- 1 Women and Children’s Health and Well-Being Project, Department of Psychiatry and Human Behavior, University of California Irvine, Orange, CA, USA
- 2 Department of Pediatrics, University of California Irvine, Orange, CA, USA
- 3 Department of Radiological Sciences, University of California Irvine, Irvine, CA, USA
- 4 Center for Functional Onco-Imaging, University of California Irvine, Irvine, CA, USA
- 5 Department of Obstetrics and Gynecology, University of California Irvine, Orange, CA, USA
- 6 Department of Obstetrics and Gynecology, Cedars Sinai Medical Center, Los Angeles, CA, USA
Disruptions to brain development associated with shortened gestation place individuals at risk for the development of behavioral and psychological dysfunction throughout the lifespan. The purpose of the present study was to determine if the benefit for brain development conferred by increased gestational length exists on a continuum across the gestational age spectrum among healthy children with a stable neonatal course. Neurodevelopment was evaluated with structural magnetic resonance imaging in 100 healthy right-handed 6- to 10-year-old children born between 28 and 41 gestational weeks with a stable neonatal course. Data indicate that a longer gestational period confers an advantage for neurodevelopment. Longer duration of gestation was associated with region-specific increases in gray matter density. Further, the benefit of longer gestation for brain development was present even when only children born full term were considered. These findings demonstrate that even modest decreases in the duration of gestation can exert profound and lasting effects on neurodevelopment for both term and preterm infants and may contribute to long-term risk for health and disease.
Introduction
The prenatal period is a time of rapid change during which fetal organs and organ systems are forming and are susceptible to both organizing and disorganizing influences. These influences on the fetus have been described as programming; the process by which a stimulus or insult during a vulnerable developmental period has a long-lasting or permanent effect (Nathanielsz, 1999). Prenatal events that regulate length of gestation and risk for preterm birth similarly shape the trajectory of fetal development. The rapid pace of fetal brain development renders it especially vulnerable to such exposures. Disruptions to brain development increase the risk for both psychopathology and cognitive delays (Wright et al., 2000; Shaw et al., 2007; Kyriakopoulos and Frangou, 2009). Although it is widely recognized that medically compromised infants born at very early gestational ages experience pervasive insults to the developing brain (Nosarti et al., 2000; Peterson et al., 2003; Delobel-Ayoub et al., 2009), healthy late preterm and early term infants typically are viewed as low risk and rarely considered for developmental follow-up. This issue was highlighted in recent investigations documenting both increased morbidity and mortality during the neonatal period (Tita et al., 2009; Zhang and Kramer, 2009) and decreased cognitive abilities in childhood (Yang et al., 2010) among full term infants delivered prior to 39 gestational weeks. Further, there is evidence that late preterm infants (i.e., those born after 34 gestational weeks) are more likely to experience gray matter injury (Billiards et al., 2006) and more likely to display cognitive and behavioral deficits during infancy and childhood compared with children born full term (McCarton et al., 1996; De Haan et al., 2000; Petrini et al., 2009; van Baar et al., 2009). This evidence suggests that the relation between gestational age at birth and brain development is present across the gestational age spectrum and not confined solely to very early preterm infants.
Disruptions to brain development associated with shortened gestation place individuals at risk for the development of behavioral and psychological dysfunction throughout the lifespan (Schothorst et al., 2007; Gozzo et al., 2009; Janssens et al., 2009). Individuals who are born early have an increased risk for the development of various psychopathologies including attention deficit hyperactivity disorder and affective disorders (Aarnoudse-Moens et al., 2009; Johnson et al., 2010). The purpose of the present study was to determine if the benefit for brain development conferred by increased gestational length exists on a continuum across the gestational age spectrum. Specifically, the association was investigated between gestational age at birth and brain development among 100, 6- to 10-year-old children who were born between 28 and 41 gestational weeks and had a stable neonatal course.
