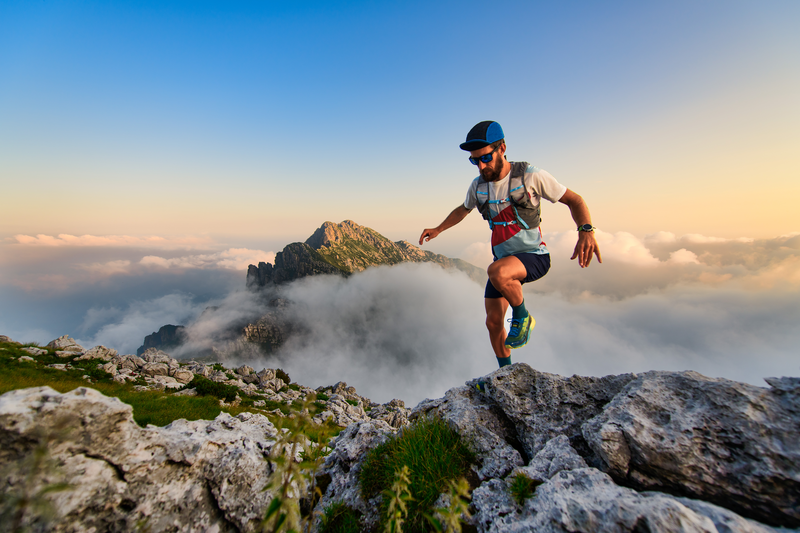
94% of researchers rate our articles as excellent or good
Learn more about the work of our research integrity team to safeguard the quality of each article we publish.
Find out more
REVIEW article
Front. Psychol. , 12 May 2015
Sec. Cognitive Science
Volume 6 - 2015 | https://doi.org/10.3389/fpsyg.2015.00622
The last decade has witnessed a spurt of new publications documenting sleep's essential contribution to the brains ability to form lasting memories. For the declarative memory domain, slow wave sleep (the deepest sleep stage) has the greatest beneficial effect on the consolidation of memories acquired during preceding wakefulness. The finding that newly encoded memories become reactivated during subsequent sleep fostered the idea that reactivation leads to the strengthening and transformation of the memory trace. According to the active system consolidation account, trace reactivation leads to the redistribution of the transient memory representations from the hippocampus to the long-lasting knowledge networks of the cortex. Apart from consolidating previously learned information, sleep also facilitates the encoding of new memories after sleep, which probably relies on the renormalization of synaptic weights during sleep as suggested by the synaptic homeostasis theory. During wakefulness overshooting potentiation causes an imbalance in synaptic weights that is countered by synaptic downscaling during subsequent sleep. This review briefly introduces the basic concepts and central findings of the research on sleep and memory, and discusses implications of this lab-based work for everyday applications to make the best possible use of sleep's beneficial effect on learning and memory.
Our modern society has formed an ill-advised but strong belief that sleep is an annoying habit that should be kept at a strict minimum to enhance productivity. However, recent research indicates that sleep's function goes beyond rest and replenishment (in itself a good reason to sleep) and constitutes a state of active offline information processing essential to the appropriate functioning of learning and memory. Memory is established in three stages; new memories are initially acquired (encoding), become strengthened and reorganized (consolidation), and are finally recalled (retrieval; Figure 1 upper panel). In 1924, Jenkins and Dallenbach expanded on Ebbinghaus (1885) studies of forgetting curves and reported that sleep after encoding of nonsense syllables supports memory consolidation, inasmuch as it reduces forgetting compared to an interval containing only wakefulness (Jenkins and Dallenbach, 1924). This line of research was revisited several times during the last century (Ekstrand, 1967; Yaroush et al., 1971; Barrett and Ekstrand, 1972; Fowler et al., 1973; Benson and Feinberg, 1975; Ekstrand et al., 1977) and progress was aided substantially by the identification of sleep stages with distinct psychophysiological properties (Figure 2; Aserinsky and Kleitman, 1953; Dement and Kleitman, 1957). During the last two decades, interest in the topic has re-emerged and we have witnessed an upsurge of publications dissecting sleep's role for memory (for a comprehensive review see Rasch and Born, 2013). One of the most widely studied models of sleep's role for memory consolidation, according to the active system consolidation theory, is that of an active neuronal replay of memory representations during slow wave sleep, strengthening memory traces encoded during preceding wakefulness (Figure 1 upper panel; Diekelmann and Born, 2010; Oudiette and Paller, 2013). This theory has recently received support by findings showing that sleep following learning increases synaptic spines specifically associated with prior learning experience (Euston and Steenland, 2014; Yang et al., 2014). Apart from the consolidating effect, sleep has an additional function in the restoration of learning capabilities (Van Der Werf et al., 2009; Antonenko et al., 2013), which may also be linked to the downscaling of synapses that were potentiated during prior wakefulness (Tononi and Cirelli, 2003, 2006, 2014). Of note, this review does not touch on the subject of learning during sleep, which to the best of our knowledge has not been shown in the declarative memory domain. Empirical work suggests that encoding during sleep in humans, if at all, is only possible for conditioning (Ikeda and Morotomi, 1996; Arzi et al., 2012, 2014; Cox et al., 2014).
Figure 1. Schematic of the proposed memory processes. Upper panel: Memory processes can be divided into three steps (Encoding, Consolidation, and Retrieval). Encoding is the process of information uptake during learning (in this case of card pair locations of a concentration game) and is most effective during wakefulness, while retrieval is the process of recalling the memorized content at a later time point. Consolidation takes place after encoding and is necessary to transform the initially labile traces into enduring representations. During sleep after learning, sleep-dependent memory consolidation strengthens the acquired memory traces by reactivating (“replaying”) them. Lower panel: During wake encoding, information is taken up by the sense organs and flows to the hippocampus via the association cortices. In the hippocampus a trace is formed fast but only for temporary storage (thick lines in the hippocampus represent the hippocampal trace, dashed lines in the cortex represent the corresponding cortical trace). During slow wave sleep the traces in the hippocampus are reactivated leading to their integration into the long-term store of the cortex (active system consolidation), where they are strengthened through synaptic consolidation (the consolidated trace is represented by thick lines in the cortex). Additionally, processes of synaptic downscaling during sleep (shown as the hippocampal trace vanishing) lead to the preparation of the brain to encode new information during the next wake phase (again depicted by thick and dashed lines in hippocampus and cortex, respectively). While the synaptic downscaling hypothesis has been most rigorously applied to cortical learning tasks (Tononi and Cirelli, 2014), recent research has provided evidence for downscaling in the hippocampus during REM sleep (Grosmark et al., 2012). Processes of local synaptic strengthening may work in concert with global downscaling processes to provide reliable and efficient memory traces (Born and Feld, 2012). Bottom panel is adapted from Diekelmann and Born (2010).
Figure 2. Overview over sleep stages. (A) Sleep, defined as a readily reversible state of reduced responsiveness to the environment, is a phenomenon reported in all animals—from humans to flies and mollusks—that have been systematically examined so far (Borbély and Achermann, 2000; Huber et al., 2004b; Cirelli and Tononi, 2008; Vorster et al., 2014). Human sleep is traditionally dichotomized into rapid-eye-movement (REM) sleep and non-rapid-eye-movement (NonREM) sleep and prototypically consists of 90 min cycles, during which NonREM sleep and REM sleep alternate. REM sleep (green) is characterized by rapid eye movements, a mixed frequency EEG and an inhibition of muscle tone. NonREM sleep can be further subdivided into sleep stages 1–4 (S1–S4), which resemble the depth of sleep, inasmuch as responsiveness to external stimuli is minimal in sleep stage 4. S1 is a transitory stage that only makes up a small amount of a night's sleep (<10%). S2 is determined by the occurrence of sleep spindles (waxing and waning activity of 10–15 Hz) and K-complexes (a sharp negative high-amplitude deflexion followed by a slower positive wave). S3 and S4 combine to slow wave sleep (SWS, blue) that is characterized by large amounts (>20%) of slow wave activity (0.5–4 Hz) in the EEG. (B) In rats, place cells express a specific firing pattern dependent on the rat's location within its environment. When running through a maze (Run) this leads to a sequential firing pattern that represents the sequence of locations the rat passes in the maze (each row represent one cell, the upper panel shows spikes across time during one lap, the lower panel shows averaged activity). During slow wave sleep (Sleep) after training on an alternation task in the maze the firing sequence is replayed in a time-compressed manner (adapted from Ji and Wilson, 2007). This and other similar experiments are evidence that representations encoded during wakefulness are replayed during subsequent sleep. (C) Recent evidence suggests that the hallmark oscillation of SWS, the sleep slow oscillation (<1 Hz), which is generated in cortical areas, coordinates replay in the hippocampus (accompanied by sharp wave/ripple activity generated in the hippocampus) and plasticity-promoting spindle activity (generated in the thalamus). The coordination of these oscillations enables the gradual transfer of the transient memory representations from the hippocampus to their long-term storage sites within the cortex. The upper trace depicts the unfiltered surface EEG and below, the surface EEG filtered in the slow oscillation and the spindle band are shown (spindles typically occur in the down-to-up transition of the slow oscillation). The inset shows sharp-wave/ripple activity, which is typically nested in the troughs of spindles.
