- 1Department of Psychology, University of Montreal, Montreal, QC, Canada
- 2CHU Sainte-Justine Research Center, Montreal, QC, Canada
- 3Functional Neuroimaging Unit, University of Montreal’s Geriatric Institute, Montreal, QC, Canada
- 4Center for Advanced Research in Sleep Medicine, Hôpital du Sacré-Cœur de Montréal, Montreal, QC, Canada
A large body of longitudinal research provides compelling evidence for the critical role of early attachment relationships in children’s social, emotional, and cognitive development. It is expected that parent–child attachment relationships may also impact children’s brain development, however, studies linking normative caregiving experiences and brain structure are scarce. To our knowledge, no study has yet examined the associations between the quality of parent–infant attachment relationships and brain morphology during childhood. The aim of this preliminary study was to investigate the prospective links between mother–infant attachment security and whole-brain gray matter (GM) volume and thickness in late childhood. Attachment security toward the mother was assessed in 33 children when they were 15 months old. These children were then invited to undergo structural magnetic resonance imaging at 10–11 years of age. Results indicated that children more securely attached to their mother in infancy had larger GM volumes in the superior temporal sulcus and gyrus, temporo-parietal junction, and precentral gyrus in late childhood. No associations between attachment security and cortical thickness were found. If replicated, these results would suggest that a secure attachment relationship and its main features (e.g., adequate dyadic emotion regulation, competent exploration) may influence GM volume in brain regions involved in social, cognitive, and emotional functioning through experience-dependent processes.
Introduction
Seminal work by Harlow and Harlow (1962) suggested that the primate tendency to attach to a caregiver is innate and does not merely reflect physiological needs. Human children attach to a caregiver who is physically present, even if the caregiver does not fulfill a primary physical need, such as feeding, and even if the caregiver adopts abusive behaviors (Bowlby, 1956; Ainsworth, 1967; Cyr et al., 2010). Attachment is a specific, preferential, and enduring emotional tie between an infant and a caregiver, promoting survival and allowing children to feel safe and protected (Bowlby, 1969/1982). Infant attachment is expressed by behaviors such as separation distress, greeting reactions upon reunion, and the tendency to turn to a specific caregiver for reassurance when distressed (Sroufe, 1979; Cassidy, 2016). These innate, universal behavioral tendencies are driven by a biologically based attachment system (Cassidy, 2016). Importantly, however, they are subsequently gradually modulated by caregiver responses, progressively leading to the development of individual differences in the expression and organization of infant attachment behavior (Ainsworth et al., 1978; Fearon and Belsky, 2016). These individual differences are considered to index the “quality” or “security” of attachment relationships. Specifically, a critical tenet of attachment theory is that securely attached children have confident expectations of themselves as being able to solicit the caregiver’s proximity, and of the caregiver as being responsive and available when needed (Bowlby, 1973, 1988). In contrast, infants develop insecure attachments over the course of interactions with caregivers who have difficulty responding adequately to their emotional needs (see meta-analysis by De Wolff and Van IJzendoorn, 1997). Hence, virtually all children become attached to a caregiver, but not all develop secure attachment relationships (Cassidy, 2016).
Decades of longitudinal research have supported the notion that individual differences in infant and child attachment security to primary caregivers are of critical importance for child social, emotional, and cognitive development. Several meta-analyses suggest that variations in attachment security are associated with individual differences in a range of child outcomes: higher attachment security (as compared to insecurity) is associated with better social competence (Groh et al., 2014, 2017a), emotion understanding (Cooke et al., 2016), quality of peer relationships (Pallini et al., 2014), language competence (Van IJzendoorn et al., 1995), as well as fewer internalizing (Groh et al., 2012, 2017a; Madigan et al., 2013) and externalizing behavior problems (Fearon et al., 2010; Groh et al., 2017a).
Such associations between attachment security and child social, emotional, and cognitive development are sometimes interpreted as suggesting that attachment experiences influence the development of children’s brain structures underlying socio-emotional and cognitive functioning (Gunnar et al., 2006; Belsky and de Haan, 2011; Tottenham, 2014). Indeed, although many brain development processes, such as neuronal differentiation, synaptogenesis, and pruning, are guided largely by biological factors (Rakic, 1988), caregiving experiences can also shape brain development in two ways. Experience-expectant processes refer to development that occurs in response to experiences that are typically shared by all members of a species (Greenough et al., 1987). Caregiver presence is expected in humans, and indeed caregiver deprivation is associated with alterations of brain structure and function (Eisenberger and Cole, 2012; Tottenham, 2012). Closer to our purposes, experience-dependent processes refer to brain development that varies from one person to another as a result of specific individual experiences (Greenough et al., 1987). For example, animal studies indicate that variations in the quality of caregiving have long-term consequences for brain development, notably in brain areas that support stress regulation, social behaviors, and reward processing (Meaney, 2001; Yu et al., 2013; Peña et al., 2014). Accordingly, it is plausible that the security of parent–child attachment, as an important indicator of the quality of the early caregiving environment, may contribute to shaping the developing brain.
This hypothesis is sensible when considering that a core feature of a secure attachment relationship is that the caregiver acts as a secure base from which the child can confidently explore, and seek proximity when distressed (Ainsworth, 1985). During exploration, securely attached children can freely play an active, purposeful role in exploring the surrounding social and physical world, which provides rich stimulation for the developing brain. When, however, they encounter a distressing event during exploration (e.g., hurting oneself while playing), the very nature of their secure attachment relationship allows these children to return to their caregiver for help and soothing (Ainsworth, 1985), which gradually fosters the development of emotion regulation (Calkins, 2004; Cole et al., 2004). Overall, secure attachment relationships are believed to favor both confident exploration and effective emotion regulation in children, which are likely to influence structural development in brain regions involved in a range of social, emotional, and cognitive functions. In fact, it has been proposed that the quality of the attachment bond between children and their caregivers is especially likely to be associated with brain structures underpinning social functioning (Rilling and Young, 2014; Tottenham, 2014), known as the “social brain” and including the superior temporal sulcus, medial prefrontal and anterior cingulate cortices, inferior frontal gyrus, anterior insula, as well as the amygdala (Blakemore, 2008; Adolphs, 2009).
Yet, in contrast to the abundance of research linking attachment security to behavioral outcomes, the links between brain structure and child attachment are still poorly understood (Coan, 2016). Indirect evidence comes from studies of children exposed to maltreatment, which suggest that severely adverse caregiving experiences can lead to morphological alterations in brain regions underpinning social, emotional, and cognitive functions later in life (Teicher and Samson, 2016). Children and adults exposed to childhood maltreatment present abnormal brain volumes and thickness compared to non-exposed individuals in several brain regions (see Lim et al., 2014; Riem et al., 2015, for meta-analyses; Kelly et al., 2013; Whittle et al., 2013; Teicher and Samson, 2016). Nonetheless, these findings should be considered alongside the numerous confounding factors that characterize maltreating families (e.g., poor mental and physical health, poverty, poor quality of sleep, prenatal drug and alcohol use; Edwards et al., 2003; Hussey et al., 2006; Smith et al., 2007; Cuddihy et al., 2013). Given that these factors also influence brain development (Jednoróg et al., 2012; Goodkind et al., 2015; Urrila et al., 2017), the poor quality of parent–child relationships may or may not be the cause of the structural abnormalities observed in the brains of maltreated children (Belsky and de Haan, 2011). Studies in the general population are required to fully understand the association between caregiving experiences and brain morphology.
In contrast to the relatively large body of research on maltreatment, empirical evidence for links between normative variations in parent–child relationship quality and brain development in typically developing children is scarce, and almost all relevant studies have examined parental behavior rather than parent–child relationship quality per se. Overall, these studies suggest that normative variations in different dimensions of parental behavior are associated with differences in gray matter (GM) volume and thickness in several brain regions, although directionality varies. Specifically, higher maternal sensitivity has been found to relate to larger subcortical GM volume in infants (Sethna et al., 2017), but also to smaller hippocampal volumes and to (marginally) smaller amygdalar volume in infants (Rifkin-Graboi et al., 2015). Kok et al.’s (2015, 2017) longitudinal studies suggested that parental sensitivity in infancy was not associated with hippocampal and amygdalar volumes in school-aged children, but was associated with larger total GM volume as well as thicker cortex in the bilateral middle frontal gyri, precentral gyri, and left postcentral gyrus. Greater maternal support during the preschool years is associated with larger hippocampal volumes in school-aged children (Luby et al., 2012, 2013, 2016), while self-reported parental praise is related to larger left insula in children aged 5–18 years (Matsudaira et al., 2016). The presence of more positive maternal behavior has been linked to decreased volumetric development in the right amygdala as well as accelerated cortical thinning in the right anterior cingulate and bilateral prefrontal cortices in adolescence (Whittle et al., 2014). On the other hand, negative aspects of parental behavior (e.g., self-reported hostility and observed aggressive behavior) are related to smaller total GM volume (Lévesque et al., 2015) and attenuated cortical thinning in the right superior frontal, superior parietal, and supramarginal gyri, as well as a reduced volumetric development in the left nucleus accumbens in adolescence (Whittle et al., 2016). These brain structures are crucial for children’s social, emotional, and cognitive development, given that they underpin social cognition, emotion regulation, threat detection, attention monitoring, stress regulation and reward processing (Meaney, 2001; Dölen and Malenka, 2014; Frank et al., 2014; Kalisch and Gerlicher, 2014; Deen et al., 2015).