Materials and Methods
Participants
Participants included 100 right-handed children (49 girls, 51 boys) between the ages of 6 and 10 years (mean = 8.1 ± 1.1) who were born at one of two hospitals in the greater Los Angeles area (UC Irvine Medical Center, or Long Beach Memorial Medical Center) and were recruited from ongoing protocols of development. Children born at term (n = 67) and preterm (n = 33) were included to permit evaluation of a range of gestational lengths. Children were eligible for participation if they were between 6 and 10 years of age, right-handed (determined using a modified version of the Edinburgh Handedness Inventory (Oldfield, 1971), singletons, born at greater than 28 weeks gestational age (determined based on current ACOG guidelines, 2009). Our low risk sample comprised children with a stable neonatal course (Median Apgar score = 9, range 7–10) and without known congenital, chromosomal, or genetic anomalies (e.g., trisomy 21), neonatal illness (e.g., respiratory distress, mechanical ventilation over 48 hours or sepsis). Participants had no evidence of intraventricular hemorrhage (determined by ultrasound), periventricular leukomalacia, and/or low-pressure ventriculomegaly in the newborn period and normal neurologic findings, including normal ventricle size, at assessment. Further, at 6–10 years of age, no emotional or physical problems were reported in a structured interview using the MacArthur Health and Behavior Questionnaire (Armstrong and Goldstein, 2003). Parents and children gave informed consent for all aspects of the protocol, which was approved by the Institutional Review Board for protection of human subjects.
Background Information
Table 1 displays birth outcome, demographic, and neurodevelopmental characteristics of this sample. Gestational age at birth was not significantly related to birth weight percentile. Nor was it significantly associated with maternal age, maternal education, maternal IQ (determined with the Perceptual Reasoning Scale of the Wechsler Adult Intelligence Scale), household income, child race/ethnicity, or child birth order at the time of the study visit (all ps > 0.21).
MRI Acquisition
Structural MRI scans were acquired on a 3-T Philips Achieva system. To minimize head motion, padding was placed around the head. Ear protection was given to all children. To further increase compliance and reduce motion, children were fitted with headphones and allowed to watch the movie of their choice while in the scanner. Following the scanner calibration and pilot scans, a high resolution T1 anatomical scan was acquired in the sagittal plane with 1 mm3 isotropic voxel dimensions. An inversion-recovery spoiled gradient recalled acquisition (IR-SPGR) sequence with the following parameters was applied: Repetition rate (TR) = 11 ms, Echo time (TE) = 3.3 ms, Inversion time (TI) = 1100 ms, Turbo field echo factor (TFE) = 192, Number of slices: 150, no SENSE acceleration, Flip angle = 18°, Shot interval (time from inversion pulse to the center of acquisition) = 2200 ms. Acquisition time for this protocol was 7 min.
Processing of MRI Data
All processing and analysis of MRI data was completed using SPM5 software1 (SPM5 The Wellcome Department of Imaging Neuroscience, University College London). The structural images were bias field corrected, and segmented using an integrated generative model (unified segmentation, Ashburner and Friston, 2005) Unified segmentation involves alternating between segmentation, bias field correction, and normalization to obtain local optimal solutions for each process. The pediatric CCHMC a priori templates (Wilke et al., 2002) were used to segment and normalize (affine and 16 iteration non-linear transformations) the children’s images. The resulting images were modulated to correct voxel signal intensities for the amount of volume displacement during normalization. The normalized and segmented images were averaged across the children’s datasets to produce gray matter, white matter, and CSF sample specific a priori templates. The process was then repeated using the sample specific a priori templates resulting in subject specific deformation maps for VBM analysis of 1-mm isotropic voxels. The normalized, segmented, and modulated images were then smoothed using a 12-mm kernel to ensure that the data were normally distributed and to limit the number of false positive findings (Salmond et al., 2002). Coordinates of the most significant voxel within each cluster were converted from original Montreal Neurological Institute (MNI) coordinates to those of the Talairach brain atlas (Talairach and Tournoux, 1988) using the mni2tal utility (GPL)2. Anatomical locations of the significant areas are based on the best estimate from the Talairach atlas using the Talairach Daemon Client3.