While we are only starting to understand the neuronal mechanisms that underlie these processes, the following sections give a brief overview of some of the most persuasive findings in an attempt to translate them into behavioral guidelines for optimizing memory function by sleep. Importantly, rather than giving a state of the art overview of all findings and developments in the rapidly expanding field of sleep and memory (for recent comprehensive reviews see, Diekelmann and Born, 2010; Lewis and Durrant, 2011; Saletin and Walker, 2012; Abel et al., 2013; Inostroza and Born, 2013; Oudiette and Paller, 2013; Prince and Abel, 2013; Rasch and Born, 2013; Stickgold and Walker, 2013), this review focuses on the most established findings to aid their adaptation into education, therapeutic practice and performance improvement in the elderly. Additionally, we hope to encourage an increase in applied research of sleep and memory, which is still in its beginnings.
The theory of active system consolidation can explain how sleep is able to strengthen, transform and redistribute the information that was encoded during prior wakefulness (Figure 1 lower panel; Diekelmann and Born, 2010; Rasch and Born, 2013). During wakefulness new information is encoded in parallel into the hippocampus, which serves as a fast-learning temporary store, and into distributed cortical areas that represent the slow-learning long-term store. Because the cortex takes longer to form direct connections between single elements of a memory, the hippocampus initially acts as a hub to bind the cortical representations (Winocur et al., 2010; Battaglia et al., 2011). During sleep, the new memory representations become re-activated in the hippocampus as well as in the cortex. The hippocampus thereby serves as a trainer for the cortical long-term store by reactivating the cortical representations repeatedly so that the cortical connections become strengthened and eventually become independent of the hippocampus.
Such a neuronal “replay” of learning-related activity during sleep was first observed in rats. After a spatial learning experience that activated specific place cells in the hippocampus, those cells that had fired together during learning displayed correlated firing again during subsequent sleep (Wilson and McNaughton, 1994). Importantly, replay in the hippocampus follows the same sequence as during wakefulness and is also coordinated with replay in the cortex (Figure 2B; Skaggs and McNaughton, 1996; Ji and Wilson, 2007). Other brain regions show replay of learning-related neuronal activity during sleep as well, such as motor cortex, striatum, and thalamus (Ribeiro et al., 2004; Lansink et al., 2008, 2009; Gulati et al., 2014; Harris, 2014). Signs of reactivation were also found in humans using brain imaging techniques such as functional magnetic resonance imaging (fMRI). In these studies, participants learned tasks that activated specific brain regions and similar activation patterns were re-expressed during subsequent sleep (Maquet et al., 2000; Peigneux et al., 2004). Memory reactivations are accompanied by high-frequency oscillatory events (ripples, 100–200 Hz) in the local field potential of the hippocampus that together with sharp-waves form sharp-wave/ripple complexes (Figure 2C, Buzsaki, 1986, 1989; Nadasdy et al., 1999). The sleep slow oscillations (large amplitude waves <1 Hz in the EEG) that dominate slow wave sleep are generated in the cortex and synchronize these memory reactivations in the hippocampus (Sirota et al., 2003; Buzsaki and Draguhn, 2004; Molle and Born, 2011). This synchronization effects that the reactivated memory information reaches the cortex together with sleep spindles (10–15 Hz oscillations, Mölle et al., 2011; Bergmann et al., 2012). The plasticity enhancing effect of spindles in the cortex facilitates the integration of the new memories into the long term store (Ribeiro et al., 2007) benefiting the recall of these memories at a later point. These oscillations and the associated memory reactivations are partly dependent on the specific constellation of hormones and neurotransmitters during sleep, such as the low cholinergic tone during slow wave sleep (Hasselmo, 1999; Gais and Born, 2004), which allows information to flow from the hippocampus to the neocortex. While there are also accounts of memory reactivation during wakefulness and REM sleep, it seems to be the specific combination of the oscillatory and neuromodulatory environment found during slow wave sleep that enables consolidation through reactivation in the hippocampus and cortex (Diekelmann and Born, 2010).
Beyond the strengthening of memories, sleep also reorganizes and transforms memories. This reorganization of memory can facilitate generalization and the abstraction of the gist, i.e., invariant features or rules, from encoded information (Gomez et al., 2006; Durrant et al., 2011; Lewis and Durrant, 2011; Inostroza and Born, 2013). Sleep can even help to gain insight and find new solutions to problems. Wagner et al. (2004) used a number reduction task that afforded participants to perform a series of numerical transformations. Unbeknown to the participants there was a hidden rule in the task. As soon as they discovered this rule they could use a short cut to solve the task. Almost three times more participants in the sleep condition gained insight into this hidden rule compared to the wake condition. Further, evidence for a reorganization of memory during sleep comes from studies in which false memories were provoked by asking participants to learn lists of words that all pertained to an associated word (the gist word) that was not on the list. More gist words were falsely assigned as true memories by those participants that slept during the retention interval compared to those who remained awake (Payne et al., 2009; Diekelmann et al., 2010), suggesting that sleep helped extracting the general meaning of the word lists.
Next to the idea of active system consolidation, the most influential theory of sleep's function for memory is the synaptic homeostasis hypothesis (Tononi and Cirelli, 2003, 2006, 2014). According to this hypothesis, the widespread synaptic potentiation occurring at encoding of information during wakefulness leads to increased demands of space and energy in the brain. If this development remained unchecked the brain would shortly reach the limits of its encoding and/or upkeep capabilities. Sleep is essential to renormalize synaptic weights (termed “synaptic downscaling”) and has therefore been suggested to be “the price we pay for plasticity” (Cirelli and Tononi, 2008; Tononi and Cirelli, 2014). Indeed, overall synaptic spines as well as markers for synaptic potentiation increase across periods of wakefulness and decrease during periods of sleep (Vyazovskiy et al., 2008; Maret et al., 2011). Computer simulations have established the theoretical effectiveness of slow wave activity (0.5–4 Hz) to renormalize synapses (Hill and Tononi, 2005; Esser et al., 2007; Sullivan and de Sa, 2008), and potentiating synapses by extended learning (Huber et al., 2004a; Schmidt et al., 2006) or by transcranial magnetic stimulation (Huber et al., 2007, 2008) induces local increases of slow wave activity. Conversely, immobilizing a human's arm reduces slow wave activity (Huber et al., 2006) and slow wave sleep deprivation impairs visuo-motor learning (Hill et al., 2008; Aeschbach, 2009; Landsness et al., 2009). In correspondence with the general finding that slow wave activity is homeostatically regulated (Borbély and Achermann, 2000) this line of research indicates that slow wave activity may play a major role for synaptic homeostasis (Massimini et al., 2009; Vassalli and Dijk, 2009). However, it has also been shown that learning increases sleep spindles (Gais et al., 2002), and since the synaptic homeostasis theory makes no explicit claims as to the specific mechanisms involved (Tononi and Cirelli, 2012), their exact nature as well as their association with specific sleep stages remain to be determined (Born and Feld, 2012; Chauvette et al., 2012; Grosmark et al., 2012).
A direct consequence of synaptic downscaling during sleep can be seen in the improvement of learning after a period containing sleep compared to a period containing only wakefulness (McDermott et al., 2003). In a nap study, Mander and colleagues tested their participants' ability to learn new information twice in 1 day, once at noon and a second time in the evening. One group of subjects was allowed to take a nap in between the two sessions and another group stayed awake. As expected, encoding ability deteriorated across the day in the wake subjects, while subjects who took a nap even slightly improved encoding performance at the second session (Mander et al., 2011). Impairments in encoding ability were also observed in participants who were sleep-deprived for one night, with these impairments being associated with reduced hippocampal activation (Yoo et al., 2007). In line with the suggestion of the synaptic homeostasis hypothesis that slow waves might be responsible for synaptic downscaling, it was shown that suppressing slow wave sleep in elderly participants reduced their ability to encode new information the next morning (Van Der Werf et al., 2009).
Although a considerable number of findings suggest that sleep plays a pivotal role for the normal functioning of human memory, research on the application of these effects of sleep on memory is still at its beginning. The translation of the basic science findings into real world contexts can not only help improve our learning and memory capacities in everyday life but is also a promising avenue for possible applications in aging, clinical settings, and education. Based on the available evidence, the following sections offer potential strategies of optimizing sleep's beneficial influence on memory in applied contexts.
