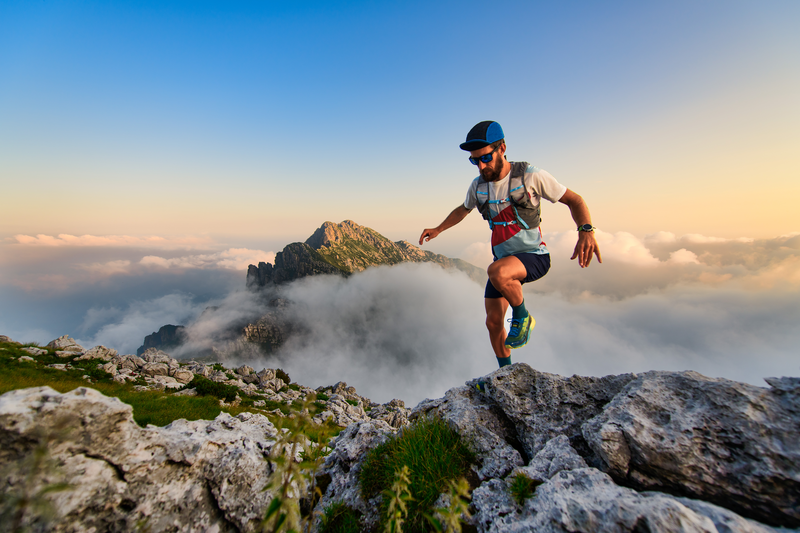
94% of researchers rate our articles as excellent or good
Learn more about the work of our research integrity team to safeguard the quality of each article we publish.
Find out more
ORIGINAL RESEARCH article
Front. Psychol. , 19 August 2011
Sec. Movement Science
volume 2 - 2011 | https://doi.org/10.3389/fpsyg.2011.00194
The purpose of this training study was to determine the magnitude of strength gains following a high-intensity resistance training (i.e., improvement of neuromuscular coordination) that can be achieved by imagery of the respective muscle contraction imagined maximal isometric contraction (IMC training). Prior to the experimental intervention, subjects completed a 4-week standardized strength training program. 3 groups with different combinations of real maximum voluntary contraction (MVC) and mental (IMC) strength training (M75, M50, M25; numbers indicate percentages of mental trials) were compared to a MVC-only training group (M0) and a control condition without strength training (CO). Training sessions (altogether 12) consisted of four sets of two maximal 5-s isometric contractions with 10 s rest between sets of either MVC or IMC training. Task-specific effects of IMC training were tested in four strength exercises commonly used in practical settings (bench pressing, leg pressing, triceps extension, and calf raising). Maximum isometric voluntary contraction force (MVC) was measured before and after the experimental training intervention and again 1 week after cessation of the program. IMC groups (M25, M50, M75) showed slightly smaller increases in MVC (3.0% to 4.2%) than M0 (5.1%), but significantly stronger improvements than CO (−0.2%). Compared to further strength gains in M0 after 1 week (9.4% altogether), IMC groups showed no “delayed” improvement, but the attained training effects remained stable. It is concluded that high-intensity strength training sessions can be partly replaced by IMC training sessions without any considerable reduction of strength gains.
Traditionally, mental training has been applied to tasks with mainly coordinative affordances. It is based on vivid mental images containing a perceptual experience in the absence of overt behavior and therefore with no sensory input (Annett, 1995). Often, mental imagery of motor actions relies on a first-person perspective including specific kinesthetic sensations (Munzert and Zentgraf, 2009). In this case, it is denoted as motor imagery (Decety, 1995; Jeannerod, 2001; Sirigu and Duhamel, 2001). Meta-analyses show that not only highly cognitive but also primarily motor tasks benefit considerably from mental training (Feltz and Landers, 1983; Driskell et al., 1994). Yue and Cole (1992) extended the applicability of mental training to the field of strength training by demonstrating a significant effect of motor imagery in an isometric force production task.
In the Yue and Cole study, two groups of participants completed a 4-week training of isometric force production with the little finger by either physical maximum voluntary contraction (MVC) or mental training imagined maximal isometric contraction (IMC). Both groups showed a comparable strength gain (MVC: 29.8%, IMC: 22%) that differed significantly from a control group with no training (3.7%). Moreover, strength gains were also found for the contralateral side not included in the training regime. This transfer to the contralateral part of the body highlights the importance of central neural processes in strength production, because peripheral processes (e.g., on the muscular level) are unlikely to substantially contribute to strength gains on the contralateral side (Carroll et al., 2006; Lee and Carroll, 2007).