Total Gray Matter Analysis
Total gray matter volume for each child was estimated based on the volumes of the segmented and modulated images. The number of non-zero voxels in the image was multiplied by the voxel values to obtain an estimate of total gray matter volume. Partial correlation analyses were performed to test the association between gestational age at birth and total gray matter volume controlling for age at assessment. Gestational age at birth was not correlated with total gray matter volume [r(100) = 0.15, p = 0.13].
Voxel-Based Morphometry Analysis
Voxel-based morphometry (VBM) is an automated structural analysis used to detect regional morphologic differences in brain images obtained by MRI. Unlike volumetric tracing methods, VBM does not depend on user-defined brain regions, but rather presents a comprehensive localization of gray matter density differences between groups (Ashburner and Friston, 2000). The relation between gestational age at birth, measured continuously, and regional gray matter density was determined using a multiple regression with gestational age at birth as the predictor of interest and child age and total gray matter volume as covariates. Consequently, the analysis detected regional differences rather than overall, large-scale variations in gray matter. Relative threshold masking (threshold > 0.3) was used to minimize gray–white matter boundary effects, and implicit masking was used to disregard voxels with 0 values. The statistics for VBM analyses were normalized to Z score. The threshold for the statistical parametric maps was p < 0.001. Clusters were considered significant if they contained at least 100 voxels and remained significant after false discovery rate (FDR) correction for multiple comparisons (p < 0.05) as recommended by Genovese et al. (2002).
These analyses were applied to the entire sample to evaluate associations between gestational age at birth measured continuously and morphological differences in gray matter among children born between 28 and 41 gestational weeks. Significant clusters of voxels, where longer gestation was associated with higher gray matter density, identified in this initial analysis were used as regions of interest in subgroup analyses. For the empirically identified regions of interest, the association between gestational age at birth and gray matter density was determined separately among the children born at term 37–41 gestational weeks and preterm 28–36 gestational weeks. Significance was set at p < 0.05 after Bonferonni correction.
Results
Is Gestational Age at Birth Associated with Morphological Changes in Regional Gray Matter?
As shown in Figure 1, VBM analyses revealed that children born at later gestational ages showed regionally specific differences in gray matter during childhood predominantly in the temporal lobes after controlling for overall brain volume. Regions with associations that achieved statistical significance after FDR correction are shown in Table 2 and Figure 2. Longer gestation was associated with significant, mostly bilateral, increases in gray matter density in several temporal regions including the superior (BA 22, 41, and 42) and middle temporal gyrus (BA 21) and extending ventrally to the inferior temporal gyrus and the occipitotemporal gyrus/fusiform gyrus (BA 37, bilaterally and BA 20, right only) and medially to the insula. Associations between greater gestational age at birth and increased gray matter density during childhood extend dorsally to the inferior and superior parietal lobule (BA 40, bilaterally and BA 7, left only). Longer gestation was additionally associated with increases in gray matter bilaterally in the cerebellum, but only significant on the left side after FDR correction. Associations between gestational age at birth and gray matter also were revealed in subcortical regions including the caudate, but these are not discussed further because they were not significant after FDR correction (p = 0.058).
