- 1Department of Psychiatry and Psychotherapy, St. Hedwig Hospital, Charité – Universitätsmedizin Berlin, Berlin, Germany
- 2Department of Biological Psychology, Christian-Albrechts-University Kiel, Kiel, Germany
- 3Physikalisch-Technische Bundesanstalt, Berlin, Germany
- 4Center for Stroke Research Berlin, Charité – Universitätsmedizin Berlin, Berlin, Germany
- 5Department of Psychiatry and Psychotherapy, University Medical Center Hamburg-Eppendorf, Hamburg, Germany
Clinical studies suggest aberrant neurotransmitter concentrations in the brains of patients with schizophrenia (SCZ). Numerous studies have indicated deviant glutamate concentrations in SCZ, although the findings are inconsistent. Moreover, alterations in glutamate concentrations could be linked to personality traits in SCZ. Here, we examined the relationships between personality dimensions and glutamate concentrations in a voxel encompassing the occipital cortex (OCC) and another voxel encompassing the left superior temporal sulcus (STS). We used proton magnetic resonance spectroscopy to examine glutamate concentrations in the OCC and the STS in 19 SCZ and 21 non-psychiatric healthy control (HC) participants. Personality dimensions neuroticism, extraversion, openness, agreeableness and conscientiousness were assessed using the NEO-FFI questionnaire. SCZ compared to HC showed higher glutamate concentrations in the STS, reduced extraversion scores, and enhanced neuroticism scores. No group differences were observed for the other personality traits and for glutamate concentrations in the OCC. For the SCZ group, glutamate concentrations in STS were negatively correlated with the neuroticism scores [r = -0.537, p = 0.018] but this was not found in HC [r(19) = 0.011, p = 0.962]. No other significant correlations were found. Our study showed an inverse relationship between glutamate concentrations in the STS and neuroticism scores in SCZ. Elevated glutamate in the STS might serve as a compensatory mechanism that enables patients with enhanced concentrations to control and prevent the expression of neuroticism.
Introduction
A large proportion of people diagnosed with schizophrenia (SCZ) show a chronic progression of positive and negative symptoms, cognitive impairments, as well as social dysfunction (Millan et al., 2016). Aberrant neurotransmission involving the neurotransmitter glutamate likely contributes to the development and progression of this disorder (Javitt and Zukin, 1991; Coyle, 1996). The glutamate hypothesis posits changes in glutamatergic synapses and alterations in glutamatergic pathways in SCZ (Coyle, 2006; Javitt, 2010; Kantrowitz and Javitt, 2010; Poels et al., 2014a,b). In support of this hypothesis, various studies have suggested a link between glutamate and psychosis (Egerton et al., 2012; Wijtenburg et al., 2015; Treen et al., 2016).
Glutamatergic synapses are found in the entire brain. However, alterations in glutamate-mediated neurotransmission and glutamate concentrations in SCZ seem to be confined to specific brain regions (Gallinat et al., 2016). To date, only few studies with SCZ patients have examined glutamatergic neurotransmission in the temporal lobe (Atagün et al., 2015; Hugdahl et al., 2015). For example, Hugdahl et al. (2015) reported decreased glutamate levels in the temporal lobe in SCZ compared to HC. However, the authors also found increased glutamate concentrations in a subgroup of SCZ patients with auditory hallucinations. In addition, another study has measured brain metabolites in the left superior temporal cortex in small participant samples but did not find differences in glutamate concentrations between SCZ and HC (Atagün et al., 2015). The superior temporal cortex is crucially involved in the processing and integration of information from multiple sensory modalities (Maier et al., 2008; Keil and Senkowski, 2018). Moreover, processing in the STS has an impact on perception (Balz et al., 2016). Hence, it is possible that interindividual differences at the synaptic and systemic levels in the superior temporal cortex also shape personality traits (Lu et al., 2014; Ikeda et al., 2015; Tseng et al., 2015; Li et al., 2017; Riccelli et al., 2017). For example, one study in healthy adults revealed a positive correlation between neuroticism scores and cortical thickness in the STS (Riccelli et al., 2017). In addition, the study showed negative correlations between both neuroticism and extraversion scores with the STS surface area and cortical volume. Another study using EEG reported an increase in functional connectivity between the insular cortex and STS during the processing of unpleasant emotions (Ikeda et al., 2015). Moreover, the study revealed that neural responses to unpleasant emotional stimuli in the inferior temporal gyrus were higher in participants with high neuroticism compared to participants with low neuroticism scores. Thus, differences in the STS architecture as well as in information processing in this area seem to be related to personality traits.
Studies investigating the Big Five personality traits in SCZ have consistently shown enhanced neuroticism and diminished extraversion scores (Berenbaum and Fujita, 1994; Gurrera et al., 2000; Camisa et al., 2005; Boyette et al., 2013; Ohi et al., 2016). Personality traits in SCZ relate to clinical symptoms, cognitive deficits, and impaired social function (Herrán et al., 2006; Kirihara et al., 2012; Gurrera et al., 2014; Compton et al., 2015). In addition, personality traits affect both the onset and the progression of SCZ (Van Os and Jones, 2001; Gleeson et al., 2005; Lönnqvist et al., 2009). Furthermore, post-mortem studies have indicated that glutamatergic neurobiological mechanisms might contribute to the susceptibility to neuroticism (Hettema et al., 2006; Ohi et al., 2017). Hence, it may be that alterations in the glutamatergic system relate to the SCZ psychopathology, as expressed in altered personality traits.
Thus far, no study has examined the link between glutamate concentration and personality traits in the superior temporal lobe. Recent magnetic resonance spectroscopy (MRS) studies have suggested both increased and decreased glutamate concentrations in the occipital lobe of SCZ patients (Chang et al., 2007; Thakkar et al., 2017). However, others studies did not find such alterations (Goto et al., 2012; Marsman et al., 2014). While higher and lower glutamate concentrations have been frequently reported in various brain areas of patients compared to control participants (Marsman et al., 2013; Poels et al., 2014a; Wijtenburg et al., 2015; Merritt et al., 2016), the functional significance of these alterations for the SCZ pathology are not well understood. In this study we examined glutamate concentrations in the OCC and the left STS of SCZ and healthy control (HC) participants. We used proton MRS to examine glutamate concentrations. Additionally, we assessed the Big Five personality dimensions using the NEO-FFI questionnaire (Costa and McCrae, 1992). We computed correlations between glutamate concentrations and those personality dimensions that differed between groups. This was carried out separately for each voxel and group. Since findings in the literature are relatively inconsistent, we hesitated deriving specific hypotheses regarding alterations in glutamate concentration in SCZ for the two obtained voxels. For the personality traits, we expected higher neuroticism scores and lower extraversion scores in SCZ than in HC. Finally, we explored whether alterations in glutamate concentrations serve as a marker for personality traits, especially neuroticism and extraversion, in SCZ and HC.
Materials and Methods
Participants
Twenty-three patients with the diagnosis schizophrenia were recruited from outpatient units of the Charité – Universitätsmedizin Berlin. The ICD-10 criteria, as used in Germany to classify psychiatric disorders, were applied. In addition, 23 healthy control participants matched for age, education, gender, and handedness, were recruited for the study. As it was not possible to recruit an equal amount of male and female SCZ patients that fitted our inclusion criteria, we made sure to maintain the same ratio for the HC group. The study was conducted in accordance with the Declaration of Helsinki and approved by the ethics committee of the Charité – Universitätsmedizin Berlin. All participants were additionally screened for mental disorders with the German version of the Structural Clinical Interview for DSM-IV-TR (SCID; First et al., 2002). This was done to assure that there were no co-morbidities in the patient group and no psychiatric disorders in the control group. Three SCZ elected not to complete the MRS scan. Furthermore, MRS data could not be obtained from one SCZ and two HC due to scanner malfunction. These participants were excluded from further analysis. The clinical diagnosis was assessed by a senior psychiatrist at the recruiting institution. All participants provided written informed consent, had normal hearing, normal or corrected to normal vision, and no other neurological disorders, alcohol or substance abuse. A random sample of 40% of all participants underwent a multi-drug screening test. Severity of symptoms in SCZ was assessed with the Positive and Negative Syndrome Scale (PANSS; Kay et al., 1987). To test cognitive performance, the Brief Assessment of Cognition in Schizophrenia (BACS; Keefe et al., 2004) was administered. The five major personality dimensions (Neuroticism; Extraversion; Openness; Agreeableness; Conscientiousness) were assessed with the NEO-FFI questionnaire (Costa and McCrae, 1989, 1992). Table 1 provides an overview on demographic data, cognitive performance, and clinical scores of the study participants.
