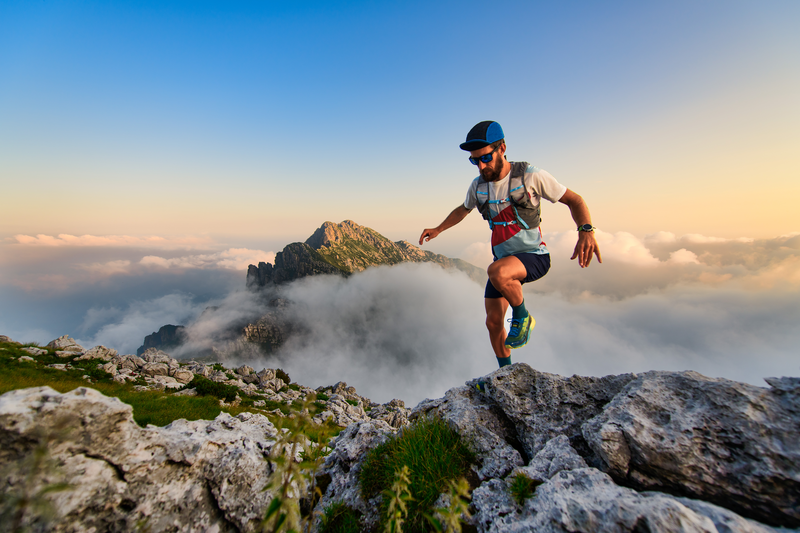
95% of researchers rate our articles as excellent or good
Learn more about the work of our research integrity team to safeguard the quality of each article we publish.
Find out more
SYSTEMATIC REVIEW article
Front. Psychol. , 29 January 2021
Sec. Movement Science
Volume 12 - 2021 | https://doi.org/10.3389/fpsyg.2021.528352
Background: Positive effects of acute exercise on cognitive performances in general inspired research that investigated the effects of acute exercise on specific cognitive subdomains. Many existing studies examined beneficial effects of acute exercise on subsequent set shifting performance in healthy adults. Set shifting, a subdomain of executive function, is the ability to switch between different cognitive sets. The results of existing studies are inconsistent. Therefore, a meta-analysis was conducted that pooled available effect sizes. Additionally, moderator analyses were carried out to identify covariates that determine the magnitude of exercise-induced set shifting benefits.
Methods: Medline, PsycINFO, and SPORTDiscus were searched for eligible studies. Hedges' g corrected standardized mean difference values were used for analyses. Random-effects weights were applied to pool effects. Potential moderation of the effect of acute exercise on subsequent set shifting performance by exercise intensity, type of exercise, participants' age, and type of control group were examined.
Results: Twenty-two studies (N = 1,900) were included into analysis. All aggregated effect sizes ranged from small to moderate. Overall, a small significant beneficial effect was revealed (g = −0.32, 95 % CI −0.45 to −0.18). Heterogeneity of included effect sizes was moderate and significant (T2 = 0.0715, I2 = 46.4%, (p < 0.0016). Moderator analyses revealed a larger average effect in older adults than for studies examining younger adults (−0.42 vs. −0.29). Light exercise (−0.51) led to larger effects than moderate (−0.24) or vigorous exercise (−0.29). Studies testing acute exercise against active control groups showed a noticeably smaller average effect (−0.13) than studies that used passive (−0.38) or cognitive engaging control groups (−0.34). Interestingly, application of resistance or aerobic exercise led to no different average effect sizes (−0.30 vs. −0.32). However, none of the tested covariates reached statistical significance.
Conclusion: Acute exercise improves subsequent set shifting performance. However, effect sizes are small, making the relevance for everyday life questionable. The results indicate that older adults benefit more from acute exercise than younger adults do. Light intensity exercise seems most effective while the type of exercise does not seem to influence the magnitude of effects. Research designs with active control groups show the smallest average effect, raising concerns about placebo effects.
PROSPERO registration number: CRD42019138799
The investigated effects of acute exercise on the cardiovascular, respiratory, musculoskeletal and other organic systems (Mathews and Fox, 1976) inspired research to examine whether acute exercise has an influence on cognitive functioning as well (Tomporowski and Ellis, 1986). After several years, studies revealed beneficial effects of acute exercise on the central nervous system. Among others, it increases prefrontal oxygenation (Endo et al., 2013), cortical activation (Yanagisawa et al., 2010), neurotrophin (Schmolesky et al., 2013) and catecholamine expression (Chmura et al., 1994), and it improves the metabolic status of cerebral neurons (Dalsgaard et al., 2004). These positive physiological adaptations to acute exercise within the central nervous system once again raised the question whether cognitive performances might also benefit as a result even more. In particular, potential effects of acute exercise on subsequent higher cognitive performances are in the focus of today's research interest. Accordingly, this meta-analysis focuses on the potential effects of acute exercise on subsequent set shifting performance. Set Shifting is a subcomponent of executive function (Pennington and Ozonoff, 1996; Miyake et al., 2000; Alvarez and Emory, 2006; Diamond, 2013) and is often used synonymously for the more common term “task switching,” which is owed to vague minor differences in the definitions of both terms (Rogers and Monsell, 1995; Rushworth et al., 2005; Ravizza and Carter, 2008; Koch et al., 2018). Set shifting implies the ability to switch between cognitive sets by altering behavior and actions due to changing conditions (Cools, 2001). In other words, being capable of moving back and forth between tasks, operations or mental sets (Miyake et al., 2000). This distinguishes set shifting from multitasking, which is defined as a cognitive process involved in performing two or more tasks at the same time (Koch et al., 2018). Set shifting was shown to predict problem solving abilities (Senn et al., 2004) and is required for social competence as well (Bierman et al., 2008).
Still, the exact neurobiological mechanisms by which the process of set shifting occurs are still unknown (Ludyga et al., 2016; Pontifex et al., 2019). However, it was shown that the prefrontal and posterior parietal cortices are mainly involved in making set shifts possible (Miyake et al., 2000; Pa et al., 2010) and that the dorsolateral prefrontal cortex is more active in exactly these situations (Meyer et al., 1998; MacDonald et al., 2000). Due to the above-mentioned neurobiological influence acute exercise has on the central nervous system in addition to the reported involvement of different cortical areas, potential effects of acute exercise on subsequent set shifting performance are assumed (Chang et al., 2012). Often examined neurobiological factors on set shifting performance are catecholamines (Arnsten, 2011) and the brain-derived neurotrophic factor (BDNF) (Nakazato et al., 2009; Gajewski et al., 2011; van der Kolk et al., 2015). Both factors are shown to react strongly to acute exercise (Knaepen et al., 2010; Schmolesky et al., 2013). It is important to note that this meta-analysis focusses on the effect of acute exercise on behavioral set shifting performance and not on potential neurobiological moderators.
Many studies exist that examine the effects of acute exercise on subsequent behavioral set shifting performance (Coles and Tomporowski, 2008; Barenberg et al., 2015; Crush and Loprinzi, 2017). The results are inconsistent. While several studies showed beneficial effects (Murray and Russoniello, 2012; Jaffery et al., 2018; Naderi et al., 2018), other studies show no effects (Frith et al., 2017) or even detrimental effects (Basso et al., 2015). In an attempt to clarify this disparity, this meta-analysis is the first to examine potential moderators that might influence the effect of an acute exercise bout on subsequent set shifting performance. Differences in the intensity of applied exercise might explain the inconsistency of results. It is assumed that intensity is a crucial covariate that determines quality and magnitude of physiological adaptations to acute exercise within the central nervous system (Knaepen et al., 2010; McMorris, 2016). Another potential moderator of the effect of acute exercise on subsequent set shifting is the type of exercise. Resistance and aerobic exercise represent distinct spectra of exercise defined by different physical demands, e.g., cardiovascular, metabolic, or musculoskeletal (Pontifex et al., 2009).
