- 1The Molecular & Behavioral Neuroscience Institute, University of Michigan, Ann Arbor, MI, United States
- 2Department of Psychiatry, University of Michigan, Ann Arbor, MI, United States
- 3National Institute on Alcohol Abuse and Alcoholism, National Institutes of Health, Bethesda, MD, United States
Heterogeneity within Major Depressive Disorder (MDD) has hampered identification of biological markers (e.g., intermediate phenotypes, IPs) that might increase risk for the disorder or reflect closer links to the genes underlying the disease process. The newer characterizations of dimensions of MDD within Research Domain Criteria (RDoC) domains may align well with the goal of defining IPs. We compare a sample of 25 individuals with MDD compared to 29 age and education matched controls in multimodal assessment. The multimodal RDoC assessment included the primary IP biomarker, positron emission tomography (PET) with a selective radiotracer for 5-HT1A [(11C)WAY-100635], as well as event-related functional MRI with a Go/No-go task targeting the Cognitive Control network, neuropsychological assessment of affective perception, negative memory bias and Cognitive Control domains. There was also an exploratory genetic analysis with the serotonin transporter (5-HTTLPR) and monamine oxidase A (MAO-A) genes. In regression analyses, lower 5-HT1A binding potential (BP) in the MDD group was related to diminished engagement of the Cognitive Control network, slowed resolution of interfering cognitive stimuli, one element of Cognitive Control. In contrast, higher/normative levels of 5-HT1A BP in MDD (only) was related to a substantial memory bias toward negative information, but intact resolution of interfering cognitive stimuli and greater engagement of Cognitive Control circuitry. The serotonin transporter risk allele was associated with lower 1a BP and the corresponding imaging and cognitive IPs in MDD. Lowered 5HT 1a BP was present in half of the MDD group relative to the control group. Lowered 5HT 1a BP may represent a subtype including decreased engagement of Cognitive Control network and impaired resolution of interfering cognitive stimuli. Future investigations might link lowered 1a BP to neurobiological pathways and markers, as well as probing subtype-specific treatment targets.
Introduction
An enduring, but incomplete observation in MDD (Major Depressive Disorder) is of serotonin dysfunction. Serotonin dysfunction is a corollary of the monoamine hypothesis, positing that MDD is associated with relative depletion of monoamines including catecholamines (e.g., dopamine and noradrenaline) and tryptamine (e.g., serotonin) (Wijaya et al., 2018). Parallel and sometimes convergent reports spanning neurochemistry, behavioral pharmacology, neuroimaging and gene have implicated serotonergic dysfunction in MDD. However, while such dysfunction can be thought of as a typical, it is very clearly not a universal characteristic of MDD (Albert and Lemonde, 2004; Kalia, 2005; Surtees et al., 2006). Evidence that serotonin function is relevant in a subset of those with MDD includes a number of different avenues of exploration. First, affective experience and emotional regulation are more dramatically altered after acute tryptophan depletion (ATD), more so in persons with personal or family history of MDD (Rogers et al., 2003; Fusar-Poli et al., 2006; Neumeister et al., 2006; Spring et al., 2007; van der Veen et al., 2007). Second, selective serotonergic reuptake inhibitors (SSRIs) are more effective than placebo in a majority of controlled clinical trials (Rush et al., 2006; Trivedi et al., 2006). Third, functional loci at genes that mediate serotonergic function have been implicated in MDD both alone, and via interaction with early life stress (Hariri et al., 2002, 2006; Sen et al., 2004; Neumeister et al., 2006; Surtees et al., 2006; Gotlib et al., 2008; Mak et al., 2013). Fourth, some of these functional variants in genes (modulating serotonin function) alter brain responses to emotion and brain connectivity (Dannlowski et al., 2008; Kalin et al., 2008; Elton et al., 2014; Wessa and Lois, 2015). These alternations align with a model of how inherited variations contribute to risk for MDD. Fifth, manipulations of serotonin function and/or use of agents with serotonergic effects within animal models can simulate depression and anxiety-like behaviors (Albert and Lemonde, 2004; Bert et al., 2008; Borg, 2008). Sixth, depression is associated with negative cognitive changes including memory and executive function impairments (Burt et al., 1995; Snyder, 2013; Yu et al., 2018), negative affect related to control, success, and rejection (Yeo et al., 2017) and increased negative schema (Stange et al., 2017; Lim et al., 2018). As such, there is continuing pursuit of domains affected in MDD that can be linked to serotonergic function and genes. There is also interest in whether these dimensional features may define a more homogeneous subset for further exploration and targeted treatment. A short review of the relevant links of serotonin dysfunction in MDD and of potential multimodal intermediate phenotypes [IPs (Burmeister et al., 2008; Kalin et al., 2008; Tan et al., 2008; Langenecker et al., 2010; Webb et al., 2016)] is conducted to integrate these separate lines of inquiry.
Imaging Studies of 5-HT1A Function
Evidence of abnormal 5-HT (5-hydroxytyptamine refers to G protein coupled receptors and ligand-gated ion channels, also known as serotonin receptors) function in MDD is building, including for 5-HT1A specifically (1A is a subtype of 5-HT receptor which is the most widespread 5-HT receptor, including within cortex and medial temporal structures). Past human imaging studies of 5-HT1A binding potential (BP) have focused on areas of binding where serotonin receptors are more densely populated, including the raphe, as well as frontal, cingulate and medial temporal cortices (Marazziti et al., 1994; Oquendo et al., 2003; Parsey et al., 2006, 2010; Drevets et al., 2007; Selvaraj et al., 2017; Kranz et al., 2018). 5-HT1A receptors regulate the firing of 5-HT neurons presynaptically in the raphe nuclei and are expressed postsynaptically in many different cortical and subcortical brain regions (Schlumpf et al., 1987; Kaufman et al., 2015; Zanderigo et al., 2018). In the cortex, there are inhibitory properties of the postsynaptic 5-HT1A receptors (Gross et al., 2002; Borg, 2008), plus regulation of the release of glutamate in subcortical structures (Czyrak et al., 2003). A recent review of concentrations of transporter (5HTT), 5-HT1A, and 5-HT2A receptors reflect the fact that 5-HTTs are densely populated in subcortical, pre-synaptic regions, whereas, 5-HT1A and 5-HT2A receptors are more dense in cortical regions (Kranz et al., 2010; Kautzky et al., 2018). These same cortical areas support a number of cognitive and affective processes and both the regions and the processes they support are heavily implicated in MDD (Teasdale and Dent, 1987; Heller and Nitschke, 1998; Hugdahl et al., 2003; Phillips et al., 2003, 2008; Drevets et al., 2007; Langenecker et al., 2007c, 2010, 2014; Porter et al., 2007; Disner et al., 2011).
Positron emission tomography (PET) studies, typically utilizing the selective radiotracer for 5-HT1A receptors, [11C]WAY-100635, have noted lowered levels of 5-HT1A receptor availability (BPND) within these regions in MDD as well as alterations in 5-HT1A availability pre- and post-treatment (Bhagwagar et al., 2004; Drevets et al., 2007; Moses-Kolko et al., 2007; Hirvonen et al., 2008; Kautzky et al., 2017). Lowered 5-HT1A BP in MDD is the general pattern observed; however, utilizing arterial sampling or a cortical reference region can make a significant impact on the direction of effects [higher or lower (Drevets et al., 2007; Parsey et al., 2010)]. As such, careful verification of reference region/marker equivalence between MDD and healthy comparison (HC) groups is important for PET studies with this radiotracer. Lower brainstem SERT BP was reported in an additional study of depressed suicide attempters (Nye et al., 2013). 5-HT1A disruptions have also been reported in high-risk offspring of those with MDD (Milak et al., 2018).
Animal Models of 5-HT1A in MDD
Given the limited number and variability across human in vivo studies, we briefly review the role of 5-HT1A in the pathophysiology of MDD as seen in animal models of depression and human postmortem studies. Animal studies have primarily reported increased 5-HT1A function after chronic SSRI administration (Haddjeri et al., 1998) and increases in anxious and depressive behaviors after 5-HT1A blockade, depletion, or knockout (Olivier et al., 2001;Akil, 2005; Zhang et al., 2006; Richardson et al., 2010). Novel antidepressants including agomelatine and vortioxetine induced modulation of brain-derived neurotrophic factor (BDNF) which is a neurotrophin that serves as a survival factor for neurons (Lu et al., 2018a,b). In a related study, BDNF knock out mice showed a significant attenuation of 5-HT1A receptor function (Hensler et al., 2007). Acute stress results in decreased 5-HT1A mRNA in the hippocampus (Lopez et al., 1999) and those with knockout or blockade demonstrate memory dysfunction (Sarnyai et al., 2000). Animals with 5-HT1A antagonist acute injection into the dorsal raphe show enhanced social defeat behavior (Cooper et al., 2008).
Stress-sensitive cynomolgus monkeys exhibit a reduced number of 5-HT1A receptors in dorsal raphe after stress exposure (Lima et al., 2009). Similarly, exposure to peer-rearing in rhesus monkeys as an early life stressor generally results in lower in vivo 5-HT1A receptor concentrations (Spinelli et al., 2009). Likewise, chronic psychosocial stress in tree shrews results in decreased 5-HT1A receptors in prefrontal cortex, hippocampus, and parietal cortex (Flugge, 1995).
Human Postmortem and Anatomical Studies
Furthermore, in human postmortem studies, lower hippocampal 5-HT1A mRNA is demonstrated in MDD subjects, with death by accident, assault, suicide, or cardiac causes (Lopez et al., 1998) and reduced 5-HT1A receptors in amygdala and hippocampus in suicide completers (Cheetham et al., 1990). Finally, 5-HT1A receptor density is related to gray matter volume cortical thickness in many prefrontal and parietal regions in HCs, but not in MDD (Pillai et al., 2018; Zanderigo et al., 2018). Notably, one recent study used PET binding to subdivide clusters in 5-HT function for anatomical parcellation and alignment with resting state networks (Kautzky et al., 2018). Both 5-HT1A and 5-HT2A demonstrate cortical; distribution and alignment with dorsal attention and frontoparietal networks (clusters 2 and 3), suggesting that is alignment between monamine function and cortical networks.
Tryptophan Depletion and Effects on Cognitive Control and Related Functions in MDD
A potentially convergent line of study is a possibility that divergent serotonergic function for some individuals with MDD is related to abnormalities in executive function and affective processing – these are broad domains within Research Domain Criteria [RDoC (Cuthbert, 2005)] that may constitute IPs for MDD. Executive functioning domains include conceptual reasoning, inhibitory control, verbal fluency, interference resolution, working memory – components of the Cognitive Control network. Difficulties in these skills are present in MDD (Austin et al., 1999; Channon and Green, 1999; Rogers et al., 2004; Langenecker et al., 2007b; Snyder, 2013) and lead to work-related disability and productivity loss (Lee et al., 2018). Links between these executive function skills (and cognitive control network function) and serotonergic function are conducted by reducing synthesis of 5-HT centrally via ATD (Lamar et al., 2009; Smith et al., 1999). ATD has also been shown to result in disrupted affective processing and networks (Phillips et al., 2003), increasing negative emotional experience and decreases in positive affective experience [Roiser et al., 2007; Spring et al., 2007; van der Veen et al., 2007]. ATD also disrupts social cooperation (Wood et al., 2006). Some affective domains of interest for MDD are enhanced memory for negative information and disrupted accuracy in processing of facial emotions [Gur et al., 1992; Langenecker et al., 2005, 2007b; Hsu et al., 2010].
In summary, there is a distinct possibility that disrupted 5-HT1A receptor mediated mechanisms might translate to affective and cognitive domains of dysfunction for MDD. Multimodal studies, encouraged by RDoC, can address convergence of multiple different assays. Here, we hypothesized that lower 5-HT1A BP in MDD [Hypothesis (Hyp) 1] maybe related to dysfunction in affective (bottom-up, Hyp 2) and executive (top-down, Hyp 3) domains (Langenecker et al., 2010, 2014; Disner et al., 2011). These affective dysfunction domains included negative memory bias, (Bradley et al., 1996; Hsu et al., 2010) and impaired emotion categorization (Gur et al., 1992; Langenecker et al., 2005, 2007b). We used executive dysfunction domains - previously identified factors in individuals with bipolar disease, similar to domains reported in MDD (Rogers et al., 2004; Snyder, 2013). These factors do not align perfectly with the RDoC domains within Cognitive Systems, although we note that the RDoC domains are suggestive and not prescriptive (Insel et al., 2010; Sanislow et al., 2010). The broader goal is to utilize dimensional, factor-driven analysis in studies where these are experimentally advantageous over DSM categories. Here, Cognitive Control subsumes the elements of (1) speed (Verbal Fluency and Processing Speed), (2) speed in the context of distracting or competing stimuli (Processing Speed with Interference Resolution), (3) stopping a prepotent response (e.g., regulation, here Inhibitory Control), and (4) balance of decision making within multistimulus sets and changing rules (Conceptual Reasoning and Set-Shifting) (Langenecker et al., 2010; Ryan et al., 2013).
We further investigated relationships of fMRI BOLD responses during a Cognitive Control task based upon 5-HT1A BP in the MDD sample, including fMRI BOLD responses based upon degree of 5-HT1A BPND in the MDD sample [Hyp 4 of lowered 5-HT1A BPND correlated with lowered activation in Cognitive Control region(s)]. Cross-modality comparisons are relatively rare in MDD (multimodal imaging can be simultaneous or on separate days), but they illustrate the value in integrating localization, function, and neurotransmitter density (Kalin et al., 2008; Selvaraj et al., 2017; Hamilton et al., 2018; Kranz et al., 2018; Piel et al., 2018). Analyses were also conducted with the HC group to verify the general or MDD specific nature of these relationships (Hyp 5). Exploratory analyses with genetic variants related to serotonergic function were also conducted (Hyp 6) (Wojnar et al., 2009; Villafuerte et al., 2009; Mak et al., 2013; Kautzky et al., 2017; Norgaard et al., 2017; Piel et al., 2018; Zanderigo et al., 2018).
