- 1BioMotion Center, Institute of Sports and Sports Science, Karlsruhe Institute of Technology, Karlsruhe, Germany
- 2Department of Prosthodontics, Dental School, University of Heidelberg, Heidelberg, Germany
- 3Research Group Biomechanics, Institute for Mechanics, Karlsruhe Institute of Technology, Karlsruhe, Germany
- 4Institute of Biomechanics and Orthopaedics, German Sport University Cologne, Cologne, Germany
- 5ARCUS Clinics Pforzheim, Pforzheim, Germany
Postural control is crucial for most tasks of daily living, delineating postural orientation and balance, with its main goal of fall prevention. Nevertheless, falls are common events, and have been associated with deficits in muscle strength and dynamic postural stability. Recent studies reported on improvements in rate of force development and static postural control evoked by jaw clenching activities, potentially induced by facilitation of human motor system excitability. However, there are no studies describing the effects on dynamic stability. The present study, therefore, aimed to investigate the effects of submaximum jaw clenching on recovery behavior from forward loss of balance. Participants were 12 healthy young adults, who were instructed to recover balance from a simulated forward fall by taking a single step while either biting at a submaximum force or keeping the mandible at rest. Bite forces were measured by means of hydrostatic splints, whereas a 3D motion capture system was used to analyze spatiotemporal parameters and joint angles, respectively. Additionally, dynamic stability was quantified by the extrapolated CoM concept, designed to determine postural stability in dynamic situations. Paired t-tests revealed that submaximum biting did not significantly influence recovery behavior with respect to any variable under investigation. Therefore, reductions in postural sway evoked by submaximum biting are obviously not transferable to balance recovery as it was assessed in the present study. It is suggested that these contradictions are the result of different motor demands associated with the abovementioned tasks. Furthermore, floor effects and the sample size might be discussed as potential reasons for the absence of significances. Notwithstanding this, the present study also revealed that bite forces under both conditions significantly increased from subjects’ release to touchdown of the recovery limb. Clenching the jaw, hence, seems to be part of a common physiological repertoire used to improve motor performance.
Introduction
Postural control is crucial for most activities of daily living, and comprises the neuromuscular control of postural orientation and postural equilibrium; the latter is commonly referred to as balance. Whereas postural orientation involves the positioning of the body’s segments in space with respect to gravity, postural equilibrium delineates the ability to control the center of mass (CoM) within the base of support (BoS) (Woollacott and Shumway-Cook, 2002; Macpherson and Horak, 2013).
The main function of the postural control system is to maintain stability, and thus to prevent any falls resulting from internal or external forces (Horak and Macpherson, 1996). However, due to the large variety of structures involved in this complex sensorimotor process, falls are common and serious events, potentially leading to severe injuries or even to death. Most of these falls occur during locomotion, such as tripping or slipping while walking (Blake et al., 1988). Investigations on postural control and fall related events, hence, are a particular issue of concern – for the scientific community, for the public health care system, and also for the fall-prone persons and patients themselves.
Do et al. (1982) were the first to introduce an experimental paradigm for assessing recovery behavior during forward loss of balance. This paradigm, in which subjects are suddenly released from a static forward lean angle, is still frequently used (Wojcik et al., 2001; Karamanidis and Arampatzis, 2007; Arampatzis et al., 2008; Karamanidis et al., 2008; Curtze et al., 2010; Barrett et al., 2012; Carty et al., 2012; Cheng et al., 2014; Graham et al., 2014), and has been shown to evince postural deficits in diverse populations. In this context, force potential of leg extensor muscles (Karamanidis et al., 2008), effective control of the whole body CoM (Barrett et al., 2012), as well as step length and step velocity (Carty et al., 2012) have been identified as important variables for dynamic postural stability.
In recent years, several studies reported on the potential benefits of jaw clenching on human postural control. Thereby, a significant decrease in center of pressure (CoP) displacements induced by submaximum bite forces has been revealed by posturographic analyses (Hellmann et al., 2011; Ringhof et al., 2015b). These reductions in postural sway were accompanied by decreased sway of cranial body segments (Ringhof et al., 2015b), concomitant with alterations in muscular co-contraction patterns and systematic reductions in joint motions of the lower extremities (Hellmann et al., 2015). Modulation of somatosensory input, particularly for the neck muscles (Abrahams, 1977), and facilitation of human motor system excitability (Boroojerdi et al., 2000) were suggested to be the main causes for these improvements. In addition, facilitating effects on ankle extensor and flexor muscles (Miyahara et al., 1996; Takada et al., 2000), concomitant with attenuated reciprocal Ia inhibition from the pretibial muscles to the soleus muscle (Takada et al., 2000), which have been reported as a result of concurrent jaw clenching activities, might have contributed to the abovementioned stabilizing effects. Neuroanatomical connections and projections of the trigeminal nerve to structures associated with postural control (Ruggiero et al., 1981; Buisseret-Delmas et al., 1999; Devoize et al., 2010) are thought to form the basis for these findings.
Whereas the effects of jaw clenching on static postural control are merely consistent, there is no clear evidence as to whether dental occlusion in general affects postural sway; and also the mechanisms supporting this potential effect are still debated, and far from having reached a consensus (Cuccia and Caradonna, 2009; Michelotti et al., 2011; Manfredini et al., 2012). On the one hand, there are several studies in which significant sway reductions were observed, depending on the relative position of the mandible. Specifically, CoP displacements were found to be significantly decreased when the mandible was in symmetric centric relation as compared to intercuspal or lateral occlusion (Gangloff et al., 2000; Bracco et al., 2004; Sakaguchi et al., 2007). Contradictory results are provided by Ferrario et al. (1996), reporting that postural sway was not significantly influenced by five dental positions, either in healthy women or in women with temporomandibular disorders and asymmetric malocclusion. Perinetti (2006, 2007) confirmed these findings in terms of non-significant differences between intercuspidation and mandibular rest positions under eyes open and eyes closed conditions, disputing any relationship at the posturography level between dental occlusion and body sway. Some of this work has been criticized, however, primarily because of weak experimental designs and lack of control conditions. Moreover, in most of the publications, unfortunately, descriptions of the experimental design are inadequate. Some of the weak points are the lack of information concerning the generated bite forces and the mandibular positions during the experiments. In particular, when assessing the impact of dental occlusion on postural control, the actual oral motor activity mostly remained unknown. Furthermore, there is no international consensus about the definition of a physiological centric jaw relation (Keshvad and Winstanley, 2000). The common used phrase of symmetric positioning of the mandible in centric relation is, thus, not meaningful, and the jaw positions as experimental conditions are not comparable (Hellmann et al., 2015).