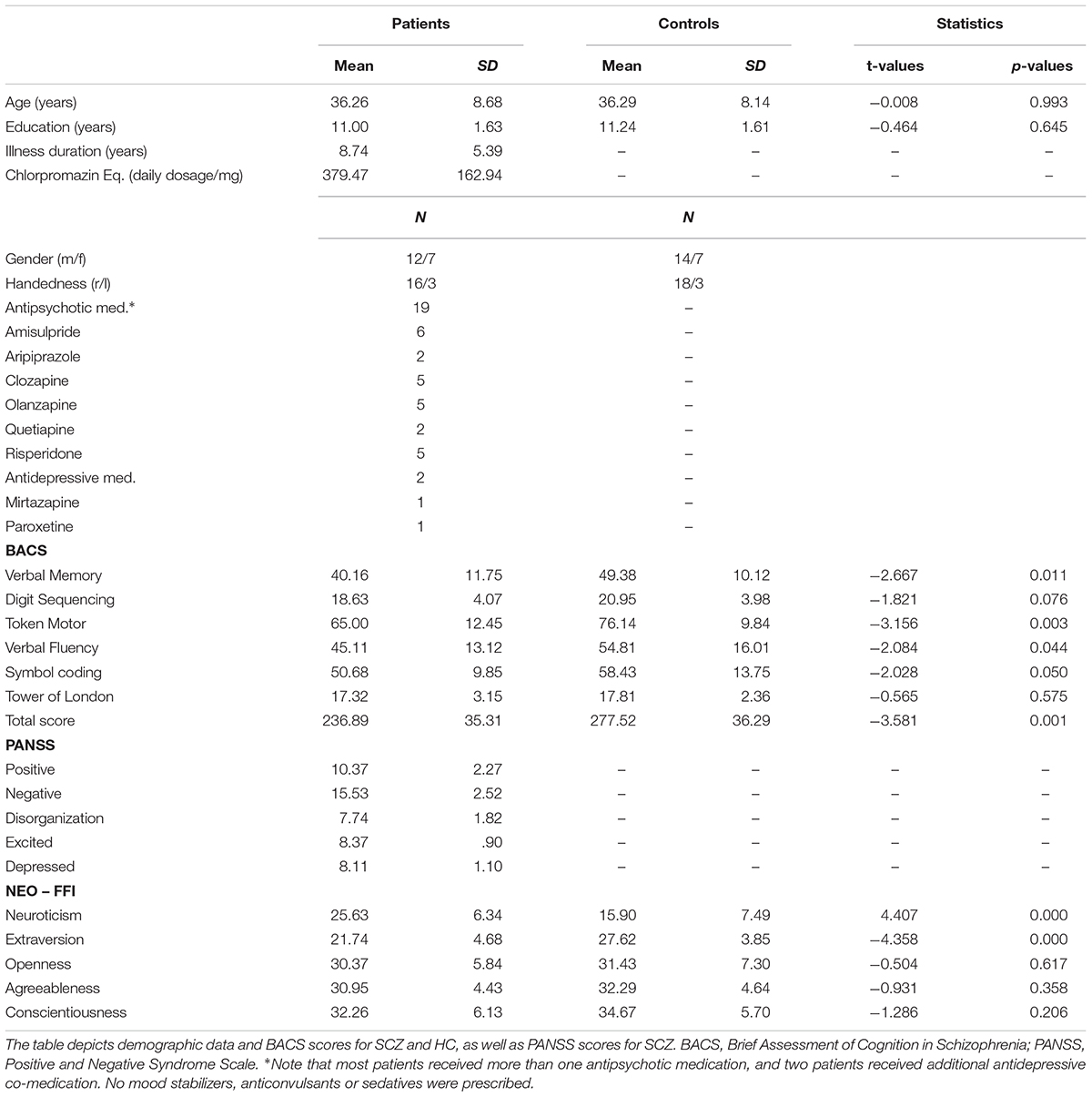
TABLE 1. Demographic data, positive and negative syndromes, and cognitive scores in the study participants.
MR Methods
MR images and spectra were collected on a 3 T Verio (Siemens Healthcare, Erlangen, Germany). Anatomical images were acquired using a three-dimensional T1-weighted magnetization prepared gradient-echo sequence (MPRAGE) with an isotropic resolution of 1.0 mm, a repetition time (TR) of 2.3 s, an echo time (TE) of 3.03 ms, an inversion time (TI) of 900 ms, and a flip angle of 9°. The volume of interest (VOI = 20 mm × 30 mm × 20 mm) for single voxel MRS, encompassing the left STS, was positioned below the upper bank of the temporal cortex (Figure 1A). The second VOI (30 mm × 20 mm × 20 mm) was positioned centrally, encompassing the occipital cortex (OCC) (Figure 1B). The transmitter radiofrequency (RF) voltage was calibrated for the individual VOI, followed by adjustment of first- and second-order shims using FAST(EST)MAP (Gruetter and Boesch, 1992; Gruetter and Tkac, 2000). Spin echo full intensity acquired localized (SPECIAL) (Mlynárik et al., 2006) spectra were acquired. The short echo time of the SPECIAL sequence enables the determination of a large number of metabolites and yields a high precision for the detection of glutamate (Mekle et al., 2009; Schubert et al., 2017). The sequence was used with water suppression by VAPOR (variable power radio frequency pulses with optimized relaxation delays; Tkac et al., 1999), and six outer volume suppression slices placed around the spectroscopic voxel to saturate outside spins. For each metabolite spectrum the number of acquisitions (NA) was 256 with TR = 3 s and TE = 8.5 ms. Following this scan, a spectrum without water suppression was recorded (NA = 8).
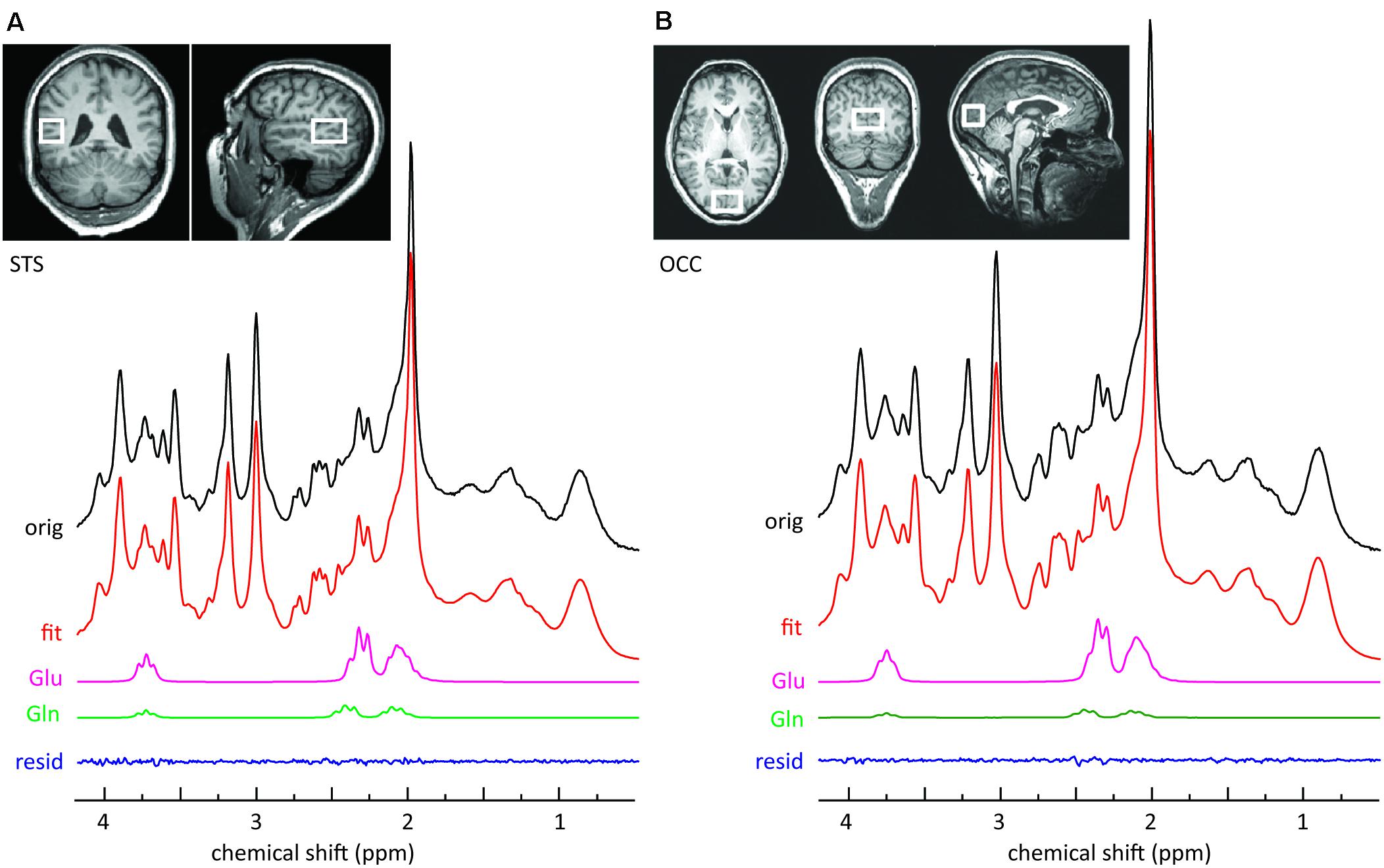
FIGURE 1. Sample MRS spectra from the left STS voxel (A) and the OCC voxel (B). The upper panels illustrate the location of the voxel on T1-weighted images. The top row of each panel represents the original SPECIAL spectrum. Also shown are the overall fits and the fitted components of interest. The small residuals reflect the high quality of the fits.