Materials and Methods
Participants
Twenty-nine HC and 25 patients with MDD were recruited via newspaper advertisements, campus fliers, and word of mouth with Institutional Review Board (IRB)–approved written informed consent consistent with the Declaration of Helsinki at the University of Michigan. Diagnosis was confirmed with the Structured Clinical Interview for Diagnostic and Statistical Manual [DSM-IV (American Psychiatric Association, 1994)]. HC subjects were required to be below 5 and MDD subjects above 15 on the Hamilton Rating Scale for Depression for study entry [HRSD, 17 item scale (Hamilton, 1960)], using conservative thresholds for sensitivity and specificity (Naarding et al., 2002; Romera et al., 2011; Sawamura et al., 2018). The groups did not differ in age, sex, years of formal education, or intellectual ability [(Shipley, 1946), all p’s > 0.15, Table 1]. Other evidence of neurological or psychiatric disorders, other than generalized anxiety and/or social/specific phobia, panic disorder in the MDD sample was exclusionary. Cigarette smokers and those with alcohol abuse or who had used illegal drugs in the past 2 years were excluded. Patients with MDD were unmedicated and had been medication-free for a minimum of 6 months for all potentially psychoactive medications (mean 25.7 months, 14 medication naïve).
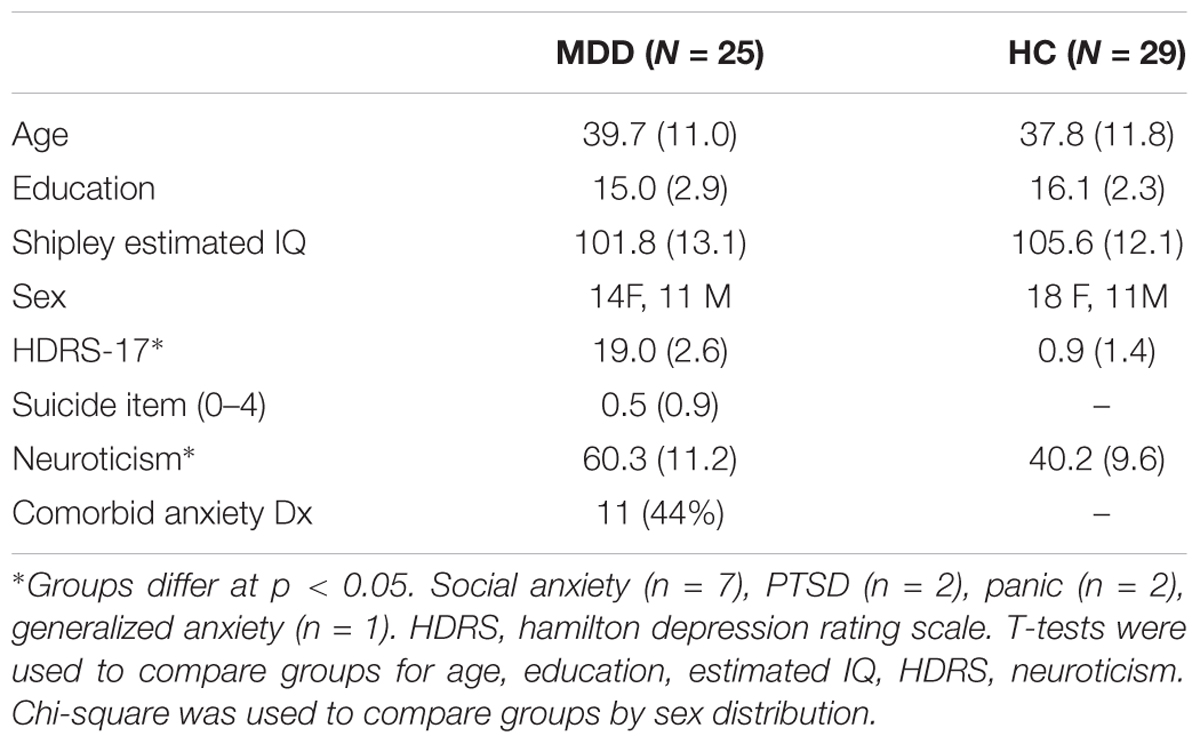
Table 1. Demographic and clinical information for participants with major depressive disorder and matching healthy control adults.
Overall Procedure
Neuropsychological measures were typically captured within several days after the intake and diagnosis. fMRI and PET were collected on average 9.5 days (SD = 36.8) apart for participants. The SD is large because 2 MDD and 2 HC participants discontinued and then restarted the study about 3–4 months apart. 85% of participants completed all evaluations within 1 month.
PET Scanning and Processing Procedures
Positron emission tomography scanning was conducted using [11C]Way100635. PET procedures were similar to those described previously (Mickey et al., 2008). PET images were acquired with a Siemens/CTI HR+ scanner in three-dimensional mode with septa retracted. [carbonyl- 11C]WAY-100635, a specific 5-HT1A receptor antagonist, was synthesized at high specific activity (Hwang et al., 1999). The tracer was administered as a bolus followed by continuous infusion to more rapidly achieve steady-state conditions. Eighteen scans of increasing duration (0.5–10 min) were acquired over a period of 90 min. Raw PET images were co-registered and smoothed with a Gaussian filter (4 mm FWHM). Smoothed images were transformed voxel-by-voxel into parametric maps of tracer transport (K1 ratio) and specific binding [distribution volume ratio (DVR)] using a modified Logan graphical analysis, with bilateral cerebellar white matter (excluding the vermis) as the reference region (Logan et al., 1996). Non-displaceable binding potential (BPND) was defined as BPND = DVR-1 = k2Bmax/KD, where Bmax is the total receptor concentration, KD is the dissociation constant, and k2 is the extracellular concentration of tracer (assumed to be a small and constant value) (Stange et al., 2017). Two individuals (one control and one MDD) showed visible binding in the cerebellum and (as a result) anomalously low global BPND (2.4–3.0 SDs below the mean, illustrated in Figure 1), and were excluded.
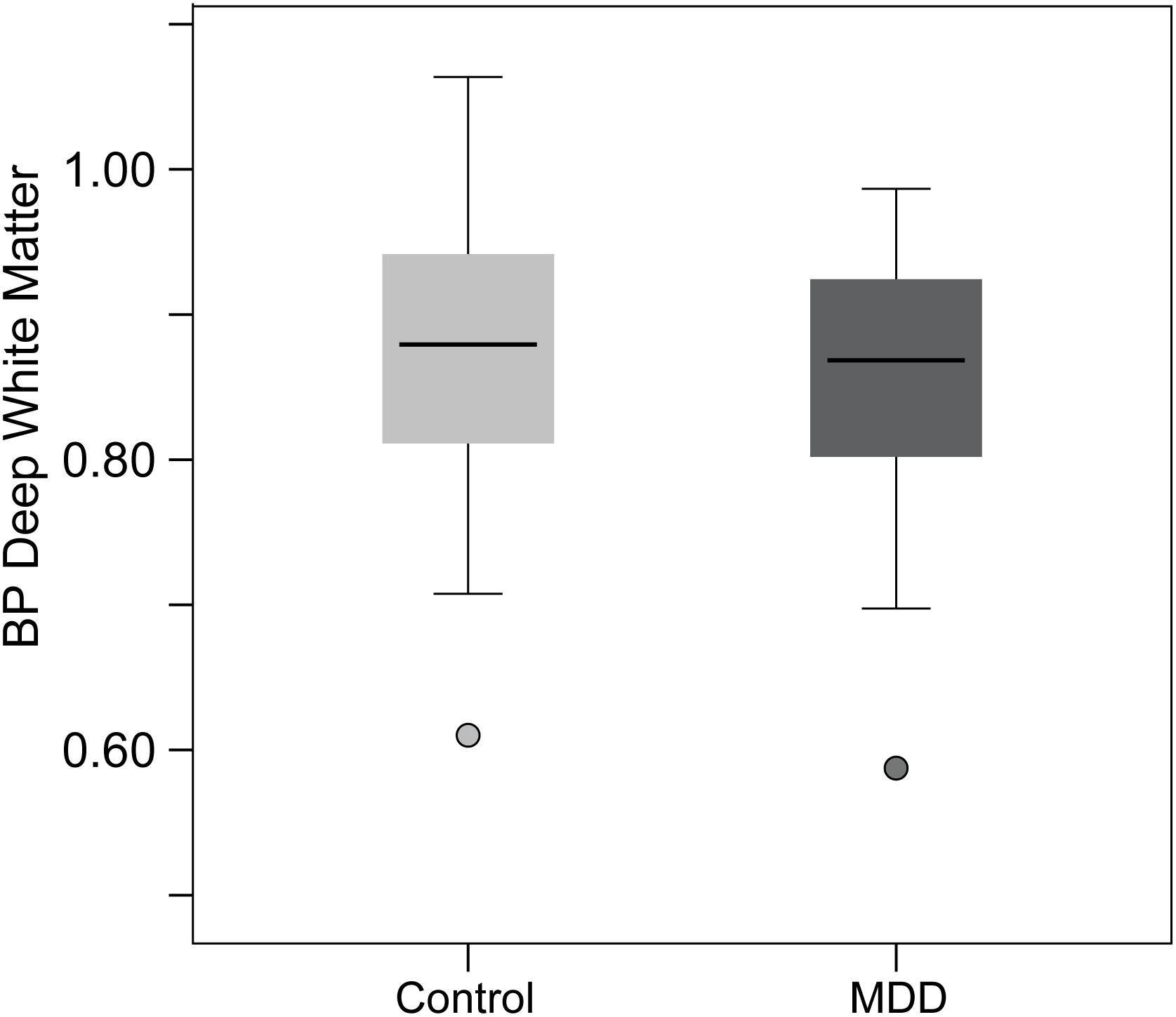
Figure 1. Figure illustrating the 5-HT1A BP values in the deep white matter (DWM) control region. Note that the two individuals (one MDD and one HC) with abnormally high binding potential (BP) in the cerebellar reference region resulted in low values in the DWM region. These two individuals were excluded from all analyses and the MDD and HC did not differ in DWM BP, indicating equivalence in the reference region. Further, no results were changed by including DWM BP as a covariate in analyses.
Positron emission tomography images were coregistered with MRI images to allow anatomical localization of PET data. Coregistration was accomplished for each subject by alignment of K1 images with MRI SPGR images using co-registration within SPM2. MRI data were subsequently transformed into standardized coordinates (International Consortium for Brain Mapping; Montreal Neurological Institute) by linear and non-linear warping, and the resulting transformation matrix was applied to parametric PET images.
Although not central to the current study or hypotheses, we specifically addressed the concern that lowered 5-HT1A BPND in MDD is a function of differences between HC and MDD in the cerebellar white matter reference region. Without an arterial reference point, we instead added a deep white matter (DWM) ROI within the centrum semiovale for test comparisons between MDD and HC subjects. This technique capitalizes on modeling DWM as a constant in the equation. Without any receptors, DWM would be a constant including noise – the only variable free to vary in the equation is BP within the cerebellar white matter reference region (including noise). There were no significant differences between groups in 5-HT1A BPND in the DWM of the centrum semiovale [t(41) = 0.55, p = 0.59]. The reference region BP was equivalent between groups, increasing confidence that effects reported herein are contingent upon inherent regional differences in BP between MDD and HC groups in the regions specified (Figure 1).
Candidate Affective and Executive Domains Relevant to MDD
The processing speed with interference resolution includes the trail making test, digit symbol substitution test, stroop color-word test, and response time to targets from the parametric go/no-go test. The parametric go/no-go test was programmed in EPrime 2 completed before the scanning session for practice (Langenecker, 2001; Langenecker et al., 2007a,b,c, 2018a; Votruba and Langenecker, 2013). It was also completed during fMRI. There are three levels of difficulty, including a 3 target Go-only condition, and 2 target alternating target Go/No-go condition, and a 2 target alternating target Go/No-go condition. There are 68 “lure” events so that correct and incorrect rejections of lures can be modeled and analyzed separately.
There are also less prominent potential domains/factors, less strongly linked to risk for MDD or BD, comprising Verbal Fluency with Processing Speed, Inhibitory Control, and Conceptual Reasoning and Set Shifting, and tests from these factors have been demonstrated to be dysfunctional in previous studies of MDD (see Langenecker et al., 2009 for a review). Negative Memory Bias (NMB) was calculated as a subtraction of percentage of negative words recognized from the percentage of neutral words recognized from within the Emotion Words task programmed in EPrime 2 (Hsu et al., 2010). In addition to the Negative Memory Bias, we also used performance accuracy in Emotion Classification of faces as potential Affective Processing domains in MDD that would be linked to abnormal 5-HT1A BPND (Langenecker et al., 2005).
MRI for Co-registration of PET Images and Collection of fMRI BOLD
One hundred twenty-four high-resolution SPGR axial anatomic images [TE = 5 ms; TR (repetition time) = 24 ms, 45 degree flip angle, NEX (number of excitations) = 2, slice thickness = 1.2 or 1.3 mm, FOV = 24 cm, matrix size = 256 × 256] were performed on each subject with a GE 3T Signa scanner for coregistration of PET images.
The Go/No-go task is a cognitive control task that has been used extensively by our group with fMRI, including in healthy aging, MDD, and bipolar disorder (for review, see Votruba and Langenecker, 2013). The fMRI task includes event-related models for correctly responded “go” events or Hits, correctly rejected “no-go” events or Rejections, and incorrectly responded “no-go” events, or Commissions, modeled with the hemodynamic response function. The steps for processing the data and model building include slice timing, physiological correction, coregistration, normalization, smoothing with a 5 mm FWHM Gaussian filter, and building individual models using SPM2 as described previously (Langenecker et al., 2007c). Contrasts were set up to define activation for Hits, Rejections, and Commissions in a fast event-related model. Imaging parameters include a TR of 2000 ms, FOV of 22 cm, with a 3.0 T GE Signa scanner using a standard radio frequency coil and T2∗- weighted pulse sequence. The images were collected using a forward-reverse spiral sequence with 29 axial slices of 4 mm.
Defining Regions of Significant Effect in 5-HT1A, and Low and Normal MDD Groups
Differences between groups in 5-HT1A BPND will be extracted from regions of significant effects (RSEs). 1st 5-HT1A BPND levels will be converted to z scores based upon mean and standard deviation of BPND levels for the HC group for each RSE. Then the z scores will be averaged across all RSEs to create a mean Z Group RSE variable across all post-synaptic 5-HT1A regions that differ between groups. Mean Z group RSE will be used as predictor variable in subsequent analyses with performance and fMRI IPs. We will use mean 5-HT1A BPND PET results in RSEs to define low and normal 5-HT1A BPND MDD groups in relation to HC 5-HT1A BPND in the RSEs. These two MDD groups will then be compared to identify regions for fMRI analyses in the imaging contrasts (Commissions, Correct Rejections, Hits) for the Parametric Go/No-go test.
Genotyping for 5HTTLPR and MAO-A
In addition, for exploratory purposes, the relative impact for 5-HTTLPR and MAO-A genotype were evaluated, genes with a significant biological relationship with 5-HT1A BP.
