- 1Vanderbilt Memory & Alzheimer’s Center, Department of Neurology, Vanderbilt University Medical Center, Nashville, TN, United States
- 2Vanderbilt Brain Institute, Vanderbilt University, Nashville, TN, United States
- 3Vanderbilt University School of Medicine, Vanderbilt University, Nashville, TN, United states
- 4Department of Biostatistics, Vanderbilt University Medical Center, Nashville, TN, United States
- 5Division of Cardiovascular Medicine, Department of Medicine, Vanderbilt University Medical Center, Nashville, TN, United States
- 6Vanderbilt Heart Imaging Core Lab, Vanderbilt Translational and Clinical Cardiovascular Research Center, Vanderbilt University Medical Center, Nashville, TN, United States
- 7Department of Neurology, Vanderbilt University Medical Center, Nashville, TN, United States
- 8Vanderbilt Genetics Institute, Vanderbilt University Medical Center, Nashville, TN, United States
Background: Subclinical reductions in cardiac output correspond to lower cerebral blood flow (CBF), placing the brain at risk for functional changes.
Objectives: This study aims to establish the consequences of reduced cardiac output on longitudinal cognitive outcomes in aging adults.
Methods: Vanderbilt Memory and Aging Project participants free of clinical dementia and heart failure (n = 306, 73 ± 7, 58% male) underwent baseline echocardiography to assess cardiac output (L/min) and longitudinal neuropsychological assessment at baseline, 18 months, 3 and 5 years. Linear mixed-effects regressions related cardiac output to trajectory for each longitudinal neuropsychological outcome, adjusting for age, sex, race/ethnicity, education, body surface area, Framingham Stroke Risk Profile score, apolipoprotein E (APOE) ε4 status and follow-up time. Models were repeated, testing interactions with cognitive diagnosis and APOE-ε4 status.
Results: Lower baseline cardiac output related to faster declines in language (β = 0.11, p = 0.01), information processing speed (β = 0.31, p = 0.006), visuospatial skills (β = 0.09, p = 0.03), and episodic memory (β = 0.02, p = 0.001). No cardiac output x cognitive diagnosis interactions were observed (p > 0.26). APOE-ε4 status modified the association between cardiac output and longitudinal episodic memory (β = 0.03, p = 0.047) and information processing speed outcomes (β = 0.55, p = 0.02) with associations stronger in APOE-ε4 carriers.
Conclusion: The present study provides evidence that even subtle reductions in cardiac output may be associated with more adverse longitudinal cognitive health, including worse language, information processing speed, visuospatial skills, and episodic memory performances. Preservation of healthy cardiac functioning is important for maintaining optimal brain aging among older adults.
Introduction
Altered cardiac hemodynamics have been associated with cerebral blood flow (CBF) disturbances (Jefferson et al., 2017), smaller brain volumes (Sabayan et al., 2015), and poorer cognitive performances (Sabayan et al., 2015; Kresge et al., 2018), particularly among APOE-ε4 carriers (Bown et al., 2019). Prior studies, however, have been cross-sectional (Sabayan et al., 2015; Jefferson et al., 2017; Kresge et al., 2018; Bown et al., 2019) with limited investigation into the connection between subclinical cardiac dysfunction and longitudinal consequences, especially cognitive trajectories among aging adults. Given cross-sectional evidence, associations between cardiac function and longitudinal cognition may exist.
Reduced cardiac output is associated with reduced CBF (Jefferson et al., 2017) which may result in damaging cascades in the brain due to inadequate nutrient delivery and oxidative stress. The medial temporal lobes (Chen et al., 2011; Roy et al., 2017) as well as the superior frontal and orbito-frontal gyri (Chen et al., 2011; Roy et al., 2017) are susceptible to reductions in blood flow with aging (Chen et al., 2011) and heart failure (Roy et al., 2017), and the hippocampus, a structure of the medial temporal lobes, is vulnerable to blood brain barrier breakdown with aging (Montagne et al., 2015). Of course, in addition to regional susceptibility to reduced blood supply, certain regions demand more energy which creates additional vulnerabilities (Hawkins et al., 1979; Payabvash et al., 2011). Cognitive functions specific to regions of the brain that are particularly susceptible to blood flow reductions may be compromised in the context of hemodynamic dysregulation. The medial temporal lobes and frontal-subcortical networks, which are regions sensitive to hypoperfusion, are responsible for episodic memory (Tulving and Markowitsch, 1998), executive function (Cummings, 1998), and information processing (Cummings, 1998) and therefore these functions are vulnerable to subtle but chronic hemodynamic dysregulation over time.
This study aimed to characterize the association between subclinical cardiac dysfunction and cognitive trajectory in aging adults. Specifically, we examined whether baseline cardiac output relates to longitudinal cognitive performance among older adults free of clinical dementia, stroke, or heart failure at study entry. Based on prior work (Jefferson et al., 2010; Sabayan et al., 2015; Kresge et al., 2018), we hypothesized lower baseline cardiac output would relate to worse cognitive trajectories over the follow-up period, especially episodic memory as assessed by the Biber Figure Learning Test (Glosser et al., 2002) and California Verbal Learning Test-II (Delis et al., 2000), information processing speed as assessed by the Delis Kaplan Executive Function System (DKEFS) Number Sequencing Test (Delis et al., 2001) and Wechsler Adult Intelligence Scale 4th Edition (WAIS-IV) Digit Symbol Test (Wechsler, 2008), and executive function as assessed by the DKEFS Number-letter Switching (Delis et al., 2001), DKEFS Tower Test (Delis et al., 2001), DKEFS Color-Word Inhibition (Delis et al., 2001), and Letter Fluency Test (Benton et al., 1994). We investigated whether associations would be modified by the apolipoprotein E (APOE) ε4 allele or cognitive diagnosis. While APOE-ε4 is a genetic susceptibility marker for AD (Martins et al., 2005) it is also a molecular moderator of vascular damage (Halliday et al., 2016). Thus, we hypothesized that APOE-ε4 carriers would have stronger associations between baseline cardiac output and cognitive decline compared to non-carriers. We also hypothesized that participants with mild cognitive impairment (MCI), a clinical prodrome of dementia, would have stronger associations compared to participants with normal cognition due to extensive neuropathology underlying cognitive symptoms. The presumed neuropathology of MCI participants disrupts normal physiology and creates vulnerability to hemodynamic dysfunction.
Materials and Methods
Study Cohort
The Vanderbilt Memory and Aging Project (VMAP) is a longitudinal study investigating vascular health and aging. Inclusion required participants to be age 60 years or older, speak English, have intact auditory and visual acuity, and have a study partner. Exclusion criteria included MRI contraindication, history of neurological disease (e.g., stroke, epilepsy), prevalent heart failure, major psychiatric illness, head injury with loss of consciousness greater than 5 min and systemic or terminal illness (e.g., cancer) that could adversely affect follow-up participation.
