations compared to those employed during the actual performance (Afonso et al., 2012).
To perform a controlled and precise movement, an accurate vision is essential (Roca et al., 2011; Williams et al., 2011; Laby and Appelbaum, 2021). In fact, these were the results of the meta-analysis of Mann et al. (2007); however, 15 more years passed, and more studies emerged. Hence, in a recent review (Laby and Appelbaum, 2021), it was highlighted that studies in this field offer promising but incomplete evidence that performance on visual assessments may correlate with game performance in competitive situations, not allowing to make strong conclusions. Therefore, the aim of the present study was to conduct a review with meta-analysis to reduce the doubts around this topic to seek a clear and stronger conclusion about the differences between experts and non-experts on visual search strategies in sports. Differences are expected to be found between experts and novices, with experts showing fewer fixations, but longer gaze durations and quiet eyes. This could also help to further understand the importance and how to implement new interventions to develop visual search in sports training.
Methods
This systematic review with meta-analysis followed the PRISMA 2020 (Page et al., 2021) and Cochrane's guidelines (Higgins et al., 2019).
Eligibility criteria
We considered only studies published in peer-reviewed journals, with no limitations regarding date or language. The eligibility criteria were set according to the PECOS (Participants, Exposure, Comparator, Outcome, Study design) framework (Morgan et al., 2018):
Participants
Healthy team sport athletes engaged in regular practice, from any sex, age, or competitive level, specifically classified a priori as expert or novice in the original research (i.e., if they were classified after the experiment, based on one of the tests, the study would be excluded). Alternative classifications were acceptable (e.g., more vs. less skilled, starters vs. non-starters, and more vs. less experienced), as long as the spirit of the comparison is preserved.
Exposure
Exposure to in situ or film-based match/game scenarios in which visual search behavior is assessed through eye-tracking technology (e.g., mobile, head-mounted eye trackers typically used in dynamic settings). Visual occlusion paradigms (i.e., where the timing of the occlusion is purposefully manipulated, instead of just having a final occlusion) and effects generated by implementing training programs and/or manipulating task constraints were not considered. In the same vein, studies where the participants had to engage in non-naturalistic actions (e.g., moving a joystick or pressing a button) were excluded.
Comparator
At least one group with an expert level was different from the main group (e.g., if one group is composed of experts, the other should be composed of novice athletes, and vice-versa).
Outcomes
The studies had to include at least one of the following outcomes: number of fixations, number of fixations per second, fixation location (i.e., areas of interest or interest areas), fixation duration, fixation duration per location, fixation order/sequence, visual field (i.e., area covered by the sum of central vision and peripheral vision), quiet eye duration, microsaccade and/or saccade amplitudes, durations, peak velocities, and accelerations.
Study design
Any study design, if at least one group of experts and one group of novices are included.
Information sources
The databases of EBSCO (Academic Search Complete, Academic Search Ultimate, APA PsycArticles, and APA PsycINFO), PubMed, Scopus, SPORTDiscus, and Web of Science were used to perform the searches. On 1 October 2021, a data search was performed. No filters were used during the searches. After the automatic search, a manual search was conducted using the reference lists of the included articles. The final list was sent to two external experts (both with Ph.D. and publications related to the topic) for checking and identification of possible missing relevant articles. The experts suggested including three different studies; however, those papers were already been screened by authors and excluded as they did not fit the search requirements. Reviews about the topic were also consulted aiming to identify potential original articles that fit the scope; the general search strategy was complemented with “review” in the title, and searches were performed on PubMed and Google Scholar on 18 October 2021. Parallel to the list of included articles, an additional search for errata/corrections/corrigenda and retractions of the included studies was conducted (Higgins et al., 2019).
Search strategy
Free text terms and Boolean operators (AND/OR) were applied to the title or abstract. No filters or limits were used. Some databases only perform wildcard searches (i.e., using the symbol *) if words have a minimum of four letters, which was considered in our general search strategy:
Vision OR visual* OR eye OR eyes OR gaze OR gazing OR ocular OR oculomotor OR decision* OR anticipa* OR “quiet eye” OR saccad* OR “eye task” AND Sport* OR athlet* AND expert* OR novice OR skill* OR experience*
The fourth line of code was applied to full text/all text/any field (depending on the database):
“eye-track*” OR “eye track*” OR “fixation track*” OR “fixation-track*” OR “gaze-track*” OR “gaze track*” OR “eye movement”
Full search strategies and details for each database are presented in Table 1.
Selection process
JA and HS independently screened each record. Disagreements were decided by IT. Automated removal of duplicates was performed using EndNoteTM 20.2 for Mac (ClarivateTM), but further manual removal of duplicates was required.
Data collection process
RL and HC independently collected data. In case of disagreements, IT provided arbitrage. No automation tools were used.
Data management
Data items
Primary outcomes
The primary outcomes were number of fixations, number of fixations per second, fixation location, fixation duration, fixation duration per location, visual field, quiet eye duration, microsaccade and/or saccade amplitudes, durations, peak velocities, and accelerations.
Secondary outcomes (when assessed)
The secondary outcomes were task reaction time, the efficacy of decision-making, and accuracy of motor responses.
Additional variables:
(i) Experiment-related variables: experimental setting (i.e., film-based, in situ, or both; in the case of film-based studies, report the area of the projection), description of exposure, eye-tracking specifications (model, sampling rate, gaze resolution, and noise), study's definition of fixation and/or saccades and microsaccades, and frequency of calibration (i.e., how many trials before re-calibration).
(ii) Sample-related variables: specific sport, a competitive level (or equivalent concepts, such as training, experience, and/or skill level), age, sex, and years of practice.
(iii) Other variables: study location (i.e., country), competing interests, and funding.
Risk of bias assessment of studies
Since the studies were non-randomized by nature (i.e., experts were compared with novices) and the term exposure was more appropriate than intervention, Cochrane's RoBANS tool (Park et al., 2011) was used to assess the risk of bias arising from (i) selection of participants; (ii) confounding variables; (iii) measurement of exposure; (iv) blinding of outcome assessment; (v) incomplete outcome data; and (vi) selective outcome reporting. The risk of bias was considered similar for all primary outcomes, since data emerge from the same eye tracker device in each study. Therefore, only one risk of bias assessment was performed per study.
Data synthesis
For continuous variables (e.g., fixation duration, fixation duration per location, visual field, quiet eye duration, microsaccade and saccade amplitudes, durations, peak velocities, and accelerations), studies were meta-analytically aggregated if three or more (Claudino et al., 2021) relatively homogeneous studies were available for the same outcome measure, with the main comparison being between experts and novices. A similar approach was planned for counts-related variables (e.g., number of fixations and number of fixations per second) by using statistical approaches previously proposed to combine dichotomous and continuous data (Higgins and Thomas, 2021). Effect sizes (ES; Hedge's g) were calculated using means and SDs from each dependent variable. For studies that reported standard errors, SDs were calculated by multiplying the standard error with the square root of the sample size (Higgins and Green, 2011; Lee et al., 2015). Depending on the outcome unit of measurement (e.g., degrees vs. radians) reported among included studies for meta-analysis, standard mean differences (SMDs) were also planned to be used.
The weight of trials was proportional to their individual standard errors through the application of an inverse variance random-effects model, as heterogeneity was expected (Deeks et al., 2008; Kontopantelis et al., 2013). The ES values were presented with 95% confidence intervals (95% CIs). The ES magnitudes were interpreted using the following scale: <0.2, trivial; 0.2–0.6, small; >0.6–1.2, moderate; >1.2–2.0, large; >2.0–4.0, very large; and >4.0, extremely large (Hopkins et al., 2009). The impact of study heterogeneity was assessed using the I2 statistic, with values of <25, 25–75%, and >75% representing low, moderate, and high levels, respectively (Higgins and Thompson, 2002).
Nominal variables (i.e., fixation location) were presented as original frequencies and percentages of the total. Locations were classified into seven categories, based on (Afonso et al., 2014; Roca et al., 2018) ball, opponent with the ball, opponent without the ball, teammate with the ball, teammate without the ball, functional space (i.e., visual pivots), and unclassified. Comparisons between experts and novices were performed using chi-square tests using the original frequencies (Schober and Vetter, 2019), with the effect size being calculated through Cramér (1946). Cramer's V is interpreted as a correlation: (McHugh, 2013), using arbitrary thresholds: very weak (0–0.19), weak (0.2–0.39), moderate (0.40–0.59), strong (0.6–0.79), and very strong (0.8–1) (The BMJ, 2021). For assessing the specific cells where differences emerged, adjusted standardized residuals were calculated, with |1.96| implying the cell had a number of cases significantly larger (or smaller, if negative) than expected (Agresti, 2002). Monte Carlo correction was used in cases where >20% of the cells had expected counts <5 (Irene et al., 2021).