Using the same task as Yue and Cole (1992), Smith et al. (2003) also found significant effects of mental training with remarkable strength gains (23.3%). Another study on the IMC effect to a larger muscle group (elbow flexion) resulted in a somewhat smaller increase of strength with a gain of 13.5% (Ranganathan et al., 2004). An effect of imagery motor training was also found on torque production of ankle plantar flexor muscles (Zijdewind et al., 2003). In this study, IMC training produced a strength gain of 36.3%. As the control group without training showed improvements of 14%, a 22% strength gain could be attributed to IMC training. Reiser (2005) demonstrated a smaller but significant increase of strength (5.7%) following IMC training on an isometric bench pressing task. More specifically, strength gains were restricted to the first training sessions and did not lead to the same improvements as physical training. Table 1 summarizes studies on the effect of imagined muscle contraction training (IMC).
Table 1. Schedule of mental training in different studies and size of IMC effect (proportional modification). Studies are split into small muscle groups (finger abduction) and large (close to everyday) muscle groups or strength exercises (bench press, elbow flexion, plantar flexion).
Some clinical studies also provide evidence for IMC training effects on neuromuscular activation. For example, Newsom et al. (2003) have shown that strength losses occurring during immobilization of joints can be reduced significantly by motor imagery. Periods of muscle unloading can lead to a rapid loss of muscle mass (Hortobáyi et al., 2000; Mulder et al., 2006). However, strength losses as a consequence of muscular inactivity in the first 2 weeks after immobilization result mainly from the reduced ability of the neural system to activate muscle fibers and to a lesser extent from muscle atrophy (Deschenes et al., 2002).
A large number of brain mapping studies have shown that the same neural areas are activated during either physical or mental simulation of motor actions. This holds not only for cortical areas such as the premotor cortex (PMC), the supplementary motor area (SMA), and the primary motor cortex (M1), but also for subcortical areas such as the basal ganglia and the cerebellum (Lotze et al., 1999; Jeannerod, 2001; Lafleur et al., 2002; Munzert et al., 2008). Considering force production, Ranganathan et al. (2004) showed imagery-induced enhancements of the EEG signal associated with the SMA and the contralateral sensory motor areas after successful IMC intervention. In this context, it is essential to note that the magnitude of force production is directly proportional to the amplitude of the respective brain signal (Dai et al., 2001). Similar findings have been reported in other EEG studies (Romero et al., 2000; Siemionow et al., 2000). These neurophysiological findings can be taken as a strong argument that IMC strength gains are caused primarily by central neural processes. Further evidence for this view comes from studies demonstrating an increase of neural activity in the contralateral motor cortex depending on the level of mental effort (Ranganathan et al., 2002).
Nonetheless, it is still unclear to what extent IMC effects shown in laboratory experiments can be transferred across specific field conditions. One particular practical application might be to reduce the demands on the musculoskeletal system associated with “heavy load training” sessions. In order to improve maximal voluntary neural activation, maximal force training sessions are typically applied with very high loads (>90% 1RM). Hence, IMC training could be a suitable way of contributing to a reduction of stress on passive structures (e.g., avoiding overtraining) while simultaneously preserving maximal voluntary neural activation.
Because it is not clear how far strength training sessions can be replaced by IMC training without a substantial reduction of strength gains, the objective of the present study was to determine what would be a useful proportion of physical and mental training. The level of effectiveness of mental training within physical training was tested by using different ratios of physical to mental training (3:1, 2:2, 1:3). These conditions were then compared to exclusive physical training without any mental rehearsal. In general, we predict similar strength gains for the IMC groups compared to the exclusively physical training group. To address applied problems in sports settings, subjects completed IMC interventions after a 4-week standardized training program.
All subjects completed the same 4-week resistance training with submaximal loads prior to the actual intervention followed by a supervised 4-week training program consisting of maximal isometric contractions (MVC) and IMC respectively (see Figure 1A). Three groups performed different combinations of physical (MVC) and mental (IMC) strength training (M75, M50, M25; numbers indicate percentages of mental trials; see Figure 1B). These groups were compared to a group with MVC training only (M0). Experimental groups M75, M50, M25, and M0 performed the same total number of trials either physically and/or mentally. Each subject practiced two of the four exercises (see below). The first exercise in each training session was determined at random. The two non-practiced exercises then served as a within-subject control condition without strength training (CO).
Figure 1. (A) The temporal structure of the experimental procedure. (B) Experimental groups (M75, M50, M25) performing different ratios of mental (IMC) and physical (MVC) training (3:1, 2:2, 1:3) for the same number of trials were compared with a MVC-only training group (M0).
Maximum isometric voluntary contraction force (MVC) was measured before and after the intervention and again 1 week after cessation of the program. Groups were matched in terms of pretest performance.
A total of 43 healthy sport students (20 female) from the Institute of Sports Science of the Justus Liebig University Giessen, Germany (mean age = 22.7 years, SD = 2.3 years) participated in this study. The subjects had experience with strength training (mean 2 h/week) and were familiar with the exercises, but none of them had recently undertaken an isometric high-resistance training. Testing with the movement imagery questionnaire (MIQ; Hall and Martin, 1997) revealed that all subjects could rehearse vivid kinesthetic images of their motor actions. A mean score above 3.0 on a scale from 1 (very easy to image) to 7 (very difficult to image) was set as a cutoff criterion for participation. All subjects gave their informed consent for the study, and the experimental protocol was conducted in accordance with the declaration of Helsinki.