The timing of sleep has been shown to influence sleep's effect on memory, inasmuch as a shorter interval between sleep and learning of declarative tasks enhances the effect of sleep on memory (Gais et al., 2006; Talamini et al., 2008; Payne et al., 2012b). Comparing retention intervals that differed with respect to the timing of sleep, Gais et al. (2006) found that sleep that follows within 3 h after encoding is more beneficial to the consolidation of English-German vocabulary than sleep following after 10 h. Thus, to make the best use of sleep's memory improving effect, sleep should follow encoding within a few hours or, alternatively, the contents that were learned during the day could be rehearsed shortly before bedtime. With regard to the amount of sleep that is necessary to boost memory, several studies have shown that declarative memory even benefits from rather short periods of sleep. 3-h episodes of sleep during the first half of the night are sufficient to prompt the beneficial effect of sleep on declarative memory (Yaroush et al., 1971; Barrett and Ekstrand, 1972; Fowler et al., 1973; Plihal and Born, 1997; Tucker and Fishbein, 2009) and even naps can be used to improve memory, albeit, in a more dose-dependent manner (Lahl et al., 2008). Although some studies found memory improvements of very short naps of only a few minutes (Lahl et al., 2008), longer periods of 60–90 min of sleep provide better outcomes (Diekelmann et al., 2012). This offers the opportunity to time short naps after intensive periods of learning to benefit consolidation and subsequent learning. However, even though short amounts of sleep can help the initial consolidation of new memories, naps cannot replace a good night's sleep but may rather be introduced in addition to nocturnal sleep. Some types of memories even require a whole night of sleep, including the cyclic occurrence of different sleep stages, to become optimally consolidated (Diekelmann and Born, 2010).
Memory consolidation during sleep is selective in preferentially facilitating those memories that are in some way relevant for the future. Wilhelm et al. (2011) showed that merely the information that memory contents will have to be recalled on the next day can decide its access to sleep-dependent consolidation. In this study participants learned a declarative word pair association task in the evening. After encoding, only one of two groups was informed that they would have to retrieve the word pairs the next morning. While the informed group showed the expected improvement in retention performance after sleep, the uninformed group did not and was on par with an informed group that did not sleep during the retention interval. This effect is confirmed by sleep's enhancement of prospective memories of tasks to be performed in the future (Scullin and McDaniel, 2010; Diekelmann et al., 2013a,b). Similar findings were observed when subjects anticipated a monetary reward for performing well in a procedural finger sequence tapping task (Fischer and Born, 2009). Emotionality of the learning content is a further feature that signals relevance for the individual and leads to stronger effects of sleep-dependent memory consolidation (Wagner et al., 2001; Sterpenich et al., 2007, 2009; Payne et al., 2008, 2012a; Groch et al., 2013, 2014). However, there seem to be conflicting mechanisms of REM sleep for consolidating emotional content and SWS for declarative content in some tasks (Payne et al., 2008; Groch et al., 2014). To optimize the beneficial effect of sleep on memory, the relevance of the learning material should be very evident to the learner and might be increased by additional incentives.
Sleep also benefits the learning of declarative tasks such as word pair associations and object locations in children (Wilhelm et al., 2008). A recent study in preschoolers shows that learning new words from storybooks that are read to them is increased in children that are allowed to nap after hearing the story (Williams and Horst, 2014). Even infants of 6–16 months learn new word meanings or novel actions better if they nap during the retention interval (Friedrich et al., 2015; Seehagen et al., 2015). For the extraction of explicit knowledge, sleep might even be more beneficial in children than in adults. Wilhelm and colleagues trained adults and 8–11-year-old children to press a specific sequence of buttons on a button-box (Wilhelm et al., 2013). None of the participants were aware of the underlying sequence of button presses during training before sleep. While both children and adults gained more explicit awareness of the sequence after sleep compared to a wake interval, children clearly outperformed the adults after sleep with almost all children being able to explicitly generate the entire 8-element sequence. Interestingly, this beneficial effect of sleep on knowledge may even extend into highly demanding education such as medical school (Ahrberg et al., 2012; Genzel et al., 2013). In a questionnaire study, sleep behavior (especially during the pre-exam period), together with the results in the pre-clinical board exam, turned out as the strongest predictor for the final grade in the medical exam (Genzel et al., 2013). Improving sleep in children and students might therefore be a promising target to improve school performance (Ribeiro and Stickgold, 2014).
Older adults typically show a dramatic reduction in the amount of slow wave sleep, with this reduction being associated with reduced sleep-dependent memory consolidation (Backhaus et al., 2007). The importance of slow wave sleep for memory consolidation in the elderly was shown in a cross-sectional study (Mander et al., 2013b). In this study young and elderly adults learned a declarative word pair task before going to sleep. Overnight retention was predicted by slow wave activity, which in turn was associated with prefrontal gray matter volume. The authors argue that the age-related reduction in gray matter volume causes the decline of slow wave generation in the brain, which in turn compromises sleep's ability to consolidate memories. In another study from the same group, it was shown that the reduction in sleep spindles observed in the elderly is predictive of impairments of new learning (of an episodic task) and hippocampal activation after sleep (Mander et al., 2013a). Importantly, in these studies it remains unclear if sleep is causal to the observed impairments. Clarifying this question will be essential to identify therapeutic targets for improving sleep and memory in the elderly.
Many memory-related psychiatric disorders, such as post-traumatic stress disorder (PTSD), are associated with altered sleep profiles (Germain, 2013; Steiger et al., 2013), suggesting that sleep might play a role in the etiology of these disorders. Considering that behavioral therapy is effective in many anxiety disorders and relies on learning mechanisms, its success may be further improved by using sleep treatments. Particularly in phobias diminished extinction of conditioned fear responses is often a problem. Sleep benefits the generalization of the extinction of conditioned fear (Pace-Schott et al., 2009) and this finding has been applied to simulated exposure therapy showing that sleep can be used to consolidate and generalize extinction of spider fear responses (Pace-Schott et al., 2012). Recently, Kleim et al. (2013) were able to show that napping for 90 min after an exposure therapy session can reduce self-reported fear and negative cognition at a 1-week follow-up assessment in patients suffering from spider phobia. Hence, applying sleep as a non-invasive method might prove to be a highly useful and inexpensive tool to boost the efficiency of psychotherapy.
Apart from the knowledge about how to make best use of natural sleep for memory functions, recent research approaches have also produced a number of insights of how to enhance memory processing during sleep beyond its natural boundaries. Although there are still certain methodological and ethical restraints (for a topic specific review see, Diekelmann, 2014), memory enhancement during sleep might prove worthwhile in daily contexts as well as in clinical applications.
Recent research suggests that memory replay can be biased by external cues presented during sleep that had been present during prior encoding (Bendor and Wilson, 2012). In their seminal paper, Rasch et al. (2007) used odor cues to trigger memory reactivation processes during sleep in humans. Participants were asked to encode locations of picture pairs shown on a computer screen (similar to the game concentration, Figure 1 upper panel) while they smelled the scent of roses. During subsequent slow wave sleep participants were re-exposed to this odor or an odorless vehicle. Those participants who had smelled the odor during learning and during slow wave sleep showed increased recall of the card locations at retrieval compared to the vehicle condition. Memory was not improved if participants received the odor only during sleep but not during prior encoding, and odor re-exposure was also not effective during wakefulness and post-learning REM sleep. Since this report, the paradigm has been extended to show that specific auditory cues associated with picture-locations (e.g. the sound “meow” associated with the picture of a cat at a specific location), likewise foster memory reactivation if presented during slow wave sleep, increasing subsequent recall performance (Rudoy et al., 2009; van Dongen et al., 2012). Additionally, it was shown that auditory cues during sleep can enhance the retention of non-declarative tasks by using a melody for cueing a sequence of button presses in a motor skill task (Antony et al., 2012). Interestingly, this effect is highly specific, inasmuch as participants only perform better on that part of the sequence that has been cued during sleep, while performance on the un-cued part is unchanged (Schonauer et al., 2014). Finally, it was demonstrated that the strengthening effect of reactivation cues for memory is specific for the sleep state and that presentation of the same cues during wakefulness can even labilize and disturb the memory trace (Diekelmann et al., 2011). Both odor cues and tones are stimuli that are easy to apply in the home environment to improve individual memory capacities. For example, students might consider using fragrance lights or aromatic lamps during learning and subsequent sleep, or re-play previously learned spoken vocabulary during sleep. Care should be taken, however, to make sure that these stimuli do not disturb sleep per se.