At eligibility, participants completed a medical history and record review, Clinical Dementia Rating interview, and neuropsychological assessment. Participants were enrolled if they had normal cognition (NC) or met diagnostic criteria for MCI which requires all of the following criteria be fulfilled: (a) a cognitive concern by a participant, informant, or clinician, (b) impairment in at least one cognitive domain, (c) independence in functional abilities, and (d) no dementia (Albert et al., 2011). At enrollment, each participant completed a comprehensive evaluation, including fasting blood draw, physical examination, clinical interview, medication review, neuropsychological assessment, echocardiogram, and cardiac magnetic resonance. Identical procedures were repeated at each time point, including 18 months, 3 and 5 years, for longitudinal follow-up of the cohort. Participants were excluded from the current study for missing baseline echocardiogram, baseline covariate data, or neuropsychological data across all timepoints. See Figure 1 for participant selection details. Three hundred and six participants included in the study were seen at baseline and 278 had more than one timepoint of data for a mean follow-up period of 3.5 ± 1.5 years. Two hundred and seventy participants were seen at 18-months, 256 participants at 3-year, and 133 participants at 5-year follow-ups.
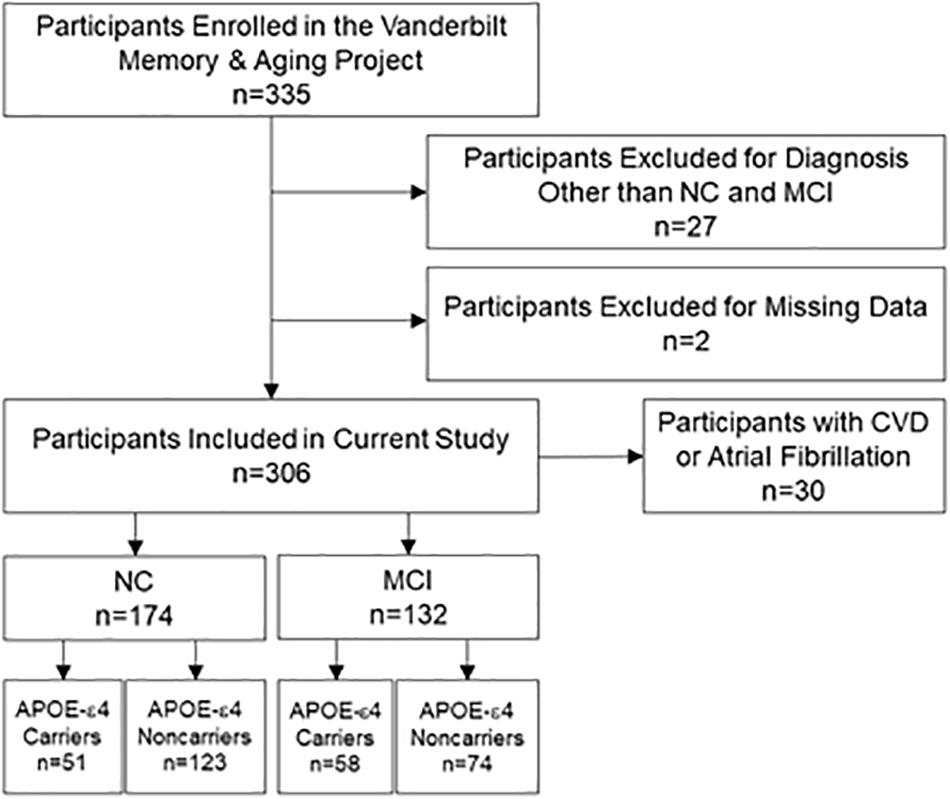
Figure 1. Participant inclusion and exclusion details. Missing data categories at baseline are mutually exclusive and included missing at least one neuropsychological outcome (n = 1) and missing covariate data (n = 1). Sensitivity analyses excluded participants with CVD or atrial fibrillation. APOE-ε4, apolipoprotein E ε4. CVD, cardiovascular disease. MCI, mild cognitive impairment. NC, normal cognition.
Standard Protocol Approvals, Registrations, and Participant Consents
The protocol was approved by the Vanderbilt University Medical Center Institutional Review Board. Written informed consent was obtained from all participants at each time point prior to data collection. Due to participant consent restrictions in data sharing, a subset of data is available for purposes of reproducing results or replicating procedures. Data, analytic methods, and study materials can be obtained by contacting the corresponding author.
Echocardiography
Standard 2-dimensional, M-mode, and Doppler transthoracic echocardiography was performed by a single research sonographer at the Vanderbilt University Medical Center Clinical Research Center on a Philips IE33 cardiac ultrasound machine (Philips Medical, Andover, MD). Digital images with measurements were confirmed by board-certified cardiologists (DKG, LAM) using commercially available software (HeartLab; AGFA Healthcare, Greenville, SC). All raters were blinded to clinical information.
Image acquisition and quantification were performed according to standards from the American Society of Echocardiography (Lang et al., 2015). Stroke volume was calculated from the left ventricular outflow tract velocity time integral and diameter, and cardiac output was calculated as stroke volume x heart rate. Final values were derived from a single cardiac cycle for participants in normal sinus rhythm or the average of three consecutive cardiac cycles in the presence of atrial fibrillation.
Neuropsychological Assessment
All participants underwent a comprehensive neuropsychological protocol at each time point, including Boston Naming Test (Kaplan et al., 1983), Animal Naming (Goodglass and Kaplan, 1983), WAIS-IV Coding (Wechsler, 2008), DKEFS Number Sequencing (Delis et al., 2001), DKEFS Tower Test (Delis et al., 2001), DKEFS Color-Word Inhibition (Delis et al., 2001), DKEFS Number-Letter Switching (Delis et al., 2001), Letter Fluency (FAS) (Benton et al., 1994), Hooper Visual Organization Test (Hooper, 1983), Biber Figure Learning Test (Glosser et al., 2002), and California Verbal Learning Test-II (Delis et al., 2000). Measures were carefully selected to preclude floor or ceiling effects and were not utilized for screening, diagnosis, or selection of participants for the study. To minimize multiple comparisons, composite z-scores were derived separately for episodic memory and executive function performances which leveraged item-level data from the multiple tests mentioned above to create bifactor latent variable models which fit the data well (i.e., root mean square error of approximation: 0.09 for memory and 0.03 for executive function) (Kresge et al., 2018).