Four training exercises were performed that differed in terms of extremity (upper vs. lower) and complexity (single-joint vs. multijoint). Exercises were the bench press (upper, multi), leg press (lower, multi), triceps extension (upper, single), and calf raise (lower, single). To compare IMC and physical training, the respective strength gains are given as percentages, because subjects trained different exercises (resulting in different absolute strength gains). This makes it possible to summarize the four strength exercises in the statistical analysis. Training and test exercises were identical in the treatment groups but not in the control condition.
The measurement of maximum isometric contractions force was carried out on standard strength training devices. For test contractions, subjects performed two 5-s maximal efforts separated by a 90-s resting interval. Instruction focused on gradually increasing force to a peak after approximately 2 s (Figures 2 and 3). Subjects practiced a small number of training trials (between three and eight) to familiarize themselves with the procedure.
Figure 2. Force traces from an individual test session illustrating maximum force production in leg pressing and calf raising.
Figure 3. Force traces from an individual test session illustrating maximum force production in and bench pressing and triceps extension.
Bench pressing was performed on a multipress. Using steel chains and carbines, the barbell was attached to the frame of the bench press in order to produce isometric conditions. The chain length was adjusted individually so that subjects could train with an arm and elbow flexion of approximately 90°. Working positions could be reproduced precisely by means of markings on the barbell and a device to adjust the horizontal distance of the subject relative to the barbell.
Leg pressing was tested on a sled 45° leg press. Again, the sled could be fixed to produce isometric conditions. Chains were adjusted so that subjects achieved a 100° angle at their knees. Triceps extension force was measured unilaterally. Subjects were seated on a preacher bench with their upper arm supported. They grasped a handle attached to a cable robe fixed behind them above the head. The length of the cable was adjusted so that subjects reached a 90° angle with their elbow.
To measure the force of the calf raise, subjects sat on a calf-raise machine with their upper thighs placed under a fixed leg pad just above the knees.
A linkage construction was used to produce isometric working conditions. This consisted of steel chains linked by carbines and rigidly coupled with a force sensor system (System DigiMax, Fa. mechaTronic GmbH, Hamm, Germany). The signal from the calibrated force sensor was sampled at 1000 Hz. To remove high-frequency fluctuations from the force signal, data were filtered using a second-order Butterworth filter. Based on visual inspection of the filtering results, the cutoff frequency was set at 6 Hz, which allows adequate quantification of the maximum force value. For each exercise, the peak force produced was measured twice. The highest contraction force obtained from the recorded test trials was taken as the MVC force. All tested exercises revealed high correlations between the two MVC measurements at each measurement time point (all r > 0.93), indicating adequate reliability.
Prior to the intervention, all subjects performed a 4-week standardized training program twice a week under the individual supervision of one of the investigators. Subjects trained four sets of each of the two exercises performing dynamic executions using a “15RM” load. The 15RM load was determined at the first training session, using a multiple repetition protocol (Fleck and Kraemer, 2004). Training loads were then increased according to strength increments. Relative changes of weights from the second to the last “submaximal” training session were used to quantify strength gains in relation to the standardized training program. Because this training phase was also used to familiarize subjects with the training procedure, it was possible to rule out any interpretation of the strength gains in the following intervention as being a result of repeated measurements (Rutherford and Jones, 1986; Fleck and Kraemer, 2004).
The experimental training sessions (12 in total) consisted either of MVC or IMC training three times each week for 4 weeks. It should be noted that all exercises during experimental intervention and testing were isometric. MVC training included four series of two maximal 5-s isometric contractions with a 10-s rest between contractions and a 90-s rest between series. IMC training was arranged in the same temporal pattern. Durations for active and mental trials were standardized regardless of differences to durations in dynamical task settings. All subjects completed their IMC sessions individually under the supervision of one of the investigators. They were instructed to imagine maximal contraction efforts as vividly as possible, using kinesthetic imagery (“you should imagine the sensation associated with a contraction effort, but your muscles must stay relaxed”). A preference for kinesthetic versus visual imagery is found in almost all instructions for IMC procedures. This approach is underpinned by studies showing that motor imagery is more effective when associated with “motor” tasks than visual imagery (Féry, 2003). Following the instruction “position,” subjects imagined the starting position. “Start” marked the beginning of an effort, and “OK” was the signal to finish the imagined contraction. The timing of the contractions was instructed verbally. Most subjects preferred to close their eyes during imaged contractions. Visual controls were used to ensure that no actual muscle contractions were done during the mental training. It is possible to observe even small muscle contractions in the respective muscle groups. In the few cases in which a contraction was visible, feedback was given telling the subject to relax the muscles. This procedure has been applied in a previous study on IMC training (Reiser, 2005) and has proved itself. More exactly but costly EMG method to control for any muscular activity during imagined contractions was not used. Therefore, it can not be completely excluded that very small activities (background EMG) occurred. However, it was shown several times that subjects are well able to realize IMC instructions with muscle activation levels (normalized to MVC EMG) near zero (Yue and Cole, 1992; Ranganathan et al., 2004), indicating inactive muscles during IMC training.