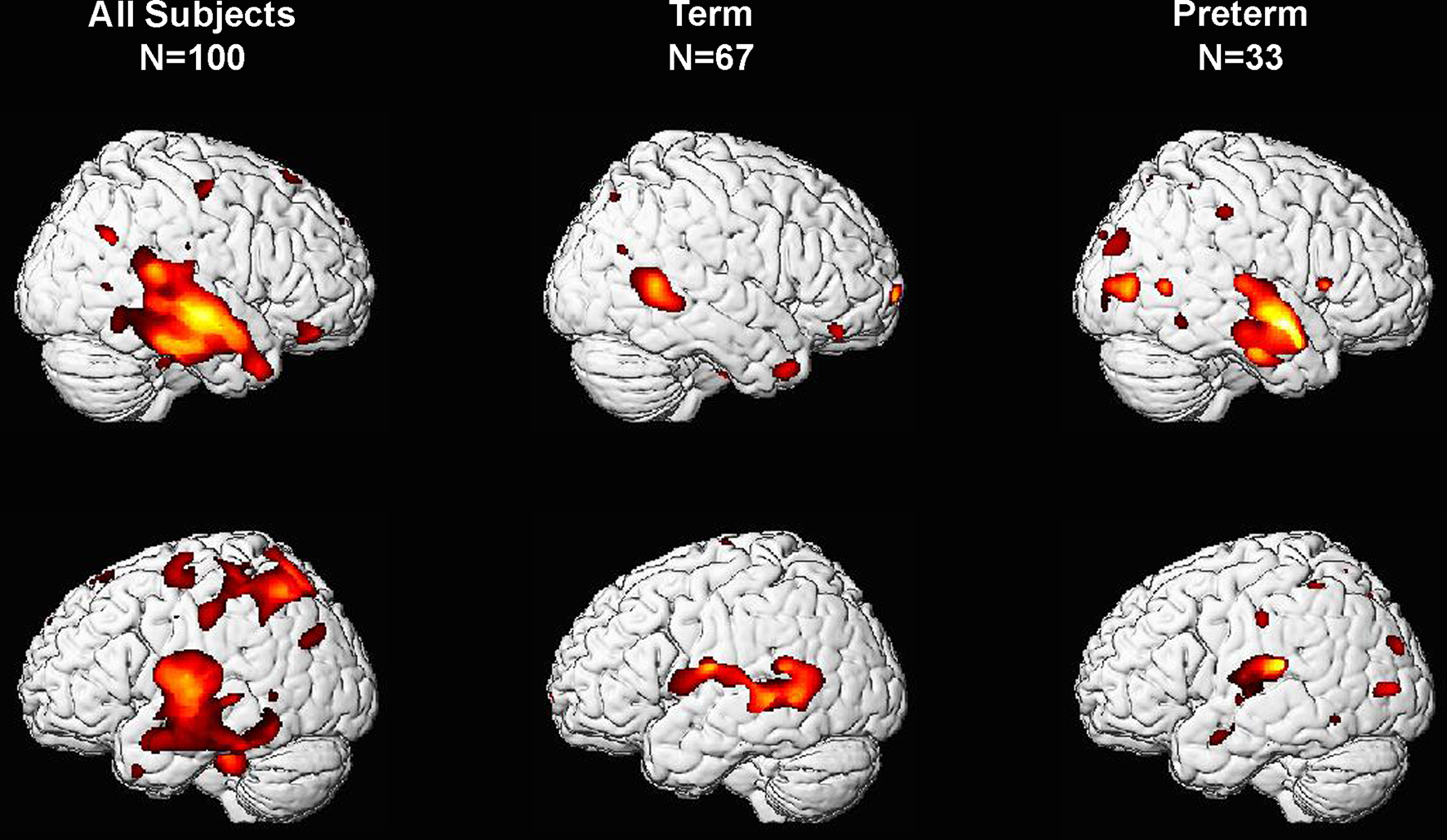
Figure 1. Three-dimensional representation of regions of increased gray matter probability associated with longer gestation for right-handed children born between 28 and 41 weeks. Column 1 presents data from the complete sample, column 2 includes only children born at term (37–41 weeks gestational age) and column 3 includes only children born preterm (28–36 weeks gestational age).