Apart from features of the exercise regimen, characteristics of examined participants might also explain inconsistency of existing studies that examined the effect of acute exercise on subsequent set shifting performance. Executive function performances are considered to decrease during older adulthood (Brydges et al., 2013; Pettigrew and Martin, 2014). On the contrary, young healthy adults seem to be at the peak of their cognitive performance (Zelazo et al., 2004). There is evidence, which indicates that individuals with lower baseline cognitive performance benefit most from acute exercise (Sibley and Beilock, 2007; Drollette et al., 2014; Dimitrova et al., 2017). Therefore, it is conceivable that participants' age plays a moderating role in exercise-induced set shifting benefits.
Finally, methodological features might explain variability of reported effects of acute exercise on subsequent set shifting. Particularly, the type of applied control group was discussed. It was hypothesized that in research designs with passive control groups expectation-driven placebo effects might play a role (Oberste et al., 2019).
In this systematic review and meta-analysis, an overview of the current research of the effects of acute exercise on subsequent set shifting performance in healthy adults is provided. Moreover, moderator analyses are carried out to identify covariates that determine the magnitude of exercise-induced set shifting benefits. The intensity of the applied exercise, the type of exercise, participants' age, and the type of control group are checked for moderation of the effect of acute exercise on subsequent set shifting performance.
This meta-analysis followed the PRISMA reporting guidelines (Moher et al., 2009). We provide the PRISMA checklist in the Supplementary Material to this article. The key features of this review were registered in advance on PROSPERO. (registration number: CRD42019138799).
Studies were eligible for this review if they were published peer-reviewed in English language. The year of publication was not a limiting factor. Eligibility criteria for this review were defined based on the PICOS approach:
Studies were included if they investigated healthy adults. Adult was defined as 18 years of age or older. Studies were excluded if they investigated participants under 18 years of age, ill individuals, or animals.
We included studies that applied a single aerobic or resistance exercise session of 5–60 min duration and of a light, moderate or vigorous intensity. Resistance and aerobic exercise were understood as defined by the American College of Sports Medicine (ACSM). They define aerobic exercise as any activity that uses large muscle groups, can be maintained continuously and is rhythmic in nature (Pescatello et al., 2014). Resistance exercise includes free weights, machines with stacked weights or pneumatic resistance, and even resistance bands (Pescatello et al., 2014). The division into light, moderate and vigorous intensity was based on the definitions by Norton et al. (2010) for the aerobic exercises (Norton et al., 2010), and by Jovanovic (2013) for the resistance exercises (Jovanović and Flanagan, 2014).
Trials were included if they compared the effects of acute exercise against a control condition that did not exceed the threshold for light intensity as defined by Norton et al. (2010) and Jovanović and Flanagan (2014). Studies were excluded if they compared only the effects of two exercise treatment groups without a control group.
Studies were included if they measured behavioral set shifting performance as a primary or secondary endpoint. Studies were excluded if they measured behavioral set shifting performance only during exercise.
Studies were included if they applied a randomized crossover study design or a randomized controlled trial study design. Studies with other designs were excluded.
using one of the following neuropsychological tests for assessing set shifting performance were included in this meta-analysis. The Trail Making Test (De Oliveira-Souza et al., 2000; Moll et al., 2002; Sánchez-Cubillo et al., 2009), the Wisconsin Card Sorting Task (Konishi et al., 1998; Gamboz et al., 2009), the Switch Trial test (Rogers and Monsell, 1995), the Dimension Switching Task (Boucard et al., 2012), the Plus-Minus Task (Boucard et al., 2012), the More Odd Task (Chen et al., 2014), the Task Switching Test (Dai et al., 2013) and the Visual Switch Task (Tomporowski and Ganio, 2006) are defined as valid measures of set shifting performance. A detailed description including the instructions for each of the above stated tests can be found in the Supplementary Material.
The literature search was last updated on the 25th of May 2020, using the electronic databases Medline, SPORTDiscus and PsychINFO via EBSCO host. To determine relevant studies, the terms “exercise,” “sport,” “physical activity,” “physical exertion,” “physical training,” “running,” “jogging,” “walking,” “bicycling,” “strength training” were combined with “cognition,” “executive function,” “set shifting,” “reaction time” and “attention.” The exact search algorithm can be found in the Supplementary Material to this review. The literature search was conducted independently by two members of the review team (S.S. and M.O.). Inconsistencies were solved by discussion.
For included studies, the number of participants in each group, as well as the group means and standard deviations of measures of set shifting performance were extracted. Moreover, we extracted information of potential moderators (exercise intensity, type of exercise, participants' age, and type of control group). If measures were not reported in the full-text of the study, the corresponding author was contacted, and the raw data was requested. If the results were presented in form of a graphic, data was extracted using the WebPlotDigitizer (Tsafnat et al., 2014). For studies with multiple treatment arms that did not deviate regarding here tested moderators, the data was summarized according to the methods suggested by Higgins and Deeks (2011). In case of a repeated testing of the participants, the measurement taken first was used. Data extraction was assessed independently by two members of the review team (S.S. and M.O.). Disagreements were solved by discussion.
To assess methodological quality, two authors (S.S. and M.O.) independently rated each included study according to the Physiotherapy Evidence Database (PEDro) scale. The PEDro scale consists of the following 11 items: (1) eligibility criteria, (2) random allocation, (3) concealed allocation, (4) baseline comparability, (5) blinding of subjects, (6) blinding of therapists, (7) blinding of assessors, (8) completeness of follow-up, (9) intention-to-treat-analysis, (10) between group statistical comparisons, (11) point estimates and variability. However, in trials investigating effects of physical exercise interventions true blinding is difficult to achieve for both, participants and for therapists. Therefore, in this review the criteria (5) blinding of subjects and (6) blinding of therapists have not been taken into account (de Morton, 2009) and the highest possible PEDro score that can be achieved is nine instead of 11. If a criterion is clearly met, it is rewarded with one point and is rated as “low risk of bias.” If a criterion is not fulfilled, it is rated as “high risk of bias” and no point is given. If the respective study does not share the information needed for rating, it is rated as “unclear risk of bias.” The initial level of agreement between the ratings by the authors was excellent [intraclass correlation coefficient [ICC] = 0.93]. Discrepancies were solved by discussion and checking the full text.
Potential moderation of exercise intensity was investigated. Intensities of acute exercise were classified into (1) light, (2) moderate, and (3) vigorous exercise intensity. An acute aerobic exercise session was defined as light, if the article reported a rating of perceived exertion between 8 and 10 on the Borg scale, a heart rate between 40 and 55% of maximum heart rate, a heart rate between 20 and 40% of heart rate reserve, or an oxygen uptake between 20 and 40% of maximum oxygen uptake. An acute aerobic exercise session was defined as moderate, if the article reported a rating of perceived exertion between 11 and 13 on the Borg Scale, a heart rate between 56 and 70% of maximum heart rate, a heart rate between 41 and 60% of heart rate reserve, or an oxygen uptake between 41 and 60% of maximum oxygen uptake. An acute aerobic exercise session was defined as vigorous, if the article reported a rating of perceived exertion between 14 and 16 on the Borg Scale, a heart rate between 71 and 90% of maximum heart rate, a heart rate between 61 and 85% of heart rate reserve, or an oxygen uptake between 60 and 85% of maximum oxygen uptake (Norton et al., 2010). An acute resistance exercise session was defined as light, if the article reported an exercise intensity of 61–70% of the participant's individual repetition maximum. It was defined as moderate, if the article reported an exercise intensity of 71–80% of the participants‘ individual repetition maximum. Finally, an acute resistance exercise session was defined as vigorous, if the article reported an exercise intensity of 81–100% of the participants‘ individual repetition maximum (Jovanović and Flanagan, 2014).