Analytical Plan
Body surface area was calculated according to the DuBois formula (Du Bois and Du Bois, 1989): weight (kg)0.425 × height (cm)0.725 × 0.007184. Systolic blood pressure was the mean of two measurements. Diabetes mellitus included fasting blood glucose ≥ 126 mg/dL, hemoglobin A1c ≥ 6.5%, or oral hypoglycemic or insulin medication use. Medication review determined anti-hypertensive medication usage. Left ventricular hypertrophy was determined using echocardiogram as left ventricle mass index > 95 g/m2 in women or > 115 g/m2 in men. Atrial fibrillation included self-report with supporting evidence from echocardiogram, cardiac magnetic resonance, or prior surgery or medication usage to treat atrial fibrillation. Self-report was used to ascertain current cigarette smoking (yes/no within the year before baseline). Prevalent CVD from self-report with supporting medical record evidence included angina, coronary heart disease, or myocardial infarction (heart failure was an exclusion criterion at study enrollment). Framingham Stroke Risk Profile (FSRP) score was calculated by applying points by sex for age, systolic blood pressure accounting for anti-hypertensive medication usage, diabetes mellitus, current cigarette smoking, left ventricular hypertrophy, prevalent CVD, and atrial fibrillation (D’Agostino et al., 1994). APOE genotyping was performed using a TaqMan assay on DNA extracted from whole-blood samples as described previously (Jefferson et al., 2016), and APOE-ε4 carrier status was defined as positive (ε2/ε4, ε3/ε4, ε4/ε4) or negative (ε2/ε2, ε2/ε3, ε3/ε3).
Linear mixed-effects regression models related baseline cardiac output to longitudinal neuropsychological performance (one test per model), including an interaction with time to follow-up between baseline and last follow-up visit (in years) as the term of interest. We model the trajectory of cognition using these linear mixed-effect regression models, where terms involving follow-up time capture cognitive decline. Based on known associations with cardiovascular function and brain health, a series of covariates were identified a priori, including age (Wisdom et al., 2012), sex (Prabhavathi et al., 2014; McCarrey et al., 2016), education (Chen et al., 2019), race/ethnicity (Leigh et al., 2016), FSRP (excluding points for age) (Wolf et al., 1991; D’Agostino et al., 1994), and APOE-ε4 carrier status (Rawle et al., 2018). Additional covariates included body surface area (to adjust for individual differences in cardiac output) and follow-up time. Sensitivity analyses were performed excluding participants with prevalent CVD and atrial fibrillation, adjusting for cognitive diagnosis as well as outliers above 4 standard deviations. To test hypotheses related to APOE-ε4 status, models were repeated with a cardiac output x follow-up time x APOE-ε4 carrier status interaction term with follow-up models stratified by APOE-ε4 carrier status (APOE-ε4 carrier and non-carrier) with identical covariates. To test hypotheses related to cognitive diagnosis, models were repeated with a cardiac output x follow-up time x cognitive diagnosis interaction term with follow-up models stratified by cognitive diagnosis (NC and MCI). Lower order terms were included in all interaction models. To determine if outliers were driving the results, additional models were calculated excluding predictor or outcome values > 4 standard deviations from the group mean. Significance was set a priori at p < 0.05. False discovery rate (FDR) correction for multiple comparisons was performed using the Benjamini-Hochberg procedure adjusting for seven tests evaluated in planned analyses. All analyses were conducted using R 3.5.21.
Results
Participant Characteristics
The final sample included 306 participants with 174 NC and 132 MCI participants. The mean sample age at baseline was 73 ± 7 years (ranging 60–92 years), 58% were men, and 87% self-identified as non-Hispanic white. Cardiac output values ranged 2.0–8.7 L/min (5.0 ± 1.3) and did not differ between NC and MCI participants (p = 0.58). Sample characteristics are presented in Table 1. As expected, the MCI participants had worse baseline performance on all neuropsychological measures (Table 1) and greater annual longitudinal decline across all measures compared to NC participants (Table 2). Of the 306 participants included in the current study, 278 had more than one time point of data with a mean 3.5 ± 1.5 year follow-up for the sample which differed by participant group (NC = 3.8 ± 1.4, MCI = 3.0 ± 1.6, p < 0.001). Individuals with one time point of data (n = 28) were on average older (p = 0.004), less educated (p < 0.001), had a higher Framingham Stroke Risk Profile score (p = 0.02), and performed worse on all baseline cognitive measures (p < 0.03) than participants with multiple time points. Over the follow-up period, 33 participants developed incident dementia (defined as a change in Clinical Dementia Rating global score), eight experienced incident stroke, and two participants developed incident heart failure.
Cardiac Output and Longitudinal Neuropsychological Performances
Lower baseline cardiac output related to faster decline over the follow-up (defined as a negative trajectory of cognition, where terms involving follow-up time capture cognitive decline) for Boston Naming Test (β = 0.11, p = 0.01), Coding (β = 0.31, p = 0.006), Hooper Visual Organization Test (β = 0.09, p = 0.03), and episodic memory composite performances (β = 0.02, p = 0.001). Cardiac output was unrelated to longitudinal trajectory for the remaining neuropsychological measures (p > 0.12). See Table 3 for details and Figure 2 for illustrations of significant results. In sensitivity analyses excluding participants with prevalent CVD and atrial fibrillation, as well as excluding outliers, results were similar (data not shown). Results were similar when including cognitive diagnosis as a covariate. Results continued to meet the significance threshold when correcting for FDR using the Benjamini-Hochberg procedure except for Hooper Visual Organization Test (p = 0.05).
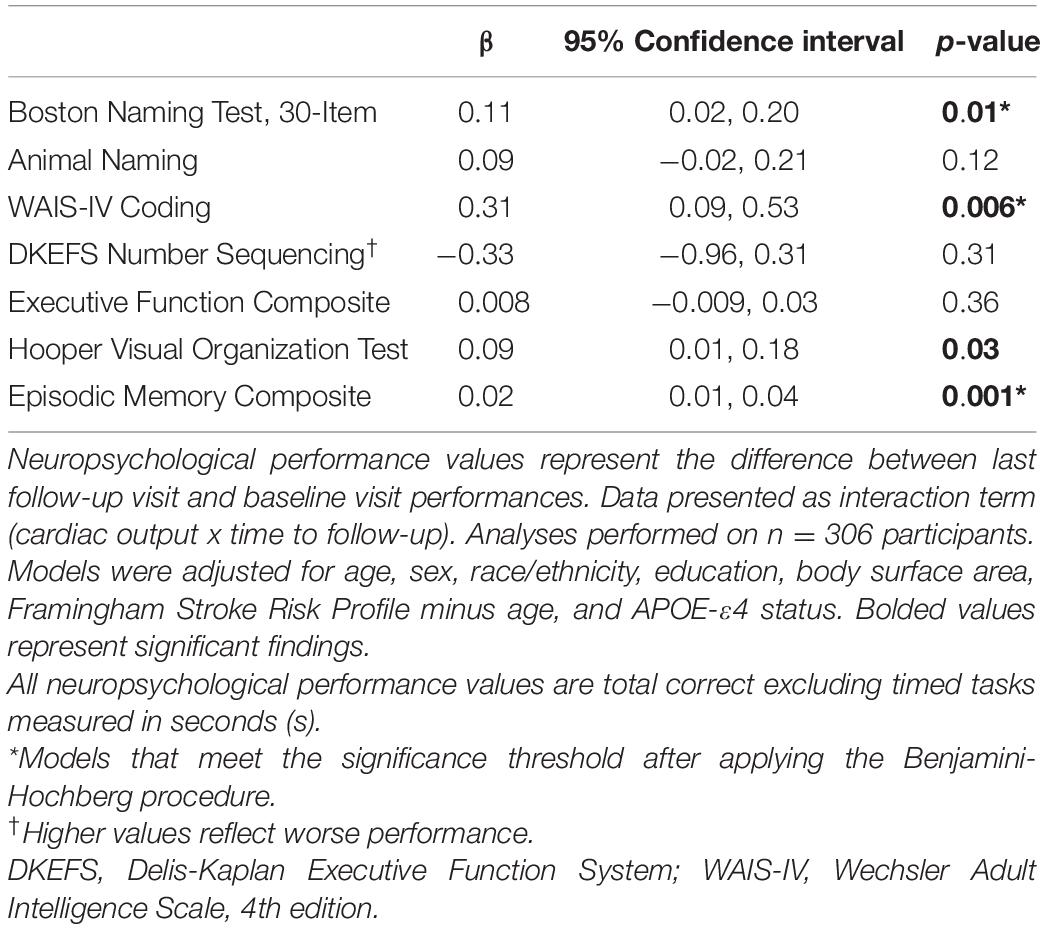
Table 3. Main effect results relating baseline cardiac output to longitudinal neuropsychological performances.
