- 1School of Psychology, University of Wollongong, Wollongong, NSW, Australia
- 2Illawarra Health and Medical Research Institute, University of Wollongong, Wollongong, NSW, Australia
Schizotypal traits are of interest and importance in their own right and also have theoretical and clinical associations with schizophrenia. These traits comprise attenuated psychotic symptoms, social withdrawal, reduced cognitive capacity, and affective dysregulation. The link between schizotypal traits and psychotic disorders has long since been debated. The status of knowledge at this point is such schizotypal traits are a risk for psychotic disorders, but in and of themselves only confer liability, with other risk factors needing to be present before a transition to psychosis occurs. Investigation of schizotypal traits also has the possibility to inform clinical and research pursuits concerning those who do not make a transition to psychotic disorders. A growing body of literature has investigated the genetic underpinnings of schizotypal traits. Here, we review association, family studies and describe genetic disorders where the expression of schizotypal traits has been investigated. We conducted a thorough review of the existing literature, with multiple search engines, references, and linked articles being searched for relevance to the current review. All articles and book chapters in English were sourced and reviewed for inclusion. Family studies demonstrate that schizotypal traits are elevated with increasing genetic proximity to schizophrenia and some chromosomal regions have been associated with schizotypy. Genes associated with schizophrenia have provided the initial start point for the investigation of candidate genes for schizotypal traits; neurobiological pathways of significance have guided selection of genes of interest. Given the chromosomal regions associated with schizophrenia, some genetic disorders have also considered the expression of schizotypal traits. Genetic disorders considered all comprise a profile of cognitive deficits and over representation of psychotic disorders compared to the general population. We conclude that genetic variations associated with schizotypal traits require further investigation, perhaps with targeted phenotypes narrowed to assist in refining the clinical end point of significance.
Introduction
Schizotypal personality comprises stable behaviors characterized by persistent attenuated psychotic symptoms. These include unusual perceptual experiences, odd beliefs, unusual speech (in tone, intonation, or use of specific words), social anxiety, suspiciousness/paranoia, and isolated fully formed psychotic symptoms. The rate of clinically diagnosed schizotypal personality disorder (SPD) is approximately 4% in the general population (Pulay et al., 2009). Differentiation between schizotypal traits and SPD reflects the degree of self-recognized impairment to occupational and interpersonal functioning, frequency, and severity of symptom presentation. There are an increasing number of studies suggesting detectable reductions in interpersonal and occupational functioning in those displaying schizotypal traits (e.g., Waldeck and Miller, 2000; McGurk et al., 2013). When used as a screen in the general population, schizotypal traits are associated with depression, bipolar and affective and non-affective psychotic disorders longitudinally (e.g., Miller et al., 2002; Kwapil et al., 2013). Progression rates from SPD to a psychotic disorder are approximately 40% over 2 years (Nordentoft et al., 2006), double the risk reported in more recent studies concerned with transition from an at risk mental state (ARMS) (Fusar-Poli et al., 2013). Clinically, schizotypal traits are part of the prodrome or ARMS criteria (e.g., Salokangas and McGlashan, 2008) and SPD has now been moved from personality disorders into the Schizophrenia Spectrum and Other Psychotic Disorders section of Diagnostic and Statistical manual of Mental Disorders (DSM-V; American Psychiatric Association., 2013). The factors which determine whether someone moves from non-clinical schizotypal traits to the longitudinal expression of a clinical disorder are as yet unknown.
As well as the clinical presentation of SPD, researchers and clinicians are becoming increasingly interested in the expression of schizotypal traits or schizotypy in the general population. Clinical psychotic disorders are etiologically complex. Genetic heritability of schizotypy has been estimated around 30 to 50% within families (Claridge and Hewitt, 1987; Kendler and Hewitt, 1992; Kendler et al., 1993; Cardno et al., 1999; Chang et al., 2002; Linney et al., 2003; Macar et al., 2012). Similarly to schizophrenia susceptibility, schizotypy seems to be polygenic, with each gene likely conferring only a small to moderate risk, and interacting against background environmental factors (Grant et al., 2013; Brambilla et al., 2014). This level of complexity leads to the hypothesis that the same biological factors that underpin schizotypy in the general population are also relevant to more severe clinical disorders such as SPD and psychotic disorders. The so-called psychosis continuum suggests that risk factors for psychotic disorders will operate in a similar manner in subclinical schizotypy as in the clinical end points. Given that clinical psychotic disorders conceivably occur after a cascade and interaction between multiple biological, environmental, and psychological factors, investigating the effects of psychosis-risk-factors in subclinical schizotypes provides a window into etiological factors prior to disease progression.
During the last decade, a growing literature has emerged identifying genetic markers associated with schizotypy. We will outline the evidence for the proximity of schizotypal traits to genetic liability for psychotic disorders reporting notable specific genetic markers, such as single nucleotide polymorphisms (SNPs), associated with schizotypal traits. These studies provide evidence for population level variations in genetic bases underpinning the expression of schizotypal traits. The few linkage studies which have investigated the chromosomal regions conferring liability for schizotypal traits within families will be outlined. Collectively, these studies will delineate the evidence for schizotypal traits demonstrating heritability and co-occurrence with schizophrenia. Next, there are some genetic disorders which report rates of psychotic disorders with higher prevalence than the general population. These disorders encompass chromosomal regions which are of interest in schizophrenia. Consideration will be given to genetic disorders where expressions of schizotypal traits have been reported. These genetic disorders have well characterized genomic abnormalities and therefore further assist in pointing to chromosomal regions of significance for schizotypal trait expression.
We conducted a thorough search in the selection of articles for the current paper. We made use of PubMed, Scopus, Web of Science, PsycINFO, and Medline for the identification of articles. Key search terms were generated on the basis of the main concepts within each section, and the key words have been included in parenthesis after subsequent subheadings. All articles available in English were reviewed for inclusion. We excluded studies which considered psychotic experiences in subclinical populations, or who considered psychotic experiences after an event (e.g., stress, cannabis use). We also excluded studies which had considered the expression of schizotypal traits in the presence of bipolar risk. There is substantial debate considering the relationship between psychotic disorders and bipolar disorder which is worthy of a review in itself in order to do it justice. These publications (Mahon et al., 2013) were based within the debate of this relationship and were therefore not included in the current articles. For each section, references and similar articles were then searched to determine whether references had been missed. This was particularly relevant to the section on Schizotypal Traits and Genetic Disorders because this represented a small body of literature. Our last search of the literature was conducted in August 2016.
Heritability and Linkage Studies for Schizotypal Traits
Expression of Schizotypal Traits in Families (Schizotypy, Schizotypal, Psychosis, Psychotic, Family, Familial, Relatives, Siblings, Twins, Parental)
The expression of schizotypal traits in relatives of patients with schizophrenia presents the opportunity to investigate whether schizotypal traits are an intermediate phenotype (Lenzenweger, 2013) for psychotic disorders. Intermediate phenotypes are heritable and underpinned by biological mechanisms. They lie between the biological risk and disease end point of interest; in this case schizotypal traits are predominantly considered for their relevance for psychosis risk. An intermediate phenotype is thought to lie mid-distance between genetic risk and the clinical end point of interest. Unsurprisingly, given we make this assumption about schizotypal traits, predisposition to schizophrenia spectrum disorders is considered to be highly heritable, demonstrated through patterns of risk in twins, and other relatives (Tsuang, 2000; Nguyen et al., 2003). First degree relatives presumably have some of the risk genes since they are at a 10-fold increased risk for developing the disorder and share approximately fifty per cent of their genes with their proband relatives. Our goal was to identify whether consistent findings pointed to a reliable relationship between familial liability and schizotypal expression. The results of schizotypal traits expressed in non-psychotic relatives of schizophrenia spectrum patients are summarized in Table 1. Preliminary findings from family studies suggest a relationship between familial-genetic liability for schizophrenia and schizotypal traits. Schizotypy has been implicated as a key expression of risk in the relationship between familial liability and schizophrenia (Lenzenweger, 2006).
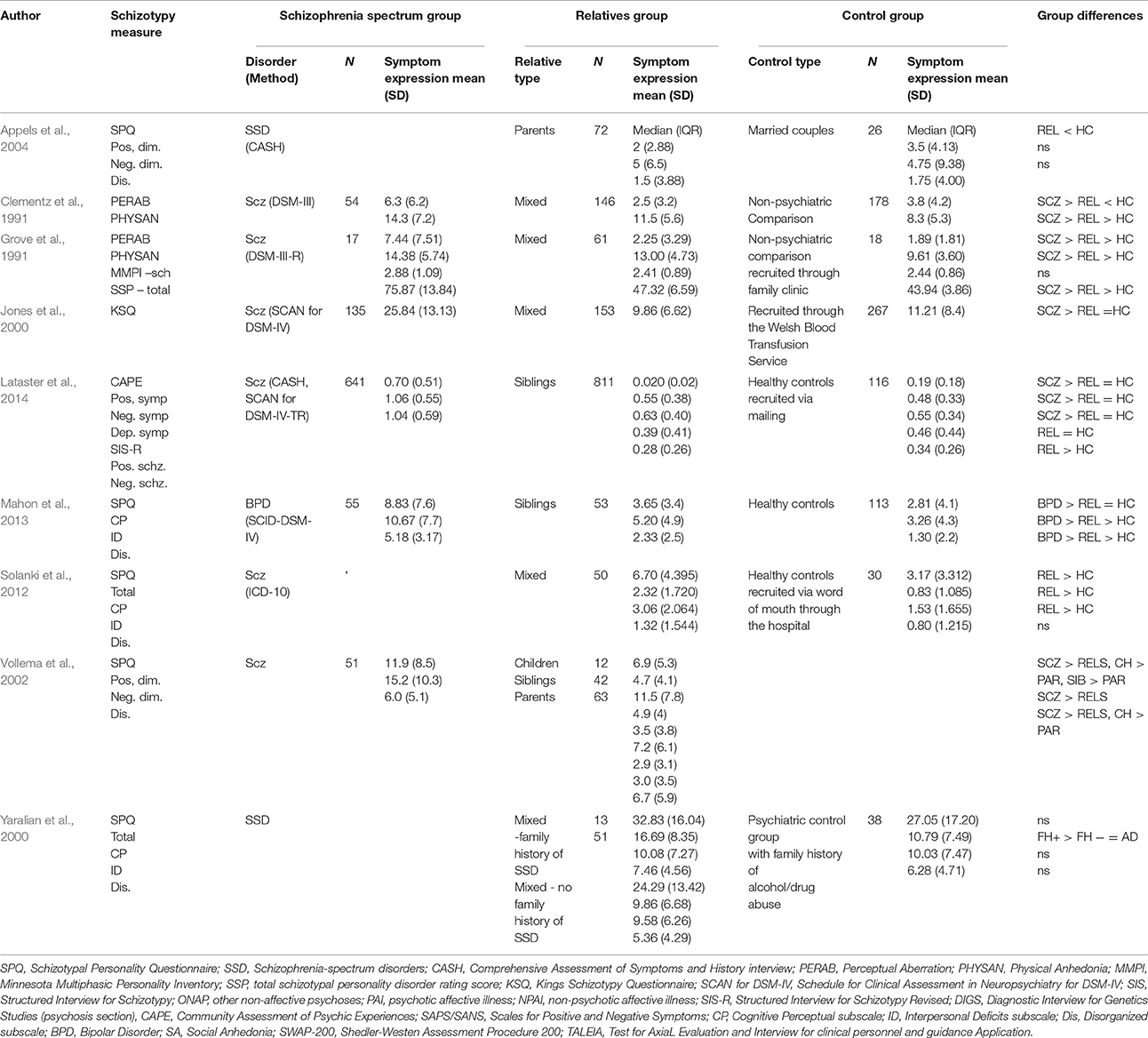
Table 1. Summary of expression and presence of schizotypy symptoms of studies involving relatives of Schizophrenia Spectrum probands.