It has now been attempted to translate the memory cueing paradigm to fear conditioning tasks, which opens the possibility of enhancing efficiency of exposure therapy. In one of these studies participants who had undergone olfactory contextual fear conditioning were re-exposed to the olfactory context cue during subsequent slow wave sleep. Compared to the control condition without odor during sleep, these participants showed greater extinction of contextual fear in the next morning (Hauner et al., 2013). Likewise, subjects who were fear conditioned on a neutral tone and were re-presented this tone during slow wave sleep, showed reduced fear responses to the tone after sleep (He et al., 2014). Two similar studies in rodents, however, showed a greater fear response after re-exposure of the conditioned stimulus during sleep (Rolls et al., 2013; Barnes and Wilson, 2014). Although much more research is needed to clarify these controversial findings and to identify possible boundary conditions and adverse effects (for an opinion on the contradictory nature of these results see, Diekelmann and Born, 2015), this method bears great potential for applications in clinical contexts, e.g., for the treatment of anxiety disorders.
Slow oscillations are the brain oscillations that have been most closely linked to sleep-dependent memory processing. Intensifying these slow oscillations with electrical or auditory stimulation has been shown to enhance learning and memory consolidation (Marshall et al., 2006; Ngo et al., 2013b). In the ground-breaking study by Marshall and colleagues, participants learned a word pair association task in the evening. During subsequent sleep they were subjected to trans-cranial electric stimulation (TES) at the same frequency as natural slow oscillations (~0.75 Hz). This stimulation did not only intensify slow oscillations as well as spindle activity, but also increased the efficacy of sleep to consolidate the previously learned word pairs (Marshall et al., 2006). Using the same stimulation at the same frequency (~0.75 Hz) during wakefulness increases theta activity and improves encoding of new learning material, while consolidation remains unchanged (Kirov et al., 2009). Finally, applying this slow oscillation stimulation during a daytime nap improves the encoding of new information afterward (Antonenko et al., 2013), suggesting that slow wave activity during prior sleep frees space for new learning after sleep. Even though some consumer devices for TES have become available (e.g., http://www.foc.us/, http://thebrainstimulator.net), the possibility to affect sleep's slow oscillations by auditory stimulation may offer a more elegant and less invasive approach for the bedroom (Ngo et al., 2013a). Ngo et al. timed short (50 ms) bursts of white noise on the slow oscillation up-states, which increased the amount of consecutive slow oscillations as well as spindle activity coupled to the slow oscillations. When compared to a sham condition, the stimulation improved sleep-dependent consolidation of declarative word pair memory (Ngo et al., 2013b). Such an auditory stimulation of slow oscillations could be applied in the home environment to boost memory performance with relatively little effort. Very recently it has been shown that slow wave sleep can even be enhanced by hypnosis (Cordi et al., 2014, 2015). This method may offer the possibility of enhancing sleep without recurring to invasive and potentially sleep-disturbing techniques.
Many neurotransmitters and hormones that are known to be implicated in learning and memory show characteristic changes across the sleep-wake cycle. It therefore stands to reason to apply certain drugs targeting specific neurotransmitter systems during sleep to enhance memory. GABA A modulators, for example, have long been known to induce sleep and have thus been used extensively to treat sleep disorders. Yet, these drugs may not induce natural sleep but rather light sleep with low amounts of slow wave sleep and REM sleep (Lancel, 1999). The GABA re-uptake inhibitor tiagabine, on the other hand, increases the occurrence of slow wave sleep, but unfortunately decreases sleep spindles and even deteriorates consolidation of a procedural motor skill task (Feld et al., 2013b). The GABA A positive modulator zolpidem has been shown to have a more beneficial profile, as it increases the amount of sleep spindles and improves memory consolidation of word pairs (Kaestner et al., 2013; Mednick et al., 2013). However, other authors do not find this effect or even observed a detrimental effect of zolpidem on declarative memory consolidation during sleep (Melendez et al., 2005; Hall-Porter et al., 2014). Recently it was shown that the NMDA receptor co-agonist d-cycloserine can enhance the consolidation of word pair associations if given before a retention interval containing sleep (Feld et al., 2013a). D-cycloserine has also been discussed as a cognitive enhancer to improve efficacy of cognitive behavior therapy to attenuate anxiety disorders (Otto et al., 2007; Hofmann et al., 2012), hence the application of d-cycloserine during retention sleep after successful exposure sessions may increase the therapeutic value of this drug. Other pharmacological treatments have likewise been shown to affect memory formation during sleep more or less beneficially, such as the noradrenaline reuptake inhibitor reboxetine (Rasch et al., 2009; Gais et al., 2011), the cytokine interleukin-6 (Benedict et al., 2009), and the dopamine D2-like receptor agonist pramipexole (Feld et al., 2014). Generally, drugs that increase sleep and memory are potentially interesting for clinical applications, but should presently not be considered for non-therapeutic sleep and memory enhancement, as the potential adverse effects remain completely unclear.
Sleep is not idle time. Sleep is an active state during which the brain processes information acquired during the previous day and prepares itself for the demands of the next day. Long-term memory is formed during sleep by a process that strengthens memory traces, reorganizes them, and integrates them into established knowledge networks. These processes do not only store previously acquired memories but also renew the capacity for new learning after sleep. Considering this backdrop, it is alarming how society still views sleep as a luxury. Sleep is not only a necessity. Rather we should start recognizing sleep as a worthy and skillful operator that helps our brain to work at its best. This review has discussed several ways of how to make optimal use of sleep's helpful hand in learning and memory. It is quite clear that improved sleeping conditions will have a higher impact on overall productivity than increasing the mere presence at work or at the study table. The ideal strategy to acquire new information should consider that sleep is important for memory. For students it is probably not effective to skip sleep in order to have more hours to learn for a specific subject. And for finding elegant solutions to complex problems the popular proverb may offer good advice: sleep on it.
The authors declare that the research was conducted in the absence of any commercial or financial relationships that could be construed as a potential conflict of interest.
This work was supported by a grant from the Deutsche Forschungsgemeinschaft SFB 654 “Plasticity and Sleep.”
Abel, T., Havekes, R., Saletin, J. M., and Walker, M. P. (2013). Sleep, plasticity and memory from molecules to whole-brain networks. Curr. Biol. 23, R774–R788. doi: 10.1016/j.cub.2013.07.025
Ahrberg, K., Dresler, M., Niedermaier, S., Steiger, A., and Genzel, L. (2012). The interaction between sleep quality and academic performance. J. Psychiatr. Res. 46, 1618–1622. doi: 10.1016/j.jpsychires.2012.09.008
Antonenko, D., Diekelmann, S., Olsen, C., Born, J., and Molle, M. (2013). Napping to renew learning capacity: enhanced encoding after stimulation of sleep slow oscillations. Eur. J. Neurosci. 37, 1142–1152. doi: 10.1111/ejn.12118
Antony, J. W., Gobel, E. W., O'Hare, J. K., Reber, P. J., and Paller, K. A. (2012). Cued memory reactivation during sleep influences skill learning. Nat. Neurosci. 15, 1114–1116. doi: 10.1038/nn.3152
Arzi, A., Holtzman, Y., Samnon, P., Eshel, N., Harel, E., and Sobel, N. (2014). Olfactory aversive conditioning during sleep reduces cigarette-smoking behavior. J. Neurosci. 34, 15382–15393. doi: 10.1523/JNEUROSCI.2291-14.2014
Arzi, A., Shedlesky, L., Ben-Shaul, M., Nasser, K., Oksenberg, A., Hairston, I. S., et al. (2012). Humans can learn new information during sleep. Nat. Neurosci. 15, 1460–1465. doi: 10.1038/nn.3193
Aserinsky, E., and Kleitman, N. (1953). Regularly occurring periods of eye motility, and concomitant phenomena, during sleep. Science 118, 273–274. doi: 10.1126/science.118.3062.273
Backhaus, J., Born, J., Hoeckesfeld, R., Fokuhl, S., Hohagen, F., and Junghanns, K. (2007). Midlife decline in declarative memory consolidation is correlated with a decline in slow wave sleep. Learn. Mem. 14, 336–341. doi: 10.1101/lm.470507
Barnes, D. C., and Wilson, D. A. (2014). Slow-wave sleep-imposed replay modulates both strength and precision of memory. J. Neurosci. 34, 5134–5142. doi: 10.1523/JNEUROSCI.5274-13.2014
Barrett, T. R., and Ekstrand, B. R. (1972). Effect of sleep on memory. 3. Controlling for time-of-day effects. J. Exp. Psychol. 96, 321–327. doi: 10.1037/h0033625
Battaglia, F. P., Benchenane, K., Sirota, A., Pennartz, C. M., and Wiener, S. I. (2011). The hippocampus: hub of brain network communication for memory. Trends Cogn. Sci. 15, 310–318. doi: 10.1016/j.tics.2011.05.008
Bendor, D., and Wilson, M. A. (2012). Biasing the content of hippocampal replay during sleep. Nat. Neurosci. 15, 1439–1444. doi: 10.1038/nn.3203
Benedict, C., Scheller, J., Rose-John, S., Born, J., and Marshall, L. (2009). Enhancing influence of intranasal interleukin-6 on slow-wave activity and memory consolidation during sleep. FASEB J. 23, 3629–3636. doi: 10.1096/fj.08-122853
Benson, K., and Feinberg, I. (1975). Sleep and memory: retention 8 and 24 hours after initial learning. Psychophysiology 12, 192–195. doi: 10.1111/j.1469-8986.1975.tb01275.x
Bergmann, T. O., Molle, M., Diedrichs, J., Born, J., and Siebner, H. R. (2012). Sleep spindle-related reactivation of category-specific cortical regions after learning face-scene associations. Neuroimage 59, 2733–2742. doi: 10.1016/j.neuroimage.2011.10.036
Borbély, A. A., and Achermann, P. (2000). “Sleep homeostasis and models of sleep regulation,” in Principles and Practice of Sleep Medicine, eds M. H. Kryger, T. Roth, W. C. Dement (Philadelphia, PA: WB Saunders Company), 377–90.