5HTTLPR
Genotyping protocols were performed according to Lesch et al. (1996). The 5-HTTLPR assay discriminates between two functional 5-HTT promoter alleles, visualized as DNA bands of 528 bp and 484 bp (long and short alleles, l and s, respectively). Genotypes were grouped in accordance with in vitro data on a reduced transcriptional activity of the dominating s allele that leads to a decrease in central 5-HT turnover (Bennett et al., 2002). Ten individuals did not have 5-HTTLPR genotype obtained (6 HC, 4 MDD).
MAO-A
Genomic DNA was purified from blood using standard methods. The MAOA promoter region that contains the upstream VNTR polymorphism (Sabol et al., 1998) was amplified from 10 ng genomic DNA using the primer sequences: Forward 5 CCCAGGCTGCTCCAGAAACATG-3 and Reverse 5′-GTTCGGGACCTGGGCAGTTGTG-3′. Because of the high GC content in the VNTR region, amplification was performed using Invitrogen’s PlatinumTaq and PCRX. Twenty-three individuals (12 HC, 11 MDD) did not have MAO-A genotype obtained.
Statistical Analyses
First, we identified MDD specific regions of low 5-HT1A BPND as described in the results section. We compared MDD and HC groups in 5-HT1A BPND using a combined threshold of p < 0.001 and a cluster minimum of 80mm3 was used between groups t-test in SPM2 (Table 2 and Figure 5). Mean 5-HT1A BPND was extracted for these Regions of Significant Effects (RSEs) and BPND was used in group specific linear regressions in SPSS 22 with behavioral performance measures of affective processing (Negative Memory Bias, Emotion Categorization) and executive functioning (Processing Speed with Interference Resolution, Verbal Fluency with Processing Speed, Inhibitory Control, and Conceptual Reasoning and Set Shifting) factors/scores were evaluated as converging, multimodal candidate IPs using MANOVA in SPSS 22. fMRI BOLD activation differences were also investigated in SPM5 factorial model comparisons of normal and low 5-HT1A BPND MDD groups based upon mean 1A BPND (with DWM BP as a covariate of no interest). Relationships between fMRI BOLD signal differences were evaluated subsequently with correlations with RDoC Domains scores in SPSS 22. These domains were also evaluated in exploratory analyses for serotonin-related genetic effects using 5-HTTLPR and MAO-A.
Results
Defining Regions of Low 5-HT1A Binding Potential in the MDD Group
Regions of significant BP differences between HC and MDD groups (HC > MDD) were used to define low 5-HT1A BPND MDD regions of significant effect (hereafter RSE). The whole brain analyses in MDD vs HC with 5-HT1A BPND indicated six RSEs of lower 5-HT1A BPND in the MDD group relative to the HC group. These regions, predominantly temporal, are reported in Table 2 and Figure 5, defining 10–20% reduction in post-synaptic 5-HT1A BPND in MDD across these regions. There were no regions where MDD group had greater BPND relative to the HC group. Half of the MDD group was below the 5th percentile of 5-HT1A BPND for the Z normed average of the RSEs relative to the HC group.
Impact of Low 5HT1a BPND on Executive Functioning and Affective Processing IPs in MDD
We investigated the linear relationship of IPs (e.g., Processing Speed with Interference Resolution, Inhibitory Control, and Negative Memory Bias) with mean rank 5-HT1A BP using regression in SPSS 22. Negative Memory Bias accounted for 16.4% (no difference after accounting for age) and Processing Speed with Interference Resolution accounted for 26.3% (35.4% after accounting for age effects) of mean rank BPND in the MDD group (covarying DWM DVR, p’s = 0.074, 0.029, respectively, Figure 2). No other IPs were significantly related to mean rank 5-HT1A BPND in the MDD group. In the control group, <1% variance in processing speed with interference resolution or negative memory bias was accounted for by mean rank BPND. Negative memory bias and processing speed with interference resolution were non-significantly correlated (r = -0.33, p = 0.17).
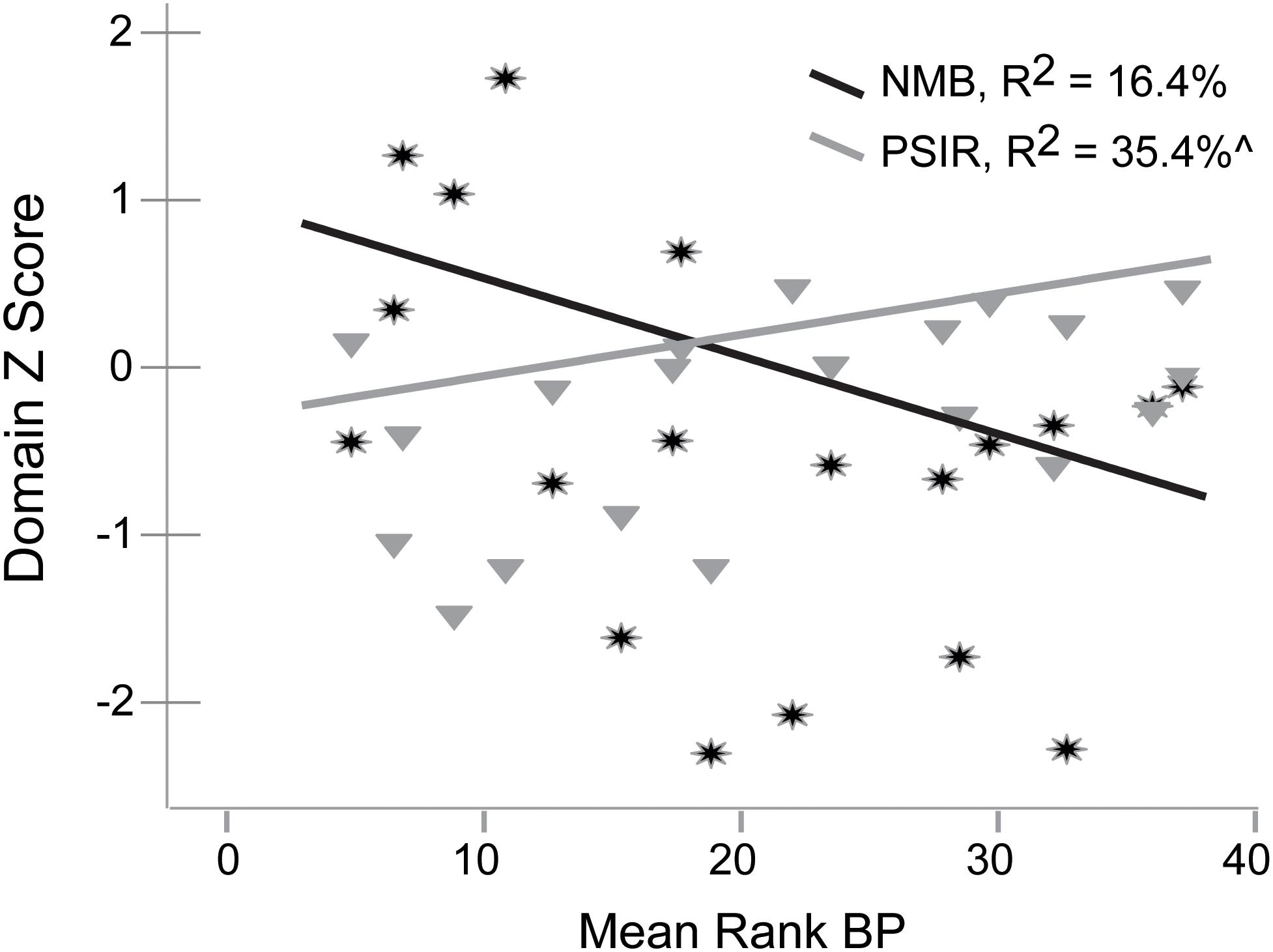
Figure 2. Relationships of Negative Memory Bias (NMB) and Processing Speed with Interference Resolution (PSIR) variables with mean rank 5-HT1A BP in the MDD group.
Lowered Mean 5-HT1A BP in Relation to fMRI BOLD Responses to Hits, Rejections, and Commissions Within the MDD Group
It was expected that abnormalities in executive functioning domains based upon mean 5-HT1A BPND would also be related to BOLD fMRI differences. As this has not been evaluated in published studies, there was no clear expectation of hyper or hypo (our hypothesis) activation during the Cognitive Control task for low vs normal 5-HT1A BP groups. These contrasts in SPM5 included in separate models for BOLD responses during Hits, Correct Rejections, and Commissions. Rejections and Commissions are used to calculate Inhibitory Control, which would be expected to be related to 5-HT1A BPND based upon relationships illustrated in Figure 3. Processing speed with interference resolution includes response speed to Hits and Conceptual Reasoning with Set Shifting includes Hit accuracy, suggesting that these Hit events should also be related to 5-HT1A BPND. There were some individuals without fMRI scans, some with abnormal DVR in the cerebellum (see section “Materials and Methods”), and some with low IQ, leaving, 17 MDD subjects available for fMRI analyses (divided into normal and low, in a model with 19 HCs). Whole brain analyses were conducted using combined height and extent thresholds with 3dClustSim (p < 0.005, k > 55, 1000 Monte Carlo simulations, p < 0.05 whole brain adjusted).
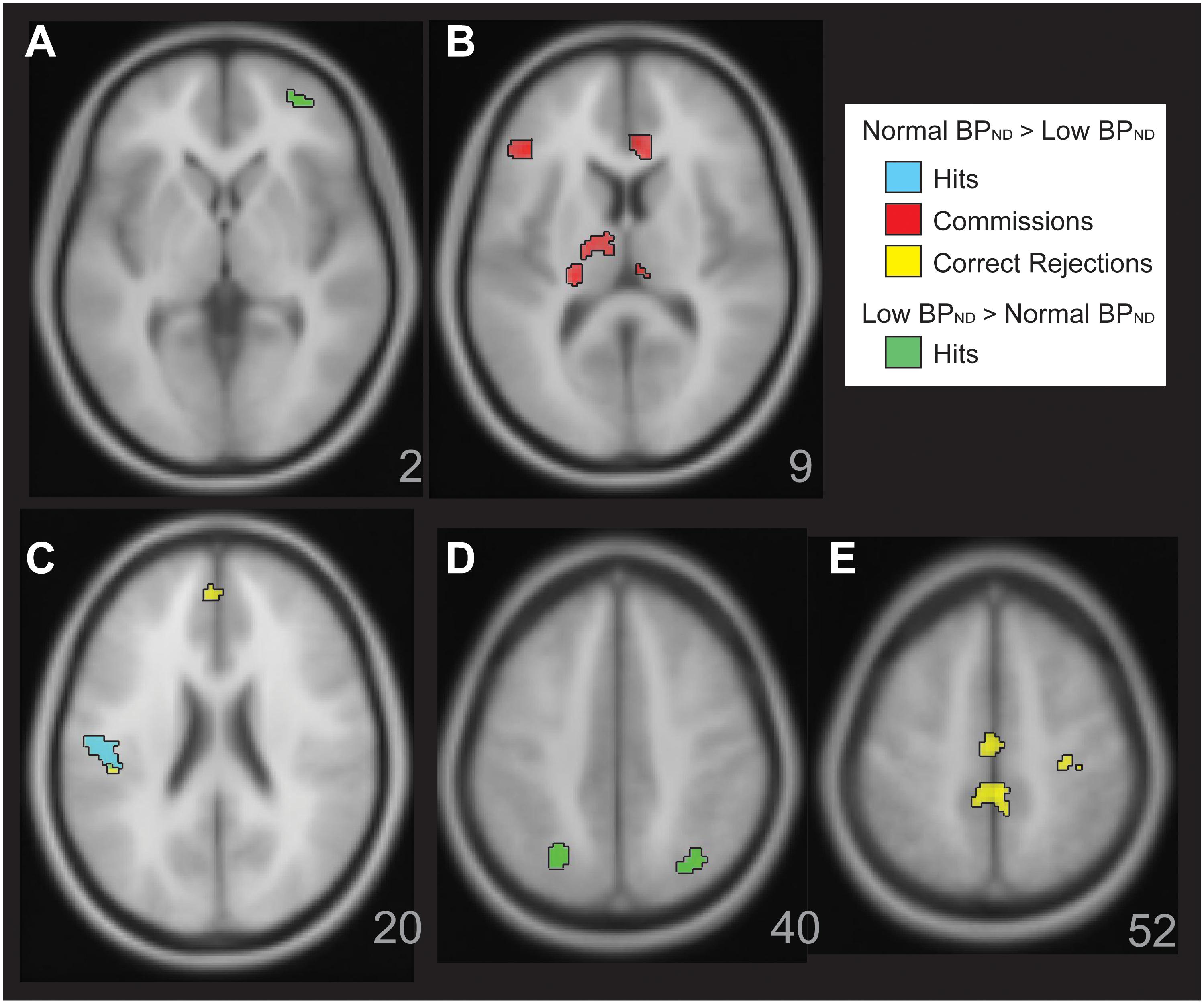
Figure 3. Figure illustrates significant BOLD activation relationships with 5HT-1a BP in the MDD group. There was greater BOLD activation with normal 5-HT1A BP MDD in the Parametric Go/No-go test. This is shown for correct Hits (Panel C,D, cyan), for Commissions (Panel B, red), Correct Rejections (Panels C,E, yellow). There were also a few areas of greater activation for correct Hits with low 5-HT1A BP in the MDD group (Panels A,D, green).
Within the MDD sample, there was a general pattern of greater activation with increasing mean 5-HT1A BPND. For Correct Rejections (yellow, Figure 3, Panels C, E), this was observed in dorsal anterior cingulate, postcentral gyrus, left posterior insula, and mid cingulate gyrus RSEs. There was increasing activation in rostral anterior cingulate, left inferior frontal gyrus, bilateral dorsal medial thalamus, and pulvinar RSEs with greater mean 5-HT1A BPND in relation to Commissions. There was greater activation for Hits in a left posterior insula RSE related to mean 5-HT1A BPND (cyan, Figure 3, Panel C). The exception to this general pattern of increased activation with increasing mean 5-HT1A BP in MDD was observed for Hits in bilateral superior parietal lobule and right anterior inferior frontal gyrus, where there was decreasing activation as mean 5-HT1A BPND increased (green, Figure 3, Panels A and D).