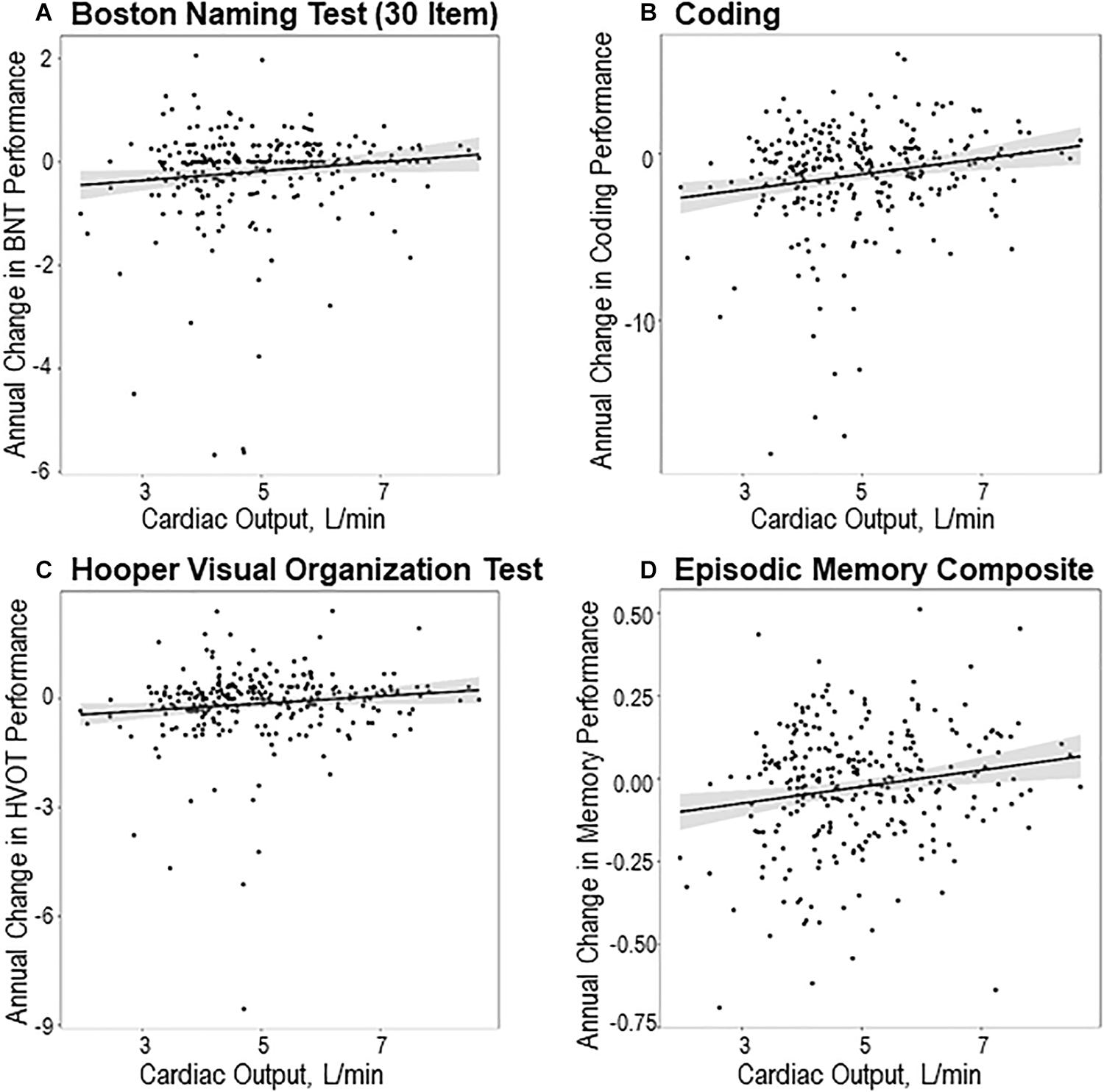
Figure 2. Cardiac output and longitudinal neuropsychological performances. Cardiac output and longitudinal neuropsychological performances, including (A) Boston Naming Test (30-Item), (B) Coding, (C) Hooper Visual Organization Test, and (D) episodic memory composite. Depicted are unadjusted scatter plots. Solid black line reflects fitted (predicted) values of annual change in cognitive outcomes (y-axis) corresponding to baseline cardiac output (x-axis). Shading reflects 95% confidence interval. All displayed plots are statistically significant (p < 0.05) and all models except for Hooper Visual Organization Test (p = 0.05) survive correction for false discovery rate. All participants in primary analyses are included in the scatter plots.
Cardiac Output × Cognitive Diagnosis and Longitudinal Neuropsychological Performances
No cardiac output× cognitive diagnosis interactions were observed for any longitudinal neuropsychological performances (p > 0.26). See Supplementary Table 1. Stratified models of participants with MCI were similarly null (p > 0.20) except for the episodic memory composite (β = 0.03, p = 0.02), which does not meet the significance threshold when correcting for FDR. Stratified models in NC participants revealed an association between lower baseline cardiac output and faster decline on Boston Naming Test (β = 0.07, p = 0.003), Coding (β = 0.26, p = 0.02), and Hooper Visual Organization Test performances (β = 0.06, p = 0.02). All of these results meet the significance threshold when correcting for FDR. Results were similar when excluding participants for prevalent CVD and atrial fibrillation, when including cognitive diagnosis as a covariate, and when excluding outliers (data now shown).