In light of the growing literature pertaining to specific dimensions of parental behavior and brain morphology, it is surprising that almost no research has focused directly on the quality of the parent–child dyadic relationship, of which attachment security is perhaps the best documented and most widely recognized indicator. Given that the quality of parenting behavior is moderately associated with parent–child attachment security (De Wolff and Van IJzendoorn, 1997), the body of literature presented above suggests that parent–child attachment security may relate to children’s brain morphology. Yet, to our knowledge, only two studies have examined the relations between brain structure and the quality of parent–child attachment relationships, and both focused on subcortical volumes (amygdala, hippocampus, caudate nucleus, thalamus) once participants reached adulthood. These studies suggest that poorer attachment quality to mother in infancy (assessed with the Strange Situation Procedure; SSP, Ainsworth et al., 1978) relates to larger volume of the amygdalae in adulthood (Moutsiana et al., 2015; Lyons-Ruth et al., 2016). These two longitudinal studies highlight the potentially long-lasting link between early parent–child attachment and subcortical brain structure. However, it is not known whether the longitudinal links are already apparent during childhood, whether the direction of association is stable, and whether attachment may also relate to other brain regions. These are important questions in light of increasing evidence that developmental considerations play a crucial role in the links between caregiving experiences and regional brain development, including directionality of such links (Tottenham and Sheridan, 2009; Teicher et al., 2016). For example, higher-quality parenting is associated with smaller hippocampal volumes in infants and children [Luby et al., 2012, 2013, 2016; Rifkin-Graboi et al., 2015; but see Sethna et al.’s (2017) results indicating larger subcortical GM volume]; however, higher-quality parenting is associated with larger hippocampal volume in adolescence (albeit in a sample of children exposed to cocaine during gestation; Rao et al., 2010).
Building on previous studies (Moutsiana et al., 2015; Lyons-Ruth et al., 2016), the current report examines the longitudinal associations between mother–infant attachment security and whole-brain GM volume and thickness in late childhood. Previous studies have used an a priori regions-of-interests approach to investigate the links between parent–child relationship quality and brain structure, which may limit the scope of the conclusions that can be drawn; a whole-brain approach was therefore used here. We assessed early mother–child attachment security with the Attachment Behavior Q-Sort (AQS; Waters and Deane, 1985), which yields a continuous score for attachment security rather than assignment to a particular attachment category. This approach maximizes statistical power by affording excellent detection of fine individual differences, and may be especially appropriate in the context of small sample sizes (Groh et al., 2017b). Psychometric work also suggests that a dimensional approach is coherent with the underlying structure of individual differences in attachment (Fraley and Spieker, 2003). Given the scarcity of literature on attachment security and brain morphology in typically developing children, and the fact that a large number of brain regions have been variously linked to caregiving experiences, the statistical analyses were exploratory and no a priori hypotheses were formulated with regards to the location of putative associations or the direction of associations, considering also that some aspects of brain development trajectories follow an inverted U-shape (Shaw et al., 2008; Giedd et al., 2015).
Materials and Methods
Participants
Participants included in the present study (n = 33) were followed annually as part of a larger longitudinal research project that documents the prospective associations between the early caregiving environment and several facets of child development (see Bélanger et al., 2015). In the present study, we report on attachment security assessed at 15 months of age (T1; M = 15.65, SD = 0.97, range = 14.50 – 18.00) and structural magnetic resonance imaging (MRI) data collected when children were 10–11 years of age (T2, M = 10.59, SD = 0.46, range = 10.0 – 11.67 years). The study was approved by the local research ethics committee of aging-neuroimaging of the CIUSSS du Centre-Sud-de-l’île-de-Montréal and all families provided written informed consent for participation.
Families were recruited from random birth lists of a large Canadian metropolitan area, provided by the Ministry of Health and Social Services. Inclusion criteria for participation were full-term pregnancy (i.e., at least 37 weeks of gestation) and the absence of any known physical or mental disability, severe developmental delay in the infant, acquired brain injury, and standard MRI counter-indications. For the current analyses, 64 families were invited to participate in structural MRI when children reached 10 years of age; among them, 35 (54.69%) agreed to participate. Families who agreed to participate (n = 35) did not differ from those who refused (n = 29) in terms of family income, parental age, education, and ethnicity, as well as child attachment security to mother in infancy (all ps > 0.21, see Table 1). Of the 35 families who agreed to take part in the MRI protocol, one child was excluded from the analyses because of excessive head motion (translation > 2.5 mm or rotation > 2.5 degrees) and one because of suspected neuropathology. Consequently, data from 33 children [20 girls and 13 boys; χ2(1) = 1.46, p = 0.23] were used in the analyses. Group comparisons between families included in the analyses (n = 33) and those who declined the MRI protocol (n = 29) were not significant.
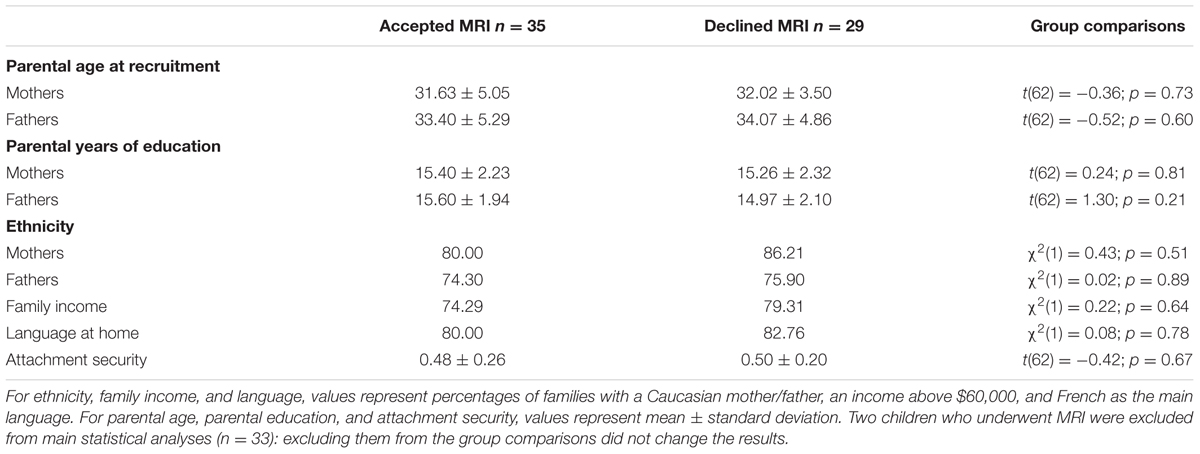
TABLE 1. Sociodemographic information and attachment security scores for families who accepted vs. declined participation in the magnetic resonance imaging (MRI) protocol.
Attachment Security Assessment
Mother–infant attachment security was assessed at T1 using the Attachment Behavior Q-Sort (AQS; Waters and Deane, 1985). The observer-version of the AQS is considered one of the gold-standard measures of attachment (Van IJzendoorn et al., 2004) as it shows excellent construct validity, converging with attachment security assessed with the SSP, with child socio-emotional adaptation, and with maternal sensitivity (see Van IJzendoorn et al., 2004; Cadman et al., 2017 for meta-analytic evidence), while also demonstrating discriminant validity with respect to child temperament (Cadman et al., 2017). In fact, meta-analytic data suggest that the AQS is more closely related to child outcomes than the SSP (Fearon et al., 2010), which makes it an instrument of choice to study putative associations between early attachment and brain morphology.
In this study, trained research assistants observed infant behaviors throughout a 70- to 90-min home visit modeled after the work of Pederson and Moran (1995). This visit was purposely designed to create a situation during which maternal attention was solicited by both infant demands and research-related tasks (e.g., mothers had to fill in questionnaires while infants were not cared for by the research assistant). This aimed at challenging mothers’ capacity to divide their attention between competing demands, thus reproducing the natural conditions of daily life when caring for an infant. Restricting maternal availability to infant demands is a classic trigger of the attachment system in infancy (Ainsworth et al., 1978). The research assistants completed the AQS immediately after the visit. In order to maximize the reliability of the observations performed during these home visits, which was central to this study, we followed Pederson and Moran’s (1995) recommendations for training our home visitors. Research assistants first attended a 2-day training workshop on techniques of home visiting and structured observation of mother–infant interactions. They reviewed several videotapes to practice coding the AQS. The assistants then performed their first few home visits with a more experienced colleague, and the two completed the AQS together. When the junior home visitors were deemed ready to lead home visits independently, the next two or three visits were followed by a debriefing session with an experienced graduate student, to review the salient elements of the visit before scoring the AQS. Inter-rater reliability testing (described below) took place only after assistants had successfully completed this training.
The AQS consists of 90 items measuring the quality of the child’s attachment behaviors toward a specific figure (the mother in this case). Each item of the AQS describes a potential child behavior. Based on observations performed during the entire home visit, research assistants sorted those behaviors into nine clusters of 10 items each, ranging from “very similar” to “very unlike” the observed child’s behaviors. The global score for attachment security consists of the correlation between the observer’s sort of the 90 items and a criterion sort for the prototypically secure infant (Waters and Deane, 1985). Attachment security scores can thus range from -1.0 (highly insecure) to 1.0 (highly secure). Prototypical security represents a fluid balance between exploration of the environment and appropriate reliance on the caregiver for support when needed. To examine inter-rater reliability, 23.1% of the home visits were conducted by two research assistants, who then completed the AQS independently. Agreement between the two raters’ sorts was satisfactory, intra-class correlation (ICC) = 0.71.
Pubertal Status
A parent-report version of the rating scale for pubertal development (Carskadon and Acebo, 1993) was completed at the time of the MRI (T2). Parents evaluated their child’s pubertal development using a scale ranging from 1 = “not yet started” to 4 = “seems completed.” Children’s pubertal status was derived from three items for both boys (body hair growth, voice change, facial hair growth) and girls (body hair growth, breast development, menarche), as described by Carskadon and Acebo (1993).
Structural Magnetic Resonance Imaging
Acquisition
Neuroimaging data were collected at T2 using a 32-channel head coil on a Siemens 3 Tesla scanner (MAGNETOM Trio, Siemens, Erlangen, Germany). Structural data were acquired using a three-dimensional T1-weighted 4-echo magnetization-prepared rapid gradient-echo sequence [3D-T1-4echo-MPRAGE sagittal; repetition time (TR): 2530 ms; first echo time (TE): 1.64 ms; echo spacing ΔTE: 1.86 ms; flip angle: 7°; 176 slices; slice thickness: 1 mm; no gap; matrix: 256 × 256; field of view (FoV): 256 mm; in-plane resolution: 1 mm× 1 mm; duration: 363 s].