We further investigated dimensional, linear links between these multimodal IPs using pairwise correlations between the mean rank 5-HT1A BPND, mean rank for the combined fMRI BOLD RSEs (by condition), and behavioral performance parameters. These fMRI BOLD RSE clusters were combined by condition and group difference for purposes of data reduction, with the resulting mean Z BOLD RSE scores highly correlated with all individual clusters (r’s > 0.59 for Commission clusters, r’s > 0.68 for Correct Rejection clusters, r’s > 0.78 for Hits clusters, p’s < 0.001). As illustrated in Figure 4, the mean Z fMRI BOLD RSEs were significantly correlated with mean rank 5-HT1A BP for Hit BOLD RSE Normal > Low (r = 0.73, p < 0.001, Figure 4, Panel A), Hits mean Z BOLD RSEs Low > Normal (r = -0.80, p = 0.0001, Panel B), Commissions mean Z BOLD RSEs Normal > Low (r = 0.87, p = 0.0001, Panel E), and Rejections mean Z BOLD RSEs Normal > Low (r = 0.77, p = 0.0001, Panel C). PSIR Z score was positively correlated with fMRI BOLD Hit RSE (Normal > Low, r = 0.54, p = 0.02) and fMRI BOLD Commission mean Z RSEs (Normal > Low, r = 0.53, p = 0.03, Panel F). Negative Memory Bias Z was significantly positively correlated with fMRI BOLD Hit mean Z RSEs (Low > Normal, r = 0.50, p = 0.04) and negatively with fMRI BOLD Rejections mean Z RSEs (Normal > Low, r = -0.59, p = 0.01, Panel D). Information from 19 HCs with all modalities of measurement included – are added to these scatterplots (Figure 4) for comparison. The scatterplots indicate individual level differences in fMRI for Cognitive Control that relate to 5-HT1A BPND and PSIR.
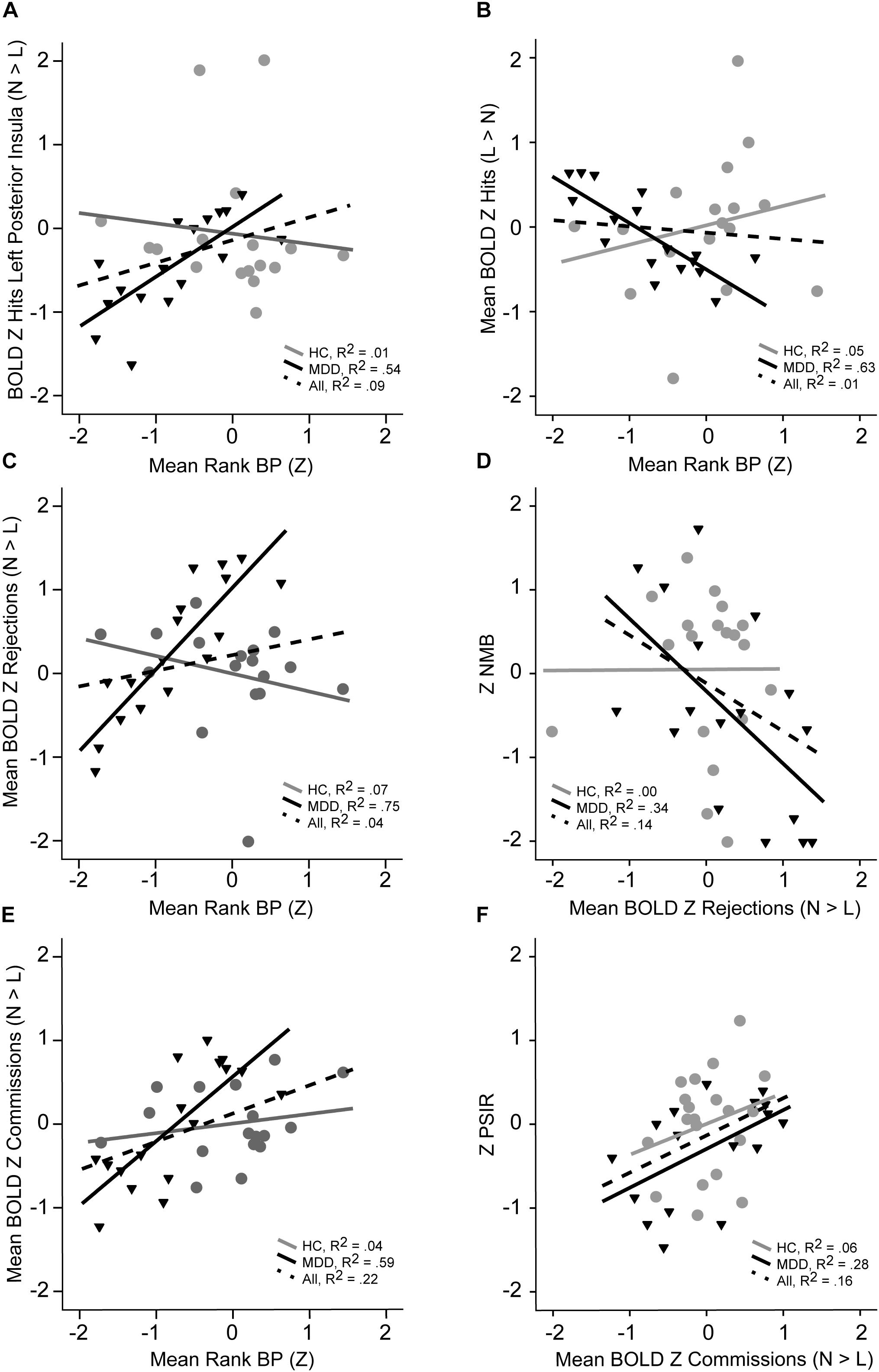
Figure 4. Illustration of linear relationships by group for 5-HT1A BP, fMRI BOLD signal, and neuropsychological performance measures (MDD in black, HC in gray, dashed line for both). Panel A shows the relationship between BOLD activation or Hits in left posterior insula with mean 5-HT1A BP rank. Panel B illustrates the relationship between mean BOLD signal RSEs for correct Hits that are greater in normal relative to lower 5-HT1A BP in MDD with mean 5-HT1A BP rank. Panel C depicts the relationship between mean BOLD for correct rejections and mean 5-HT1A BP rank. The relationship between mean BOLD for correct rejections and NMB in shown in Panel D. Panel E illustrates the mean BOLD for commission errors with mean 5-HT1A BP rank. The relationship between mean BOLD for commission errors and processing speed with interference resolution is shown in Panel F.
Exploratory Analyses of 5-HTTLPR and MAO-A Effects in Executive Functioning and Affective Processing IPs
Next, we expected that the low functioning forms of either the MAO-A and 5HTTLPR genotypes would be associated with poorer performance irrespective of group for candidate genes in exploratory analyses. Given the small sample size, and the relatively weak link between Cognitive/Affective IPs and functional polymorphisms that might impact 5-HT1A, the probability of type II error is high. The MANCOVA for 5-HTTLPR (diagnosis as covariate) was significant for Conceptual Reasoning and Set Shifting [F(1,29) = 4.29, p = 0.047, E2 = 0.13] and Processing Speed with Interference Resolution [F(1,29) = 5.93, p = 0.02, E2 = 0.17], with poorer performance in s allele carriers irrespective of group status. For definition of high and low functioning MAO-A genes, the intermediate genotype group of women was placed into the low functioning allele group based upon prior results (Austin et al., 1999) There was a significant effect for genotype on Conceptual Reasoning and Set Shifting [F(1,26) = 5.73, p = 0.02, E2 =0 .18] and a trend for Emotion Categorization [F(1,26) = 3.35, p = 0.08, E2 = 0.11]. Those with low function alleles for MAO-A performed better on Conceptual Reasoning and Set Shifting and marginally worse on Emotion Categorization.
5-HT1A BPND Links to 5-HTTLPR Genotype and Relationship With PSIR
Next, we evaluated specifically the effect of 5-HTLPPR genotype on 5-HT1A BPND reductions, covarying for disease group. Group results based upon disease (from Table 2) and genotype are displayed in Figure 5 (Panels A and B). Those with the 5-HTTLPR s allele, irrespective of disease status, exhibited lower 5-HT1A BPND in fronto-temporal regions, overlapping with temporal regions that were lower in those with MDD. There were additional frontal regions of lower 5-HT1A BPND in s allele carriers irrespective of diagnosis.
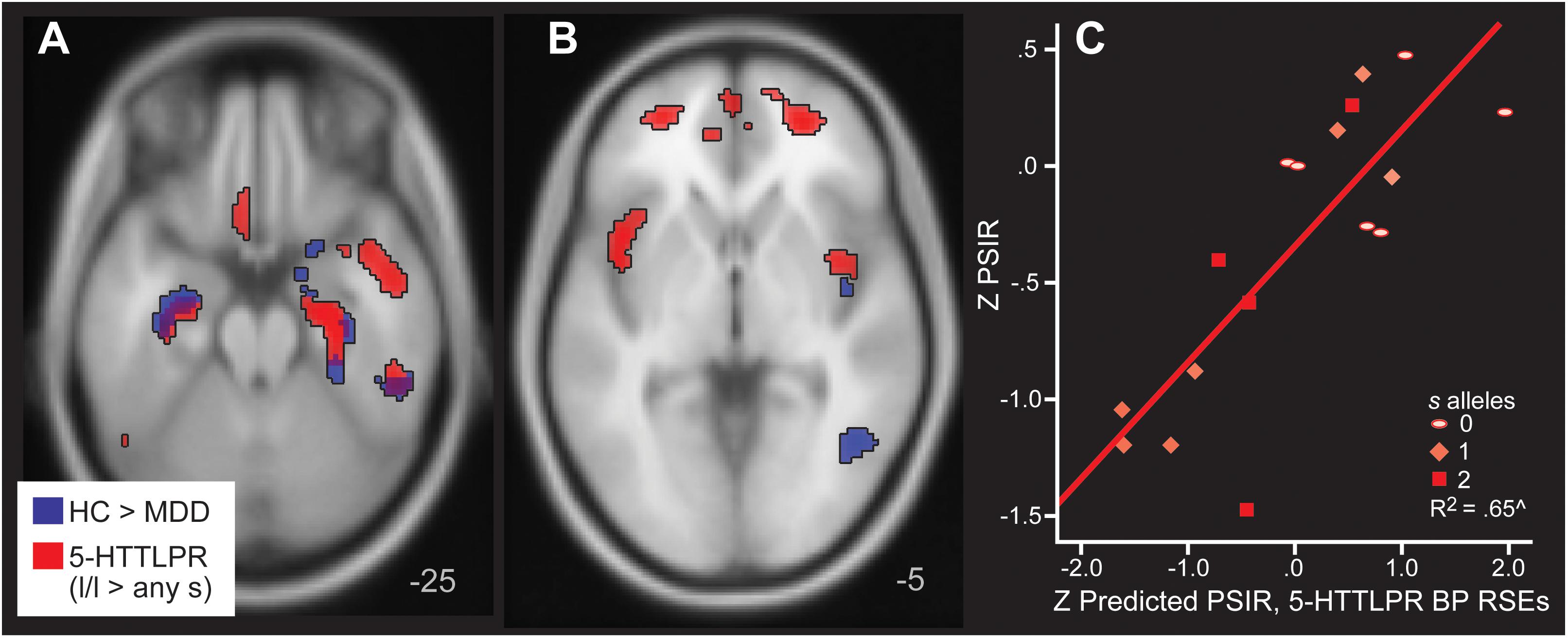
Figure 5. Areas of greater 5-HT1A BP in HC relative to MDD (blue) and in l homozygotes for 5-HTTLPR relative to s/s or l/s (red) in Panels A,B. Note that the blue clusters are the same as those listed in Table 2, which were used to define the regions of significant effect that defined mean rank BP regressors. They are included for comparison with the 5-HTTLPR analysis here to show the similarities in location and direction. Panel C depicts the actual and predicted PSIR values. The predictions are based upon mean 5-HT1A BP values from the regions of significant effect (RSEs) in the 5-HTTLPR analysis.
Mean rank order BP based upon 5-HTTLPR was averaged across all 16 RSEs of greater BP in l/l homozygotes relative to the s allele carriers. Regression was used to predict Processing Speed with Interference Resolution based upon 5-HTTLPR and also using mean rank BP from the 5-HTTLPR RSEs. Fifty-two percent of Processing Speed with Interference Resolution was explained by 5-HT1A BPND regions with significantly low BP extracted in those with an s allele (covarying age and DWM DVR, B = 0.79, p = 0.001, Figure 5, Panel C). 5-HT1A RSEs defined by MDD vs control and by 5-HTTLPR were highly correlated (r = 0.88, p < 0.001) in 5-HT1A BPND. MDD and 5-HTTLPR s allele were retained as independent variables in this analysis. No other regression models reached significance when using mean rank BP from the 5-HTTLPR RSEs to predict Negative Memory Bias, Emotion Categorization, Inhibitory Control, or Conceptual Reasoning with Set Shifting.
Discussion
The present study is the first to link abnormal 5-HT1A BPND measures in unmedicated, symptomatic patients with MDD to objective performance and imaging markers of illness, in this case interference resolution, a component of Cognitive Control. The separation of interference resolution performance by 5-HT1A levels is marked, with a medium-large effect size. The abnormal 5-HT1A BPND is also related to fMRI BOLD hypoactivation changes in a Cognitive Control domain during Inhibitory Control, the Parametric Go/No-Go Test. The impact of 5-HTTLPR genotype upon interference resolution and 5-HT1A is modest and significant. The results follow previous studies showing links between 5-HT1A BPND values with clinical factors such as anxiety symptoms, treatment outcome, genetics, and sex (Bhagwagar et al., 2004; Drevets et al., 2007; Hirvonen et al., 2008; Miller et al., 2008; Parsey et al., 2010). There is also evidence that lower 5-HT1A levels are present when there are increased depression symptoms in the context of epilepsy, Parkinson disease and in chronic stress without depression (Jovanovic et al., 2008). Using objective, but simpler, performance measures to identify subjects with a higher probability of abnormal 5-HT1A BPND could have substantial benefits for clinical, genetic and research studies. The executive functioning measures used to derive the processing speed with interference resolution variables are inexpensive to administer and are easily employed in subject recruitment (even clinical) settings (Langenecker et al., 2007b; Dawson et al., 2017). These measures could be used to select individuals for treatments or research protocols that specifically target 5-HT1A receptor functioning and for subtype-specific pharmacotherapy treatment trials. Analogs of these performance measures are already present in animal models to further aid in strategies for better understanding the neurobiology and genetics of depression and for new treatment development.
In the data presented it was striking that Cognitive Control, and not Negative Memory Bias (inverted effect) or Emotion Categorization, was related to lower 5-HT1A BPND in MDD. Indeed, recent studies have demonstrated that executive functioning measures, an umbrella domain term that includes Cognitive Control, are perhaps most critical in understanding increased risk for MDD, and are observed in the remitted state, and in family relatives of those with mood disorders (Clark et al., 2005; Bora et al., 2009; Peters et al., 2017). A recent review illustrated how executive dysfunction for those with MDD is substantial and fairly consistent across well-powered studies (Rogers et al., 2004). Some existing literature, although mainly with small N studies, suggest that executive functioning is also a good predictor of treatment response and functioning in MDD (Kampf-Sherf et al., 2004; Taylor et al., 2006; Jaeger et al., 2007; Dawson et al., 2017). Executive functioning also can be used to predict recurrence (Langenecker et al., 2018a) and workplace disability (Lee et al., 2018).
