- UTokyo Center for Integrative Science of Human Behavior (CiSHuB), Center for Evolutionary Cognitive Sciences, Graduate School of Arts and Sciences, The University of Tokyo, Tokyo, Japan
This perspective article discusses the importance of evidence-based psychotherapy and highlights the usefulness of near-infrared spectroscopy (NIRS) in assessing the effects of psychotherapeutic interventions as a future direction of clinical psychology. NIRS is a safe and non-invasive neuroimaging technique that can be implemented in a clinical setting to measure brain activity via a simple procedure. This article discusses the possible benefits and challenges of applying NIRS for this purpose, and the available methodology based on previous studies that used NIRS to evaluate psychotherapeutic effects. Furthermore, this perspective article suggests alternative methodologies that may be useful, namely, the single- and multi-session evaluations using immediate pre- and post-intervention measurements. These methods can be used to evaluate state changes in brain activity, which can be derived from a single session of psychotherapeutic interventions. This article provides a conceptual schema important in actualizing NIRS application for evidence-base psychotherapy.
Introduction
Evidence-Based Psychotherapy
Evidence-based practice in psychology (EBPP), which is defined by the American Psychological Association as “the integration of the best available research with clinical expertise in the context of patient characteristics, culture, and preferences” (American Psychological Association Presidential Task Force on Evidence-Based Practice, 2006), has been receiving increasing attention. EBPP aims to facilitate effective psychological practice based on empirically supported clinical principles. Although it includes a broad range of psychological practices (e.g., assessment, case formulation, psychotherapeutic relationships, and interventions), this article discusses the merits and challenges specifically in the context of an evidence-based approach to psychotherapy.
Throughout history, there has been a long-standing debate regarding the empirical evidence of psychotherapeutic effects (Chambless and Ollendick, 2001; Lilienfeld, 2007; Kazdin, 2008; Gaudiano and Miller, 2013; Emmelkamp et al., 2014; Cook et al., 2017). It could be misleading to depend on subjective interpretations and heuristic decisions with respect to psychotherapeutic events or effects; rather, the importance of scientific perspectives and empirically supported decision-making for psychotherapy implementation has been emphasized. In this regard, research has also provided ample evidence on the efficacy or effectiveness of various psychotherapeutic interventions, although most of these studies have focused on cognitive-behavioral therapy (e.g., Butler et al., 2006; Hofmann S.G. et al., 2012).
Various research methods are currently available to examine psychotherapeutic effects. Among them, self-report measures (e.g., questionnaires and interviews) and behavioral tasks (e.g., cognitive tests) are most frequently used in research and routine clinical practice. These methods are convenient to use and have provided substantial evidence for the effects of various psychotherapeutic modalities, when used properly. Contrarily, neuroimaging is being increasingly used for the assessment of psychotherapeutic effects in general research. Its reliability and validity are not sufficient compared with conventional methods, and its clinical use is often a target of debate. However, since psychiatric symptoms are associated with structural and/or functional abnormalities, and because psychotherapeutic interventions could lead to detectable structural and/or functional changes, neuroimaging has gained attention as another tool for assessing psychotherapeutic effects (Etkin et al., 2005; Roffman et al., 2005; Linden, 2008; Barsaglini et al., 2014). The information obtained from neuroimaging assessments has been expected to elucidate the mechanisms involved in disease pathogenesis, to monitor psychotherapeutic effects, and predict follow-up results (Etkin et al., 2005; Roffman et al., 2005; Barsaglini et al., 2014). Since neuroimaging is a biology-based technique, it evaluates different aspects from those assessed by conventional methods, and is thus expected to provide additional information.
Aims of This Perspective Article
Previous research on psychotherapeutic effect evaluation using neuroimaging indices was mostly based on functional magnetic resonance imaging (fMRI) and positron emission tomography (PET). These neuroimaging techniques have high spatial resolution and are suitable in basic research for evaluating whole brain areas and neural mechanisms. However, their use for evidence-based psychotherapy is still limited partly because the preparation and procedure required to evaluate ongoing psychotherapy effects in a clinical setting may not be easily incorporated. Further, using these methods is expensive, which may impede the obtention of repeated measurements for detailed information of psychotherapeutic effects, although psychotherapeutic sessions often occur several times over the span of weeks or months.
Near-infrared spectroscopy (NIRS), which is a well-known, safe, and non-invasive functional neuroimaging technique, allows for assessment of brain activity using a simple procedure. Some NIRS systems are compact and portable and can be easily installed in a clinical setting. Portable systems usually require only a few minutes for attachment and preparation. Once NIRS systems are acquired, there is typically no additional cost for each measurement, which is useful for repeated measurements. Moreover, NIRS systems are relatively tolerant to body and head movements, allowing participants to comfortably sit on a chair during NIRS measurements. Therefore, the use of NIRS is suitable for a broad range of participants, including children and patients who have difficulty remaining immobile, such as those with attention-deficit hyperactivity disorder (ADHD) (Yasumura et al., 2014).
Given these characteristics, NIRS has been used for on-site measurements in various environments and applications, and researchers have suggested that NIRS systems can be useful tools in clinical psychology (Roffman et al., 2005; Adorni et al., 2016). However, their potential in the field of psychotherapy has not been fully realized yet. Despite psychotherapy being effective in clinical practice, there are many gaps regarding its effects and mechanisms. Through its unique characteristics, NIRS may be useful for providing additional or detailed information concerning these aspects. However, the methodology for examining psychotherapeutic effects using NIRS is not well organized. Therefore, this article discusses some of the possible benefits and challenges of applying this technique in psychotherapy and the available methodologies based on previous studies that have used NIRS to examine psychotherapeutic effects. Further, this perspective article proposes a methodology that could be useful in future research. In this way, the aim of this article was to provide a conceptual schema of methodology that may help facilitate evidence-based psychotherapy and highlight future directions for clinical psychology.
This perspective article initially introduces basic NIRS system characteristics (see section “Basic characteristics of NIRS”) and subsequently focuses on its application (see section “Agendas for NIRS application”). Thereafter, future directions of clinical psychology in terms of the application of NIRS for evidence-based psychotherapy are discussed in the following sections: “Methodology for evaluation of psychotherapeutic effects using NIRS,” “NIRS index for evaluation of psychotherapeutic effects,” and “Considerations for the use of NIRS as a practical clinical instrument.” Furthermore, the limitations and possibilities associated with the use of NIRS for evidence-based psychotherapy are discussed (see section “Limitations and possibilities”). This article conforms to the Declaration of Helsinki and was approved by the University of Tokyo (approval no. 694).
Characteristics of NIRS and Agendas for NIRS Application
Basic Characteristics of NIRS
Near-infrared spectroscopy monitors changes in hemoglobin oxygenation states by employing near-infrared light ranging from approximately 700 to 900 nm. Near-infrared light within this range is transparent when passing through body tissues, but is absorbed by hemoglobin in the blood, which attenuates the light. Cerebral blood flow (CBF) increases upon a regional and transient neural activation, a phenomenon known as neurovascular coupling (Roy and Sherrington, 1890). This leads to an increase in oxygenated hemoglobin (oxyHb) levels and a simultaneous decrease in deoxygenated hemoglobin (deoxyHb) levels (Obrig et al., 1996), which affect the amount of light attenuation. Light attenuation is measured, using emitters and detectors that are attached to the head surface, and converted to changes in concentrations of oxyHb and deoxyHb using the modified Beer-Lambert law (Delpy et al., 1988). The concentration changes correspond to the amount of brain activity in that particular region.
The measurement methods may differ depending on the NIRS system. However, in most systems, light emitters and detectors, both known as optodes, are attached at specific positions on the head surface based on internationally standardized methods for electroencephalography electrode positioning (e.g., the 10–20 system) (Jasper, 1958). The optodes are usually fixed 3 cm apart from each other using a holder or cap (Figure 1). Changes in oxyHb and deoxyHb concentrations are measured at each channel located in the middle of an emitter and detector, approximately 2 cm below the scalp. The number and locations of measurable channels differ depending on the NIRS system. Various types of NIRS systems, including portable systems and systems equipped with one or several channels, are available.
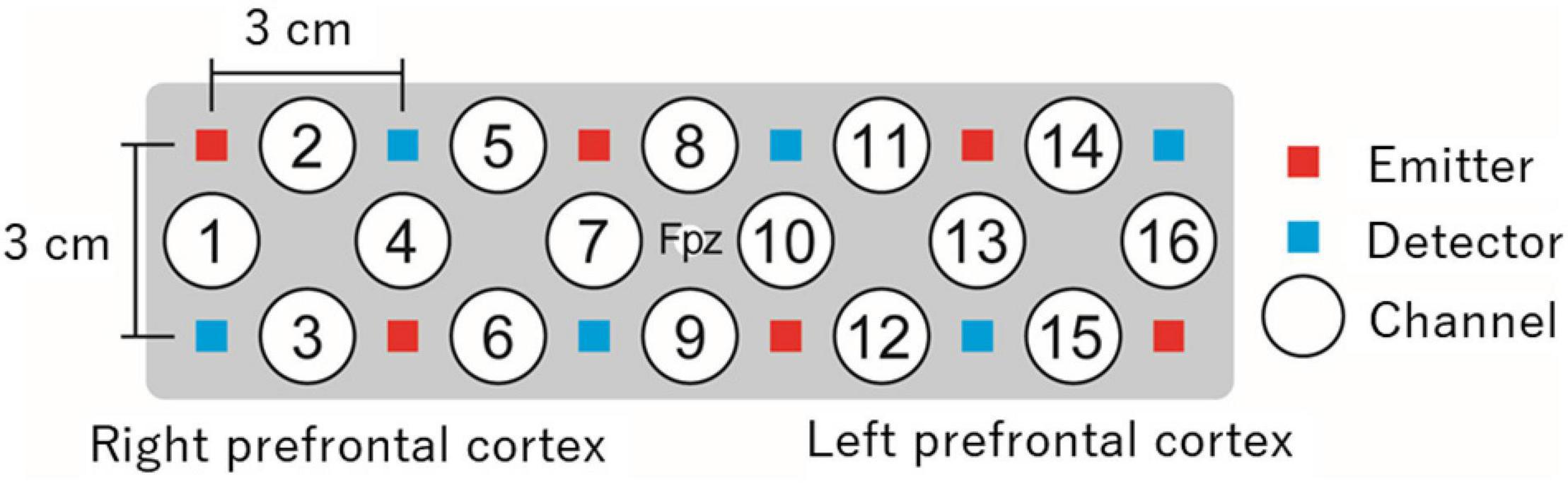
Figure 1. An example of prefrontal measurement with 16-channel NIRS. In this example, a holder is placed on the head surface such that its center matches the Fpz location in the international 10–20 system. Changes in oxyHb and deoxyHb concentrations are measured from approximately 2 cm below the scalp by 16 independent channels. NIRS, near-infrared spectroscopy; oxyHb, oxygenated hemoglobin; deoxyHb, deoxygenated hemoglobin.