Born, J., and Feld, G. B. (2012). Sleep to upscale, sleep to downscale: balancing homeostasis and plasticity. Neuron 75, 933–935. doi: 10.1016/j.neuron.2012.09.007
Buzsaki, G. (1986). Hippocampal sharp waves: their origin and significance. Brain Res. 398, 242–252. doi: 10.1016/0006-8993(86)91483-6
Buzsaki, G. (1989). Two-stage model of memory trace formation: a role for “noisy” brain states. Neuroscience 31, 551–570. doi: 10.1016/0306-4522(89)90423-5
Buzsaki, G., and Draguhn, A. (2004). Neuronal oscillations in cortical networks. Science 304, 1926–1929. doi: 10.1126/science.1099745
Chauvette, S., Seigneur, J., and Timofeev, I. (2012). Sleep oscillations in the thalamocortical system induce long-term neuronal plasticity. Neuron 75, 1105–1113. doi: 10.1016/j.neuron.2012.08.034
Cirelli, C., and Tononi, G. (2008). Is sleep essential? PLoS Biol. 6:e216. doi: 10.1371/journal.pbio.0060216
Cordi, M. J., Hirsiger, S., Merillat, S., and Rasch, B. (2015). Improving sleep and cognition by hypnotic suggestion in the elderly. Neuropsychologia 69, 176–182. doi: 10.1016/j.neuropsychologia.2015.02.001
Cordi, M. J., Schlarb, A. A., and Rasch, B. (2014). Deepening sleep by hypnotic suggestion. Sleep. 37, 1143–1152. doi: 10.5665/sleep.3778
Cox, R., Korjoukov, I., de Boer, M., and Talamini, L. M. (2014). Sound asleep: processing and retention of slow oscillation phase-targeted stimuli. PLoS ONE 9:e101567. doi: 10.1371/journal.pone.0101567
Dement, W., and Kleitman, N. (1957). Cyclic variations in EEG during sleep and their relation to eye movements, body motility, and dreaming. Electroencephalogr. Clin. Neurophysiol. 9, 673–690. doi: 10.1016/0013-4694(57)90088-3
Diekelmann, S. (2014). Sleep for cognitive enhancement. Front. Syst. Neurosci. 8:46. doi: 10.3389/fnsys.2014.00046
Diekelmann, S., Biggel, S., Rasch, B., and Born, J. (2012). Offline consolidation of memory varies with time in slow wave sleep and can be accelerated by cuing memory reactivations. Neurobiol. Learn. Mem. 98, 103–111. doi: 10.1016/j.nlm.2012.07.002
Diekelmann, S., and Born, J. (2010). The memory function of sleep. Nat. Rev. Neurosci. 11, 114–126. doi: 10.1038/nrn2762
Diekelmann, S., and Born, J. (2015). Cueing fear memory during sleep - to extinguish or to enhance fear? Sleep 38, 337–339. doi: 10.5665/sleep.4484
Diekelmann, S., Born, J., and Wagner, U. (2010). Sleep enhances false memories depending on general memory performance. Behav. Brain Res. 208, 425–429. doi: 10.1016/j.bbr.2009.12.021
Diekelmann, S., Buchel, C., Born, J., and Rasch, B. (2011). Labile or stable: opposing consequences for memory when reactivated during waking and sleep. Nat. Neurosci. 14, 381–386. doi: 10.1038/nn.2744
Diekelmann, S., Wilhelm, I., Wagner, U., and Born, J. (2013a). Sleep improves prospective remembering by facilitating spontaneous-associative retrieval processes. PLoS ONE 8:e77621. doi: 10.1371/journal.pone.0077621
Diekelmann, S., Wilhelm, I., Wagner, U., and Born, J. (2013b). Sleep to implement an intention. Sleep 36, 149–153. doi: 10.5665/sleep.2322
Durrant, S. J., Taylor, C., Cairney, S., and Lewis, P. A. (2011). Sleep-dependent consolidation of statistical learning. Neuropsychologia 49, 1322–1331. doi: 10.1016/j.neuropsychologia.2011.02.015
Ekstrand, B. R. (1967). Effect of sleep on memory. J. Exp. Psychol. 75, 64–72. doi: 10.1037/h0024907
Ekstrand, B. R., Barrett, T. R., West, J. N., and Maier, W. G. (1977). “The effect of sleep on human long-term memory,” in Neurobiology of Sleep and Memory, eds R. Drucker-Colin, J. McGaugh (New York, NY: Academic Press), 419–38.
Esser, S. K., Hill, S. L., and Tononi, G. (2007). Sleep homeostasis and cortical synchronization: I. Modeling the effects of synaptic strength on sleep slow waves. Sleep 30, 1617–1630.
Euston, D. R., and Steenland, H. W. (2014). Neuroscience. Memories–getting wired during sleep. Science 344, 1087–1088. doi: 10.1126/science.1255649
Feld, G. B., Besedovsky, L., Kaida, K., Munte, T. F., and Born, J. (2014). Dopamine D2-like receptor activation wipes out preferential consolidation of high over low reward memories during human sleep. J. Cogn. Neurosci. 26, 2310–2320. doi: 10.1162/jocn_a_00629
Feld, G. B., Lange, T., Gais, S., and Born, J. (2013a). Sleep-dependent declarative memory consolidation–unaffected after blocking NMDA or AMPA receptors but enhanced by NMDA coagonist D-cycloserine. Neuropsychopharmacology 38 2688–2697. doi: 10.1038/npp.2013.179
Feld, G. B., Wilhelm, I., Ma, Y., Groch, S., Binkofski, F., Molle, M., et al. (2013b). Slow wave sleep induced by GABA agonist tiagabine fails to benefit memory consolidation. Sleep 36, 1317–1326. doi: 10.5665/sleep.2954
Fischer, S., and Born, J. (2009). Anticipated reward enhances offline learning during sleep. J. Exp. Psychol. Learn. Mem. Cogn. 35, 1586–1593. doi: 10.1037/a0017256
Fowler, M. J., Sullivan, M. J., and Ekstrand, B. R. (1973). Sleep and memory. Science 179, 302–304. doi: 10.1126/science.179.4070.302
Friedrich, M., Wilhelm, I., Born, J., and Friederici, A. D. (2015). Generalization of word meanings during infant sleep. Nat. Commun. 6, 6004. doi: 10.1038/ncomms7004
Gais, S., and Born, J. (2004). Low acetylcholine during slow-wave sleep is critical for declarative memory consolidation. Proc. Natl. Acad. Sci. U.S.A. 101, 2140–2144. doi: 10.1073/pnas.0305404101
Gais, S., Lucas, B., and Born, J. (2006). Sleep after learning aids memory recall. Learn. Mem. 13, 259–262. doi: 10.1101/lm.132106
Gais, S., Molle, M., Helms, K., and Born, J. (2002). Learning-dependent increases in sleep spindle density. J. Neurosci. 22, 6830–6834.