Subgroup and/or sensitivity analyses were performed depending on the number of studies available in each comparison: (i) sex, (ii) sport, (iii) age groups, (iv) risk of bias, and (v) experimental setting (i.e., in situ vs. film-based). All analyses were carried out using the Comprehensive Meta-Analysis software (Version 2.0; Biostat, Englewood, NJ, USA) and IBM SPSS for Mac (Version 27; IBM, Armonk, NY, USA). The level of statistical significance was set at p < 0.05.
Risk of reporting bias
The risk of reporting bias was explored for continuous variables (≥10 studies per outcome) (Sterne et al., 2011) using the Egger's test (Egger et al., 1997), with p < 0.05 implying the risk of bias. To adjust for the risk of reporting bias, a sensitivity analysis was conducted using the trim and fill method (Duval and Tweedie, 2000), with L0 as the default estimator for the number of missing studies (Shi and Lin, 2019). Computation of meta-regression was planned with at least 10 studies per covariate (Higgins et al., 2019).
Results
Study selection
Automated searches retrieved 6,257 records, of which 985 were duplicates. Titles and abstracts of 5,272 were screened, and 45 required full-text analysis. Of these, 23 were excluded due to not fulfilling eligibility criteria regarding participants (n = 6; Vaeyens et al., 2007a; Sáez-Gallego et al., 2013; Laffer et al., 2019; Cardoso et al., 2021a,b; Vítor de Assis et al., 2021), exposure (n = 6; Park, 2003; Jafarzadehpur et al., 2007; Núñez et al., 2009; Cañal-Bruland et al., 2011; Lex et al., 2015; Millard et al., 2020), comparators (n = 11; Shank and Haywood, 1987; Helsen and Starkes, 1999; Nagano et al., 2004; Zhang and Watanabe, 2005; North et al., 2009; Lee, 2010; van der Kamp, 2011; Roca et al., 2013; Wu et al., 2013; Uchida et al., 2014; Gorman et al., 2015), or outcomes (n = 1; Vater et al., 2019). Table 2 provides details about the 22 studies included in our review (Williams et al., 1994; Vickers, 1996b; Williams and Davids, 1997, 1998; Martell and Vickers, 2004; Vaeyens et al., 2007a; McRobert et al., 2009, 2011; Schorer and Baker, 2009; Roca et al., 2011; Afonso et al., 2012; Afonso and Mesquita, 2013; Rivilla-García et al., 2013; Vansteenkiste et al., 2014; Krzepota et al., 2016; Castro et al., 2017; Sarpeshkar et al., 2017; Klostermann et al., 2018; Abellán et al., 2019; Moeinirad et al., 2020; Natsuhara et al., 2020; Ribeiro et al., 2021). A manual search within the reference lists of these studies revealed 15 potentially relevant titles, of which 11 had appeared in our database searches. The abstracts of the remaining four studies were screened, with one study requiring full-text analysis and ending up fulfilling all eligibility criteria (Afonso and Mesquita, 2013). The search for reviews retrieved 10 relevant reviews on the topic, where 14 potentially relevant titles were identified. Of these, 10 titles had appeared in our initial searches, but four did not. Screening of their abstracts showed they did not fulfill all eligibility criteria. Two experts on the topic were consulted to ensure that all studies on the topic would be included. The suggestions made by experts were studies that had already passed through our analysis and had been excluded. No errata or retractions were found for any of the included studies.
Importantly, the studies of McRobert et al. (2009, 2011) were part of the same trial, and so were analyzed conjointly. Likewise, the studies of Williams et al. (1994) and Williams and Davids (1997) were also part of the same trial, and were analyzed conjointly.
Study characteristics
Table 2 summarizes all the characteristics of the studies included in this review. Most of the studies (~73%, corresponding to 16 studies) included only men (Williams et al., 1994; Williams and Davids, 1997, 1998; Vaeyens et al., 2007a; McRobert et al., 2009, 2011; Schorer and Baker, 2009; Roca et al., 2011; Rivilla-García et al., 2013; Krzepota et al., 2016; Castro et al., 2017; Sarpeshkar et al., 2017; Klostermann et al., 2018; Abellán et al., 2019; Moeinirad et al., 2020; Natsuhara et al., 2020). Conversely, the remaining studies only included women, as detailed in Table 2. Although the majority of the studies included experts or considered highly skilled athletes, six of them also analyzed youth athletes (Vaeyens et al., 2007a; Schorer and Baker, 2009; Castro et al., 2017; Sarpeshkar et al., 2017; Abellán et al., 2019; Ribeiro et al., 2021). The sports studies varied among soccer (n = 8), volleyball (n = 4), basketball (n = 3), cricket (n = 3), handball (n = 3), and ice hockey (n = 1); nevertheless, 13 studies conducted laboratory procedures. Considering the primary outcome, two main topics were analyzed: visual behavior (Williams et al., 1994; Vickers, 1996b; Williams and Davids, 1997, 1998; Martell and Vickers, 2004; McRobert et al., 2009, 2011; Afonso et al., 2012; Rivilla-García et al., 2013; Vansteenkiste et al., 2014; Krzepota et al., 2016; Sarpeshkar et al., 2017; Klostermann et al., 2018; Abellán et al., 2019; Moeinirad et al., 2020; Ribeiro et al., 2021) and the decision-making (Vaeyens et al., 2007a; Schorer and Baker, 2009; Roca et al., 2011; Afonso and Mesquita, 2013; Castro et al., 2017; Natsuhara et al., 2020). Considering the second outcome, the focus was mainly to characterize and compare between different levels of expertise, the number of fixations, and the number of locations, with some studies including verbal reports and the quiet eye analysis.
Risk of bias in individual studies
The Risk of Bias Assessment Tool for Non-Randomized Studies (RoBANS) was used to assess the risk of bias in included studies. This tool contains guidelines for evaluation based on six categories, each to be assessed as “high risk”, unclear risk, or “low risk” (Kim et al., 2013). The RoBANS assessment was conducted by two authors independently (JA and AFS). Disagreements were resolved by consensus or consultation with a third assessor (IT) when required.
Considering the participants' selection, a low risk of bias was identified in 70% of the studies. The remaining 30% presented high risk because the expertise or skill level was pre-stipulated by experts, without an objective referral to the playing level. Likewise, a low risk of bias was reported in 70% of the studies when analyzing the issue of confounding variables, since most trials provided familiarization with the testing procedures. However, in some studies, there was no familiarization with testing procedures, which may have influenced the results, due to a learning effect. Although most studies had a low risk of bias for incomplete outcome data (75%), studies often only reported the final sample, and there was no clear indication of whether the included participants were part of a larger sample of the initially recruited group. Occasionally, only a small subset of visual search data would be selected for analysis. The major concerns with the risk of bias were related to the measurement (eye-tracking data) and the building outcome assessment, both presenting a high risk in 55% of the studies. In the first issue, it was observed that the digitalization of images was often performed by a single tester, unblinded to the skill level. However, often this was complemented by a second assessment, by independent raters. When this was not the case, the risk of bias in measurement became high. Regarding the blinding outcome assessment, it was registered that, usually, testers were not blinded, but in some studies, a second, independent tester provided inter-rater reliability calculations. In cases where this did not happen, we judged the study to be at high risk for blinding outcome assessment. Finally, considering the selective outcome reporting, no study had a pre-registered or pre-published protocol against which to compare the manuscript. Therefore, 90% of the studies were found to be unclear regarding whether the reporting outcome was complete or selective.
Chronological age
Twenty studies provided data for chronological age, involving 20 expert and 20 novice groups (pooled n = 474). Results showed a moderate effect, with greater chronological age for expert athletes when compared to novice athletes (ES = 0.66; 95% CI = 0.12–1.20; p = 0.017; Figure 1; I2 = 86.9%; Egger test = 0.0139, with adjusted value at ES = 1.03; 95% CI = 0.43–1.64). When results were analyzed as per athletes' involvement in their respective sports (Figure 2), no significant moderator effect was noted for the type of sport (p = 0.290 between groups), involving basketball (three studies; I2 = 88.0%), soccer (nine studies; I2 = 89.3%), and volleyball (four studies; I2 = 90.1%) athletes. Other sports were not included in the moderator analysis, as less than three studies were available.