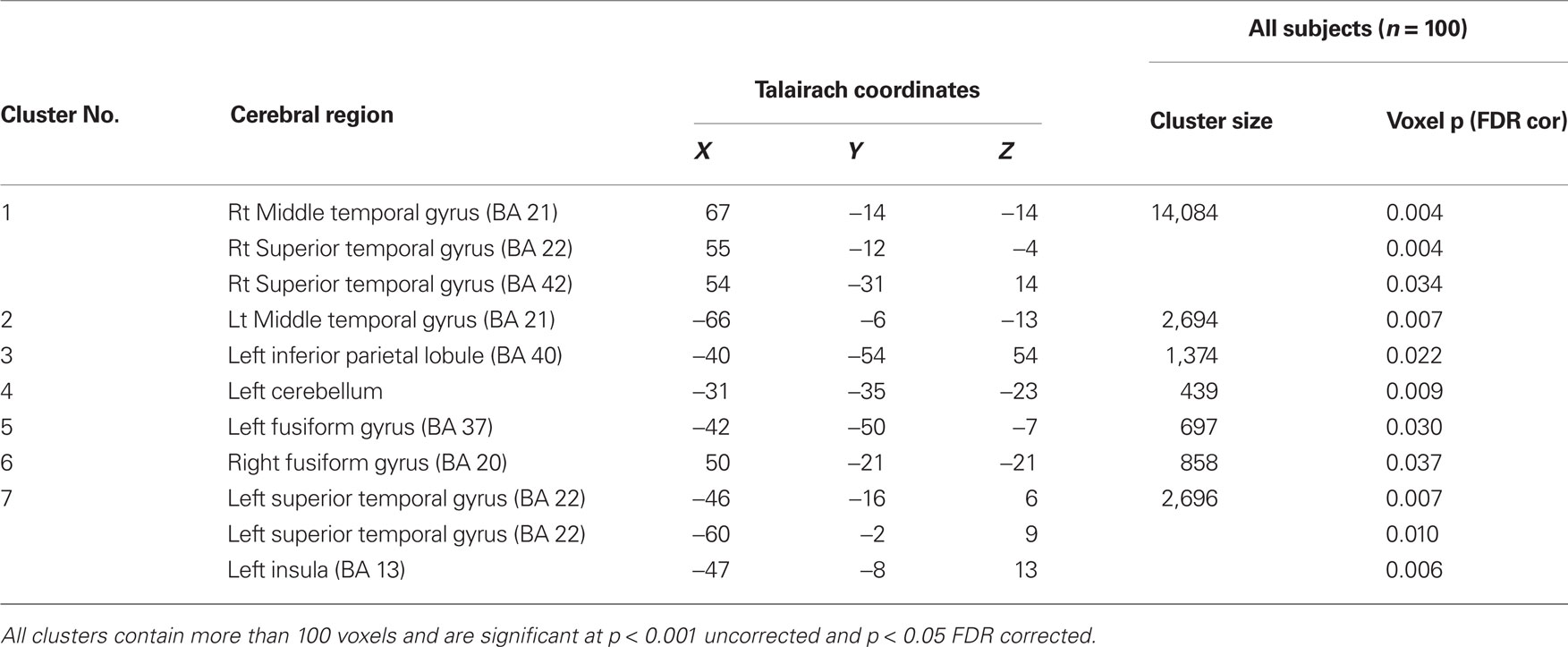
Table 2. Gestational age at birth and associations with gray matter density among right-handed 6- to 10-year-old children born between 28 and 41 gestational weeks.