Agendas for NIRS Application
To use NIRS for the evaluation of psychotherapeutic effects, several considerations must be taken into account; while certain challenges may emerge during actualization and will need to be addressed. In this section, some of these considerations are discussed.
Because near-infrared light reaches a maximum depth of approximately 2 cm below the head surface, the areas of the brain that can be studied with NIRS are limited. NIRS is usually only applicable for surface cortical regions, although variations in cortical thickness may generate discrepancies depending on the brain region and individuals (Okamoto et al., 2004). This limitation restricts the types of research questions that can be investigated using NIRS. Nevertheless, the measurable areas cover the prefrontal cortex, which engages in essential higher cognitive functions (Smith and Jonides, 1999), such as executive functions that include updating (ability to monitor information and update working memory representations), inhibition (ability to inhibit prominent responses), and shifting (ability to shift mental sets) (Miyake et al., 2000; Alvarez and Emory, 2006; Friedman et al., 2008; Minzenberg et al., 2009; Best and Miller, 2010; Hofmann W. et al., 2012). These functions are critical for understanding the dysfunctions associated with psychiatric disorders, as will be discussed later. Moreover, because a particular region of the brain is associated with multiple functions, different brain activities can be observed in the same area using an experimental control. For example, the dorsolateral prefrontal cortex (DLPFC) engages in executive functions, as well as participates in decision making (Krain et al., 2006) and social cognitions such as theory of mind (Kalbe et al., 2010). Thus, despite the area limitation, NIRS can be used to assess various important functions using an appropriate experimental control.
The fact that a particular brain region involves multiple functions indicates that it may be challenging to distinguish brain functions based on regional CBF (rCBF) changes using NIRS alone. For example, the medial prefrontal cortex (MPFC) regions were found to be involved in both regulation of emotion (brain activity for emotion regulation, such as appraisal and suppression; Diekhof et al., 2011; Nelson et al., 2015) and responses to emotional material (brain activity for responses to emotional stimulation; Phan et al., 2002; Ochsner et al., 2009). Therefore, rCBF changes in the MPFC could be interpreted due to either function. Nevertheless, underlying functions related to rCBF changes need to be identified without information from deep brain regions (e.g., the limbic system, which constitutes the center of emotional processing), using NIRS alone (Ozawa et al., 2014, 2019; Ozawa and Hiraki, 2017). To address these concerns, adequate basic research is required before the application of NIRS in clinical practice. Complementary use of other techniques, such as fMRI (Kirilina et al., 2012; Sato et al., 2013; Haeussinger et al., 2014) and physiological measurements (Balconi et al., 2015; Pinti et al., 2015), can be beneficial.
Finally, NIRS signals are easily affected by the activity of peripheral physiological processes controlled by the autonomic nervous system (e.g., heart rate, respiration, and blood pressure) (Kirilina et al., 2012; Haeussinger et al., 2014) that involve the continuous contraction and relaxation of innervated blood vessels (Söderstrom et al., 2003). In particular, task-evoked physiological changes in extracerebral blood vessels, such as changes in skin blood flow, cause artifacts in NIRS signals (Takahashi et al., 2011; Kirilina et al., 2012). For example, artifacts are frequently observed during tasks that affect respiration (e.g., verbal fluency tasks) (Takahashi et al., 2011), emotional processing (e.g., picture presentation) (Minati et al., 2009), and body movement (e.g., arm raising) (Minati et al., 2011). These risks may be reduced by contriving experimental designs. Performing tasks during baseline instead of resting has been suggested to examine intended differences by reducing possible confounding factors (Minagawa et al., 2017). The risks may be further reduced by controlling factors that cause artifacts. For example, performing adequate preliminary practices or creating a relaxed resting state at the beginning (using deep breathing or attentional control, for example) may promote stabilization of arousal. Body movement during tasks can be reduced using body supports such as a chin or arm rest. Furthermore, various analytical methods have been developed to reduce physiological artifacts (Scholkmann et al., 2014; Tak and Ye, 2014), including independent component analysis (Kohno et al., 2007), spatial filtering (Zhang et al., 2016), and the hemodynamic modality separation method (Yamada et al., 2012). For the hemodynamic modality separation method, a free data analysis package is currently available (The National Institute of Advanced Industrial Science and Technology, 2019).
Future Directions in Clinical Psychology
Methodology for Evaluation of Psychotherapeutic Effects Using NIRS
There are several possible methods for evaluating psychotherapeutic effects using NIRS systems (Tables 1, 2). One is to measure brain activity during a psychotherapeutic intervention or a similar condition. This allows for detection of the neural mechanisms underlying the intervention through real-time monitoring during the intervention. This method is particularly suitable with NIRS and has been used in various forms of psychotherapy (Tables 1, 2), such as during mindfulness meditation (Gundel et al., 2018; Rosenbaum et al., 2020a), interpersonal brain synchronization between the counselor and client (Zhang et al., 2018, 2020), attention training with metacognitive therapy (Rosenbaum et al., 2018), exposure to stimuli for specific phobia (Landowska et al., 2018; Rosenbaum et al., 2020b), role lettering in writing therapy (Okamoto et al., 2010), and trauma-related recall with eye movement desensitization (Ohtani et al., 2009; Amano and Toichi, 2016). Nevertheless, this method restricts body movement during the intervention, which could disrupt performance, depending on the type of psychotherapy. Hence, this method has limited applicability for psychotherapeutic effect evaluation.
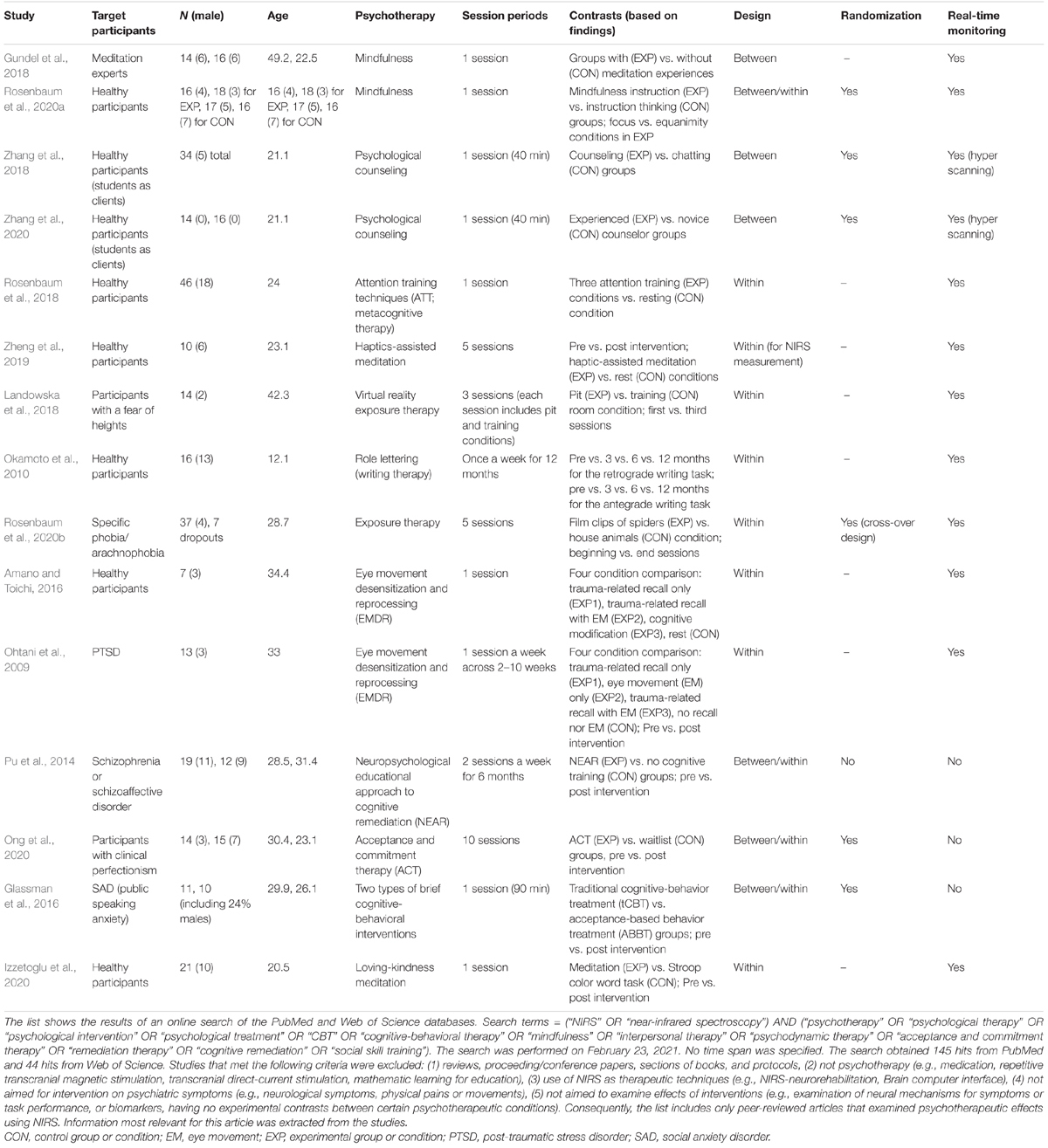
Table 1. Experimental designs of studies that evaluated psychotherapeutic effects using hemodynamic response changes.