After each session, subjects were asked to rate the vividness of their kinesthetic images on a scale from 1 (no imagery could be performed) to 5 (vivid imagery could be performed). The ranking scale was constructed following the scale of the MIQ, but is a five and not a seven stage scale. This rating procedure had already been applied in a previous study on IMC training (Reiser, 2005). To familiarize subjects with the general procedure and to facilitate the generation of kinesthetic images, the IMC procedure was rehearsed in a pretraining session.
Maximum voluntary contraction gains of the individual exercises did not differ, either at Posttest 1, F(3, 84) = 0.812, p = 0.491, nor at Posttest 2, F(3, 67) = 0.215, p = 0.886. Therefore average percentage gain in strength across the both trained, respectively the two both untrained exercises within a subject were used for statistical analysis.
A 2 × 2 analysis of variance (ANOVA) with the between factor IMC groups and with repeated measures for the comparison of individual differences between trained and not trained exercises was used to test for training effects, that means for different alterations from Pretest to Posttest 1 and from Pretest to Posttest 2, respectively. To test for group effects between the two posttests, that is, to determine whether MVC gains in the IMC groups differed from Posttest 1 to Posttest 2, a 2 × 2 ANOVA with the between factor IMC groups and with repeated measures for differences between Posttest 1 and Posttest 2 was computed.
The significance level for statistical analyses was set at p < 0.05. Effect sizes and d were calculated to evaluate the magnitude of the treatment effects as well as the practical significance. Data are presented as means ± SE.
Irrespective of their later treatment, all subjects first completed a standardized (physical) training program. Training with submaximal loads in these sessions led to considerable strength gains as reflected in increasingly heavier training weights, t(85) = 9.04, p < 0.001, d = 1.00 with weight adjustments ranging comparably between 21.3% and 29.6%, F(3, 82) = 0.49, p = 0.69.
The experimental intervention started with 12 subjects within each of the four groups. A total of 43 subjects completed the pre- and posttest (Posttest 1) for the IMC/MVC training and 35 subjects completed a second posttest (Posttest 2). Figures 4 and 5 shows the percentage increases of MVC for all groups. Throughout, strength gains for the trained exercises were higher compared to the CO condition. For Posttest 1, as well as for Posttest 2, an ANOVA with repeated measures for percentage strength gains of IMC conditions contrasted with control conditions reveals a significant within practice effect [Posttest1: F(1, 39) = 10.46, p = 0.002, Posttest 2: F(1, 31) = 5.83, p = 0.022,
]. The M0 group, which carried out a complete physical training, achieved the strongest improvements at Posttest 1 (4.3%) and Posttest 2 (8.3%). Strength gains in the groups with mental training rates (M25, M50, M75) were slightly lower (between 3.0 and 4.2% for Posttest 1; between 2.6 and 4.0% for Posttest 2, respectively) compared to the M0 condition. However, for both Posttests, the interaction group × practice was not significant [Posttest 1: F(3, 39) = 0.27, p = 0.85,
Posttest 2: F(3, 31) = 0.57, p = 0.64,
]. No significant group effect was found, neither for [Posttest 1, F(3, 39) = 0.004, p = 1.00,
nor for Posttest 2, F(3, 31) = 1.68, p = 0.192,
]. At Posttest 2 the M0 group shows a somewhat greater improvement compared to the groups with mental training proportions (M25, M50, M75). However, the group × measuring time interaction was not significant, F(3, 31) = 1.63, p = 0.202,
Figure 4. Bar graphs comparing relative changes of isometric maximum force from pretest to Posttest 1. The values shown are expressed as mean ± SE. Data from the different exercises are pooled within each experimental group (M0, M25, M50, M75). Note: one half of each group trained single-joint exercises (calf raise, triceps extension); the other half, multijoint exercises (leg press, bench press). Non-practiced exercises (multijoint or single-joint exercises) were taken as a control condition.
Figure 5. Bar graphs comparing relative changes of isometric maximum force from pretest to Posttest 2. The values shown are expressed as mean ± SE. Data from the different exercises are pooled within each experimental group (M0, M25, M50, M75). Note: one half of each group trained single-joint exercises (calf raise, triceps extension); the other half, multijoint exercises (leg press, bench press). Non-practiced exercises (multijoint or single-joint exercises) were taken as a control condition.