Pre-processing
Pre-processing for the voxel-based morphometry (VBM) and the surface-based morphometry (SBM) analyses were performed using the SPM12 package (Statistical Parametric Mapping, Institute of Neurology, London, United Kingdom) and the CAT12 Toolbox1 running on MATLAB version R2016a (MathWorks, Inc., Natick, MA, United States). For VBM, T1-weighted images were segmented into GM, white matter (WM), and cerebrospinal fluid (CSF) using age-appropriate stereotaxic tissue probability maps (NIHPD 7.5-13.5 asymmetric2; Fonov et al., 2011). Pediatric templates were used to minimize the potential confounds introduced by developmental differences in cortical morphometry (Yoon et al., 2009). Next, the segments were spatially normalized to the Montreal Neurological Institute (MNI) space with a voxel size of 1.5 mm × 1.5 mm × 1.5 mm. Finally, the resulting GM maps were modulated and smoothed with 8-mm full-width-at-half-maximum (FWHM) smoothing kernels. For SBM, T1-weighted images were segmented and spatially normalized as for VBM. The cortical surface was reconstructed from volumetric data using the projection-based thickness method. The cortical thickness maps were resampled onto the cortical surface and smoothed with a standard 15-mm FWHM smoothing kernel.
Statistical Analyses
The threshold-free cluster enhancement (TFCE) method implemented in CAT12 was used to identify statistically significant clusters. TFCE is a cluster-based thresholding method that overcomes the problem of choosing an arbitrary cluster-forming threshold, while keeping the sensitivity advantage of cluster-based thresholding (Smith and Nichols, 2009). TFCE uses a permutation approach that maximizes statistical power in small sample studies (Pernet et al., 2015). Using 5,000 permutations and non-parametric testing, a voxel-wise p-value map is produced. An explicit GM mask based on the mean normalized GM images of all participants was used to ensure that the analyses were restricted to GM. Resulting statistical maps were thresholded at p < 0.05 corrected for multiple comparisons by false discovery rate (FDR; Chumbley et al., 2010).
Main Analyses
The main analyses focused on GM volume and thickness. A multiple regression analysis was performed using CAT12 to predict GM volumes in late childhood from attachment security in infancy, after accounting for confounding variables (described below). Similar analyses were performed to predict cortical thickness, and right and left hemispheres were analyzed separately. In order to account for differences in overall brain size, total intracranial volume (ICV) was controlled for in the VBM analyses (Barnes et al., 2010; Malone et al., 2015). As ICV is not related to cortical thickness (Toro et al., 2008; Winkler et al., 2010), it was not controlled for in the SBM analyses. Child age and sex, pubertal status, as well as maternal education are associated with cortical volume and thickness (Barnes et al., 2010; Blakemore et al., 2010; Jednoróg et al., 2012), and were therefore included as covariates in both the VBM and SBM analyses.
The AQS score was missing for one child. In line with recommendations for best practices for handling missing data, multiple imputation was employed to estimate the missing value (Enders, 2010) using the Markov Chain Monte Carlo procedure (Geyer, 1992) in SPSS software version 24.0 (IBM Corp., Armonk, NY, United States). Ten imputations were used and then averaged to maximize the precision of imputed data (Graham, 2009; Enders, 2010). To reach maximal accuracy, the imputations were performed based on the original 64 families using child sex and age at T1, as well as parental age and education at the time of recruitment as predictors in the imputation equation.
Results
Descriptive Statistics
At the time of initial recruitment (when children were 7 months old; n = 33), mothers and fathers were, respectively, on average 31.73 (SD = 5.10) and 33.27 (SD = 5.00) years old and had on average 15.36 (SD = 2.28; range 10–18) and 15.58 (SD = 2.00; range 11–17) years of education. The families’ average income fell in the $60,000 to $79,000 bracket. The majority of mothers (78.80%) and fathers (75.80%) were Caucasian. Most families had French as their first language (78.80%). Attachment security scores at T1 varied from -0.28 to 0.75 (M = 0.49, SD = 0.26).
At T2, almost half of the children (45%) were pre-pubertal, two were early pubertal (6%), 15 were mid-pubertal (45%), and one was post-pubertal (3%). Average brain volumetric data were: 1529.34 cm3 (SD = 106.58, range = 1317.63–1854.37) for total ICV; 833.27 cm3 (SD = 53.40, range = 725.37–987.76) for total GM volume; 442.40 cm3 (SD = 44.44, range = 367.07–552.58) for total WM volume; and 253.67 cm3 (SD = 23.68, range = 205.73–314.04) for total CSF. Table 2 displays the bivariate correlations among attachment security, child age and sex, pubertal status, maternal education, and volumetric data (total ICV, total GM volume, total WM volume, and total CSF). No outliers were identified on any of the attachment or anatomical measures.
Voxel-Based Morphometry
Multiple regression analysis indicated that after accounting for child age, sex, pubertal status, maternal education, and total ICV, children who were more securely attached to their mother in infancy had larger GM volumes in the right hemisphere covering the superior temporal sulcus and gyrus, extending to the middle temporal gyrus, and into the temporo-parietal junction. Increased GM volume in the left superior temporal sulcus and in the bilateral precentral gyri was also related to higher attachment quality (see Figure 1 and Table 3). No significant supra-threshold voxels were found for negative contrasts.
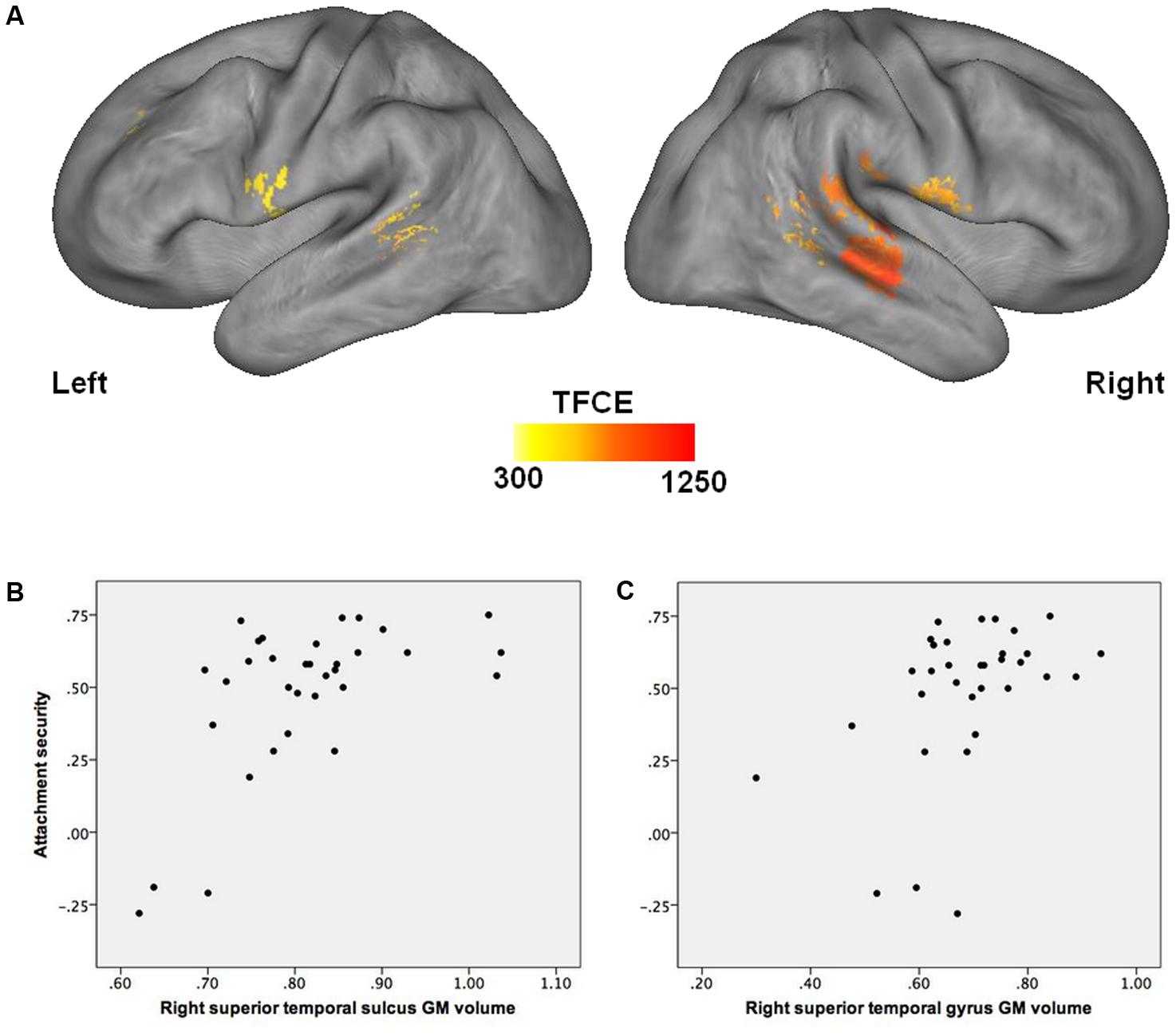
FIGURE 1. Association between attachment security in infancy and GM volume in late childhood. (A) Higher attachment security in infancy is associated with greater GM volume in the right superior temporal sulcus and gyrus, temporo-parietal junction, and precentral gyrus, as well as in the left superior temporal sulcus and precentral gyrus (FDR corrected, p < 0.05), after accounting for child age, sex, pubertal status, maternal education, and total intracranial volume. (B) Correlation between attachment security in infancy and GM volume in the right superior temporal sulcus in late childhood [x = 45; y = –21; z = –4]. (C) Correlation between attachment security in infancy and GM volume in the right superior temporal gyrus in late childhood [x = 68; y = –33; z = 20]. GM, gray matter.