If the water line width calculated from a fit to the water spectrum after shimming was greater than or equal to 9.5 Hz, all corresponding MRS data were excluded from further analysis. The mean water line width (±standard deviation) of the remaining dataset (n = 40) was determined to be 8.5 (±1.3) Hz in STS and 7.6 (±1.1) in OCC. Linewidth did not significantly differ between SCZ and HC. For the analysis of the SPECIAL data, the spectra were corrected for frequency drift during acquisition and analyzed using LCModel (Provencher, 1993) with a simulated basis set containing 20 metabolites.
The unsuppressed water spectrum was used for eddy current correction and referencing the metabolite spectrum to the internal water concentration. Cramér-Rao lower bounds of the LCModel fits were 4.3 ± 0.7% for glutamate in the STS and 4.6 ± 1.3% for glutamate in the OCC (n = 40). Glutamate amplitudes were corrected for relaxation using T1 and T2 values determined at 3T (Schubert et al., 2004; Mullins et al., 2008). To correct the in vivo concentrations for the amount of cerebrospinal fluid (CSF) in the selected VOI, segmentation of the T1-weighted images was performed using statistical parametric mapping (SPM8; Litvak et al., 2011). Pixels in the VOI were classified according to their probability calculated by SPM8 to belong to one of the tissue types: CSF, gray matter, or white matter. The average glutamate concentrations calculated in this manner were 8.64 ± 1.27 mmol/l in STS and 7.68 ± 1.02 mmol/l in OCC.
Data Analysis
For SCZ and HC, the NEO-FFI scores were evaluated for each personality dimension. In a first step, we compared glutamate concentrations in the STS and OCC between SCZ and HC. In this comparison we used a Bonferroni-corrected alpha-level of 0.025 (0.05/2). In the next step personality dimensions between both groups were compared using two-sample t-tests. Here, a Bonferroni-corrected alpha-level of 0.01 (0.05/5) was used. Significant group differences were followed up by analyses of Pearson correlation between glutamate concentrations and NEO-FFI scores. In this comparison we used a Bonferroni-corrected alpha-level of 0.025 (0.05/2) within SCZ and HC. Fisher Z-values were calculated to compare correlation coefficients between groups. In addition, if the analysis revealed significant correlations between glutamate concentrations and NEO-FFI scores, we investigated whether they were mediated by the factors age and gender in the control group and by the factors age, gender and medication in the patients group. To statistically control for these factors partial correlation analyses were conducted. In patients the medication dosage was converted to chlorpromazine equivalent (CPZE) level (Gardner et al., 2010). For exploratory purposes, in the SCZ group, glutamate values were also related to psychopathology scores (PANSS) using Pearson correlations.
Results
SCZ showed higher glutamate concentrations in STS compared to HC [M = 9.193, SD = 1.106 vs. M = 8.151, SD = 1.231, t(38) = 2.806, p = 0.008; Figure 2A]. However, no significant differences were observed for occipital glutamate concentrations [M = 7.705, SD = 1.255 vs. M = 7.652, SD = 7.777, t(38) = 0.160, p = 0.874]. Across groups, there were no differences in glutamate concentrations between female and male participants [female (n = 14): M = 21.21, SD = 8.496 vs. male (n = 26): M = 20.15, SD = 8.582, t(38) = -0.374, p = 0.710]. The analysis of personality traits revealed enhanced neuroticism scores [M = 25.63, SD = 6.344 vs. M = 15.90, SD = 7.489, t(38) = 4.407, p < 0.001] and reduced extraversion scores [M = 21.74, SD = 4.677 vs. M = 27.62, SD = 3.853, t(38) = -4.358, p < 0.001] in SCZ compared to HC (Figure 2B). No significant group differences were found for the other personality traits [Openness: M = 30.37, SD = 5.842 vs. M = 31.43, SD = 7.298, t(38) = -0.504, p = 0.617; Agreeableness: M = 30.95, SD = 4.428 vs. M = 32.29, SD = 4.638, t(38) = -0.931, p = 0.358; Conscientiousness: M = 32.26, SD = 6.127 vs. M = 34.67, SD = 5.695, t(38) = -1.286, p = 0.206]. Across groups, no significant differences in neuroticism and extraversion scores were found between female and male participants [neuroticism: female (n = 14): M = 21.21, SD = 8.496 vs. male (n = 26): M = 20.15, SD = 8.582, t(38) = -0.374, p = 0.710; extraversion: female (n = 14): M = 25.29, SD = 5.239 vs. male (n = 26): M = 24.58, SD = 5.194, t(38) = -0.410, p = 0.684].
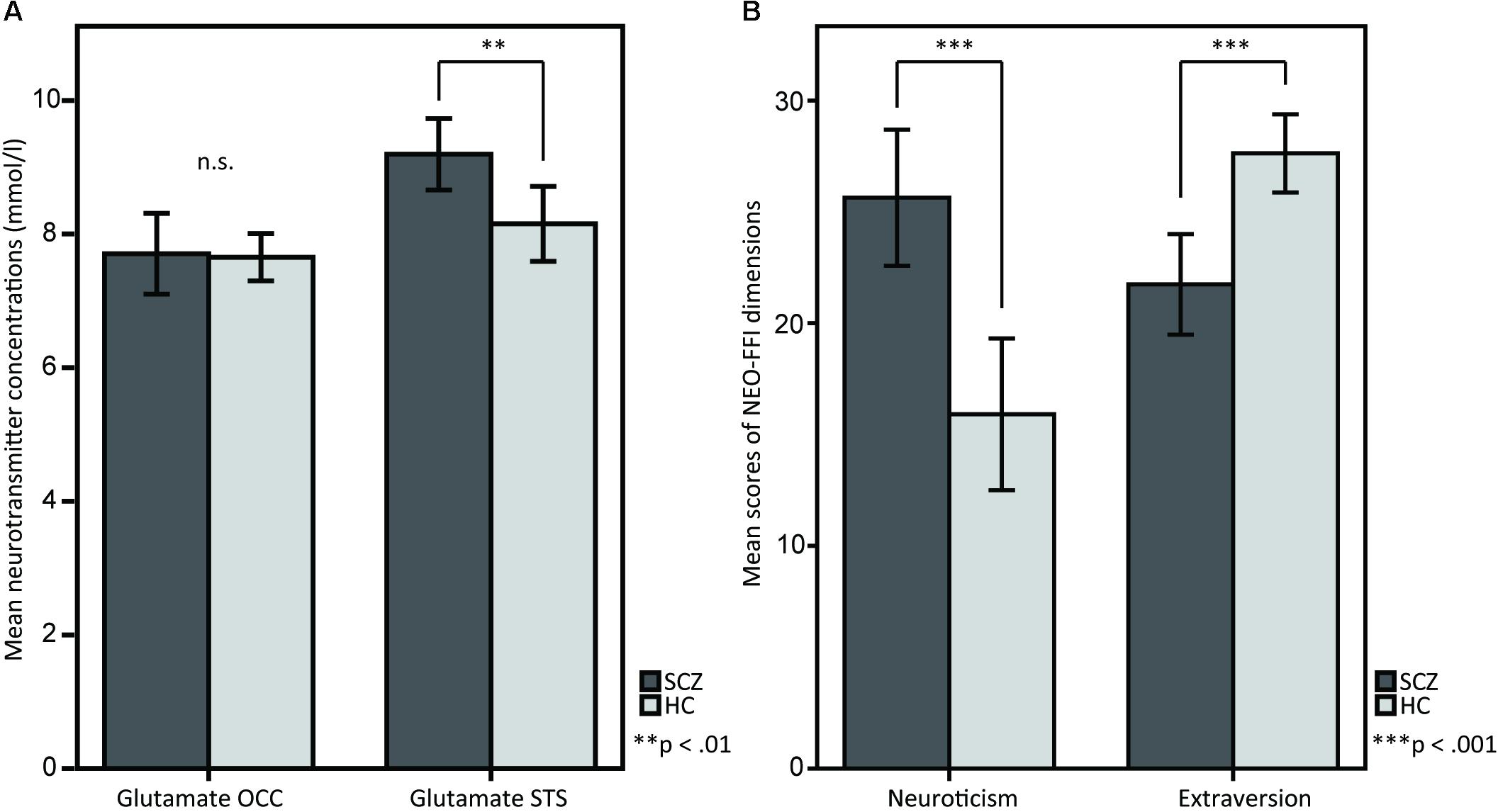
FIGURE 2. T-tests between SCZ and HC for (A) glutamate in the OCC and glutamate in the STS, and for (B) the NEO-FFI dimensions neuroticism and extraversion. (A) The barplots show OCC and STS mean glutamate concentrations in SCZ and HC. Glutamate concentrations in the STS are elevated in SCZ compared to HC, whereas OCC glutamate concentrations do not show any differences. (B) The barplots show differences between SCZ and HC in the personality dimensions neuroticism and extraversion, measured by the NEO-FFI.