To test whether the training effects were influenced by the initial strength (training level at the start of the IMC/MVC sessions), correlations between the absolute MVC values at Pretest and percentage strength gains at Posttest 1 were computed for each of the four exercises. There are consistently negative correlations between the Pretest MVC values and percentage strength gains. This means that the strength gains were as higher the lower initial strength level was. However, only for the calf raise a moderate and significant correlation with r = −0.61 (p = 0.005, n = 20) was found. For all other exercises non-significant correlations ranged from r = −0.15 to r = −0.28.
Expressed in SE (Figures 4 and 5), strength gains as a result of IMC training differed considerably between subjects, indicating a need to search for other, not yet systematically examined factors. Therefore, a supplementary data analysis was conducted to explore further variables influencing IMC effects. First, it was analyzed whether gender impacted on strength gains. However, summary data on all groups with mental training rates (M25, M50, M75), MVC increased almost equally in both females (3.6%) and males (3.5%), indicating that IMC effects were not influenced by gender, t(32) = 0.013, p = 0.99. Second, we examined whether strength gains were influenced by vividness of motor imagery. At the end of each session, subjects were asked to give a global evaluation of imagery during the IMC session. Individual mean ratings ranged from 3.0 to 4.3 (M = 3.6, SD = 0.4). The correlation between strength gains and vividness of imagery was r = 0.30 (p = 0.041, n = 34) in Posttest 1 and r = 0.40 (p = 0.019, n = 27) in Posttest 2. The finding that IMC effects were moderately affected by subjects’ self-reported vividness of mental imagery was confirmed by comparing subjects with different vividness of imagery. A median split of vividness of imagery values was used to divide subjects of mental training groups M25, M50, and M75 into good and excellent IMC performers. As depicted in Figure 6 better overall strength improvement was found for excellent imagers compared to good imagers. A t-test for strength gains revealed a significant difference in strength gains for groups with different imagery ability, t(32) = 1.75, p = 0.045.
Figure 6. Strength gains for good vs. excellent IMC imagers. Data from the mental imagery groups (M25, M50, M75) are pooled. The values shown (relative changes of isometric maximum force from pretest to Posttest 1) are expressed as mean ± SE.
In competitive sports, particularly sports with weight categories, training often aims to increase maximal voluntary strength without increasing body mass. As a consequence, heavy resistance “neural” sessions and also plyometric exercises are typically applied with the aim of optimizing the neural activation of the muscles. This leads to some increase even in the maximal force per cross-sectional area of the muscle, and it has been linked to a centrally represented learning effect observed not only in highly trained athletes but also in recreationally trained and untrained persons (Saltin and Gollnick, 1983). We examined training regimes that implied different ratios of MVC and IMC with the aim to assess the centrally induced training effects of strength training.
We preferred isometric strength training in this study for two reasons: first, maximal contractions produces strong increases in the maximal voluntary neural contraction of the muscles, whereas muscular hypertrophy of trained muscles remain insignificant. Second, relevant training variables can be determined and controlled very well in isometric strength training conditions, yielding high internal validity of the study. Due to this reason, and taking into account test specificity effects, isometric strength testing was assessed to evaluate IMC strength gains.
A comparison of the groups immediately after the training intervention (Posttest 1) underlines the expectation that considerable maximum strength gains can be achieved with a combination of “high-intensity” mental and physical strength training units. Different rates (75, 50, 25%) of IMC lead to nearly the same improvements as physical strength training alone. If neuromuscular training units are indicated, physical training units can be replaced by mental training units without any significant performance reduction. This holds for short-term effects as revealed by findings on the posttest immediately after the IMC training sessions. The results underline the concept of a functional equivalence between motor imagery and motor performance (Lotze and Halsband, 2006; Munzert and Zentgraf, 2009; Munzert et al., 2009). Considering that early strength gains, that is, improvements within the first 3–4 weeks, are based on neural adaptations (Sale, 1992; Enoka, 1997; Chilibeck et al., 1998; Akima et al., 1999), it can be concluded that – at least for athletes at the recreational level – IMC training leads to comparable adaptations. Further investigations will have to consider whether IMC training represent a reasonable (supplementary) method to improve muscle strength in highly trained athletes, too. In this context, it will also be necessary to investigate whether IMC training is adequate when executing dynamic strength exercises.
Since a cessation of heavy resistance training has been shown to enhance strength during the very first week after detraining (Schlumberger and Schmidtbleicher, 1998), IMC effects were measured again after a 1 week rest period without any strength training. Compared with the strength gain in the 100% physical training group (M0) at Posttest 2, strength effects are slightly smaller in the IMC groups. However, the IMC strength gains achieved in Posttest 1 also remain stable 1 week later in Posttest 2. Actually, it is not unlikely that a small delayed effect on maximal force in the M0 group – the group with the highest volumes of (real) resistance training preceding detraining – was observed, which may caused predominantly by (additional) adaptations at the muscular level. One may argue that peripheral adaptations (hypertrophy) could contribute after 6 or 7 weeks of training at the earliest (our intervention lasted 4-weeks). However, recent studies show that an increased muscle protein synthesis during the post load phase can be observed already after one single strength training unit, from which strength gains could theoretically result (Phillips, 2000).