SPQ, Schizotypal Personality Questionnaire; SSD, Schizophrenia-spectrum disorders; CASH, Comprehensive Assessment of Symptoms and History interview; PERAB, Perceptual Aberration; PHYSAN, Physical Anhedonia; MMPI, Minnesota Multiphasic Personality Inventory; SSP, total schizotypal personality disorder rating score; KSQ, Kings Schizotypy Questionnaire; SCAN for DSM-IV, Schedule for Clinical Assessment in Neuropsychiatry for DSM-IV; SIS, Structured Interview for Schizotypy; ONAP, other non-affective psychoses; PAI, psychotic affective illness; NPAI, non-psychotic affective illness; SIS-R, Structured Interview for Schizotypy Revised; DIGS, Diagnostic Interview for Genetics Studies (psychosis section), CAPE, Community Assessment of Psychic Experiences; SAPS/SANS, Scales for Positive and Negative Symptoms; CP, Cognitive Perceptual subscale; ID, Interpersonal Deficits subscale; Dis, Disorganized subscale; BPD, Bipolar Disorder; SA, Social Anhedonia; SWAP-200, Shedler-Westen Assessment Procedure 200; TALEIA, Test for AxiaL Evaluation and Interview for clinical personnel and guidance Application
However, it is important to understand whether genetic factors alone drive elevated rates of schizotypal traits in relatives of patients with psychotic disorders. To date there are no methodologically sound population studies (with large sample sizes, controls for confounds, and for the direction of the relationship) that explore this association. There are however two studies that prospectively examine this relationship on a smaller level (Mata et al., 2000, 2003). The first published in 2000, interviewed mothers of 90 patients with schizophrenia and 121 of their psychiatrically healthy relatives with the Present State Examination (PSE; Wing et al., 1974). The authors were interested in childhood personality, social adjustment of the mothers, and schizotypal traits in relatives as assessed by the Kings Schizotypy Questionnaire (KSQ; Williams, 1993), International Personality Disorder Examination (IPDE; World Health Organization., 1992) and Venables' Survey of Attitudes and Experiences Scale (SAE; Venables et al., 1990). Positive syndrome in patients was correlated with greater scores for relatives on the three schizotypy scales; however patient syndromes did not have a relationship with their corresponding schizotypal trait (i.e., positive schizotypy to positive syndrome in patients). The second study published in 2003 sought to elucidate the link between schizotypal traits in relatives and psychopathological syndromes in patients with psychoses. Interviews were conducted with 172 patients admitted with psychosis (using the PSE) and 263 of their psychiatrically healthy relatives (using the SAE, KSQ, and IPDE). Schneiderian symptoms in patients were related to higher scores on both positive and negative schizotypal features in relatives.
These studies collectively point to schizotypal traits being highly expressed in relatives of patients with psychotic disorders. There is one point of limitation which needs to be kept in mind for studies where parent-child dyads are used in research. Given that we do not understand the heritability of schizophrenia risk, it is not possible to be certain that the parent conferring the genetic risk is included in the study (unless of course they have a diagnosed psychotic disorder themselves). This may potentially lead to weakened associations between relative dyads for schizotypal traits. Indeed, consideration needs to be given to second degree relatives and their potential role in conferring genetic liability for schizotypal traits. A study which examines schizotypal traits within an extended family, including multiple generations and first and second degree relatives, would be interesting to determine where strongest associations are found between individuals. Although these studies suggest schizotypal traits are a significant factor in the genetic risk for psychotic disorders they do not highlight specific chromosomal regions. The two studies from Mata et al. (2000) and Mata et al. (2003), suggest that profiles of symptoms in patients do not relate to their corresponding subclinical schizotypal traits, for example positive psychotic symptoms were specifically associated with positive schizotypal traits. This would suggest, schizotypal traits provide an overall risk for psychosis, rather than vulnerability toward subtypes of specific psychotic symptomatology.
Linkage Studies with Schizotypal Traits (Schizotypy, Schizotypal, Psychosis, Psychotic, Family, Familial, Relatives, Siblings, Twins, Parental, Linkage, Candidate, Plus Specific Genes Highlighted by Review of Initial Literature)
Most studies which examine candidate genes involved in schizotypal genetic susceptibility are based on the results of previous studies of schizophrenia. Only a few studies have performed genetic analyses on schizotypy in relatives of patients with schizophrenia (Kendler et al., 1995; Fogelson et al., 1999; Bergman et al., 2000; Miller et al., 2002; Mata et al., 2003; Fanous et al., 2007; Lien et al., 2010). Four of these studies included the Structured Interview Schizotypy or a modified version of it to assess schizotypy in tested individuals (Kendler et al., 1995; Miller et al., 2002; Fanous et al., 2007; Lien et al., 2010). Linkage studies in families have highlighted chromosomic regions of interest related to the psychosis continuum. Existing studies suggest that both schizophrenia and schizotypal traits share some common chromosome regions (such as 4q, 5q; 6p, 6q, 8p, 9q, 10p, 10q, 11q, 15q), with an average significance generally lower in schizotypal traits than schizophrenia, regardless of ethnicity of the sample and schizotypy measure used (Levinson et al., 2000; Baron, 2001; DeLisi et al., 2002; Linney et al., 2003; Fanous et al., 2007; Xu et al., 2009; Lien et al., 2010). However, an Irish study reported higher significance schizotypal traits compared to patients with schizophrenia, highlighting chromosomal regions 5q, 9q, and 10p (Fanous et al., 2007). When an analysis was performed using a combination of both schizophrenia and schizotypy factors in a population of Taiwan relatives, both negative schizophrenia and negative schizotypal traits were linked with chromosomal region 10q22.3 while the ones for positive schizophrenia and positive schizotypy were specific to 5q14.2 and 11q23.3 (Lien et al., 2010).
From the reviewed studies, schizotypal traits do seem to be expressed at higher levels in relatives of patients with psychotic disorders compared to the general population. It is less clear whether patients and their relatives share a vulnerability to expressing the same subtypes of symptoms, such as common expression of positive trait/symptomatology. Linkage studies point to some commonality in symptom expression between patients and relatives with corresponding chromosomal regions; however the two family correlational studies did not support this. The small number of existing linkage studies, suggest the same chromosomal regions are implicated in both schizotypal traits and psychotic disorders. Subsequently the candidate genes with loci within these regions of interest are worthy of investigation in case-control studies.
Schizotypal Trait Genes: Association Studies (Schizotypy, Schizotypal, Psychosis, Psychotic, Candidate Genes, Dopamine, Glutamate, Serotonin, GABA, Plus Specific Genes Highlighted through an Initial Literature Search)
Several schizophrenia genes have been identified through systematic fine mapping in regions implicated by linkage analysis (Liu et al., 2002) and through follow up analysis of linkage peaks to systematically identify candidate genes (Hennah et al., 2003; Duan et al., 2004; Petryshen et al., 2005; Pimm et al., 2005). Due to the small number of linkage studies investigating schizotypal traits, the candidate genes previously associated with schizophrenia drive the focus of SNP selection in schizotypal trait studies. For a disorder such as schizophrenia, which appears to be genetically complex, genetic markers including SNPs provide a plausible mechanism to underpin the expression of attenuated intermediary phenotypes such as schizotypal traits to be presented within a healthy population. An inherent assumption in this area is that each genetic marker will confer a degree of risk for the expression of psychotic disorders, and it is an accumulation of risk alleles in a dosing manner which sets background genetic liability. Overall results from literature search of schizotypy and candidate genes can be found in Table 2.
Among these candidate genes, dystrobrevin-binding protein 1 gene (DTNBP1) located on chromosome 6q22.3 has been associated with schizophrenia in diverse populations (Bray et al., 2005; Tochigi et al., 2006; Tosato et al., 2007; Vilella et al., 2008). This gene codes for a synaptic protein dysbindin 1 which is found presynaptically. Dysbindin seems to be involved in the exocytotic glutamate release and cognition processes, in particular memory (Burdick et al., 2006; Talbot et al., 2006; Bhardwaj et al., 2009; Hashimoto et al., 2010). SNPs within this gene are associated with lower levels of dysbindin expression in post-mortem brain studies (Talbot et al., 2004, 2006), increased risk for schizophrenia in case-control studies (Straub et al., 2002) and with paranoid schizotypy in a Caucasian sample (Stefanis et al., 2008). In reference to glutamatergic functioning, P250 protein is also important given that it plays a significant part in N-methyl-D-aspartate receptor (NMDAR) mediated spine development. This protein was found to be highly enriched in the post-synaptic densities of neurons and is co-localized with the NR2B subunit of the NMDAR. NDMAR plays an essential role in the synaptic plasticity and memory performance. SNPs in the P250 gene have been previously proposed to be involved in schizophrenia vulnerability (Ohi et al., 2012). In a Japanese cohort, genetic variant (rs2298599) in the P250 gene was also found to be significantly associated with high scoring of schizotypal traits. NRG1 function is mediated by a class of receptor tyrosine kinases including erbB4 (Li et al., 2007), and has been shown to associate with schizophrenia (Law et al., 2007) with altered NRG1/erbB4 signaling being reported in the brains of schizophrenia patients (Hahn et al., 2006). Two studies have investigated SNPs within this gene (Stefanis et al., 2011; Zammit et al., 2014), and results have been inconsistent with only one SNP (rs4673628) associating with positive schizotypy.