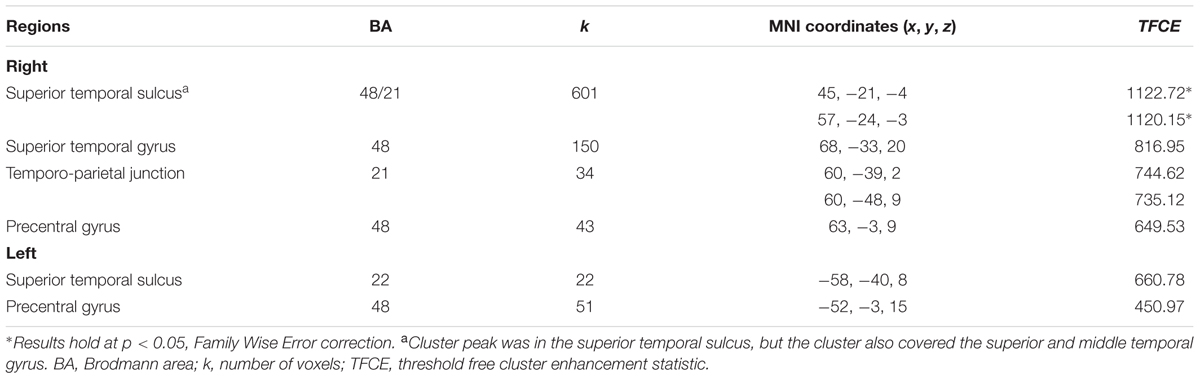
TABLE 3. Regional volumes significantly associated with attachment security in infancy (p < 0.05, False Discovery Rate correction).
Surface-Based Morphometry
Multiple regression analysis indicated that attachment security in infancy was not significantly related to cortical thickness in late childhood, neither positively nor negatively, over and above child age, sex, pubertal status, and maternal education (p > 0.001, uncorrected).
Discussion
To our knowledge, this is the first study to examine the prospective associations between attachment security in infancy and whole-brain GM volume and thickness in childhood. The main findings indicate that children who were more securely attached to their mother in infancy (15 months) had larger GM volume in the bilateral superior temporal sulci, right superior temporal gyrus, right temporo-parietal junction, and the bilateral precentral gyri in late childhood (10–11 years). These results survived a multiple comparisons correction after controlling for several potentially confounding variables. No significant association was found between attachment security and cortical thickness. Consistent with animal studies indicating that enriched caregiving is associated with optimal brain development (Greenough et al., 1987; Van Praag et al., 2000; Meaney, 2001), the current study provides rare data in humans consistent with the idea that attachment relationships may affect children’s brain development, as reflected by larger GM volume in the frontal and temporal lobes. Moreover, these results contribute to the emerging literature indicating that variations in the quality of caregiving experiences within the normative range are associated with child brain morphology (Luby et al., 2012, 2016; Whittle et al., 2014, 2016; Kok et al., 2015, 2017).
Specifically, better quality mother–child relationships in infancy were found to be predictive of larger GM volume in the superior and middle temporal gyri, superior temporal sulci, temporo-parietal junction, and precentral gyri in late childhood. This appears to be the first evidence of a relation between a direct observational measure of caregiving quality (whether parenting or attachment) and GM volume in these specific brain regions. The lack of prior comparable findings may be partly expected, given that no previous studies have investigated the association between parent–child relationship quality or parental behaviors and whole-brain GM volume in a pediatric community sample. However, the current findings are broadly consistent with studies reporting smaller GM volume or surface in the superior and middle temporal gyri of maltreated children (Hanson et al., 2010; De Brito et al., 2013; Kelly et al., 2013, 2015; Lim et al., 2014). These areas are critical for processing emotional stimuli (Allison et al., 2000), a function that is impaired in maltreated children, as indicated by event-related potential and functional MRI studies (see da Silva Ferreira et al., 2014 for a systematic review; Pollak et al., 2001). Importantly, the preliminary results presented here suggest that even normative variations in relationship quality may have a long-lasting impact on the development of these brain regions. This is a promising first step, but independent replication is necessary.
In contrast to previous studies reporting an association between parental behavior and cortical thickness in typically developing children (Whittle et al., 2014, 2016; Kok et al., 2015), no significant association was found in this sample between attachment security in infancy and cortical thickness in late childhood. Methodological differences, such as the modest sample size and related limited statistical power in the current study, may account for this discrepancy. Developmental considerations may also be at play. Brain volume and thickness follow an inverted U-shape developmental trajectory characterized by an increase during childhood, a region-specific peak in late childhood and early adolescence, and a subsequent decrease (Shaw et al., 2008; Giedd et al., 2015). Results reported here are age-specific (10–11 years); in a younger or older sample, results may be different. Previous work by Kok et al. (2015) indicates that maternal sensitivity in early childhood is associated with brain volume and thickness in 8-year-old children. Moreover, as Teicher and Samson (2016) underscore, caregiving experiences may not relate to brain structures at one specific period of development, but rather, they may be associated with the trajectory of brain development over time (see also Whittle et al., 2014). Thus, one intriguing possibility that could be investigated in future work is that attachment security may not relate to cortical thickness at specific ages, but rather to the rhythm of cortical thickening and then thinning over time. Alternatively, the findings may be theoretically meaningful, indicating for instance that, although related to an extent, parenting behavior and parent–child attachment may have a different impact on brain development. Of note, we did not find links between attachment and amygdalar volume as observed by Moutsiana et al. (2015) and Lyons-Ruth et al. (2016). In addition to the different composition of the samples studied and the different attachment measure used, developmental considerations may again underlie discrepant findings, given that the previous studies found links between early attachment and amygdalar volume in adulthood. Longitudinal designs including repeated MRI would be useful to more accurately depict the developmental aspects of the brain-attachment associations.
Attachment and the Developing Brain: Proposed Mechanisms
Children more securely attached to their primary caregivers are exposed to a variety of experiences that differ from those characterizing insecurely attached children. These experiences may influence children’s brain development in regions involved in social, cognitive, and emotional functioning. A central way in which the experience of securely attached infants differs from that of their insecurely attached counterparts is with regards to the quality of the emotion regulation provided by the caregiver. Indeed, one of the hallmarks of a secure attachment relationship is the caregiver’s capacity to provide adequate external regulation when the infant encounters an affectively challenging situation during exploration (e.g., frustration when faced with a complex toy, fear of a large dog in the park). As a result, securely attached children are exposed to repeated experiences of successful regulation in emotionally taxing situations, which provides a strong basis for the gradual development of self-regulation (Calkins, 2004; Cole et al., 2004). The superior and middle temporal gyri are activated when subjects need to down-regulate their negative affect (Ochsner et al., 2004; Frank et al., 2014), and extensive evidence from human and non-human primates points to a crucial role for the superior temporal gyrus and sulcus for processing emotional faces stimuli (Britton et al., 2006; Pagliaccio et al., 2013). If replicated, the current findings would suggest that the repeated experiences of successful emotion regulation that characterize secure attachment relationships may promote optimal development in brain regions that subsume socio-emotional regulation, such as the superior and middle temporal gyri, through experience-dependent processes.
An alternative hypothesis for the observed relation between attachment and brain structure pertains to one of the central notions of attachment theory, that of “internal working models” (Bowlby, 1969/1982, 1973, 1980, 1988). These models consist of mental representations of self and others, which are thought to be shaped by daily interactions with primary caregivers. The repeated experiences of responsive care that characterize secure attachment are believed to promote the development of positive internal working models of self and others (Bretherton and Munholland, 2016). It is theorized that these models are progressively internalized, becoming an integral part of the child’s personality, and are increasingly generalized to new relationships, guiding behavior and interpretation in new social situations and helping children correctly anticipate future social interactions (Bretherton and Munholland, 2016). Empirical evidence indeed shows that securely attached infants develop positive expectations about social interactions (Johnson et al., 2010; Biro et al., 2015). Importantly, the superior and middle temporal gyri, temporo-parietal junction, and precentral gyrus are involved in the representation and elaboration of past and future events (Kelley et al., 2002; Addis et al., 2007; Spreng et al., 2009; Holland et al., 2011; Jacques et al., 2011) and representation of self and others (Ruby and Decety, 2001; Ochsner et al., 2004). The positive expectations about social relationships characterizing secure attachment working models may lead securely attached children to engage more confidently in social interactions. Thus, these children are likely to be more frequently engaged in stimulating social interactions which may result in recurrent activation of brain regions involved in the representation of self and others in social contexts. As such, secure attachment could promote the optimal structural development of the superior and middle temporal gyri, temporo-parietal junction, and precentral gyrus.
Lastly, social perception and social cognition may play a role in the attachment-brain structure links uncovered here. Social perception is an important basis for the development of attachment relationships. In order to effectively attain a caregiver’s proximity, children have to adapt their attachment behaviors toward their caregivers according to the context, caregiver location, and the specific characteristics of the caregiver with whom they are interacting (Cassidy, 2016; Sroufe, 2016). Recognizing the caregiver’s face and affective state as well as following his or her eye gaze and movements support the contextual adaptation of infant attachment behavior for proximity seeking. As such, empirical evidence indicates that higher levels of attachment security in infancy are associated with better emotion recognition skills up to 10 years later (Steele et al., 2008). Assuming that future research replicates the current results, the association between attachment security and GM volume in the superior and middle temporal gyri may therefore be related to the importance of these brain regions for social perception, such as the detection of faces, eye gaze, and biological motion (Puce et al., 1998; Allison et al., 2000; Haxby et al., 2000; Engell and Haxby, 2007; Saygin, 2007). It is possible that securely attached children are more successful in adapting their attachment behaviors to the context by recruiting temporal regions involved in social perception, which in turn promotes the development of these regions. The value of attachment security for complex social cognitive processes and social functioning is also well established (Thompson, 2016), and numerous studies have underscored the role of the superior temporal sulcus and gyrus, middle temporal gyrus, and the temporo-parietal junction in theory of mind, moral reasoning, and empathy (see Bzdok et al., 2012, for a meta-analysis). Larger GM volumes in the superior and middle temporal gyri have been related to more optimal social skills, such as better emotion recognition (Shdo et al., 2017) and better ability to predict others’ behavior based on mental states (Powell et al., 2014). Conversely, reduced GM thickness or volume in the superior and middle temporal gyri has been associated with lack of empathy and compassion and severity of conduct disorder symptomatology (Huebner et al., 2008; Fahim et al., 2011; Wallace et al., 2014). Overall, these studies suggest that GM volume and functional activity in the superior temporal sulcus and gyrus, middle temporal gyrus, and temporo-parietal junction are closely linked with social cognition and social functioning, of which attachment security is a well-known predictor (Thompson, 2016). Social cognitive experiences embedded in secure attachment relationships provide children with a more sophisticated understanding of the psychological dimensions of social interactions (Thompson, 2016) and may therefore contribute to shaping the structural development of regions involved in social cognition, such as the superior temporal sulcus and gyrus, the middle temporal gyrus, and the temporo-parietal junction.