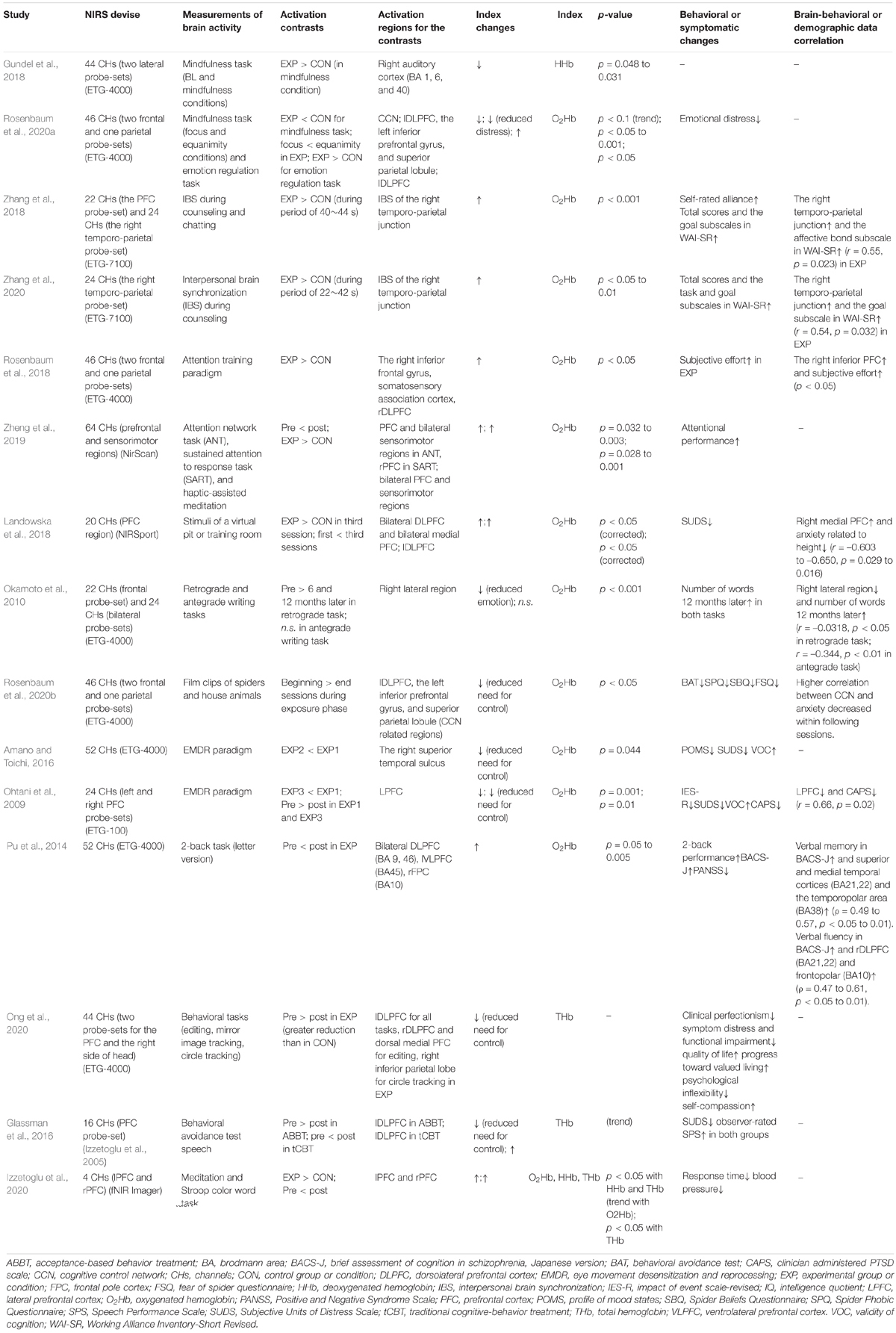
Table 2. Brain and behavioral results of studies that evaluated psychotherapeutic effects using hemodynamic response changes.
Second, psychotherapeutic effects can be assessed by comparing pre-intervention rCBF changes with post-intervention rCBF changes during task implementation, thereby detecting changes in brain function over a series of sessions. This method has typically been used for the examination of empirical evidence of psychotherapeutic effects, often employing structurally established experimental designs (including randomized controlled trials). For neuroimaging modalities, fMRI (Haut et al., 2010; Bor et al., 2011; Yoshimura et al., 2014) is frequently used; however, it is also used with NIRS (Tables 1, 2). For example, the effects of the neuropsychological educational approach to cognitive remediation were assessed by pre- and post-comparison of the oxyHb changes during 2-back tasks (Pu et al., 2014). Similarly, the effects of haptic-assisted meditation were evaluated using the attention network and sustained attention to response tasks (Zheng et al., 2019); further, the effects of acceptance and commitment therapy were assessed using behavioral tasks including editing, mirror image tracking, and circle tracking (Ong et al., 2020).
Third, pre- and post-intervention measurements of rCBF changes could be interposed in a single session (Figure 2). Although the previous method measures trait changes derived from a series of sessions, this method measures state changes derived from a single session. In comparison with pre- and post-intervention measurements over a series of sessions, performing measurements in one session would have the following benefits:
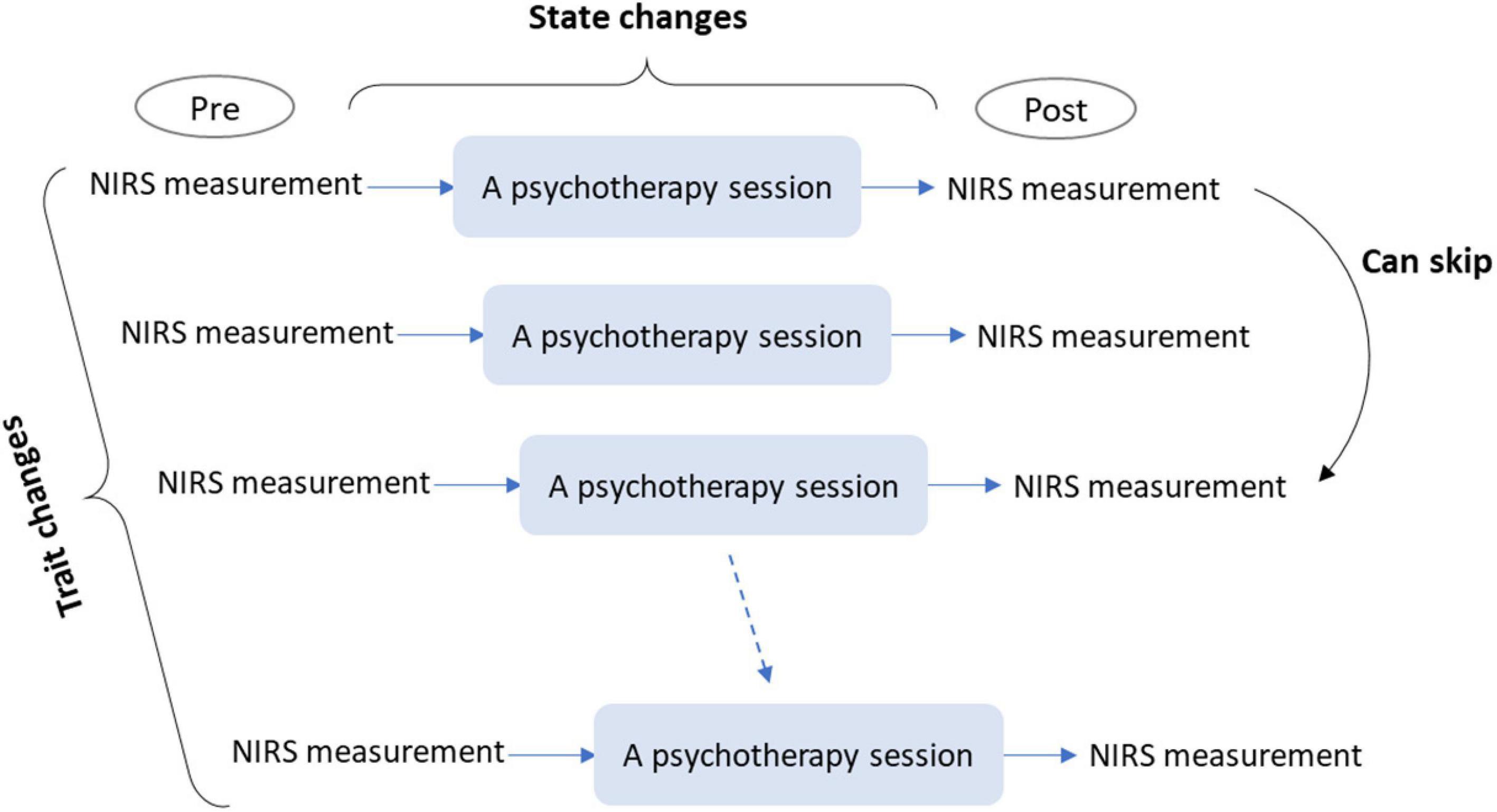
Figure 2. A conceptual schema for single- and multi-session evaluations of psychotherapeutic effects using NIRS. Single-session evaluation can be conducted by immediate pre- and post-intervention measurements of rCBF changes. This evaluation allows for the measurement of state changes in brain activity induced by a single session. Multi-session evaluation can be conducted by repetitive single-session evaluations, which allows for the examination of trait changes in brain activity induced by a psychotherapy program. NIRS, near-infrared spectroscopy; rCBF, regional cerebral blood flow.
• Physical and mental conditions of patients, which constantly vary, are less likely to influence the obtained signals.