Genes involved in the regulation of dopamine have been a strong candidate due to dopamine's documented role in schizophrenia and schizotypal traits (e.g., Woodward et al., 2011; Seeman and Seeman, 2014). Considerable attention has been given to the catechol-O-methyltransferase gene (or COMT). This gene codes for an enzyme involved in the breakdown of catecholamines, including dopamine, at the synapse. A single variation from G to A at codon 158 of COMT (rs4680) results in a valine (Val) to methionine (Met) substitution in the amino acid sequence of the protein. This change leads to a functional alteration in the activity of the enzyme: Low (Met), Medium (Val/Met) and High (Val). Several studies have reported an association between high activity allele and patients with schizophrenia (Glatt et al., 2003; Gogos and Gerber, 2006). Authors suggest that the high activity enzyme leads to lower dopamine activity in the cortex and can account for both the negative and cognitive symptoms found in schizophrenia. This SNP has been associated with higher schizotypy scores in several gene-wide association studies (GWAS) (Caucasian population: Avramopoulos et al., 2002; Schürhoff et al., 2007; Smyrnis et al., 2007; Grant et al., 2013; de Castro-Catala et al., 2015. Chinese population: Ma et al., 2007) but not all (Caucasian population: Arias et al., 2010; Grant et al., 2014; Zammit et al., 2014). A key regulator of the levels of dopamine in the synapses in brain is the dopamine transporter protein (DAT), encoded by gene SLC6A3 (located at 5p15.3). Unlike COMT which operates within the synapse, DAT reuptakes the neurotransmitter into the presynaptic neuron. Given the functional importance to neurotransmission of this gene, it has been comparatively under investigated in relation to schizotypy. The functional polymorphism of interest is located at the 3′UTR region of SLC6A3 in a form of a Variable Number Tandem Repeat (VNTR) of 40 bp. Rare allele 9 carriers of this VNTR were found with schizotypal features (Grant et al., 2013), however due to the limited sample this result has to be considered with caution. Rather than just focusing on the factors involved in breaking down or taking up neurotransmitters, attention has also been given to SNPs coding for dopamine receptors. These SNPs can lead to functional and structural alterations in dopamine (e.g,. D'Souza and Craig, 2006; Michealraj et al., 2014). Gurvich et al. (2016) found that dopaminergic receptor D1 was linked to increased scores on schizotypy factors that resemble negative symptoms in clinically diagnosable schizophrenia, while Grant et al. (2014) reported that SNPs in the dopaminergic receptors D4 and D5 were negatively correlated with schizotypal features. These authors concluded that schizotypy is associated with dopamine dysregulation. However, the results for dopamine receptors as a whole are mixed, with Grant et al. (2013) and Taurisano et al. (2014) finding an association with schizotypy at the D2 receptor, which conflicts with the findings of Leach et al. (2013) and Montag et al. (2015).
Several other functional SNPs with relevance to neurotransmission and brain maturation have also been investigated in relation to schizotypy. A factor considered essential for supporting neuronal growth and promoting neuronal survival is brain-derived neurotrophic factor (BDNF). BDNF has been widely investigated for its relevance to schizophrenia (Kheirollahi et al., 2016). Proline is an amino acid which is found in excess in patients with schizophrenia. There is a SNP of interest in the gene coding for proline oxidase enzyme, which is able to metabolize (PRODH). Additionally, a SNP coding for D-Amino Acid Oxidase enzyme involved in glutamatergic neurotransmission (DAAO), has been demonstrated to be associated with schizophrenia in case-control studies (Caldinelli et al., 2013). One study in a Chinese population (Yang et al., 2013), found no association between these SNPs and schizotypy scores. Similarly, after correction for multiple comparisons, no significant associations were observed between SNPs in DAAO gene and schizotypy in a Greek military healthy cohort (Stefanis et al., 2007). The same cohort was employed to evaluate risk factors for schizotypy for selected SNPs in RGS4 gene. The RGS4 gene codes for Regulator of G protein signaling, which is involved in intracellular signaling in glutamatergic and dopaminergic receptors. Genetic variant for rs951436 and rs2661319 were associated with negative schizotypy and total schizotypy respectively, however none of the tested SNPs were associated with cognitive indices, despite the seeming functional importance of RGS4 (Stefanis et al., 2008).
Looking at other candidate neuronal receptors, genetic variants for CACNA1C gene has been of interest to some investigators. This gene codes for a sub-unit of the calcium channel which regulates the calcium influx into the cells; a mechanism which has implications for learning and memory. One SNP in this gene (rs1006737) has been previously associated with schizophrenia (Porcelli et al., 2015). In a Caucasian sample, schizotypal paranoid ideation was associated with this SNP (Roussos et al., 2011). In another study from the same group, the same genetic variant was positively associated with interview assessed schizotypal personality disorder paranoid ideation and unusual experiences (Roussos et al., 2013). Given the replication of an association between schizotypal traits and this SNP it is worthy of investigation in future studies.
An increasing area of exploration in association studies is the zinc finger (ZNF) family. The ZNF family plays roles in the binding of DNA- and RNA proteins; where amino acids are folded into a single structural unit around a zinc atom to stabilize the fold (Sun et al., 2015). They are involved in multiple functions, including transcriptional activation, protein folding, RNA packaging, lipid binding, and DNA recognition (Laity et al., 2001). Recently, focus has been given to specific SNPs of ZNF804A (e.g., rs1344706, rs4667001, and rs728534) with a meta-analysis by Zhu et al. (2014) showing an association with schizophrenia risk in both Asian and Caucasian populations. When investigating these SNPs in reference to schizotypy, we see mixed results with some studies finding a significant association with schizotypy (Yasuda et al., 2011; Stefanis et al., 2013) and others not (Leach et al., 2013).
There is burgeoning interest in whether genes inherited from the maternal and paternal lines have differing effects, known as imprinting. This has been partly driven by consistent findings for increasing paternal age being related to psychosis risk (Foutz and Mezuk, 2015; Martin et al., 2015) and speculation on what mechanism may be underpinning this. Additionally, the role of imprinting in psychiatric genetics is becoming more widely speculated upon and a point for consideration within psychotic disorders (Crespi, 2008). In support of this the imprinted gene LRRTM1 (Leucine-rich repeat transmembrane neuronal 1) was reported to be a risk factor for mental illness such as schizophrenia when the gene was inherited from the father (Francks et al., 2007). LRRTM1 plays a significant role in brain development and reflects a variable profile of maternal regulation (Francks et al., 2007). Given that schizophrenia and schizotypal traits are considered to be neurodevelopmental, this gene may be of functional significance. Three SNPs (rs1007371, rs1446109, rs723524) in the LRRTM1 gene were examined in a student Canadian cohort for their association with a brief measure of schizotypy (Leach et al., 2014). However, none of the tested SNPs were significantly associated with schizotypy after correction for multiple comparisons. Given the gene's significance for neurodevelopment and maturation of the brain, potentially through the lifespan, this requires further investigation.
To some extent linkage studies have led to SNPs previously associated with schizophrenia being investigated for their relevance to schizotypal traits. The findings from the SNP studies are mixed, at best. However, they are similarly mixed using patient groups, where a concentrated expression of biological risk is found. The advantage of examining schizotypy in healthy populations is the potential to achieve large sample sizes, often compromised in patient case-control studies. However, there are caveats to such an approach. First the genetic risk will be “watered-down” in the attenuated schizotypal traits. Assuming a polygenic approach to risk, this will mean each individual will carry fewer at risk alleles. Secondly, there is as much heterogeneity in the expression of schizotypal traits in the healthy population as there is in the presentation of symptoms in patient populations. Thirdly, it is possible that schizotypes also carry protective SNPs which decrease the likelihood of transition to psychosis, this complicates a scenario which we know represents the convergence of multifaceted risk markers, only some of which are biological in nature. To date, few studies have taken advantage of the possibility of using large sample sizes to investigate the genetic underpinnings of schizotypy. There may be both pragmatic and theoretical concerns at play. Some still appear to question the utility of schizotypy to investigate psychotic disorders, questioning its role in risk for psychosis as well as the possibility it represents a useful intermediate phenotype. Others question the degree to which it is of interest in itself. These continued concerns being voiced in the literature ensure that obtaining funding to investigate the biological underpinnings of schizotypy can be difficult; restricting the investigation of the genetic underpinnings to often already established population databases where measures are limited and phenotypic refinement not possible. Finally, there are sample overlaps in the studies summarized here. Multiple research groups need to investigate these questions, each with their own approach to ensure that thorough and representative research eventuates in the future.
Genetic Disorders Reporting Schizotypal Traits (Schizotypy, Schizotypal, Psychosis, Psychotic, Schizophrenia, Genetic Disorders, Developmental Disorders)
Alongside the traditional methods of linkage, family studies and SNPs examining the underpinnings of the psychosis continuum, an alternative approach exists. There are a number of genetic disorders, with well-characterized genetic deletions or insertions, where rates of schizophrenia are considered to be higher than those found in the general population. In these instances, the genetic mutations are established, often with documented physical and psychological implications. It is possible that consideration of these disorders in relation to schizotypal traits would assist in providing additional evidence for the biological mechanisms underpinning the expression of these traits. Interestingly, given the current diagnostic manuals, individuals with well-defined genetic variations cannot receive a diagnosis of schizophrenia. However, some debate surrounds whether psychotic symptom presentation in those with genetic variations differs from the strict definition of schizophrenia (e.g., Bassett et al., 2003). A brief overview of genetic disorders with elevated rates of schizophrenia, where consideration has been given to schizotypal traits will be outlined.
Perhaps the most well-known genetic disorder associated with psychotic disorders is Chromosome 22q11.2 deletion syndrome. Deletion of 22q11.2 underpins a group of syndromes including DiGeorge syndrome, velocardiofacial syndrome (VCFS; also called Shprintzen syndrome), Opitz G/BBB syndrome and Conotruncal anomaly face syndrome. Chromosome 22q11.2 deletion syndrome is associated with physical problems in the cleft palate, heart, kidneys, lungs, autoimmune problems, and distinctive facial features. Many individuals have lower IQ than the general population, are developmentally delayed and have cognitive deficits. The deletion occurs in 1:5000 live births (Botto et al., 2003); although some argue rates may be much higher, given the heterogeneity of presentation it is possible those with the deletion will receive an alternative diagnosis and may not receive genetic testing. There is substantial evidence documenting elevated rates of schizophrenia in adolescence and adulthood in those with 22q11.2 deletion syndrome when compared to the general population. In addition, they appear to be at greater risk for developing schizophrenia than other learning disabled individuals (Gothelf et al., 2007). The existence of subclinical psychotic symptoms in children and adolescents with 22q11.2 deletion syndrome has clinical predictive validity for future psychotic disorders (Gothelf et al., 2007). Indeed, Debbané et al. (2006) reported 28% of their child and adolescent sample had psychotic symptoms. Consequently, the examination of schizotypal features in 22q11.2 deletion syndrome individuals is clinically valid as a potential preventative measure in this group as well as providing additional information concerning the pathogenesis of the psychosis.
Monks et al. (2014) reported rates of schizotypy to be higher in a 22q11.2 deletion sample compared to controls, even when participants with a current psychotic disorder were excluded from the 22q11.2 deletion group. Adolescents with 22q11.2 deletion syndrome have been reported to have elevated interpersonal schizotypal traits compared to controls, and the assessment of these traits appeared to be consistent over a three year period (Fonseca-Pedrero et al., 2016). In addition, higher levels of negative schizotypal traits have been reported in those with 22q11.2 deletion when compared to controls, even after controlling for anxiety, depression and full scale intelligence (Schneider et al., 2015). There are a number of genes captured by the 22q11.2 deletion area, these include: COMT, PRODH, zinc finger gene (ZDHHC8) and armadillo repeat protein deleted in velocardiofacial syndrome (ARVCF; see Harrison and Weinberger, 2005; Prasad et al., 2008 for reviews). Polymorphisms in these genes have been varyingly associated with cognitive deficits, schizophrenia, high schizotypy and psychotic symptoms both in psychiatric populations and in 22q11.2 samples. However, these associations have not been consistently reported; and, to date, it is difficult to determine whether negative findings are due to sample differences (e.g., cultural variation in allelic frequency), sex differences, phenotypic heterogeneity or low statistical power.