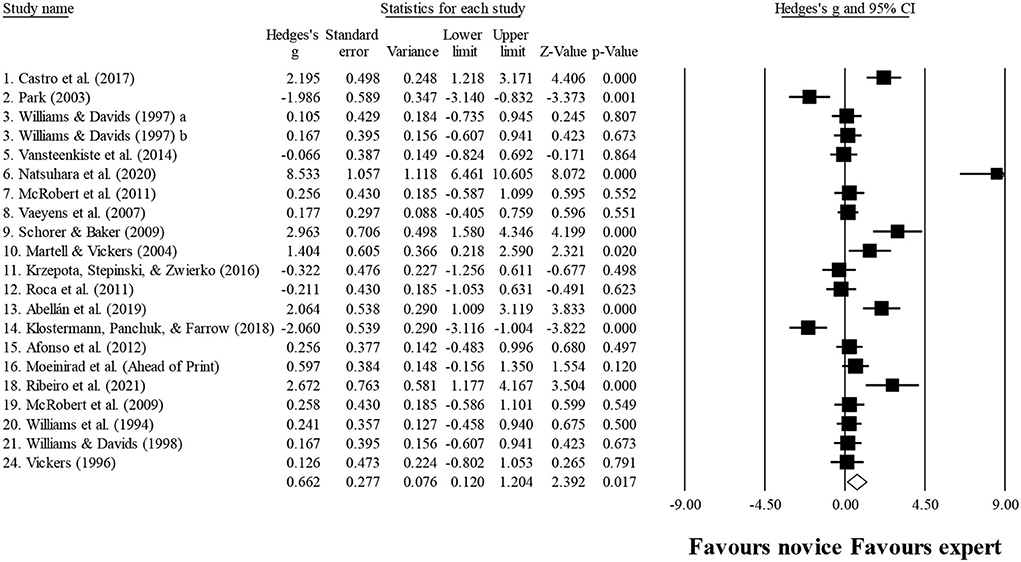
Figure 2. Chronological age: greater chronological age was noted for expert athletes compared to novice athletes. Black squares: individual studies. Its size represents their relative weights. White rhomboid: summary value.
Years of experience
Sixteen studies provided data for years of experience, involving 16 expert and 16 novice groups (pooled n = 363). Results showed a moderate effect, with greater years of experience for expert athletes when compared to novice athletes (ES = 1.13; 95% CI = 0.69–1.57; p < 0.001; Figure 3; I2 = 73.9%; Egger test = 0.007, with adjusted value at ES = 1.21, 95% CI = 0.76–1.65). When results were analyzed as per athletes' involvement in their respective sports (Figure 4), no significant moderator effect was noted for the type of sport (p = 0.835 between groups), involving basketball (three studies; I2 = 89.7%) and soccer (six studies; I2 = 81.0%) athletes. Other sports were not included in the moderator analysis, as less than three studies were available.
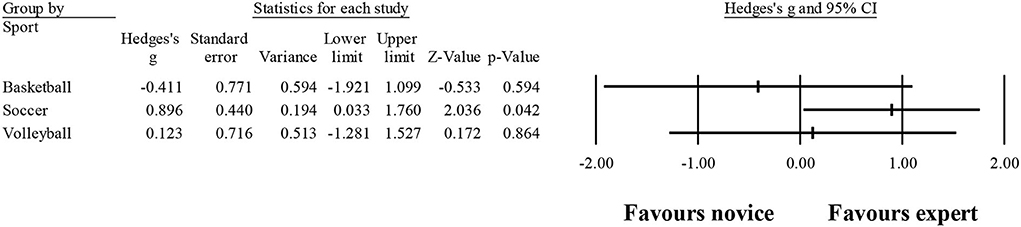
Figure 3. Chronological age moderated by type of sport: no significant moderator effect was noted for the type of sport (p = 0.290 between groups).
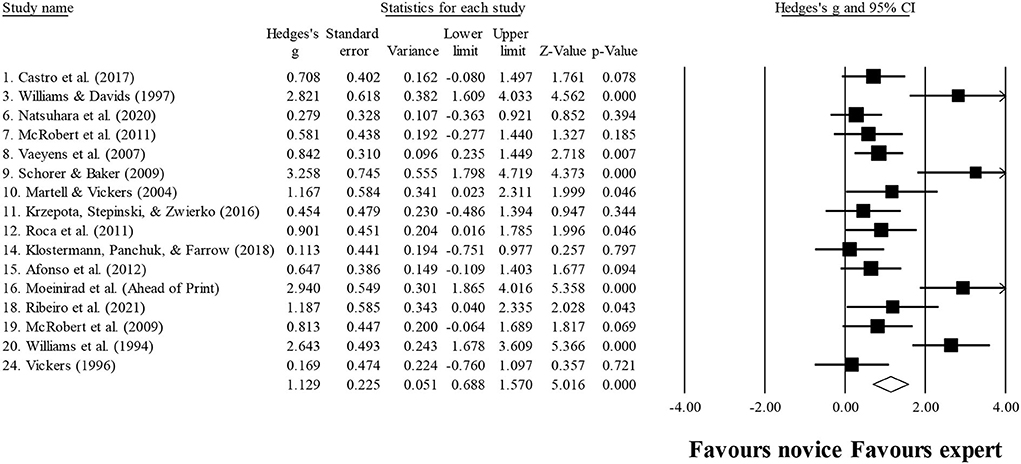
Figure 4. Years of experience: greater years of experience were noted for expert athletes compared to novice athletes. Black squares: individual studies. Its size represents their relative weights. White rhomboid: summary value.
Number of fixations
Ten studies provided data for the number of fixations, involving 10 expert and 10 novice groups (pooled n = 218). Results showed a small effect, with greater (although not significant) number of fixations for expert athletes when compared to novice athletes (ES = 0.39; 95% CI = −0.32 to 1.11; p = 0.280; Figure 5; I2 = 84.4%; Egger test = 0.373). When results were analyzed as per athletes' involvement in their respective sports (Figure 6), a moderator effect (although not significant; p = 0.078 between groups) was noted for the type of sport, involving soccer (four studies; I2 = 92.1%) and volleyball (three studies; I2 = 39.4%) athletes. Other sports were not included in the moderator analysis, as less than three studies were available.
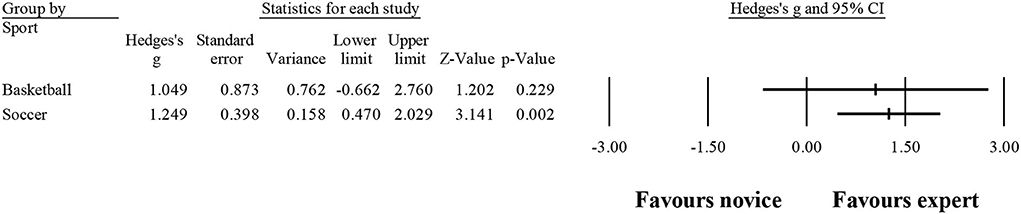
Figure 5. Years of experience moderated by type of sport: no significant moderator effect was noted for the type of sport (p = 0.835 between groups).
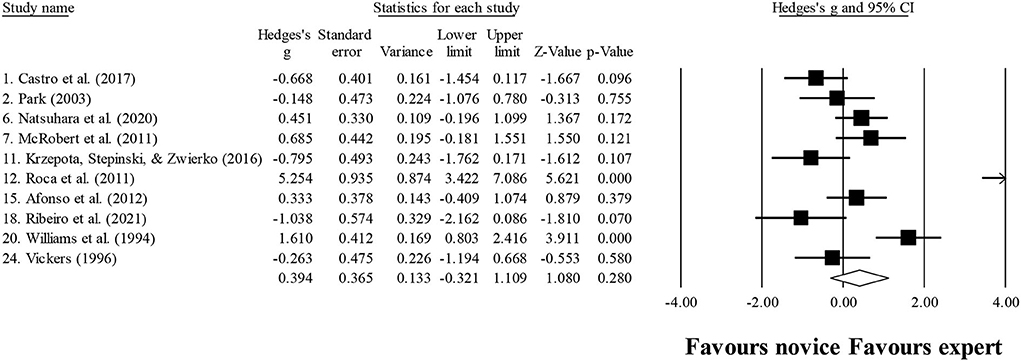
Figure 6. Number of fixations: greater (although not significant) number of fixations was noted for expert athletes compared to novice athletes. Black squares: individual studies. Its size represents their relative weights. White rhomboid: summary value.
Fixation duration
Ten studies provided data for fixation duration, involving 11 expert and 11 novice groups (pooled n = 246). Results showed a small effect, with lower (although no significant) fixation duration for expert athletes when compared to novice athletes (ES = −0.21; 95% CI = −0.72 to 0.31; p = 0.435; Figure 7; I2 = 75.1%; Egger test = 0.509). When results were analyzed as per athletes' involvement in their respective sports (Figure 8), no moderator effect (p = 0.138 between groups) was noted for the type of sport, involving soccer (six studies; I2 = 82.7%) and volleyball (three studies; I2 = 0.0%) athletes. Other sports were not included in the moderator analysis, as less than three studies were available.