A recent review of 5-HT1A receptor studies suggested that there is a weak relationship between 5-HT1A and cognitive function (Borg, 2008). One of four studies in healthy controls have illustrated a relationship between 5-HT1A BPND and cognitive performance (Yasuno, 2004). A pilot study in those with Alzheimer’s disease, mild cognitive impairment (MCI), or neither suggested a relationship of decreased 5-HT1A BPND with poorer MMSE in the entire sample, and with learning and memory in the healthy control and MCI participants (Kepe et al., 2006). Another study suggests that gray matter thickness in key limbic regions is positively associated with 5-HT1A (Kraus et al., 2012). A similar study shows these associations in fronto-limbic regions (Zanderigo et al., 2018). In our control sample we replicate the pattern of non-significant relationships of 5-HT1A BPND to cognitive and affective measures. However, in MDD, we demonstrate a significant positive relationship of 5-HT1A with Negative Memory Bias and Inhibitory Control, and a significant negative relationship of 5-HT1A with Processing Speed with Interference Resolution.
fMRI BOLD signal changes for correct rejections and errors of commission resulted in hypoactivation in critical regulatory and inhibitory regions for those with lower 5-HT1A BPND. These low BOLD signals were related to Negative Memory Bias and Processing Speed with Interference Resolution. Notably, those MDD with lower 5-HT1A BPND levels show increasing difficulties with poorer set-shifting and processing speed. In contrast and with an intriguing result, MDD subjects with higher/normative 5-HT1A BPND levels exhibited significant Negative Memory Bias and increased activation in regulatory regions during successful rejection. As a result, increased need for recruitment in the mid-dorsal and rostral anterior cingulate for successful rejection may reflect poorer Inhibitory Control in those without lower 5-HT1A BPND levels. This observation is confirmed by performance above normal levels in Inhibitory Control as 5-HT1A BPND levels decreased. Those with higher/normative5-HT1A BPND 5-HT1A BPND levels tended to have worse Negative Memory Bias, Inhibitory Control, and increased BOLD recruitment for successful rejection of prepotent stimuli. Although the sample is quite small, these results reaffirm with other work that there likely many circuits, neurotransmitters, and behaviors associated with subtypes of MDD that are heretofore unclear (Webb et al., 2016; Kling et al., 2018).
Further, 5-HTTLPR appears to be related to both 5-HT1A BPND levels, and executive functioning performance, irrespective of illness. Notably, samples of this small size often suffer from difficulty with replication and should be interpreted very cautiously. There are some clues, however, that interference resolution might explain the inconsistent findings in emotion processing studies of 5-HTTLPR from other studies as well. For example, affective processing and executive regulation can be in dynamic opposition to one another in some contexts, or in the case of psychiatric illness, there may be excessive responses in the former and weaker control in the latter (Phillips et al., 2003; Kampf-Sherf et al., 2004; Langenecker et al., 2007c, 2014). Presence of the low functioning alleles of 5-HTTLPR may reflect a relatively weaker executive functioning system, resulting in a stronger environmental dependence in the development of and execution of emotion regulation (Jacobs et al., 2006; Dannlowski et al., 2008; Lohoff et al., 2014; Piel et al., 2018). In non-stressful environments, this weakness is less likely to result in problematic outcomes, but could still result in excessive responses to negative emotional stimuli (Sen et al., 2004; Surguladze et al., 2008). This pattern of diminished regulation skill in those carrying the short allele may be exaggerated in MDD (Dannlowski et al., 2008). In high demand, high stress, negative environments, there may be greater difficulty in regulating negative emotional responses, and greater difficulty in shifting from one emotional state to another for those with low functioning 5-HTTLPR alleles (Jacobs et al., 2006; Neumeister et al., 2006; Piel et al., 2018). The lack of regulation or emotional flexibility to environmental demands could then perpetuate depressive symptoms.
Use of screening tools like PSIR measures, with knowledge of convergent results with the 5-HT1A BPND levels may be one dimensional way of increasing the homogeneity in MDD samples. Such increased homogeneity could lead to more targeted, precision medicine trials. For example, preselecting individuals based upon poor Cognitive Control could lead to a larger percentage with low 5-HT1A BPND, facilitating identification of related biomarkers for treatment. As we already know that weaker CC is a predictor of poor treatment response, greater likelihood of recurrence, these individuals might benefit from different treatment algorithms [e.g., TMS trials (Kampf-Sherf et al., 2004; Januel et al., 2006; Siegle et al., 2006; Langenecker et al., 2007c,d, 2018a,b; Levkovitz et al., 2009; Drysdale et al., 2016; Crane et al., 2017; Dawson et al., 2017; Natania et al., 2018)].
It is also notable that Emotion Categorization accuracy, although related at a trend level to MAO-A genotype, was not related to 5-HT1A BPND levels or 5-HTTLPR. More surprisingly, higher mean rank BP was associated with greater Negative Memory Bias in MDD. Those with lower 5-HT1A BPND exhibited no evidence of a Negative Memory Bias, suggesting that using mean rank BP to illustrate dimensional functioning in MDD could be defined in part by the segregation of Affective and Executive Domains. In reality, though, risk for MDD is likely defined by affective dysfunction, executive dysfunction, or dysfunction in both systems, and it is unlikely to be so clearly illustrated based upon results from one ligand. Several recent studies reported mixed results in links between serotonin genes and emotion reactivity (Kranz et al., 2018; Piel et al., 2018).
The main limitation of the study is the sample size. We were able to obtain a relatively large sample for a multimodal study, and we were also able to find strong biological links between different measurement modalities. Samples of this size only reliably find large and very large effect sizes. We did obtain robust results consistent with our hypotheses. Another potential limitation of the present study relates to the broader difficulty within the field to agree upon the best reference strategy for calculating 5-HT1A BPND. Although we have shown equivalence in our reference region between groups. There are challenges to the reference region approach that appear to be surmountable by excluding gray matter and vermis, such as in this work (Parsey et al., 2010), and verifying equivalence of the reference region, as we have done. Animal models, especially those of 5-HT1A knockout mice, suggest that there may be lower 5-HT1A availability in MDD and similar states, resulting in decreased serotonergic regulation. Likewise, postmortem data also suggests decreased 5-HT1A mRNA in MDD. These findings are contrasted with others suggesting that higher 5-HT1A in mice can mimic aspects of autism and not anxiety/depression. We contend that the present results strengthen evidence that lower 5-HT1A BPND is a viable IP in MDD. This is in part upon the clear lack of BP difference in a deep white matter region, the presence of a behavioral performance correlate, links to fMRI BOLD, and link to a genetic marker 5-HTTLPR. At the very least, the fact that 5-HT1A BPND is altered in some individuals with MDD is clear. Until the discrepant reference region/correction methods can be resolved, directionality is still contested. Individual studies will have to demonstrate equivalence of reference ranges/structures. Furthermore, newer radioligands such as (Elton et al., 2014) MPFF might have more sensitive and stable properties for investigation of 5-HT1A BPND with MDD subjects absent these reference region concerns (Lothe et al., 2012).
In addition, fMRI is expensive and requires extensive equipment for set-up and analysis. A lower cost alternative to measure hemoglobin changes during task may be functional near-infrared spectroscopy (fNIRS) (Ho et al., 2016). As a number of the imaging regions identified here were cortical, it is possible that fNIRS could be used less expensively and with a broader range of patients to understand the relationship of hemodynamic changes to lowered 5-HT1A function and cognitive control (McKendrick et al., 2015). Finally, neuropsychological testing, fMRI and PET were typically collected on separate days and locations. The anatomical cross-localization could be off in such instances, and the measurements could be weakened by day-session specific parameters (Selvaraj et al., 2017; Hamilton et al., 2018). As the relationships were quite robust, there may have been additional links that were missed.
5-HT1A function has a number of associations with other chronic diseases that are often comorbid with MDD, including coronary artery disease and obesity (Vickers and Dourish, 2004; Ramage and Villalon, 2008; Quek et al., 2017; Ho et al., 2018), and changes in 5-HT1A function are coassociated with changes in pro-inflammatory cytokines including interleukin-1 beta (IL-1β), IL-17, and tumor necrosis factor – alpha (TNF-α) (Aune et al., 1993; Lu et al., 2017; Ng et al., 2018). These studies suggest that 5-HT1A shares functions that are related to, but extend beyond the phenotype of MDD, which in light of the present study, further confirms a need for homogeneous subsets that can be used to explore specific biological pathways for illness and recovery.
Conclusion
In conclusion, the present study offers promising new evidence that biomarkers for MDD can be found and objectively measured. The heterogeneity of MDD has been problematic in pursuing these biomarkers, and identification of subtypes of MDD may, in the end, prove to be the most fruitful in linking biomarkers, to phenotypes, to genetic risks, and ultimately to personalized medicine. The phenotypic heterogeneity of MDD, combined with prior attempts at a “one size fits all” biomarker approach to MDD has been one limiting factor in this complex illness. Future work can capitalize upon the relationship between 5-HT1A BPND abnormalities and executive functioning in MDD. These links could also be pursued more broadly in other psychiatric conditions within the RDoC initiative, as executive functioning disruption is not specific to MDD.
Ethics Statement
The work was approved by the University of Michigan IRB. Written informed consent was obtained from all participants.
Author Contributions
SL designed the study, analyzed the data, and wrote and edited the manuscript. BM performed the PET and cognitive analyses, and wrote and edited the manuscript. PE, SK, and TL performed the PET analysis, and wrote and edited the manuscript. SS performed the genetics analysis, and wrote and edited the manuscript. KE performed the analysis, and wrote and edited the manuscript. MH and SR wrote and edited the manuscript. DH performed the fMRI and PET analyses, and wrote and edited the manuscript. RK designed PET, and edited the manuscript. SW and HA designed the study and edited the manuscript. DG performed the genetics, analysis and edited the manuscript. MB designed the study, performed the genetics analysis, and edited the manuscript. J-KZ designed the study, and wrote and edited the manuscript.
Funding
NIMH grants P01 MH42251 (J-KZ, Elizabeth A. Young, HA), K23 MH074459 (SL), and the National Alliance for Research on Schizophrenia and Depression (NARSAD, SL, J-KZ). SL was supported by RDoC grant MH101487. Also supported by the General Clinical Research Center at the University of Michigan (NIH grant RR00042), now MICH-R CTSA (UL1RR024986).
Conflict of Interest Statement
The authors declare that the research was conducted in the absence of any commercial or financial relationships that could be construed as a potential conflict of interest.
Acknowledgments
We thank Virginia Murphy-Weinberg, RN, MS, Emily M. Briceño, Leslie M. Guidotti-Breting, Allison M. Kade and the technologists of the PET Center and fMRI Laboratory at the University of Michigan for their assistance in the performance of the studies. We thank Colin Hodgkinson, NIAAA, NIH for assistance in genetic analyses.
References
Akil, H. (2005). Stressed and depressed. Nature Nat. Medicine Med. 11, 116–118. doi: 10.1038/nm0205-116
Albert, P. R., and Lemonde, S. (2004). 5-HT1A receptors, gene repression, and depression: guilt by association. Neuroscientist 10, 575–593. doi: 10.1177/1073858404267382
American Psychiatric Association. (1994). Diagnostic and Statistical Manual of Mental Disorders, 4th Edn. Washington, DC: American Psychiatric Association.
Aune, T. M., McGrath, K. M., Sarr, T., Bombara, M. P., and Kelley, K. A. (1993). Expression of 5HT1a receptors on activated human T cells. Regulation of cyclic AMP levels and T cell proliferation by 5-hydroxytryptamine. J. Immunol. 151, 1175–1183.
Austin, M. P., Wilhelm, K., Parker, G., Hickie, I., Brodaty, H., Chan, J., et al. (1999). Cognitive function in depression : a distinct pattern of frontal impairment in melancholia? Psychological Psychol. Medicine Med. 29, 73–85.
Bennett, A. J., Lesch, K. P., Heils, A., Long, J. C., Lorenz, J. G., Shoaf, S. E., et al. (2002). Early experience and serotonin transporter gene variation interact to influence primate CNS function. Mol. Psychiatry 7, 118–122. doi: 10.1038/sj.mp.4000949
Bert, B., Fink, H., Rothe, J., Walstab, J., and Bonisch, H. (2008). Learning and memory in 5-HT(1A)-receptor mutant mice. Behav. Brain Res. 195, 78–85. doi: 10.1016/j.bbr.2008.02.028
Bhagwagar, Z., Rabiner, E. A., Sargent, P. A., Grasby, P. M., and Cowen, P. J. (2004). Persistent reduction in brain serotonin1A receptor binding in recovered depressed men measured by positron emission tomography with [11C]WAY-100635. Mol. Psychiatry 9, 386–392. doi: 10.1038/sj.mp.4001401
Bora, E., Yucel, M., and Pantelis, C. (2009). Cognitive endophenotypes of bipolar disorder: a meta-analysis of neuropsychological deficits in euthymic patients and their first-degree relatives. J. Affect. Disord. 113, 1–20. doi: 10.1016/j.jad.2008.06.009
Borg, J. (2008). Molecular imaging of the 5-HT1A receptor in relation to human cognition. Behav. Brain Res. 195, 103–111. doi: 10.1016/j.bbr.2008.06.011
Bradley, B. P., Mogg, K., and Millar, N. (1996). Implicit memory bias in clinical and non-clinical depression. Behav. Res. Ther. 34, 865–879. doi: 10.1016/S0005-7967(96)00074-5
Burmeister, M., McInnis, M. G., and Zollner, S. (2008). Psychiatric genetics: progress amid controversy. Nat. Rev. Genet. 9, 527–540. doi: 10.1038/nrg2381
Burt, D. B., Zembar, M. J., and Niederehe, G. (1995). Depression and memory impairment: a meta-analysis of the association, its pattern, and specificity. Psychol. Bull. 117, 285–305. doi: 10.1037/0033-2909.117.2.285
Channon, S., and Green, P. S. (1999). Executive function in depression: the role of performance strategies in aiding depressed and non-depressed participants. J. Neurol. Neurosurg. Psychiatry. 66, 162–171.