Limitations
The results presented here must be interpreted in the context of some limitations. First, the longitudinal but non-experimental design precludes causal inference and determination of directionality. The possibility that larger GM volumes in the superior and middle temporal gyri, the superior temporal sulci, the temporo-parietal junction, and the precentral gyri were already present in these children in infancy, and may have predisposed them to develop secure attachment to their mothers, cannot be excluded. In fact, given that developmental processes are transactional by nature (Sameroff, 2009), it is reasonable to expect that any caregiving-brain associations are probably bidirectional, reflecting the action of mutual reciprocal influences between parent and child (Serbin et al., 2015). The non-experimental design also leaves open some third-variable explanations, notably the possibility that shared genes between mother and child may be partly responsible for the links observed. This is unlikely to have played a major role in the current results though, given that several genetically informed studies show that the variance in mother–child attachment security (O’Connor and Croft, 2001; Bokhorst et al., 2003; Roisman and Fraley, 2008) and the variance in maternal caregiving behavior (Roisman and Fraley, 2008) are almost entirely attributable to environmental influences, with small to negligible genetic contributions. Other third-variable explanations are possible though, one of which being that caregiving experiences (e.g., exposure to higher parental sensitivity) could influence both the quality of attachment relationships and the development of corresponding brain regions. Second, the small sample size and the use of several covariates reduced statistical power, potentially leading to underestimation of the links between attachment and brain volumes and thickness. Clearly, replication in larger independent samples is necessary to confirm the links reported here, especially for clusters in the right temporo-parietal junction and precentral gyrus, as well as in the left precentral gyrus and superior temporal sulcus, due to the small number of voxels contained in these clusters. Third, the attachment measure used in the current study does not allow the assessment of attachment disorganization (the most extreme form of attachment insecurity, assessed exclusively through the SSP), which one study found to be related to amygdalar volume (Lyons-Ruth et al., 2016). Disorganized attachment relationships are associated with the development of psychopathology, poor emotion regulation skills, and poor relationships with peers and adults (Lyons-Ruth and Jacobvitz, 2016), which could be reflected in children’s brain morphology, especially in brain regions known to be involved in socio-emotional functioning (limbic system, social brain). Fourth, we did not assess father–child attachment security, which may differentially influence brain development given that fathers have unique contributions to children’s social and cognitive development (see Cabrera and Tamis-LeMonda, 2013). However, Kok et al. (2015) reported that the associations between parental sensitivity and brain morphology were similar for mothers and fathers in their sample.
Conclusion
This 9-year longitudinal study suggests that better mother–child attachment quality in infancy is related to greater GM volume in the superior temporal sulcus and gyrus, the temporo-parietal junction, and precentral gyrus in late childhood, whereas no associations with measures of cortical thickness were found. This appears to be the first study to investigate the link between infant-caregiver attachment quality and brain morphometry in childhood. The use of a gold-standard observational measure of attachment security in the ecological context of the family home, along with whole-brain analyses using a pediatric template, enabled the identification of novel associations between attachment and brain regions involved in social, cognitive, and emotional functioning, and these associations were robust to several important covariates. Although preliminary and in need of replication, the present results provide further evidence that infant quality of attachment toward a primary caregiver is important not only for children’s social, emotional, and cognitive functioning, but may also be involved in their brain development. As in previous studies focusing on maltreatment (e.g., da Silva Ferreira et al., 2014; Puetz et al., 2017), future research in normative samples could test, using other methodologies (e.g., diffusion tensor imaging, functional connectivity, event-related potentials), the breadth of the links between attachment security in infancy and brain morphology and functions.
Ethics Statement
The research ethics committee of aging-neuroimaging of the CIUSSS du Centre-Sud-de-l’île-de-Montréal approved the study. The goal of the study was explained to children and their parents, who signed a detailed inform consent form. We report how we determined our sample size, all manipulations, and all measures in the study.
Author Contributions
ÉL participated in data collection, performed statistical analyses, and drafted the initial manuscript. AB designed the study, wrote parts of the manuscript and revised it for intellectual content. MB contributed to designing the study, edited and revised the manuscript for intellectual content. FD contributed to data analyses and interpretation, wrote parts of the manuscript and revised it for intellectual content. VD participated in data collection and methodological choices, and revised the manuscript for intellectual content. All authors gave their final approval of the manuscript to be published and agreed to be accountable for all aspects of the work.
Funding
This work was supported by grants awarded to AB by the Canadian Institutes of Health Research [MOP-119390], the Social Sciences and Humanities Research Council of Canada [410-2010-1366], and the Fonds de Recherche du Québec - Société et Culture [2012-RP-144923].
Conflict of Interest Statement
The authors declare that the research was conducted in the absence of any commercial or financial relationships that could be construed as a potential conflict of interest.
Acknowledgments
The authors wish to acknowledge Natasha Ballen, Marie-Ève Bélanger, Stéphanie Bordeleau, Andrée-Anne Bouvette-Turcot, Catherine Cimon-Paquet, Isabelle Demers, Marie Deschênes, Christine Gagné, Sarah Hertz, Véronique Jarry-Boileau, Jessica Laranjo, Élodie Larose-Grégoire, Nadine Marzougui, Célia Matte-Gagné, Rachel Perrier, Émilie Rochette, Marie-Soleil Sirois, Émilie Tétreault, and Natasha Whipple for help with data collection. The authors also want to express special gratitude to the participating families of the Grandir Ensemble project who generously opened their homes to us. The authors thank Andre van der Kouwe from the Massachusetts General Hospital for the use of the MPRAGE 4-echo sequence.
Footnotes
References
Addis, D. R., Wong, A. T., and Schacter, D. L. (2007). Remembering the past and imagining the future: common and distinct neural substrates during event construction and elaboration. Neuropsychologia 45, 1363–1377. doi: 10.1016/j.neuropsychologia.2006.10.016
Adolphs, R. (2009). The social brain: neural basis of social knowledge. Annu. Rev. Psychol. 60, 693–716. doi: 10.1146/annurev.psych.60.110707.163514
Ainsworth, M. D. S. (1967). Infancy in Uganda: Infant Care and the Growth of Attachment. Baltimore, MD: Johns Hopkins University Press.
Ainsworth, M. D. S. (1985). Patterns of infant–mother attachment: antecedents and effects on development. Bull. N. Y. Acad. Med. 61, 771–791.
Ainsworth, M. D. S., Blehar, M. C., Waters, E., and Wall, S. (1978). Patterns of Attachment. Hillsdale, NJ: Lawrence Erlbaum Associates.
Allison, T., Puce, A., and McCarthy, G. (2000). Social perception from visual cues: role of the STS region. Trends Cogn. Sci. 4, 267–278. doi: 10.1016/S1364-6613(00)01501-1
Barnes, J., Ridgway, G. R., Bartlett, J., Henley, S. M., Lehmann, M., Hobbs, N., et al. (2010). Head size, age and gender adjustment in MRI studies: a necessary nuisance? Neuroimage 53, 1244–1255. doi: 10.1016/j.neuroimage.2010.06.025
Bélanger, M.-É., Bernier, A., Simard, V., Bordeleau, S., and Carrier, J. (2015). Objective and subjective measures of sleep among preschoolers: disentangling attachment security and dependency. Monogr. Soc. Res. Child Dev. 80, 125–140. doi: 10.1111/mono.12148
Belsky, J., and de Haan, M. (2011). Annual research review: parenting and children’s brain development: the end of the beginning. J. Child Psychol. Psychiatry 52, 409–428. doi: 10.1111/j.1469-7610.2010.02281.x
Biro, S., Alink, L. R., Huffmeijer, R., Bakermans-Kranenburg, M. J., and Van IJzendoorn, M. H. (2015). Attachment and maternal sensitivity are related to infants’ monitoring of animated social interactions. Brain Behav. 5:e00410. doi: 10.1002/brb3.410
Blakemore, S. J. (2008). The social brain in adolescence. Nat. Rev. Neurosci. 9, 267–277. doi: 10.1038/nrn2353
Blakemore, S. J., Burnett, S., and Dahl, R. E. (2010). The role of puberty in the developing adolescent brain. Hum. Brain Mapp. 31, 926–933. doi: 10.1002/hbm.21052
Bokhorst, C. L., Bakermans-Kranenburg, M. J., Fearon, R. P., Van IJzendoorn, M. H., Fonagy, P., and Schuengel, C. (2003). The importance of shared environment in mother–infant attachment security: a behavioral genetic study. Child Dev. 74, 1769–1782. doi: 10.1046/j.1467-8624.2003.00637.x
Bowlby, J. (1988). A Secure Base: Clinical Applications of Attachment Theory. New York, NY: Basic Books.
Bretherton, I., and Munholland, K. A. (2016). “The internal working model construct in light of contemporary neuroimaging research,” in Handbook of Attachment: Theory, Research, and Clinical Applications, eds J. Cassidy and P. R. Shaver (New York, NY: Guilford Press), 102–127.
Britton, J. C., Phan, K. L., Taylor, S. F., Welsh, R. C., Berridge, K. C., and Liberzon, I. (2006). Neural correlates of social and nonsocial emotions: an fMRI study. Neuroimage 31, 397–409. doi: 10.1016/j.neuroimage.2005.11.027
Bzdok, D., Schilbach, L., Vogeley, K., Schneider, K., Laird, A. R., Langner, R., et al. (2012). Parsing the neural correlates of moral cognition: ALE meta-analysis on morality, theory of mind, and empathy. Brain Struct. Funct. 217, 783–796. doi: 10.1007/s00429-012-0380-y
Cabrera, N. J., and Tamis-LeMonda, C. S. (2013). Handbook of Father Involvement: Multidisciplinary Perspectives. New York, NY: Taylor and Francis.