Included studies were subdivided in two different subgroups based on whether an (1) aerobic exercise session or a (2) resistance exercise session was applied.
Studies were divided into those that examined (1) young adults (18–34 years of age) and those that examined (2) older adults (35+ years of age).
The included studies were subdivided according to the used control group paradigm. According to Pontifex et al., we distinguished (1) active control, (2) cognitive engaged control, and (3) passive control groups. Active control groups are physically active, but at such a low intensity, that there is no increase in heart rate. Passive control groups are physically inactive and non-supervised. Cognitive engaging control groups are instructed to perform activities with cognitive stimulation, like watching TV, reading a book, or scrolling on a smartphone.
For data analyses, “R” software with the “meta” package (Schwarzer and Carpenter, 2015) was used. As primary meta-analysis, we examined the effect of acute exercise on subsequent set shifting performance in healthy adults. We calculated the “bias corrected Hedges' g standardized mean difference” (SMD) between control group and exercise group at post-intervention for each study. The SMDs were pooled across studies using a random effects model. Interpretation of the size of pooled SMD followed Cohen's classification. Consequently, SMD values of 0.2, 0.5, and 0.8 were interpreted as small, moderate, and large effect sizes, respectively (Cohen, 2013). We quantified “between study heterogeneity,” calculating the T2 and the Higgins' I2 statistic. Higgins' I2 values of 25, 50, and 75% were interpreted as low, moderate and large proportion of between-study heterogeneity, respectively (Higgins et al., 2003). We conducted sensitivity analyses by excluding studies rated “high risk of bias” on PEDro scale items. Based on subgroup analyses, we examined the moderation potential of the above-described variables.
Through electronic database search, 6,859 publications were identified. The search of the references of recent meta-analyses (Chang et al., 2012; Verburgh et al., 2014; Ludyga et al., 2016) and included studies resulted in 5 additional records. After duplicate removal, titles and abstracts of 4,811 publications were screened. Four thousand, six hundred fifty-five records were excluded because the above defined eligibility criteria for inclusion in this review were not met. One-hundred and fifty-six publications remained, full texts were obtained and read. At this point, 134 studies were excluded for the following reasons: no healthy subjects, no measurement of set shifting performance, no control condition, assessment during exercise. Twenty-two studies, comprising the data from 1,900 participants, were included in a qualitative synthesis and meta-analysis. An overview of the selection process is provided as a PRISMA diagram in Figure 1.
Of the 22 included studies, nine studies applied a randomized controlled crossover and 13 studies applied a randomized controlled design. Twelve studies had multiple exercise treatment arms eligible for inclusion in this meta-analysis. Set shifting measures reported in included studies were: averaged or absolute reaction time to complete a defined number of trials, or the number of trials participants processed within a defined time frame. On average, the studies reached a PEDro summary score of 6.52 (SD = 0.93), which relates to a good average methodological quality of included studies (Teasell et al., 2007). An overview of the PEDro rated study quality is depicted in Figure 2. The PEDro ratings for each item and each included study can be found in the Supplementary Material to this article. An extensive summary of characteristics and PEDro summary scores of included studies can be found in Table 1.
Figure 2. Overview of the PEDro rated study quality (green = low risk of bias, yellow = unclear risk of bias, red = high risk of bias).
Table 1. Overview of studies included into meta-analysis on the aftereffect of acute exercise on set shifting performance in healthy adults.
It should be noted that negative SMDs represent a beneficial effect of acute exercise compared to control treatment as it reflects a decrease in time needed to complete the task. Thirty-five effect sizes from 22 studies were included in the analysis (1,900 participants). A small to moderate beneficial effect of acute aerobic exercise on subsequent set shifting performance was revealed (k = 35, Hedges' g = −0.32, 95% CI −0.45 to −0.18, p < 0.0001). A moderate heterogeneity amongst the included effect sizes was detected (T2 = 0.0715, I2 = 46.4%). Q-test revealed statistical significance of heterogeneity (p < 0.0016), supporting the assumption of random effects. No evident outliers were identified. An overall forest plot is presented in Figure 3.
Figure 3. The effect of acute exercise on subsequent set shifting performance compared to control in healthy adults: Overview of all included studies.
One or more included studies were rated “high risk of bias” for the PEDro scale items “eligibility criteria and source,” “baseline comparability, and “point estimates and variability.” If the studies that did not give sufficient information concerning eligibility criteria and source were excluded from analysis, the aggregated effect did not decrease while heterogeneity slightly increased compared to initial analysis (k = 32, Hedges' g = −0.39, 95% CI = −0.59 to −0.18, p < 0.001, T2 = 0.2521, I2 = 75.5%). If the studies that did not show comparable baseline results were excluded from analysis, the aggregated effect increased substantially. Again, heterogeneity increased (k = 30, Hedges' g = −0.43, 95% CI = −0.68 to −0.23, p < 0.001, T2 = 0.2931, I2 = 77.5%). If the studies that did not provide point estimates and/or variability were removed from the analysis, the aggregated effect turned out to be larger while the heterogeneity increased (k = 30, Hedges' g = −0.46, 95% CI = −0.68 to −0.23, p < 0.001, T2 = 0.2931, I2 = 75.5%). If all studies that were rated “high risk of bias” in at least one item of the PEDro scale were excluded from analysis, the aggregated effect increased just as the heterogeneity did (k = 21, Hedges' g = −0.52, 95% CI = −0.84 to −0.19, p < 0.001, T2 = 0.4476, I2 = 81.1%).
Table 2 provides a summary of the moderator analyses. The forest plots to subgroup analyses, which are not depicted in this article, are provided in the Supplementary Material to this article.
Six studies chose to examine the effects of a light exercise intensity on subsequent set shifting performance (k = 7, Hedges' g = −0.51, 95% CI −0.83 to −0.19, T2 = 0.0742, I2 = 37.8%). Eight studies had their participants perform an exercise bout of moderate intensity prior to measurement of set shifting performance (k = 9, Hedges' g = −0.24, 95% CI −0.50 to 0.03, T2 = 0.0742, I2 = 0%). Nineteen studies investigated the effects of a vigorous exercise intensity on subsequent set shifting performance (k = 19, Hedges' g = −0.29, 95% CI −0.47 to −0.11, T2 = 0.0742, I2 = 61.7%). No significant difference between subgroups was detected (Q-between = 1.83, df = 2, p = 0.4014).
In 19 included studies, participants performed a bout of acute aerobic exercise (k = 27, Hedges' g = −0.32, 95% CI −0.48 to −0.17, T2 = 0.0754, I2 = 47.6%) while four studies subjected their participants to an acute bout of resistance exercise (k = 8, Hedges' g = −0.30, 95% CI −0.58 to −0.03, T2 = 0.0754, I2 = 47.4%). Subgroups summary effects did not differ significantly from each other (Q-between = 0.02, df = 1, p = 0.8977).