In conclusion, the contradictory reports merely are a consequence of diverse, potentially affecting experimental conditions and/or task instructions. The findings concerning the effects of jaw clenching on postural control are mostly consistent, however. Notwithstanding this, previous studies exclusively focused on the influence of force-controlled biting on postural sway under static conditions, i.e., upright unperturbed stance. To the best of the authors’ knowledge, there are no reports describing the effects of jaw clenching on postural stability in dynamic situations; which is much more related to the risk of falling than static postural control (Rubenstein, 2006).
The purpose of this study, therefore, was to investigate the effects of submaximum jaw clenching on dynamic stability and lower extremity joint kinematics during balance recovery after forward loss of balance. This methodological approach comprises components of postural control, muscular strength and reaction time. As clenching of the jaw was shown to significantly improve reflex facilitation (Miyahara et al., 1996; Takada et al., 2000), static postural control (Hellmann et al., 2011, 2015; Ringhof et al., 2015b), force production, and rate of force development (Forgione et al., 1991; Hiroshi, 2003; Ebben et al., 2008), it was hypothesized that submaximum biting would lead to improved balance recovery in terms of increased dynamic stability. We also hypothesized a concomitant decrease in joint flexion angles of the knee and hip of the recovery limb at touchdown as well as during the subsequent stance phase.
Materials and Methods
Subjects
Twelve healthy young adults, 10 males and 2 females, with a mean age of 21.8 ± 1.8 years (height: 1.78 ± 0.04 m; mass: 72.85 ± 2.35 kg) participated in the study. All participants were naïve to the experiments, and presented with full dentition (except for third molars) in neutral occlusion. None of them had any self-reported muscular or neurological diseases that could have affected their ability to perform the experiments.
The study was approved by the Ethics Committee of the German Sport University Cologne (no. 38/12), and written informed consent was given by all subjects.
Experimental Procedure
To evaluate the effects of jaw clenching on balance recovery, a crossover design was applied. The experimental design included a balance recovery task in the form of a simulated forward fall, and two oral motor tasks: force-controlled biting and non-biting. The order of oral motor tasks was counterbalanced across the subjects, i.e., half of the sample started with force-controlled biting, whereas the others first performed the non-biting control condition.
Oral Motor Tasks
Force-controlled biting (FB) was conducted at submaximum bite forces of 150 N, corresponding to mean individual maximum voluntary contraction of the masseter of 15.07 ± 4.47%. This bite force is in accordance with previous experiments (Hellmann et al., 2011), revealing that submaximum biting significantly affected postural sway in upright unperturbed stance. To monitor the bite forces, a hydrostatic system consisting of liquid-filled pads fixed to the maxilla was used. Biting on the pads resulted in increased hydrostatic pressure, which was presented to the subjects as numerical real-time feedback on a display positioned directly in front of them. Detailed information on the hydrostatic system and the oral splints can be obtained from Hellmann et al. (2011) and Ringhof et al. (2015b).
In the non-biting control condition (NB) the oral device was worn as well, but the subjects were asked to keep their mandible in a resting position, that is consciously applying no bite force, and monitoring this condition by looking at the feedback screen.
Balance Recovery Task
Forward falls were simulated by an experimental approach that has been previously reported by Do et al. (1982), and Karamanidis and Arampatzis (2007). Within this test, subjects were instructed to attempt to recover balance by taking a rapid single step after being suddenly released from an inclined forward posture (Wojcik et al., 2001; Karamanidis et al., 2008).
In the present study, the forward-inclined position was attained by a horizontal cable that was attached to a safety harness worn by the subjects around the trunk. At the other end, the horizontal cable was connected to an electromagnetic system, which could be manually released by the investigators (Figure 1). To avoid any injuries resulting from falls, the safety harness additionally was attached to a ceiling-mounted rope, which prevented contact of any body part, other than the feet, with the ground (Karamanidis et al., 2008).
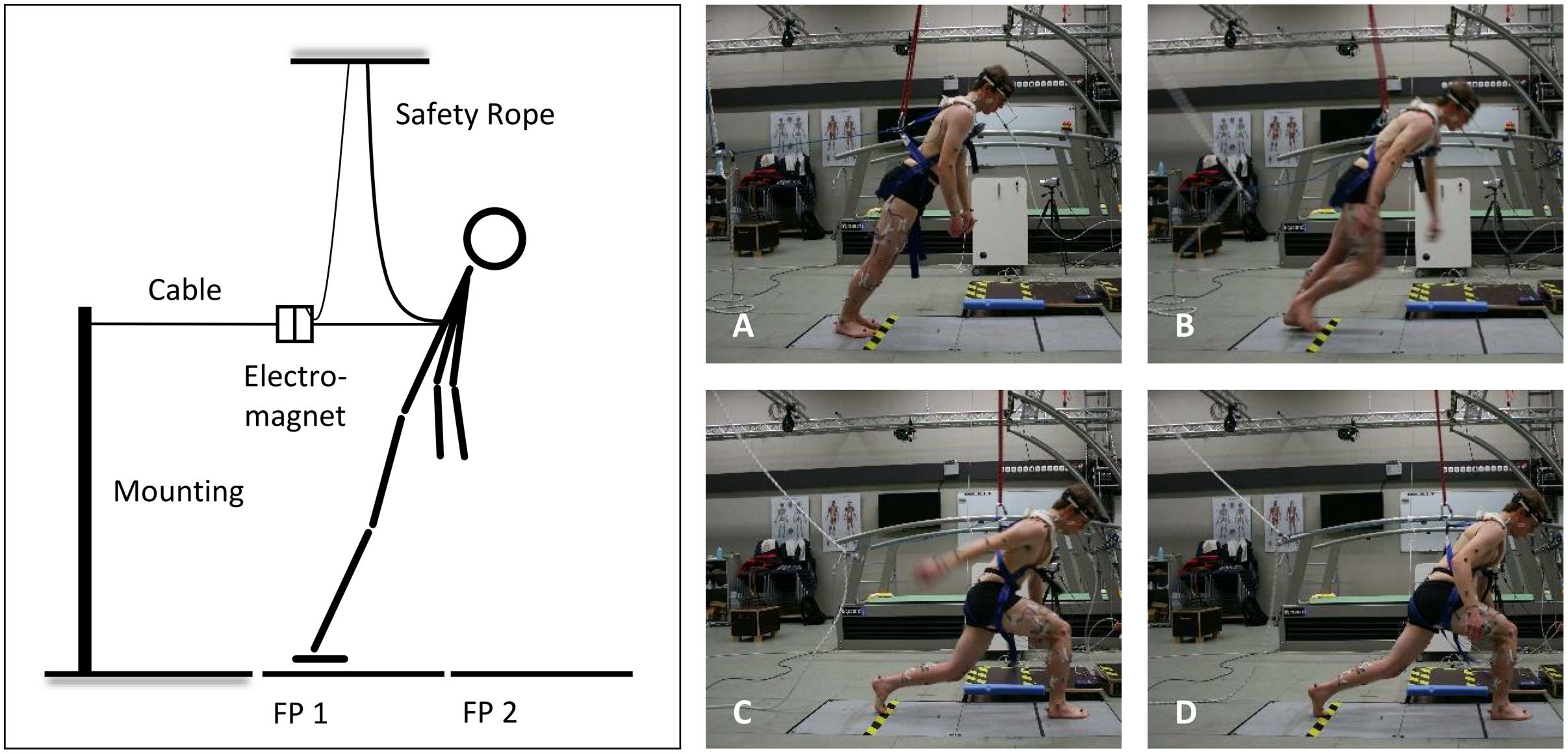
FIGURE 1. Schematic illustration of the experimental setup (left) and the analyzed time points (right): release of the subject (A), toe-off from the ground of the recovery limb (B), touchdown of the recovery limb (C), and 500 ms after touchdown (D); FP, force plate.