• Immediate measurement will facilitate the capture of subtle changes induced by a single session.
• Determination of the effectiveness of a single session is possible.
Although this method has rarely been used, previous studies have utilized it to evaluate the effects of brief cognitive-behavioral interventions for public speaking anxiety (Glassman et al., 2016) and loving-kindness meditation in healthy participants (Izzetoglu et al., 2020). This method demands immediate and repetitive measurements, preferably performed on-site; therefore, it would be particularly suitable with NIRS. The present article suggests that the method is useful in the application of NIRS for evaluating psychotherapeutic effects.
Finally, pre- and post-intervention measurements in a single session can be extended to multi-sessions (Figure 2). This requires repetitive measurements over a series of sessions and would be feasible only with NIRS. It can facilitate the investigation of changes in effects over a series of sessions (e.g., whether initial or latter sessions were more effective). Since most psychotherapeutic approaches involve multiple sessions, this investigation would be beneficial for most approaches.
For single- and multi-session evaluations of psychotherapeutic effects using NIRS, immediate measurement is important since it measures state changes in brain activity, which is suitable with NIRS. To perform immediate measurements in a clinical setting, an easy and simple process for NIRS measurement is needed; the NIRS system should be attached quickly to the patient or client’s head. Furthermore, simple and short tasks are required; implementation of the task should not disrupt the psychotherapy session or become a burden for the patient or client.
NIRS Index for Evaluation of Psychotherapeutic Effects
The NIRS index, which can be used to assess psychotherapeutic effects, should be carefully examined in future studies. Setting a certain norm which can be applied for broad psychiatric disorders or symptoms may be challenging since each disorder or symptom may have unique characteristics. However, the present article suggests the most likely candidates for NIRS indices which can be used to evaluate psychotherapeutic effects since certain tendencies have already been indicated in previous studies. The most likely candidate for a NIRS index would be the concentration changes of oxyHb in the lateral prefrontal cortex (LPFC) region, including the DLPFC, during executive tasks. LPFC activity is known to increase with the employment of executive functions, such as working memory, inhibition, and emotional control. Table 3 lists some previous findings regarding prefrontal activities during executive tasks in patients with psychiatric disorders, in contrast to healthy participants.
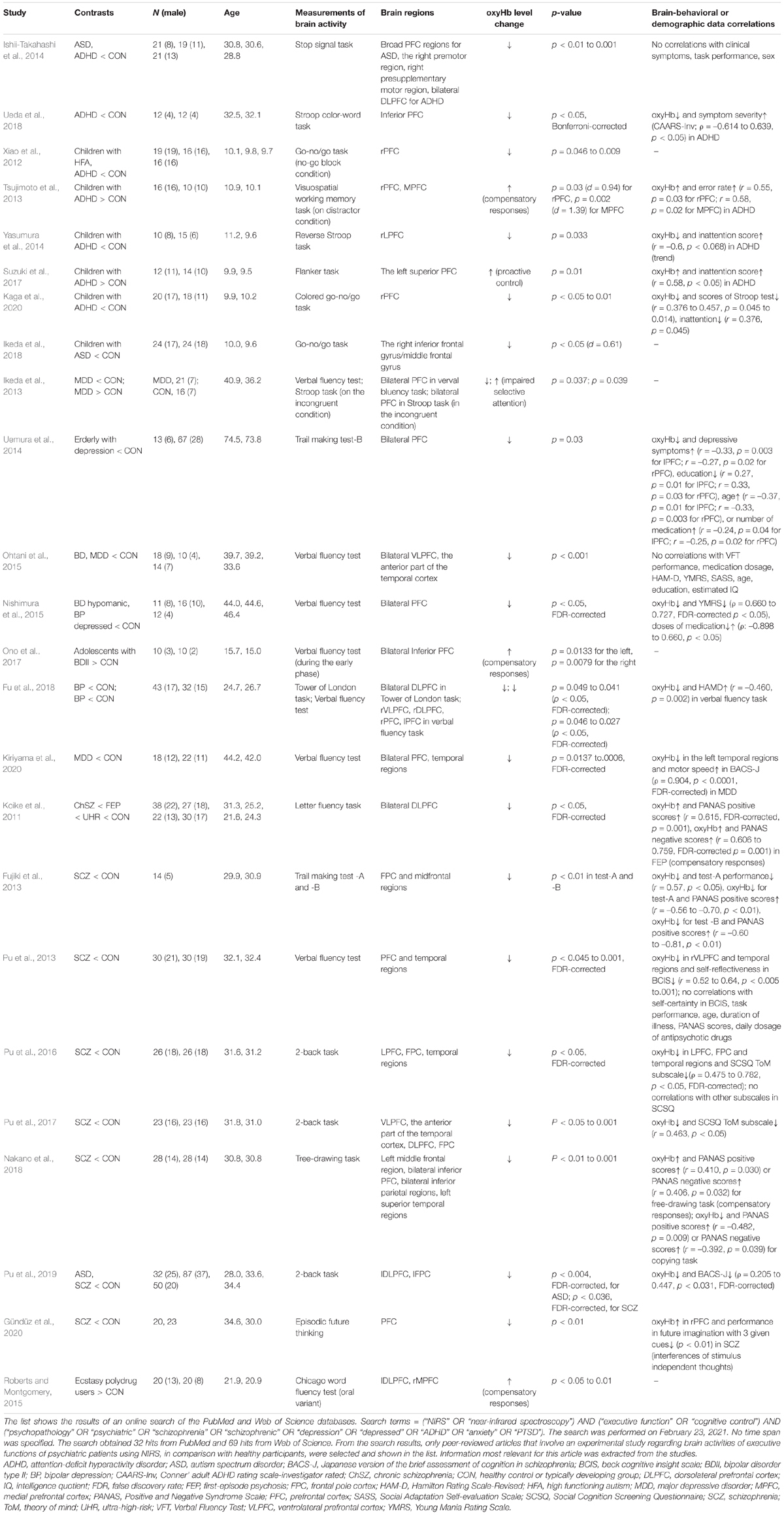
Table 3. OxyHb levels changes during executive tasks in psychiatric patients in comparison with healthy controls.
Patients with various psychiatric disorders typically have executive dysfunction and frequently demonstrate hypoactivation of the LPFC relative to healthy controls during executive tasks, such as the n-back task, go/no-go task, trail making test, and Stroop task. This has been observed using NIRS (Table 3) in addition to fMRI or PET studies [meta-analysis of schizophrenia (Minzenberg et al., 2009), major depressive disorder (MDD) (Snyder, 2013), and various participants (McTeague et al., 2017)]. In such cases, activation of the LPFC region serves as an index for successful psychotherapy. Cognitive remediation therapy has been found to increase DLPFC activity during n-back tasks in patients with schizophrenia, as assessed using NIRS (Pu et al., 2014) and fMRI (Haut et al., 2010).
In contrast, hyperactivation of the LPFC during executive tasks may be observed in some psychiatric patients. Firstly, patients with an anxiety disorder or post-traumatic disorder (PTSD) have been found to show hyperactivation of the LPFC when fearful or under stress (Ohtani et al., 2009; Rosenbaum et al., 2020b; Tables 1, 2). It has been suggested that this is because these individuals must exert significant effort to inhibit excessive emotion. In such cases, deactivation of the LPFC region is an index for improvement (Ohtani et al., 2009; Okamoto et al., 2010; Amano and Toichi, 2016; Glassman et al., 2016; Ong et al., 2020; Rosenbaum et al., 2020a,b; Table 2). Implementation of exposure therapy reduced the LPFC activity while watching videos of spiders in patients with arachnophobia (Rosenbaum et al., 2020b; Tables 1, 2). Similarly, eye movement desensitization and reprocessing have been found to reduce LPFC activity during the recall of traumatic memories (Ohtani et al., 2009; Tables 1, 2). Secondly, patients with ADHD may sometimes show increased LPFC activity during demanding inhibitory tasks such as Stroop tasks (Tsujimoto et al., 2013; Suzuki et al., 2017; Table 3). It has been suggested that this may occur because of a compensatory mechanism; when a task is demanding with high difficulty, increased efforts are required, leading to excessive brain activity (Tsujimoto et al., 2013). The compensatory mechanism has been also indicated in patients with schizophrenia (Koike et al., 2011; Nakano et al., 2018), bipolar disorder (Ono et al., 2017), and ecstasy polydrug users (Roberts and Montgomery, 2015) when tasks are demanding or symptoms are severe. Nevertheless, it has been suggested that this phenomenon can be distinguished by the lack of associated behavioral differences (Roberts and Montgomery, 2015). Finally, increased PFC activity may also be observed due to excessive cognitive processing caused by impaired selective attention or dysfunction of the default mode network (Ikeda et al., 2013). Patients with MDD showed increased bilateral PFC activity during Stroop tasks (Ikeda et al., 2013). However, activation attributed to this factor is more likely associated with the MPFC rather than with the LPFC considering that the MPFC is a core region of the default mode network (e.g., Gusnard and Raichle, 2001; Gusnard et al., 2001).
With regard to task selection, typical executive tasks that are robust and relatively consistent would be appropriate for clinical assessment. Habituation (e.g., Thompson, 2009), in such forms as a response decrement in brain activity as reported in fMRI studies (e.g., Breiter et al., 1996; Wright et al., 2001), can be caused by repetitive implementation of tasks, especially for multi-session interventions. Considering the basic properties of habituation (Thompson, 2009), this may be reduced by the following efforts. Task difficulty, which can be initially adjusted for each individual, should not be too easy, considering that a weaker stimulus is likely to cause habituation. The frequency of measurements can be decreased by omitting them at some sessions. Alternatively, it is also possible that tasks that differ from the assessment task are administered as dummy tasks to avoid monotony caused by repetition of a single type of task, considering that presentation of another stimulus may prevent or provide recovery from habituation to target stimuli. Moreover, use of a control task (e.g., 1-back task for n-back) for baseline data instead of rest may eliminate confounding (habituation) effects that can be caused by sensory adaptation (e.g., visual and tactile sensations) given that differences in rCBF changes between control and experimental tasks may computationally exclude the confounding effects due to sensory adaptation.