In the next step of the analysis, personality traits that showed significant group differences, i.e., Extraversion and Neuroticism, were correlated with glutamate concentrations in the STS and OCC. This was done separately for the SCZ and HC groups. Within the SCZ group, the glutamate concentrations correlated negatively with the neuroticism scores [r(17) = -0.537, p = 0.018] (Figure 3A). Higher glutamate concentrations were related to lower neuroticism scores. However, no such correlation was found in HC [r(19) = 0.011, p = 0.962]. Importantly, the correlation coefficients for the relationships between neuroticism scores and glutamate concentrations in the STS differed significantly between groups [Fisher Z = -1.714, p = 0.043]. Next, we conducted a partial correlation analysis with the control variables age, gender and medication, i.e., CPZE level, between glutamate concentration in the STS and neuroticism scores in SCZ. The analysis revealed a significant relationship [r(14) = -0.518, p = 0.040], demonstrating that age, gender, and medication did not substantially mediate the negative correlation between glutamate concentration in the STS and neuroticism scores in SCZ.
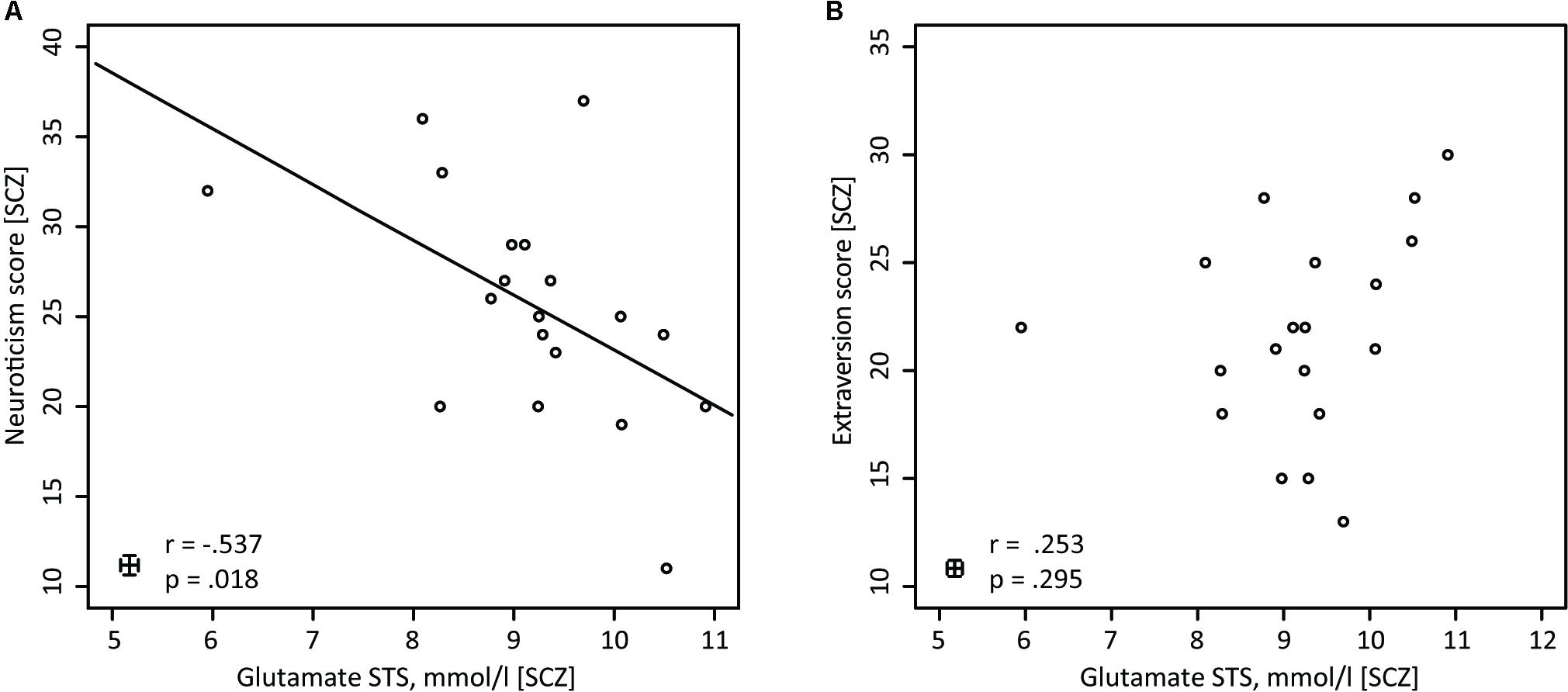
FIGURE 3. Pearson correlations for SCZ between glutamate (STS) and neuroticism, and between glutamate (STS) and extraversion. (A) In SCZ glutamate concentrations in the STS are negatively correlated with neuroticism (n = 19). (B) In SCZ glutamate concentrations in the STS do not show any correlations with the NEO-FFI extraversion score (n = 19).
Further analyses revealed that for both groups, there were no significant correlations between glutamate concentrations in the STS and extraversion scores [SCZ: r(17) = 0.253, p = 0.295 (Figure 3B); HC: r(19) = 0.394, p = 0.077]. In addition, the correlation analysis between glutamate concentrations in the occipital lobe and neuroticism scores [SCZ: r(17) = -0.412, p = 0.079; HC: r(19) = 0.136, p = 0.557], as well as between glutamate concentration in the occipital lobe and extraversion scores [SCZ: r(17) = -0.014, p = 0.954; HC: r(19) = -0.102, p = 0.659], did not reveal any significant relationships.
No relationship between glutamate and schizophrenia symptoms, as obtained through the PANSS, was found (for all scales p > 0.05). Moreover, there was no relationship between glutamate concentration and cognitive capacity, measured by the BACS (for all scales p > 0.05).
Discussion
In this study we examined glutamate concentrations in the STS and OCC in SCZ and HC. We observed that glutamate concentrations in the STS were significantly higher for SCZ than HC, while there were no group differences in glutamate concentrations in the OCC. Additionally, we obtained measures of the Big Five personality traits. In line with previous research, we found increased neuroticism scores and reduced extraversion scores in SCZ compared to HC. Interestingly, within SCZ glutamate levels in the STS correlated negatively with neuroticism scores.
Patients Exhibit Higher STS Glutamate Concentrations Than Healthy Controls
SCZ compared to HC showed higher glutamate concentrations in the STS, which is a key association area in the human brain. However, we did not find group differences in glutamate concentrations in the visual cortex. The lack of glutamate concentration differences between SCZ and HC in the visual cortex corresponds with a study by Marsman et al. (2014), who examined glutamate concentrations in the parieto-occipital cortex using a 7 T MRI scanner. The study revealed no significant group differences in glutamate concentrations in the parieto-occipital cortex. Previous studies measuring glutamate showed inconsistent results: Depending on the selected brain area, some studies did not find glutamatergic aberrations in SCZ (Marsman et al., 2013; Poels et al., 2014a,b; Wijtenburg et al., 2015; Merritt et al., 2016), while others did find both increased and decreased glutamate concentrations in SCZ (Marsman et al., 2013; Poels et al., 2014a,b; Wijtenburg et al., 2015; Merritt et al., 2016). In the STS, however, clear results have been missing so far. To our knowledge, only one previous study has examined glutamate concentrations in SCZ in the left STS in a small study sample (Atagün et al., 2015). The study did not reveal significant differences in glutamate concentrations between groups, perhaps due to a lack of statistical power. The results of the current study show, for the first time, increased glutamate concentrations in the left STS in SCZ compared to HC.
Recent research indicates that alterations in glutamatergic neurotransmission in SCZ do not start during the course of the disorder but are already prevalent before the visible manifestation of symptoms (Millan et al., 2016). For example, Egerton et al. (2012) suggested that genetic and environmental risk factors for psychosis can interact with glutamatergic neurotransmission and can consequently lead to the onset of SCZ. Furthermore, several studies have suggested that individuals at high-risk for developing a psychotic disorder show alterations in glutamate concentrations with lower glutamate concentrations in thalamic and higher glutamate concentration in medial frontal regions prior to the onset of SCZ (Tibbo et al., 2004; Stone et al., 2009; Fusar-Poli et al., 2011; Wijtenburg et al., 2015).
Generally, glutamate levels, e.g., as measured in thalamic regions and the anterior cingulate cortex, seem to be elevated in early stages of the psychosis as well as unmedicated first episode SCZ patients (Théberge et al., 2002; Egerton et al., 2012; Marsman et al., 2013; Wijtenburg et al., 2015). In contrast, the results in chronic, medicated patients are less clear (Egerton and Stone, 2012; Marsman et al., 2013; Poels et al., 2014a,b; Wijtenburg et al., 2015). Some studies have suggested that glutamate concentrations in various regions increase with age, or medication intake (Wijtenburg et al., 2015). However, in the present study we did not find an influence of age, or medication on glutamate concentrations in the left STS. The meta-analysis by Merritt et al. (2016) could also not confirm a relationship between glutamate concentration and age, antipsychotic treatment or symptom severity. Hence, our results suggest that glutamate concentrations do not seem to be directly related to those factors. Moreover, our study provides evidence that elevated glutamate concentrations in the STS are prevalent in SCZ even in medicated chronic patients.