Cheetham, S. C., Crompton, M. R., Katona, C. L., and Horton, R. W. (1990). Brain 5-HT1 binding sites in depressed suicides. Psychopharmacology (Berl) 102, 544–548. doi: 10.1007/BF02247138
Clark, L., Sarna, A., and Goodwin, G. M. (2005). Impairment of executive function but not memory in first-degree relatives of patients with bipolar I disorder and in euthymic patients with unipolar depression. Am. J. Psychiatry 162, 1980–1982. doi: 10.1176/appi.ajp.162.10.1980
Cooper, M. A., McIntyre, K. E., and Huhman, K. L. (2008). Activation of 5-HT1A autoreceptors in the dorsal raphe nucleus reduces the behavioral consequences of social defeat. Psychoneuroendocrinology 33, 1236–1247. doi: 10.1016/j.psyneuen.2008.06.009
Crane, N. A., Jenkins, L. M., Bhaumik, R., Dion, C., Gowins, J. R., Mickey, B. J., et al. (2017). Multidimensional prediction of treatment response to antidepressants with cognitive control and functional MRI. Brain 140, 472–486. doi: 10.1093/brain/aww326
Cuthbert, B. N. (2005). Dimensional models of psychopathology: research agenda and clinical utility. J. Abnorm. Psychol. 114, 565–569. doi: 10.1037/0021-843X.114.4.565
Czyrak, A., Czepiel, K., Mackowiak, M., Chocyk, A., and Wedzony, K. (2003). Serotonin 5-HT1A receptors might control the output of cortical glutamatergic neurons in rat cingulate cortex. Brain Res. 989, 42–51. doi: 10.1016/S0006-8993(03)03352-3
Dannlowski, U., Ohrmann, P., Bauer, J., Deckert, J., Hohoff, C., Kugel, H., et al. (2008). 5-HTTLPR biases amygdala activity in response to masked facial expressions in major depression. Neuropsychopharmacology 33, 418–424. doi: 10.1038/sj.npp.1301411
Dawson, E. L., Caveney, A. F., Meyers, K. K., Weisenbach, S. L., Giordani, B., Avery, E. T., et al. (2017). Executive functioning at baseline prospectively predicts depression treatment response. The Primary Prim. Care Companion 19, e1–e7. doi: 10.4088/PCC.16m01949
Disner, S. G., Beevers, C. G., Haigh, E. A., and Beck, A. T. (2011). Neural mechanisms of the cognitive model of depression. Nat. Rev. Neurosci. 12, 467–477. doi: 10.1038/nrn3027
Drevets, W. C., Thase, M. E., Kolko-Moses, E. L., Price, J., Frank, E., and Kupfer, D. J. (2007). Serotonin-1A receptor imaging in recurrent depression: replication and literature review. Nucl. Med. Biol. 34, 865–877. doi: 10.1016/j.nucmedbio.2007.06.008
Drysdale, A. T., Grosenick, L., Downar, J., Dunlop, K., Mansouri, F., Meng, Y., et al. (2016). Resting-state connectivity biomarkers define neurophysiological subtypes of depression. Nat. Med. 23, 28–38. doi: 10.1038/nm.4246
Elton, A., Tripathi, S. P., Mletzko, T., Young, J., Cisler, J. M., James, G. A., et al. (2014). Childhood maltreatment is associated with a sex-dependent functional reorganization of a brain inhibitory control network. Hum. Brain Mapp. 35, 1654–1667. doi: 10.1002/hbm.22280
Flugge, G. (1995). Dynamics of central nervous 5-HT1A-receptors under psychosocial stress. J. Neurosci. 15, 7132–7140. doi: 10.1523/JNEUROSCI.15-11-07132.1995
Fusar-Poli, P., Allen, P., McGuire, P., Placentino, A., Cortesi, M., and Perez, J. (2006). Neuroimaging and electrophysiological studies of the effects of acute tryptophan depletion: a systematic review of the literature. Psychopharmacology 188, 131–143. doi: 10.1007/s00213-006-0493-1
Gotlib, I. H., Joormann, J., Minor, K. L., and Hallmayer, J. (2008). HPA axis reactivity: a mechanism underlying the associations among 5-HTTLPR, stress, and depression. Biological Biol. Psychiatry The Serotonin Transporter Gene and Stress Reactivity: Reflections on Altered Amygdala Reactivity 63, 847–851. doi: 10.1016/j.biopsych.2007.10.008
Gross, C., Zhuang, X., Stark, K., Ramboz, S., Oosting, R., Kirby, L., et al. (2002). Serotonin1A receptor acts during development to establish normal anxiety-like behaviour in the adult. Nature 416, 396–400. doi: 10.1038/416396a
Gur, R. C., Edwin, R., Gur, R., Zwil, A., Heimberg, C., and Kraemer, H. (1992). Facial emotion discrimination: II Behavioral findings in depression. Psychiatry Research Res. 42, 241–251. doi: 10.1016/0165-1781(92)90116-K
Haddjeri, N., Blier, P., and de Montigny, C. (1998). Montigny, Long-term antidepressant treatments result in a tonic activation of forebrain 50HT1A receptors. Journal J. of Neuroscience. 18, 10150–10156. doi: 10.1523/JNEUROSCI.18-23-10150.1998
Hamilton, J. P., Sacchet, M. D., Hjørnevik, T., Chin, F. T., Shen, B., Kämpe, R., et al. (2018). Striatal dopamine deficits predict reductions in striatal functional connectivity in major depression: a concurrent 11C-raclopride positron emission tomography and functional magnetic resonance imaging investigation. Translational Transl. Psychiatry 8, :264. doi: 10.1038/s41398-018-0316-2
Hamilton, M. (1960). A rating scale for depression. J. Neurol. Neurosurg. Psychiatry 23, 56–62. doi: 10.1136/jnnp.23.1.56
Hariri, A. R., Drabant, E. M., and Weinberger, D. R. (2006). Imaging genetics: perspectives from studies of genetically driven variation in serotonin function and corticolimbic affective processing. Biol. Psychiatry 59, 888–897. doi: 10.1016/j.biopsych.2005.11.005
Hariri, A. R., Mattay, V. S., Tessitore, A., Kolachana, B., Fera, F., Goldman, D., et al. (2002). Serotonin transporter genetic variation and the response of the human amygdala. Science 297, 400–403. doi: 10.1126/science.1071829
Heller, W., and Nitschke, J. (1998). The puzzle of regional brain activity in depression and anxiety: the importance of subtypes and co-morbidity. Cognition Cogn. & Emotion. 12, 421–447. doi: 10.1080/026999398379664
Hensler, J. G., Advani, T., and Monteggia, L. M. (2007). Regulation of serotonin-1A receptor function in inducible brain-derived neurotrophic factor knockout mice after administration of corticosterone. Biol. Psychiatry 62, 521–529. doi: 10.1016/j.biopsych.2006.10.015
Hirvonen, J., Karlsson, H., Kajander, J., Lepola, A., Markkula, J., Rasi, H., et al. (2008). Decreased brain serotonin 5-HT1A receptor availability in medication-naive patients with major depressive disorder: an in-vivo imaging study using PET and [carbonyl-11C]WAY-100635. International Int. Journal J. of Neuropsychopharmacology. 11, 465–476. doi: 10.1017/S1461145707008140
Ho, C. S., Zhang, M. W., and Ho, R. C. (2016). Optical topography in psychiatry: a chip off the old block or a new look beyond the mind-brain frontiers? Front. Psychiatry 7:74. doi: 10.3389/fpsyt.2016.00074
Ho, R. C. M., Chua, A. C., Tran, B. X., Choo, C. C., Husain, S. F., Vu, G. T., et al. (2018). Factors associated with the risk of developing coronary artery disease in medicated patients with major depressive disorder. International Int. Journal J. of Environmental. Research Res. and Public Health 15, :E2073. doi: 10.3390/ijerph15102073
Hsu, D. T., Langenecker, S., Kennedy, S., Zubieta, J., and Heitzeg, M. M. (2010). fMRI BOLD responses to negative stimuli in the prefrontal cortex are dependent on levels of recent negative life stress in major depressive disorder. Psychiatry Research:. Neuroimaging 183, 7202–208. doi: 10.1016/j.pscychresns.2009.12.002
Hugdahl, K., Rund, B. R., Lund, A., Asbjornsen, A., Egeland, J., Landro, N. I., et al. (2003). Attentional and executive dysfunctions in schizophrenia and depression: evidence from dichotic listening performance. Biol. Psychiatry 53, 609–616. doi: 10.1016/S0006-3223(02)01598-6
Hwang, D. R., Simpson, N. R., Montoya, J., Man, J. J., and Laruelle, M. (1999). An improved one-pot procedure for the preparation of [11C-carbonyl]-WAY100635. Nucl. Med. Biol. 26, 815–819. doi: 10.1016/S0969-8051(99)00056-6
Insel, T., Cuthbert, B., Garvey, M., Heinssen, R., Pine, D. S., Quinn, K., et al. (2010). Research domain criteria (RDoC): toward a new classification framework for research on mental disorders. Am. J. Psychiatry 167, 748–751. doi: 10.1176/appi.ajp.2010.09091379
Jacobs, N., Kenis, G., Peeters, F., Derom, C., Vlietinck, R., and van, J. (2006). Os, stress-related negative affectivity and genetically altered serotonin transporter function: evidence of synergism in shaping risk of depression. Arch. Gen. Psychiatry 63, 989–996. doi: 10.1001/archpsyc.63.9.989
Jaeger, J., Berns, S., Loftus, S., Gonzalez, C., and Czobor, P. (2007). Neurocognitive test performance predicts functional recovery from acute exacerbation leading to hospitalization in bipolar disorder 87. Bipolar. Disord. 9, 93–102. doi: 10.1111/j.1399-5618.2007.00427.x
Januel, D., Dumortier, G., Verdon, C. M., Stamatiadis, L., Saba, G., Cabaret, W., et al. (2006). A double-blind sham controlled study of right prefrontal repetitive transcranial magnetic stimulation (rTMS): therapeutic and cognitive effect in medication free unipolar depression during 4 weeks. Progress Prog. in Neuro-Psychopharmacology psychopharmacol. and Biological. Psychiatry 30, 126–130.
Jovanovic, H., Lundberg, J., Karlsson, P., Cerin, A., Saijo, T., Varrone, A., et al. (2008). Sex differences in the serotonin 1A receptor and serotonin transporter binding in the human brain measured by PET. Neuroimage 39, 1408–1419. doi: 10.1016/j.neuroimage.2007.10.016
Kalia, M. (2005). Neurobiological basis of depression: an update. Metabolism 54, 24–27. doi: 10.1016/j.metabol.2005.01.009
Kalin, N. H., Shelton, S. E., Fox, A. S., Rogers, J., Oakes, T. R., and Davidson, R. J. (2008). The serotonin transporter genotype is associated with intermediate brain phenotypes that depend on the context of eliciting stressor. Mol. Psychiatry 13, 1021–1027. doi: 10.1038/mp.2008.37
Kampf-Sherf, O., Zlotogorski, Z., Gilboa, A., Speedie, L., Lereya, J., Rosca, P., et al. (2004). Neuropsychological functioning in major depression and responsiveness to selective serotonin reuptake inhibitors antidepressants. Journal J. of Affective. Disorders Disord. 82, 453–459. doi: 10.1016/j.jad.2004.02.006
Kaufman, J., Sullivan, G. M., Yang, J., Ogden, R. T., Miller, J. M., Oquendo, M. A., et al. (2015). Quantification of the serotonin 1A receptor using PET: identification of a potential biomarker of major depression in males. Neuropsychopharmacology 40, 1692–1699. doi: 10.1038/npp.2015.15
Kautzky, A., Hahn, A., Godbersen, G. M., Gryglewski, G., James, G. M., Sigurdardottir, H. L., et al. (2018). Parcellation of the human cerebral cortex based on molecular targets in the serotonin system quantified by positron emission tomography in vivo. Cerebral Cereb. Cortex 29, 372–382. doi: 10.1093/cercor/bhy249
Kautzky, A., James, G. M., Philippe, C., Baldinger-Melich, P., Kraus, C., Kranz, G. S., et al. (2017). The influence of the rs6295 gene polymorphism on serotonin-1A receptor distribution investigated with PET in patients with major depression applying machine learning. Transl. Psychiatry 7, :e1150. doi: 10.1038/tp.2017.108
Kepe, V., Barrio, J. R., Huang, S. C., Ercoli, L., Siddarth, P., Shoghi, K., et al. (2006). Serotonin 1A receptors in the living brain of Alzheimer’s disease patients. Proc. Natl. Acad. Sci. U.S.A. 103, 702–707. doi: 10.1073/pnas.0510237103
Kling, L. R., Bessette, K. L., DelDonno, S. R., Ryan, K. A., Drevets, W. C., McInnis, M. G., et al. (2018). Cluster analysis with MOODS-SR illustrates a potential bipolar disorder risk phenotype in young adults with remitted major depressive disorder. Bipolar Disord. 20, 697–707. doi: 10.1111/bdi.12693
Kranz, G. S., Hahn, A., Kraus, C., Spies, M., Pichler, V., Jungwirth, J., et al. (2018). Probing the association between serotonin-1A autoreceptor binding and amygdala reactivity in healthy volunteers. Neuroimage 171, 1–5. doi: 10.1016/j.neuroimage.2017.12.092
Kranz, G. S., Kasper, S., and Lanzenberger, R. (2010). Reward and the serotonergic system. Neuroscience 166, 1023–1035. doi: 10.1016/j.neuroscience.2010.01.036
Kraus, C., Hahn, A., Savli, M., Kranz, G. S., Baldinger, P., Hoflich, A., et al. (2012). Serotonin-1A receptor binding is positively associated with gray matter volume — A multimodal neuroimaging study combining PET and structural MRI. Neuroimage 63, 1091–1098. doi: 10.1016/j.neuroimage.2012.07.035
Lamar, M., Cutter, W. J., Rubia, K., Brammer, M., Daly, E. M., Craig, M. C., et al. (2009). 5-HT, prefrontal function and aging: fMRI of inhibition and acute tryptophan depletion. Neurobiol. Aging 30, 1135–1146. doi: 10.1016/j.neurobiolaging.2007.09.013
Langenecker, S. A. (2001). The neuroanatomy of inhibitory control in healthy aging: Evidence from eventrelated fMRI. Available at: https://epublications.marquette.edu/dissertations/AAI3049934
Langenecker, S. A., Bieliauskas, L. A., Rapport, L. J., Zubieta, J. K., Wilde, E. A., and Berent, S. (2005). Face emotion perception and executive functioning deficits in depression. J. Clin. Exp. Neuropsychol. 27, 320–333. doi: 10.1080/13803390490490515720
Langenecker, S. A., Briceno, E. M., Hamid, N. M., and Nielson, K. A. (2007a). An evaluation of distinct volumetric and functional MRI contributions toward understanding age and task performance: a study in the basal ganglia. Brain Res. 1135, 58–68.