At the beginning of each trial, the subjects stood barefoot with both feet on a force plate (AMTI, model BP600900, 1,000 Hz; Advanced Mechanical Technology, Watertown, MA, USA), and were then moved in a forward-inclined position. The angle of this leaning position was individually adjusted for each subject within a pilot trial prior to the measurements. Thereby, the lean angle – defined as the angle between the vertical in the sagittal plane and the line connecting the CoM and the center of the ankle joint – was gradually increased until the subjects no longer felt able to recover balance by taking a single step. Once the lean angle was determined, this angle was maintained throughout all recovery trials. The mean angle of the forward lean was 36.15 ± 1.38°, evoking a mean horizontal force component of 29.65 ± 2.99% of the subject’s body weight; which is very similar to the loads used by Karamanidis et al. (2008), Barrett et al. (2012), and Carty et al. (2012).
In this position, with heels touching the ground and arms hanging at their sides, the subjects were asked to concurrently perform the oral motor tasks. The respective bite force had to be maintained for at least 2 s until the investigators randomly released the electromagnetic system within a timeframe of 5 s. Once the forward fall was initiated, the subjects were encouraged to restore balance by taking a rapid single step placing their recovery limb properly in front of their other limb.
After one familiarization trial, for each test condition five trials were conducted.
Measurements
All data collected were simultaneously recorded by a Vicon motion capture system (Vicon Motion Systems; Oxford Metrics Group, Oxford, UK). As indicated above, bite forces were measured by means of a hydrostatic system, sampling at 1,000 Hz. Besides, kinematic data were captured by use of thirteen infrared cameras (Vicon MX camera system, 200 Hz) and 39 passive reflective markers (diameter 14 mm). The reflective markers were placed on the subjects’ skin in accordance with the Vicon Plug-In Gait full-body marker set. Based on this, mathematical human multibody models (Kadaba et al., 1990; Davis et al., 1991) allowed for the definition of rigid body segments and its CoM, and the calculation of kinematic parameters, such as joint angles.
Data Analysis
As all participants were able to successfully recover balance with a single step, all five trials were included in the analyses based on which mean values were calculated for each test condition. Hereto, data were processed using MATLAB R2014b (The MathWorks, Natick, MA, USA).
Initially, the time series were filtered by use of a fourth-order Butterworth low-pass filter with a cut-off frequency of 8 Hz. To determine the potential effects of FB on balance recovery, thereafter, for each trial four time points were identified (Figure 1): release of the subject (Release, 1A), toe-off from the ground of the recovery limb (Toe-Off, 1B), touchdown of the recovery limb (Touchdown, 1C), and 500 ms after touchdown (TD+500, 1D) (Karamanidis et al., 2008). For time-normalized analyses, additionally two main phases of recovery were defined: the Falling phase covered the time interval from Release until Touchdown (normalized from –100 to 0%), and the Stance phase involved the period between Touchdown and TD+500 (normalized from 0 to 100%).
Spatiotemporal Parameters
Based on the abovementioned time points, subjects’ response time and duration of recovery were determined. The response time was considered as the time interval from Release until Toe-Off, and the duration of recovery was indicated by the time interval from Release until Touchdown. Further, the step length, defined as the linear distance between the initial and final toe position in anteroposterior direction, was calculated.
Joint Angles
Joint kinematics were analyzed in sagittal plane for the hip, knee, and ankle joints of the recovery limb. Specifically, mean joint angles at Touchdown and maximum joint flexion angles (dorsiflexion angle in terms of the ankle joint) during the Stance phase were investigated.
Dynamic Stability
Postural stability was quantified by the extrapolated CoM concept (Hof et al., 2005). This concept is based on the inverted pendulum model of balance and allows to determine postural stability in dynamic situations (Hof et al., 2005; Arampatzis et al., 2008; Hof, 2008; Curtze et al., 2010). Hereto, the margin of stability (MoS) in anteroposterior direction was calculated as it has been proposed by Hof et al. (2005):
in which pBoS is the anterior boundary of the BoS (projection of the anteroposterior position of the toe from the recovery limb on the ground), and xCoM is the extrapolated CoM in the anteroposterior direction. The extrapolated CoM in turn was calculated as follows:
where pCoM is the anteroposterior component of the CoM (projection of the anteroposterior position of the CoM on the ground), vCoM is the anteroposterior velocity of the CoM, g is the acceleration of gravity, and l is the distance between the CoM and the center of the ankle joint in the sagittal plane (Hof, 2008).
The extrapolated CoM concept suggests that postural stability in anteroposterior direction is maintained when the projection of the extrapolated CoM is located within the BoS, i.e., the MoS shows positive values (Hof et al., 2005; Karamanidis et al., 2008). A loss of dynamic stability in turn is indicated by negative values of the MoS, i.e., in cases where the extrapolated CoM exceeds the anterior boundary of the BoS. The moment the MoS changed from negative to positive values (subsequently referred to as stability point), therefore was depicted as the main outcome parameter. In addition, dynamic stability was calculated for the moments of Touchdown and TD+500.
Statistics
All statistical tests were performed by use of IBM SPSS Statistics 22.0 (IBM Corporation, Armonk, NY, USA). First, Kolmogorov–Smirnov tests were applied to confirm the normality of data distribution. Mauchly’s tests of sphericity were then conducted to determine whether the assumption of sphericity was violated. When this did occur, Greenhouse–Geisser estimates were used to correct for any violations.