Patients Rate Themselves Higher in Neuroticism and Lower in Extraversion Than Healthy Controls
In line with our hypothesis, SCZ patients had higher neuroticism values and lower extraversion values than HC. Thus, our findings are in line with previous studies about personality trait differences in SCZ (Berenbaum and Fujita, 1994; Gurrera et al., 2000; Camisa et al., 2005; Boyette et al., 2013; Ohi et al., 2016). A number of studies have suggested that personality differences between SCZ and healthy individuals are present even before the onset of psychotic symptoms (Keshavan et al., 2005; Fryers and Brugha, 2013; Ohi et al., 2016). Furthermore, high neuroticism scores and low extraversion scores have been associated with an increased risk of developing SCZ (Van Os and Jones, 2001; Krabbendam et al., 2002; Goodwin et al., 2003; Gleeson et al., 2005; Krug et al., 2008; Lönnqvist et al., 2009; Boyette et al., 2013, 2015; Ohi et al., 2016). High neuroticism trait scores may reflect a vulnerability to distress, which could contribute to the development of SCZ (Nuechterlein et al., 1994; Boyette et al., 2013). Recently, Ridgewell et al. (2017) discovered a relationship between neuroticism and quality of life in SCZ. If neuroticism is constantly higher in individuals who are at high risk for developing SCZ, and if elevated neuroticism scores shape general health and the onset of SCZ, then elevated neuroticism trait scores could be a predictive marker for individuals who are at risk of developing SCZ or progressing to a psychotic phase. Future research should examine the predictive value of enhanced neuroticism scores for the development of SCZ.
Neuroticism and extraversion might be a manifestation of short-term symptoms that occur in SCZ. To test this assumption, Boyette et al. (2015) investigated the 3-year stability of the Big Five personality traits and found that transient psychotic symptoms and psychotic relapse did not substantially affect personality traits in patients with psychotic disorders. In addition, Kentros et al. (1997) failed to show a relationship between positive symptoms and personality trait scores in SCZ, although a slight influence of negative symptoms on personality traits was observed. Thus, these studies suggest that short-term symptoms in SCZ have a relatively modest impact on personality traits in SCZ.
When investigating personality traits in SCZ, one might argue that patients are unable to provide a reliable self-assessment on their personality. However, some studies have addressed this issue and found that SCZ patients have the same ability to rate their own personality as compared with healthy individuals (Bell et al., 2007). Bell et al. (2007) investigated the validity of self-report questionnaires for SCZ patients and found that standardized personality questionnaires, such as the NEO-FFI are valid even for patients with poor insight. Furthermore, Suslow et al. (2014) found that not only the explicit but also the implicit self-representation in SCZ is different from HC in the direction of higher neuroticism and lower extraversion in SCZ. Hence, we conclude that the personality traits measured in the current study provide a reliable measure of the Big Five personality dimensions in both study groups. Personality trait scores are relatively stable over time and do not seem to be related to the severity of the illness or to the current symptomatology of acute psychosis symptoms. Therefore, we argue that obtained alterations in personality trait scores, i.e., elevated neuroticism and diminished extraversion in SCZ reflect a trait rather than a state effect (Kentros et al., 1997; Gurrera et al., 2000; Beauchamp et al., 2006, 2011; Boyette et al., 2015).
A Negative Correlation Between STS Glutamate Concentrations and Neuroticism Can Be Found in Schizophrenic Patients
In SCZ neuroticism scores, but not extraversion scores, correlated negatively with STS glutamate concentrations. The higher the STS glutamate concentration in SCZ, the lower the neuroticism scores were. Interestingly, glutamate concentrations in the STS were linked to neuroticism in SCZ, but not in HC. HC did not show significant correlations between STS glutamate and neuroticism or extraversion.
So far, only a few studies have discovered negative correlations between glutamate concentrations and symptoms of psychiatric disorders in SCZ. For example, Egerton et al. (2014), who examined individuals with ultra high risk of psychosis, found a negative relationship between thalamic glutamate concentration and the severity of positive symptoms – particularly the severity of abnormal thought content and the severity of perceptual abnormalities. Furthermore, longitudinal data in that study revealed a correlation between lower thalamic glutamate concentrations and successive worsening of symptoms over time. However, our study did not reveal any correlations of glutamate with PANSS or BACS ratings, which indicates that acute psychopathology is independent from glutamate concentration levels in the STS and the OCC. Notably, other studies also reported a lack of any significant relationship between acute psychotic symptoms and glutamate concentrations, e.g., in the anterior cingulate cortex or dorsolateral prefrontal cortex (Chiu et al., 2017; Ćurčić-Blake et al., 2017).
The observed effects in the current study were relatively weak and therefore require replication with a larger sample. Moreover, it is not clear if an enhancement in glutamate concentrations actually contributes to enhanced neural activity of glutamatergic neurons. Nevertheless, we could speculate that higher STS glutamate concentrations might be a compensatory mechanism that protects individuals with SCZ from otherwise higher neuroticism traits. Studies suggested that high neuroticism scores might have a negative impact on functioning and quality of life (Ridgewell et al., 2017). An elevated glutamate concentration in the STS in SCZ might therefore compensate the otherwise distinct personality differences and lead to better overall functioning. However, this assumption requires further testing.
Glutamate is the primary excitatory neurotransmitter in the human brain, hence, constantly elevated glutamate concentrations could lead to structural abnormalities and dangerous neurotoxic effects in the SCZ brain (Marsman et al., 2013; Plitman et al., 2016). Long-term studies are necessary to monitor changes in glutamatergic neurotransmission over time in SCZ and to check for damages caused by glutamatergic aberrations. If SCZ is linked to aberrant glutamatergic neurotransmission, then medication that can affect glutamatergic neurotransmission may be a promising psychopharmacological intervention target. The observation that glutamate is linked to and affects personality could be used as a treatment option to reduce symptoms of psychotic and other psychiatric disorders (Carlsson, 2001; Rosenberg et al., 2004; Hoerst et al., 2010; Neill and Frodl, 2012). However, since SCZ is a very heterogeneous disorder, it is possible that different types of treatment will be necessary to help to diminish the diverse symptoms in this patient group (Chavez-Noriega et al., 2002; Howes and Kapur, 2014; Poels et al., 2014b; Beck et al., 2016). Promising results supporting this therapeutic avenue propose that SCZ who do not respond to dopaminergic medication reveal normal dopaminergic levels, but altered glutamate levels (Demjaha et al., 2014). Consequently, drugs that target the glutamatergic system could help in individuals who do not respond to standard medication in SCZ, which mostly targets the dopaminergic system.
Conclusion
Our study revealed that glutamate concentration in the STS and neuroticism in SCZ are linked. Patients with SCZ who exhibit higher glutamate concentrations in the STS show lower neuroticism and vice versa. We propose that glutamate could serve as a compensatory mechanism in the SCZ brain. Specifically, enhanced glutamate concentrations in the association cortex might enable patients to control and subsequently prevent the expression of neuroticism. Considering that modulations in neurotransmission also affect personality in SCZ, monitoring and moderating the glutamatergic concentration might help to better understand SCZ psychopathology. Consequently, addressing the glutamatergic dysfunction might help individuals with schizophrenia to overcome some of the limitations resulting from their higher neuroticism trait scores.
Author Contributions
JB conceived the experiment, recorded data, analyzed data, discussed data, and wrote the manuscript. YRR conceived the experiment, recorded data, analyzed data, and wrote the manuscript. JK conceived the experiment, analyzed data, discussed data, and wrote the manuscript. FS and RM recorded data, analyzed data, and wrote the manuscript. BI analyzed data and wrote the manuscript. CM discussed the data and wrote the manuscript. JG conceived the experiment and wrote the manuscript. DS conceived the experiment, discussed data, and wrote the manuscript.
Funding
This work was supported by grants from the German Research Foundation (DFG) (GA707/6-1 to JG, KE1828/2-1 to JK, and SE1859/4-1 to DS) and the European Union (ERC-2010-StG-20091209 to DS). We acknowledge support from the DFG and the Open Access Publication Fund of Charité – Universitätsmedizin Berlin.
Conflict of Interest Statement
The authors declare that the research was conducted in the absence of any commercial or financial relationships that could be construed as a potential conflict of interest.
Acknowledgments
We would like to thank Tobias Bernklau, Melissa Henjes, Markus Koch, and Paulina Schulz for their assistance in data collection. We would particularly like to thank Semiha Aydin for acquisition and analysis of MR data.