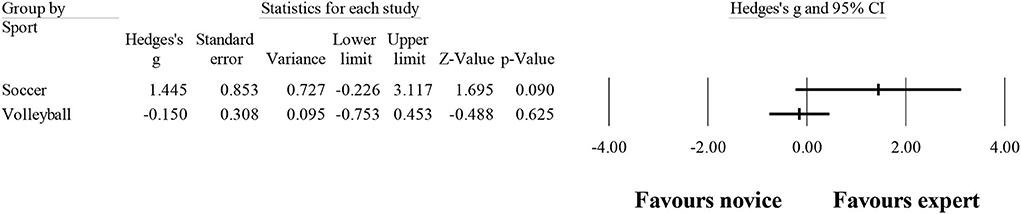
Figure 7. Number of fixations moderated by type of sport: no significant moderator effect was noted for the type of sport (p = 0.078 between groups).
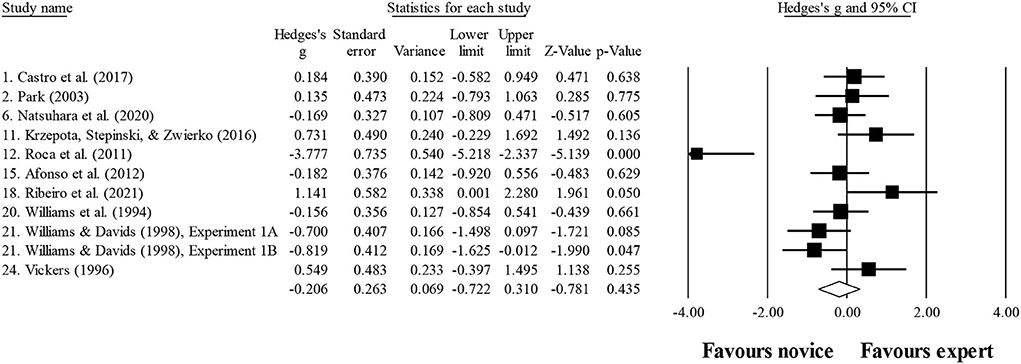
Figure 8. Fixation duration: similar fixation duration was noted for expert athletes compared to novice athletes. Black squares: individual studies. Its size represents their relative weights. White rhomboid: summary value.
Quiet eye duration
Three studies provided data for quiet eye duration, involving eight expert and eight novice groups (pooled n = 174). Results showed a small effect, with greater (although no significant) quiet eye duration for expert athletes when compared to novice athletes (ES = 0.34; 95% CI = −0.44 to 1.11; p = 0.396; Figure 9; I2 = 82.9%). Moderator analyses as per athletes' type of sport were precluded, as less than three studies were available.
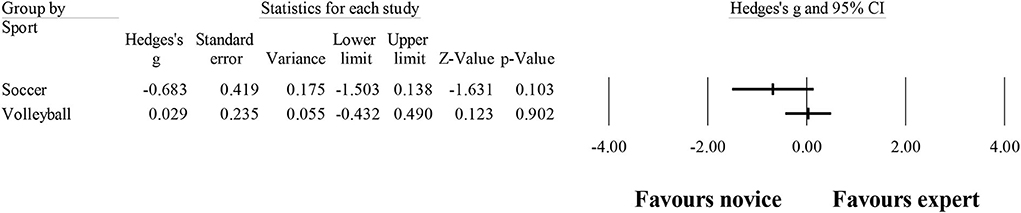
Figure 9. Fixation duration moderated by type of sport: no significant moderator effect was noted for type of sport (p = 0.138 between groups).
Other outcomes
The fixation duration per location, fixation order/sequence, visual field (i.e., area covered by the sum of central vision and peripheral vision), and microsaccade and/or saccade amplitudes, durations, peak velocities, and accelerations were not included in the present meta-analysis as initially planned, since not enough or no information was given in the included studies that would allow analysis and subsequent discussion and conclusion.
Discussion
The present study aimed to review and conduct a meta-analysis to examine the differences between experts and non-experts in visual search. In contrast to what was hypothesized, results showed that the ability to distinguish experts from novices was not so clear regarding the variables analyzed. This could be due to the strategies chosen in each study, which were specific to each scenario, and when grouping all together, it was lost information within non-representative averages. Considering the participants included, not surprisingly, it was shown that experts were older and accumulated more years of practice. The analysis, by sport, revealed a moderate effect only on the number of fixations. Altogether, these results seem to highlight that chronological age and years of practice could really improve the athletes' level, and a visual search analysis should be conducted regarding the sport. Nevertheless, more studies need to be conducted in different sports to strengthen further conclusions.
A possible variable that could affect our results was the ambiguous definition of an expert athlete. In fact, in a review by Swann et al. (2015), at least eight different criteria were used to define an expert athlete. The most frequent criteria to distinguish an elite from a non-elite was if the athlete had an international and/or national competitive level. The athletes' experience occupied the second place (49% of the sample), and the professionalism characteristic took the third place, with almost 30% of the studies included. Clearly, such imprecision in the criteria used to define athletes as expert threatens the validity of research on expertise in sport. More recently, McKay et al. (2022) reinforce the importance of a clear definition of athlete's level, highlighting that athletic success might be explained by different attributes, with the athletic caliber influencing intervention results. Therefore, the authors presented a framework of five levels, with clear items to classify participants, which could help in future studies if we are really getting information from expert athletes. In fact, this could be the reason why, in the present study, the experts were shown to be older and with more years of practice than novices.
The number of fixations, which is characterized as the time spent looking at a particular location, provides information about the attention span and the time needed to process the stimulus/object (Hüttermann et al., 2018). It has been suggested that expert athletes exhibit a distinct gaze behavior, leading to an optimization in the visual information collected and maximizing the coupling between perception and action (Klostermann and Moeinirad, 2020). Indeed, in the reviews of Mann et al. (2007) and Gegenfurtner et al. (2011), it was found that expert athletes presented fewer fixations with longer durations, showing that they focus on different, but more task-relevant information sources. However, in a recent review in the field (Klostermann and Moeinirad, 2020), these findings were not corroborated. A similar pattern among different skill levels was found for both the number and the duration of the fixations. In that same review, a comparison between expert and intermediate skill athletes was performed (n = 41 studies), as well as between experts and novices (n = 32 studies). In addition, it was also found that the same number of studies noticed a significant negative result in the number of fixations when comparing experts with intermediate and novice athletes (Klostermann and Moeinirad, 2020).
These confusing outcomes were also expressed in the present meta-analysis, shown in Figure 6. In fact, in the sample of 218 evaluated athletes, a small effect (non-significant: ES = 0.39; p = 0.280) was observed, not corroborating the older studies in the field. This could be the result of a great heterogeneity found (I2 = 84.4%) in the present analysis. It is possible that sports scenarios do not always require the same search strategies, to cover the average data. Although this finding does not inhibit experts from using the best strategy for each specific scenario, it may imply a greater number of fixations in one instead of another scenario. Nevertheless, when exploring the number of fixations in each sport, moderate effects were found (although not significant; p = 0.078 between groups). However, two main concerns should be highlighted: (i) only three studies in volleyball and four studies in soccer were included, and (ii) the results were quite different for each sport, as shown in Figure 7.
Regarding the fixation duration, in the study of Klostermann et al. (2018) and Moeinirad et al. (2020), a similar number of studies were found to have noticed significant and non-significant results. However, in the present study, more studies were registered, revealing nonsignificant (e.g., Park, 2003; McRobert et al., 2011; Castro et al., 2017; Natsuhara et al., 2020) than significant results (Williams et al., 1994; Williams and Davids, 1997, 1998; Roca et al., 2011), with a small effect observed when analyzing all studies together (Figure 8) and no effect when separated by sport (Figure 9). Once again, this could be due to the heterogeneity observed in the included study (I2 = 75.1%), showing that there is a lot of dispersion in the results.
Longer duration on quiet eye has been reported when comparing experts to intermediate and novice athletes (Mann et al., 2007; Gegenfurtner et al., 2011; Klostermann and Moeinirad, 2020). The capacity to predict performance has been associated with expertise (Lebeau et al., 2016), since it was suggested that during this period, task-relevant environmental cues are processed and motor programs are retrieved and coordinated for task success (Vickers, 1996a,b). These findings seem to be in line with those exhibited in Figure 10, although only a small effect was proven to exist (ES = 0.34; p = 0.396), instead of a moderate and large effect considered in previous studies (e.g., Lebeau et al., 2016). Nevertheless, in the present study, although experts showed a trend to spend more time in the quiet eye, that value was not significant. It should be noted that this variable also showed high heterogeneity values (I2 = 82.9%). In addition, in line with the Klostermann and Moeinirad (2020) review, in contrast with other gaze measures, no study with significant negative results was found, but only a trend for a higher quiet eye duration in an undefended basketball game situation was reported (Klostermann et al., 2018).