Sixteen studies included in this meta-analysis investigated the effect of acute exercise on subsequent set shifting performance in younger adults (age 18–34) (k = 26, Hedges' g = −0.29, 95% CI −0.44 to −0.13, T2 = 0.0764, I2 = 23.1%). In contrast, five studies examined the effect of acute exercise on subsequent set shifting performance in older adults (age 35+) (k = 9, Hedges' g = −0.42, 95% CI −0.72 to −0.13, T2 = 0.0764, I2 = 52.8%). The forest plot to this subgroup analysis is depicted in Figure 4. The difference between the subgroups' summary effects did not reach statistical significance (Q-between =0.60, df = 1, p = 0.4383).
Three studies included in this meta-analysis decided to use a physically active control group as a standard of comparison to the acute exercise condition (k = 6, Hedges' g = −0.13, 95% CI −0.46 to 0.20, T2 = 0.0742, I2 = 0%). Twelve studies used a cognitive engaging control treatment (k=16, Hedges' g = −0.34, 95% CI −0.53 to −0.15, T2 = 0.0742, I2 = 71.7%). Nine studies compared the acute exercise condition with a physically inactive and therefore passive control treatment (k=13, Hedges' g = −0.38, 95% CI −0.62 to −0.14, T2 =0.0742, I2 = 0%). The difference between subgroups summary effects was not statistically significant (Q-between = 1.49, df = 2, p = 0.4742).
This meta-analysis showed significant small to moderate effects of acute aerobic exercise on subsequent set shifting performance in healthy adults (g = −0.32). Sensitivity analyses show that this effect is unlikely due to bias of individual studies. Whether the small to moderate benefit in time when shifting between two different tasks is of practical relevance is difficult to evaluate. In special situations, where fast set-shifting performance is required, as in professional e-sports, a small speed advantage might make a decisive difference. By contrast, in the context of average everyday work life in an office for example, these exercise-induced benefits will hardly mean a noticeable improvement.
In addition, this meta-analysis investigated the influence of potential moderators. Light exercise intensity showed a moderate effect on subsequent set shifting performance (g = −0.51). However, moderate (g = −0.24) and vigorous exercise intensity (g = −0.29) showed only a small to moderate effect. This result may be explained by the different physiological responses to acute exercise at light and moderate/vigorous intensity. Moderate and vigorous exercise induce more fatigue and dehydration, which are assumed to have a disadvantageous effect on subsequent cognitive performances (Cian et al., 2001). Results of existing meta-analyses investigating the effect of the exercise intensity on executive function are inconsistent. Contrasting to the present analysis, McMorris and Hale (2012) showed a bigger improvement in inhibition, working memory and set shifting performances following an exercise session of moderate intensity than of light or vigorous intensity (McMorris and Hale, 2012). In contrast, Loprinzi and Kane (2015) could not show any differences between a light, moderate or vigorous exercise intensity on set shifting performance at all (Loprinzi and Kane, 2015). While it still remains unclear which exercise intensity should be chosen to achieve the highest improvement in cognitive performances in general, this meta-analysis suggests that a light exercise intensity leads to the highest increase in subsequent set shifting performance.
The comparison of a single bout of aerobic exercise with a single bout of resistance exercise resulted in nearly identical beneficial effects on subsequent set shifting performance. Acute aerobic (g = −0.32) and resistance exercise (g = −0.30) both have a small to moderate beneficial effect on subsequent set shifting performance. This indicates that the previously mentioned differences regarding physical demands do not seem to play a decisive role in the extent to which each type of exercise has an influence on set shifting performance. Therefore, the focus seems to lie on the subsequent physical effects that both types of exercise have in common. Both lead to an increase of cerebral blood flow, which is considered to facilitate waste clearing and corresponding metabolic resource availability. Previous studies support the idea that these effects may ease cognitive processing (Delp et al., 2001; Pereira et al., 2007). Furthermore, both types of exercise lead to a release of catecholamines, which is seen to have an influence on set shifting (Robbins and Roberts, 2007; Bondi et al., 2010), and working memory (McMorris et al., 2016) as well. There is however almost no difference in the extent of the effects both types of exercise have on subsequent set shifting performance.
Although not statistically significant, the results revealed that older adults (g = −0.42) benefit noticeably more from an acute exercise bout than younger adults (g = −0.29) do. One potential explanation for these differences could be different baseline performances in young healthy and older adults. Previous studies showed the most pronounced benefits of acute exercise on executive function, especially on inhibition and working memory, in individuals with low baseline cognitive performance (Sibley and Beilock, 2007; Drollette et al., 2014). According to statements by Zelazo et al. (2004) there is a “rise and fall” of executive function across the human life span, which indicates peak performance during young adulthood and lower performance in older adulthood (Zelazo et al., 2004). Therefore, older adults, with lower baseline performance, might benefit more from acute exercise than young adults with higher baseline set shifting performance. A possible reason for the reduced increase in set shifting performance following a single bout of acute exercise in younger adults is the occurrence of a ceiling effect. Since cognitive performance is expected reaching its peak in the age group of young adults (Zelazo et al., 2004), there seems less room for improvement (Kamijo et al., 2007; O'Leary et al., 2011; Bullock and Giesbrecht, 2014). The results of this meta-analysis with respect to the participants' age were in accordance with these assertions regarding cognitive performance in general. Therefore, it can be assumed that they hold true for the specific subdomain set shifting as well.
Acute exercise led to a moderately higher set shifting performance when compared to passive (g = −0.38) or cognitive engaged (g = −0.34) control groups. By contrast, there was only a very small improvement when acute exercise was compared to active control groups (g = −0.13). These differences in subgroups‘ effect sizes could be explained by the potential occurrence of a placebo effect. In studies investigating the effect of acute physical activity, participants are aware of the physical demands they are being exposed to. Therefore, participants of the active control group are more likely to believe that they are part of the experimental condition, which controls better for expectations of cognitive benefits between the experimental and control group. Conversely, it is more likely for the participants in the passive control group to realize that they are part of the control condition. Therefore, differences in expectations for cognitive benefits between experimental and passive/cognitive engaged control groups are perceivable. Expectations play a key role in the placebo effect (Brown, 2015). The results of this analysis indicate that the magnitude of the effect of acute exercise on set shifting might be overestimated due to Placebo-effect. However, it should also be noted that the use of a placebo or an active control group in a trial might also lead to reduced effects in treatment groups. Negative expectations of the participants due to the chance of receiving the placebo are responsible for that. This effect has been named the “lessebo effect” (Mestre et al., 2014). Despite these differences of effect sizes in each subgroup, none of these results could be regarded as statistically significant. This might be explained by the small number of included effect sizes (35 effect sizes from 22 studies), and the resulting low statistical power. However, it diminishes the generalizability of the here achieved results.
The here presented results must be interpreted against the background of limitations. The results of this meta-analysis are only valid within the boundaries of the stated in- and exclusion criteria. Moreover, this meta-analysis was not able to differentiate between local and global switch cost as a separately primary endpoint. Since only three (Coles and Tomporowski, 2008; Barenberg et al., 2015; Naderi et al., 2018) of the 22 included studies used a variation of the Task-Switching Paradigm as neuropsychological test and therefore could report results for either global or local switch cost, a quantitative analysis for each variable was not possible. Future studies in this field should apply neuropsychological tests that allow a differentiation between local and global switch cost to enable further knowledge in this scientific field.