A disorder which has also been investigated for its similarities to schizophrenia is that of Fragile X syndrome (for example see Kelemen et al., 2013). In Fragile X there is a mutation of multiple CGG repeats on the X chromosome located in an area coding for a protein which appears to be fundamental to healthy brain development and functioning. The phenotypic characteristics of the disorder are developmental delays and cognitive impairments. Additionally, carriers (particularly males) can express psychomotor and cognitive difficulties as they age. Although in children Fragile X is most closely associated with autism spectrum and other pervasive developmental disorders (e.g., Franke et al., 1996; Tsiouris and Brown, 2004), some studies have suggested there may be a link with psychotic disorders. Given the overlapping pathophysiology of dysregulation in BDNF, glutamate and gamma-Aminobutyric acid (GABA) shared by Fragile X and schizophrenia, the potential for common causal pathways seems plausible. A number of studies have also reported associations between elevated rates of schizotypal traits and Fragile X chromosomal abnormalities (Reiss et al., 1988; Kerby and Dawson, 1994; Sobesky et al., 1994; Franke et al., 1996). However, given the levels of social anxiety and withdrawal in those with Fragile X syndrome it would be worthwhile further investigating which aspects of schizotypy are elevated in this syndrome. The overlapping pathophysiology with schizophrenia certainly makes the area of the chromosome affected in Fragile X of significance for those concerned schizophrenia etiology. However, phenotypic presentation, particularly in males, is more developmentally pervasive than the later presentation of symptoms in psychotic disorders. Researchers have linked the expression of Fragile X protein to symptoms and age of onset of schizophrenia (Hossein and Folsom, 2011; Kovács et al., 2013). Therefore, Fragile X protein levels deserve consideration in schizotypal individuals.
Another sex chromosome linked disorder of interest is Klinefelter syndrome where individuals have a 47 XXY karyotype, possessing an extra X chromosome. It occurs in approximately 1 in 670 male births (Bojesen et al., 2003). Many of the genes on the additional X chromosome are inactive which accounts for the relatively mild presentation of difficulties in Klinefelter's compared to other genetic disorders. However, there are some genes on the X chromosome which are homologous with those found on the Y chromosome and consequently are expressed. Males with Klinefelter's tend to be tall in stature, experience difficulties in hormonal expression, experience gynecomastia and infertility. Cognitive deficits in verbal domains (including dyslexia) are most commonly reported (e.g., Fales et al., 2003). The brain structural abnormalities found in Klinefelter's individuals are similar to those reported in patients with schizophrenia, including temporal lobe abnormalities, enlarged ventricles and structurally and functionally reduced or reversed asymmetries (e.g., Reiss et al., 2000; Itti et al., 2003; DeLisi et al., 2005). Approximately, 0.8–1% of male inpatients with schizophrenia are found to have an XXY karyotype (DeLisi et al., 1994). Rates of psychotic symptoms (DeLisi et al., 1994) and psychotic disorders (Bojesen et al., 2006; Cederlöf et al., 2014) are elevated in Klinefelter's individuals compared to the general population (although see Mors et al., 2001). Studies have reported that schizotypal traits are higher in those with Klinefelter's when compared to healthy controls (Van Rijn et al., 2006, 2009; Verhoeven and Egger, 2011). Some authors have suggested that the association between Klinefelter's and schizotypal traits is a function of the cognitive disorganization and verbal deficits found in the genetic syndrome. However, those with Klinefelter's also have elevated diagnoses of autism spectrum, ADHD and bipolar disorders; consequently, the higher schizotypal scores could be an expression of vulnerability to psychopathology in general rather than a demonstrated specificity for psychotic disorders.
Genetic disorders in some ways allow for the possibility of minimizing heterogeneity of genetic abnormalities within a well characterized subpopulation to enable exploration of the consequent phenotypic presentations. However, as yet it is poorly understood how the general genetic background would act to mediate or moderate deletions and insertions. Therefore, the possibility of the effects of epistatic interactions shaping phenotypic expression of genetic disorders needs to be more fully explored. One commonality in presentation of the genetic disorders highlighted above is cognitive deficits. It would be tempting to conclude that the associations with psychotic disorders and schizotypal traits are through a common causal pathway relating to cognitive vulnerabilities in verbal abilities and executive function. However, other developmental disorders such as Downs Syndrome also display cognitive deficits and yet do not have an over representation of psychotic disorders; thus a seemingly simple hypothesis is unsupported given that it cannot be generalized. Further work is needed to determine which aspects of the genetic disorders are particularly predictive of schizotypal traits and whether cognitive deficits, social difficulties or common biological pathways are the crucial factors in determining risk for schizotypal traits.
Conclusions and Future Directions
The selection of genetic variations investigated for their association with schizotypal traits has been largely shaped by studies concerned with schizophrenia. These include microsatellite, SNPs, insertions and deletions in neurobiological pathways known to be aberrant in psychotic disorders, including dopamine, glutamate and GABA. The authors were surprised by the relative paucity of papers which considered the genetic underpinnings of schizotypal traits. Unlike the clinical end point of psychotic disorders, schizotypal traits can be readily, cheaply and efficiently characterized using well validated and established measures (Mason, 2015). In contrast to psychotic symptoms, schizotypal traits are stable by virtue of the fact they are defined as a personality construct. Whilst some aspects of the behaviors comprising schizotypal traits may fluctuate, such as the presentation of isolated psychotic symptoms, the core of schizotypal traits will be consistently detectable over multiple time points. Schizotypal traits have been found to be associated with similar environmental factors, such as urbanicity, to those reported for elevating risk for psychotic disorder (e.g., Nelson et al., 2013). Collectively these factors shape a strong argument for considering schizotypal traits as being related to schizophrenia. Many of the studies included in this review have considered schizotypal traits as a whole (although see Mata et al., 2000, 2003). We have referred to schizotypal traits as plural because they comprise a number of behaviors and psychological phenomena. In addition, theorists in this area emphasize either the individual difference or clinical relevance of these traits. These considerations lead to differences in the measures used and whether differing dimensions (positive, negative, unusual experiences, cognitive disorganization, interpersonal) are emphasized. It is possible that these differences in measurement account for some of the inconsistencies in genetic association studies. Indeed, some of the dimensions of schizotypal traits could be more likely to be underpinned by stable genetic factors. For instance, disorganization and interpersonal schizotypal traits have a stability to them which suggests biological underpinnings are more plausible. Whilst a propensity toward unusual perceptual experiences may be further “down stream” of genetic influences, given that they are likely triggered by factors such as stress or substance use. Linkage studies have not as yet provided evidence for the genomic components which confer risk for schizotypal traits within families. A gene-dosing relationship is implied between psychotic disorders and schizotypal traits, given that there are elevated levels in relatives of patients with schizophrenia compared to the general population. This suggests further investigation of the biological correlates of schizotypal traits is warranted.
However, whether these investigations should be exclusively guided by the pathophysiology of schizophrenia could be a matter of debate. Given the assumption that schizotypal traits exist on a continuum with psychotic disorders, it seems likely that at least some of the genetic liability for schizophrenia should underpin schizotypal traits; yet, this is fraught with difficulties. Schizotypal individuals will likely possess some of the genetic liability for psychotic disorders but also some biological protective factors which assist in preventing transition to a diagnosable psychotic disorder. Although we recognize that risk for psychotic disorders is an interaction between genetic and environmental factors, existing evidence points to a strong role for genetic and biological vulnerability. In addition, it is possible that schizotypal traits present a broad phenotype for the liability to serious mental health disorders in general rather than psychotic disorders per se. The evidence accumulated here from genetic disorders may provide intriguing evidence for this. Schizotypal traits may be elevated in genetic disorders which over express psychotic disorders, however, these same genetic disorders also over represent pervasive developmental disorders, ADHD and bipolar disorder.
The authors could not find any papers which examined the interaction between genes and the environment for schizotypal traits. From a theoretical perspective this may be due to the assumed stability in a personality trait. A longitudinal study could track the effects of childhood events on the differing trajectories theorized to occur with schizotypal traits in response to environmental factors (Raine, 2006). Alternatively, shorter longitudinal follow up periods could reveal environmental factors in adulthood which exacerbate the presentation of schizotypal traits. These studies could consider the effects of particular genetic variations highlighted in this review. Such a design also has the potential to identify protective as well as risk genes. The investigation of gene-environment interactions on schizotypal traits is certainly an area for development. There are a number of papers considering gene-environment interactions for psychotic symptoms (e.g., Alemany et al., 2011; Ramsay et al., 2013). This perhaps suggests a way forward may be to refine the measurement of the schizotypal phenotype with the addition of state-like measures capturing psychotic symptoms separately. In this way gene-environment interactions could investigate effects along the psychosis continuum for expression of psychotic symptoms against a background risk of schizotypal traits. Whilst theoretically robust this is statistically complex and would require a relatively large sample size in order to have sufficient power to detect what, will likely be small effects.
The genetic disorders reviewed highlight the potential role for cognitive deficits being an additional factor for psychosis risk. From the perspective of investigation of genetic underpinnings, cognitive performance has many appealing features. It is objectively definable on the basis of performance rather than self-report; and, it is closer to the expression of genes than collections of (personality) behaviors, given that it can be linked to particular brain areas. Cognitive performance has been linked more consistently to genetic variation (e.g., Park and Waldman, 2014; Heck et al., 2014). Cognitive deficits can be linked to the structural brain abnormalities reported in genetic disorders. This provides a link from well characterized genetic polymorphisms to changes in brain structure which could underpin the cognitive deficits found in genetic disorders. Brain structure abnormalities have been found in those with schizotypal traits who are otherwise healthy individuals (e.g., Smallman et al., 2014). Therefore, in those characterized for both their schizotypal and genetic profiles, we could examination associations between carefully selected genetic polymorphisms, gray and white matter density and cognitive performance. In this way, research can begin to close the gap between the neurodevelopmental effects of the gene, their cognitive outcomes and whether these differ with schizotypal traits. Cognitive deficits are found across a number of genetic and mental health disorders; consequently, they do not represent an alternative single end point for genetic studies. Rather they offer a way to refine a complex and heterogeneous phenotype like schizotypy. For instance, schizotypes who have poorer cognitive performance could represent a subtype that may be genetically enriched for risk. Distinguishing their genetic profile from schizotypes with intact cognitive performance could, therefore, begin to tease out the complexities of psychosis liability where we see multiple factors converging.