Cardiac Output × APOE-ε4 and Longitudinal Neuropsychological Performances
Cardiac output× APOE-ε4 carrier status modified the association between cardiac output and longitudinal Coding (β = 0.55, p = 0.02) and episodic memory composite performances (β = 0.03, p = 0.047) but neither of these measures met the significance threshold when correcting for FDR. See Table 4 for details and Supplementary Figure 1 for illustrations. No interactions were observed for the remaining measures (p > 0.08). Among the APOE-ε4 positive participants, stratified results suggested lower baseline cardiac output was related to faster decline on Coding (β = 0.71, p = 0.01) and episodic memory composite performances (β = 0.05, p = 0.004). Both findings survive correction for FDR. Among the APOE-ε4 negative subgroup, cardiac output was unrelated to any neuropsychological performance (p > 0.07). Significant APOE-ε4 interaction models were attenuated when excluding participants with prevalent CVD and atrial fibrillation but remained when excluding outliers. Results for models stratified by APOE-ε4 status were similar when excluding participants for prevalent CVD and atrial fibrillation (data not shown), and when excluding outliers. When including diagnosis as a covariate an additional significant interaction with APOE-ε4 status was found with Boston Naming Test (β = 0.19, p = 0.047).
Discussion
Among community-dwelling older adults free of clinical dementia, stroke, and heart failure, lower cardiac output at study entry related to faster decline in language, information processing speed, visuospatial skill, and episodic memory over the mean 3.5-year follow-up period. Cognitive diagnosis did not modify these associations, but a modest 3-way interaction amongst cardiac output, follow-up time, and APOE-ε4 was found, such that lower cardiac output at study entry was associated with worse longitudinal trajectory for information processing speed and episodic memory performances in APOE-ε4 carriers.
Our observations are among the first to extend prior cross-sectional findings that lower resting cardiac output relates to worse cognitive performance in community-dwelling older adults (Jefferson et al., 2010; Sabayan et al., 2015; Bown et al., 2019) by providing evidence that subtle reductions in cardiac output also relate to faster cognitive decline in domains of language, information processing, visuospatial skill, and episodic memory. Subtle reductions in cardiac output correspond to reduced CBF delivery as recently reported by our group (Jefferson et al., 2017). Chronic lower blood flow delivery to the brain could create a gradual metabolic energy crisis for neurons (Shuang Wan et al., 2016) and oxidative stress (Cacciamani et al., 2017) that concurrently promotes increased tau phosphorylation (Melov et al., 2007), mitochondrial dysfunction (Lu et al., 2004), and astrocyte dysregulation (Furuta et al., 2017). These changes are known to drive neurodegeneration, but lower blood flow could affect the brain through other pathways by inducing blood-brain barrier dysregulation (Krueger et al., 2017) or neuroinflammation (Miyanohara and Kakae, 2018), both of which might contribute to clinical symptoms and subsequent dementia. These possible pathways align with prior epidemiological evidence linking lower levels of cardiac output to incident dementia (Jefferson et al., 2015). The present study serves to further highlight the importance of maintaining a cardiovascular healthy lifestyle and its translation to enhanced brain health.
Tests of language, information processing speed, visuospatial skills, and episodic memory were all implicated in main effect models relating baseline cardiac output to longitudinal cognitive trajectory. Language abilities generally localize to the temporal lobes (Spitsyna et al., 2006) but the Boston Naming Test, a word-retrieval task implicated in this study, requires the convergence of semantic memory (Schwartz, 2014), lexical selection (Schwartz, 2014) and visual-perceptual processing (Soble et al., 2016) and can be indicative of a global phenomenon. Still, there may be specific brain regions that are more susceptible to hemodynamic fluctuations than others. For example, regions that are likely to have higher metabolic energy demand (Hawkins et al., 1979; Payabvash et al., 2011) or are located in territories that are difficult to perfuse are more vulnerable (Howard et al., 1987). A large portion of energy expenditure in the brain occurs at synapses (Attwell and Laughlin, 2001) and regions with high neuronal density could be more vulnerable to oligemia or hypoperfusion. The temporal lobes mediate memory and language functions, have higher synaptic density in older adults with normal cognition (Brown et al., 1998), and have a less extensive network of collateral blood vessels in humans (Liebeskind, 2003), which may increase vulnerability to alterations in CBF delivery. Additionally, basal ganglia nuclei, which mediate information processing speed (Cummings, 1998), are some of the most susceptible brain regions to ischemic damage in the context of hypoperfusion (Payabvash et al., 2011) due to being located in the internal watershed region of the brain (Pugh and Lipsitz, 2002). Future research incorporating multi-modal neuroimaging techniques should evaluate regional vulnerabilities to hemodynamic fluctuations and metabolic demands that may result in subsequent structural damage.
While we posit a direct association between cardiac function and brain health to explain the current results, another possible explanation is that findings reflect a top-down phenomenon where early brain changes associated with evolving neuropathology may be responsible for subtle changes in cardiac function. AD pathology develops up to 20 years before clinical symptoms manifest (Bateman et al., 2012) and corresponding chemical changes could affect autonomic nervous system functions (Engelhardt and Laks, 2008). Alternatively, the current findings could be the result of an epiphenomenon or unknown variables that drive changes in both cardiac function and cognition. Additional research is needed to better understand the underlying mechanism(s) accounting for these findings.
Cognitive diagnosis did not modify the association between baseline cardiac output and longitudinal neuropsychological performances. These longitudinal null results align with previous cross-sectional null results (Bown et al., 2019) and suggest that reduced cardiac output has a comparable effect on longitudinal cognitive trajectory regardless of cognitive status. The pathology underlying clinical symptoms in MCI may differ from the processes underlying the association between cardiac output and cognitive decline (Ye et al., 2015). Results presented here may have differed had we assessed our MCI participants years ago prior to clinical symptom onset or if we followed the cohort for a longer period of time (Jefferson et al., 2015).
We observed a subtle interaction between baseline cardiac output and APOE-ε4 carrier status on longitudinal information processing speed and episodic memory performances. Interestingly, episodic memory performance localizes to the medial temporal lobes (Tulving and Markowitsch, 1998) while information processing abilities rely on frontal-subcortical circuits (Cummings, 1998). As described above, both the basal ganglia and medial temporal lobes are regions that are susceptible to alterations in blood flow delivery. Therefore, APOE-ε4, a moderator of cerebrovascular damage (Halliday et al., 2016), may further increase the susceptibility of the temporal lobes and basal ganglia to damage associated with reduced cardiac output but caution must be taken with such interpretations of the present results. The two significant interactions do not survive correction for false discovery and are attenuated when excluding participants with prevalent CVD and atrial fibrillation, suggesting results may be spurious or driven by less healthy individuals. The mostly null interactions with APOE-ε4 carrier status in a longitudinal study of older adults could highlight a phenomenon where APOE-ε4 exerts its effects earlier in the aging process and becomes less relevant later in life (Blacker et al., 1997). This age-effect phenomenon is corroborated by recent cross-sectional results of an interaction between cardiac output and APOE-ε4 status on cognition for which effects were much more robust (Bown et al., 2019). Additional research is warranted to better understand the intersection of AD genetic risk and vascular health on risk for cognitive decline.