The IMC effect sizes in our study are smaller compared with other IMC studies (see Table 1). In order to meet the specific requirements of a performance-oriented training, an established model of periodization with a prior 4-week standardized training program was administered to all subjects. This leads to higher pretest levels in our study, supporting the plausibility of smaller strength gains compared with other IMC studies. In exercises of the upper extremities our IMC groups achieved a gain of approximately 6%. A similar increase in strength is also reported by Reiser (2005). The marginal strength increase in the control conditions shows that subjects are familiar with the test situation, and that observed strength gains in the IMC conditions are therefore the result of the specific treatment. Again in this context, we would like to emphasize that subjects were very well familiarized with the strength training exercises before they started with the actual IMC intervention. It is therefore plausible to assume that significant adaptations also in neural function resulted from the 4-week strength training preceding the IMC training, which should markedly reduce the probability of further “non-specific” (not IMC-induced) neural adaptations.
Finally, we shall discuss two outcomes related to the effect sizes of our IMC procedures that have both methodological and practical implications. First, as noted above, there is a considerable variability in strength gains within the groups. In the present study, we examined four sport-related strength exercises that differed in terms of extremity and complexity. Although strength gains of the individual exercises following the IMC/MVC interventions did not differ in our study, it cannot be ruled out, that IMC training effects are influenced by specific task demands. Lebon et al. (2010), for example, found an large IMC effect on the MVC and on the maximum number of repetitions for the leg press but no effects for the bench press, when mental imagery was implemented supplementarily during the rest periods to the physical training. Additionally, from a more applied perspective, it is well known that subjects adapt differently to strength training interventions. Individual physiological responses to physical loads may be one reason for such differences.
However, IMC effects may also be influenced by individual differences in vividness of imagery. A supplementary data analysis revealed a moderate positive correlation between subjects’ subjective reports of vividness of motor imagery and strength gains. It has to be considered that imagery abilities do not differ greatly between participants. Therefore, correlations seem to underestimate the underlying connection between both variables. A median split of participants into good and excellent imagers revealed that excellent imagers showed better overall improvements of strength in IMC conditions. To our knowledge, this is the first time that a relationship between imagery ability and strength gains following IMC has been found. A relation between imagery abilities and motor learning processes in a more general sense have been stated for theoretical reasons (Munroe et al., 2000). However, only a few studies examined this relation empirically (Goss et al., 1986; Rodgers et al., 1991). Vividness of imagery reflects the richness of the evoked representation in working memory (Baddeley and Andrade, 2000; Munzert and Zentgraf, 2009) and is correlated with differential neural activation in motor-related brain areas (Guillot et al., 2008; Lorey et al., 2011). Therefore, subjective ratings of the vividness of imagery may reflect how good the internal simulation of maximum isometric contraction worked.
Second, compared with other IMC studies, the total duration of the imagined muscle contractions is rather small (see Table 1). This is due to the methodological decision to compare real and imagined contractions of the same duration. Whereas the number of maximal physical contractions should not increase considerably because of the accompanying acute fatigue in the neuromuscular system (Güllich and Schmidtbleicher, 1999), the number of imagined contractions during comparable training sessions can be enhanced significantly. Thus, it is worth mentioning that the strongest strength improvements have been found in studies examining long total IMC durations. Therefore, it can be expected that larger IMC strength gains can be achieved quite simply by prolonging IMC duration.
Naturally, strength training cannot be carried out without highly intensive real effort. However, high-intensity isometric strength training sessions can be partly replaced by IMC training sessions without any considerable reduction in strength gains. This holds at least for athletes at the recreational level. Moreover, we have shown that IMC training is also effective after a prior 4-week physical strength training phase. Our findings support the assumption that IMC training is an adequate supplementary method for improving muscle strength. This type of training may be advantageous for situations aiming to optimize the neural activation of the exercised muscles. In order to test the generalizability of the IMC effect future studies need to address training modalities that more closely represent actual training practice (e.g., dynamic strength training) and hence have a greater transfer to athletic or other skilled movement.
The authors declare that the research was conducted in the absence of any commercial or financial relationships that could be construed as a potential conflict of interest.
This research was supported by a grant from the Federal Institute of Sport Sciences, Germany (VF 07/05/11/2005).
The authors declare that all experiments performed in the study do comply with the current laws of Germany.
Akima, H., Takahashi, H., Kuno, S. Y., Masuda, K., Masuda, T., Shimojo, H., Anno, I., Itai, Y., and Katsuta, S. (1999). Early phase adaptations of muscle use and strength to isokinetic training. Med. Sci. Sports Exerc. 31, 588–594.