Finally, although these NIRS indexes can be used for the evaluation of psychotherapeutic effects in general research, it is preferable that they are used in addition to existing methods. The validity of NIRS indexes need to be examined using multiple lines of indexes from existing methods. Since these indexes assess different aspects, they may show inconsistency. For example, changes in brain activity can be detected without changes in self-report measures and vice versa. However, a holistic evaluation would provide information that can more thoroughly examine the results.
Considerations for the Use of NIRS as a Practical Clinical Instrument
Near-infrared spectroscopy indexes can be used for the evaluation of psychotherapeutic effects within research settings; however, they are not yet applicable for routine clinical practice at the present stage. The applicability or usefulness of biomarkers (such as neuroimaging) may differ between the clinical research framework and core clinical framework (Albert et al., 2011), and translating them to routine clinical practices would still be premature (Dubois et al., 2014; Jack et al., 2018; Canevelli et al., 2019).
The above discussion indicates that methods with high levels of correlation between the brain and behavior with respect to psychiatric diagnoses (e.g., depression) are currently insufficient, and their relationship remains to be well characterized. Moreover, conventional methods, such as self-report and clinical data (e.g., behavioral data), are more strongly correlated with clinical markers (e.g., relapse, life-satisfaction, mortality, and suicide). Although there is neuroimaging evidence on psychotherapeutic effects, these results were obtained in a research setting rather than in routine clinical practice.
In order to translate the NIRS method to routine clinical practice, there needs to be adequate research results showing that NIRS is not harmful, and that it is reliable, valid, and beneficial to existing methods. Moreover, significant and important decisions in actual clinical practice and research settings (e.g., treatment choices) need to be based on adequate evidence from existing methods. In the field of psychiatric disorders, these aforementioned criteria remain unmet, and the validity of NIRS methods has not been adequately researched.
Nevertheless, studies using NIRS to investigate psychotherapeutic effects has started to increase in recent years, as shown in this systematic search (Tables 1, 2). Most of these studies have found psychotherapeutic effects based on changes in hemodynamic responses. Furthermore, the results of the systematic search show that the signs of improvement, indicated by the NIRS index, are relatively consistent with data shown with the behavioral or symptomatic changes (Table 2). Statistically significant correlations between the NIRS index and the behavioral or demographic data have also been frequently found, as shown in Tables 2, 3. Although these findings were obtained within a research framework, they indicate that the reliability and validity of NIRS to evaluate psychotherapeutic effects have been increasing recently. Although this field is still in an early developmental stage, it has great potential to grow in the future.
Limitations and Possibilities
First, the pre- and post-intervention measurements interposed in a single session capture state changes in brain activity rather than trait changes. Hence, observed changes may be transient. It is unclear whether repeated state changes induced by multi-session implementations could lead to the trait changes that are usually considered as the outcome of psychotherapy. Measurements in multi-sessions should be able to address this question. Second, multi-session evaluations may be affected by changes in the patient’s or client’s mental and physical conditions. For example, naturalistic negative mood was found to correlate with lower activation in the DLPFC region during verbal working memory tasks in healthy participants (Aoki et al., 2011; Sato et al., 2014). However, the relationship between mental and physical conditions and rCBF changes in a single session can be examined by employing other assessment methods (e.g., self-report and interview). Third, NIRS application can also be beneficial in fields other than clinical psychology, including psychiatry and neurology. The effects of treatment methods, such as the repetitive transcranial magnetic stimulation and the transcranial direct-current stimulation, can be evaluated using methodologies similar with the ones discussed in this article. The use of NIRS as brain-machine interface also has high potential as an intervention tool for certain neurological (e.g., locked-in syndrome; Hong et al., 2018) and psychiatric symptoms (e.g., ADHD symptoms with NIRS-neurorehabilitation; Hudak et al., 2017; Kimmig et al., 2019). Fourth, due to the limited number of used search words and databases in the systematic search, Tables 1, 2 include only limited number of available studies which examined psychotherapeutic effects using NIRS; however, they provided information that helped the organization of the primary methodologies. Fifth, NIRS application can be useful for various psychotherapies, including ones not found in the current search results. Particularly, single- and multi-session evaluations using NIRS may be beneficial when other methodologies are less likely to be undertaken. For example, few methodologies may be available for some psychotherapeutic interventions, such as play therapy, sand therapy, and art-related therapy, compared to other psychotherapeutic approaches, such as cognitive behavioral therapy. Further, compared to adults without disorders, the available methodologies for children and some patients with difficulties in grasping their mental and physical conditions are limited. These issues are related to methodological availability. Single- and multi-session evaluations using NIRS provide another opportunity for such cases and may show brain-based evidence. Finally, this article provides a conceptual schema for the evaluation of psychotherapeutic effects using NIRS. Therefore, the suggested methodology and the NIRS index remain only a possibility. More detailed examination based on each psychiatric disorder and type of psychotherapy is warranted in future studies to approach actualization of evidence-based psychotherapy.
Conclusion
This article highlights the possibility of using NIRS for evidence-based psychotherapy as a future direction in clinical psychology, it discussed some of the benefits and challenges of applying this technique for this purpose, and organized the available methodology based on a review of primary studies that have used NIRS to examine psychotherapeutic effects. Furthermore, this perspective article proposed that single- and multi-session evaluations with NIRS as alternative methodologies for the evaluation of psychotherapeutic effects. Since these methods capture state changes in brain activity, immediate measurements are important. Changes in oxyHb levels of the LPFC region during typical executive tasks were suggested as potential NIRS indexes. Although more detailed examination is needed in future studies, single- and multi-session evaluations using NIRS may provide additional information which can be derived from psychotherapeutic interventions.
Author Contributions
SO provided all perspectives and prepared the manuscript.
Funding
This study was supported by grants from the UTokyo Center for Integrative Science of Human Behavior (CiSHuB).
Conflict of Interest
The author declares that the research was conducted in the absence of any commercial or financial relationships that could be construed as a potential conflict of interest.
Publisher’s Note
All claims expressed in this article are solely those of the authors and do not necessarily represent those of their affiliated organizations, or those of the publisher, the editors and the reviewers. Any product that may be evaluated in this article, or claim that may be made by its manufacturer, is not guaranteed or endorsed by the publisher.
Acknowledgments
I am deeply grateful to Dr. Toshikazu Hasegawa for the invaluable support and contributions provided in the conception of this article. I also would like to thank the continuous support received by the UTokyo Center for Integrative Science of Human Behavior (CiSHuB) and University of Tokyo Institute for Diversity & Adaptation of Human Mind (UTIDAHM).
References
Adorni, R., Gatti, A., Brugnera, A., Sakatani, K., and Compare, A. (2016). Could fNIRS promote neuroscience approach in clinical psychology? Front. Psychol. 7:456. doi: 10.3389/fpsyg.2016.00456
Albert, M. S., DeKosky, S. T., Dickson, D., Dubois, B., Feldman, H. H., Fox, N. C., et al. (2011). The diagnosis of mild cognitive impairment due to Alzheimer’s disease: recommendations from the National Institute on Aging-Alzheimer’s Association workgroups on diagnostic guidelines for Alzheimer’s disease. Alzheimers Dement. 7, 270–279. doi: 10.1016/j.jalz.2011.03.008