Langenecker, S. A., Caveney, A. F., Giordani, B., Young, E. A., Nielson, K. A., Rapport, L. J., et al. (2007b). The sensitivity and psychometric properties of a brief computer-based cognitive screening battery in a depression clinic. Psychiatry Research Res. 152, 143–154.
Langenecker, S. A., Kennedy, S. E., Guidotti, L. M., Briceno, E. M., Own, L. S., Hooven, T., et al. (2007c). Frontal and limbic activation during inhibitory control predicts treatment response in major depressive disorder. Biol. Psychiatry 62, 1272–1280.
Langenecker, S. A., Zubieta, J. K., Young, E. A., Akil, H., and Nielson, K. A. (2007d). A task to manipulate attentional load, set-shifting, and inhibitory control: convergent validity and test-retest reliability of the Parametric Go/No-Go Test. J. Clin. Exp. Neuropsychol. 29, 842–853.
Langenecker, S. A., Jacobs, R. H., and Passarotti, A. M. (2014). Current neural and behavioral dimensional constructs across mood disorders. Current Curr. Behavioral Behav. Neuroscience Neurosci. ReportsRep.∗ 1, 114–153.
Langenecker, S. A., Jenkins, L. M., Stange, J. P., Chang, Y. S., DelDonno, S. R., Bessette, K. L., et al. (2018a). Cognitive control neuroimaging measures differentiate between those with and without future recurrence of depression. Neuroimage: Clinical Clin. 20, 1001–1009. doi: 10.1016/j.nicl.2018.10.004
Langenecker, S. A., Klumpp, H., Peters, A. T., Crane, N. A., DelDonno, S. R., Bessette, K. L., et al. (2018b). Multidimensional imaging techniques for prediction of treatment response in major depressive disorder. Progress Prog. in Neuro-psychopharmacology. and Biological. Psychiatry doi: 10.1016/j.pnpbp.2018.07.001 [Epub ahead of print].
Langenecker, S. A., Lee, J., and Bieliauskas, L. A. (2009). “Neuropsychology of depression, and related mood disorders,” in Neuropsychological Assessment of Neuropsychiatric and Neuromedical Disorders, ed. I. G. K. Adams (Oxford: Oxford University Press).
Langenecker, S. A., Saunders, E. F. H., Kade, A. M., Ransom, M. T., and McInnis, M. G. (2010). Intermediate cognitive phenotypes in bipolar disorder. J. Affect. Disord. 122, 285–293. doi: 10.1016/j.jad.2009.08.018
Lee, Y., Rosenblat, J. D., Lee, J., Carmona, N. E., Subramaniapillai, M., Shekotikhina, M., et al. (2018). Efficacy of antidepressants on measures of workplace functioning in major depressive disorder: a systematic review. J. Affect. Disord. 227, 406–415. doi: 10.1016/j.jad.2017.11.003
Lesch, K. P., Bengel, D., Heils, A., Sabol, S. Z., Greenberg, B. D., Petri, S., et al. (1996). Association of anxiety-related traits with a polymorphism in the serotonin transporter gene regulatory region. Science 274, 1527–1531. doi: 10.1126/science.274.5292.1527
Levkovitz, Y., Harel, E. V., Roth, Y., Braw, Y., Most, D., Katz, L. N., et al. (2009). Deep transcranial magnetic stimulation over the prefrontal cortex: evaluation of antidepressant and cognitive effects in depressive patients. Brain Stimulation Stimul. 2, 188–200. doi: 10.1016/j.brs.2009.08.002
Lim, C. R., Barlas, J., and Ho, R. C. M. (2018). The effects of temperament on depression according to the schema model: a scoping review. International Int. Journal J. of Environmental. Research Res. and Public Health 15, :E1231. doi: 10.3390/ijerph15061231
Lima, F. B., Centeno, M. L., Costa, M. E., Reddy, A. P., Cameron, J. L., and Bethea, C. L. (2009). Stress sensitive female macaques have decreased fifth Ewing variant (Fev) and serotonin-related gene expression that is not reversed by citalopram. Neuroscience 164, 676–691. doi: 10.1016/j.neuroscience.2009.08.010
Logan, J., Fowler, J. S., Volkow, N. D., Wang, G. J., Ding, Y. S., and Alexoff, D. L. (1996). Distribution volume ratios without blood sampling from graphical analysis of PET data. J. Cereb. Blood Flow Metab. 16, 834–840. doi: 10.1097/00004647-199609000-00008
Lohoff, F. W., Hodge, R., Narasimhan, S., Nall, A., Ferroro, T. N., Mickey, B. J., et al. (2014). Functional genetic variants in the vesicular monoamine transporter 1 (VMAT1) modulate emotion processing. Mol. Psychiatry 19, 129–139. doi: 10.1038/mp.2012.193
Lopez, J. F., Chalmers, D. T., Little, K. Y., and Watson, S. J. (1998). Regulation of serotonin 1A, glucocorticoid, and mineralocorticoid receptor in rat and human hippocampus: implications for the neurobiology of depression. Biol. Psychiatry 43, 547–573. doi: 10.1016/S0006-3223(97)00484-8
Lopez, J. F., Liberzon, I., Vazquez, D. M., Young, E. A., and Watson, S. J. (1999). Serotonin 1A receptor messenger RNA regulation in the hippocampus after acute stress. Biol. Psychiatry 45, 934–937. doi: 10.1016/S0006-3223(98)00224-8
Lothe, A., Saoud, M., Bouvard, S., Redout, J., Lerond, J., and Ryvlin, P. (2012). 5-HT1A receptor binding changes in patients with major depressive disorder before and after antidepressant treatment: a pilot [18F]MPPF positron emission tomography study. Psychiatry Research:. Neuroimaging 203, 103–104. doi: 10.1016/j.pscychresns.2011.09.001
Lu, Y., Ho, C. S., Liu, X., Chua, A. N., Wang, W., McIntyre, R. S., et al. (2017). Chronic administration of fluoxetine and pro-inflammatory cytokine change in a rat model of depression. PLoS One 12:e0186700. doi: 10.1371/journal.pone.0186700
Lu, Y., Ho, C. S., McIntyre, R. S., Wang, W., and Ho, R. C. (2018a). Agomelatine-induced modulation of brain-derived neurotrophic factor (BDNF) in the rat hippocampus. Life Sci. 210, 177–184. doi: 10.1016/j.lfs.2018.09.003
Lu, Y., Ho, C. S., McIntyre, R. S., Wang, W., and Ho, R. C. (2018b). Effects of vortioxetine and fluoxetine on the level of Brain Derived Neurotrophic Factors (BDNF) in the hippocampus of chronic unpredictable mild stress-induced depressive rats. Brain Res. Bull. 142, 1–7. doi: 10.1016/j.brainresbull.2018.06.007
Mak, K. K., Kong, W. Y., Mak, A., Sharma, V. K., and Ho, R. C. (2013). Polymorphisms of the serotonin transporter gene and post-stroke depression: a meta-analysis. J. Neurol. Neurosurg. Psychiatry 84, 322–328. doi: 10.1136/jnnp-2012-303791
Marazziti, D., Marracci, S., Palego, L., Rotondo, A., Mazzanti, C., Nardi, I., et al. (1994). Localization and gene expression of serotonin 1A (5HT1A) receptors in human brain postmortem. Brain Res. 658, 55–59. doi: 10.1016/S0006-8993(09)90010-5
McKendrick, R., Parasuraman, R., and Ayaz, H. (2015). Wearable functional near infrared spectroscopy (fNIRS) and transcranial direct current stimulation (tDCS): expanding vistas for neurocognitive augmentation. Front. Syst. Neurosci. 9:27. doi: 10.3389/fnsys.2015.00027
Mickey, B. J., Ducci, F., Hodgkinson, C., Langenecker, S. A., Goldman, D., and Zubieta, J. K. (2008). Monoamine oxidase A genotype predicts human serotonin 1A receptor availability in vivo. Journal J. for Neuroscience. 28, 11354–11359. doi: 10.1523/JNEUROSCI.2391-08.2008
Milak, M. S., Pantazatos, S., Rashid, R., Zanderigo, F., DeLorenzo, C., Hesselgrave, N., et al. (2018). Higher 5-HT1A autoreceptor binding as an endophenotype for major depressive disorder identified in high risk offspring - A pilot study. Psychiatry Research. Neuroimaging 276, 15–23. doi: 10.1016/j.pscychresns.2018.04.002
Miller, J. M., Oquendo, M. A., Ogden, R. T., Mann, J. J., and Parsey, R. V. (2008). Serotonin transporter binding as a possible predictor of one-year remission in major depressive disorder. J. Psychiatr. Res. 42, 1137–1144. doi: 10.1016/j.jpsychires.2008.01.012
Moses-Kolko, E. L., Price, J. C., Thase, M. E., Meltzer, C. C., Kupfer, D. J., Mathis, C. A., et al. (2007). Measurement of 5-HT1A receptor binding in depressed adults before and after antidepressant drug treatment using positron emission tomography and [11C]WAY-100635. Synapse∗ 61, 523–530. doi: 10.1002/syn.20398
Naarding, P., Leentjens, A. F., van Kooten, F., and Verhey, F. R. (2002). Disease-specific properties of the Rating Scale for Depression in patients with stroke, Alzheimer’s dementia, and Parkinson’s disease. J. Neuropsychiatry Clin. Neurosci. 14, 329–334. doi: 10.1176/jnp.14.3.329
Natania, A. C., Alvaro, V., Masoud, K., Runa, B., Kelly, A. R., David, F. M., et al. (2018). Developing dimensional, pandiagnostic inhibitory control constructs with self-report and neuropsychological data. Assessment doi: 10.1177/1073191118754704 [Epub ahead of print].
Neumeister, A., X-Hu, Z., Luckenbaugh, D. A., Schwarz, M., Nugent, A. C., Bonne, O., et al. (2006). Differential effects of 5-HTTLPR genotypes on the behavioral and neural responses to tryptophan depletion in patients with major depression and controls. Arch. Gen. Psychiatry 63, 978–986. doi: 10.1001/archpsyc.63.9.978
Ng, A., Tam, W. W., Zhang, M. W., Ho, C. S., Husain, S. F., McIntyre, R. S., et al. (2018). IL-1beta, IL-6, TNF- alpha and CRP in elderly patients with depression or Alzheimer’s disease: systematic review and meta-analysis. Scientific Sci. Reports Rep. 8, :12050. doi: 10.1038/s41598-018-30487-6
Norgaard, M., Ganz, M., Svarer, C., Fisher, P. M., Churchill, N. W., Beliveau, V., et al. (2017). Brain networks implicated in seasonal affective disorder: a neuroimaging PET study of the serotonin transporter. Frontiers Front. in Neuroscience. 11:614. doi: 10.3389/fnins.2017.00614
Nye, J. A., Purselle, D., Plisson, C., Voll, R. J., Stehouwer, J. S., Votaw, J. R., et al. (2013). Decreased brainstem and putamen SERT binding potential in depressed suicide attempters using [11C]-zient PET imaging. Depress. Anxiety 30, 902–907. doi: 10.1002/da.22049
Olivier, B., Pattij, T., Wood, S. J., Oosting, R., Sarnyai, Z., and Toth, M. (2001). The 5-HT1A receptor knockout mouse and anxiety. Behav. Pharmacol. 12, 439–450. doi: 10.1097/00008877-200111000-00004
Oquendo, M. A., Placidi, G. P., Malone, K. M., Campbell, C., Keilp, J., Brodsky, B., et al. (2003). Positron emission tomography of regional brain metabolic responses to a serotonergic challenge and lethality of suicide attempts in major depression. Arch. Gen. Psychiatry 60, 14–22. doi: 10.1001/archpsyc.60.1.14
Parsey, R. V., Ogden, R. T., Miller, J. M., Tin, A., Hesselgrave, N., Goldstein, E., et al. (2010). Higher serotonin 1A binding in a second major depression cohort: modeling and reference region considerations. Biol. Psychiatry 68, 170–178. doi: 10.1016/j.biopsych.2010.03.023
Parsey, R. V., Oquendo, M. A., Ogden, R. T., Olvet, D. M., Simpson, N., Huang, Y. Y., et al. (2006). Altered serotonin 1A binding in major depression: a [carbonyl-C-11]WAY100635 positron emission tomography study. Biol. Psychiatry 59, 106–113. doi: 10.1016/j.biopsych.2005.06.016
Peters, A. T., Jacobs, R. H., Crane, N. A., Ryan, K. A., Weisenbach, S. L., Ajilore, O., et al. (2017). Domain-specific impairment in cognitive control among remitted youth with a history of major depression. Early Intervention Interv. in Psychiatry 11, 383–392. doi: 10.1111/eip.12253
Phillips, M. L., Drevets, W. C., Rauch, S. L., and Lane, R. (2003). Neurobiology of emotion perception I: the neural basis of normal emotion perception. Biol. Psychiatry 54, 504–514. doi: 10.1016/S0006-3223(03)00168-9
Phillips, M. L., Ladouceur, C. D., and Drevets, W. C. (2008). A neural model of voluntary and automatic emotion regulation: implications for understanding the pathophysiology and neurodevelopment of bipolar disorder. Mol. Psychiatry 13, 829–857. doi: 10.1038/mp.2008.65
Piel, J. H., Lett, T. A., Wackerhagen, C., Plichta, M. M., Mohnke, S., Grimm, O., et al. (2018). The effect of 5-HTTLPR and a serotonergic multi-marker score on amygdala, prefrontal and anterior cingulate cortex reactivity and habituation in a large, healthy fMRI cohort. Eur. Neuropsychopharmacol. 28, 415–427. doi: 10.1016/j.euroneuro.2017.12.014
Pillai, R. L. I., Malhotra, A., Rupert, D. D., Wechsler, B., Williams, J. C., Zhang, M., et al. (2018). Relations between cortical thickness, serotonin 1A receptor binding, and structural connectivity: a multimodal imaging study. Hum. Brain Mapp. 39, 1043–1055. doi: 10.1002/hbm.23903
Porter, R. J., Bourke, C., and Gallagher, P. (2007). Neuropsychological impairment in major depression: its nature, origin and clinical significance. Aust. N. Z. J. Psychiatry 41, 115–128. doi: 10.1080/00048670601109881
Quek, Y. H., Tam, W. W. S., Zhang, M. W. B., and Ho, R. C. M. (2017). Exploring the association between childhood and adolescent obesity and depression: a meta-analysis. Obesity Obes. Reviews :. an official journal of the International Association for the Study of Obesity 18, 742–754. doi: 10.1111/obr.12535
Ramage, A. G., and Villalon, C. M. (2008). 5-hydroxytryptamine and cardiovascular regulation. Trends in Pharmacological. Sciences Sci. 29, 472–481.