Baddeley, A., and Andrade, J. (2000). Working memory and the vividness of imagery. J. Exp. Psychol. Gen. 129, 126–145.
Carroll, T. J., Herbert, R. D., Munn, J., Lee, M., and Gandevia, S. C. (2006). Contralateral effects of unilateral strength training: evidence and possible mechanisms. J. Appl. Physiol. 101, 1514–1522.
Chilibeck, P. D., Calder, A. W., Sale, D. G., and Webber, C. E. (1998). A comparison of strength and muscle mass during resistance training in young women. Eur. J. Appl. Physiol. 77, 170–175.
Dai, T. H., Liu, J. Z., Sahgal, V., Brown, R. W., and Yue, G. H. (2001). Relationship between muscle output and functional MRI-measured brain activation. Exp. Brain Res. 140, 290–300.
Deschenes, M. R., Giles, J. A., McCoy, R. W., Volek, J. S., Gomez, A. L., and Kraemer, W. J. (2002). Neural factors account for strength decrements observed after short-term muscle unloading. Am. J. Physiol. Regul. Integr. Comp. Physiol. 282, R578–R583.
Driskell, J. E., Copper, C., and Moran, A. (1994). Does mental practice enhance performance? J. Appl. Psychol. 79, 481–492.
Feltz, D. L., and Landers, D. M. (1983). The effects of mental practice on motor skill learning and performance: a meta-analysis. J. Sport Psychol. 5, 25–57.
Féry, Y.-A. (2003). Differentiating visual and kinesthetic imagery in mental practice. Can. J. Exp. Psychol. 57, 1–10.
Fleck, S. J., and Kraemer, W. J. (2004). Designing Resistance Training Programs. Champaign, IL: Human Kinetics.
Goss, S., Hall, C., and Buckolz, E. (1986). Imagery ability and the acquisition and retention of movements. Mem. Cogn. 14, 469–477.
Guillot, A., Collet, C., Nguyen, V., Malouin, F., Richards, C., and Doyon, J. (2008). Functional neuroanatomical networks associated with expertise in motor imagery. Neuroimage 41, 1471–1483.
Güllich, A., and Schmidtbleicher, D. (1999). Struktur der Kraftfähigkeiten und ihrer Trainingsmethoden [Structure of motor strength and the training methods]. Dtsch. Z. Sportmed. 50, 223–234.
Hall, C. R., and Martin, K. E. (1997). Measuring movement imagery abilities: a revision of the movement imagery questionnaire. J. Ment. Imagery 21, 143–154.
Herbert, D., and Gandevia, S. C. (1998). Effects of real and imagined training on voluntary muscle activation during maximal isometric contractions. Acta Physiol. Scand. 163, 361–369.
Hortobáyi, T., Dempsey, D., Fraser, D., Zheng, D., Hamilton, G., Lambert, J., and Dohm, L. (2000). Changes in muscle strength, muscle fibre size and myofibrillar gene expression after immobilization and retraining in humans. J. Physiol. (Lond.) 524, 293–304.
Jeannerod, M. (2001). Neural simulation of action: a unifying mechanism for motor cognition. Neuroimage 14, 103–109.
Lafleur, M. F., Jackson, P. L., Malouin, F., Richards, C. L., Evans, A. C., and Doyon, J. (2002). Motor learning produces parallel dynamic functional changes during the execution and imagination of sequential foot movements. Neuroimage 16, 142–157.
Lebon, F., Collet, C., and Guillot, A. (2010). Benefits of motor imagery training on muscle strength. J. Strength Cond. Res. 24, 1680–1687.
Lee, M., and Carroll, T. J. (2007). Cross education. Possible mechanisms for the contralateral effects of unilateral resistance training. Sports Med. 37, 1–14.
Lotze, M., Montoya, P., Erb, M., Hülsmann, E., Flor, H., Klose, U., Birbaumer, N., and Grodd, W. (1999). Activation of cortical and cerebellar motor areas during executed and imagined hand movements:an fmri study. J. Cogn. Neurosci. 11, 491–501.
Mulder, E. R., Stegeman, D. F., Gerrits, K. H., Paalman, M. I., Rittweger, J., Felsenberg, D., and de Haan, A. (2006). Strength, size and activation of knee extensors followed during 8 weeks of horizontal bed rest and the influence of a countermeasure. Eur. J. Appl. Physiol. 97, 706–715.
Munroe, K., Giacobbi, J., Peter, R., Hall, C., and Weinberg, R. (2000). The four ws of imagery use: where, when, why, and what. Sport Psychol. 14, 119–137.
Munzert, J., Lorey, B., and Zentgraf, K. (2009). Cognitive motor process: the role of motor imagery in the study of motor representations. Brain Res. Rev. 60, 306–326.
Munzert, J., and Zentgraf, K. (2009). Motor imagery and its implications for understanding the motor system. Prog. Brain Res. 174, 219–229.