Although the ordering was counterbalanced across the subjects, which minimized the likelihood of confounding, preliminary analyses were conducted to evaluate whether the order of exposure had an effect on the variables under investigation. However, repeated measures ANOVAs indicated that neither the order of presentation nor the trial number within both test conditions had been influential.
To check whether the subjects met the requested oral motor tasks, one-sample t-tests contrasted the intended and actual bite forces at subjects’ Release for both oral motor tasks. Differences in bite forces between oral motor tasks [FB, NB] and between time points [Release, Touchdown] were investigated by two-way repeated measures ANOVA. Finally, the effects of oral motor tasks on spatiotemporal parameters, joint angles, and dynamic stability were assessed by paired t-tests, separately run for each dependent variable under investigation.
All data are presented as mean values and 95% confidence intervals (mean ± CI95%). Effect sizes were determined using Cohen’s d (small effect: d = 0.20; medium effect: d = 0.50; large effect d = 0.80) or partial eta squared (small effect: ; medium effect: ; large effect ) in case of t-tests and ANOVAs, respectively (Cohen, 1988; Richardson, 2011). For all statistical tests, the level of significance was set a priori to p = 0.05.
Results
Bite Forces
The time-normalized bite forces from Release until TD+500 are shown in Figure 2. Descriptively, bite forces under FB conditions increased from 150 N at Release to over 200 N at Toe-Off, with a subsequent decrease to baseline values. But, also in NB slight increases in bite force from Release to Toe-Off and to Touchdown were observed.
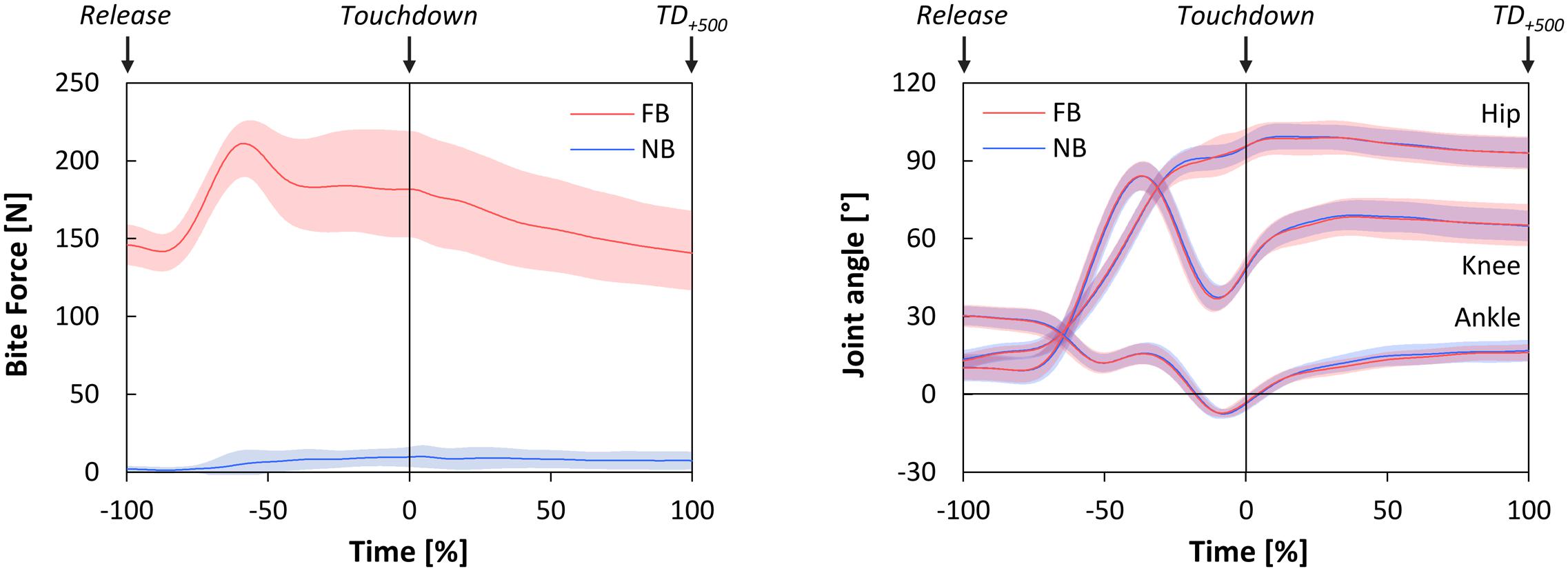
FIGURE 2. Time-normalized bite forces (left), and time-normalized joint angles of the hip, knee, and ankle joints in sagittal plane (right) during Falling phase and Stance phase as functions of oral motor tasks (FB, force-controlled biting; NB, non-biting). Falling phase: from Release until Touchdown (normalized from –100 to 0%); Stance phase: from Touchdown until 500 ms after Touchdown (TD+500) (normalized from 0 to 100%). All data are presented as mean ± CI95.
Statistical tests revealed no significant deviations of the actual bite forces from the intended bite forces at Release [FB: p = 0.515; NB: p = 0.056], and thus confirmed the compliance with the oral motor tasks. On the other hand, significant main effects of oral motor tasks [p < 0.001, ] and time points [p = 0.006, ] were indicated by two-way repeated measures ANOVA. Post hoc analysis (paired t-tests) revealed that bite forces were statistically higher under FB as compared to NB, both at Release [t(11) = 26.03, p < 0.001, d = 9.64] and at Touchdown [t(11) = 12.92, p < 0.001, d = 4.82]. In addition, bite forces under both oral motor tasks significantly increased from Release to Touchdown [FB: t(11) = 3.03, p = 0.011, d = 0.96; NB: t(11) = 2.94, p = 0.014, d = 1.03].
Spatiotemporal Parameters
Spatiotemporal parameters were analyzed to provide a general view on the effects of jaw clenching on balance recovery. However, the response time and the duration of recovery were both not significantly influenced by oral motor tasks. Moreover, there was no significant difference between the subjects’ step length in the two testing conditions (Table 1).
Joint Angles
The time-normalized joint angles of the hip, knee, and ankle joints in the sagittal plane are illustrated in Figure 2. All joint angles at Touchdown were statistically unaffected by oral motor tasks. Additionally, maximum joint flexion angles in Stance phase showed no significant differences between FB and NB (Table 2).