References
Atagün, M. I., Şikoğlu, E. M., Can, S. S., Karakaş-Uğurlu, G., Ulusoy-Kaymak, S., Çayköylü, A., et al. (2015). Investigation of Heschl’s gyrus and planum temporale in patients with schizophrenia and bipolar disorder: a proton magnetic resonance spectroscopy study. Schizophr. Res. 161, 202–209. doi: 10.1016/j.schres.2014.11.012
Balz, J., Keil, J., Roa Romero, Y., Mekle, R., Schubert, F., Aydin, S., et al. (2016). GABA concentration in superior temporal sulcus predicts gamma power and perception in the sound-induced flash illusion. Neuroimage 125, 724–730. doi: 10.1016/j.neuroimage.2015.10.087
Beauchamp, M.-C., Lecomte, T., Lecomte, C., Leclerc, C., and Corbière, M. (2006). Do people with a first episode of psychosis differ in personality profiles? Schizophr. Res. 85, 162–167. doi: 10.1016/j.schres.2006.03.026
Beauchamp, M.-C., Lecomte, T., Lecomte, C., Leclerc, C., and Corbière, M. (2011). Personality traits in early psychosis: relationship with symptom and coping treatment outcomes. Early Interv. Psychiatry 5, 33–40. doi: 10.1111/j.1751-7893.2010.00198.x
Beck, K., Javitt, D. C., and Howes, O. D. (2016). Targeting glutamate to treat schizophrenia: lessons from recent clinical studies. Psychopharmacology 233, 2425–2428. doi: 10.1007/s00213-016-4318-6
Bell, M., Fiszdon, J., Richardson, R., Lysaker, P., and Bryson, G. (2007). Are self-reports valid for schizophrenia patients with poor insight? Relationship of unawareness of illness to psychological self-report instruments. Psychiatry Res. 151, 37–46. doi: 10.1016/j.psychres.2006.04.012
Berenbaum, H., and Fujita, F. (1994). Schizophrenia and personality: exploring the boundaries and connections between vulnerability and outcome. J. Abnorm. Psychol. 103, 148–158. doi: 10.1037/0021-843X.103.1.148
Boyette, L.-L., Korver-Nieberg, N., Verweij, K., Meijer, C., Dingemans, P., Cahn, W., et al. (2013). Associations between the Five-Factor Model personality traits and psychotic experiences in patients with psychotic disorders, their siblings and controls. Psychiatry Res. 210, 491–497. doi: 10.1016/j.psychres.2013.06.040
Boyette, L.-L., Nederlof, J., Meijer, C., de Boer, F., and de Haan, L. (2015). Three year stability of Five-Factor Model personality traits in relation to changes in symptom levels in patients with schizophrenia or related disorders. Psychiatry Res. 229, 539–544. doi: 10.1016/j.psychres.2015.05.057
Camisa, K. M., Bockbrader, M. A., Lysaker, P., Rae, L. L., Brenner, C. A., and O’Donnell, B. F. (2005). Personality traits in schizophrenia and related personality disorders. Psychiatry Res. 133, 23–33. doi: 10.1016/j.psychres.2004.09.002
Carlsson, M. L. (2001). On the role of prefrontal cortex glutamate for the antithetical phenomenology of obsessive compulsive disorder and attention deficit hyperactivity disorder. Prog. Neuropsychopharmacol. Biol. Psychiatry 25, 5–26. doi: 10.1016/S0278-5846(00)00146-9
Chang, L., Friedman, J., Ernst, T., Zhong, K., Tsopelas, N. D., and Davis, K. (2007). Brain metabolite abnormalities in the white matter of elderly schizophrenic subjects: implication for glial dysfunction. Biol. Psychiatry 62, 1396–1404. doi: 10.1016/j.biopsych.2007.05.025
Chavez-Noriega, L. E., Schaffhauser, H., and Campbell, U. C. (2002). Metabotropic glutamate receptors: potential drug targets for the treatment of schizophrenia. Curr. Drug Targets CNS Neurol. Disord. 1, 261–281. doi: 10.2174/1568007023339337
Chiu, P. W., Lui, S. S. Y., Hung, K. S. Y., Chan, R. C. K., Chan, Q., Sham, P. C., et al. (2017). In vivo gamma-aminobutyric acid and glutamate levels in people with first-episode schizophrenia: a proton magnetic resonance spectroscopy study. Schizophr. Res. 193, 295–303. doi: 10.1016/j.schres.2017.07.021
Compton, M. T., Bakeman, R., Alolayan, Y., Maria, P., Bernardini, F., Broussard, B., et al. (2015). Personality domains, duration of untreated psychosis, functioning, and symptom severity in first-episode psychosis. Schizophr. Res. 168, 113–119. doi: 10.1016/j.schres.2015.06.028
Costa, P. T., and McCrae, R. R. (1989). NEO PI/FFI Manual Supplement for use with the NEO Personality Inventory and the NEO Five-Factor Inventory. Odessa, FL: Psychological Assessment Resources, Inc.
Costa, P. T., and McCrae, R. R. (1992). Neo PI-R Professional Manual: Revised NEO Personality Inventory (NEO PI-R) and NEO Five-Factor Inventory (NEO-FFI). Odessa, FL: Psychological Assessment Resources, Inc.
Coyle, J. T. (1996). The glutamatergic dysfunction hypothesis for schizophrenia. Harv. Rev. Psychiatry 3, 241–253. doi: 10.3109/10673229609017192
Coyle, J. T. (2006). Glutamate and schizophrenia: beyond the dopamine hypothesis. Cell. Mol. Neurobiol. 26, 365–384. doi: 10.1007/s10571-006-9062-8
Ćurčić-Blake, B., Bais, L., Sibeijn-Kuiper, A., Pijnenborg, H. M., Knegtering, H., Liemburg, E., et al. (2017). Glutamate in dorsolateral prefrontal cortex and auditory verbal hallucinations in patients with schizophrenia: a 1H MRS study. Prog. Neuropsychopharmacol. Biol. Psychiatry 78, 132–139. doi: 10.1016/j.pnpbp.2017.05.020
Demjaha, A., Egerton, A., Murray, R. M., Kapur, S., Howes, O. D., Stone, J. M., et al. (2014). Antipsychotic treatment resistance in schizophrenia associated with elevated glutamate levels but normal dopamine function. Biol. Psychiatry 75, e11–e13. doi: 10.1016/j.biopsych.2013.06.011
Egerton, A., Fusar-Poli, P., and Stone, J. M. (2012). Glutamate and psychosis risk. Curr. Pharm. Des. 18, 466–478. doi: 10.2174/138161212799316244
Egerton, A., and Stone, J. M. (2012). The glutamate hypothesis of schizophrenia: neuroimaging and drug development. Curr. Pharm. Biotechnol. 13, 1500–1512. doi: 10.2174/138920112800784961
Egerton, A., Stone, J. M., Chaddock, C. A., Barker, G. J., Bonoldi, I., Howard, R. M., et al. (2014). Relationship between brain glutamate levels and clinical outcome in individuals at ultra high risk of psychosis. Neuropsychopharmacology 39, 2891–2899. doi: 10.1038/npp.2014.143
First, M. B., Spitzer, R. L., Gibbon, M., and Williams, J. B. W. (2002). Structured Clinical Interview for DSM-IV-TR Axis I Disorders, Research Version, Non-Patient Edition. (SCID-I/NP). New York, NY: New York State Psychiatric Institute.
Fryers, T., and Brugha, T. (2013). Childhood determinants of adult psychiatric disorder. Clin. Pract. Epidemiol. Ment. Health 9, 1–50. doi: 10.2174/1745017901309010001
Fusar-Poli, P., Stone, J. M., Broome, M. R., Valli, I., Mechelli, A., McLean, M. A., et al. (2011). Thalamic glutamate levels as a predictor of cortical response during executive functioning in subjects at high risk for psychosis. Arch. Gen. Psychiatry 68, 881–890. doi: 10.1001/archgenpsychiatry.2011.46
Gallinat, J., McMahon, K., Kühn, S., Schubert, F., and Schaefer, M. (2016). Cross-sectional study of glutamate in the anterior cingulate and hippocampus in schizophrenia. Schizophr. Bull. 42, 425–433. doi: 10.1093/schbul/sbv124
Gardner, D. M., Murphy, A. L., O’Donnell, H. O., Centorrino, F., and Baldessarini, R. J. (2010). International consensus study of antipsychotic dosing. Am. J. Psychiatry 167, 686–693. doi: 10.1176/appi.ajp.2009.09060802
Gleeson, J. F., Rawlings, D., Jackson, H., and Mcgorry, P. (2005). Agreeableness and neuroticism as predictors of relapse after first-episode psychosis: a prospective follow-up study. J. Nerv. Ment. Dis. 193, 160–169. doi: 10.1097/01.nmd.0000154841.99550.d3
Goodwin, R. D., Fergusson, D. M., and Horwood, L. J. (2003). Neuroticism in adolescence and psychotic symptoms in adulthood. Psychol. Med. 33, 1089–1097. doi: 10.1017/S0033291703007888
Goto, N., Yoshimura, R., Kakeda, S., Nishimura, J., Moriya, J., Hayashi, K., et al. (2012). Six-month treatment with atypical antipsychotic drugs decreased frontal-lobe levels of glutamate plus glutamine in early-stage first-episode schizophrenia. Neuropsychiatr. Dis. Treat. 8, 119–122. doi: 10.2147/NDT.S25582
Gruetter, R., and Boesch, C. (1992). Fast, noniterative shimming of specially localized signals. In vivo analysis of the magnetic field along axes. J. Magn. Reson. 96, 323–334.