Munzert, J., Zentgraf, K., Stark, R., and Vaitl, D. (2008). Neural activation in cognitive motor processes: comparing motor imagery and observation of gymnastic movements. Exp. Brain Res. 188, 437–444.
Newsom, J., Knight, P., and Balnave, R. (2003). Use of mental imagery to limit strength loss after immobilization. J. Sport. Rehabil. 12, 249–258.
Phillips, S. M. (2000). When do repeated bouts of resistance exercise become training? Can. J. Appl. Physiol. 25, 185–193.
Ranganathan, V. K., Kuykendall, T., Siemionow, V., and Yue, G. H. (2002). Level of mental effort determines training-induced strength increases. Abstr. Soc. Neurosci. 32, 768.3.
Ranganathan, V. K., Siemionow, V., Liu, J. Z., Sahgal, V., and Yue, G. H. (2004). From mental power to muscle power – gaining strength by using the mind. Neuropsychologia 42, 944–956.
Reiser, M. (2005). Kraftgewinne durch Vorstellung maximaler Muskelkontraktionen [Strength gains by motor imagery of maximal muscle contractions]. Z. Sportpsychologie 12, 11–21.
Rodgers, W., Hall, C., and Buckolz, E. (1991). The effects of an imagery training program on imagery ability, imagery use and figure skating performance. J. Appl. Sport Psychol. 3, 109–125.
Romero, D. H., Lacourse, M. G., Lawrence, K. R., Schandler, S., and Cohen, M. J. (2000). Event-related potentials as a function of movement parameter variations during motor imagery and isometric action. Behav. Brain Res. 117, 83–96.
Lorey, B., Pilgramm, S., Bischoff, M., Stark, R., Vaitl, D., Kindermann, S., Munzert, J., and Zentgraf, K. (2011). Activation of the parieto-premotor network is associated with vivid motor imagery – a parametric fMRI study. PLoS ONE 6, e20368.
Rutherford, O. M., and Jones, D. A. (1986). The role of learning and coordination in strength training. Eur. J. Appl. Physiol. 55, 100–105.
Sale, D. G. (1992). “Neural adaptations to strength training,” in Strength and Power in Sport, ed. P. V. Komi (Oxford: Blackwell Scientific Publications), 249–265.
Saltin, B., and Gollnick, P. D. (1983). “Skeletal muscle adaptability: significance for metabolism and performance,” in Skeletal Muscle. Handbook of Physiology, ed. L. D. Peachey (Bethesda, MD: American Physiological Society), 555–631.
Schlumberger, A., and Schmidtbleicher, D. (1998). Zeitlich verzögerte Effekte beim Krafttraining [Time-delayed effects of strength training]. Leistungssport 28, 33–38.
Siemionow, V., Yue, G. H., Ranganathan, V. K., Liu, J. Z., and Sahgal, V. (2000). Relationship between motor activity-related cortical potential and voluntary muscle activation. Exp. Brain Res. 133, 303–311.
Sirigu, A., and Duhamel, J. R. (2001). Motor and visual imagery as two complementary but neurally dissociable mental processes. J. Cogn. Neurosci. 13, 910–919.
Smith, D., Collins, D., and Holmes, P. (2003). Impact and mechanism of mental practice effects on strength. Int. J. Sport Exercise Psychol. 1, 293–306.
Yue, G., and Cole, K. J. (1992). Strength increases from the motor program: comparison of training with maximal voluntary and imagined muscle contractions. J. Neurophysiol. 67, 1114–1123.
Keywords: motor imagery, mental training, maximum voluntary contraction, strength training
Citation: Reiser M, Büsch D and Munzert J (2011) Strength gains by motor imagery with different ratios of physical to mental practice. Front. Psychology 2:194. doi: 10.3389/fpsyg.2011.00194
Received: 11 February 2011;
Accepted: 01 August 2011;
Published online: 19 August 2011.
Edited by:
Jared Porter, Southern Illinois University, USAReviewed by:
Will Wu, California State University, USACopyright: © 2011 Reiser, Büsch and Munzert. This is an open-access article subject to a non-exclusive license between the authors and Frontiers Media SA, which permits use, distribution and reproduction in other forums, provided the original authors and source are credited and other Frontiers conditions are complied with.
*Correspondence: Mathias Reiser, Institute of Sport Science, Justus Liebig University Giessen, Kugelberg 62, 35394 Giessen, Germany. e-mail:bWF0aGlhcy5yZWlzZXJAc3BvcnQudW5pLWdpZXNzZW4uZGU=
Disclaimer: All claims expressed in this article are solely those of the authors and do not necessarily represent those of their affiliated organizations, or those of the publisher, the editors and the reviewers. Any product that may be evaluated in this article or claim that may be made by its manufacturer is not guaranteed or endorsed by the publisher.
Research integrity at Frontiers
Learn more about the work of our research integrity team to safeguard the quality of each article we publish.