Alvarez, J. A., and Emory, E. (2006). Executive function and the frontal lobes: a meta-analytic review. Neuropsychol. Rev. 16, 17–42. doi: 10.1007/s11065-006-9002-x
Amano, T., and Toichi, M. (2016). Possible neural mechanisms of psychotherapy for trauma-related symptoms: cerebral responses to the neuropsychological treatment of post-traumatic stress disorder model individuals. Sci. Rep. 6:34610. doi: 10.1038/srep34610
American Psychological Association Presidential Task Force on Evidence-Based Practice (2006). Evidence-based practice in psychology. Am. Psychol. 61, 271–285. doi: 10.1037/0003-066X.61.4.271
Aoki, R., Sato, H., Katura, T., Utsugi, K., Koizumi, H., Matsuda, R., et al. (2011). Relationship of negative mood with prefrontal cortex activity during working memory tasks: an optical topography study. Neurosci. Res. 70, 189–196. doi: 10.1016/j.neures.2011.02.011
Balconi, M., Grippa, E., and Vanutelli, M. E. (2015). What hemodynamic (fNIRS), electrophysiological (EEG) and autonomic integrated measures can tell us about emotional processing. Brain Cogn. 95, 67–76. doi: 10.1016/j.bandc.2015.02.001
Barsaglini, A., Sartori, G., Benetti, S., Pettersson-Yeo, W., and Mechelli, A. (2014). The effects of psychotherapy on brain function: a systematic and critical review. Prog. Neurobiol. 114, 1–14. doi: 10.1016/j.pneurobio.2013.10.006
Best, J. R., and Miller, P. H. (2010). A developmental perspective on executive function. Child Dev. 81, 1641–1660. doi: 10.1111/j.1467-8624.2010.01499.x
Bor, J., Brunelin, J., d’Amato, T., Costes, N., Suaud-Chagny, M. F., Saoud, M., et al. (2011). How can cognitive remediation therapy modulate brain activations in schizophrenia? An fMRI study. Psychiatry Res. Neuroimaging 192, 160–166. doi: 10.1016/j.pscychresns.2010.12.004
Breiter, H. C., Etcoff, N. L., Whalen, P. J., Kennedy, W. A., Rauch, S. L., Buckner, R. L., et al. (1996). Response and habituation of the human amygdala during visual processing of facial expression. Neuron 17, 875–887. doi: 10.1016/s0896-6273(00)80219-6
Butler, A. C., Chapman, J. E., Forman, E. M., and Beck, A. T. (2006). The empirical status of cognitive-behavioral therapy: a review of meta-analyses. Clin. Psychol. Rev. 26, 17–31. doi: 10.1016/j.cpr.2005.07.003
Canevelli, M., Bacigalupo, I., Gervasi, G., Lacorte, E., Massari, M., Mayer, F., et al. (2019). Methodological issues in the clinical validation of biomarkers for Alzheimer’s disease: the paradigmatic example of CSF. Front. Aging Neurosci. 11:282. doi: 10.3389/Fnagi.2019.00282
Chambless, D. L., and Ollendick, T. H. (2001). Empirically supported psychological interventions: controversies and evidence. Ann. Rev. Psychol. 52, 685–716. doi: 10.1146/annurev.psych.52.1.685
Cook, S. C., Schwartz, A. C., and Kaslow, N. J. (2017). Evidence-based psychotherapy: advantages and challenges. Neurotherapeutics. 14, 537–545. doi: 10.1007/s13311-017-0549-4
Delpy, D. T., Cope, M., Vanderzee, P., Arridge, S., Wray, S., and Wyatt, J. (1988). Estimation of optical pathlength through tissue from direct time of flight measurement. Phys. Med. Biol. 33, 1433–1442. doi: 10.1088/0031-9155/33/12/008
Diekhof, E. K., Geier, K., Falkai, P., and Gruber, O. (2011). Fear is only as deep as the mind allows: a coordinate-based meta-analysis of neuroimaging studies on the regulation of negative affect. Neuroimage. 58, 275–285. doi: 10.1016/j.neuroimage.2011.05.073
Dubois, B., Feldman, H. H., Jacova, C., Hampel, H., Molinuevo, J. L., Blennow, K., et al. (2014). Advancing research diagnostic criteria for Alzheimer’s disease: the IWG-2 criteria. Lancet Neurol. 13, 614–629. doi: 10.1016/S1474-4422(14)70090-0
Emmelkamp, P. M. G., David, D., Beckers, T., Muris, P., Cuijpers, P., Lutz, W., et al. (2014). Advancing psychotherapy and evidence-based psychological interventions. Int. J. Methods Psychiatr. Res. 23, 58–91. doi: 10.1002/mpr.1411
Etkin, A., Phil, M., Pittenger, C., Polan, H. J., and Kandel, E. R. (2005). Toward a neurobiology of psychotherapy: basic science and clinical applications. J. Neuropsychiatry Clin. Neurosci. 17, 145–158. doi: 10.1176/appi.neuropsych.17.2.145
Friedman, N. P., Miyake, A., Young, S. E., DeFries, J. C., Corley, R. P., and Hewitt, J. K. (2008). Individual differences in executive functions are almost entirely genetic in origin. J. Exp. Psychol. Gen. 137, 201–225. doi: 10.1037/0096-3445.137.2.201
Fu, L., Xiang, D., Xiao, J., Yao, L., Wang, Y., Xiao, L., et al. (2018). Reduced prefrontal activation during the tower of London and verbal fluency task in patients with bipolar depression: a multi-channel NIRS study. Front. Psychiatry. 9:214. doi: 10.3389/fpsyt.2018.00214
Fujiki, R., Morita, K., Sato, M., Kamada, Y., Kato, Y., Inoue, M., et al. (2013). Reduced prefrontal cortex activation using the trail making test in schizophrenia. Neuropsychiatr. Dis. Treat. 9, 675–685. doi: 10.2147/ndt.s43137
Gaudiano, B. A., and Miller, I. W. (2013). The evidence-based practice of psychotherapy: facing the challenges that lie ahead. Clin. Psychol. Rev. 33, 813–824. doi: 10.1016/j.cpr.2013.04.004
Glassman, L. H., Forman, E. M., Herbert, J. D., Bradley, L. E., Foster, E. E., Izzetoglu, M., et al. (2016). The effects of a brief acceptance-based behavioral treatment versus traditional cognitive-behavioral treatment for public speaking anxiety: an exploratory trial examining differential effects on performance and neurophysiology. Behav. Modif. 40, 748–776. doi: 10.1177/0145445516629939
Gundel, F., von Spee, J., Schneider, S., Haeussinger, F. B., Hautzinger, M., Erb, M., et al. (2018). Meditation and the brain–Neuronal correlates of mindfulness as assessed with near-infrared spectroscopy. Psychiatry Res. Neuroimaging 271, 24–33. doi: 10.1016/j.pscychresns.2017.04.002
Gündüz, H., Baran, Z., Kir, Y., Sedes Baskak, N., and Baskak, B. (2020). Investigation of the cortical activity during episodic future thinking in schizophrenia: a functional near-infrared spectroscopy study. Behav. Neurosci. 134, 344–357. doi: 10.1037/bne0000377
Gusnard, D. A., Akbudak, E., Shulman, G. L., and Raichle, M. E. (2001). Medial prefrontal cortex and self-referential mental activity: relation to a default mode of brain function. Proc. Natl. Acad. Sci. U.S.A. 98, 4259–4264. doi: 10.1073/pnas.071043098
Gusnard, D. A., and Raichle, M. E. (2001). Searching for a baseline: functional imaging and the resting human brain. Nat. Rev. Neurosci. 2, 685–694. doi: 10.1038/35094500
Haeussinger, F. B., Dresler, T., Heinzel, S., Schecklmann, M., Fallgatter, A. J., and Ehlis, A. C. (2014). Reconstructing functional near-infrared spectroscopy (fNIRS) signals impaired by extra-cranial confounds: an easy-to-use filter method. Neuroimage 95, 69–79. doi: 10.1016/j.neuroimage.2014.02.035
Haut, K. M., Lim, K. O., and MacDonald, A. (2010). Prefrontal cortical changes following cognitive training in patients with chronic schizophrenia: effects of practice, generalization, and specificity. Neuropsychopharmacology 35, 1850–1859. doi: 10.1038/npp.2010.52
Hofmann, S. G., Asnaani, A., Vonk, I. J., Sawyer, A. T., and Fang, A. (2012). The efficacy of cognitive behavioral therapy: a review of meta-analyses. Cognit. Ther. Res. 36, 427–440. doi: 10.1007/s10608-012-9476-1
Hofmann, W., Schmeichel, B. J., and Baddeley, A. D. (2012). Executive functions and self-regulation. Trends Cogn. Sci. 16, 174–180. doi: 10.1016/j.tics.2012.01.006
Hong, K. S., Khan, M. J., and Hong, M. J. (2018). Feature extraction and classification methods for hybrid fNIRS-EEG brain-computer interfaces. Front. Hum. Neurosci. 12:246. doi: 10.3389/fnhum.2018.00246
Hudak, J., Blume, F., Dresler, T., Haeussinger, F. B., Renner, T. J., Fallgatter, A. J., et al. (2017). Near-infrared spectroscopy-based frontal lobe neurofeedback integrated in virtual reality modulates brain and behavior in highly impulsive adults. Front. Hum. Neurosci. 11:425. doi: 10.3389/fnhum.2017.00425
Ikeda, E., Shiozaki, K., Ikeda, H., Suzuki, M., and Hirayasu, Y. (2013). Prefrontal dysfunction in remitted depression at work reinstatement using near-infrared spectroscopy. Psychiatry Res. 214, 254–259. doi: 10.1016/j.pscychresns.2013.07.009
Ikeda, T., Tokuda, T., Monden, Y., Hirai, M., Mizushima, S. G., Nagashima, M., et al. (2018). Hypoactivation of the right prefrontal cortex underlying motor-related inhibitory deficits in children with autism spectrum disorder: a functional near-infrared spectroscopy study. Jpn. Psychol. Res. 60, 251–264. doi: 10.1111/jpr.12204
Ishii-Takahashi, A., Takizawa, R., Nishimura, Y., Kawakubo, Y., Kuwabara, H., Matsubayashi, J., et al. (2014). Prefrontal activation during inhibitory control measured by near-infrared spectroscopy for differentiating between autism spectrum disorders and attention deficit hyperactivity disorder in adults. Neuroimage Clin. 4, 53–63. doi: 10.1016/j.nicl.2013.10.002
Izzetoglu, M., Izzetoglu, K., Bunce, S., Ayaz, H., Devaraj, A., Onaral, B., et al. (2005). Functional near-infrared neuroimaging. IEEE Trans. Neural Syst. Rehabil. Eng. 13, 153–159. doi: 10.1109/TNSRE.2005.847377