Gruetter, R., and Tkac, I. (2000). Field mapping without reference scan using asymmetric echo-planar techniques. Magn. Reson. Med. 43, 319–323. doi: 10.1002/(SICI)1522-2594(200002)43:2<319::AID-MRM22>3.0.CO;2-1
Gurrera, R. J., Mccarley, R. W., and Salisbury, D. (2014). Cognitive task performance and symptoms contribute to personality abnormalities in first hospitalized schizophrenia. J. Psychiatr. Res. 55, 68–76. doi: 10.1016/j.jpsychires.2014.03.022
Gurrera, R. J., Nestor, P. G., and O’Donnell, B. F. (2000). Personality traits in schizophrenia: comparison with a community sample. J. Nerv. Ment. Dis. 188, 31–35. doi: 10.1097/00005053-200001000-00006
Herrán, A., Sierra-biddle, D., Cuesta, M. J., Sandoya, M., and Vázquez-Barquero, J. L. (2006). Can personality traits help us explain disability in chronic schizophrenia Psychiatry Clin. Neurosci. 60, 538–545. doi: 10.1111/j.1440-1819.2006.01577.x
Hettema, J. M., An, S. S., Neale, M. C., Bukszar, J., van den Oord, E. J., Kendler, K. S., et al. (2006). Association between glutamic acid decarboxylase genes and anxiety disorders, major depression, and neuroticism. Mol. Psychiatry 11, 752–762. doi: 10.1038/sj.mp.4001845
Hoerst, M., Weber-Fahr, W., Tunc-Skarka, N., Ruf, M., Bohus, M., Schmahl, C., et al. (2010). Correlation of glutamate levels in the anterior cingulate cortex with self-reported impulsivity in patients with borderline personality disorder and healthy controls. Arch. Gen. Psychiatry 67, 946–954. doi: 10.1001/archgenpsychiatry.2010.93
Howes, O. D., and Kapur, S. (2014). A neurobiological hypothesis for the classification of schizophrenia: type A (hyperdopaminergic) and type B (normodopaminergic). Br. J. Psychiatry 205, 1–3. doi: 10.1192/bjp.bp.113.138578
Hugdahl, K., Craven, A. R., Nygård, M., Løberg, E.-M., Øystein Berle, J., Johnsen, E., et al. (2015). Glutamate as a mediating transmitter for auditory hallucinations in schizophrenia: a (1)H MRS study. Schizophr. Res. 161, 252–260. doi: 10.1016/j.schres.2014.11.015
Ikeda, S., Mizuno-matsumoto, Y., Canuet, L., Ishii, R., Aoki, Y., Hata, M., et al. (2015). Emotion regulation of neuroticism: emotional information processing related to psychosomatic state evaluated by electroencephalography and exact low-resolution brain electromagnetic tomography. Neuropsychobiology 71, 34–41. doi: 10.1159/000368119
Javitt, D. C. (2010). Glutamatergic theories of schizophrenia. Isr. J. Psychiatry Relat. Sci. 47, 4–16.
Javitt, D. C., and Zukin, S. R. (1991). Recent advances in the phencyclidine model of schizophrenia. Am. J. Psychiatry 148, 1301–1308. doi: 10.1176/ajp.148.10.1301
Kantrowitz, J. T., and Javitt, D. C. (2010). N-methyl-d-aspartate (n.d.) receptor dysfunction or dysregulation: the final common pathway on the road to schizophrenia? Brain Res. Bull. 83, 108–121. doi: 10.1016/j.brainresbull.2010.04.006
Kay, S. R., Fiszbein, A., and Opler, L. A. (1987). The positive and negative syndrome scale (PANSS) for schizophrenia. Schizophr. Bull. 13, 261–276. doi: 10.1093/schbul/13.2.261
Keefe, R. S., Goldberg, T. E., Harvey, P. D., Gold, J. M., Poe, M. P., and Coughenour, L. (2004). The brief assessment of cognition in schizophrenia: reliability, sensitivity, and comparison with a standard neurocognitive battery. Schizophr. Res. 68, 283–297. doi: 10.1016/j.schres.2003.09.011
Keil, J., and Senkowski, D. (2018). Neural oscillations orchestrate multisensory processing. Neuroscientist doi: 10.1177/1073858418755352 [Epub ahead of print].
Kentros, M., Smith, T. E., Hull, J., McKee, M., Terkelsen, K., and Capalbo, C. (1997). Stability of personality traits in schizophrenia and schizoaffective disorder: a pilot project. J. Nerv. Ment. Dis. 185, 549–555. doi: 10.1097/00005053-199709000-00003
Keshavan, M. S., Diwadkar, V. A., Montrose, D. M., Rajarethinam, R., and Sweeney, J. A. (2005). Premorbid indicators and risk for schizophrenia: a selective review and update. Schizophr. Res. 79, 45–57. doi: 10.1016/j.schres.2005.07.004
Kirihara, K., Kasai, K., Tada, M., Nagai, T., Kawakubo, Y., Yamasaki, S., et al. (2012). Neurophysiological impairment in emotional face processing is associated with low extraversion in schizophrenia. Prog. Neuropsychopharmacol. Biol. Psychiatry 37, 270–275. doi: 10.1016/j.pnpbp.2012.02.012
Krabbendam, L., Janssen, I., Bak, M., Bijl, R. V., de Graaf, R., and van Os, J. (2002). Neuroticism and low self-esteem as risk factors for psychosis. Soc. Psychiatry Psychiatr. Epidemiol. 37, 1–6. doi: 10.1007/s127-002-8207-y
Krug, A., Markov, V., Leube, D., Zerres, K., Eggermann, T., Nöthen, M. M., et al. (2008). Genetic variation in the schizophrenia-risk gene neuregulin1 correlates with personality traits in healthy individuals. Eur. Psychiatry 23, 344–349. doi: 10.1016/j.eurpsy.2008.03.004
Li, T., Yan, X., Wang, J., Li, Q., Li, H., and Li, J. (2017). Neuronal correlates of individual differences in the Big Five personality traits: evidences from cortical morphology and functional homogeneity. Front. Neurosci. 11:414. doi: 10.3389/fnins.2017.00414
Litvak, V., Mattout, J., Kiebel, S., Phillips, C., Henson, R., Kilner, J., et al. (2011). EEG and MEG data analysis in SPM8. Comput. Intell. Neurosci. 2011:852961. doi: 10.1155/2011/852961
Lönnqvist, J.-E., Verkasalo, M., Haukka, J., Nyman, K., Tiihonen, J., Laaksonen, I., et al. (2009). Premorbid personality factors in schizophrenia and bipolar disorder: results from a large cohort study of male conscripts. J. Abnorm. Psychol. 118, 418–423. doi: 10.1037/a0015127
Lu, F., Huo, Y., Li, M., Chen, H., Liu, F., Wang, Y., et al. (2014). Relationship between personality and gray matter volume in healthy young adults: a voxel-based morphometric study. PLoS One 9:e88763. doi: 10.1371/journal.pone.0088763
Maier, J. X., Chandrasekaran, C., and Ghazanfar, A. A. (2008). Integration of bimodal looming signals through neuronal coherence in the temporal lobe. Curr. Biol. 18, 963–968. doi: 10.1016/j.cub.2008.05.043
Marsman, A., Mandl, R. C. W., Klomp, D. W. J., Bohlken, M. M., Boer, V. O., Andreychenko, A., et al. (2014). GABA and glutamate in schizophrenia: a 7 T 1H-MRS study. Neuroimage Clin. 6, 398–407. doi: 10.1016/j.nicl.2014.10.005
Marsman, A., van den Heuvel, M. P., Klomp, D. W. J., Kahn, R. S., Luijten, P. R., and Hulshoff Pol, H. E. (2013). Glutamate in schizophrenia: a focused review and meta-analysis of 1H-MRS studies. Schizophr. Bull. 39, 120–129. doi: 10.1093/schbul/sbr069
Mekle, R., Mlynárik, V., Gambarota, G., Hergt, M., Krueger, G., and Gruetter, R. (2009). MR spectroscopy of the human brain with enhanced signal intensity at ultrashort echo times on a clinical platform at 3T and 7T. Magn. Reson. Med. 61, 1279–1285. doi: 10.1002/mrm.21961
Merritt, K., Egerton, A., Kempton, M. J., Taylor, M. J., and McGuire, P. K. (2016). Nature of glutamate alterations in schizophrenia: a meta-analysis of proton magnetic resonance spectroscopy studies. JAMA Psychiatry 73, 665–674. doi: 10.1001/jamapsychiatry.2016.0442
Millan, M. J., Andrieux, A., Bartzokis, G., Cadenhead, K., Dazzan, P., Fusar-Poli, P., et al. (2016). Altering the course of schizophrenia: progress and perspectives. Nat. Rev. Drug Discov. 15, 485–515. doi: 10.1038/nrd.2016.28