Another limitation is that meta-analytic subgroups analysis is observational. This means that randomization of participants in single studies does not apply to distinguishing between subgroups of studies. Therefore, systematic differences between subgroups concerning other factors than the subgroup defining cannot be ruled out. Several included studies conducted the neuropsychological testing of set shifting performance repeatedly for different time intervals after exercise cessation. To avoid severe issues with double counts and unit-of-analysis-error in our analysis, these multiple treatment arms were combined to a single pair-wise comparison following Higgins and Deeks' recommendations (Higgins and Deeks, 2011). Therefore, this meta-analysis could not investigate the influence of the different time intervals of test administration on set shifting performance. Future studies should continue to systematically investigate potential moderators with regards to the timing of test administration. This meta-analysis does not give a final answer on the internal validity of research on acute exercise induced benefits for set shifting performance in healthy adults. Potential moderation of widespread methodological shortcomings in that field, like e.g., “concealed allocation” and “blinding of assessors” remain unclear. Future meta-analyses should investigate the moderating influence of these factors. They should also apply a higher methodological standard to increase their internal validity. Also, a two, or more, factor analysis that investigates potential interaction effects between moderators is beyond the scope of our article. Also, lacking theory-driven approaches to the effects of exercise on cognition limit advances in this research field. Although research has started to investigate promising neurobiological factors, the underlying neurobiological mechanisms are still unknown. A deeper understanding of these mechanisms is important for more precise hypotheses, which aspects of cognition may be influenced or even immune to physical activity. As the understanding of biological processes responsible for changes in executive functions is still limited, further research on these underlying mechanisms is encouraged. Finally, this meta-analysis only focused on the effect of acute exercise on subsequent set shifting performance. Long-term exercise interventions are likely to lead to more pronounced positive effects because they are comprised of repetitive acute sessions. Future meta-analyses should focus on acute and long-term exercise and investigate the underlying neurobiological mechanisms.
This meta-analysis shows that a single bout of acute exercise improves subsequent set shifting performance in healthy adults. However, effects are of small size making relevance for everyday life is questionable. Older adults show moderate beneficial effects, but also young adults being at their peak of cognitive performance still benefit from an acute exercise session. The type of exercise does not influence magnitude of effect sizes, which implies that the improvement in subsequent set shifting performance is due to physical adaptations to the exercise, regardless of its type. Moderate and vigorous exercise intensities both lead too small to moderate beneficial effects in subsequent set shifting performance, while a light exercise intensity results in a moderate beneficial effect. Participants who are part of an active control group show higher improvements in set shifting performance than participants of a passive or cognitive engaged control group, which could be a result of an expectation driven placebo effect.
MO, SS, WB, and PZ planned the project. MO and SS conducted literature search, data extraction, and risk of bias assessment. Inconsistencies were resolved in consultation with WB and PZ. MO conducted data analyses. MO and SS wrote the manuscript, and concerning underlying neurobiological effects of acute aerobic exercise. SS communicated with study authors in case of missing data. WB supervised. All authors proofread the manuscript.
The authors declare that the research was conducted in the absence of any commercial or financial relationships that could be construed as a potential conflict of interest.
We would like to thank James Bonorris for proofreading the manuscript.
The Supplementary Material for this article can be found online at: https://www.frontiersin.org/articles/10.3389/fpsyg.2021.528352/full#supplementary-material
Aguirre-Loaiza, H., Arenas, J., Arias, I., Franco-Jímenez, A., Barbosa-Granados, S., Ramos-Bermúdez, S., et al. (2019). Effect of acute physical exercise on executive functions and emotional recognition: analysis of moderate to high intensity in young adults. Front. Psychol. 10:2774. doi: 10.3389/fpsyg.2019.02774
Alvarez, J. A., and Emory, E. (2006). Executive function and the frontal lobes: a meta-analytic review. Neuropsychol. Rev. 16, 17–42. doi: 10.1007/s11065-006-9002-x
Alves, C. R. R., Gualano, B., Takao, P. P., Avakian, P., Fernandes, R. M., Morine, D., et al. (2012). Effects of acute physical exercise on executive functions: a comparison between aerobic and strength exercise. J. Sport Exerc. Psychol. 34, 539–549. doi: 10.1123/jsep.34.4.539
Arnsten, A. F. T. (2011). Catecholamine influences on dorsolateral prefrontal cortical networks. Biol. Psychiatry 69, e89–e99. doi: 10.1016/j.biopsych.2011.01.027
Bae, S., and Masaki, H. (2019). Effects of acute aerobic exercise on cognitive flexibility required during task-switching paradigm. Front. Hum. Neurosci. 13:260. doi: 10.3389/fnhum.2019.00260
Barenberg, J., Berse, T., and Dutke, S. (2015). Ergometer cycling enhances executive control in task switching. J. Cogn. Psychol. 27, 692–703. doi: 10.1080/20445911.2015.1024256
Basso, J. C., Shang, A., Elman, M., Karmouta, R., and Suzuki, W. A. (2015). Acute exercise improves prefrontal cortex but not hippocampal function in healthy adults. J. Int. Neuropsychol. Soc. 21, 791–801. doi: 10.1017/S135561771500106X
Bierman, K. L., Nix, R. L., Greenberg, M. T., Blair, C., and Domitrovich, C. E. (2008). Executive functions and school readiness intervention: impact, moderation, and mediation in the Head Start REDI program. Dev. Psychopathol. 20, 821–843. doi: 10.1017/S0954579408000394
Bondi, C. O., Jett, J. D., and Morilak, D. A. (2010). Beneficial effects of desipramine on cognitive function of chronically stressed rats are mediated by alpha1-adrenergic receptors in medial prefrontal cortex. Prog. Neuropsychopharmacol. Biol. Psychiatry 34, 913–923. doi: 10.1016/j.pnpbp.2010.04.016
Boucard, G. K., Albinet, C. T., Bugaiska, A., Bouquet, C. A., Clarys, D., and Audiffren, M. (2012). Impact of physical activity on executive functions in aging: a selective effect on inhibition among old adults. J. Sport Exerc. Psychol. 34, 808–827. doi: 10.1123/jsep.34.6.808
Brown, W. A. (2015). Expectation, the placebo effect and the response to treatment. R. I. Med. J. 98, 19–21.
Brush, C. J., Olson, R. L., Ehmann, P. J., Osovsky, S., and Alderman, B. L. (2016). Dose-response and time course effects of acute resistance exercise on executive function. J. Sport Exerc. Psychol. 38, 396–408. doi: 10.1123/jsep.2016-0027
Brydges, C. R., Anderson, M., Reid, C. L., and Fox, A. M. (2013). Maturation of cognitive control: delineating response inhibition and interference suppression. PLoS ONE. 8e69826. doi: 10.1371/journal.pone.0069826
Bullock, T., and Giesbrecht, B. (2014). Acute exercise and aerobic fitness influence selective attention during visual search. Front. Psychol. 5.
Chang, Y.-K., and Etnier, J. L. (2009). Effects of an acute bout of localized resistance exercise on cognitive performance in middle-aged adults: A randomized controlled trial study. Psychol. Sport Exerc. 10, 19–24. doi: 10.1016/j.psychsport.2008.05.004
Chang, Y. K., Labban, J. D., Gapin, J. I., and Etnier, J. L. (2012). “The effects of acute exercise on cognitive performance: a meta-analytic review”: Corrigendum. Brain Res. 1470:159. doi: 10.1016/j.brainres.2012.06.039
Chen, A. G., Yan, J., Yin, H. C., Pan, C. Y., and Chang, Y. K. (2014). Effects of acute aerobic exercise on multiple aspects of executive function in preadolescent children. Psychol. Sport Exerc. 5:1290. doi: 10.1016/j.psychsport.2014.06.004
Chen, F. T., Etnier, J. L., Wu, C. H., Cho, Y. M., Hung, T. M., and Chang, Y. K. (2018). Dose-response relationship between exercise duration and executive function in older adults. J. Clin. Med. 7:279. doi: 10.3390/jcm7090279
Chmura, J., Nazar, K., and Kaciuba-Uściłko, H. (1994). Choice reaction time during graded exercise in relation to blood lactate and plasma catecholamine thresholds. Int. J. Sports Med. 15, 172–176.