The genetic underpinnings of schizotypal traits have received limited attention; there has been substantial focus on general psychosis risk rather than an emphasis on schizotypal traits specifically. As such there is the opportunity for further investigation and exploration. Using the investigations of psychotic disorders represents a start point for identification of genes of interest for schizotypal traits but focus needs to be widened. Investigations now are examining the genes associated with particular symptoms with minimal attention to diagnostic category (e.g., Craddock et al., 2009; Hamshere et al., 2009). This broader scope represents a way forward for the investigation of schizotypal traits. As such, perhaps we need to consider the genetic underpinnings of the differing dimensions of schizotypal traits rather than focusing on the intermediate phenotype as a whole. Given that schizotypy is heterogeneous, more refinement of the clinical end point of interest needs considering; the inclusion of a cognitive or psychotic symptom measure could help to narrow the specificity for genetic association studies.
Author Contributions
EW Contribution to the conception and design of the review, literature search for acquisition of studies, drafting of section 2.1 and 3 of the manuscript, critical review, editing, and formatting of the full manuscript and final approval of the version to be submitted. FF Contribution to the conception and design of the review, literature search for section 2.2, drafting of section 2.2, and 3, Critical review of manuscript and final approval of the version to be submitted. MS Contribution to the conception and design of the review, literature search for acquisition of studies for inclusion in the review, drafting of section 3, and final approval of the version to be submitted. EB Contribution to the conception and design of the review, literature search for acquisition of studies, drafting of sections 1, 4, and 5. Critical review of sections 2.1, 2.2, and 3. Editorial review of full manuscript and final approval of the version to be submitted. All authors agree to be accountable for all aspects of the work
Funding
EB is funded by the NARSAD Young Investigator Award, in kind support from GW Pharmaceuticals, and two internal grants for the University of Wollongong FF is funded by an NHMRC grant.
Conflict of Interest Statement
The authors declare that the research was conducted in the absence of any commercial or financial relationships that could be construed as a potential conflict of interest.
References
American Psychiatric Association. (2013). Diagnostic and Statistical Manual of Mental Disorders (5th ed.). Washington, DC: American Psychiatric Association.
Alemany, S., Arias, B., Aguilera, M., Villa, H., Moya, J., Ibáñez, M. I., et al. (2011). Childhood abuse, the BDNF-Val66Met polymorphism and adult psychotic-like experiences. Br. J. Psychiat. 199, 38–42. doi: 10.1192/bjp.bp.110.083808
Appels, M. C., Sitskoorn, M. M., Vollema, M. G., and Kahn, R. S. (2004). Elevated levels of schizotypal features in parents of patients with a family history of schizophrenia spectrum disorders. Schizophr. Bull. 30, 781–790. doi: 10.1093/oxfordjournals.schbul.a007131
Arias, B., Fatjo-Vilas, M., Estrada, G., Aguilera, Mc., Mitjans, M., Moya, J., et al. (2010). The analysis of genetic variability at COMT, CNR1, CNR2 and FAAH genes on cannabis use, schizotypy and psychotic-like experiences: a study in a Spanish general population. Schizophr. Res. 117, 307–308. doi: 10.1016/j.schres.2010.02.515
Avramopoulos, D., Stefanis, N. C., Hantoumi, I., Smyrnis, N., Evdokimidis, I., and Stefanis, C. N. (2002). Higher scores of self reported schizotypy in healthy young males carrying the COMT high activity allele. Mol. Psychiatry 7, 706–711. doi: 10.1038/sj.mp.4001070
Baron, M. (2001). Genetics of schizophrenia and the new millennium: progress and pitfalls. Am. J. Hum. Genet. 68, 299–312. doi: 10.1086/318212
Bassett, A. S., Chow, E., AbdelMalik, P., Gheorghiu, M., Husted, J., and Weksberg, R. (2003). The schizophrenia phenotype in 22Q deletion syndrome. Schizophr. Res. 60, 78. doi: 10.1016/S0920-9964(03)80618-7
Bergman, A. J., Silverman, J. M., Harvey, P. D., Smith, C. J., and Siever, L. J. (2000). Schizotypal symptoms in the relatives of schizophrenia patients: an empirical analysis of the factor structure. Schizophr. Bull. 26, 577–586. doi: 10.1093/oxfordjournals.schbul.a033479
Bhardwaj, S. K., Baharnoori, M., Sharif-Askari, B., Kamath, A., Williams, S., and Srivastava, L. K. (2009). Behavioral characterization of dysbindin-1 deficient sandy mice. Behav. Brain Res. 197, 435–441. doi: 10.1016/j.bbr.2008.10.011
Bojesen, A., Juul, S., Birkebæk, N. H., and Gravholt, C. H. (2006). Morbidity in Klinefelter syndrome: a Danish register study based on hospital discharge diagnoses. J. Clin. Endocrinol. Metab. 91, 1254–1260. doi: 10.1210/jc.2005-0697
Bojesen, A., Juul, S., and Gravholt, C. H. (2003). Prenatal and postnatal prevalence of Klinefelter syndrome: a national registry study. J. Clin. Endocrinol. Metab. 88, 622–626. doi: 10.1210/jc.2002-021491
Botto, L. D., May, K., Fernhoff, P. M., Correa, A., Coleman, K., Rasmussen, S. A., et al. (2003). A population-based study of the 22q11.2 deletion: phenotype, incidence, and contribution to major birth defects in the population. Pediatrics 112, 101–107. doi: 10.1542/peds.112.1.101
Brambilla, P., Fagnani, C., Cecchetto, F., Medda, E., Bellani, M., Salemi, M., et al. (2014). Genetic and environmental bases of the interplay between magical ideation and personality. Psychiatry Res. 215, 453–459. doi: 10.1016/j.psychres.2013.11.021
Bray, N. J., Preece, A., Williams, N. M., Moskvina, V., Buckland, P. R., Owen, M. J., et al. (2005). Haplotypes at the dystrobrevin binding protein 1 (DTNBP1) gene locus mediate risk for schizophrenia through reduced DTNBP1 expression. Hum. Mol. Genet. 14, 1947–1954. doi: 10.1093/hmg/ddi199
Burdick, K. E., Lencz, T., Funke, B., Finn, C. T., Szeszko, P. R., Kane, J. M., et al. (2006). Genetic variation in DTNBP1 influences general cognitive ability. Hum. Mol. Genet. 15, 1563–1568. doi: 10.1093/hmg/ddi481
Caldinelli, L., Sacchi, S., Molla, G., Nardini, M., and Pollegioni, L. (2013). Characterization of human DAAO variants potentially related to an increased risk of schizophrenia. Biochim. Biophys. Acta 1832, 400–410. doi: 10.1016/j.bbadis.2012.11.019
Cardno, A. G., Marshall, E. J., Coid, B., Macdonald, A. M., Ribchester, T. R., Davies, N. J. et al. (1999). Heritability estimates for psychotic disorders: the maudsley twin psychosis study. Arch. Gen. Psychiatry 56, 162–168. doi: 10.1001/archpsyc.56.2.162
Cederlöf, M., Ohlsson Gotby, A., Larsson, H., Serlachius, E., Boman, M., Långström, N., et al. (2014). Klinefelter syndrome and risk of psychosis, autism and ADHD. J. Psychiatr. Res. 48, 128–130. doi: 10.1016/j.jpsychires.2013.10.001
Chang, C.-J., Chen, W. J., Liu, S. K., Cheng, J. J., Yang, W.-C. O., Chang, H.-J., et al. (2002). Morbidity risk of psychiatric disorders among the first degree relatives of schizophrenia patients in Taiwan. Schizophr. Bull. 28, 379–392. doi: 10.1093/oxfordjournals.schbul.a006947
Claridge, G., and Hewitt, J. K. (1987). A biometrical study of schizotypy in a normal population. Pers. Individ. Dif. 8, 303–312. doi: 10.1016/0191-8869(87)90030-4
Clementz, B. A., Grove, W. M., Katsanis, J., and Iacono, W. G. (1991). Psychometric detection of schizotypy: perceptual aberration and physical anhedonia in relatives of schizophrenics. J. Abnorm. Psychol. 100, 607–612. doi: 10.1037/0021-843X.100.4.607
Craddock, N., O'Donovan, M. C., and Owen, M. J. (2009). Psychosis genetics: Modeling the relationship between schizophrenia, bipolar disorder and mixed (or “schizoaffective”) psychoses. Schizophr. Bull. 35, 482–490. doi: 10.1093/schbul/sbp020
Crespi, B. (2008). Genomic imprinting in the development and evolution of psychotic spectrum conditions. Biol. Rev. 83, 441–493. doi: 10.1111/j.1469-185x.2008.00050.x
D'Souza, U. M., and Craig, I. W. (2006). Functional polymorphisms in dopamine and serotonin pathway genes. Hum. Mutat. 27, 1–13. doi: 10.1002/humu
Debbané, M., Glaser, B., David, M. K., Feinstein, C., and Eliez, S. (2006). Psychotic symptoms in children and adolescents with 22q11.2 deletion syndrome: Neuropsychological and behavioral implications. Schizophr. Res. 84, 187–193. doi: 10.1016/j.schres.2006.01.019
de Castro-Catala, M., Barrantes-Vidal, N., Sheinbaum, T., Moreno-Fortuny, A., Kwapil, T. R., and Rosa, A. (2015). COMT-by sex interaction effect on psychosis proneness. J. Biomed. Biotechnol. 2015:829237. doi: 10.1155/2015/829237
DeLisi, L. E., Friedrich, U., Wahlstrom, J., Boccio-Smith, A., Forsman, A., Eklund, K., et al. (1994). Schizophrenia and sex chromosome anomalies. Schizophr. Bull. 20, 495–505. doi: 10.1093/schbul/20.3.495
DeLisi, L. E., Maurizio, A. M., Svetina, C., Ardekani, B., Szulc, K., Nierenberg, J., et al. (2005). Klinefelter's syndrome (XXY) as a genetic model for psychotic disorders. Am. J. Med. Genet. 135, 15–23. doi: 10.1002/ajmg.b.30163
DeLisi, L. E., Shaw, S. H., Crow, T. J., Shields, G., Smith, A. B., Larach, V. W., et al. (2002). A genome-wide scan for linkage to chromosomal regions in 382 sibling pairs with schizophrenia or schizoaffective disorder. Am. J. Psychiatry 159, 803–812. doi: 10.1176/appi.ajp.159.5.803
Duan, J., Martinez, M., Sanders, A. R., Hou, C., Saitou, N., Kitano, T., et al. (2004). Polymorphisms in the trace amine receptor 4 (TRAR4) gene on chromosome 6q23.2 are associated with susceptibility to schizophrenia. Am. J. Hum. Gen. 75, 624–638. doi: 10.1086/424887
Fales, C. L., Knowlton, B. J., Holyoak, K. J., Geschwind, D. H., Swerdloff, R. S., and Gonzalo, I. G. (2003). Working memory and relational reasoning in Klinefelter syndrome. J. Int. Neuropsychol. Soc. 9, 839–846. doi: 10.1017/s1355617703960036
Fanous, A. H., Neale, M. C., Gardner, C. O., Webb, B. T., Straub, R. E., O'Neill, F., et al. (2007). Significant correlation in linkage signals from genome-wide scans of schizophrenia and schizotypy. Mol. Psychiatry 12, 958–965. doi: 10.1038/sj.mp.4001996
Fogelson, D. L., Nuechterlein, K. H., Asarnow, R. F., Payne, D. L., Subotnik, K. L., and Giannini, C. A. (1999). The factor structure of schizophrenia spectrum personality disorders: signs and symptoms in relatives of psychotic patients from the UCLA family members study. Psychiatry Res. 87, 137–146. doi: 10.1016/S0165-1781(99)00086-4
Fonseca-Pedrero, E., Debbané, M., Schneider, M., Badoud, D., and Eliez, S. (2016). Schizotypal traits in adolescents with 22q11.2 deletion syndrome: validity, reliability and risk for psychosis. Psychol. Med. 46, 1005–1013. doi: 10.1017/S0033291715002500
Foutz, J., and Mezuk, B. (2015). Advanced paternal age and risk of psychotic-like symptoms in adult offspring. Schizophr. Res. 165, 123–127. doi: 10.1016/j.schres.2015.04.014
Francks, C., Maegawa, S., Laurén, J., Abrahams, B. S., Velayos-Baeza, A., Medland, S. E., et al. (2007). LRRTM1 on chromosome 2p12 is a maternally suppressed gene that is associated paternally with handedness and schizophrenia. Mol. Psychiatry 12, 1129–1057. doi: 10.1038/sj.mp.4002053
Franke, P., Maier, W., Hautzinger, M., Weiffenbach, O., Gänsicke, M., Iwers, B., et al. (1996). Fragile-X carrier females: evidence for a distinct psychopathological phenotype? Am. J. Med. Genet. 64, 334–339.