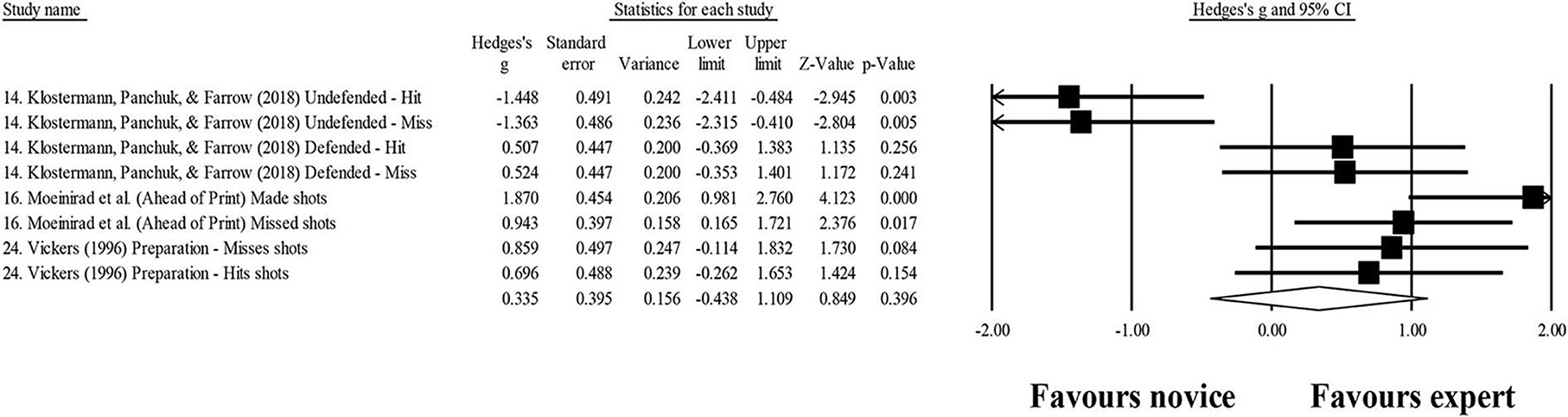
Figure 10. Quiet eye duration: similar quiet eye duration was noted for expert athletes compared to novice athletes. Black squares: individual studies. Its size represents their relative weights. White rhomboid: summary value.
It seems that over the last years, the results reported in this field have changed. In fact, the number of studies that revealed nonsignificant results has overwhelmed the number of studies with significant positive results (Klostermann and Moeinirad, 2020). To explain this finding, researchers have suggested that the main reason for these different results can be attributed to the advances in technology, specifically more accurate and reliable gaze data (Kredel et al., 2017; Orquin and Holmqvist, 2018). Moreover, recent developments in eye trackers allowed to conduct studies in a more ecological and thus more representative environment (Orquin and Holmqvist, 2018), which has been found to affect gaze behavior (e.g., Dicks et al., 2010; van Maarseveen et al., 2015). In fact, as we could observe in Table 2, different eye-tracker brands and specifications were used in different studies. Nevertheless, in future studies, more attention must be paid to the athletes' classification, to have a normalization of the data and the certainty that we are evaluating experts and novices.
It should be noted that the major limitation inherent to the present study was the method used to classify and characterize experts and novices. However, this difficulty and diversity of criteria have already been reported and discussed elsewhere (Chi, 2006; Swann et al., 2015; McKay et al., 2022), which may confuse the results and conclusions drawn.
Data availability statement
The original contributions presented in the study are included in the article/supplementary material, further inquiries can be directed to the corresponding author/s.
Author contributions
AFS, JA, and EM-C: conceptualization and supervision. AFS, JA, AS, NP, and FG: writing. RL, HC, IT, and HS: search and paper screening. RR-C: statistical analysis. AS and JA: project administration. AK and AO: writing revision. All authors have read and agreed to the published version of the manuscript.
Acknowledgments
The authors gratefully acknowledge the experts in the field, Andre Roca and McRobert Allistair, for helping in reviewing the final list of studies and their suggestions.
Conflict of interest
The authors declare that the research was conducted in the absence of any commercial or financial relationships that could be construed as a potential conflict of interest.
Publisher's note
All claims expressed in this article are solely those of the authors and do not necessarily represent those of their affiliated organizations, or those of the publisher, the editors and the reviewers. Any product that may be evaluated in this article, or claim that may be made by its manufacturer, is not guaranteed or endorsed by the publisher.
References
Abellán, J., Sáez-Gallego, N. M., Savelsbergh, G. J. P., and Contreras, O. R. (2019). Interception of a corner kick from the constraints-led aproach. Rev. Int. Med. Cienc. Act. Física El Deporte 19, 225–241. doi: 10.15366/rimcafd2019.74.004
Afonso, J., Garganta, J., Mcrobert, A., Williams, A. M., and Mesquita, I. (2012). The perceptual cognitive processes underpinning skilled performance in volleyball: evidence from eye-movements and verbal reports of thinking involving an in situ representative task. J. Sports Sci. Med. 11, 339.
Afonso, J., Garganta, J., McRobert, A., Williams, M., and Mesquita, I. (2014). Visual search behaviours and verbal reports during film-based and in situ representative tasks in volleyball. Eur. J. Sport Sci. 14, 177–184. doi: 10.1080/17461391.2012.730064
Afonso, J., and Mesquita, I. (2013). Skill-based differences in visual search behaviours and verbal reports in a representative film-based task in volleyball. Int. J. Perform. Anal. Sport 13, 669–677. doi: 10.1080/24748668.2013.11868679
Araujo, D., and Davids, K. (2016). Team synergies in sport: theory and measures. Front. Psychol. 7, 1449. doi: 10.3389/fpsyg.2016.01449
Araújo, D., Hristovski, R., Seifert, L., Carvalho, J., and Davids, K. (2019). Ecological cognition: expert decision-making behaviour in sport. Int. Rev. Sport Exerc. Psychol. 12, 1–25. doi: 10.1080/1750984X.2017.1349826
Cañal-Bruland, R., Lotz, S., Hagemann, N., Schorer, J., and Strauss, B. (2011). Visual span and change detection in soccer: An expertise study. J. Cogn. Psychol. 23, 302–310. doi: 10.1080/20445911.2011.496723
Cardoso, F. S. L., Afonso, J., Roca, A., and Teoldo, I. (2021a). The association between perceptual-cognitive processes and response time in decision making in young soccer players. J. Sports Sci. 39, 926–935. doi: 10.1080/02640414.2020.1851901
Cardoso, F. S. L., García-Calvo, T., Patrick, T., Afonso, J., and Teoldo, I. (2021b). How does cognitive effort influence the tactical behavior of soccer players? Percept. Mot. Skills 128, 851–864. doi: 10.1177/0031512521991405
Cardoso, F. S. L., -Villora, S., Guilherme, J., and Teoldo, I. (2019). Young soccer players with higher tactical knowledge display lower cognitive effort. Percept. Mot. Skills, 126, 499–514. doi: 10.1177/0031512519826437
Castro, H. D. O., Costa, G. D. C., Praça, G. M., Campbell, C. S., and Greco, P. J. (2017). Análise das fixações visuais e tomada de decisão de atletas de voleibol das categorias infanto e juvenil. Rev. Bras. Ciênc. Mov. 25, 51–59. doi: 10.31501/rbcm.v25i1.6337
Chi, M. T. H. (2006). “The Cambridge handbook of expertise and expert performance,” in Two Approaches to the Study of Experts' Characteristics, eds K. A. Ericsson, N. Charness, P. Feltovich, and R. R. Hoffman (Cambridge: Cambridge University Press), 21–38.
Claudino, J. G., Afonso, J., Sarvestan, J., Lanza, M. B., Pennone, J., Filho, C. A. C., et al. (2021). Strength training to prevent falls in older adults: a systematic review with meta-analysis of randomized controlled trials. J. Clin. Med. 10, 3184. doi: 10.3390/jcm10143184
Dalton, K. (2021). The quiet eye in sports performance—Is the quiet eye the ultimate explanation or only the beginning? Optomet. Vis. Sci. 98, 732–737. doi: 10.1097/OPX.0000000000001728
Deeks, J. J., Higgins, J. P., and Altman, D. G. (2008). “Analysing data and undertaking meta-analyses,” in Cochrane Handbook for Systematic Reviews of Interventions, eds J. P. Higgins, and S. Green (The Cochrane Collaboration, 243–296.