Cian, C., Barraud, P. A., Melin, B., and Raphel, C. (2001). Effects of fluid ingestion on cognitive function after heat stress or exercise-induced dehydration. Int. J. Psychophysiol. Off. J. Int. Organ. Psychophysiol. 42, 243–251. doi: 10.1016/s0167-8760(01)00142-8
Cohen, J. (2013). Statistical Power Analysis for the Behavioral Sciences, 2nd Edn. Hoboken, NJ: Taylor and Francis. Available online at: http://gbv.eblib.com/patron/FullRecord.aspx?p=1192162
Coles, K., and Tomporowski, P. D. (2008). Effects of acute exercise on executive processing, short-term and long-term memory. J. Sports Sci. 26, 333–344. doi: 10.1080/02640410701591417
Cools, R. (2001). Mechanisms of cognitive set flexibility in Parkinson's disease. Brain. 124, 2503–2512. doi: 10.1093/brain/124.12.2503
Córdova, C., Silva, V. C., Moraes, C. F., Simões, H. G., and Nóbrega, O. T. (2009). Acute exercise performed close to the anaerobic threshold improves cognitive performance in elderly females. Brazil. J. Med. Biol. Res. 42, 458–464. doi: 10.1590/s0100-879x2009000500010
Crush, E. A., and Loprinzi, P. D. (2017). Dose-response effects of exercise duration and recovery on cognitive functioning. Percept. Mot. Skills 124, 1164–1193. doi: 10.1177/0031512517726920
Dai, C.-T., Chang, Y.-K., Huang, C.-J., and Hung, T.-M. (2013). Exercise mode and executive function in older adults: an ERP study of task-switching. Brain Cogn. 83, 153–162. doi: 10.1016/j.bandc.2013.07.007
Dalsgaard, M. K., Quistorff, B., Danielsen, E. R., Selmer, C., Vogelsang, T., and Secher, N. H. (2004). A reduced cerebral metabolic ratio in exercise reflects metabolism and not accumulation of lactate within the human brain. J. Physiol. 554(Pt 2), 571–578. doi: 10.1113/jphysiol.2003.055053
de Morton, N. A. (2009). The PEDro scale is a valid measure of the methodological quality of clinical trials: a demographic study. Aust. J. Physiother. 55, 129–133. doi: 10.1016/S0004-9514(09)70043-1
De Oliveira-Souza, R., Moll, J., Passman, L. J., Cunha, F. C., Paes, F., Adriano, M. V., et al. (2000). Trail making and cognitive set-shifting. Arq. Neuropsiquiatr. 58, 826–829. doi: 10.1590/s0004-282x2000000500006
Delp, M. D., Armstrong, R. B., Godfrey, D. A., Harold Laughlin, M., David Ross, C., and Keith Wilkerson, M. (2001). Exercise increases blood flow to locomotor, vestibular, cardiorespiratory and visual regions of the brain in miniature swine. J. Physiol. 533, 849–859. doi: 10.1111/j.1469-7793.2001.t01-1-00849.x
Diamond, A. (2013). Executive functions. Annu. Rev. Psychol. 64, 135–168. doi: 10.1146/annurev-psych-113011-143750
Dimitrova, J., Hogan, M., Khader, P., O'Hora, D., Kilmartin, L., Walsh, J. C., et al. (2017). Comparing the effects of an acute bout of physical exercise with an acute bout of interactive mental and physical exercise on electrophysiology and executive functioning in younger and older adults. Aging Clin. Exp. Res. 29, 959–967. doi: 10.1007/s40520-016-0683-6
Douris, P. C., Handrakis, J. P., Apergis, D., Mangus, R. B., Patel, R., Limtao, J., Platonova, S., Gregorio, A., and Luty, E. (2018). The effects of aerobic exercise and gaming on cognitive performance. J. Hum. Kinet. 61, 73–83. doi: 10.1515/hukin-2017-0134
Drollette, E. S., Scudder, M. R., Raine, L. B., Moore, R. D., Saliba, B. J., Pontifex, M. B., et al. (2014). Acute exercise facilitates brain function and cognition in children who need it most: An ERP study of individual differences in inhibitory control capacity. Dev. Cogn. Neurosci. 7, 53–64. doi: 10.1016/j.dcn.2013.11.001
Endo, K., Matsukawa, K., Liang, N., Nakatsuka, C., Tsuchimochi, H., Okamura, H., et al. (2013). Dynamic exercise improves cognitive function in association with increased prefrontal oxygenation. J. Physiol. Sci. JPS 63, 287–298. doi: 10.1007/s12576-013-0267-6
Frith, E., Sng, E., and Loprinzi, P. D. (2017). Randomized controlled trial evaluating the temporal effects of high-intensity exercise on learning, short-term and long-term memory, and prospective memory. Eur. J. Neurosci. 46, 2557–2564. doi: 10.1111/ejn.13719
Gajewski, P. D., Hengstler, J. G., Golka, K., Falkenstein, M., and Beste, C. (2011). The Met-allele of the BDNF Val66Met polymorphism enhances task switching in elderly. Neurobiol. Aging 32, 2327.e7–2327.e19. doi: 10.1016/j.neurobiolaging.2011.06.010
Gamboz, N., Borella, E., and Brandimonte, M. A. (2009). The role of switching, inhibition and working memory in older adults' performance in the Wisconsin Card Sorting Test. Aging, Neuropsychol. Cogn. 16, 260–284. doi: 10.1080/13825580802573045
Higgins, J. P., and Deeks, J. J. (2011). Chapter 07: selecting studies and collecting data. Cochrane Handb. Syst. Rev. Interv. 67, 275–281. doi: 10.1590/S1519-69842007000200012
Higgins, J. P. T., Thompson, S. G., Deeks, J. J., and Altman, D. G. (2003). Measuring inconsistency in meta-analyses testing for heterogeneity. BMJ. 2003:327. doi: 10.1136/bmj.327.7414.557
Hwang, J., Brothers, R. M., Castelli, D. M., Glowacki, E. M., Chen, Y. T., Salinas, M. M., et al. (2016). Acute high-intensity exercise-induced cognitive enhancement and brain-derived neurotrophic factor in young, healthy adults. Neurosci. Lett. 630, 247–253. doi: 10.1016/j.neulet.2016.07.033
Jaffery, A., Edwards, M. K., and Loprinzi, P. D. (2018). The effects of acute exercise on cognitive function: solomon experimental design. J. Prim. Prev. 39, 37–46. doi: 10.1007/s10935-017-0498-z
Jovanović, M., and Flanagan, E. P. (2014). Researched applications of velocity based strength training. J. Aust. Strength Cond. 22, 58–69.