Our study has several strengths, including a longitudinal study design, a community-based cohort free of clinical dementia, stroke, and heart failure at baseline, a comprehensive neuropsychological protocol capturing a diverse range of cognitive outcomes across domains, a reliable imaging technique that reflects the clinical standard for quantifying cardiac output, and a core laboratory for processing all measurements by technicians blinded to clinical information. Additionally, our findings were adjusted for established vascular risk factors and remained consistent in sensitivity analyses excluding participants with prevalent CVD and atrial fibrillation. Despite these strengths, we must note a few limitations. First, our cohort is not reflective of the general population because participants were on average predominantly white, college-educated, older, and relatively cardiac healthy. In fact, participants who withdrew were on average clinically less healthy than participants who were retained over the follow-up, which likely biases our results to the null hypothesis. Generalizability of results to other races, ethnicities, ages, or adults with poorer health is unknown, though it is plausible that in a cohort with more vascular risk factors or cardiac dysfunction, associations might be stronger. Finally, multiple comparisons were used, increasing the likelihood of false-positive findings. We attempted to mitigate this limitation by employing the Benjamini-Hochberg procedure to control for the FDR, and main effect models for language, information processing, and episodic memory continued to meet the significance threshold (p < 0.03).
In summary, among community-dwelling older adults, subclinical reductions in systemic blood flow, as assessed by cardiac output, relate to worse longitudinal cognition, particularly in information processing speed, language, visuospatial skills, and episodic memory. Associations were statistically independent of concurrent vascular risk factors captured by FSRP, CVD, or atrial fibrillation and present among a cohort of participants free of clinical stroke, dementia, and heart failure at study entry. Results from the present study argue that vascular measures, such as cardiac output, have important implications in the maintenance of cognitive health with age. Further investigation into the underlying connection between cardiac output and abnormal brain aging may reveal molecular pathways for prevention or therapeutic purposes. Therapies aimed at improving cardiovascular integrity, including lifestyle interventions, should be investigated in more detail as one way to mitigate cognitive decline.
Data Availability Statement
Due to participant consent restrictions in data sharing, a subset of data is available for purposes of reproducing results or replicating procedures. Data, analytic methods, and study materials can be obtained by contacting the corresponding author.
Ethics Statement
The studies involving human participants were reviewed and approved by the Vanderbilt University Institutional Review Board. The patients/participants provided their written informed consent to participate in this study.
Author Contributions
CB and RD: lumped in with the other grouping that contributed analysis and interpretation of data as well as manuscript composition. OK, DL, FC, EM, KO, DG, and LM: analysis and interpretation of data and manuscript composition. KP: acquisition of data, analysis and interpretation of data, study supervision and manuscript composition. TH and KG: study concept and design, acquisition of data, analysis and interpretation of data, study supervision and manuscript composition. AJ: secured funding, study concept and design, acquisition of data, analysis and interpretation of data, study supervision and manuscript composition. All authors contributed to the article and approved the submitted version.
Funding
This research was supported by F31-AG066358 (CB), T32-AG058524 (CB), T32-GM007347 (EM), F30-AG064847 (EM), F31-AG059345 (FC), Howard Hughes Medical Institute James H. Gilliam Fellowship for Advanced Study (FC), F32-AG058395 (KO), Alzheimer’s Association IIRG-08-88733 (AJ), R01-AG034962 (AJ), R01-HL111516 (AJ), R01-AG056534 (AJ), R01-NS100980 (AJ), K24-AG046373 (AJ), K01-AG049164 (TH), K23-AG045966 (KG), UL1-TR000445 (Vanderbilt Clinical Translational Science Award), S10-OD023680 (Vanderbilt’s High-Performance Computer Cluster for Biomedical Research), the Vanderbilt Memory and Alzheimer’s Center and the Vanderbilt Alzheimer’s Disease Research Center (P20-AGAG068082).
Conflict of Interest
The authors declare that the research was conducted in the absence of any commercial or financial relationships that could be construed as a potential conflict of interest.
Acknowledgments
We would like to thank the dedicated Vanderbilt Memory and Aging Project participants, their loved ones, and our devoted staff and trainees who contributed to recruitment, screening, and enrollment of the cohort.
Supplementary Material
The Supplementary Material for this article can be found online at: https://www.frontiersin.org/articles/10.3389/fpsyg.2020.569355/full#supplementary-material
Footnotes
References
Albert, M. S., Dekosky, S. T., Dickson, D., Dubois, B., Feldman, H. H., Fox, N. C., et al. (2011). The diagnosis of mild cognitive impairment due to Alzheimer’s disease: recommendations from the National Institute on Aging-Alzheimer’s Association workgroups on diagnostic guidelines for Alzheimer’s disease. Alzheimer’s Dement. 7, 270–279. doi: 10.1016/j.jalz.2011.03.008
Attwell, D., and Laughlin, S. B. (2001). An energy budget for signaling in the grey matter of the brain. J. Cereb. Blood Flow Metab. 21, 1133–1145. doi: 10.1097/00004647-200110000-00001
Bateman, R. J., Xiong, C., Benzinger, T. L. S., Fagan, A. M., Goate, A., Fox, N. C., et al. (2012). Clinical and biomarker changes in dominantly inherited Alzheimer’s disease. N. Engl. J. Med. 367, 795–804. doi: 10.1056/NEJMoa1202753
Benton, A. L., Hamsher, K., and Sivan, A. B. (1994). Multilingual Aphasia Examination. Iowa City, IA: AJA Associates.