Gais, S., Rasch, B., Dahmen, J. C., Sara, S., and Born, J. (2011). The memory function of noradrenergic activity in non-REM sleep. J. Cogn. Neurosci. 23, 2582–2592. doi: 10.1162/jocn.2011.21622
Genzel, L., Ahrberg, K., Roselli, C., Niedermaier, S., Steiger, A., Dresler, M., et al. (2013). Sleep timing is more important than sleep length or quality for medical school performance. Chronobiol. Int. 30, 766–771. doi: 10.3109/07420528.2012.763132
Germain, A. (2013). Sleep disturbances as the hallmark of PTSD: where are we now? Am. J. Psychiatry 170, 372–382. doi: 10.1176/appi.ajp.2012.12040432
Gomez, R. L., Bootzin, R. R., and Nadel, L. (2006). Naps promote abstraction in language-learning infants. Psychol. Sci. 17, 670–674. doi: 10.1111/j.1467-9280.2006.01764.x
Groch, S., Wilhelm, I., Diekelmann, S., and Born, J. (2013). The role of REM sleep in the processing of emotional memories: evidence from behavior and event-related potentials. Neurobiol. Learn. Mem. 99, 1–9. doi: 10.1016/j.nlm.2012.10.006
Groch, S., Zinke, K., Wilhelm, I., and Born, J. (2014). Dissociating the contributions of slow-wave sleep and rapid eye movement sleep to emotional item and source memory. Neurobiol. Learn. Mem. doi: 10.1016/j.nlm.2014.08.013. [Epub ahead of print].
Grosmark, A. D., Mizuseki, K., Pastalkova, E., Diba, K., and Buzsaki, G. (2012). REM sleep reorganizes hippocampal excitability. Neuron 75, 1001–1007. doi: 10.1016/j.neuron.2012.08.015
Gulati, T., Ramanathan, D. S., Wong, C. C., and Ganguly, K. (2014). Reactivation of emergent task-related ensembles during slow-wave sleep after neuroprosthetic learning. Nat. Neurosci. 17, 1107–1113. doi: 10.1038/nn.3759
Hall-Porter, J. M., Schweitzer, P. K., Eisenstein, R. D., Ahmed, H. A., and Walsh, J. K. (2014). The effect of two benzodiazepine receptor agonist hypnotics on sleep-dependent memory consolidation. J. Clin. Sleep Med. 10, 27–34. doi: 10.5664/jcsm.3352
Harris, K. D. (2014). Sleep replay meets brain-machine interface. Nat. Neurosci. 17, 1019–1021. doi: 10.1038/nn.3769
Hasselmo, M. E. (1999). Neuromodulation: acetylcholine and memory consolidation. Trends Cogn. Sci. 3, 351–359. doi: 10.1016/S1364-6613(99)01365-0
Hauner, K. K., Howard, J. D., Zelano, C., and Gottfried, J. A. (2013). Stimulus-specific enhancement of fear extinction during slow-wave sleep. Nat. Neurosci. 16, 1553–1555. doi: 10.1038/nn.3527
He, J., Sun, H. Q., Li, S. X., Zhang, W. H., Shi, J., Ai, S. Z., et al. (2014). Effect of Conditioned stimulus exposure during slow wave sleep on fear memory extinction in humans. Sleep 38, 423–431. doi: 10.5665/sleep.4502
Hill, S., and Tononi, G. (2005). Modeling sleep and wakefulness in the thalamocortical system. J. Neurophysiol. 93, 1671–1698.
Hill, S., Tononi, G., and Ghilardi, M. F. (2008). Sleep improves the variability of motor performance. Brain Res. Bull. 76, 605–611. doi: 10.1016/j.brainresbull.2008.02.024
Hofmann, S. G., Sawyer, A. T., and Asnaani, A. (2012). D-cycloserine as an augmentation strategy for cognitive behavioral therapy for anxiety disorders: an update. Curr. Pharm. Des. 18, 5659–5662. doi: 10.1152/jn.00915.2004
Huber, R., Esser, S. K., Ferrarelli, F., Massimini, M., Peterson, M. J., and Tononi, G. (2007). TMS-induced cortical potentiation during wakefulness locally increases slow wave activity during sleep. PLoS ONE 2:e276. doi: 10.1371/journal.pone.0000276
Huber, R., Ghilardi, M. F., Massimini, M., Ferrarelli, F., Riedner, B. A., Peterson, M. J., et al. (2006). Arm immobilization causes cortical plastic changes and locally decreases sleep slow wave activity. Nat. Neurosci. 9, 1169–1176. doi: 10.1038/nn1758
Huber, R., Ghilardi, M. F., Massimini, M., and Tononi, G. (2004a). Local sleep and learning. Nature 430, 78–81. doi: 10.1038/nature02663
Huber, R., Hill, S. L., Holladay, C., Biesiadecki, M., Tononi, G., and Cirelli, C. (2004b). Sleep homeostasis in Drosophila melanogaster. Sleep 27, 628–639.
Huber, R., Maatta, S., Esser, S. K., Sarasso, S., Ferrarelli, F., Watson, A., et al. (2008). Measures of cortical plasticity after transcranial paired associative stimulation predict changes in electroencephalogram slow-wave activity during subsequent sleep. J. Neurosci. 28, 7911–7918. doi: 10.1523/JNEUROSCI.1636-08.2008
Ikeda, K., and Morotomi, T. (1996). Classical conditioning during human NREM sleep and response transfer to wakefulness. Sleep 19, 72–74.
Inostroza, M., and Born, J. (2013). Sleep for preserving and transforming episodic memory. Annu. Rev. Neurosci. 36, 79–102. doi: 10.1146/annurev-neuro-062012-170429
Jenkins, J. G., and Dallenbach, K. M. (1924). Obliviscence during sleep and waking. Am. J. Psychol. 35, 605–612. doi: 10.2307/1414040
Ji, D., and Wilson, M. A. (2007). Coordinated memory replay in the visual cortex and hippocampus during sleep. Nat. Neurosci. 10, 100–107. doi: 10.1038/nn1825
Kaestner, E. J., Wixted, J. T., and Mednick, S. C. (2013). Pharmacologically increasing sleep spindles enhances recognition for negative and high-arousal memories. J. Cogn. Neurosci. 25, 1597–1610. doi: 10.1162/jocn_a_00433
Kirov, R., Weiss, C., Siebner, H. R., Born, J., and Marshall, L. (2009). Slow oscillation electrical brain stimulation during waking promotes EEG theta activity and memory encoding. Proc. Natl. Acad. Sci. U.S.A. 106, 15460–15465. doi: 10.1073/pnas.0904438106
Kleim, B., Wilhelm, F. H., Temp, L., Margraf, J., Wiederhold, B. K., and Rasch, B. (2013). Sleep enhances exposure therapy. Psychol. Med. 44, 1511–1519. doi: 10.1017/S0033291713001748
Lahl, O., Wispel, C., Willigens, B., and Pietrowsky, R. (2008). An ultra short episode of sleep is sufficient to promote declarative memory performance. J. Sleep Res. 17, 3–10. doi: 10.1111/j.1365-2869.2008.00622.x
Lancel, M. (1999). Role of GABAA receptors in the regulation of sleep: initial sleep responses to peripherally administered modulators and agonists. Sleep 22, 33–42.
Landsness, E. C., Crupi, D., Hulse, B. K., Peterson, M. J., Huber, R., Ansari, H., et al. (2009). Sleep-dependent improvement in visuomotor learning: a causal role for slow waves. Sleep 32, 1273–1284.
Lansink, C. S., Goltstein, P. M., Lankelma, J. V., Joosten, R. N., McNaughton, B. L., and Pennartz, C. M. (2008). Preferential reactivation of motivationally relevant information in the ventral striatum. J. Neurosci. 28, 6372–6382. doi: 10.1523/JNEUROSCI.1054-08.2008
Lansink, C. S., Goltstein, P. M., Lankelma, J. V., McNaughton, B. L., and Pennartz, C. M. (2009). Hippocampus leads ventral striatum in replay of place-reward information. PLoS Biol. 7:e1000173. doi: 10.1371/journal.pbio.1000173
Lewis, P. A., and Durrant, S. J. (2011). Overlapping memory replay during sleep builds cognitive schemata. Trends Cogn. Sci. 15, 343–351. doi: 10.1016/j.tics.2011.06.004
Mander, B. A., Rao, V., Lu, B., Saletin, J. M., Ancoli-Israel, S., Jagust, W. J., et al. (2013a). Impaired prefrontal sleep spindle regulation of hippocampal-dependent learning in older adults. Cereb. Cortex. doi: 10.1093/cercor/bht188
Mander, B. A., Rao, V., Lu, B., Saletin, J. M., Lindquist, J. R., Ancoli-Israel, S., et al. (2013b). Prefrontal atrophy, disrupted NREM slow waves and impaired hippocampal-dependent memory in aging. Nat. Neurosci. 16, 357–364. doi: 10.1038/nn.3324
Mander, B. A., Santhanam, S., Saletin, J. M., and Walker, M. P. (2011). Wake deterioration and sleep restoration of human learning. Curr. Biol. 21, R183–R184. doi: 10.1016/j.cub.2011.01.019
Maquet, P., Laureys, S., Peigneux, P., Fuchs, S., Petiau, C., Phillips, C., et al. (2000). Experience-dependent changes in cerebral activation during human REM sleep. Nat. Neurosci. 3, 831–836. doi: 10.1038/77744
Maret, S., Faraguna, U., Nelson, A. B., Cirelli, C., and Tononi, G. (2011). Sleep and waking modulate spine turnover in the adolescent mouse cortex. Nat. Neurosci. 14, 1418–1420. doi: 10.1038/nn.2934
Marshall, L., Helgadottir, H., Molle, M., and Born, J. (2006). Boosting slow oscillations during sleep potentiates memory. Nature 444, 610–613. doi: 10.1038/nature05278
Massimini, M., Tononi, G., and Huber, R. (2009). Slow waves, synaptic plasticity and information processing: insights from transcranial magnetic stimulation and high-density EEG experiments. Eur. J. Neurosci. 29, 1761–1770. doi: 10.1111/j.1460-9568.2009.06720.x
McDermott, C. M., LaHoste, G. J., Chen, C., Musto, A., Bazan, N. G., and Magee, J. C. (2003). Sleep deprivation causes behavioral, synaptic, and membrane excitability alterations in hippocampal neurons. J. Neurosci. 23, 29 9687–9695.