Cadman, T., Diamond, P. R., and Fearon, R. P. (2017). Reassessing the validity of the attachment Q-sort: an updated meta-analysis. Infant Child Dev. (in press). doi: 10.1002/icd.2034
Calkins, S. D. (2004). “Early attachment processes and the development of self regulation,” in Handbook of Self Regulation: Research, Theory and Applications, eds R. F. Baumeister and K. D. Vohs (New York, NY: Guilford Press), 324–339.
Carskadon, M. A., and Acebo, C. (1993). A self-administered rating scale for pubertal development. J. Adolesc. Health 14, 190–195. doi: 10.1016/1054-139X(93)90004-9
Cassidy, J. (2016). “The nature of child’s tie,” in Handbook of Attachment: Theory, Research, and Clinical Applications, eds J. Cassidy and P. R. Shaver (New York, NY: Guilford Press), 3–24.
Chumbley, J., Worsley, K., Flandin, G., and Friston, K. (2010). Topological FDR for neuroimaging. Neuroimage 49, 3057–3064. doi: 10.1016/j.neuroimage.2009.10.090
Coan, J. A. (2016). “Toward a neuroscience of attachment,” in Handbook of Attachment: Theory, Research, and Clinical Applications, eds J. Cassidy and P. R. Shaver (New York, NY: Guilford Press), 242–269.
Cole, P. M., Martin, S. E., and Dennis, T. A. (2004). Emotion regulation as a scientific construct: challenges and directions for child development research. Child Dev. 75, 317–333. doi: 10.1111/j.1467-8624.2004.00673.x
Cooke, J. E., Stuart-Parrigon, K. L., Movahed-Abtahi, M., Koehn, A. J., and Kerns, K. A. (2016). Children’s emotion understanding and mother–child attachment: a meta-analysis. Emotion 16, 1102–1106. doi: 10.1037/emo0000221
Cuddihy, C., Dorris, L., Minnis, H., and Kocovska, E. (2013). Sleep disturbance in adopted children with a history of maltreatment. Adopt. Fostering 37, 404–411. doi: 10.1177/0308575913508715
Cyr, C., Euser, E. M., Bakermans-Kranenburg, M. J., and Van IJzendoorn, M. H. (2010). Attachment security and disorganization in maltreating and high-risk families: a series of meta-analyses. Dev. Psychopathol. 22, 87–108. doi: 10.1017/S0954579409990289
da Silva Ferreira, G. C., Crippa, J. A., and de Lima Osorio, F. (2014). Facial emotion processing and recognition among maltreated children: a systematic literature review. Front. Psycholol. 5:1460. doi: 10.3389/fpsyg.2014.01460
De Brito, S. A., Viding, E., Sebastian, C. L., Kelly, P. A., Mechelli, A., Maris, H., et al. (2013). Reduced orbitofrontal and temporal grey matter in a community sample of maltreated children. J. Child Psychol. Psychiatry 54, 105–112. doi: 10.1111/j.1469-7610.2012.02597.x
De Wolff, M. S., and Van IJzendoorn, M. H. (1997). Sensitivity and attachment: a meta-analysis on parental antecedents of infant attachment. Child Dev. 68, 571–591. doi: 10.1111/j.1467-8624.1997.tb04218.x
Deen, B., Koldewyn, K., Kanwisher, N., and Saxe, R. (2015). Functional organization of social perception and cognition in the superior temporal sulcus. Cereb. Cortex 25, 4596–4609. doi: 10.1093/cercor/bhv111
Dölen, G., and Malenka, R. C. (2014). The emerging role of nucleus accumbens oxytocin in social cognition. Biol. Psychiatry 76, 354–355. doi: 10.1016/j.biopsych.2014.06.009
Edwards, V. J., Holden, G. W., Felitti, V. J., and Anda, R. F. (2003). Relationship between multiple forms of childhood maltreatment and adult mental health in community respondents: results from the adverse childhood experiences study. Am. J. Psychiatry 160, 1453–1460. doi: 10.1176/appi.ajp.160.8.1453
Eisenberger, N. I., and Cole, S. W. (2012). Social neuroscience and health: neurophysiological mechanisms linking social ties with physical health. Nat. Neurosci. 15, 669–674. doi: 10.1038/nn.3086
Engell, A. D., and Haxby, J. V. (2007). Facial expression and gaze-direction in human superior temporal sulcus. Neuropsychologia 45, 3234–3241. doi: 10.1016/j.neuropsychologia.2007.06.022
Fahim, C., He, Y., Yoon, U., Chen, J., Evans, A., and Pérusse, D. (2011). Neuroanatomy of childhood disruptive behavior disorders. Aggress. Behav. 37, 326–337. doi: 10.1002/ab.20396
Fearon, R. P., Bakermans-Kranenburg, M. J., Van IJzendoorn, M. H., Lapsley, A. M., and Roisman, G. I. (2010). The significance of insecure attachment and disorganization in the development of children’s externalizing behavior: a meta-analytic study. Child Dev. 81, 435–456. doi: 10.1111/j.1467-8624.2009.01405.x
Fearon, R. P., and Belsky, J. (2016). “Precursors of attachment security,” in Handbook of Attachment: Theory, Research, and Clinical Applications, eds J. Cassidy and P. R. Shaver (New York, NY: Guilford Press), 291–313.
Fonov, V., Evans, A. C., Botteron, K., Almli, C. R., McKinstry, R. C., Collins, D. L., et al. (2011). Unbiased average age-appropriate atlases for pediatric studies. Neuroimage 54, 313–327. doi: 10.1016/j.neuroimage.2010.07.033
Fraley, R. C., and Spieker, S. J. (2003). Are infant attachment patterns continuously or categorically distributed? A taxometric analysis of strange situation behavior. Dev. Psychol. 39, 387–404. doi: 10.1037/0012-1649.39.3.387
Frank, D. W., Dewitt, M., Hudgens-Haney, M., Schaeffer, D. J., Ball, B. H., Schwarz, N. F., et al. (2014). Emotion regulation: quantitative meta-analysis of functional activation and deactivation. Neurosci. Biobehav. Rev. 45, 202–211. doi: 10.1016/j.neubiorev.2014.06.010
Giedd, J. N., Raznahan, A., Alexander-Bloch, A., Schmitt, E., Gogtay, N., and Rapoport, J. L. (2015). Child psychiatry branch of the National Institute of Mental Health longitudinal structural magnetic resonance imaging study of human brain development. Neuropsychopharmacology 40, 43–49. doi: 10.1038/npp.2014.236
Goodkind, M., Eickhoff, S. B., Oathes, D. J., Jiang, Y., Chang, A., Jones-Hagata, L. B., et al. (2015). Identification of a common neurobiological substrate for mental illness. JAMA Psychiatry 72, 305–315. doi: 10.1001/jamapsychiatry.2014.2206
Graham, J. W. (2009). Missing data analysis: making it work in the real world. Annu. Rev. Psychol. 60, 549–576. doi: 10.1146/annurev.psych.58.110405.085530
Greenough, W. T., Black, J. E., and Wallace, C. S. (1987). Experience and brain development. Child Dev. 58, 539–559.
Groh, A. M., Fearon, R. P., Bakermans-Kranenburg, M. J., Van IJzendoorn, M. H., Steele, R. D., and Roisman, G. I. (2014). The significance of attachment security for children’s social competence with peers: a meta-analytic study. Attach. Hum. Dev. 16, 103–136. doi: 10.1080/14616734.2014.883636
Groh, A. M., Fearon, R. P., Van IJzendoorn, M. H., Bakermans-Kranenburg, M. J., and Roisman, G. I. (2017a). Attachment in the early life course: meta-analytic evidence for its role in socioemotional development. Child Dev. Perspect. 11, 70–76. doi: 10.1111/cdep.12213
Groh, A. M., Propper, C., Mills-Koonce, R., Moore, G. A., Calkins, S., and Cox, M. (2017b). Mothers’ physiological and affective responding to infant distress: unique antecedents of avoidant and resistant attachments. Child Dev. doi: 10.1111/cdev.12912 [Epub ahead of print].