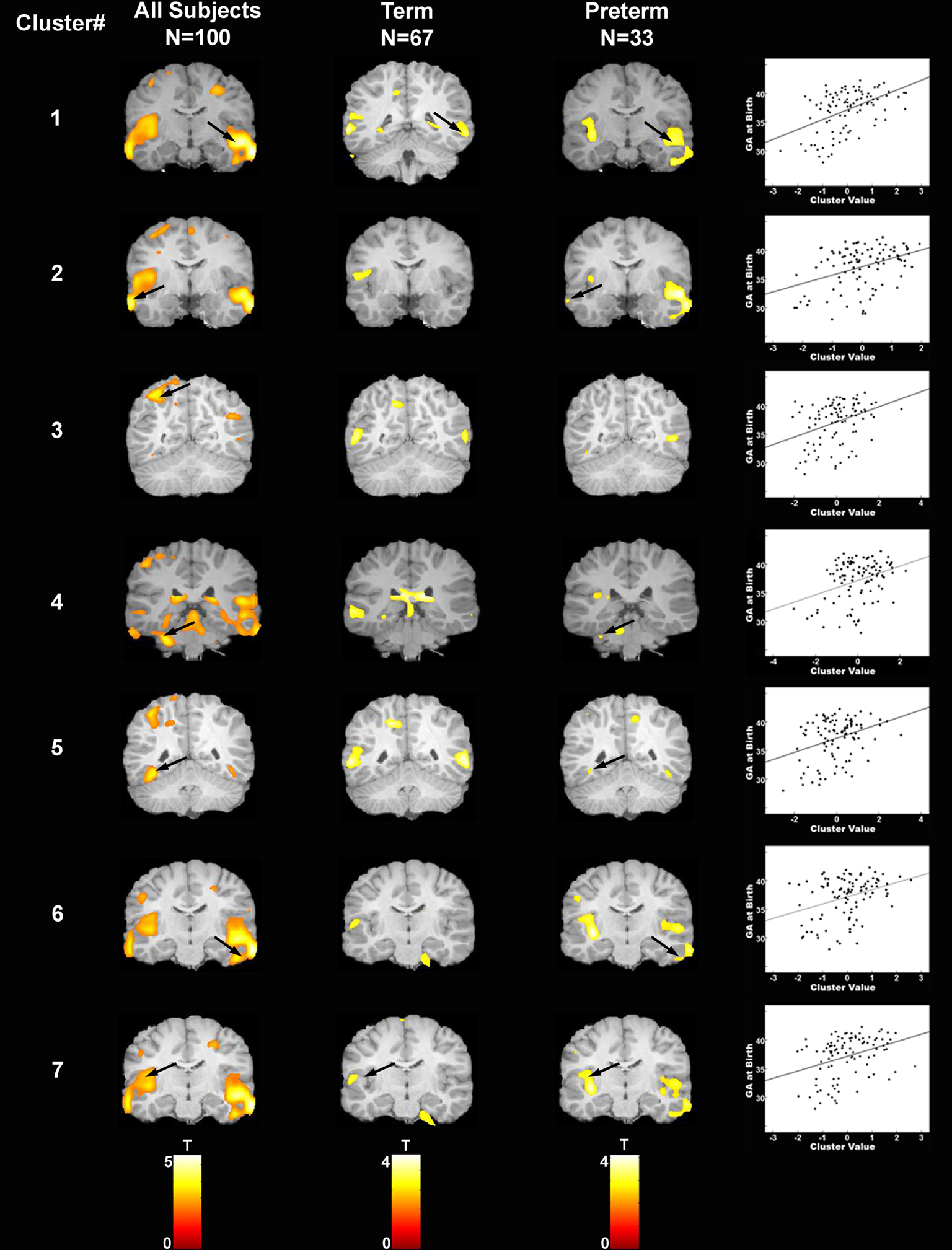
Figure 2. Areas of increased gray matter density in association with longer gestation are displayed for each of the seven regions of interest for: (i) the complete sample (column 1), (ii) children born at term (column 2), and (iii) children born preterm (column 3). Arrows indicate clusters that were significantly associated with gestational age at birth after correction for multiple comparisons. Scatter plots are presented for illustrative purposes only to demonstrate the linear nature of the association between gestational age at birth (y axis) and the cluster value (x axis) for each of the seven regions of interest. Note that children born at term and children born preterm contribute to the significant association between gestational age at birth and gray matter density in region 1. However, the area of association between gestational age at birth and gray matter density among the children born at term extends slightly more posterior to the area of significant associations among the children born preterm. For this reason, different slices are shown for the term and preterm children for this cluster only.