Mednick, S. C., McDevitt, E. A., Walsh, J. K., Wamsley, E., Paulus, M., Kanady, J. C., et al. (2013). The critical role of sleep spindles in hippocampal-dependent memory: a pharmacology study. J. Neurosci. 33, 4494–4504. doi: 10.1523/JNEUROSCI.3127-12.2013
Melendez, J., Galli, I., Boric, K., Ortega, A., Zuniga, L., Henriquez-Roldan, C. F., et al. (2005). Zolpidem and triazolam do not affect the nocturnal sleep-induced memory improvement. Psychopharmacology (Berl). 181, 21–26. doi: 10.1007/s00213-005-2228-0
Mölle, M., Bergmann, T. O., Marshall, L., and Born, J. (2011). Fast and slow spindles during the sleep slow oscillation: disparate coalescence and engagement in memory processing. Sleep 34, 1411–1421. doi: 10.5665/sleep.1290
Molle, M., and Born, J. (2011). Slow oscillations orchestrating fast oscillations and memory consolidation. Prog. Brain Res. 193, 93–110. doi: 10.1016/B978-0-444-53839-0.00007-7
Nadasdy, Z., Hirase, H., Czurko, A., Csicsvari, J., and Buzsaki, G. (1999). Replay and time compression of recurring spike sequences in the hippocampus. J. Neurosci. 19, 9497–9507.
Ngo, H. V., Claussen, J. C., Born, J., and Molle, M. (2013a). Induction of slow oscillations by rhythmic acoustic stimulation. J. Sleep Res. 22, 22–31. doi: 10.1111/j.1365-2869.2012.01039.x
Ngo, H. V., Martinetz, T., Born, J., and Molle, M. (2013b). Auditory closed-loop stimulation of the sleep slow oscillation enhances memory. Neuron 78, 545–553. doi: 10.1016/j.neuron.2013.03.006
Otto, M. W., Basden, S. L., Leyro, T. M., McHugh, R. K., and Hofmann, S. G. (2007). Clinical perspectives on the combination of D-cycloserine and cognitive-behavioral therapy for the treatment of anxiety disorders. CNS Spectr. 12, 51–6, 9–61.
Oudiette, D., and Paller, K. A. (2013). Upgrading the sleeping brain with targeted memory reactivation. Trends Cogn. Sci. 17, 142–149. doi: 10.1016/j.tics.2013.01.006
Pace-Schott, E. F., Milad, M. R., Orr, S. P., Rauch, S. L., Stickgold, R., and Pitman, R. K. (2009). Sleep promotes generalization of extinction of conditioned fear. Sleep 32, 19–26.
Pace-Schott, E. F., Verga, P. W., Bennett, T. S., and Spencer, R. M. (2012). Sleep promotes consolidation and generalization of extinction learning in simulated exposure therapy for spider fear. J. Psychiatr. Res. 46, 1036–1044. doi: 10.1016/j.jpsychires.2012.04.015
Payne, J. D., Chambers, A. M., and Kensinger, E. A. (2012a). Sleep promotes lasting changes in selective memory for emotional scenes. Front. Integr. Neurosci. 6:108. doi: 10.3389/fnint.2012.00108
Payne, J. D., Schacter, D. L., Propper, R. E., Huang, L. W., Wamsley, E. J., Tucker, M. A., et al. (2009). The role of sleep in false memory formation. Neurobiol. Learn. Mem. 92, 327–334. doi: 10.1016/j.nlm.2009.03.007
Payne, J. D., Stickgold, R., Swanberg, K., and Kensinger, E. A. (2008). Sleep preferentially enhances memory for emotional components of scenes. Psychol. Sci. 19, 781–788. doi: 10.1111/j.1467-9280.2008.02157.x
Payne, J. D., Tucker, M. A., Ellenbogen, J. M., Wamsley, E. J., Walker, M. P., Schacter, D. L., et al. (2012b). Memory for semantically related and unrelated declarative information: the benefit of sleep, the cost of wake. PLoS ONE 7:e33079. doi: 10.1371/journal.pone.0033079
Peigneux, P., Laureys, S., Fuchs, S., Collette, F., Perrin, F., Reggers, J., et al. (2004). Are spatial memories strengthened in the human hippocampus during slow wave sleep? Neuron 44, 535–545. doi: 10.1016/j.neuron.2004.10.007
Plihal, W., and Born, J. (1997). Effects of early and late nocturnal sleep on declarative and procedural memory. J. Cogn. Neurosci. 9, 534–547. doi: 10.1162/jocn.1997.9.4.534
Prince, T. M., and Abel, T. (2013). The impact of sleep loss on hippocampal function. Learn. Mem. 20, 558–569. doi: 10.1101/lm.031674.113
Rasch, B., and Born, J. (2013). About sleep's role in memory. Physiol. Rev. 93, 681–766. doi: 10.1152/physrev.00032.2012
Rasch, B., Buchel, C., Gais, S., and Born, J. (2007). Odor cues during slow-wave sleep prompt declarative memory consolidation. Science 315, 1426–1429. doi: 10.1126/science.1138581
Rasch, B., Pommer, J., Diekelmann, S., and Born, J. (2009). Pharmacological REM sleep suppression paradoxically improves rather than impairs skill memory. Nat. Neurosci. 12, 396–397. doi: 10.1038/nn.2206
Ribeiro, S., Gervasoni, D., Soares, E. S., Zhou, Y., Lin, S. C., Pantoja, J., et al. (2004). Long-lasting novelty-induced neuronal reverberation during slow-wave sleep in multiple forebrain areas. PLoS Biol. 2:E24. doi: 10.1371/journal.pbio.0020024
Ribeiro, S., Shi, X., Engelhard, M., Zhou, Y., Zhang, H., Gervasoni, D., et al. (2007). Novel experience induces persistent sleep-dependent plasticity in the cortex but not in the hippocampus. Front. Neurosci. 1, 43–55. doi: 10.3389/neuro.01.1.1.003.2007
Ribeiro, S., and Stickgold, R. (2014). Sleep and school education. Trends Neurosci. Educ. 3, 18–23. doi: 10.1016/j.tine.2014.02.004
Rolls, A., Makam, M., Kroeger, D., Colas, D., de Lecea, L., and Heller, H. C. (2013). Sleep to forget: interference of fear memories during sleep. Mol. Psychiatry 18, 1166–1170. doi: 10.1038/mp.2013.121
Rudoy, J. D., Voss, J. L., Westerberg, C. E., and Paller, K. A. (2009). Strengthening individual memories by reactivating them during sleep. Science 326, 1079. doi: 10.1126/science.1179013
Saletin, J. M., and Walker, M. P. (2012). Nocturnal mnemonics: sleep and hippocampal memory processing. Front. Neurol. 3:59. doi: 10.3389/fneur.2012.00059
Schmidt, C., Peigneux, P., Muto, V., Schenkel, M., Knoblauch, V., Munch, M., et al. (2006). Encoding difficulty promotes postlearning changes in sleep spindle activity during napping. J. Neurosci. 26, 8976–8982. doi: 10.1523/JNEUROSCI.2464-06.2006
Schonauer, M., Geisler, T., and Gais, S. (2014). Strengthening procedural memories by reactivation in sleep. J. Cogn. Neurosci. 26, 143–153. doi: 10.1162/jocn_a_00471
Scullin, M. K., and McDaniel, M. A. (2010). Remembering to execute a goal: sleep on it! Psychol. Sci. 21, 1028–1035.