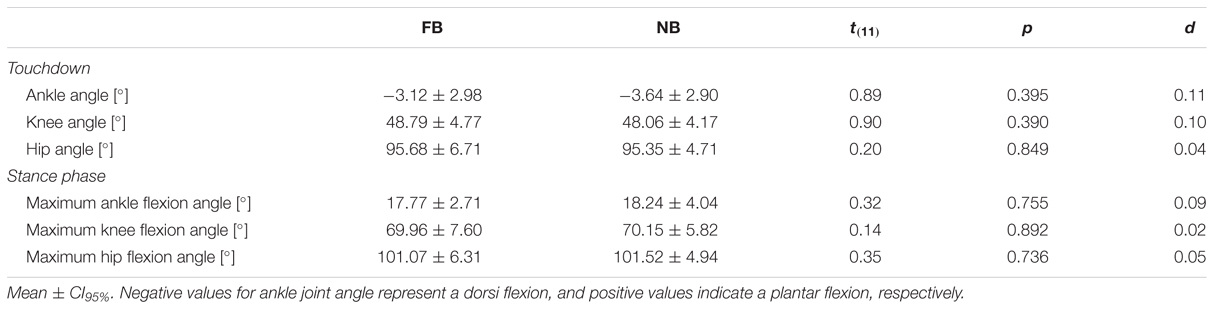
TABLE 2. Joint flexion angles at Touchdown, and maximum joint flexion angles during Stance phase for force-controlled biting (FB) and non-biting (NB).
Dynamic Stability
Figure 3 shows the time-normalized data of the MoS, the extrapolated CoM, and the anterior boundary of the BoS. The stability point was obtained at -23.88 ± 2.33% and -23.22 ± 2.21% of the falling phase for FB and NB, respectively. In particular, paired t-test indicated no significant difference in this point between testing conditions [t(11) = 1.48, p = 0.168, d = 0.19]. Further, FB did not significantly affect the anterior boundary of the BoS, the anteroposterior component and velocity of the CoM, the extrapolated CoM, and the MoS; neither at Touchdown nor at TD+500 (Table 3).
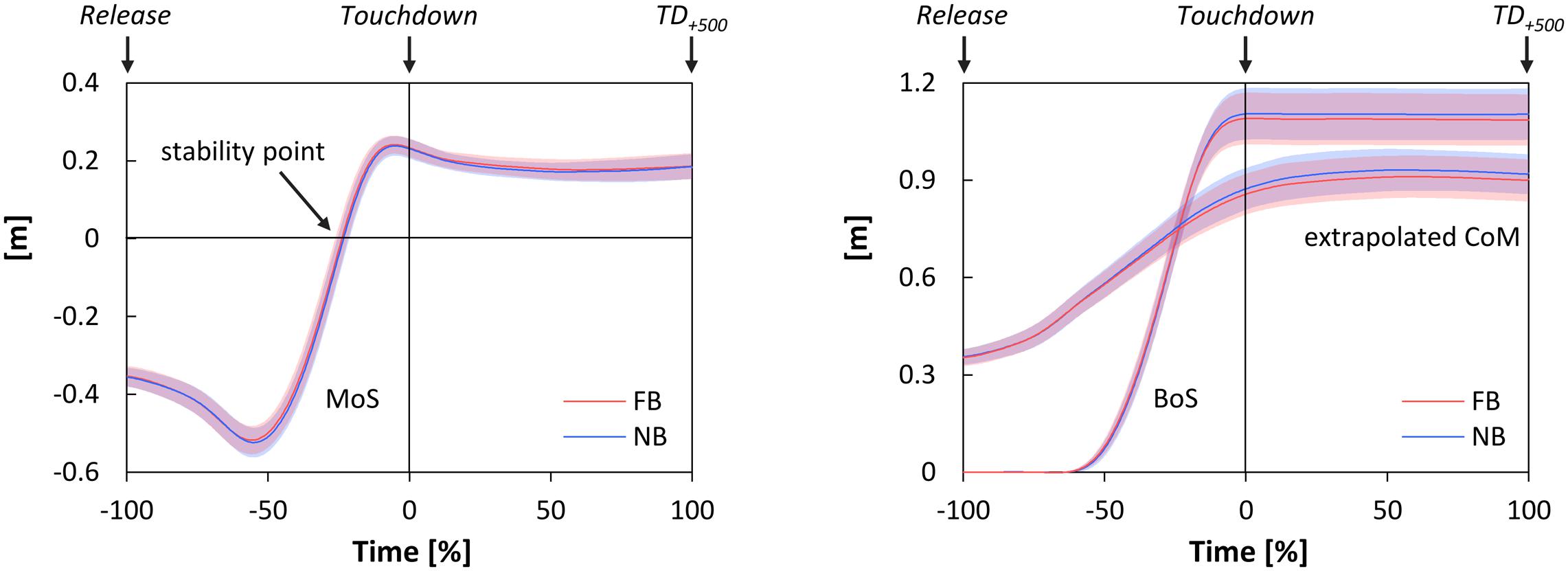
FIGURE 3. Time-normalized data of the margin of stability (MoS, left), the extrapolated center of mass (extrapolated CoM), and the anterior boundary of the base of support (BoS, both right) during Falling phase and Stance phase as functions of oral motor tasks (FB, force-controlled biting; NB, non-biting). Falling phase: from Release until Touchdown (normalized from –100 to 0%); Stance phase: from Touchdown until 500 ms after Touchdown (TD+500) (normalized from 0 to 100%); stability point: time point the MoS changes from negative to positive values. All data are presented as mean ± CI95.
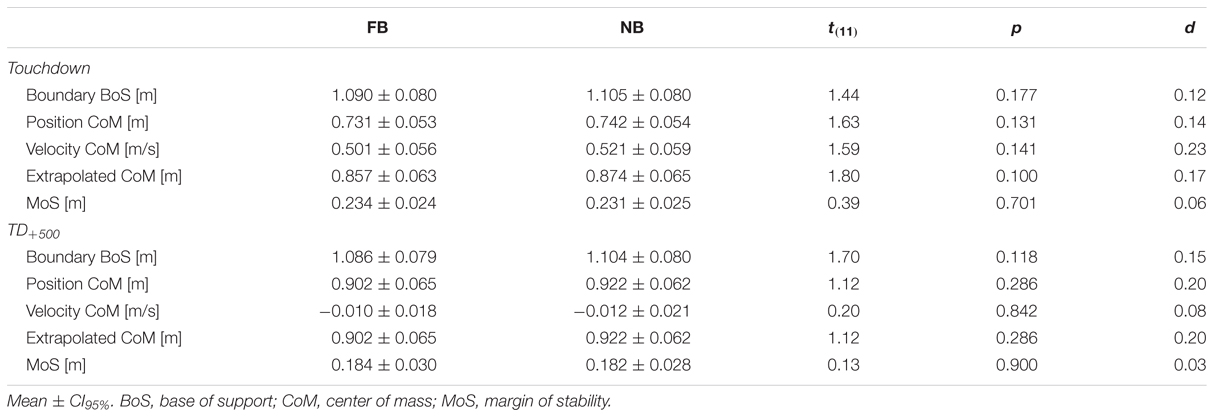
TABLE 3. Stability parameters at Touchdown and 500 ms after Touchdown (TD+500) for force-controlled biting (FB) and non-biting (NB).