The scatter plots in Figure 2 illustrate the association between gestational age at birth and the cluster value for each of the seven regions of interest (calculated using SPM’s volume of interest, VOI tool; Brett et al., 2002). As indicated, associations are present across the gestational age spectrum and are not simply determined by preterm birth status. This suggests that there are regionally specific incremental increases in gray matter associated with increases in gestational length. To further evaluate this question, subgroup analyses examining the children born at term and preterm separately were performed for the identified regions of interest (Figure 2). For term infants, longer gestation within the range of 37–41 weeks was associated with significant bilateral increases in gray matter density in the superior (BA 22) and middle temporal gyrus (BA 21). Significant associations were additionally observed in the left parietal lobe (BA 7). For preterm infants significant associations between longer gestation and increased gray matter were observed bilaterally in the superior (BA 22, 41) and middle temporal gyrus (BA 21) and extending ventrally to the inferior temporal gyrus and the occipitotemporal gyrus/fusiform gyrus (BA 37, left only and BA 20, right only) and medially to the insula. Significant associations were additionally observed in the left parietal lobe (BA 40) and the left cerebellum.
Discussion
These results indicate that there are regionally specific associations between gestational length and brain morphology at 6–10 years of age. The consequences of gestational age at birth on brain development are observed both across the full gestational range in our sample and within the constricted gestational age range of infants born at term (greater than 37 weeks). Children in this cohort had a stable neonatal course, did not experience common perinatal risk factors such as mechanical ventilation, intraventricular hemorrhage, or periventricular leukomalacia. Our findings indicate that, among healthy, low risk populations, shortened gestation, even within the boundaries of normal term delivery, has persisting influences on neurological development.
Rapid changes in the development and organization of the fetal brain occur throughout the entire course of gestation (Bourgeois, 1997; Volpe, 2008). The associations between gestational age at birth and child neurodevelopment reported here are likely due to prenatal influences on processes that mature during the third trimester. Our findings in this normal healthy sample support the argument that prenatal experiences (e.g., exposure to maternal and placental hormones) alter the trajectory of fetal brain development (Davis and Sandman, 2010). The advantage of longer gestation for neurodevelopment is arguably a direct result of prenatal influences that benefit the developing fetal nervous system during the third trimester and these influences will need to be evaluated in future studies. During the last third of human pregnancy the fetal brain is forming secondary and tertiary gyri, and exhibiting neuronal differentiation, dendritic arborization, axonal elongation, synapse formation and collateralization, and myelination (Bourgeois, 1997; Volpe, 2008). Synapse formation during this period accelerates to a rate of approximately 40,000 synapses per minute (Bourgeois, 1997). Linear increases in total gray matter volume of 1.4% per week are seen from 29 to 41 gestational weeks (Huppi et al., 1998) and approximately 50% of the increase in cortical volume occurs between 34 and 40 gestational weeks (Adams-Chapman, 2006; Kinney, 2006). The benefits of longer gestation are most apparent in the temporal cortex, one of the latest brain regions to mature (Hill et al., 2010), where synaptogenesis and then gyrification begin and progress rapidly throughout the third trimester (van der Knaap et al., 1996; Huttenlocher and Dabholkar, 1997). Interestingly, the benefits of longer gestation for development of the temporal cortex were apparent even for children born at term (37–41 gestational weeks). The affected area extends more posterior among term as compared to preterm children; perhaps due to differences in the developmental stage at the time of delivery resulting in alterations to the trajectory of neurodevelopment. The neurological consequences of shortened gestation observed in our low risk population of healthy children follow a similar pattern described for medically compromised children born at extremely early gestational ages with a high risk of neonatal morbidity and mortality (Kesler et al., 2006). Further, these data are consistent with the recently described cognitive and behavioral deficits observed among children born during the late preterm and early term periods (Kramer, 2009; van Baar et al., 2009; Yang et al., 2010).