Seehagen, S., Konrad, C., Herbert, J. S., and Schneider, S. (2015). Timely sleep facilitates declarative memory consolidation in infants. Proc. Natl. Acad. Sci. U.S.A. 112, 1625–1629. doi: 10.1073/pnas.1414000112
Sirota, A., Csicsvari, J., Buhl, D., and Buzsaki, G. (2003). Communication between neocortex and hippocampus during sleep in rodents. Proc. Natl. Acad. Sci. U.S.A. 100, 2065–2069. doi: 10.1073/pnas.0437938100
Skaggs, W. E., and McNaughton, B. L. (1996). Replay of neuronal firing sequences in rat hippocampus during sleep following spatial experience. Science 271, 1870–1873. doi: 10.1126/science.271.5257.1870
Steiger, A., Dresler, M., Kluge, M., and Schussler, P. (2013). Pathology of sleep, hormones and depression. Pharmacopsychiatry 46(Suppl. 1), S30–S35. doi: 10.1055/s-0033-1337921
Sterpenich, V., Albouy, G., Boly, M., Vandewalle, G., Darsaud, A., Balteau, E., et al. (2007). Sleep-related hippocampo-cortical interplay during emotional memory recollection. PLoS Biol. 5:e282. doi: 10.1371/journal.pbio.0050282
Sterpenich, V., Albouy, G., Darsaud, A., Schmidt, C., Vandewalle, G., Dang Vu, T. T., et al. (2009). Sleep promotes the neural reorganization of remote emotional memory. J. Neurosci. 29, 5143–5152. doi: 10.1523/JNEUROSCI.0561-09.2009
Stickgold, R., and Walker, M. P. (2013). Sleep-dependent memory triage: evolving generalization through selective processing. Nat. Neurosci. 16, 139–145. doi: 10.1038/nn.3303
Sullivan, T. J., and de Sa, V. R. (2008). Sleeping our way to weight normalization and stable learning. Neural Comput. 20, 3111–3130. doi: 10.1162/neco.2008.04-07-502
Talamini, L. M., Nieuwenhuis, I. L., Takashima, A., and Jensen, O. (2008). Sleep directly following learning benefits consolidation of spatial associative memory. Learn. Mem. 15, 233–237. doi: 10.1101/lm.771608
Tononi, G., and Cirelli, C. (2003). Sleep and synaptic homeostasis: a hypothesis. Brain Res. Bull. 62, 143–150. doi: 10.1016/j.brainresbull.2003.09.004
Tononi, G., and Cirelli, C. (2006). Sleep function and synaptic homeostasis. Sleep Med. Rev. 10, 49–62. doi: 10.1016/j.smrv.2005.05.002
Tononi, G., and Cirelli, C. (2012). Time to Be SHY? Some comments on sleep and synaptic homeostasis. Neural Plasticity 2012:415250. doi: 10.1155/2012/415250
Tononi, G., and Cirelli, C. (2014). Sleep and the price of plasticity: from synaptic and cellular homeostasis to memory consolidation and integration. Neuron 81, 12–34. doi: 10.1016/j.neuron.2013.12.025
Tucker, M. A., and Fishbein, W. (2009). The impact of sleep duration and subject intelligence on declarative and motor memory performance: how much is enough? J. Sleep Res. 18, 304–312. doi: 10.1111/j.1365-2869.2009.00740.x
Van Der Werf, Y. D., Altena, E., Schoonheim, M. M., Sanz-Arigita, E. J., Vis, J. C., De Rijke, W., et al. (2009). Sleep benefits subsequent hippocampal functioning. Nat. Neurosci. 12, 122–123. doi: 10.1038/nn.2253
van Dongen, E. V., Takashima, A., Barth, M., Zapp, J., Schad, L. R., Paller, K. A., et al. (2012). Memory stabilization with targeted reactivation during human slow-wave sleep. Proc. Natl. Acad. Sci. U.S.A. 109, 10575–10580. doi: 10.1073/pnas.1201072109
Vassalli, A., and Dijk, D. J. (2009). Sleep function: current questions and new approaches. Eur. J. Neurosci. 29, 1830–1841. doi: 10.1111/j.1460-9568.2009.06767.x
Vorster, A. P., Krishnan, H. C., Cirelli, C., and Lyons, L. C. (2014). Characterization of sleep in Aplysia californica. Sleep 37, 1453–1463. doi: 10.5665/sleep.3992
Vyazovskiy, V. V., Cirelli, C., Pfister-Genskow, M., Faraguna, U., and Tononi, G. (2008). Molecular and electrophysiological evidence for net synaptic potentiation in wake and depression in sleep. Nat. Neurosci. 11, 200–208. doi: 10.1038/nn2035
Wagner, U., Gais, S., and Born, J. (2001). Emotional memory formation is enhanced across sleep intervals with high amounts of rapid eye movement sleep. Learn. Mem. 8, 112–119. doi: 10.1101/lm.36801
Wagner, U., Gais, S., Haider, H., Verleger, R., and Born, J. (2004). Sleep inspires insight. Nature 427, 352–355. doi: 10.1038/nature02223
Wilhelm, I., Diekelmann, S., and Born, J. (2008). Sleep in children improves memory performance on declarative but not procedural tasks. Learn. Mem. 15, 373–377. doi: 10.1101/lm.803708
Wilhelm, I., Diekelmann, S., Molzow, I., Ayoub, A., Molle, M., and Born, J. (2011). Sleep selectively enhances memory expected to be of future relevance. J. Neurosci. 31, 1563–1569. doi: 10.1523/JNEUROSCI.3575-10.2011
Wilhelm, I., Rose, M., Imhof, K. I., Rasch, B., Buchel, C., and Born, J. (2013). The sleeping child outplays the adult's capacity to convert implicit into explicit knowledge. Nat. Neurosci. 16, 391–393. doi: 10.1038/nn.3343
Williams, S. E., and Horst, J. S. (2014). Goodnight book: sleep consolidation improves word learning via storybooks. Front. Psychol. 5:184. doi: 10.3389/fpsyg.2014.00184
Wilson, M. A., and McNaughton, B. L. (1994). Reactivation of hippocampal ensemble memories during sleep. Science 265, 676–679. doi: 10.1126/science.8036517
Winocur, G., Moscovitch, M., and Bontempi, B. (2010). Memory formation and long-term retention in humans and animals: convergence towards a transformation account of hippocampal-neocortical interactions. Neuropsychologia 48, 2339–2356. doi: 10.1016/j.neuropsychologia.2010.04.016
Yang, G., Lai, C. S., Cichon, J., Ma, L., Li, W., and Gan, W. B. (2014). Sleep promotes branch-specific formation of dendritic spines after learning. Science 344, 1173–1178. doi: 10.1126/science.1249098
Yaroush, R., Sullivan, M. J., and Ekstrand, B. R. (1971). Effect of sleep on memory. II. Differential effect of the first and second half of the night. J. Exp. Psychol. 88, 361–366. doi: 10.1037/h0030914
Keywords: sleep, memory, learning, consolidation, applied research
Citation: Feld GB and Diekelmann S (2015) Sleep smart—optimizing sleep for declarative learning and memory. Front. Psychol. 6:622. doi: 10.3389/fpsyg.2015.00622
Received: 17 September 2014; Accepted: 27 April 2015;
Published: 12 May 2015.
Edited by:
Snehlata Jaswal, Indian Institute of Technology Jodhpur, IndiaReviewed by:
Beat Meier, University of Bern, SwitzerlandCopyright © 2015 Feld and Diekelmann. This is an open-access article distributed under the terms of the Creative Commons Attribution License (CC BY). The use, distribution or reproduction in other forums is permitted, provided the original author(s) or licensor are credited and that the original publication in this journal is cited, in accordance with accepted academic practice. No use, distribution or reproduction is permitted which does not comply with these terms.
*Correspondence: Susanne Diekelmann, Institute for Medical Psychology and Behavioral Neurobiology, University of Tübingen, Otfried-Müller-Straße 25, 72076 Tübingen, Germany,c3VzYW5uZS5kaWVrZWxtYW5uQHVuaS10dWViaW5nZW4uZGU=
†These authors have contributed equally to this work
Disclaimer: All claims expressed in this article are solely those of the authors and do not necessarily represent those of their affiliated organizations, or those of the publisher, the editors and the reviewers. Any product that may be evaluated in this article or claim that may be made by its manufacturer is not guaranteed or endorsed by the publisher.
Research integrity at Frontiers
Learn more about the work of our research integrity team to safeguard the quality of each article we publish.