Kamijo, K., Nishihira, Y., Higashiura, T., and Kuroiwa, K. (2007). The interactive effect of exercise intensity and task difficulty on human cognitive processing. Int. J. Psychophysiol. 65, 114–121. doi: 10.1016/j.ijpsycho.2007.04.001
Knaepen, K., Goekint, M., Heyman, E. M., and Meeusen, R. (2010). Neuroplasticity - exercise-induced response of peripheral brain-derived neurotrophic factor: a systematic review of experimental studies in human subjects. Sports Med. 40, 765–801. doi: 10.2165/11534530-000000000-00000
Koch, I., Poljac, E., Müller, H., and Kiesel, A. (2018). Cognitive structure, flexibility, and plasticity in human multitasking-an integrative review of dual-task and task-switching research. Psychol. Bull. 144, 557–583. doi: 10.1037/bul0000144
Konishi, S., Nakajima, K., Uchida, I., Kameyama, M., Nakahara, K., Sekihara, K., et al. (1998). Transient activation of inferior prefrontal cortex during cognitive set shifting. Nat. Neurosci. 1, 80–84. doi: 10.1038/283
Kujach, S., Olek, R. A., Byun, K., Suwabe, K., Sitek, E. J., Ziemann, E., et al. (2020). Acute sprint interval exercise increases both cognitive functions and peripheral neurotrophic factors in humans: the possible involvement of lactate. Front. Neurosci. 13:1455. doi: 10.3389/fnins.2019.01455
Loprinzi, P. D., and Kane, C. J. (2015). Exercise and cognitive function: a randomized controlled trial examining acute exercise and free-living physical activity and sedentary effects. Mayo Clin. Proc. 90, 450–460. doi: 10.1016/j.mayocp.2014.12.023
Ludyga, S., Gerber, M., Brand, S., Holsboer-Trachsler, E., and Puhse, U. (2016). Acute effects of moderate aerobic exercise on specific aspects of executive function in different age and fitness groups: a meta-analysis. Psychophysiology 53, 1611–1626. doi: 10.1111/psyp.12736
MacDonald, A. W., Cohen, J. D., Andrew Stenger, V., and Carter, C. S. (2000). Dissociating the role of the dorsolateral prefrontal and anterior cingulate cortex in cognitive control. Science (80-.). 288, 1835–1838. doi: 10.1126/science.288.5472.1835
Mathews, D. K., and Fox, E. L. (1976). The Physiological Basis of Physical Education and Athletics. xvi, 577 p. Available online at: file://catalog.hathitrust.org/Record/004423892
McMorris, T. (2016). “Exercise–cognition interaction: State of the art and future research,” in Exercise-Cognition Interaction: Neuroscience Perspectives, eds T. McMorris (San Diego, CA: Elsevier Academic Press), 459–481. Available online at: https://search.ebscohost.com/login.aspx?direct=true&db=psyh&AN=~2016-10849-022&site=ehost-live
McMorris, T., and Hale, B. J. (2012). Differential effects of differing intensities of acute exercise on speed and accuracy of cognition: a meta-analytical investigation. Brain Cogn. 80, 338–351. doi: 10.1016/j.bandc.2012.09.001
McMorris, T., Turner, A., Hale, B. J., and Sproule, J. (2016). “Beyond the catecholamines hypothesis for an acute exercise-cognition interaction: a neurochemical perspective,” in Exercise-Cognition Interaction: Neuroscience Perspectives, T. McMorris (Chichester; London; San Diego, CA; Waltham, MA; Oxford: Elsevier Academic Press; The Boulevard), 65–103. doi: 10.1016/B978-0-12-800778-5.00004-9
Mestre, T. A., Shah, P., Marras, C., Tomlinson, G., and Lang, A. E. (2014). Another face of placebo: the lessebo effect in Parkinson disease. Neurology 82, 1402–1409. doi: 10.1212/WNL.0000000000000340
Meyer, B.-U., Röricht, S., and Niehaus, L. (1998). Morphology of acallosal brains as assessed by MRI in six patients leading a normal daily life. J. Neurol. 245, 106–110. doi: 10.1007/s004150050187
Miyake, A., Friedman, N. P., Emerson, M. J., Witzki, A. H., Howerter, A., and Wager, T. D. (2000). The unity and diversity of executive functions and their contributions to complex “frontal lobe” tasks: a latent variable analysis. Cogn. Psychol. 41, 49–100. doi: 10.1006/cogp.1999.0734
Moher, D, Liberati, A, and Tetzlaff, J. A. D. (2009). Reprint-preferred reporting items for systematic reviews and meta-analyses: the PRISMA statement. Phys. Ther. 89, 873–880. doi: 10.1093/ptj/89.9.873
Moll, J., De Oliveira-Souza, R., Moll, F. T., Bramati, I. E., and Andreiuolo, P. A. (2002). The cerebral correlates of set-shifting: An fMRI study of the trail making test. Arq. Neuropsiquiatr. 60, 900–905. doi: 10.1590/S0004-282X2002000600002
Murray, N. P., and Russoniello, C. (2012). Acute physical activity on cognitive function: a heart rate variability examination. Appl. Psychophysiol. Biofeedback 37, 219–227. doi: 10.1007/s10484-012-9196-z
Naderi, A., Shaabani, F., Esmaeili, A., Salman, Z., Borella, E., and Degens, H. (2018). Effects of low and moderate acute resistance exercise on executive function in community-living older adults. Sport. Exerc. Perform. Psychol. 8, 106–122. doi: 10.1037/spy0000135
Nakazato, M., Tchanturia, K., Schmidt, U., Campbell, I. C., Treasure, J., Collier, D. A., et al. (2009). Brain-derived neurotrophic factor (BDNF) and set-shifting in currently ill and recovered anorexia nervosa (AN) patients. Psychol. Med. 39, 1029–1035. doi: 10.1017/S0033291708004108
Norton, K., Norton, L., and Sadgrove, D. (2010). Position statement on physical activity and exercise intensity terminology. J. Sci. Med. Sport. 13, 496–502. doi: 10.1016/j.jsams.2009.09.008
Oberste, M., Bloch, W., Hübner, S. T., and Zimmer, P. (2016). Do reported effects of acute aerobic exercise on subsequent higher cognitive performances remain if tested against an instructed self-myofascial release training control group? a randomized controlled trial. PLoS ONE 11:e0167818. doi: 10.1371/journal.pone.0167818
Oberste, M., Javelle, F., Sharma, S., Joisten, N., Walzik, D., Bloch, W., et al. (2019). Effects and moderators of acute aerobic exercise on subsequent interference control: a systematic review and meta-analysis. Front. Psychol. 10:2616. doi: 10.3389/fpsyg.2019.02616
O'Leary, K. C., Pontifex, M. B., Scudder, M. R., Brown, M. L., and Hillman, C. H. (2011). The effects of single bouts of aerobic exercise, exergaming, and videogame play on cognitive control. Clin. Neurophysiol. 122, 1518–1525. doi: 10.1016/j.clinph.2011.01.049
Pa, J., Possin, K. L., Wilson, S. M., Quitania, L. C., Kramer, J. H., Boxer, A. L., et al. (2010). Gray matter correlates of set-shifting among neurodegenerative disease, mild cognitive impairment, and healthy older adults. J. Int. Neuropsychol. Soc. 16, 640–650. doi: 10.1017/S1355617710000408.Gray
Pennington, B. F., and Ozonoff, S. (1996). Executive functions and developmental psychopathology. J. Child Psychol. Psychiatry. 37, 51–87. doi: 10.1111/j.1469-7610.1996.tb01380.x
Pereira, A. C., Huddleston, D. E., Brickman, A. M., Sosunov, A. A., Hen, R., McKhann, G. M., et al. (2007). An in vivo correlate of exercise-induced neurogenesis in the adult dentate gyrus. Proc. Natl. Acad. Sci. U.S.A. doi: 10.1073/pnas.0611721104
Pescatello, L. S., Arena, R., Riebe, D., and Thompson, P. D. (2014). ACSM's Guidelines for Exercise Testing and Prescription, 9th Edn. Philadelphia, PA: Wolters Kluwer/Lippincott Williams & Wilkins.