Discussion
The aim of the present study was to examine the effects of submaximum jaw clenching on dynamic postural stability and joint kinematics during balance recovery after forward loss of balance. We hypothesized that force-controlled biting would lead to improved balance recovery in terms of increased dynamic stability and lower joint flexion angles of the knee and hip at touchdown and during the subsequent stance phase. The results, however, showed that biting at a submaximum force did not significantly influence recovery behavior of healthy young adults with regard to the variables under investigation.
Previous studies on the impact of concurrent jaw clenching activities observed significant improvements in peak force and rate of force development as compared to non-clenching controls (Forgione et al., 1991; Hiroshi, 2003; Ebben et al., 2008). Further, significant reductions in CoP displacements have been described under static conditions (Hellmann et al., 2011; Ringhof et al., 2015b). To the authors’ knowledge, this study was the first to examine the effects of oral motor activities on dynamic postural stability in general, and specifically on balance recovery from forward loss of balance. Nevertheless, the present data are very similar in magnitude to those of other studies on balance recovery (Karamanidis and Arampatzis, 2007; Karamanidis et al., 2008; Curtze et al., 2010). This forms the basis for the further discussion, enabling conclusive statements with regard to dynamic postural stability. In this context, the authors attempt to provide some explanations for the lack of observed differences; without any claim to be comprehensive.
First, the absence of any biting effects might be evoked by the different motor demands associated with static postural control compared with balance recovery after simulated forward falls. The former is primarily based on fine motor control relying on feedback mechanisms, and unconscious and highly automated processes (Horak, 2006). Contrastingly, the latter requires gross motor coordination, and huge demands on explosive muscle activation and force production (Karamanidis et al., 2008). Specifically, in the scenario of simulated forward falls, this process mainly follows feedforward control. Hence, the subjects can preselect their compensatory movements, which finally reduces the contribution of reflexes and automated processes. This distinction is of particular relevance, because most effects of jaw clenching activities were considered to be caused by facilitation of reflexes and motor system excitability (Miyahara et al., 1996; Boroojerdi et al., 2000; Takada et al., 2000). Modulation of somatosensory input, particularly for the neck muscles (Abrahams, 1977) during simulated forward falls might, thus, be not an issue.
In this context, one could speculate that more ecologically valid experiments, which are representative for the analysis of real falls, might have revealed differing results. As indicated above, in simulated forward falls, the subjects are well aware of the upcoming forward fall, which increases the proportion of voluntary movement control. In everyday life, however, the subjects are mostly unaware of such tripping or slipping events. The process of recovering stability, hence, mainly follows stereotypic movement patterns, initially provoked by stimulation of the muscle spindles in the calf muscles. Consequently, recovery is primary based on reflexes or automated compensatory movements, all requiring no or only little focused attention (Macpherson and Horak, 2013). Based on the findings on reflex facilitation (Miyahara et al., 1996; Takada et al., 2000) and concurrent activation potentiation (Ebben, 2006), we hypothesize that investigation on unexpected perturbations possibly would offer ergogenic effects for concurrent clenching activities, whereas the increase in voluntary movement control evoked by the chosen study design might have contributed to the absence of any significant alterations.
On the other hand, the lack of observed differences in dynamic stability could be the result of a floor effect, whereby the perturbation for the given sample was not difficult enough. Subjects were young healthy adults, and all obtained very high stability values (MoS > 0.2 at Touchdown). Potentially, subjects with diminished postural control and/or reduced force potential as, e.g., elderly could benefit from force-controlled biting. This investigation cannot resolve this question, however.
The final factor to be considered in the interpretation of the data is the bite force. At Release bite forces under both testing conditions were maintained at the intended level, confirming the compliance with the oral motor tasks. At Touchdown, however, significant increases in bite forces for both oral motor tasks were observed. In terms of NB, the results imply that an open-mouth, non-clenching condition is an unphysiological state which is not preferred during challenging situations. This is reinforced additionally by the fact that the subjects, even when already submaximum clenching their jaw, significantly increased their bite force from 146.01 ± 13.04 N to more than 200 N at Toe-Off. Clenching the jaw, hence, seems to be part of a common physiological repertoire used to improve the neural drive to distal body segments and, by this means, to enhance performance in many ways (Ebben, 2006; Ringhof et al., 2015a). This, in turn, would suggest that many studies focusing the ergogenic effects of jaw clenching actually did not observe performance improvements when the jaw was clenched, but rather a decrease in the non-clenching condition (Hiroshi, 2003; Ebben et al., 2008).
In conclusion, the present study has shown that submaximum clenching the jaw did not significantly affect balance recovery of healthy young adults in terms of dynamic postural stability and lower extremity joint kinematics after forward loss of balance. This is probably due to the different control strategies associated with static postural control and balance recovery after simulated forward falls. One must, therefore, question whether (i) the ergogenic effects of jaw clenching are limited only to static postural control, or (ii) the contradictory results might have been evoked by the methodological approach, the bite forces itself, or the study sample. Conclusive evidence is lacking, however. On the other hand, one might argue that reductions in CoP displacement – as they have been observed in response to jaw clenching (Hellmann et al., 2011; Ringhof et al., 2015b), and centric jaw relation (Gangloff et al., 2000; Bracco et al., 2004; Sakaguchi et al., 2007) – could degrade postural control performance. According to Haddad et al. (2013), postural variability in terms of increased CoP displacements is considered to aid in the exploration of the environment and to allow to experience the boundaries of stability (van Emmerik and van Wegen, 2002). However, this explanatory behavior might be valid only as long as postural sway does not cause a loss of balance, and rather may facilitate postural control during postural perturbation, when increased CoP displacement may make it easier to regain balance. For static postural control, this assumption, therefore, should be regarded critically; particularly, as this explanatory behavior increases the risk of losing balance by decreasing the MoS.
Future studies should contrast the effects of submaximum biting in different populations, under conduction of random perturbations, and as compared to both open mouth and habitual control conditions.
Author Contributions
The corresponding author confirms that all authors were fully involved in the study and preparation of the manuscript process. Each of the authors has read and approved the final version of the manuscript, and all authors agreed to the submission to Frontiers in Psychology.
Conflict of Interest Statement
The authors declare that the research was conducted in the absence of any commercial or financial relationships that could be construed as a potential conflict of interest.