Fusar-Poli, P., Bechdolf, A., Taylor, M. J., Bonoldi, I., Carpenter, W. T., Yung, A. R., et al. (2013). At risk for schizophrenic or affective psychoses? A meta-analysis of DSM/ICD diagnostic outcomes in individuals at high clinical risk. Schizophr. Bull. 39, 923–932. doi: 10.1093/schbul/sbs060
Glatt, S. J., Faraone, S. V., and Tsuang, M. T. (2003). Association between a functional catechol O-methyltransferase gene polymorphism and schizophrenia: meta-analysis of case-control and family-based studies. Am. J. Psychiatry 160, 469–476. doi: 10.1176/appi.ajp.160.3.469
Gogos, J. A., and Gerber, D. J. (2006). Schizophrenia susceptibility genes: emergence of positional candidates and future directions. Trends Pharmacol. Sci. 27, 226–233. doi: 10.1016/j.tips.2006.02.005
Gothelf, D., Feinstein, C., Thompson, T., Gu, E., Penniman, L., Van Stone, E., et al. (2007). Risk factors for the emergence of psychotic disorders in adolescents with 22q11.2 deletion syndrome. Am. J. Psychiatry 164, 663–669. doi: 10.1176/ajp.2007.164.4.663
Grant, P., Gabriel, F., Kuepper, Y., Wielpuetz, C., and Hennig, J. (2014). Psychosis-proneness correlates with expression levels of dopaminergic genes. Eur. Psychiatry 29, 304–306. doi: 10.1016/j.eurpsy.2013.12.002
Grant, P., Kuepper, Y., Mueller, E. A., Wielpuetz, C., Mason, O. J., and Hennig, J. (2013). Dopaminergic foundations of schizotypy as measured by the German version of the Oxford-Liverpool Inventory of Feelings and Experiences (O-LIFE)-a suitable endophenotype of schizophrenia. Front. Hum. Neurosci. 7:1. doi: 10.3389/fnhum.2013.00001
Grove, W. M., Lebow, B. S., Clementz, B. A., Cerri, A., Medus, C., and Iacono, W. G. (1991). Familial prevalence and coaggregation of schizotypy indicators: a multitrait family study. J. Abnorm. Psychol. 100, 115–121. doi: 10.1037/0021-843X.100.2.115
Gurvich, C., Bozaoglu, K., Neill, E., Van Rheenen, T. E., Tan, E. J., Louise, S., et al. (2016). The dopamine D1 receptor gene is associated with negative schizotypy in a non-clinical sample. Psychiatry Res. 235, 213–214. doi: 10.1016/j.psychres.2015.11.051
Hahn, C.-G., Wang, H.-Y., Cho, D.-S., Talbot, K., Gur, R. E., Berrettini, W. H., et al. (2006). Altered neuregulin 1-erbB4 signaling contributes to NMDA receptor hypofunction in schizophrenia. Nat. Med. 12, 824–828. doi: 10.1038/nm1418
Hamshere, M. L., Green, E. K., Jones, I. R., Jones, L., Moskvina, V., Kirov, G., et al. (2009). Genetic utility of broadly defined bipolar schizoaffective disorder as a diagnostic concept. Br. J. Psychiatry 195, 23–29. doi: 10.1192/bjp.bp.108.061424
Harrison, P. J., and Weinberger, D. R. (2005). Schizophrenia genes, gene expression, and neuropathology: on the matter of their convergence. Mol. Psychiatry 10, 40–68. doi: 10.1038/sj.mp.4001558
Hashimoto, R., Noguchi, H., Hori, H., Nakabayashi, T., Suzuki, T., Iwata, N., et al. (2010). A genetic variation in the dysbindin gene (DTNBP1) is associated with memory performance in healthy controls. World J. Biol. Psychiatry 11(2 Pt 2), 431–438. doi: 10.3109/15622970902736503
Heck, A., Fastenrath, M., Ackermann, S., Auschra, B., Bickel, H., Coynel, D., et al. (2014). Converging genetic and functional brain imaging evidence links neuronal excitability to working memory, psychiatric disease, and brain activity. Neuron 81, 1203–1213. doi: 10.1016/j.neuron.2014.01.010
Hennah, W., Varilo, T., Kestilä, M., Paunio, T., Arajärvi, R., Haukka, J., et al. (2003). Haplotype transmission analysis provides evidence of association for DISC1 to schizophrenia and suggests sex-dependent effects. Hum. Mol. Gen. 12, 3151–3159. doi: 10.1093/hmg/ddg341
Hossein, F. S., and Folsom, T. D. (2011). The role of fragile X mental retardation protein in major mental disorders. Neuropharmacology 60, 1221–1226. doi: 10.1016/j.neuropharm.2010.11.011
Itti, E., Gaw Gonzalo, I. T., Boone, K. B., Geschwind, D. H., Berman, N., Pawlikowska-Haddal, A., et al. (2003). Functional neuroimaging provides evidence of anomalous cerebral laterality in adults with Klinefelter's Syndrome. Ann. Neurol. 54, 669–673. doi: 10.1002/ana.10735
Jones, L. A., Cardno, A. G., Murphy, K. C., Sanders, R. D., Gray, M. Y., McCarthy, G., et al. (2000). The Kings Schizotypy Questionnaire as a quantitative measure of schizophrenia liability. Schizophr. Res. 45, 213–221. doi: 10.1016/S0920-9964(99)00183-8
Kelemen, O., Kovács, T., and Kéri, S. (2013). Contrast, motion, perceptual integration, and neurocognition in schizophrenia: the role of fragile-X related mechanisms. Progress Neurol. 46, 92–97. doi: 10.1016/j.pnpbp.2013.06.017
Kendler, K. S., and Hewitt, J. (1992). The structure of self-report schizotypy in twins. J. Pers. Disord. 6, 1–17. doi: 10.1521/pedi.1992.6.1.1
Kendler, K. S., McGuire, M., Gruenberg, A. M., O'Hare, A. O., Spellman, M., and Walsh, D. (1993). The Roscommon Family Study III. Schizophrenia-related disorders in relatives. Arch. Gen. Psychiatry 50, 781–788.
Kendler, K. S., McGuire, M., Gruenberg, A. M., Walsh, D., and Virginia, V. (1995). Schizotypal symptoms and signs in the Roscommon Family Study. Arch. Gen. Psychiatry 52, 296–303. doi: 10.1001/archpsyc.1995.03950160046009
Kerby, D. S., and Dawson, B. L. (1994). Autistic features, personality and adaptive behaviour in males with fragile X syndrome and no autism. Am. J. Men. Retardation 98, 455–462.