Pettigrew, C., and Martin, R. C. (2014). Cognitive declines in healthy aging: evidence from multiple aspects of interference resolution. Psychol. Aging. 29, 187–204. doi: 10.1037/a0036085
Pontifex, M. B., Hillman, C. H., Fernhall, B., Thompson, K. M., and Valentini, T. A. (2009). The effect of acute aerobic and resistance exercise on working memory. Med. Sci. Sports Exerc. 41, 927–934. doi: 10.1249/MSS.0b013e3181907d69
Pontifex, M. B., McGowan, A. L., Chandler, M. C., Gwizdala, K. L., Parks, A. C., Fenn, K., et al. (2019). A primer on investigating the after effects of acute bouts of physical activity on cognition. Psychol. Sport Exerc. 40, 1–22. doi: 10.1016/j.psychsport.2018.08.015
Ravizza, S. M., and Carter, C. S. (2008). Shifting set about task switching: behavioral and neural evidence for distinct forms of cognitive flexibility. Neuropsychologia 46, 2924–2935. doi: 10.1016/j.neuropsychologia.2008.06.006
Robbins, T. W., and Roberts, A. C. (2007). Differential regulation of fronto-executive function by the monoamines and acetylcholine. Cereb. Cortex 17, 151–160. doi: 10.1093/cercor/bhm066
Rogers, R. D., and Monsell, S. (1995). Costs of a Predictable Switch Between Simple Cognitive Tasks. J. Exp. Psychol. Gen. doi: 10.1037/0096-3445.124.2.207
Rushworth, M. F. S., Passingham, R. E., and Nobre, A. C. (2005). Components of attentional set-switching. Exp. Psychol. 52, 83–98. doi: 10.1027/1618-3169.52.2.83
Sánchez-Cubillo, I., Periáñez, J. A., Adrover-Roig, D., Rodríguez-Sánchez, J. M., Ríos-Lago, M., Tirapu, J., et al. (2009). Construct validity of the Trail Making Test: Role of task-switching, working memory, inhibition/interference control, and visuomotor abilities. J. Int. Neuropsychol. Soc. 15, 438–450. doi: 10.1017/S1355617709090626
Schmolesky, M. T., Webb, D. L., and Hansen, R. A. (2013). The effects of aerobic exercise intensity and duration on levels of brain- derived neurotrophic factor in healthy men. J. Sport. Sci. Med. 12, 502–511.
Schwarck, S., Schmicker, M., Dordevic, M., Rehfeld, K., M?ller, N., and M?ller, P. (2019). Inter-individual differences in cognitive response to a single bout of physical exercise-a randomized controlled cross-over study. J. Clin. Med. 8:1101. doi: 10.3390/jcm8081101
Schwarzer, G., and Carpenter, J. R. (2015). Meta-Analysis with R (Use-R!). Springer Inter- national Publishing, Switzerland. Available online at: http://www.springer.com/gp/book/9783319214153
Senn, T. E., Espy, K. A., and Kaufmann, P. M. (2004). Using path analysis to understand executive function organization in preschool children. Dev. Neuropsychol. 26, 445–464. doi: 10.1207/s15326942dn2601_5
Sibley, B. A., and Beilock, S. L. (2007). Exercise and working memory: an individual differences investigation. J. Sport Exerc. Psychol. 29 783–791. doi: 10.1123/jsep.29.6.783
Slusher, A. L., Patterson, V. T., Schwartz, C. S., and Acevedo, E. O. (2018). Impact of high intensity interval exercise on executive function and brain derived neurotrophic factor in healthy college aged males. Physiol. Behav. 191, 116–122. doi: 10.1016/j.physbeh.2018.04.018
Teasell, R., Bayona, N., Marshall, S., Cullen, N., Bayley, M., Chundamala, J., et al. (2007). A systematic review of the rehabilitation of moderate to severe acquired brain injuries. Brain Inj. 21, 107–112. doi: 10.1080/02699050701201524
Tomporowski, P. D., and Ellis, N. R. (1986). Effects of exercise on cognitive processes: a review. Psychol. Bull. 99, 338–346. doi: 10.1037/0033-2909.99.3.338
Tomporowski, P. D., and Ganio, M. S. (2006). Short-term effects of aerobic exercise on executive processing, memory, and emotional reactivity. Int. J. Sport Exerc. Psychol. 4, 57–72. doi: 10.1080/1612197X.2006.9671784
Tsafnat, G., Glasziou, P., Choong, M. K., Dunn, A., Galgani, F., and Coiera, E. (2014). Systematic review automation technologies. Syst. Rev. 74:2014. doi: 10.1186/2046-4053-3-74
van der Kolk, N. M., Speelman, A. D., van Nimwegen, M., Kessels, R. P. C., IntHout, J., Hakobjan, M., et al. (2015). BDNF polymorphism associates with decline in set shifting in Parkinson's disease. Neurobiol. Aging 36, 1605.e1-1605.e6. doi: 10.1016/j.neurobiolaging.2014.08.023
Verburgh, L., Königs, M., Scherder, E. J. A., and Oosterlaan, J. (2014). Physical exercise and executive functions in preadolescent children, adolescents and young adults: a meta-analysis. Br. J. Sports Med. 48, 973–979. doi: 10.1136/bjsports-2012-091441
Wu, C.-H., Karageorghis, C. I., Wang, C.-C., Chu, C.-H., Kao, S.-C., Hung, T.-M., et al. (2019). Effects of acute aerobic and resistance exercise on executive function: An ERP study. J. Sci. Med. Sport 22, 1367–1372. doi: 10.1016/j.jsams.2019.07.009
Yanagisawa, H., Dan, I., Tsuzuki, D., Kato, M., Okamoto, M., Kyutoku, Y., et al. (2010). Acute moderate exercise elicits increased dorsolateral prefrontal activation and improves cognitive performance with Stroop test. Neuroimage 50, 1702–1710. doi: 10.1016/j.neuroimage.2009.12.023
Keywords: exercise, acute exercise, physical activity, cognition, set shifting, task switching, Trail Making Test and Wisconsin Card Sorting Test
Citation: Oberste M, Sharma S, Bloch W and Zimmer P (2021) Acute Exercise-Induced Set Shifting Benefits in Healthy Adults and Its Moderators: A Systematic Review and Meta-Analysis. Front. Psychol. 12:528352. doi: 10.3389/fpsyg.2021.528352
Received: 20 January 2020; Accepted: 04 January 2021;
Published: 29 January 2021.
Edited by:
Bernadette Ann Murphy, Ontario Tech University, CanadaReviewed by:
Carla Silva-Batista, University of São Paulo, BrazilCopyright © 2021 Oberste, Sharma, Bloch and Zimmer. This is an open-access article distributed under the terms of the Creative Commons Attribution License (CC BY). The use, distribution or reproduction in other forums is permitted, provided the original author(s) and the copyright owner(s) are credited and that the original publication in this journal is cited, in accordance with accepted academic practice. No use, distribution or reproduction is permitted which does not comply with these terms.
*Correspondence: Max Oberste, b2JlcnN0ZW1heEBob3RtYWlsLmNvbQ==
†These authors share first authorship
Disclaimer: All claims expressed in this article are solely those of the authors and do not necessarily represent those of their affiliated organizations, or those of the publisher, the editors and the reviewers. Any product that may be evaluated in this article or claim that may be made by its manufacturer is not guaranteed or endorsed by the publisher.
Research integrity at Frontiers
Learn more about the work of our research integrity team to safeguard the quality of each article we publish.