Dicks, M., Button, C., and Davids, K. (2010). Examination of gaze behaviors under in situ and video simulation task constraints reveals differences in information pickup for perception and action. Attent. Percept. Psychophys. 71, 706–720. doi: 10.3758/APP.72.3.706
Discombe, R. M., and Cotterill, S. T. (2015). Eye tracking in sport: a guide for new and aspiring researchers. Sport Exerc. Psychol. Rev. 11, 49–58. doi: 10.1016/j.ijpsycho.2020.05.010
Duval, S., and Tweedie, R. (2000). Trim and fill: a simple funnel-plot-based method of testing and adjusting for publication bias in meta-analysis. Biometrics 56, 455–463. doi: 10.1111/j.0006-341X.2000.00455.x
Egger, M., Smith, G. D., Schneider, M., and Minder, C. (1997). Bias in meta-analysis detected by a simple, graphical test. BMJ 315, 629–634. doi: 10.1136/bmj.315.7109.629
Ericsson, K., Anders, H. R. R., Kozbelt, A., and Williams, A. M. (2018). The Cambridge Handbook of Expertise and Expert Performance. Cambridge, UK: Cambridge University Press.
Ericsson, K. A. (1996). “The acquisition of expert performance: an introduction to some of the issues,” in The Road to Excellence: The Acquisition of Expert Performance in the Arts and Sciences, Sports, and Games, ed K. A. Ericsson (Mahwah, NJ: Erlbaum), 1–50.
Forsman, H., Blomqvist, M., Davids, K., Konttinen, N., and Liukkonen, J. (2016). The role of sport-specific play and practice during childhood in the development of adolescent Finnish team sport athletes. Int. J. Sports Sci. Coach. 11, 69–77. doi: 10.1177/1747954115624816
Gegenfurtner, A., Lehtinen, E., and Säljö, R. (2011). Expertise differences in the comprehension of visualizations: a meta-analysis of eye-tracking research in professional domains. Educ. Res. Rev. 23, 523–552. doi: 10.1007/s10648-011-9174-7
Gorman, A. D., Abernethy, B., and Farrow, D. (2015). Evidence of different underlying processes in pattern recall and decision-making. Q. J. Exp. Psychol. 68, 1813–1831. doi: 10.1080/17470218.2014.992797
Gréhaigne, J.-F., Godbout, P., and Bouthier, D. (2001). The teaching and learning of decision making in team sports. Quest 53, 59–76. doi: 10.1080/00336297.2001.10491730
Hastie, R. (2001). Problems for judgment and decision making. Annu. Rev. Psychol. 52, 653–683. doi: 10.1146/annurev.psych.52.1.653
Helsen, W. F., and Starkes, J. L. (1999). A multidimensional approach to skilled perception and performance in sport. Appl. Cogn. Psychol. 13, 1–27. doi: 10.1002/(SICI)1099-0720(199902)13:1<1::AID-ACP540>3.0.CO;2-T
Henderson, J. M. (2003). Human gaze control during real-world scene perception. Trends Cogn. Sci. 7, 498–504. doi: 10.1016/j.tics.2003.09.006
Higgins, J., and Thomas, J. (2021). Cochrane Handbook for Systematic Reviews of Interventions. Cochrane.
Higgins, J. P., Thomas, J., Chandler, J., Cumpston, M., Li, T., Page, M. J., and Welch, V. A. (2019). Cochrane Handbook for Systematic Reviews of Interventions, 2nd Edn. Chichester: John Wiley and Sons.
Higgins, J. P. T., and Green, S. (2011). Cochrane Handbook for Systematic Reviews of Interventions. Chichester, UK: Cochrane.
Higgins, J. P. T., and Thompson, S. G. (2002). Quantifying heterogeneity in a meta-analysis. Stat. Med. 21, 1539–1558. doi: 10.1002/sim.1186
Hoffman, L. G., Polan, G., and Powell, J. (1984). The relationship of contrast sensitivity functions to sports vision. J. Am. Optometr. Assoc. 55, 747–752.
Hopkins, W. G., Marshall, S. W., Batterham, A. M., and Hanin, J. (2009). Progressive statistics for studies in sports medicine and exercise science. Med. Sci. Sports Exerc. 41, 3–13. doi: 10.1249/MSS.0b013e31818cb278
Hüttermann, S., Noël, B., and Memmert, D. (2018). Eye tracking in high-performance sports: evaluation of its application in expert athletes. Int. J. Comp. Sci. Sport 17, 182–203. doi: 10.2478/ijcss-2018-0011
Irene, K., Amubuomombe, P. P., Mogeni, R., Andrew, C., Mwangi, A., and Omenge, O. E. (2021). Maternal and perinatal outcomes in women with eclampsia by mode of delivery at Riley mother baby hospital: a longitudinal case-series study. BMC Pregn. Childbirth 21, 439. doi: 10.1186/s12884-021-03875-6
Jafarzadehpur, E., Aazami, N., and Bolouri, B. (2007). Comparison of saccadic eye movements and facility of ocular accommodation in female volleyball players and non-players. Scand. J. Med. Sci. Sports 17, 186–190. doi: 10.1111/j.1600-0838.2005.00535.x
Kim, S. Y., Park, J. E., Lee, Y. J., Seo, H.-J., Sheen, S.-S., Hahn, S., et al. (2013). Testing a tool for assessing the risk of bias for nonrandomized studies showed moderate reliability and promising validity. J. Clin. Epidemiol. 66, 408–414. doi: 10.1016/j.jclinepi.2012.09.016
Klostermann, A., and Moeinirad, S. (2020). Fewer fixations of longer duration? Expert gaze behavior revisited. Ger. J. Exerc. Sport Res. 50, 146–161. doi: 10.1007/s12662-019-00616-y
Klostermann, A., Panchuk, D., and Farrow, D. (2018). Perception-action coupling in complex game play: exploring the quiet eye in contested basketball jump shots. J. Sports Sci. 36, 1054–1060. doi: 10.1080/02640414.2017.1355063
Kontopantelis, E., Springate, D. A., and Reeves, D. (2013). A re-analysis of the cochrane library data: the dangers of unobserved heterogeneity in meta-analyses. PLoS ONE 8, e69930. doi: 10.1371/journal.pone.0069930
Kowler, E. (2011). Eye movements: the past 25 years. Vision Res. 51, 1457–1483. doi: 10.1016/j.visres.2010.12.014
Kowler, E., Anderson, E., Dosher, B., and Blaser, E. (1995). The role of attention in the programming of saccades. Vision Res. 35, 1897–1916. doi: 10.1016/0042-6989(94)00279-U
Kredel, R., Vater, C., Klostermann, A., and Hossner, E. (2017). Eye-tracking technology and the dynamics of natural gaze behavior in sports: a systematic review of 40 years of research. Front. Psychol. 8, 1845. doi: 10.3389/fpsyg.2017.01845
Krzepota, J., Stepiński, M., and Zwierko, T. (2016). Gaze control in one versus one defensive situations in soccer players with various levels of expertise. Percept. Mot. Skills 123, 769–783. doi: 10.1177/0031512516664903
Laby, D. M., and Appelbaum, L. G. (2021). Vision and on-field performance: a critical review of visual assessment and training studies with athletes. Optomet. Vis. Sci. 98, 723–731. doi: 10.1097/OPX.0000000000001729
Laby, D. M., Davidson, J. L., Rosenbaum, L. J., Strasser, C., Mellman, M. F., Rosenbaum, A. L., and Kirschen, D. G. (1996). The visual function of professional baseball players. Am. J. Ophthalmol. 122, 476–485. doi: 10.1016/S0002-9394(14)72106-3
Laffer, J. C., Coutts, A. J., and Fransen, J. (2019). Effect of skill level on allocation of visual attention in volleyball blocking. J. Motor Learn. Dev. 7, 215–231. doi: 10.1123/jmld.2017-0061
Le Runigo, C., Benguigui, N., and Bardy, B. G. (2005). Perception-action coupling and expertise in interceptive actions. Hum. Mov. Sci. 24, 429–45. doi: 10.1016/j.humov.2005.06.008
Lebeau, J. C., Liu, S., Sáenz-Moncaleano, C., Sanduvete-Chaves, S., Chacón-Moscoso, S., Becker, B. J., and Tenenbaum, G. (2016). Quiet eye and performance in sport: a meta-analysis. J. Sport Exerc. Psychol. 38, 441–457. doi: 10.1123/jsep.2015-0123
Lee, D. K., In, J., and Lee, S. (2015). Standard deviation and standard error of the mean. Korean J. Anesthesiol. 68, 220. doi: 10.4097/kjae.2015.68.3.220
Lee, S. M. (2010). Does your eye keep on the ball? The strategy of eye movement for volleyball defensive players during spike serve reception. Int. J. Appl. Sports Sci. 22, 128–137. doi: 10.24985/ijass.2010.22.1.128
Lex, H., Essig, K., Knoblauch, A., and Schack, T. (2015). Cognitive representations and cognitive processing of team-specific tactics in soccer. PLoS ONE 10, e0118219. doi: 10.1371/journal.pone.0118219
Mann, D., Abernethy, B., and Farrow, D. (2010). Action specificity increases anticipatory performance and the expert advantage in natural interceptive tasks. Acta Psychol. 135, 17–23. doi: 10.1016/j.actpsy.2010.04.006