Acknowledgments
The authors express their gratitude to Katrin Wöhlken for her help with processing of the data. Further, the authors acknowledge support by Deutsche Forschungsgemeinschaft grant HE 6961/1-1 and the Open Access Publishing Fund of Karlsruhe Institute of Technology.
References
Abrahams, V. C. (1977). The physiology of neck muscles; their role in head movement and maintenance of posture. Can. J. Physiol. Pharmacol. 55, 332–338. doi: 10.1139/y77-047
Arampatzis, A., Karamanidis, K., and Mademli, L. (2008). Deficits in the way to achieve balance related to mechanisms of dynamic stability control in the elderly. J. Biomech. 41, 1754–1761. doi: 10.1016/j.jbiomech.2008.02.022
Barrett, R. S., Cronin, N. J., Lichtwark, G. A., Mills, P. M., and Carty, C. P. (2012). Adaptive recovery responses to repeated forward loss of balance in older adults. J. Biomech. 45, 183–187. doi: 10.1016/j.jbiomech.2011.10.005
Blake, A. J., Morgan, K., Bendall, M. J., Dallosso, H., Ebrahim, S. B., Arie, T. H., et al. (1988). Falls by elderly people at home: prevalence and associated factors. Age Ageing 17, 365–372. doi: 10.1093/ageing/17.6.365
Boroojerdi, B., Battaglia, F., Muellbacher, W., and Cohen, L. G. (2000). Voluntary teeth clenching facilitates human motor system excitability. Clin. Neurophysiol. 111, 988–993. doi: 10.1016/S1388-2457(00)00279-0
Bracco, P., Deregibus, A., and Piscetta, R. (2004). Effects of different jaw relations on postural stability in human subjects. Neurosci. Lett. 356, 228–230. doi: 10.1016/j.neulet.2003.11.055
Buisseret-Delmas, C., Compoint, C., Delfini, C., and Buisseret, P. (1999). Organisation of reciprocal connections between trigeminal and vestibular nuclei in the rat. J. Comp. Neurol. 409, 153–168. doi: 10.1002/(SICI)1096-9861(19990621)409:1<153::AID-CNE11>3.0.CO;2-#
Carty, C. P., Cronin, N. J., Lichtwark, G. A., Mills, P. M., and Barrett, R. S. (2012). Mechanisms of adaptation from a multiple to a single step recovery strategy following repeated exposure to forward loss of balance in older adults. PLoS ONE 7:e33591. doi: 10.1371/journal.pone.0033591
Cheng, K. B., Huang, Y. -C., and Kuo, S. -Y. (2014). Effect of arm swing on single-step balance recovery. Hum. Mov. Sci. 38, 173–184. doi: 10.1016/j.humov.2014.08.011
Cohen, J. (1988). Statistical Power Analysis for the Behavioral Sciences. Hillsdale, NJ: Lawrence Erlbaum Associates.
Cuccia, A., and Caradonna, C. (2009). The relationship between the stomatognathic system and body posture. Clinics 64, 61–66. doi: 10.1590/S1807-59322009000100011
Curtze, C., Hof, A. L., Otten, B., and Postema, K. (2010). Balance recovery after an evoked forward fall in unilateral transtibial amputees. Gait Posture 32, 336–341. doi: 10.1016/j.gaitpost.2010.06.005
Davis, R. B., Õunpuu, S., Tyburski, D., and Gage, J. R. (1991). A gait analysis data collection and reduction technique. Hum. Mov. Sci. 10, 575–587. doi: 10.1016/0167-9457(91)90046-Z
Devoize, L., Doméjean, S., Melin, C., Raboisson, P., Artola, A., and Dallel, R. (2010). Organization of projections from the spinal trigeminal subnucleus oralis to the spinal cord in the rat: a neuroanatomical substrate for reciprocal orofacial-cervical interactions. Brain Res. 1343, 75–82. doi: 10.1016/j.brainres.2010.04.076
Do, M. C., Breniere, Y., and Brenguier, P. (1982). A biomechanical study of balance recovery during the fall forward. J. Biomech. 15, 933–939. doi: 10.1016/0021-9290(82)90011-2
Ebben, W. P. (2006). A brief review of concurrent activation potentiation: theoretical and practical constructs. J. Strength Cond. Res. 20, 985–991. doi: 10.1519/R-19375.1
Ebben, W. P., Flanagan, E. P., and Jensen, R. L. (2008). Jaw clenching results in concurrent activation potentiation during the countermovement jump. J. Strength Cond. Res. 22, 1850–1854. doi: 10.1519/JSC.0b013e3181875117
Ferrario, V. F., Sforza, C., Schmitz, J. H., and Taroni, A. (1996). Occlusion and center of foot pressure variation: is there a relationship? J. Prosthet. Dent. 76, 302–308. doi: 10.1016/S0022-3913(96)90176-6
Forgione, A. G., Mehta, N. R., and Westcott, W. L. (1991). Strength and bite, Part 1: an analytical review. Cranio 9, 305–315.
Gangloff, P., Louis, J. P., and Perrin, P. P. (2000). Dental occlusion modifies gaze and posture stabilization in human subjects. Neurosci. Lett. 293, 203–206. doi: 10.1016/S0304-3940(00)01528-7
Graham, D. F., Carty, C. P., Lloyd, D. G., Lichtwark, G. A., and Barrett, R. S. (2014). Muscle contributions to recovery from forward loss of balance by stepping. J. Biomech. 47, 667–674. doi: 10.1016/j.jbiomech.2013.11.047
Haddad, J. M., Rietdyk, S., Claxton, L. J., and Huber, J. (2013). Task-dependent postural control throughout the lifespan. Exerc. Sport Sci. Rev. 41, 123–132. doi: 10.1097/JES.0b013e3182877cc8
Hellmann, D., Giannakopoulos, N. N., Blaser, R., Eberhard, L., and Schindler, H. J. (2011). The effect of various jaw motor tasks on body sway. J. Oral Rehabil. 38, 729–736. doi: 10.1111/j.1365-2842.2011.02211.x
Hellmann, D., Stein, T., Potthast, W., Rammelsberg, P., Schindler, H. J., and Ringhof, S. (2015). The effect of force-controlled biting on human posture control. Hum. Mov. Sci. 43, 125–137. doi: 10.1016/j.humov.2015.08.009
Hiroshi, C. (2003). Relation between teeth clenching and grip force production characteristics. Kokubyo Gakkai Zasshi 70, 82–88. doi: 10.5357/koubyou.70.82
Hof, A. L. (2008). The ‘extrapolated center of mass’ concept suggests a simple control of balance in walking. Hum. Mov. Sci. 27, 112–125. doi: 10.1016/j.humov.2007.08.003
Hof, A. L., Gazendam, M G J., and Sinke, W. E. (2005). The condition for dynamic stability. J. Biomech. 38, 1–8. doi: 10.1016/j.jbiomech.2004.03.025
Horak, F. B. (2006). Postural orientation and equilibrium: what do we need to know about neural control of balance to prevent falls? Age Ageing 35(Suppl. 2), ii7–ii11. doi: 10.1093/ageing/afl077
Horak, F. B. and Macpherson, J. M. (1996). “Postural orientation and equilibrium,” in Handbook of Physiology. Exercise: Regulation and Integration of Multiple Systems, eds L. B. Rowell and J. T. Shepherd (New York, NY: Oxford University Press), 255–292.