Kheirollahi, M., Kazemi, E., and Ashouri, S. (2016). Brain-derived neurotrophic factor gene Val66Met polymorphism and risk of schizophrenia: a meta-analysis of case–control studies. Cell. Mol. Neurobiol. 36, 1–10. doi: 10.1007/s10571-015-0229-z
Kovács, T., Kelemen, O., and Kéri, S. (2013). Decreased fragile X mental retardation protein (FMRP) is associated with lower IQ and earlier illness onset in patients with schizophrenia. Psychiatry Res. 210, 690–693. doi: 10.1016/j.psychres.2012.12.022
Kwapil, T. R., Gross, G. M., Silvia, P. J., and Barrantes-Vidal, N. (2013). Prediction of psychopathology and functional impairment by positive and negative schizotypy in the Chapmans' ten-year longitudinal study. J. Abnorm. Psychol. 122, 807–815. doi: 10.1037/a0033759
Laity, J. H., Lee, B. M., and Wright, P. E. (2001). Zinc finger proteins: new insights into structural and functional diversity. Curr. Opin. Struct. Biol. 11, 39–46. doi: 10.1016/S0959-440X(00)00167-6
Lataster, J., Collip, D., Ceccarini, J., Hernaus, D., Haas, D., Booij, L., et al. (2014). Familial liability to psychosis is associated with attenuated dopamine stress signaling in ventromedial prefrontal cortex. Schizophr. Bull. 40, 66–77. doi: 10.1093/schbul/sbs187
Law, A. J., Kleinman, J. E., Weinberger, D. R., and Weickert, C. S. (2007). Disease-associated intronic variants in the ErbB4 gene are related to altered ErbB4 splice-variant expression in the brain in schizophrenia. Hum. Mol. Genet. 16, 129–141. doi: 10.1093/hmg/ddl449
Leach, E. L., Hurd, P. L., and Crespi, B. J. (2013). Schizotypy, cognitive performance, and genetic risk for schizophrenia in a non-clinical population. Pers. Individ. Dif. 55, 334–338. doi: 10.1016/j.paid.2013.03.010
Leach, E. L., Prefontaine, G., Hurd, P. L., and Crespi, B. J. (2014). The imprinted gene LRRTM1 mediates schizotypy and handedness in a nonclinical population. J. Hum. Genet. 59, 332–336. doi: 10.1038/jhg.2014.30
Lenzenweger, M. F. (2006). Schizotypy an organising framework for schizoprhenia research. Curr. Dir. Psychol. Sci. 15, 162–167. doi: 10.1111/j.1467-8721.2006.00428.x
Lenzenweger, M. F. (2013). Thinking clearly about the endophenotype-intermediate phenotype-biomarker distinctions in developmental psychopathology research. Dev. Psychopathol. 25(4 Pt 2), 1347–1357. doi: 10.1017/S0954579413000655
Levinson, D. F., Holmans, P., Straub, R. E., Owen, M. J., Wildenauer, D. B., Gejman, P. V., et al. (2000). Multicenter linkage study of schizophrenia candidate regions on chromosomes 5q, 6q, 10p, and 13q: schizophrenia linkage collaborative group III. Am. J. Hum. Genet. 67, 652–663. doi: 10.1086/303041
Li, B., Woo, R.-S., Mei, L., and Malinow, R. (2007). ErbB4, a receptor of the schizophrenia-linked protein neuregulin-1, controls glutamatergic synapse maturation and plasticity. Neuron 54, 583–597. doi: 10.1016/j.neuron.2007.03.028
Lien, Y.-J., Tsuang, H.-C., Chiang, A., Liu, C.-M., Hsieh, M. H., Hwang, T.-J., et al. (2010). The multidimensionality of schizotypy in nonpsychotic relatives of patients with schizophrenia and its applications in ordered subsets linkage analysis of schizophrenia. Am. J. Med. Genet. B 153B, 1–9. doi: 10.1002/ajmg.b.30948
Linney, Y. M., Murray, R. M., Peters, E. R., MacDonald, A. M., Rijsdijk, F., and Sham, P. C. (2003). A quantitative genetic analysis of schizotypal personality traits. Psychol. Med. 33, 803–816. doi: 10.1017/S0033291703007906
Liu, H., Heath, S. C., Sobin, C., Roos, J. L., Galke, B. L., Blundell, M. L., et al. (2002). Genetic variation at the 22q11 PRODH2/DGCR6 locus presents an unusual pattern and increases susceptibility to schizophrenia. Proc. Natl. Acad. Sci. U. S. A. 99, 3717–3722. doi: 10.1073/pnas.042700699
Ma, X., Sun, J., Yao, J., Wang, Q., Hu, X., Deng, W., et al. (2007). A quantitative association study between schizotypal traits and COMT, PRODH and BDNF genes in a healthy Chinese population. Psychiatry Res. 153, 7–15. doi: 10.1016/j.psychres.2007.02.003
Macar, C., Bates, T. C., Heath, A. C., Martin, N. G., and Ettinger, U. (2012). Substantial genetic overlap between schizotypy and neuroticism: a twin study. Behav. Genet. 42, 732–742. doi: 10.1007/s10519-012-9558-6
Mahon, K., Perez-Rodriguez, M. M., Gunawardane, N., and Burdick, K. E. (2013). Dimensional endophenotypes in bipolar disorder: affective dysregulation and psychosis proneness. J. Affect. Disord. 151, 695–701. doi: 10.1016/j.jad.2013.08.003
Martin, A. K., Robinson, G., Reutens, D., and Mowry, B. (2015). Clinical and parental age characteristics of rare copy number variant burden in patients with schizophrenia. Am. J. Med. Genet. B. Neuropsychiatr. Genet. 168B, 374–382. doi: 10.1002/ajmg.b.32321
Mason, O. J. (2015). The assessment of schizotypy and its clinical relevance. Schizophr. Bull. 41, S374–S385. doi: 10.1093/schbul/sbu194
Mata, I., Gilvarry, C. M., Jones, P. B., Lewis, S. W., Murray, R. M., and Sham, P. C. (2003). Schizotypal personality traits in nonpsychotic relatives are associated with positive symptoms in psychotic probands. Schizophr. Bull. 29, 273–283. doi: 10.1093/oxfordjournals.schbul.a007004
Mata, I., Sham, P. C., Gilvarry, C. M., Jones, P. B., Lewis, S. W., and Murray, R. M. (2000). Childhood schizotypy and positive symptoms in schizophrenic patients predict schizotypy in relatives. Schizophr. Res. 44, 129–136. doi: 10.1016/S0920-9964(99)00222-4
McGurk, S. R., Mueser, K. T., Mischel, R., Adams, R., Harvey, P. D., McClure, M. M., et al. (2013). Vocational functioning in schizotypal and paranoid personality disorders. Psychiatry Res. 210, 498–504. doi: 10.1016/j.psychres.2013.06.019
Michealraj, K. A., Jatana, N., Jafurulla, Narayanan, L., Chattopadhyay, A., and Thelma, B. K. (2014). Functional characterization of rare variants in human dopamine receptor D4 gene by genotype-phenotype correlations. Neuroscience 262, 176–189. doi: 10.1016/j.neuroscience.2013.12.059
Miller, P., Byrne, M., Hodges, A. N. N., Lawrie, S. M., David, G., Owens, C., et al. (2002). Schizotypal components in people at high risk of developing schizophrenia: early findings from the Edinburgh High-Risk Study. Br. J. Psychiatry 180, 179–184. doi: 10.1192/bjp.180.2.179
Monks, S., Niarchou, M., Davies, A. R., Walters, J. T. R., Williams, N., Owen, M. J., et al. (2014). Further evidence for high rates of schizophrenia in 22q11.2 deletion syndrome. Schizophr. Res. 153, 231–236. doi: 10.1016/j.schres.2014.01.020
Montag, C., Hall, J., Plieger, T., Felten, A., Markett, S., Melchers, M., et al. (2015). The DRD3 Ser9Gly polymorphism, machiavellianism, and its link to schizotypal personality. J. Neurosci. Psychol. Econ. 8, 48–57. doi: 10.1037/npe0000034
Mors, O., Mortensen, P. B., and Ewald, H. (2001). No evidence of increased risk for schizophrenia or bipolar affective disorder in persons with aneuploidies of the sex chromosomes. Psychol. Med. 31, 425–430. doi: 10.1017/S0033291701003476
Nelson, M. T., Seal, M. L., Pantelis, C., and Phillips, L. J. (2013). Evidence of a dimensional relationship between schizotypy and schizophrenia: a systematic review. Neurosci. Biobehav. Rev. 37, 317–327. doi: 10.1016/j.neubiorev.2013.01.004
Nguyen, G. H., Bouchard, J., Boselli, M. G., Tolstoi, L. G., Keith, L., Baldwin, C., et al. (2003). DNA stability and schizophrenia in twins. Am. J. Med. Genet. 120B, 1–10. doi: 10.1002/ajmg.b.20010
Nordentoft, M., Thorup, A., Petersen, L., Øhlenschlæger, J., Melau, M., Christensen, T. Ø., et al. (2006). Transition rates from schizotypal disorder to psychotic disorder for first-contact patients included in the OPUS trial. A randomized clinical trial of integrated treatment and standard treatment. Schizophr. Res. 83, 29–40. doi: 10.1016/j.schres.2006.01.002
Ohi, K., Hashimoto, R., Nakazawa, T., Okada, T., Yasuda, Y., Yamamori, H., and Takeda, M. (2012). The p250GAP gene is associated with risk for schizophrenia and schizotypal personality traits. PLoS ONE 7:e35696. doi: 10.1371/journal.pone.0035696
Park, Y., and Waldman, I. D. (2014). Influence of the COMT val108/158met polymorphism on continuous performance task indices. Neuropsychologia 61, 45–55. doi: 10.1016/j.neuropsychologia.2014.06.008
Petryshen, T. L., Middleton, F. A., Tahl, A. R., Rockwell, G. N., Purcell, S., Aldinger, K. A., et al. (2005). Genetic investigation of chromosome 5q GABAA receptor subunit genes in schizophrenia. Mol. Psychiatry 10, 1074–1088. doi: 10.1038/sj.mp.4001739
Pimm, J., McQuillin, A., Thirumalai, S., Lawrence, J., Quested, D., Bass, N., et al. (2005). The Epsin 4 gene on chromosome 5q, which encodes the clathrin-associated protein enthoprotin, is involved in the genetic susceptibility to schizophrenia. Am. J. Hum. Genet. 76, 902–907. doi: 10.1086/430095
Porcelli, S., Lee, S. J., Han, C., Patkar, A. A., Serretti, A., and Pae, C. U. (2015). CACNA1C gene and schizophrenia:a case-control and pharmacogenetic study. Psychiatry Genet. 25, 163–167. doi: 10.1097/YPG.0000000000000092
Prasad, S. E., Howley, S., and Murphy, K. C. (2008). Candidate genes and the behavioral phenotype in 22q11.2 deletion syndrome. Dev. Disabil. Res. Rev. 14, 26–34. doi: 10.1002/ddrr.5
Pulay, A. J., Stinson, F. S., Dawson, D. A., Goldstein, R. B., Chou, S. P., Huang, B., et al. (2009). Prevalence, correlates, disability, and comorbidity of DSM-IV Schizotypal Personality Disorder. Prim. Care Companion J. Clin. Psychiatry 11, 53–67. doi: 10.4088/PCC.08m00679
Raine, A. (2006). Schizotypal personality: neurodevelopmental and psychosocial trajectories. Annu. Rev. Clin. Psychol. 2, 291–326. doi: 10.1146/annurev.clinpsy.2.022305.095318
Ramsay, H., Kelleher, I., Flannery, P., Clarke, M. C., Lynch, F., Harley, M., et al. (2013). Relationship between the COMT-Val158Met and BDNF-Val66Met polymorphisms, childhood trauma and psychotic experiences in an adolescent general population sample. PLoS ONE 8:e79741. doi: 10.1371/journal.pone.0079741
Reiss, A. L., Eliez, S., Schmitt, J. E., Patwardhan, A., and Haberecht, M. (2000). Brain imaging in neurogenetic conditions: realizing the potential of behavioral neurogenetics research. Ment. Retard. Dev. Disabil. Res. Rev. 6, 186–197.
Reiss, A. L., Hagerman, R. J., Vinogradov, S., Abrams, M., and King, R. J. (1988). Psychiatric disability in female carriers of the fragile X chromosome. Arch. Gen. Psychiatry 45, 25–30. doi: 10.1001/archpsyc.1988.01800250029005
Roussos, P., Bitsios, P., Giakoumaki, S. G., McClure, M. M., Hazlett, E. A., New, A. S., et al. (2013). CACNA1C as a risk factor for schizotypal personality disorder and schizotypy in healthy individuals. Psychiatry Res. 206, 122–123. doi: 10.1016/j.psychres.2012.08.039
Roussos, P., Giakoumaki, S. G., Georgakopoulos, A., Robakis, N. K., and Bitsios, P. (2011). The CACNA1C and ANK3 risk alleles impact on affective personality traits and startle reactivity but not on cognition or gating in healthy males. Bipolar Disord. 13, 250–259. doi: 10.1111/j.1399-5618.2011.00924.x
Salokangas, R. K. R., and McGlashan, T. H. (2008). Early detection and intervention of psychosis. A review. Nordic J. Psychiatry 62, 92–105. doi: 10.1080/08039480801984008
Schneider, M., Van der Linden, M., Menghetti, S., Debbané, M., and Eliez, S. (2015). Negative and paranoid symptoms are associated with negative performance beliefs and social cognition in 22q11.2 deletion syndrome. Early Interv. Psychiatry. doi: 10.1111/eip.12224. [Epub ahead of print].