Mann, D. T., Williams, A. M., Ward, P., and Janelle, C. M. (2007). Perceptual-cognitive expertise in sport: a meta-analysis. J. Sports Exerc. Physiol. 29, 457–478. doi: 10.1123/jsep.29.4.457
Martell, S. G., and Vickers, J. N. (2004). Gaze characteristics of elite and near-elite athletes in ice hockey defensive tactics. Hum. Mov. Sci. 22, 689–712. doi: 10.1016/j.humov.2004.02.004
McHugh, M. L. (2013). The Chi-square test of independence. Biochem. Med. 25, 143–149. doi: 10.11613/BM.2013.018
McKay, A. K. A., Stellingwerff, T., Smith, E. S., Martin, D. T., Mujika, I., Goosey-Tolfrey, V. L., et al. (2022). Defining training and performance caliber: a participant classification framework. Int. J. Sports Physiol. Perform. 17, 317–331. doi: 10.1123/ijspp.2021-0451
McRobert, A. P., Ward, P., Eccles, D. W., and Williams, A. M. (2011). The effect of manipulating context-specific information on perceptual–cognitive processes during a simulated anticipation task. Br. J. Psychol. 102, 519–534. doi: 10.1111/j.2044-8295.2010.02013.x
McRobert, A. P., Williams, A. M., Ward, P., and Eccles, D. W. (2009). Tracing the process of expertise in a simulated anticipation task. Ergonomics 52, 474–483. doi: 10.1080/00140130802707824
Millard, L., Shaw, B., Breukelman, G., and Shaw, I. (2020). Visual abilities distinguish level of play in rugby. Ann. Appl. Sport Sci. 8, 1–6. doi: 10.29252/aassjournal.906
Millodot, M. (2017). Dictionary of Optometry and Vision Science. Amsterdam: Elsevier Health Sciences.
Moeinirad, S., Abdoli, B., Farsi, A., and Ahmadi, N. (2020). The role of quiet eye duration and its components in a complex far-aiming task. J. Motor Learn. Dev. 8, 516–527. doi: 10.1123/jmld.2019-0048
Morgan, R. L., Whaley, P., Thayer, K. A., and Schünemann, H. J. (2018). Identifying the PECO: a framework for formulating good questions to explore the association of environmental and other exposures with health outcomes. Environ. Int. 121 (Pt 1), 1027. doi: 10.1016/j.envint.2018.07.015
Nagano, T., Kato, T., and Fukuda, T. (2004). Visual search strategies of soccer players in one-on-one defensive situations on the field. Percept. Mot. Skills 99, 968–974. doi: 10.2466/pms.99.3.968-974
Natsuhara, T., Kato, T., Nakayama, M., Yoshida, T., Sasaki, R., Matsutake, T., and Asai, T. (2020). Decision-making while passing and visual search strategy during ball receiving in team sport play. Percept. Mot. Skills 127, 468–489. doi: 10.1177/0031512519900057
Newell, K. M. (1986). “Constraints on the development of coordination,” in Motor Development in Children: Aspects of Coordination and Control, eds M. G. Wade and H. T. A. Whiting (Dordrecht: Martinus Nijhoff). doi: 10.1007/978-94-009-4460-2_19
North, J. S., Williams, A. M., Hodges, N., Ward, P., and Ericsson, K. A. (2009). Perceiving patterns in dynamic action sequences: investigating the processes underpinning stimulus recognition and anticipation skill. Appl. Cogn. Psychol. 23, 878–894. doi: 10.1002/acp.1581
Núñez, F. J., Oña, A., Raya, A., and Bilbao, A. (2009). Differences between expert and novice soccer players when using movement precues to shoot a penalty kick. Percept. Mot. Skills 108, 139–148. doi: 10.2466/pms.108.1.139-148
Orquin, J. L., and Holmqvist, K. (2018). Threats to the validity of eye-movement research in psychology. Behav. Res. Methods 50, 1645–1656. doi: 10.3758/s13428-017-0998-z
Page, M. J., McKenzie, J. E., Bossuyt, P. M., Boutron, I., Hoffmann, T. C., Mulrow, C. D., et al. (2021). The PRISMA 2020 statement: an updated guideline for reporting systematic reviews. BMJ 372, n71. doi: 10.1136/bmj.n71
Park, J., Lee, Y., Seo, H., Jang, B., Son, H., Kim, S., et al. (2011). “Risk of bias assessment tool for non-randomized studies (RoBANS): development and validation of a new instrument,” in 19th Cochrane Colloquium (Madrid),19–22.
Park, S. (2003). Anticipation and acquiring processes of visual cues on a spiker's attack patterns and directions as a function of expertise in volleyball players. Int. J. Appl. Sports Sci. 15, 2.
Passos, P., Araújo, D., Davids, K., and Shuttleworth, R. (2008). Manipulating constraints to train decision making in rugby union. Int. J. Sports Sci. Coach. 3, 125–140. doi: 10.1260/174795408784089432
Posner, M. I. (1980). Orienting of attention. Q. J. Exp. Psychol. 32, 3–25. doi: 10.1080/00335558008248231
Ribeiro, L., Figueiredo, L., Pérez-Morales, J., Nascimento, G., Porto, D., and Greco, P. (2021). Tactical knowledge and visual search analysis of female handball athletes from different age groups. J. Phys. Educ. Sport 21, 948–955. doi: 10.7752/jpes.2021.02118
Rivilla-García, J., Muñoz Noval, A., Grande Rodriguez, I., Sanchís Almenara, M., and Sampedro Molinuevo, J. (2013). A comparative analysis of visual strategy in elite and amateur handball goalkeepers. J. Hum. Sport Exerc. 8, 743–753. doi: 10.4100/jhse.2013.8.Proc3.21
Roca, A., Ford, P., McRobert, A., and Williams, A. M. (2011). Identifying the processes underpinning anticipation and decision-making in a dynamic time-constrained-task. Cogn. Process. 12, 301–310. doi: 10.1007/s10339-011-0392-1
Roca, A., Ford, P. R., McRobert, A. P., and Williams, A. M. (2013). Perceptual-cognitive skills and their interaction as a function of task constraints in soccer. J. Sport Exerc. Psychol. 35, 144–155. doi: 10.1123/jsep.35.2.144
Roca, A., Ford, P. R., and Memmert, D. (2018). Creative decision making and visual search behavior in skilled soccer players. PLoS ONE 13, e0199381. doi: 10.1371/journal.pone.0199381
Roca, A., Williams, A. M., and Ford, P. R. (2012). Developmental activities and the acquisition of superior anticipation and decision making in soccer players. J. Sports Sci. 30, 1643–1652. doi: 10.1080/02640414.2012.701761
Rosenholtz, R. (2016). Capabilities and limitations of peripheral vision. Ann. Rev. Vis. Sci. 2, 437–457. doi: 10.1146/annurev-vision-082114-035733
Russell, S., Jenkins, D., Smith, M., Halson, S., and Kelly, V. (2019). The application of mental fatigue research to elite team sport performance: new perspectives. J. Sci. Med. Sport 22, 723–728. doi: 10.1016/j.jsams.2018.12.008
Sáez-Gallego, N. M., Vila-Maldonado, S., Abellán Hernández, J., and Contreras Jordán, O. R. (2013). Analysis of the visual search behaviour and decision making skill in blocking action in volleyball. Cuadernos Psicol. Deporte 13, 31–43. doi: 10.4321/S1578-84232013000200004
Sarpeshkar, V., Abernethy, B., and Mann, D. L. (2017). Visual strategies underpinning the development of visual–motor expertise when hitting a ball. J. Exp. Psychol. Hum. Percept. Perform. 43, 1744. doi: 10.1037/xhp0000465
Schober, P., and Vetter, T. R. (2019). Chi-square tests in medical research. Anesth. Analg. 129, 1193. doi: 10.1213/ANE.0000000000004410
Schorer, J., and Baker, J. (2009). An exploratory study of aging and perceptual-motor expertise in handball goalkeepers. Exp. Aging Res. 35, 1–19. doi: 10.1080/03610730802544641
Shank, M. D., and Haywood, K. M. (1987). Eye movements while viewing a baseball pitch. Percept. Motor Skills 64(3_suppl.), 1191–1197. doi: 10.2466/pms.1987.64.3c.1191
Shepherd, M., Findlay, J. M., and Hockey, R. J. (1986). The relationship between eye movements and spatial attention. Q. J. Exp. Psychol. 38A, 475–491. doi: 10.1080/14640748608401609
Shi, L., and Lin, L. (2019). The trim-and-fill method for publication bias. Medicine 98, e15987. doi: 10.1097/MD.0000000000015987
Sierra-Díaz, M., González-Víllora, S., Pastor-Vicedo, J., and Serra-Olivares, J. (2017). Soccer and relative age effect: a walk among elite players and young players. Sports 5, 5. doi: 10.3390/sports5010005
Starkes, J. L., and Ericsson, K. A. (2003). Expert Performance in Sports: Advances in Research on Sport Expertise. IL: Human Kinetics Champaign.