Blacker, D., Haines, J. L., Rodes, L., Terwedow, H., Go, R. C., Harrell, L. E., et al. (1997). ApoE-4 and age at onset of Alzheimer’s disease: the NIMH genetics initiative. Neurology 48, 139–147. doi: 10.1212/wnl.48.1.139
Bown, C. W., Liu, D., Osborn, K. E., Gupta, D. K., Mendes, L. A., Pechman, K. R., et al. (2019). Apolipoprotein E genotype modifies the association between cardiac output and cognition in older adults. J. Am. Heart Assoc. 8:e011146. doi: 10.1161/jaha.118.011146
Brown, D. F., Risser, R. C., Bigio, E. H., Tripp, P., Stiegler, A., Welch, E., et al. (1998). Neocortical synapse density and Braak stage in the Lewy body variant of Alzheimer disease: a comparison with classic Alzheimer disease and normal aging. J. Neuropathol. Exp. Neurol. 57, 955–960. doi: 10.1097/00005072-199810000-00007
Cacciamani, F., Tandetnik, C., Gagliardi, G., Bertin, H., Habert, M. O., Hampel, H., et al. (2017). Low cognitive awareness, but not complaint, is a good marker of preclinical Alzheimer’s disease. J. Alzheimer’s Dis. 59, 753–762. doi: 10.3233/jad-170399
Chen, J. J., Rosas, H. D., and Salat, D. H. (2011). Age-associated reductions in cerebral blood flow are independent from regional atrophy. Neuroimage 55, 468–478. doi: 10.1016/j.neuroimage.2010.12.032
Chen, Y., Lv, C., Li, X., Zhang, J., Chen, K., Liu, Z., et al. (2019). The positive impacts of early-life education on cognition, leisure activity, and brain structure in healthy aging. Aging 11, 4923–4942. doi: 10.18632/aging.102088
Cummings, J. L. (1998). Frontal-subcortical circuits and human behavior. J. Psychosom. Res. 44, 627–628.
D’Agostino, R. B., Wolf, P. A., Belanger, A. J., and Kannel, W. B. (1994). Stroke risk profile: adjustment for antihypertensive medication. The Framingham Study. Stroke 25, 40–43. doi: 10.1161/01.str.25.1.40
Delis, D. C., Kaplan, E., and Kramer, J. H. (2001). Delis-Kaplan Executive Function System (D-KEFS): Examiner’s Manual. San Antonio, TX: The Psychological Corporation.
Delis, D. C., Kramer, J., Kaplan, E., and Ober, B. (2000). California Verbal Learning Test–II, 2nd Edn. San Antonio, TX: The Psychological Corporation.
Du Bois, D., and Du Bois, E. F. (1989). A formula to estimate the approximate surface area if height and weight be known. 1916. Nutrition 5, 303–311; discussion 312–303.
Engelhardt, E., and Laks, J. (2008). Alzheimer disease neuropathology: understanding autonomic dysfunction. Dement. Neuropsychol. 2, 183–191. doi: 10.1590/s1980-57642009dn20300004
Furuta, T., Mukai, A., and Ohishi, A. (2017). Oxidative stress-induced increase of intracellular zinc in astrocytes decreases their functional expression of P2X7 receptors and engulfing activity. Metallomics 9, 1839–1851. doi: 10.1039/c7mt00257b
Glosser, G., Cole, L., Khatri, U., Dellapietra, L., and Kaplan, E. (2002). Assessing nonverbal memory with the biber figure learning test - extended in temporal lobe epilepsy patients. Arch. Clin. Neuropsychol. 17, 25–35. doi: 10.1016/s0887-6177(00)00093-7
Goodglass, H., and Kaplan, E. (1983). The Assessment of Aphasia and Related Disorders. Philadelphia, PA: Lea & Febiger.
Halliday, M. R., Rege, S. V., Ma, Q., Zhao, Z., Miller, C. A., Winkler, E. A., et al. (2016). Accelerated pericyte degeneration and blood-brain barrier breakdown in apolipoprotein E4 carriers with Alzheimer’s disease. J. Cereb. Blood Flow Metab. 36, 216–227. doi: 10.1038/jcbfm.2015.44
Hawkins, R., Hass, W. K., and Ransohoff, J. (1979). Measurement of regional brain glucose utilization in vivo using [2(-14)C] glucose. Stroke 10, 690–703. doi: 10.1161/01.str.10.6.690
Hooper, H. (1983). Hooper Visual Organization Test (HVOT). Los Angeles, CA: Western Psychological Services.
Howard, R., Trend, P., and Russell, R. W. (1987). Clinical features of ischemia in cerebral arterial border zones after periods of reduced cerebral blood flow. Arch. Neurol. 44, 934–940. doi: 10.1001/archneur.1987.00520210036016
Jefferson, A. L., Beiser, A. S., Himali, J. J., Seshadri, S., O’donnell, C. J., Manning, W. J., et al. (2015). Low cardiac index is associated with incident dementia and Alzheimer disease: the Framingham Heart Study. Circulation 131, 1333–1339. doi: 10.1161/CIRCULATIONAHA.114.012438
Jefferson, A. L., Gifford, K. A., Acosta, L. M., Bell, S. P., Donahue, M. J., Taylor Davis, L., et al. (2016). The vanderbilt memory & aging project: study design and baseline cohort overview. J. Alzheimer’s Dis. 52, 539–559. doi: 10.3233/JAD-150914
Jefferson, A. L., Himali, J. J., Beiser, A. S., Au, R., Massaro, J. M., Seshadri, S., et al. (2010). Cardiac index is associated with brain aging: the Framingham Heart Study. Circulation 122, 690–697. doi: 10.1161/CIRCULATIONAHA.109.905091
Jefferson, A. L., Liu, D., Gupta, D. K., Pechman, K. R., Watchmaker, J. M., Gordon, E. A., et al. (2017). Lower cardiac index levels relate to lower cerebral blood flow in older adults. Neurology 89, 2327–2334. doi: 10.1212/wnl.0000000000004707
Kaplan, E., Goodglass, H., and Weintraub, S. (1983). The Boston Naming Test. Philadelphia, PA: Lea & Febiger.