Mlynárik, V., Gambarota, G., Frenkel, H., and Gruetter, R. (2006). Localized short-echo-time proton MR spectroscopy with full signal-intensity acquisition. Magn. Reson. Med. 56, 965–970. doi: 10.1002/mrm.21043
Mullins, P. G., Chen, H., Xu, J., Caprihan, A., and Gasparovic, C. (2008). Comparative reliability of proton spectroscopy techniques designed to improve detection of J-coupled metabolites. Magn. Reson. Med. 60, 964–969. doi: 10.1002/mrm.21696
Neill, A. O., and Frodl, T. (2012). Brain structure and function in borderline personality disorder. Brain Struct. Funct. 217, 767–782. doi: 10.1007/s00429-012-0379-4
Nuechterlein, K. H., Dawson, M. E., Ventura, J., Gitlin, M., Subotnik, K. L., Snyder, K. S., et al. (1994). The vulnerability/stress model of schizophrenic relapse: a longitudinal study. Acta Psychiatr. Scand. Suppl. 382, 58–64. doi: 10.1111/j.1600-0447.1994.tb05867.x
Ohi, K., Shimada, T., Nitta, Y., Kihara, H., Okubo, H., Uehara, T., et al. (2016). The five-factor model personality traits in schizophrenia: a meta-analysis. Psychiatry Res. 240, 34–41. doi: 10.1016/j.psychres.2016.04.004
Ohi, K., Shimada, T., Yasuyama, T., Kimura, K., Uehara, T., and Kawasaki, Y. (2017). Spatial and temporal expression patterns of genes around nine neuroticism-associated loci. Prog. Neuropsychopharmacol. Biol. Psychiatry 77, 164–171. doi: 10.1016/j.pnpbp.2017.04.019
Plitman, E., Patel, R., Chung, J. K., Pipitone, J., Chavez, S., Reyes-Madrigal, F., et al. (2016). Glutamatergic metabolites, volume and cortical thickness in antipsychotic-naive patients with first-episode psychosis: implications for excitotoxicity. Neuropsychopharmacology 41, 2606–2613. doi: 10.1038/npp.2016.84
Poels, E. M. P., Kegeles, L. S., Kantrowitz, J. T., Javitt, D. C., Lieberman, J. A., Abi-dargham, A., et al. (2014a). Glutamatergic abnormalities in schizophrenia: a review of proton MRS findings. Schizophr. Res. 152, 325–332. doi: 10.1016/j.schres.2013.12.013
Poels, E. M. P., Kegeles, L. S., Kantrowitz, J. T., Slifstein, M., Javitt, D. C., Lieberman, J. A., et al. (2014b). Imaging glutamate in schizophrenia: review of findings and implications for drug discovery. Mol. Psychiatry 19, 20–29. doi: 10.1038/mp.2013.136
Provencher, S. W. (1993). Estimation of metabolite concentrations from localized in vivo proton NMR spectra. Magn. Reson. Med. 30, 672–679. doi: 10.1002/mrm.1910300604
Riccelli, R., Toschi, N., Nigro, S., Terracciano, A., and Passamonti, L. (2017). Surface-based morphometry reveals the neuroanatomical basis of the five-factor model of personality. Soc. Cogn. Affect. Neurosci. 12, 671–684. doi: 10.1093/scan/nsw175
Ridgewell, C., Blackford, J. U., Mchugo, M., and Heckers, S. (2017). Personality traits predicting quality of life and overall functioning in schizophrenia. Schizophr. Res. 182, 19–23. doi: 10.1016/j.schres.2016.10.007
Rosenberg, D. R., Mirza, Y., Russell, A., Tang, J., Smith, J. M., Banerjee, S. P., et al. (2004). Reduced anterior cingulate glutamatergic concentrations in childhood OCD and major depression versus healthy controls. J. Am. Acad. Child Adolesc. Psychiatry 43, 1146–1153. doi: 10.1097/01.chi.0000132812.44664.2d
Schubert, F., Gallinat, J., Seifert, F., and Rinneberg, H. (2004). Glutamate concentrations in human brain using single voxel proton magnetic resonance spectroscopy at 3 Tesla. Neuroimage 21, 1762–1771. doi: 10.1016/j.neuroimage.2003.11.014
Schubert, F., Kühn, S., Gallinat, J., Mekle, R., and Ittermann, B. (2017). Towards a neurochemical profile of the amygdala using short-TE 1 H magnetic resonance spectroscopy at 3 T. NMR Biomed. 30, 1–10. doi: 10.1002/nbm.3685
Stone, J. M., Day, F., Tsagaraki, H., Valli, I., Mclean, M. A., Lythgoe, D. J., et al. (2009). Glutamate dysfunction in people with prodromal symptoms of psychosis: relationship to gray matter volume. Biol. Psychiatry 66, 533–539. doi: 10.1016/j.biopsych.2009.05.006
Suslow, T., Lindner, C., Kugel, H., Egloff, B., and Schmukle, S. C. (2014). Using implicit association tests for the assessment of implicit personality self-concepts of extraversion and neuroticism in schizophrenia. Psychiatry Res. 218, 272–276. doi: 10.1016/j.psychres.2014.04.023
Thakkar, K. N., Rösler, L., Wijnen, J. P., Boer, V. O., Klomp, D. W. J., Cahn, W., et al. (2017). 7T proton magnetic resonance spectroscopy of gamma-aminobutyric acid, glutamate, and glutamine reveals altered concentrations in patients with schizophrenia and healthy siblings. Biol. Psychiatry 81, 525–535. doi: 10.1016/j.biopsych.2016.04.007
Théberge, J., Bartha, R., Drost, D. J., Menon, R. S., Malla, A., Takhar, J., et al. (2002). Glutamate and glutamine measured with 4.0 T proton MRS in never-treated patients with schizophrenia and healthy volunteers. Am. J. Psychiatry 159, 1944–1946. doi: 10.1176/appi.ajp.159.11.1944
Tibbo, P., Hanstock, C., Valiakalayil, A., and Allen, P. (2004). 3-T proton MRS investigation of glutamate and glutamine in adolescents at high genetic risk for schizophrenia. Am. J. Psychiatry 161, 1116–1118. doi: 10.1176/appi.ajp.161.6.1116
Tkac, I., Starcuk, Z., Choi, I., and Gruetter, R. (1999). In vivo 1H NMR spectroscopy of rat brain at 1 ms echo time. Magn. Reson. Med. 41, 649–656. doi: 10.1002/(SICI)1522-2594(199904)41:4<649::AID-MRM2>3.0.CO;2-G
Treen, D., Batlle, S., Mollà, L., Forcadell, E., Chamorro, J., Bulbena, A., et al. (2016). Are there glutamate abnormalities in subjects at high risk mental state for psychosis? A review of the evidence. Schizophr. Res. 171, 166–175. doi: 10.1016/j.schres.2016.01.005
Tseng, H., Bossong, M. G., Modinos, G., Chen, K., McGuire, P., and Allen, P. (2015). A systematic review of multisensory cognitive-affective integration in schizophrenia. Neurosci. Biobehav. Rev. 55, 444–452. doi: 10.1016/j.neubiorev.2015.04.019
Van Os, J., and Jones, P. B. (2001). Neuroticism as a risk factor for schizophrenia. Psychol. Med. 31, 1129–1134. doi: 10.1017/S0033291701004044
Keywords: schizophrenia, glutamate, neuroticism, magnetic resonance spectroscopy, superior temporal sulcus
Citation: Balz J, Roa Romero Y, Keil J, Schubert F, Ittermann B, Mekle R, Montag C, Gallinat J and Senkowski D (2018) Glutamate Concentration in the Superior Temporal Sulcus Relates to Neuroticism in Schizophrenia. Front. Psychol. 9:578. doi: 10.3389/fpsyg.2018.00578
Received: 15 January 2018; Accepted: 05 April 2018;
Published: 07 May 2018.
Edited by:
Joachim Lange, Heinrich-Heine-Universität Düsseldorf, GermanyReviewed by:
Kazutaka Ohi, Kanazawa Medical University, JapanRonald Gurrera, VA Boston Healthcare System, United States
Copyright © 2018 Balz, Roa Romero, Keil, Schubert, Ittermann, Mekle, Montag, Gallinat and Senkowski. This is an open-access article distributed under the terms of the Creative Commons Attribution License (CC BY). The use, distribution or reproduction in other forums is permitted, provided the original author(s) and the copyright owner are credited and that the original publication in this journal is cited, in accordance with accepted academic practice. No use, distribution or reproduction is permitted which does not comply with these terms.
*Correspondence: Daniel Senkowski, ZGFuaWVsLnNlbmtvd3NraUBjaGFyaXRlLmRl Johanna Balz, am9oYW5uYS5iYWx6QGNoYXJpdGUuZGU=