Kadaba, M. P., Ramakrishnan, H. K., and Wootten, M. E. (1990). Measurement of lower extremity kinematics during level walking. J. Orthop. Res. 8, 383–392. doi: 10.1002/jor.1100080310
Karamanidis, K., and Arampatzis, A. (2007). Age-related degeneration in leg-extensor muscle-tendon units decreases recovery performance after a forward fall: compensation with running experience. Eur. J. Appl. Physiol. 99, 73–85. doi: 10.1007/s00421-006-0318-2
Karamanidis, K., Arampatzis, A., and Mademli, L. (2008). Age-related deficit in dynamic stability control after forward falls is affected by muscle strength and tendon stiffness. J. Electromyogr. Kinesiol. 18, 980–989. doi: 10.1016/j.jelekin.2007.04.003
Keshvad, A., and Winstanley, R. B. (2000). An appraisal of the literature on centric relation. Part I. J. Oral Rehabil. 27, 823–833. doi: 10.1046/j.1365-2842.2000.00653.x
Macpherson, J. M., and Horak, F. B. (2013). “Posture,” in Principles of Neural Science, eds E. R. Kandel, J. H. Schwartz, T. M. Jessell, S. A. Siegelbaum, and A. J. Hudspeth (New York, NY: McGraw-Hill), 935–959.
Manfredini, D., Castroflorio, T., Perinetti, G., and Guarda-Nardini, L. (2012). Dental occlusion, body posture and temporomandibular disorders: where we are now and where we are heading for. J. Oral Rehabil. 39, 463–471. doi: 10.1111/j.1365-2842.2012.02291.x
Michelotti, A., Buonocore, G., Manzo, P., Pellegrino, G., and Farella, M. (2011). Dental occlusion and posture: an overview. Prog. Orthod. 12, 53–58. doi: 10.1016/j.pio.2010.09.010
Miyahara, T., Hagiya, N., Ohyama, T., and Nakamura, Y. (1996). Modulation of human soleus H reflex in association with voluntary clenching of the teeth. J. Neurophysiol. 76, 2033–2041.
Perinetti, G. (2006). Dental occlusion and body posture: no detectable correlation. Gait Posture 24, 165–168. doi: 10.1016/j.gaitpost.2005.07.012
Perinetti, G. (2007). Temporomandibular disorders do not correlate with detectable alterations in body posture. J. Contemp. Dent. Pract. 8, 60–67.
Richardson, J. T. (2011). Eta squared and partial eta squared as measures of effect size in educational research. Educ. Res. Rev. 6, 135–147. doi: 10.1016/j.edurev.2010.12.001
Ringhof, S., Hellmann, D., Meier, F., Etz, E., Schindler, H. J., and Stein, T. (2015a). The effect of oral motor activity on the athletic performance of professional golfers. Front. Psychol. 6:750. doi: 10.3389/fpsyg.2015.00750
Ringhof, S., Stein, T., Potthast, W., Schindler, H. -J., and Hellmann, D. (2015b). Force-controlled biting alters postural control in bipedal and unipedal stance. J. Oral Rehabil. 42, 173–184. doi: 10.1111/joor.12247
Rubenstein, L. Z. (2006). Falls in older people: epidemiology, risk factors and strategies for prevention. Age Ageing 35(Suppl. 2) ii37-ii41. doi: 10.1093/ageing/afl084
Ruggiero, D. A., Ross, C. A., and Reis, D. J. (1981). Projections from the spinal trigeminal nucleus to the entire length of the spinal cord in the rat. Brain Res. 225, 225–233. doi: 10.1016/0006-8993(81)90832-5
Sakaguchi, K., Mehta, N. R., Abdallah, E. F., Forgione, A. G., Hirayama, H., Kawasaki, T., et al. (2007). Examination of the relationship between mandibular position and body posture. Cranio 25, 237–249. doi: 10.1179/crn.2007.037
Takada, Y., Miyahara, T., Tanaka, T., Ohyama, T., and Nakamura, Y. (2000). Modulation of H reflex of pretibial muscles and reciprocal Ia inhibition of soleus muscle during voluntary teeth clenching in humans. J. Neurophysiol. 83, 2063–2070.
van Emmerik, R. E., and van Wegen, E. E. (2002). On the functional aspects of variability in postural control. Exerc. Sport Sci. Rev. 30, 177–183. doi: 10.1097/00003677-200210000-00007
Wojcik, L. A., Thelen, D. G., Schultz, A. B., Ashton-Miller, J. A., and Alexander, N. B. (2001). Age and gender differences in peak lower extremity joint torques and ranges of motion used during single-step balance recovery from a forward fall. J. Biomech. 34, 67–73. doi: 10.1016/S0021-9290(00)00152-4
Keywords: postural control, balance recovery, dynamic stability, joint kinematics, jaw clenching, craniomandibular system, biting
Citation: Ringhof S, Stein T, Hellmann D, Schindler HJ and Potthast W (2016) Effect of Jaw Clenching on Balance Recovery: Dynamic Stability and Lower Extremity Joint Kinematics after Forward Loss of Balance. Front. Psychol. 7:291. doi: 10.3389/fpsyg.2016.00291
Received: 23 November 2015; Accepted: 15 February 2016;
Published: 11 March 2016.
Edited by:
Pietro Avanzini, University of Parma, ItalyReviewed by:
Christopher K. Rhea, University of North Carolina – Greensboro, USAMaury A. Nussbaum, Virginia Polytechnic Institute and State University, USA
Copyright © 2016 Ringhof, Stein, Hellmann, Schindler and Potthast. This is an open-access article distributed under the terms of the Creative Commons Attribution License (CC BY). The use, distribution or reproduction in other forums is permitted, provided the original author(s) or licensor are credited and that the original publication in this journal is cited, in accordance with accepted academic practice. No use, distribution or reproduction is permitted which does not comply with these terms.
*Correspondence: Steffen Ringhof, c3RlZmZlbi5yaW5naG9mQGtpdC5lZHU=