Kresge, H. A., Khan, O. A., Wegener, M. A., Liu, D., Terry, J. G., Nair, S., et al. (2018). Subclinical compromise in cardiac strain relates to lower cognitive performances in older adults. J. Am. Heart Assoc. 7:e007562. doi: 10.1161/JAHA.117.007562
Krueger, M., Hartig, W., Frydrychowicz, C., Mueller, W. C., Reichenbach, A., Bechmann, I., et al. (2017). Stroke-induced blood-brain barrier breakdown along the vascular tree - no preferential affection of arteries in different animal models and in humans. J. Cereb. Blood Flow Metab. 37, 2539–2554. doi: 10.1177/0271678x16670922
Lang, R. M., Badano, L. P., Mor-Avi, V., Afilalo, J., Armstrong, A., Ernande, L., et al. (2015). Recommendations for cardiac chamber quantification by echocardiography in adults: an update from the American Society of Echocardiography and the European Association of Cardiovascular Imaging. J. Am. Soc. Echocardiogr. 28, 1–39.e14. doi: 10.1016/j.echo.2014.10.003
Leigh, J. A., Alvarez, M., and Rodriguez, C. J. (2016). Ethnic minorities and coronary heart disease: an update and future directions. Curr. Atheroscler. Rep. 18:9. doi: 10.1007/s11883-016-0559-4
Liebeskind, D. S. (2003). Collateral circulation. Stroke 34, 2279–2284. doi: 10.1161/01.str.0000086465.41263.06
Lu, T., Pan, Y., Kao, S. Y., Li, C., Kohane, I., Chan, J., et al. (2004). Gene regulation and DNA damage in the ageing human brain. Nature 429, 883–891. doi: 10.1038/nature02661
Martins, C. A., Oulhaj, A., De Jager, C. A., and Williams, J. H. (2005). APOE alleles predict the rate of cognitive decline in Alzheimer disease: a nonlinear model. Neurology 65, 1888–1893. doi: 10.1212/01.wnl.0000188871.74093.12
McCarrey, A. C., An, Y., Kitner-Triolo, M. H., Ferrucci, L., and Resnick, S. M. (2016). Sex differences in cognitive trajectories in clinically normal older adults. Psychol. Aging 31, 166–175. doi: 10.1037/pag0000070
Melov, S., Adlard, P. A., Morten, K., Johnson, F., Golden, T. R., Hinerfeld, D., et al. (2007). Mitochondrial oxidative stress causes hyperphosphorylation of tau. PLoS One 2:e536. doi: 10.1371/journal.pone.0000536
Miyanohara, J., and Kakae, M. (2018). TRPM2 channel aggravates CNS inflammation and cognitive impairment via activation of microglia in chronic cerebral hypoperfusion. J. Neurosci. 38, 3520–3533. doi: 10.1523/jneurosci.2451-17.2018
Montagne, A., Barnes, S. R., Sweeney, M. D., Halliday, M. R., Sagare, A. P., Zhao, Z., et al. (2015). Blood-brain barrier breakdown in the aging human hippocampus. Neuron 85, 296–302. doi: 10.1016/j.neuron.2014.12.032
Payabvash, S., Souza, L. C., Wang, Y., Schaefer, P. W., Furie, K. L., Halpern, E. F., et al. (2011). Regional ischemic vulnerability of the brain to hypoperfusion: the need for location specific computed tomography perfusion thresholds in acute stroke patients. Stroke 42, 1255–1260. doi: 10.1161/STROKEAHA.110.600940
Prabhavathi, K., Selvi, K. T., Poornima, K. N., and Sarvanan, A. (2014). Role of biological sex in normal cardiac function and in its disease outcome - a review. J. Clin. Diag. Res. 8, Be01–Be04. doi: 10.7860/jcdr/2014/9635.4771
Pugh, K. G., and Lipsitz, L. A. (2002). The microvascular frontal-subcortical syndrome of aging. Neurobiol. Aging 23, 421–431. doi: 10.1016/s0197-4580(01)00319-0
Rawle, M. J., Davis, D., Bendayan, R., and Wong, A. (2018). Apolipoprotein-E (Apoe) ε4 and cognitive decline over the adult life course. Transl. Psychiatry 8:18. doi: 10.1038/s41398-017-0064-8
Roy, B., Woo, M. A., Wang, D. J. J., Fonarow, G. C., Harper, R. M., and Kumar, R. (2017). Reduced regional cerebral blood flow in patients with heart failure. Eur. J. Heart Fail. 19, 1294–1302. doi: 10.1002/ejhf.874
Sabayan, B., Van Buchem, M. A., Sigurdsson, S., Zhang, Q., Harris, T. B., Gudnason, V., et al. (2015). Cardiac hemodynamics are linked with structural and functional features of brain aging: the age, gene/environment susceptibility (AGES)-Reykjavik Study. J. Am. Heart Assoc. 4:e001294. doi: 10.1161/jaha.114.001294
Schwartz, M. F. (2014). Theoretical analysis of word production deficits in adult aphasia. Philos. Trans. R. Soc. B Biol. Sci. 369, 20120390. doi: 10.1098/rstb.2012.0390
Shuang Wan, E. Y., Shaik, M. A., Adhha, A., Hoo, Ng, R. M., Thompson, C., et al. (2016). Pilot evaluation of the informant AD8 as a case-finding instrument for cognitive impairment in general practitioner clinics of Singapore: a brief report. J. Am. Med. Direct. Assoc. 17, 1147–1150. doi: 10.1016/j.jamda.2016.07.031
Soble, J. R., Marceaux, J. C., Galindo, J., Sordahl, J. A., Highsmith, J. M., O’rourke, J. J., et al. (2016). The effect of perceptual reasoning abilities on confrontation naming performance: an examination of three naming tests. J. Clin. Exp. Neuropsychol. 38, 284–292. doi: 10.1080/13803395.2015.1107030
Spitsyna, G., Warren, J. E., Scott, S. K., Turkheimer, F. E., and Wise, R. J. (2006). Converging language streams in the human temporal lobe. J. Neurosci. 26, 7328–7336. doi: 10.1523/jneurosci.0559-06.2006
Tulving, E., and Markowitsch, H. J. (1998). Episodic and declarative memory: role of the hippocampus. Hippocampus 8, 198–204. doi: 10.1002/(sici)1098-1063(1998)8:3<198::aid-hipo2>3.0.co;2-g
Wisdom, N. M., Mignogna, J., and Collins, R. L. (2012). Variability in Wechsler Adult Intelligence Scale-IV subtest performance across age. Arch. Clin. Neuropsychol. 27, 389–397. doi: 10.1093/arclin/acs041
Wolf, P. A., D’agostino, R. B., Belanger, A. J., and Kannel, W. B. (1991). Probability of stroke: a risk profile from the Framingham Study. Stroke 22, 312–318. doi: 10.1161/01.str.22.3.312
Keywords: apolipoprotein E ε4, cognitive decline, aging, mild cognitive impairment, cardiac function
Citation: Bown CW, Do R, Khan OA, Liu D, Cambronero FE, Moore EE, Osborn KE, Gupta DK, Pechman KR, Mendes LA, Hohman TJ, Gifford KA and Jefferson AL (2020) Lower Cardiac Output Relates to Longitudinal Cognitive Decline in Aging Adults. Front. Psychol. 11:569355. doi: 10.3389/fpsyg.2020.569355
Received: 05 June 2020; Accepted: 08 October 2020;
Published: 09 November 2020.
Edited by:
Sara Palermo, University of Turin, ItalyReviewed by:
Fabrizio Stasolla, Giustino Fortunato University, ItalyLouise-Ann Leyland, University College London, United Kingdom
Copyright © 2020 Bown, Do, Khan, Liu, Cambronero, Moore, Osborn, Gupta, Pechman, Mendes, Hohman, Gifford and Jefferson. This is an open-access article distributed under the terms of the Creative Commons Attribution License (CC BY). The use, distribution or reproduction in other forums is permitted, provided the original author(s) and the copyright owner(s) are credited and that the original publication in this journal is cited, in accordance with accepted academic practice. No use, distribution or reproduction is permitted which does not comply with these terms.
*Correspondence: Angela L. Jefferson, angela.jefferson@vumc.org