Sterne, J. A. C., Sutton, A. J., Ioannidis, J. P. A., Terrin, N., Jones, D. R., Lau, J., et al. (2011). Recommendations for examining and interpreting funnel plot asymmetry in meta-analyses of randomised controlled trials. BMJ 343, d4002–d4002. doi: 10.1136/bmj.d4002
Swann, C., Moran, A., and Piggott, D. (2015). Defining elite athletes: Issues in the study of expert performance in sport psychology. Psychol. Sport Exerc. 16, 3–14. doi: 10.1016/j.psychsport.2014.07.004
Uchida, Y., Kudoh, D., Higuchi, T., Honda, M., and Kanosue, K. (2013). Dynamic visual acuity in baseball players is due to superior tracking abilities. Med. Sci. Sports Exerc. 45, 319–325. doi: 10.1249/MSS.0b013e31826fec97
Uchida, Y., Mizuguchi, N., Honda, M., and Kanosue, K. (2014). Prediction of shot success for basketball free throws: visual search strategy. Eur. J. Sport Sci. 14, 426–432. doi: 10.1080/17461391.2013.866166
Vaeyens, R., Lenoir, M., Williams, A. M., Mazyn, L., and Philippaerts, R. M. (2007a). The effects of task constraints on visual search behavior and decision-making skill in youth soccer players. J. Sport Exerc. Psychol. 29, 147–169. doi: 10.1123/jsep.29.2.147
Vaeyens, R., Lenoir, M., Williams, A. M., and Philippaerts, R. M. (2007b). Mechanisms underpinning successful decision making in skilled youth soccer players: an analysis of visual search behaviors. J. Mot. Behav. 39, 395–408. doi: 10.3200/JMBR.39.5.395-408
van der Kamp, J. (2011). Exploring the merits of perceptual anticipation in the soccer penalty kick. Motor Control 15, 342–358. doi: 10.1123/mcj.15.3.342
van Maarseveen, M. J., Oudejans, R. R., and Savelsbergh, G. J. (2015). Pattern recall skills of talented soccer players: two new methods applied. Hum. Mov. Sci. 41, 59–75. doi: 10.1016/j.humov.2015.02.007
Vansteenkiste, P., Vaeyens, R., Zeuwts, L., Philippaerts, R., and Lenoir, M. (2014). Cue usage in volleyball: a time course comparison of elite, intermediate and novice female players. Biol. Sport 31, 295–302. doi: 10.5604/20831862.1127288
Vater, C., Luginbühl, S., and Magnaguagno, L. (2019). Testing the functionality of peripheral vision in a mixed-methods football field study. J. Sports Sci. 37, 2789–2797. doi: 10.1080/02640414.2019.1664100
Vickers, J. N. (1996a). Control of visual attention during the basketball free throw. Am. J. Sports Med. 24, 93–96. doi: 10.1177/036354659602406S25
Vickers, J. N. (1996b). Visual control when aiming at a far target. J. Exp. Psychol. Hum. Percept. Perform. 22, 342. doi: 10.1037/0096-1523.22.2.342
Vickers, J. N. (2007). Perception, Cognition and Decision Training: The Quiet Eye in Action. Champaign IL: Human Kinetics.
Vickers, J. N. (2009). Advances in coupling perception and action: the quiet eye as a bidirectional link between gaze, attention, and action. Progr. Brain Res. 174, 279–288. doi: 10.1016/S0079-6123(09)01322-3
Vickers, J. N., and Adolphe, R. M. (1997). Gaze behaviour during a ball tracking and aiming skill. Int. J. Sports Vis. 4, 8–27.
Vickers, J. N., Causer, J., and Vanhooren, D. (2019). The role of quiet eye timing and location in the basketball three-point shot: a new research paradigm. Front. Psychol. 10, 2424. doi: 10.3389/fpsyg.2019.02424
Vítor de Assis, J., Costa, V., Casanova, F., Cardoso, F., and Teoldo, I. (2021). Visual search strategy and anticipation in tactical behavior of young soccer players. Sci. Med. Football 5, 158–164. doi: 10.1080/24733938.2020.1823462
Voss, M. W., Kramer, A. F., Basak, C., Prakash, R. S., and Roberts, B. (2010). Are expert athletes ‘expert’in the cognitive laboratory? A meta-analytic review of cognition and sport expertise. Appl. Cogn. Psychol. 24, 812–826. doi: 10.1002/acp.1588
Williams, A. M., and Davids, K. (1995). Declarative knowledge in sport: a byproduct of experience or a characteristic of expertise? J. Sport Exerc. Psychol. 17, 259–275. doi: 10.1123/jsep.17.3.259
Williams, A. M., and Davids, K. (1997). Assessing cue usage in performance contexts: a comparison between eye-movement and concurrent verbal report methods. Behav. Res. Methods Instrum. Comp. 29, 364–375. doi: 10.3758/BF03200589
Williams, A. M., and Davids, K. (1998). Visual search strategy, selective attention, and expertise in soccer. Res. Q. Exerc. Sport 69, 111–128. doi: 10.1080/02701367.1998.10607677
Williams, A. M., Davids, K., Burwitz, L., and Williams, J. G. (1994). Visual search strategies in experienced and inexperienced soccer players. Res. Q. Exerc. Sport 65, 127–135. doi: 10.1080/02701367.1994.10607607
Williams, A. M., Ford, P. R., Eccles, D. W., and Ward, P. (2011). Perceptual-cognitive expertise in sport and its acquisition: implications for applied cognitive psychology. Appl. Cogn. Psychol. 25, 432–442. doi: 10.1002/acp.1710
Wu, Y., Zeng, Y., Zhang, L., Wang, S., Wang, D., Tan, X., et al. (2013). The role of visual perception in action anticipation in basketball athletes. Neuroscience 237, 29–41. doi: 10.1016/j.neuroscience.2013.01.048
Keywords: decision-making, expertise, attention, gaze behavior, eye movements, motor behavior
Citation: Silva AF, Afonso J, Sampaio A, Pimenta N, Lima RF, Castro HdO, Ramirez-Campillo R, Teoldo I, Sarmento H, González Fernández F, Kaczmarek A, Oniszczuk A and Murawska-Ciałowicz E (2022) Differences in visual search behavior between expert and novice team sports athletes: A systematic review with meta-analysis. Front. Psychol. 13:1001066. doi: 10.3389/fpsyg.2022.1001066
Received: 22 July 2022; Accepted: 01 August 2022;
Published: 22 September 2022.
Edited by:
Antonino Bianco, University of Palermo, ItalyReviewed by:
Javier Raya-González, Universidad Isabel I de Castilla, SpainGustavo De Conti Teixeira Costa, Universidade Federal de Goiás, Brazil
Copyright © 2022 Silva, Afonso, Sampaio, Pimenta, Lima, Castro, Ramirez-Campillo, Teoldo, Sarmento, González Fernández, Kaczmarek, Oniszczuk and Murawska-Ciałowicz. This is an open-access article distributed under the terms of the Creative Commons Attribution License (CC BY). The use, distribution or reproduction in other forums is permitted, provided the original author(s) and the copyright owner(s) are credited and that the original publication in this journal is cited, in accordance with accepted academic practice. No use, distribution or reproduction is permitted which does not comply with these terms.
*Correspondence: Ana Filipa Silva, YW5hZmlsc2lsdmFAZ21haWwuY29t