Richardson, J. W., Craige-Jones, C. P., Nguyen, T. H., Kung, H. F., Gardier, A. M., Dranovsky, A., et al. (2010). Serotonin-1A autoreceptors are necessary and sufficient for the normal formation of circuits underlying innate anxiety. Journal J. of Neuroscience. 31, 6008–6018. doi: 10.1523/JNEUROSCI.5836-10.2011
Rogers, M. A., Kasai, K., Koji, M., Fukuda, R., Iwanami, A., Nakagome, K., et al. (2004). Executive and prefrontal dysfunction in unipolar depression: a review of neuropsychological and imaging evidence. Neurosci. Res. 50, 1–11. doi: 10.1016/j.neures.2004.05.003
Rogers, R. D., Tunbridge, E. M., Bhagwagar, Z., Drevets, W. C., Sahakian, B. J., and Carter, C. S. (2003). Tryptophan depletion alters the decision-making of healthy volunteers through altered processing of reward cues. Neuropsychopharmacology 28, 153–162. doi: 10.1038/sj.npp.1300001
Roiser, J. P., Levy, J., Fromm, S. J., Wang, H., Hasler, G., Sahakian, B. J., et al. (2007). The effect of acute tryptophan depletion on the neural correlates of emotional processing in healthy volunteers. Neuropsychopharmacology 33, 1992–2006.
Romera, I., Pérez, V., Menchón, J. M., Polavieja, P., and Gilaberte, I. (2011). Optimal cutoff point of the Hamilton Rating Scale for Depression according to normal levels of social and occupational functioning. Psychiatry Research Res. 186, 133–137. doi: 10.1016/j.psychres.2010.06.023
Rush, A. J., Trivedi, M. H., Wisniewski, S. R., Nierenberg, A. A., Stewart, J. W., Warden, D., et al. (2006). Acute and longer-term outcomes in depressed outpatients requiring one or several treatment steps: a STAR∗D report. Am. J. Psychiatry 163, 1905–1917. doi: 10.1176/ajp.2006.163.11.1905
Ryan, K. A., Vederman, A. C., Kamali, M., Marshall, D., Weldon, A. L., McInnis, M. G., et al. (2013). Emotion perception and executive functioning predict work status in euthymic bipolar disorder. Psychiatry Res. 210, 472–478. doi: 10.1016/j.psychres.2013.06.031
Sabol, S. Z., Hu, S., and Hamer, D. (1998). A functional polymorphism in the monoamine oxidase A gene promoter. Hum. Genet. 103, 273–279. doi: 10.1007/s004390050816
Sanislow, C. A., Pine, D. S., Quinn, K. J., Kozak, M. J., Garvey, M. A., Heinssen, R. K., et al. (2010). Developing constructs for psychopathology research: research domain criteria. J. Abnorm. Psychol. 119, 631–639. doi: 10.1037/a0020909
Sarnyai, Z., Sibille, E. L., Pavlides, C., Fenster, R. J., McEwen, B. S., and Toth, M. (2000). Impaired hippocampal-dependent learning and functional abnormalities in the hippocampus in mice lacking serotonin(1A) receptors. Proc. Natl. Acad. Sci. U.S.A. 97, 14731–14736. doi: 10.1073/pnas.97.26.14731
Sawamura, J., Ishigooka, J., and Nishimura, K. (2018). Re-evaluation of the definition of remission on the 17-item Hamilton Depression Rating Scale based on recovery in health-related quality of life in an observational post-marketing study. Health and Quality. of Life Outcomes 16, :14. doi: 10.1186/s12955-018-0838-6
Schlumpf, M., Bruinink, A., Lichtensteiger, W., Cortes, R., Palacios, J. M., and Pazos, A. (1987). Beta-adrenergic binding sites in fetal rat central nervous system and pineal gland: their relation to other receptor sites. Developmental Dev. Pharmacology Pharmacol. and Therapeutics. 10, 422–435.
Selvaraj, S., Walker, C., Arnone, D., Cao, B., Faulkner, P., Cowen, P. J., et al. (2017). Effect of citalopram on emotion processing in humans: a combined 5-HT1A [11C]CUMI-101 PET and functional MRI study. Neuropsychopharmacology 43, 655–664. doi: 10.1038/npp.2017.166
Sen, S., Burmeister, M., and Ghosh, D. (2004a). Meta-analysis of the association between a serotonin transporter promoter polymorphism (5-HTTLPR) and anxiety-related personality traits. American Am. Journal J. of Medical. Genetics Genet. Part B: Neuropsychiatric Neuropsychiatr. Genetics Genet. 127B, 85–89.
Siegle, G. J., Carter, C. S., and Thase, M. E. (2006). Use of fMRI to predict recovery from unipolar depression with cognitive behavior therapy. American Am. Journal J. of Psychiatry 163, 735–738.
Smith, K. A., Morris, J. S., Friston, K. J., Cowen, P. J., and Dolan, R. J. (1999). Brain mechanisms associated with depressive relapse and associated cognitive impairment following acute tryptophan depletion. Br. J. Psychiatry 174, 525–529. doi: 10.1192/bjp.174.6.525
Snyder, H. R. (2013). Major depressive disorder is associated with broad impairments on neuropsychological measures of executive function: a meta-analysis and review. Psychological Psychol. Bulletin Bull. 139, 81–132. doi: 10.1037/a0028727
Spinelli, S., Chefer, S., Carson, R. E., Jagoda, E., Lang, L., Heilig, M., et al. (2009). Effects of early-life stress on serotonin(1A) receptors in juvenile Rhesus monkeys measured by positron emission tomography. Biol. Psychiatry 67, 1146–1153. doi: 10.1016/j.biopsych.2009.12.030
Spring, B., Hitsman, B., Pingitore, R., McChargue, D. E., Gunnarsdottir, D., Corsica, J., et al. (2007). Effect of tryptophan depletion on smokers and nonsmokers with and without history of major depression. Biol. Psychiatry 61, 70–77. doi: 10.1016/j.biopsych.2006.03.050
Stange, J. P., Bessette, K. L., Jenkins, L. M., Burkhouse, K. L., Peters, A. T., Feldhaus, C., et al. (2017). Attenuated intrinsic connectivity within cognitive control network among individuals with remitted depression: temporal stability and association with negative cognitive styles. Hum. Brain Mapp. 38, 2939–2954. doi: 10.1002/hbm.23564
Surguladze, S. A., Elkin, A., Ecker, C., Kalidindi, S., Corsico, A., Giampietro, V., et al. (2008). Genetic variation in the serotonin transporter modulates neural system-wide response to fearful faces. Genes Brain Behav. 7, 543–551. doi: 10.1111/j.1601-183X.2008.00390.x
Surtees, P. G., Wainwright, N. W. J., Willis, S. A. G., Luben-Owen, R., Day, N. E., and Flint, J. (2006). Social adversity, the serotonin transporter (5-HTTLPR) polymorphism and major depressive disorder. Biol. Psychiatry 59, 224–229. doi: 10.1016/j.biopsych.2005.07.014
Tan, H. Y., Callicott, J. H., and Weinberger, D. R. (2008). Intermediate phenotypes in schizophrenia genetics redux: is it a no brainer? Mol. Psychiatry 13, 233–238. doi: 10.1038/sj.mp.4002145
Taylor, B. P., Bruder, G. E., Stewart, J. W., McGrath, P. J., Halperin, J., Ehrlichman, H., et al. (2006). Psychomotor slowing as a predictor of fluoxetine nonresponse in depressed outpatients. Am. J. Psychiatry 163, 73–78. doi: 10.1176/appi.ajp.163.1.73
Teasdale, J. D., and Dent, J. (1987). Cognitive vulnerability to depression: an investigation of two hypotheses. Br. J. Clin. Psychol. 26( Pt 2), 113–126. doi: 10.1111/j.2044-8260.1987.tb00737.x
Trivedi, M. H., Rush, A. J., Wisniewski, S. R., Nierenberg, A. A., Warden, D., Ritz, L., et al. (2006). Evaluation of outcomes with citalopram for depression using measurement-based care in STAR∗D: implications for clinical practice. Am. J. Psychiatry 163, 28–40. doi: 10.1176/appi.ajp.163.1.28
van der Veen, F. M., Evers, E. A. T., Deutz, N. E. P., and Schmitt, J. A. J. (2007). Effects of acute tryptophan depletion on mood and facial emotion perception related brain activation and performance in healthy women with and without a family history of depression. Neuropsychopharmacology 32, 216–224. doi: 10.1038/sj.npp.1301212
Vickers, S. P., and Dourish, C. T. (2004). Serotonin receptor ligands and the treatment of obesity. Current Curr. Opinion Opin. in Investigational. Drugs (London, England : 2000) 5, 377–388.
Villafuerte, S. M., Vallabhaneni, K., Sliwerska, E., McMahon, F. J., Young, E. A., and Burmeister, M. (2009). SSRI response in depression may be influenced by SNPs in HTR1B and HTR1A. Psychiatr. Genet. 19, 281–291. doi: 10.1097/YPG.0b013e32832a506e
Votruba, K. L., and Langenecker, S. A. (2013). Age- and education-based normative data for the parametric Go/No-go task. J. Clin. Exp. Neuropsychol. 32, 132–146. doi: 10.1080/13803395.2012.758239
Webb, C. A., Dillon, D. G., Pechtel, P., Goer, F. K., Murray, L., Huys, Q. J., et al. (2016). Neural correlates of three promising endophenotypes of depression: evidence from the EMBARC study. Neuropsychopharmacology 41, 454–463. doi: 10.1038/npp.2015.165
Wessa, M., and Lois, G. (2015). Brain functional effects of psychopharmacological treatment in major depression: a focus on neural circuitry of affective processing. Current Curr. Neuropharmacology Neuropharmacol. 13, 466–479. doi: 10.2174/1570159X13666150416224801
Wijaya, C. S., Lee, J. J. Z., Husain, S. F., Ho, C. S. H., McIntyre, R. S., Tam, W. W., et al. (2018). Differentiating medicated patients suffering from major depressive disorder from healthy controls by spot urine measurement of monoamines and steroid hormones. International Int. Journal J. of Environmental. Research Res. and Public Health 15, :E865. doi: 10.3390/ijerph15050865
Wojnar, M., Brower, K. J., Strobbe, S., Ilgen, M., Matsumoto, H., Nowosad, I., et al. (2009). Association between Val66Met brain-derived neurotrophic factor (BDNF) gene polymorphism and post-treatment relapse in alcohol dependence. Alcohol. Clin. Exp. Res. 33, 693–702. doi: 10.1111/j.1530-0277.2008.00886.x
Wood, R. M., Rilling, J. K., Sanfey, A. G., Bhagwagar, Z., and Rogers, R. D. (2006). Effects of tryptophan depletion on the performance of an iterated Prisoner’s Dilemma game in healthy adults. Neuropsychopharmacology 31, 1075–1084. doi: 10.1038/sj.npp.1300932
Yasuno, F. (2004). [Hippocampal serotonin 1A receptor and memory function]. Seishin Shinkeigaku Zasshi 106, 1314–1322.
Yeo, S. N., Zainal, H., Tang, C. S., Tong, E. M., Ho, C. S., and Ho, R. C. (2017). Success/failure condition influences attribution of control, negative affect, and shame among patients with depression in Singapore. BMC Psychiatry 17:285. doi: 10.1186/s12888-017-1451-7
Yu, J., Lim, H. Y., Abdullah, F., Chan, H. M., Mahendran, R., Ho, R., et al. (2018). Directional associations between memory impairment and depressive symptoms: data from a longitudinal sample and meta-analysis. Psychol. Med. 48, 1664–1672. doi: 10.1017/S0033291717003154
Zanderigo, F., Pantazatos, S., Rubin-Falcone, H., Ogden, R. T., Chhetry, B. T., Sullivan, G., et al. (2018). In vivo relationship between serotonin 1A receptor binding and gray matter volume in the healthy brain and in major depressive disorder. Brain Structure Struct. and Function. 223, 2609–2625. doi: 10.1007/s00429-018-1649-6
Zhang, L., Guadarrama, L., Corona-Morales, A. A., Vega-Gonzalez, A., Rocha, L., and Escobar, A. (2006). Rats subjected to extended L-tryptophan restriction during early postnatal stage exhibit anxious-depressive features and structural changes. J. Neuropathol. Exp. Neurol. 65, 562–570. doi: 10.1097/00005072-200606000-00004
Keywords: positron emission tomography, intermediate cognitive phenotypes, major depressive disorder, serotonin, executive functioning, interference resolution, processing speed
Citation: Langenecker SA, Mickey BJ, Eichhammer P, Sen S, Elverman KH, Kennedy SE, Heitzeg MM, Ribeiro SM, Love TM, Hsu DT, Koeppe RA, Watson SJ, Akil H, Goldman D, Burmeister M and Zubieta J-K (2019) Cognitive Control as a 5-HT1A-Based Domain That Is Disrupted in Major Depressive Disorder. Front. Psychol. 10:691. doi: 10.3389/fpsyg.2019.00691
Received: 07 December 2018; Accepted: 12 March 2019;
Published: 29 March 2019.
Edited by:
Roger Ho, National University of Singapore, SingaporeReviewed by:
Cyrus S. H. Ho, National University Health System, SingaporeYanxia Lu, Agency for Science, Technology and Research (A∗STAR), Singapore
Agnieszka Anna Olszewska-Guizzo, National University of Singapore, Singapore
Syeda Fabeha Husain contributed to the review of Agnieszka Anna Olszewska-Guizzo
Copyright © 2019 Langenecker, Mickey, Eichhammer, Sen, Elverman, Kennedy, Heitzeg, Ribeiro, Love, Hsu, Koeppe, Watson, Akil, Goldman, Burmeister and Zubieta. This is an open-access article distributed under the terms of the Creative Commons Attribution License (CC BY). The use, distribution or reproduction in other forums is permitted, provided the original author(s) and the copyright owner(s) are credited and that the original publication in this journal is cited, in accordance with accepted academic practice. No use, distribution or reproduction is permitted which does not comply with these terms.
*Correspondence: Scott A. Langenecker, cy5sYW5nZW5lY2tlckBoc2MudXRhaC5lZHU=
†Present address: Scott A. Langenecker, Brian J. Mickey, Tiffany M. Love, and Jon-Kar Zubieta, Department of Psychiatry, University of Utah, Salt Lake City, UT, United States