Izzetoglu, M., Shewokis, P. A., Tsai, K., Dantoin, P., Sparango, K., and Min, K. (2020). Short-term effects of meditation on sustained attention as measured by fNIRS. Brain Sci. 10:608. doi: 10.3390/brainsci10090608
Jack, C. R., Bennett, D. A., Blennow, K., Carrillo, M. C., Dunn, B., Haeberlein, S. B., et al. (2018). NIA-AA research framework: toward a biological definition of Alzheimer’s disease. Alzheimers Dement. 14, 535–562. doi: 10.1016/j.jalz.2018.02.018
Jasper, H. H. (1958). Report of the committee on methods of clinical examination in electroencephalography: 1957. Electroencephalogr. Clin. Neurophysiol. 10, 370–375. doi: 10.1016/0013-4694(58)90053-1
Kaga, Y., Ueda, R., Tanaka, M., Kita, Y., Suzuki, K., Okumura, Y., et al. (2020). Executive dysfunction in medication-naive children with ADHD: a multi-modal fNIRS and EEG study. Brain Dev. 42, 555–563. doi: 10.1016/j.braindev.2020.05.007
Kalbe, E., Schlegel, M., Sack, A. T., Nowak, D. A., Dafotakis, M., Bangard, C., et al. (2010). Dissociating cognitive from affective theory of mind: a TMS study. Cortex 46, 769–780. doi: 10.1016/j.cortex.2009.07.010
Kazdin, A. E. (2008). Evidence-based treatment and practice: new opportunities to bridge clinical research and practice, enhance the knowledge base, and improve patient care. Am. Psychol. 63, 146–159. doi: 10.1037/0003-066X.63.3.146
Kimmig, A. S., Dresler, T., Hudak, J., Haeussinger, F. B., Wildgruber, D., Fallgatter, A. J., et al. (2019). Feasibility of NIRS-based neurofeedback training in social anxiety disorder: behavioral and neural correlates. J. Neural Transm. (Vienna) 126, 1175–1185. doi: 10.1007/s00702-018-1954-5
Kirilina, E., Jelzow, A., Heine, A., Niessing, M., Wabnitz, H., Brühl, R., et al. (2012). The physiological origin of task-evoked systemic artefacts in functional near infrared spectroscopy. Neuroimage 61, 70–81. doi: 10.1016/j.neuroimage.2012.02.074
Kiriyama, T., Tanemura, R., Nakamura, Y., Takemoto, C., Hashimoto, M., and Utsumi, H. (2020). Reduced temporal activation during a verbal fluency task is associated with poor motor speed in patients with major depressive disorder. Psychiatry Investig. 17, 804–813. doi: 10.30773/pi.2020.0045
Kohno, S., Miyai, I., Seiyama, A., Oda, I., Ishikawa, A., Tsuneishi, S., et al. (2007). Removal of the skin blood flow artifact in functional near-infrared spectroscopic imaging data through independent component analysis. J. Biomed. Opt. 12:062111. doi: 10.1117/1.2814249
Koike, S., Takizawa, R., Nishimura, Y., Takano, Y., Takayanagi, Y., Kinou, M., et al. (2011). Different hemodynamic response patterns in the prefrontal cortical sub-regions according to the clinical stages of psychosis. Schizophr. Res. 132, 54–61. doi: 10.1016/j.schres.2011.07.014
Krain, A. L., Wilson, A. M., Arbuckle, R., Castellanos, F. X., and Milham, M. P. (2006). Distinct neural mechanisms of risk and ambiguity: a meta-analysis of decision-making. Neuroimage 32, 477–484. doi: 10.1016/j.neuroimage.2006.02.047
Landowska, A., Roberts, D., Eachus, P., and Barrett, A. (2018). Within- and between-session prefrontal cortex response to virtual reality exposure therapy for acrophobia. Front. Hum. Neurosci. 12:362. doi: 10.3389/fnhum.2018.00362
Lilienfeld, S. O. (2007). Psychological treatments that cause harm. Perspect. Psychol. Sci. 2, 53–70. doi: 10.1111/j.1745-6916.2007.00029.x
Linden, D. E. (2008). Brain imaging and psychotherapy: methodological considerations and practical implications. Eur. Arch. Psychiatry Clin. Neurosci. 258, 71–75. doi: 10.1007/s00406-008-5023-1
McTeague, L. M., Huemer, J., Carreon, D. M., Jiang, Y., Eickhoff, S. B., and Etkin, A. (2017). Identification of common neural circuit disruptions in cognitive control across psychiatric disorders. Am. J. Psychiatry. 174, 676–685. doi: 10.1176/appi.ajp.2017.16040400
Minagawa, Y., Hakuno, Y., Kobayashi, A., Naoi, N., and Kojima, S. (2017). Infant word segmentation recruits the cerebral network of phonological short-term memory. Brain Lang. 170, 39–49. doi: 10.1016/j.bandl.2017.03.005
Minati, L., Jones, C. L., Gray, M. A., Medford, N., Harrison, N. A., and Critchley, H. D. (2009). Emotional modulation of visual cortex activity: a functional near-infrared spectroscopy study. Neuroreport 20, 1344–1350. doi: 10.1097/WNR.0b013e328330c751
Minati, L., Kress, I. U., Visani, E., Medford, N., and Critchley, H. D. (2011). Intra- and extra-cranial effects of transient blood pressure changes on brain near-infrared spectroscopy (NIRS) measurements. J. Neurosci. Methods 197, 283–288. doi: 10.1016/j.jneumeth.2011.02.029
Minzenberg, M. J., Laird, A. R., Thelen, S., Carter, C. S., and Glahn, D. C. (2009). Meta-analysis of 41 functional neuroimaging studies of executive function in schizophrenia. Arch. Gen. Psychiatry. 66, 811–822. doi: 10.1001/archgenpsychiatry.2009.91
Miyake, A., Friedman, N. P., Emerson, M. J., Witzki, A. H., Howerter, A., and Wager, T. D. (2000). The unity and diversity of executive functions and their contributions to complex “frontal lobe” tasks: a latent variable analysis. Cogn. Psychol. 41, 49–100. doi: 10.1006/cogp.1999.0734
Nakano, S., Shoji, Y., Morita, K., Igimi, H., Sato, M., Ishii, Y., et al. (2018). Comparison of changes in oxygenated hemoglobin during the tree-drawing task between patients with schizophrenia and healthy controls. Neuropsychiatr Dis. Treat. 14, 1071–1082. doi: 10.2147/NDT.S159984
Nelson, B. D., Fitzgerald, D. A., Klumpp, H., Shankman, S. A., and Phan, K. L. (2015). Prefrontal engagement by cognitive reappraisal of negative faces. Behav. Brain Res. 279, 218–225. doi: 10.1016/j.bbr.2014.11.034
Nishimura, Y., Takahashi, K., Ohtani, T., Ikeda-Sugita, R., Kasai, K., and Okazaki, Y. (2015). Dorsolateral prefrontal hemodynamic responses during a verbal fluency task in hypomanic bipolar disorder. Bipolar Disord. 17, 172–183. doi: 10.1111/bdi.12252
Obrig, H., Heekeren, H., Ruben, J., Wenzel, R., Ndayisaba, J. P., Dirnagl, U., et al. (1996). Continuous spectrum near-infrared spectroscopy approach in functional activation studies in the human adult. Opt. Biopsies Microsc. Tech. 2926, 58–66. doi: 10.1117/12.260820
Ochsner, K. N., Ray, R. R., Hughes, B., McRae, K., Cooper, J. C., and Weber, J. (2009). Bottom-Up and top-down processes in emotion generation: common and distinct neural mechanisms. Psychol. Sci. 20, 1322–1331. doi: 10.1111/j.1467-9280.2009.02459.x
Ohtani, T., Matsuo, K., Kasai, K., Kato, T., and Kato, N. (2009). Hemodynamic responses of eye movement desensitization and reprocessing in posttraumatic stress disorder. Neurosci. Res. 65, 375–383. doi: 10.1016/j.neures.2009.08.014
Ohtani, T., Nishimura, Y., Takahashi, K., Ikeda-Sugita, R., Okada, N., and Okazaki, Y. (2015). Association between longitudinal changes in prefrontal hemodynamic responses and social adaptation in patients with bipolar disorder and major depressive disorder. J. Affect. Disord. 176, 78–86. doi: 10.1016/j.jad.2015.01.042
Okamoto, M., Dan, H., Sakamoto, K., Takeo, K., Shimizu, K., Kohno, S., et al. (2004). Three-dimensional probabilistic anatomical cranio-cerebral correlation via the international 10–20 system oriented for transcranial functional brain mapping. Neuroimage 21, 99–111. doi: 10.1016/j.neuroimage.2003.08.026
Okamoto, Y., Morita, K., Yamamoto, A., Ishii, Y., Haruguchi, N., and Uchimura, N. (2010). Changes in task-associated cerebral blood induced by role lettering: measurement by multichannel near-infrared spectroscopy. Kurume Med. J. 57, 51–58. doi: 10.2739/kurumemedj.57.51
Ong, C. W., Hancock, A. S., Barrett, T. S., Lee, E. B., Wan, N., Gillam, R. B., et al. (2020). A preliminary investigation of the effect of acceptance and commitment therapy on neural activation in clinical perfectionism. J. Contextual Behav. Sci. 18, 152–161. doi: 10.1016/j.jcbs.2020.09.007
Ono, Y., Kikuchi, M., Nakatani, H., Murakami, M., Nishisaka, M., Muramatsu, T., et al. (2017). Prefrontal oxygenation during verbal fluency and cognitive function in adolescents with bipolar disorder type II. Asian J. Psychiatr. 25, 147–153. doi: 10.1016/j.ajp.2016.11.001
Ozawa, S., and Hiraki, K. (2017). Distraction decreases prefrontal oxygenation: a NIRS study. Brain Cogn. 113, 155–163. doi: 10.1016/j.bandc.2017.02.003
Ozawa, S., Kanayama, N., and Hiraki, K. (2019). Emotion-related cerebral blood flow changes in the ventral medial prefrontal cortex: an NIRS study. Brain Cogn. 114, 21–28. doi: 10.1016/j.bandc.2019.05.001
Ozawa, S., Matsuda, G., and Hiraki, K. (2014). Negative emotion modulates prefrontal cortex activity during a working memory task: a NIRS study. Front. Hum. Neurosci. 8:46. doi: 10.3389/Fnhum.2014.00046
Phan, K. L., Wager, T., Taylor, S. F., and Liberzon, I. (2002). Functional neuroanatomy of emotion: a meta-analysis of emotion activation studies in PET and fMRI. Neuroimage 16, 331–348. doi: 10.1006/nimg.2002.1087
Pinti, P., Cardone, D., and Merla, A. (2015). Simultaneous fNIRS and thermal infrared imaging during cognitive task reveal autonomic correlates of prefrontal cortex activity. Sci. Rep. 5:17471. doi: 10.1038/srep17471