Groh, A. M., Roisman, G. I., Van IJzendoorn, M. H., Bakermans-Kranenburg, M. J., and Fearon, R. P. (2012). The significance of insecure and disorganized attachment for children’s internalizing symptoms: a meta-analytic study. Child Dev. 83, 591–610. doi: 10.1111/j.1467-8624.2011.01711.x
Gunnar, M. R., Fisher, P. A., and Early Experience Stress and Prevention Science Network. (2006). Bringing basic research on early experience and stress neurobiology to bear on preventive interventions for neglected and maltreated children. Dev. Psychopathol. 18, 651–677. doi: 10.1017/S0954579406060330
Hanson, J. L., Chung, M. K., Avants, B. B., Shirtcliff, E. A., Gee, J. C., Davidson, R. J., et al. (2010). Early stress is associated with alterations in the orbitofrontal cortex: a tensor-based morphometry investigation of brain structure and behavioral risk. J. Neurosci. 30, 7466–7472. doi: 10.1523/jneurosci.0859-10.2010
Haxby, J. V., Hoffman, E. A., and Gobbini, M. I. (2000). The distributed human neural system for face perception. Trends Cogn. Sci. 4, 223–233. doi: 10.1016/S1364-6613(00)01482-0
Holland, A. C., Addis, D. R., and Kensinger, E. A. (2011). The neural correlates of specific versus general autobiographical memory construction and elaboration. Neuropsychologia 49, 3164–3177. doi: 10.1016/j.neuropsychologia.2011.07.015
Huebner, T., Vloet, T. D., Marx, I., Konrad, K., Fink, G. R., Herpertz, S. C., et al. (2008). Morphometric brain abnormalities in boys with conduct disorder. J. Am. Acad. Child Adolesc. Psychiatry 47, 540–547. doi: 10.1097/CHI.0b013e3181676545
Hussey, J. M., Chang, J. J., and Kotch, J. B. (2006). Child maltreatment in the United States: prevalence, risk factors, and adolescent health consequences. Pediatrics 118, 933–942. doi: 10.1542/peds.2005-2452
Jacques, P. L. S., Conway, M. A., Lowder, M. W., and Cabeza, R. (2011). Watching my mind unfold versus yours: an fMRI study using a novel camera technology to examine neural differences in self-projection of self versus other perspectives. J. Cogn. Neurosci. 23, 1275–1284. doi: 10.1162/jocn.2010.21518
Jednoróg, K., Altarelli, I., Monzalvo, K., Fluss, J., Dubois, J., Billard, C., et al. (2012). The influence of socioeconomic status on children’s brain structure. PLOS ONE 7:e42486. doi: 10.1371/journal.pone.0042486
Johnson, S. C., Dweck, C. S., Chen, F. S., Stern, H. L., Ok, S. J., and Barth, M. (2010). At the intersection of social and cognitive development: internal working models of attachment in infancy. Cogn. Sci. 34, 807–825. doi: 10.1111/j.1551-6709.2010.01112.x
Kalisch, R., and Gerlicher, A. M. (2014). Making a mountain out of a molehill: on the role of the rostral dorsal anterior cingulate and dorsomedial prefrontal cortex in conscious threat appraisal, catastrophizing, and worrying. Neurosci. Biobehav. Rev. 42, 1–8. doi: 10.1016/j.neubiorev.2014.02.002
Kelley, W. M., Macrae, C. N., Wyland, C. L., Caglar, S., Inati, S., and Heatherton, T. F. (2002). Finding the self? An event-related fMRI study. J. Cogn. Neurosci. 14, 785–794. doi: 10.1162/08989290260138672
Kelly, P. A., Viding, E., Puetz, V. B., Palmer, A. L., Mechelli, A., Pingault, J. B., et al. (2015). Sex differences in socioemotional functioning, attentional bias, and gray matter volume in maltreated children: a multilevel investigation. Dev. Psychopathol. 27(4 Pt 2), 1591–1609. doi: 10.1017/S0954579415000966
Kelly, P. A., Viding, E., Wallace, G. L., Schaer, M., De Brito, S. A., Robustelli, B., et al. (2013). Cortical thickness, surface area, and gyrification abnormalities in children exposed to maltreatment: neural markers of vulnerability? Biol. Psychiatry 74, 845–852. doi: 10.1016/j.biopsych.2013.06.020
Kok, R., Prinzie, P., Bakermans-Kranenburg, M. J., Verhulst, F. C., White, T., Tiemeier, H., et al. (2017). Socialization of prosocial behavior: gender differences in the mediating role of child brain volume. Child Neuropsychol. doi: 10.1080/09297049.2017.1338340 [Epub ahead of print].
Kok, R., Thijssen, S., Bakermans-Kranenburg, M. J., Jaddoe, V. W. V., Verhulst, F. C., White, T., et al. (2015). Normal variation in early parental sensitivity predicts child structural brain development. J. Am. Acad. Child Adolesc. Psychiatry 54, 824–831. doi: 10.1016/j.jaac.2015.07.009
Lévesque, M. L., Fahim, C., Ismaylova, E., Verner, M. P., Casey, K. F., Vitaro, F., et al. (2015). The impact of the in utero and early postnatal environments on grey and white matter volume: a study with adolescent monozygotic twins. Dev. Neurosci. 37, 489–496. doi: 10.1159/000430982
Lim, L., Radua, J., and Rubia, K. (2014). Gray matter abnormalities in childhood maltreatment: a voxel-wise meta-analysis. Am. J. Psychiatry 171, 854–863. doi: 10.1176/appi.ajp.2014.13101427
Luby, J. L., Barch, D. M., Belden, A., Gaffrey, M. S., Tillman, R., Babb, C., et al. (2012). Maternal support in early childhood predicts larger hippocampal volumes at school age. Proc. Natl. Acad. Sci. U.S.A. 109, 2854–2859. doi: 10.1073/pnas.1118003109
Luby, J. L., Belden, A., Botteron, K., Marrus, N., Harms, M. P., Babb, C., et al. (2013). The effects of poverty on childhood brain development: the mediating effect of caregiving and stressful life events. JAMA Pediatr. 167, 1135–1142. doi: 10.1001/jamapediatrics.2013.3139
Luby, J. L., Belden, A., Harms, M. P., Tillman, R., and Barch, D. M. (2016). Preschool is a sensitive period for the influence of maternal support on the trajectory of hippocampal development. Proc. Natl. Acad. Sci. U.S.A. 113, 5742–5747. doi: 10.1073/pnas.1601443113
Lyons-Ruth, K., and Jacobvitz, D. (2016). “Attachment disorganization from infancy to adulthood: neurobiological correlates, parenting contexts, and pathways to disorder,” in Handbook of Attachment: Theory, Research, and Clinical Applications, eds J. Cassidy and P. R. Shaver (New York, NY: The Guilford Press), 667–695.
Lyons-Ruth, K., Pechtel, P., Yoon, S. A., Anderson, C. M., and Teicher, M. H. (2016). Disorganized attachment in infancy predicts greater amygdala volume in adulthood. Behav. Brain Res. 308, 83–93. doi: 10.1016/j.bbr.2016.03.050
Madigan, S., Atkinson, L., Laurin, K., and Benoit, D. (2013). Attachment and internalizing behavior in early childhood: a meta-analysis. Dev. Psychol. 49, 672–689. doi: 10.1037/a0028793
Malone, I. B., Leung, K. K., Clegg, S., Barnes, J., Whitwell, J. L., Ashburner, J., et al. (2015). Accurate automatic estimation of total intracranial volume: a nuisance variable with less nuisance. Neuroimage 104, 366–372. doi: 10.1016/j.neuroimage.2014.09.034
Matsudaira, I., Yokota, S., Hashimoto, T., Takeuchi, H., Asano, K., Asano, M., et al. (2016). Parental praise correlates with posterior insular cortex gray matter volume in children and adolescents. PLOS ONE 11:e0154220. doi: 10.1371/journal.pone.0154220
Meaney, M. J. (2001). Maternal care, gene expression, and the transmission of individual differences in stress reactivity across generations. Annu. Rev. Neurosci. 24, 1161–1192. doi: 10.1146/annurev.neuro.24.1.1161
Moutsiana, C., Johnstone, T., Murray, L., Fearon, R. P., Cooper, P. J., Pliatsikas, C., et al. (2015). Insecure attachment during infancy predicts greater amygdala volumes in early adulthood. J. Child Psychol. Psychiatry 56, 540–548. doi: 10.1111/jcpp.12317
Ochsner, K. N., Knierim, K., Ludlow, D. H., Hanelin, J., Ramachandran, T., Glover, G., et al. (2004). Reflecting upon feelings: an fMRI study of neural systems supporting the attribution of emotion to self and other. J. Cogn. Neurosci. 16, 1746–1772. doi: 10.1162/0898929042947829
O’Connor, T. G., and Croft, C. M. (2001). A twin study of attachment in preschool children. Child Dev. 72, 1501–1511. doi: 10.1111/1467-8624.00362
Pagliaccio, D., Luby, J. L., Gaffrey, M. S., Belden, A. C., Botteron, K. N., and Harms, M. P. (2013). Functional brain activation to emotional and nonemotional faces in healthy children: evidence for developmentally undifferentiated amygdala function during the school-age period. Cogn. Affect. Behav. Neurosci. 13, 771–789. doi: 10.3758/s13415-013-0167-5
Pallini, S., Baiocco, R., Schneider, B. H., Madigan, S., and Atkinson, L. (2014). Early child–parent attachment and peer relations: a meta-analysis of recent research. J. Fam. Psychol. 28, 118–123. doi: 10.1037/a0035736
Pederson, D. R., and Moran, G. (1995). A categorical description of infant-mother relationships in the home and its relation to Q-sort measures of infant-mother interaction. Monogr. Soc. Res. Child Dev. 60, 111–132. doi: 10.1111/j.1540-5834.1995.tb00207.x
Peña, C. J., Neugut, Y. D., Calarco, C. A., and Champagne, F. A. (2014). Effects of maternal care on the development of midbrain dopamine pathways and reward-directed behavior in female offspring. Eur. J. Neurosci. 39, 946–956. doi: 10.1111/ejn.12479
Pernet, C. R., Latinus, M., Nichols, T. E., and Rousselet, G. A. (2015). Cluster-based computational methods for mass univariate analyses of event-related brain potentials/fields: a simulation study. J. Neurosci. Methods 250, 85–93. doi: 10.1016/j.jneumeth.2014.08.003
Pollak, S., Klorman, R., Thatcher, J., and Cicchetti, D. (2001). P3b reflects maltreated children’s reactions to facial displays of emotion. Psychophysiology 38, 267–274.
Powell, J. L., Kemp, G. J., Dunbar, R. I., Roberts, N., Sluming, V., and García-Fiñana, M. (2014). Different association between intentionality competence and prefrontal volume in left-and right-handers. Cortex 54, 63–76. doi: 10.1016/j.cortex.2014.02.010
Puce, A., Allison, T., Bentin, S., Gore, J. C., and McCarthy, G. (1998). Temporal cortex activation in humans viewing eye and mouth movements. J. Neurosci. 18, 2188–2199.