Schürhoff, F., Szöke, A., Chevalier, F., Roy, I., Méary, A., Bellivier, F., et al. (2007). Schizotypal dimensions: an intermediate phenotype associated with the COMT high activity allele. Am. J. Med. Genet. B 144, 64–68. doi: 10.1002/ajmg.b.30395
Seeman, M. V, and Seeman, P. (2014). Is schizophrenia a dopamine supersensitivity psychotic reaction. Prog. Neuropsychopharmacol. Biol. Psychiatry 48, 155–160. doi: 10.1016/j.pnpbp.2013.10.003
Smallman, R. P., Barkus, E., Azadbakht, H., Embleton, K. V., Haroon, H. A., Lewis, S. W., et al. (2014). MRI diffusion tractography study in individuals with schizotypal features: a pilot study. Psychiatry Res. 221, 49–57. doi: 10.1016/j.pscychresns.2013.10.006
Smyrnis, N., Avramopoulos, D., Evdokimidis, I., Stefanis, C. N., Tsekou, H., and Stefanis, N. C. (2007). Effect of schizotypy on cognitive performance and its tuning by COMT val158 met genotype variations in a large population of young men. Biol. Psychiatry 61, 845–853. doi: 10.1016/j.biopsych.2006.07.019
Sobesky, W. E., Hull, C. E., and Hagerman, R. J. (1994). Symptoms of schizotypal personality disorder in fragile X women. J. Am. Acad. Child Adolesc. Psychiatry 33, 247–255. doi: 10.1097/00004583-199402000-00014
Solanki, R. K., Swami, M. K., Singh, P., and Gupta, S. (2012). Identification of vulnerability among first-degree relatives of patients with schizophrenia. East Asian Arch. Psychiatry 22, 118–125.
Stefanis, N. C., Hatzimanolis, A., Avramopoulos, D., Smyrnis, N., Evdokimidis, I., Stefanis, C. N., et al. (2013). Variation in psychosis gene ZNF804A is associated with a refined schizotypy phenotype but not neurocognitive performance in a large young male population. Schizophr. Bull. 39, 1252–1260. doi: 10.1093/schbul/sbs110
Stefanis, N. C., Hatzimanolis, A., Smyrnis, N., Avramopoulos, D., Evdokimidis, I., van Os, J., et al. (2011). Schizophrenia candidate gene ERBB4: covert routes of vulnerability to psychosis detected at the population level. Schizophr. Bull. 39, 349–357. doi: 10.1093/schbul/sbr169
Stefanis, N. C., Trikalinos, T. A., Avramopoulos, D., Smyrnis, N., Evdokimidis, I., Ntzani, E. E., et al. (2008). Association of RGS4 variants with schizotypy and cognitive endophenotypes at the population level. Behav. Brain Funct. 4:46. doi: 10.1186/1744-9081-4-46
Stefanis, N. C., Trikalinos, T. A., Avramopoulos, D., Smyrnis, N., Evdokimidis, I., Ntzani, E. E., et al. (2007). Impact of schizophrenia candidate genes on schizotypy and cognitive endophenotypes at the population level. Biol. Psychiatry 62, 784–792. doi: 10.1016/j.biopsych.2006.11.015
Straub, R. E., Jiang, Y., MacLean, C. J., Ma, Y., Webb, B. T., Myakishev, M. V., et al. (2002). Genetic variation in the 6p22.3 gene DTNBP1, the human ortholog of the mouse dysbindin gene, is associated with schizophrenia. Am. J. Hum. Genet. 71, 337–348. doi: 10.1086/341750
Sun, Y., Hu, D., Liang, J., Bao, Y.-P., Meng, S.-Q., Lu, L., et al. (2015). Association between variants of zinc finger genes and psychiatric disorders: systematic review and meta-analysis. Schizophr. Res. 162, 124–137. doi: 10.1016/j.schres.2015.01.036
Talbot, K., Cho, D. S., Ong, W. Y., Benson, M., Han, L. Y., Kazi, H., et al. (2006). Dysbindin-1 is a synaptic and microtubular protein that binds brain snapin. Hum. Mol. Genet. 15, 3041–3054. doi: 10.1093/hmg/ddl246
Talbot, K., Eidem, W. L., Tinsley, C. L., Benson, M. A., Thompson, E. W., Smith, R. J., et al. (2004). Dysbindin-1 is reduced in intrinsic, glutamatergic terminals of the hippocampal formation in schizophrenia. J. Clin. Invest. 113, 1353–1363. doi: 10.1172/JCI200420425
Taurisano, P., Romano, R., Mancini, M., Giorgio, A., Di Antonucci, L. A., Fazio, L., et al. (2014). Prefronto-striatal physiology is associated with schizotypy and is modulated by a functional variant of DRD2. Front. Behav. Neurosci. 8:235. doi: 10.3389/fnbeh.2014.00235
Tochigi, M., Zhang, X., Ohashi, J., Hibino, H., Otowa, T., Rogers, M., et al. (2006). Association study of the dysbindin (DTNBP1) gene in schizophrenia from the Japanese population. Neurosci. Res. 56, 154–158. doi: 10.1016/j.neures.2006.06.009
Tosato, S., Ruggeri, M., Bonetto, C., Bertani, M., Marrella, G., Lasalvia, A., et al. (2007). Association study of dysbindin gene with clinical and outcome measures in a representative cohort of Italian schizophrenic patients. Am. J. Med. Genet. B. Neuropsychiatr. Genet. 144B, 647–659. doi: 10.1002/ajmg.b.30484
Tsiouris, J. A., and Brown, W. T. (2004). Neuropsychiatric symptoms of Fragile X Syndrome. CNS Drugs 18, 687–703. doi: 10.2165/00023210-200418110-00001
Tsuang, M. (2000). Schizophrenia: genes and environment. Biol. Psychiatry 47, 210–220. doi: 10.1016/S0006-3223(99)00289-9
Van Rijn, S., Aleman, A., De Sonneville, L., and Swaab, H. (2009). Cognitive mechanisms underlying disorganization of thought in a genetic syndrome (47,XXY). Schizophr. Res. 112, 91–98. doi: 10.1016/j.schres.2009.04.017
Van Rijn, S., Aleman, A., Swaab, H., and Kahn, R. (2006). Klinefelter's syndrome (karyotype 47, XXY) and schizophrenia-spectrum pathology. Br. J. Psychiatry 189, 459–460. doi: 10.1192/bjp.bp.105.008961
Venables, P., Wilkins, S., Mitchell, D., Raine, A., and Bailes, K. (1990). A scale for the measurement of schizotypy. Pers. Individ. Dif. 11, 481–495. doi: 10.1016/0191-8869(90)90061-U
Verhoeven, W. M., and Egger, J. I. M. (2011). Schizotypy: Key feature of Klinefelter's syndrome? BMJ Case Rep. 2011:bcr0520114279. doi: 10.1136/bcr.05.2011.4279
Vilella, E., Costas, J., Sanjuan, J., Guitart, M., De Diego, Y., Carracedo, A., et al. (2008). Association of schizophrenia with DTNBP1 but not with DAO, DAOA, NRG1 and RGS4 nor their genetic interaction. J. Psychiatr. Res. 42, 278–288. doi: 10.1016/j.jpsychires.2007.02.005
Vollema, M. G., Sitskoorn, M. M., Appels, M. C., and Kahn, R. S. (2002). Does the Schizotypal Personality Questionnaire reflect the biological-genetic vulnerability to schizophrenia? Schizophr. Res. 54, 39–45. doi: 10.1016/S0920-9964(01)00350-4
Waldeck, T. L., and Miller, L. S. (2000). Social skills deficits in schizotypal personality disorder. Psychiatry Res. 93, 237–246. doi: 10.1016/S0165-1781(00)00122-0
Williams, M. B. (1993). The Psychometric Assessment of Schizotypal Personality. London: University of London.
Wing, J. K., Cooper, J. E., and Sartorius, N. (1974). The Measurement and Classification of Psychiatric Symptoms. Cambridge: Cambridge University Press.
Woodward, N. D., Cowan, R. L., Park, S., Ansari, M. S., Baldwin, R. M., Li, R., et al. (2011). Correlation of individual differences in schizotypal personality traits with amphetamine-induced dopamine release in striatal and extrastriatal brain regions. Am. J. Psychiatry 168, 418–426. doi: 10.1176/appi.ajp.2010.10020165
World Health Organization. (1992). The International Personality Disorder Examination (IPDE). Geneva: World Health Organization.
Xu, B., Woodroffe, A., Rodriguez-Murillo, L., Roos, J. L., van Rensburg, E. J., Abecasis, G. R., et al. (2009). Elucidating the genetic architecture of familial schizophrenia using rare copy number variant and linkage scans. Proc. Natl. Acad. Sci. U.S.A. 106, 16746–16751. doi: 10.1073/pnas.0908584106
Yang, H. C., Liu, C. M., Liu, Y. L., Chen, C. W., Chang, C. C., Fann, C. S. J., et al. (2013). The DAO gene is associated with schizophrenia and interacts with other genes in the Taiwan Han Chinese population. PLoS ONE 8:e60099. doi: 10.1371/journal.pone.0060099
Yaralian, P. S., Raine, A., Lencz, T., Hooley, J. M., Bihrle, S. E., Mills, S., et al. (2000). Elevated levels of cognitive-perceptual deficits in individuals with a family history of schizophrenia spectrum disorders. Schizophr. Res. 46, 57–63. doi: 10.1016/S0920-9964(99)00239-X
Yasuda, Y., Hashimoto, R., Ohi, K., Fukumoto, M., Umeda-Yano, S., Yamamori, H., et al. (2011). Impact on schizotypal personality trait of a genome-wide supported psychosis variant of the ZNF804A gene. Neurosci. Lett. 495, 216–220. doi: 10.1016/j.neulet.2011.03.069
Zammit, S., Hamshere, M., Dwyer, S., Georgiva, L., Timpson, N., Moskvina, V., et al. (2014). A population-based study of genetic variation and psychotic experiences in adolescents. Schizophr. Bull. 40, 1254–1262. doi: 10.1093/schbul/sbt146
Keywords: polymorphisms, schizotypy, schizophrenia, psychosis risk, genetic disorders, family studies
Citation: Walter EE, Fernandez F, Snelling M and Barkus E (2016) Genetic Consideration of Schizotypal Traits: A Review. Front. Psychol. 7:1769. doi: 10.3389/fpsyg.2016.01769
Received: 17 August 2016; Accepted: 27 October 2016;
Published: 15 November 2016.
Edited by:
Kimberly J. Saudino, Boston University, USAReviewed by:
Jeffrey R. Gagne, University of Texas at Arlington, USACaroline Gurvich, Monash University, Australia
Copyright © 2016 Walter, Fernandez, Snelling and Barkus. This is an open-access article distributed under the terms of the Creative Commons Attribution License (CC BY). The use, distribution or reproduction in other forums is permitted, provided the original author(s) or licensor are credited and that the original publication in this journal is cited, in accordance with accepted academic practice. No use, distribution or reproduction is permitted which does not comply with these terms.
*Correspondence: Emma Barkus, ZWJhcmt1c0B1b3cuZWR1LmF1