Pu, S., Nakagome, K., Itakura, M., Iwata, M., Nagata, I., and Kaneko, K. (2017). Association of fronto-temporal function with cognitive ability in schizophrenia. Sci. Rep. 7:42858. doi: 10.1038/srep42858
Pu, S., Nakagome, K., Satake, T., Ohtachi, H., Itakura, M., Yamanashi, T., et al. (2019). Comparison of prefrontal hemodynamic responses and cognitive deficits between adult patients with autism spectrum disorder and schizophrenia. Schizophr. Res. 206, 420–427. doi: 10.1016/j.schres.2018.10.007
Pu, S., Nakagome, K., Yamada, T., Ikezawa, S., Itakura, M., Satake, T., et al. (2014). A pilot study on the effects of cognitive remediation on hemodynamic responses in the prefrontal cortices of patients with schizophrenia: a multi-channel near-infrared spectroscopy study. Schizophr. Res. 153, 87–95. doi: 10.1016/j.schres.2014.01.031
Pu, S., Nakagome, K., Yamada, T., Itakura, M., Satake, T., Ishida, H., et al. (2013). Association between cognitive insight and prefrontal function during a cognitive task in schizophrenia: a multichannel near-infrared spectroscopy study. Schizophr. Res. 150, 81–87. doi: 10.1016/j.schres.2013.07.048
Pu, S., Nakagome, K., Yamada, T., Itakura, M., Yamanashi, T., Yamada, S., et al. (2016). Social cognition and prefrontal hemodynamic responses during a working memory task in schizophrenia. Sci. Rep. 6:22500. doi: 10.1038/Srep22500
Roberts, C. A., and Montgomery, C. (2015). fNIRS suggests increased effort during executive access in ecstasy polydrug users. Psychopharmacology (Berl.). 232, 1571–1582. doi: 10.1007/s00213-014-3795-8
Roffman, J. L., Marci, C. D., Glick, D. M., Dougherty, D. D., and Rauch, S. L. (2005). Neuroimaging and the functional neuroanatomy of psychotherapy. Psychol. Med. 35, 1385–1398. doi: 10.1017/S0033291705005064
Rosenbaum, D., Kroczek, A. M., Hudak, J., Rubel, J., Maier, M. J., Sorg, T., et al. (2020a). Neural correlates of mindful emotion regulation in high and low ruminators. Sci. Rep. 10:15617. doi: 10.1038/s41598-020-71952-5
Rosenbaum, D., Leehr, E. J., Rubel, J., Maier, M. J., Pagliaro, V., Deutsch, K., et al. (2020b). Cortical oxygenation during exposure therapy–In situ fNIRS measurements in arachnophobia. Neuroimage Clin. 26:102219. doi: 10.1016/j.nicl.2020.102219
Rosenbaum, D., Maier, M. J., Hudak, J., Metzger, F. G., Wells, A., Fallgatter, A. J., et al. (2018). Neurophysiological correlates of the attention training technique: a component study. Neuroimage Clin. 19, 1018–1024. doi: 10.1016/j.nicl.2018.06.021
Roy, C. S., and Sherrington, C. S. (1890). On the regulation of the blood-supply of the brain. J. Physiol. 1, 85–108. doi: 10.1113/jphysiol.1890.sp000321
Sato, H., Dresler, T., Haeussinger, F. B., Fallgatter, A. J., and Ehlis, A. C. (2014). Replication of the correlation between natural mood states and working memory-related prefrontal activity measured by near-infrared spectroscopy in a German sample. Front. Hum. Neurosci. 8:37. doi: 10.3389/fnhum.2014.00037
Sato, H., Yahata, N., Funane, T., Takizawa, R., Katura, T., Atsumori, H., et al. (2013). A NIRS-fMRI investigation of prefrontal cortex activity during a working memory task. Neuroimage 83, 158–173. doi: 10.1016/j.neuroimage.2013.06.043
Scholkmann, F., Kleiser, S., Metz, A. J., Zimmermann, R., Pavia, J. M., Wolf, U., et al. (2014). A review on continuous wave functional near-infrared spectroscopy and imaging instrumentation and methodology. Neuroimage 85, 6–27. doi: 10.1016/j.neuroimage.2013.05.004
Smith, E. E., and Jonides, J. (1999). Neuroscience–storage and executive processes in the frontal lobes. Science 283, 1657–1661. doi: 10.1126/science.283.5408.1657
Snyder, H. R. (2013). Major depressive disorder is associated with broad impairments on neuropsychological measures of executive function: a meta-analysis and review. Psychol. Bull. 139, 81–132. doi: 10.1037/a0028727
Söderstrom, T., Stefanovska, A., Veber, M., and Svensson, H. (2003). Involvement of sympathetic nerve activity in skin blood flow oscillations in humans. Am. J. Physiol. Heart Circ. Physiol. 284, H1638–H1646. doi: 10.1152/ajpheart.00826.2000
Suzuki, K., Okumura, Y., Kita, Y., Oi, Y., Yamashita, Y., Goto, T., et al. (2017). Excessive hemodynamic activity in the superior frontal cortex during the flanker task in children with attention deficit hyperactivity disorder. Neuroreport 28, 828–832. doi: 10.1097/wnr.0000000000000834
Tak, S., and Ye, J. C. (2014). Statistical analysis of fNIRS data: a comprehensive review. Neuroimage 85, 72–91. doi: 10.1016/j.neuroimage.2013.06.016
Takahashi, T., Takikawa, Y., Kawagoe, R., Shibuya, S., Iwano, T., and Kitazawa, S. (2011). Influence of skin blood flow on near-infrared spectroscopy signals measured on the forehead during a verbal fluency task. Neuroimage 57, 991–1002. doi: 10.1016/j.neuroimage.2011.05.012
The National Institute of Advanced Industrial Science and Technology (2019). Human Informatics Research Institute Neurorehabilitation Research Group Software Download page. Available online at: https://unit.aist.go.jp/hiri/nrrg/english/download/index.html (Accessed July 26, 2019).
Thompson, R. F. (2009). Habituation: a history. Neurobiol. Learn. Mem. 92, 127–134. doi: 10.1016/j.nlm.2008.07.011
Tsujimoto, S., Yasumura, A., Yamashita, Y., Torii, M., Kaga, M., and Inagaki, M. (2013). Increased prefrontal oxygenation related to distractor-resistant working memory in children with attention-deficit/hyperactivity disorder (ADHD). Child Psychiatry Hum. Dev. 44, 678–688. doi: 10.1007/s10578-013-0361-2
Ueda, S., Ota, T., Iida, J., Yamamuro, K., Yoshino, H., Kishimoto, N., et al. (2018). Reduced prefrontal hemodynamic response in adult attention-deficit hyperactivity disorder as measured by near-infrared spectroscopy. Psychiatry Clin. Neurosci. 72, 380–390. doi: 10.1111/pcn.12643
Uemura, K., Shimada, H., Doi, T., Makizako, H., Park, H., and Suzuki, T. (2014). Depressive symptoms in older adults are associated with decreased cerebral oxygenation of the prefrontal cortex during a trail-making test. Arch. Gerontol. Geriatr. 59, 422–428. doi: 10.1016/j.archger.2014.07.003
Wright, C. I., Fischer, H., Whalen, P. J., McInerney, S. C., Shin, L. M., and Rauch, S. L. (2001). Differential prefrontal cortex and amygdala habituation to repeatedly presented emotional stimuli. Neuroreport 12, 379–383. doi: 10.1097/00001756-200102120-00039
Xiao, T., Xiao, Z., Ke, X., Hong, S., Yang, H., Su, Y., et al. (2012). Response inhibition impairment in high functioning autism and attention deficit hyperactivity disorder: evidence from near-infrared spectroscopy data. PLoS One. 7:e46569. doi: 10.1371/journal.pone.0046569
Yamada, T., Umeyama, S., and Matsuda, K. (2012). Separation of fNIRS signals into functional and systemic components based on differences in hemodynamic modalities. PLoS One. 7:e50271. doi: 10.1371/journal.pone.0050271
Yasumura, A., Kokubo, N., Yamamoto, H., Yasumura, Y., Nakagawa, E., Kaga, M., et al. (2014). Neurobehavioral and hemodynamic evaluation of Stroop and reverse Stroop interference in children with attention-deficit/hyperactivity disorder. Brain Dev. 36, 97–106. doi: 10.1016/j.braindev.2013.01.005
Yoshimura, S., Okamoto, Y., Onoda, K., Matsunaga, M., Okada, G., Kunisato, Y., et al. (2014). Cognitive behavioral therapy for depression changes medial prefrontal and ventral anterior cingulate cortex activity associated with self-referential processing. Soc. Cogn. Affect. Neurosci. 9, 487–493. doi: 10.1093/scan/nst009
Zhang, X., Noah, J. A., and Hirsch, J. (2016). Separation of the global and local components in functional near-infrared spectroscopy signals using principal component spatial filtering. Neurophotonics 3:1. doi: 10.1117/1.Nph.3.1.015004
Zhang, Y., Meng, T., Hou, Y., Pan, Y., and Hu, Y. (2018). Interpersonal brain synchronization associated with working alliance during psychological counseling. Psychiatry Res. Neuroimaging 282, 103–109. doi: 10.1016/j.pscychresns.2018.09.007
Zhang, Y., Meng, T., Yang, Y., and Hu, Y. (2020). Experience-dependent counselor-client brain synchronization during psychological counseling. eNeuro 7, 1–10. doi: 10.1523/ENEURO.0236-20.2020
Keywords: near-infrared spectroscopy, evidence-based practice, psychotherapy, assessment, clinical psychology, neuroimaging
Citation: Ozawa S (2021) Application of Near-Infrared Spectroscopy for Evidence-Based Psychotherapy. Front. Psychol. 12:527335. doi: 10.3389/fpsyg.2021.527335
Received: 16 January 2020; Accepted: 23 June 2021;
Published: 23 July 2021.
Edited by:
Chia-Wei Sun, National Chiao Tung University, TaiwanReviewed by:
Zhen Yuan, University of Macau, ChinaF. Gonzalez-Lima, University of Texas at Austin, United States
Copyright © 2021 Ozawa. This is an open-access article distributed under the terms of the Creative Commons Attribution License (CC BY). The use, distribution or reproduction in other forums is permitted, provided the original author(s) and the copyright owner(s) are credited and that the original publication in this journal is cited, in accordance with accepted academic practice. No use, distribution or reproduction is permitted which does not comply with these terms.
*Correspondence: Sachiyo Ozawa, c2FjaGl5by4zOTMzNjkuYUBnbWFpbC5jb20=