Puetz, V. B., Parker, D., Kohn, N., Dahmen, B., Verma, R., and Konrad, K. (2017). Altered brain network integrity after childhood maltreatment: a structural connectomic DTI-study. Hum. Brain Mapp. 38, 855–868. doi: 10.1002/hbm.23423
Rakic, P. (1988). Specification of cerebral cortex areas. Science 241, 170–176. doi: 10.1126/science.3291116
Rao, H., Betancourt, L., Giannetta, J. M., Brodsky, N. L., Korczykowski, M., Avants, B. B., et al. (2010). Early parental care is important for hippocampal maturation: evidence from brain morphology in humans. Neuroimage 49, 1144–1150. doi: 10.1016/j.neuroimage.2009.07.003
Riem, M. M., Alink, L. R., Out, D., Van IJzendoorn, M. H., and Bakermans-Kranenburg, M. J. (2015). Beating the brain about abuse: empirical and meta-analytic studies of the association between maltreatment and hippocampal volume across childhood and adolescence. Dev. Psychopathol. 27, 507–520. doi: 10.1017/S0954579415000127
Rifkin-Graboi, A., Kong, L., Sim, L. W., Sanmugam, S., Broekman, B. F., Chen, H., et al. (2015). Maternal sensitivity, infant limbic structure volume and functional connectivity: a preliminary study. Transl. Psychiatry 5:e668. doi: 10.1038/tp.2015.133
Rilling, J. K., and Young, L. J. (2014). The biology of mammalian parenting and its effect on offspring social development. Science 345, 771–776. doi: 10.1126/science.1252723
Roisman, G. I., and Fraley, R. C. (2008). A behavior–genetic study of parenting quality, infant attachment security, and their covariation in a nationally representative sample. Dev. Psychol. 44, 831–839. doi: 10.1037/0012-1649.44.3.831
Ruby, P., and Decety, J. (2001). Effect of subjective perspective taking during simulation of action: a PET investigation of agency. Nat. Neurosci. 4, 546–550. doi: 10.1038/87510
Sameroff, A. (2009). The Transactional Model of Development: How Children and Contexts Shape Each Other. Washington, DC: American Psychological Association, doi: 10.1037/11877-006
Saygin, A. P. (2007). Superior temporal and premotor brain areas necessary for biological motion perception. Brain 130, 2452–2461. doi: 10.1093/brain/awm162
Serbin, L. A., Kingdon, D., Ruttle, P. L., and Stack, D. M. (2015). The impact of children’s internalizing and externalizing problems on parenting: transactional processes and reciprocal change over time. Dev. Psychopathol. 27(4 Pt 1), 969–986. doi: 10.1017/S0954579415000632
Sethna, V., Pote, I., Wang, S., Gudbrandsen, M., Blasi, A., McCusker, C., et al. (2017). Mother-infant interactions and regional brain volumes in infancy: an MRI study. Brain Struct. Funct. 222, 2379–2388. doi: 10.1007/s00429-016-1347-1
Shaw, P., Kabani, N. J., Lerch, J. P., Eckstrand, K., Lenroot, R., Gogtay, N., et al. (2008). Neurodevelopmental trajectories of the human cerebral cortex. J. Neurosci. 28, 3586–3594. doi: 10.1523/jneurosci.5309-07.2008
Shdo, S. M., Ranasinghe, K. G., Gola, K. A., Mielke, C. J., Sukhanov, P. V., Miller, B. L., et al. (2017). Deconstructing empathy: neuroanatomical dissociations between affect sharing and prosocial motivation using a patient lesion model. Neuropsychologia doi: 10.1016/j.neuropsychologica.2017.02.010 [Epub ahead of print].
Smith, D. K., Johnson, A. B., Pears, K. C., Fisher, P. A., and DeGarmo, D. S. (2007). Child maltreatment and foster care: unpacking the effects of prenatal and postnatal parental substance use. Child Maltreat. 12, 150–160. doi: 10.1177/1077559507300129
Smith, S. M., and Nichols, T. E. (2009). Threshold-free cluster enhancement: addressing problems of smoothing, threshold dependence and localisation in cluster inference. Neuroimage 44, 83–98. doi: 10.1016/j.neuroimage.2008.03.061
Spreng, R. N., Mar, R. A., and Kim, A. S. (2009). The common neural basis of autobiographical memory, prospection, navigation, theory of mind, and the default mode: a quantitative meta-analysis. J. Cogn. Neurosci. 21, 489–510. doi: 10.1162/jocn.2008.21029
Sroufe, L. A. (1979). The coherence of individual development: early care, attachment, and subsequent developmental issues. Am. Psychol. 34, 834–841. doi: 10.1037/0003-066X.34.10.834
Sroufe, L. A. (2016). “The place of attachment in development,” in Handbook of Attachment: Theory, Research, and Clinical Applications, eds J. Cassidy and P. R. Shaver (New York, NY: The Guilford Press), 997–1011.
Steele, H., Steele, M., and Croft, C. (2008). Early attachment predicts emotion recognition at 6 and 11 years old. Attach. Hum. Dev. 10, 379–393. doi: 10.1080/14616730802461409
Teicher, M. H., and Samson, J. A. (2016). Annual research review: enduring neurobiological effects of childhood abuse and neglect. J. Child Psychol. Psychiatry 57, 241–266. doi: 10.1111/jcpp.12507
Teicher, M. H., Samson, J. A., Anderson, C. M., and Ohashi, K. (2016). The effects of childhood maltreatment on brain structure, function and connectivity. Nat. Rev. Neurosci. 17, 652–666. doi: 10.1038/nrn.2016.111
Thompson, R. A. (2016). “Early attachment and later development: reframing the questions,” in Handbook of Attachment: Theory, Research, and Clinical Applications, eds J. Cassidy and P. R. Shaver (New York, NY: The Guilford Press), 330–348.
Toro, R., Perron, M., Pike, B., Richer, L., Veillette, S., Pausova, Z., et al. (2008). Brain size and folding of the human cerebral cortex. Cereb. Cortex 18, 2352–2357. doi: 10.1093/cercor/bhm261
Tottenham, N. (2012). Human amygdala development in the absence of species-expected caregiving. Dev. Psychobiol. 54, 598–611. doi: 10.1002/dev.20531
Tottenham, N. (2014). The importance of early experiences for neuro-affective development. Curr. Top. Behav. Neurosci. 16, 109–129. doi: 10.1007/7854_2013_254
Tottenham, N., and Sheridan, M. A. (2009). A review of adversity, the amygdala and the hippocampus: a consideration of developmental timing. Front. Hum. Neurosci. 3:68. doi: 10.3389/neuro.09.068.2009
Urrila, A. S., Artiges, E., Massicotte, J., Miranda, R., Vulser, H., Bézivin-Frere, P., et al. (2017). Sleep habits, academic performance, and the adolescent brain structure. Sci. Rep. 7:41678. doi: 10.1038/srep41678
Van IJzendoorn, M. H., Dijkstra, J., and Bus, A. G. (1995). Attachment, intelligence, and language: a meta-analysis. Soc. Dev. 4, 115–128. doi: 10.1111/j.1467-9507.1995.tb00055.x
Van IJzendoorn, M. H., Vereijken, C. M. J. L., Bakermans-Kranenburg, M. J., and Riksen-Walraven, J. M. (2004). Assessing attachment security with the attachment Q-sort: meta-analytic evidence for the validity of the observer AQS. Child Dev. 75, 1188–1213. doi: 10.1111/j.1467-8624.2004.00733.x
Van Praag, H., Kempermann, G., and Gage, F. H. (2000). Neural consequences of environmental enrichment. Nat. Rev. Neurosci. 1, 191–198. doi: 10.1038/35044558
Wallace, G. L., White, S. F., Robustelli, B., Sinclair, S., Hwang, S., Martin, A., et al. (2014). Cortical and subcortical abnormalities in youths with conduct disorder and elevated callous-unemotional traits. J. Am. Acad. Child Adolesc. Psychiatry 53, 456–465. doi: 10.1016/j.jaac.2013.12.008
Waters, E., and Deane, K. E. (1985). Defining and assessing individual differences in attachment behavior: Q-methodology and the organization of behavior in infancy and early childhood. Monogr. Soc. Res. Child Dev. 50, 41–65.
Whittle, S., Dennison, M., Vijayakumar, N., Simmons, J. G., Yücel, M., Lubman, D. I., et al. (2013). Childhood maltreatment and psychopathology affect brain development during adolescence. J. Am. Acad. Child Adolesc. Psychiatry 52, 940–952. doi: 10.1016/j.jaac.2013.06.007
Whittle, S., Simmons, J. G., Dennison, M., Vijayakumar, N., Schwartz, O., Yap, M. B., et al. (2014). Positive parenting predicts the development of adolescent brain structure: a longitudinal study. Dev. Cogn. Neurosci. 8, 7–17. doi: 10.1016/j.dcn.2013.10.006
Whittle, S., Vijayakumar, N., Dennison, M., Schwartz, O., Simmons, J. G., Sheeber, L., et al. (2016). Observed measures of negative parenting predict brain development during adolescence. PLOS ONE 11:e0147774. doi: 10.1371/journal.pone.0147774
Winkler, A. M., Kochunov, P., Blangero, J., Almasy, L., Zilles, K., Fox, P. T., et al. (2010). Cortical thickness or grey matter volume? The importance of selecting the phenotype for imaging genetics studies. Neuroimage 53, 1135–1146. doi: 10.1016/j.neuroimage.2009.12.028
Yoon, U., Fonov, V. S., Perusse, D., and Evans, A. C. (2009). The effect of template choice on morphometric analysis of pediatric brain data. Neuroimage 45, 769–777. doi: 10.1016/j.neuroimage.2008.12.046
Keywords: mother–child attachment, infancy, childhood, brain development, social brain, gray matter, volumetrics, cortical thickness
Citation: Leblanc É, Dégeilh F, Daneault V, Beauchamp MH and Bernier A (2017) Attachment Security in Infancy: A Preliminary Study of Prospective Links to Brain Morphometry in Late Childhood. Front. Psychol. 8:2141. doi: 10.3389/fpsyg.2017.02141
Received: 28 July 2017; Accepted: 27 November 2017;
Published: 12 December 2017.
Edited by:
Nina Kühn-Popp, Georg Simon Ohm University of Applied Sciences Nuremberg, GermanyReviewed by:
Anne Rifkin-Graboi, Singapore Institute for Clinical Sciences (A∗STAR), SingaporePatricia Pelufo Silveira, McGill University, Canada
Tobias Schuwerk, Ludwig-Maximilians-Universität München, Germany
Copyright © 2017 Leblanc, Dégeilh, Daneault, Beauchamp and Bernier. This is an open-access article distributed under the terms of the Creative Commons Attribution License (CC BY). The use, distribution or reproduction in other forums is permitted, provided the original author(s) or licensor are credited and that the original publication in this journal is cited, in accordance with accepted academic practice. No use, distribution or reproduction is permitted which does not comply with these terms.
*Correspondence: Annie Bernier, YW5uaWUuYmVybmllckB1bW9udHJlYWwuY2E=