Affected temporal regions subserve critical cognitive processes including auditory perception and processing, comprehension of verbal language (Binder et al., 2000, 2009; Schmithorst et al., 2006; Karunanayaka et al., 2007), supramodal integration and concept retrieval (Binder et al., 2009). Evidence indicates that larger brain volume is associated with higher levels of cognitive functioning (Toga and Thompson, 2005; Sandman and Kemp, 2007). It is probable that individuals with optimal neurodevelopment in these regions will show advantages for cognitive functioning in these critical areas. Further, disruptions to neurodevelopment in these regions is associated with risk for the development of psychopathology, particularly in combination with exposures to other risk factors (Gurvits et al., 2006). Specific regions observed to be affected by shortened gestation in the present study have been associated with psychological dysfunction including psychosis (Wright et al., 2000; Honea et al., 2005) and impaired emotional processing (Kober et al., 2008; Craig, 2009). Importantly, there is evidence that alterations in morphology can be detected prior to the emergence of psychosis (Crossley et al., 2009; Goldstein et al., 2009) and may be a risk factor for the development of later psychopathology. The current findings suggest that even modest decreases in gestational length are associated with disruptions to neurodevelopment and that this is likely associated with consequences for wellbeing across the lifespan.
These novel findings demonstrate that across the spectrum of gestational ages at birth, longer gestation benefits neurodevelopment. Strengths of this study include the large sample size and the characterization of brain development among a low risk sample of children born preterm and term. However, because of the retrospective design, postnatal experiences and exposures known to influence children’s development may not have been fully assessed and it is plausible that these may moderate prenatal effects (Smith et al., 2006; Bergman et al., 2010). Notably, the pattern of associations reported here cannot be accounted for by postnatal sociodemographic influences that are known to have strong influences on children’s development including maternal education or household income because these were not associated with gestational age at birth.
The gestational age when the transition from intrauterine to extrauterine life occurs in normal healthy births can profoundly alter the course of brain development with long-lasting consequences. The significant linear association between gestational age at birth and brain development in young children challenges the commonly held assumption of a “non-linear” developmental course that defines fetal maturity as occurring at 37 gestational weeks. Our findings emphasize that there is a benefit for the developing brain of increased gestational length throughout the course of fetal development. In addition to providing new information about the importance of longer gestation beyond 37 gestational weeks for brain development, this finding has implications for medical decisions involving assisted deliveries. The decision about when to deliver a fetus, especially after 37 weeks, rarely involves concerns about the fetal nervous system. The findings reported here suggest that the neurological maturity of the fetus should enter the decision algorithm because even modest increases in gestational length have significant and long-lasting influences on the structure and function of the nervous system.
Conflict of Interest Statement
The authors declare that the research was conducted in the absence of any commercial or financial relationships that could be construed as a potential conflict of interest.
Acknowledgments
This research was supported by NIH R01 HD050662 to Elysia Poggi Davis and NIH R01 HD048947 to Curt A. Sandman. The authors wish to thank the families who participated in this project. The assistance of Megan Blair, Natalie Hernandez, Christina Canino, Christine Preslicka, and Susan Jackman is gratefully acknowledged.
Footnotes
References
Aarnoudse-Moens, C. S., Weisglas-Kuperus, N., van Goudoever, J. B., and Oosterlaan, J. (2009). Meta-analysis of neurobehavioral outcomes in very preterm and/or very low birth weight children. Pediatrics 124, 717–728.
ACOG. (2009). ACOG Practice Bulletin No. 101: ultrasonography in pregnancy. Obstet. Gynecol. 113(Pt 1), 451–461.
Adams-Chapman, I. (2006). Neurodevelopmental outcome of the late preterm infant. Clin. Perinatol. 33, 947–964.
Armstrong, J. M., and Goldstein, L. H. (2003). Manual for the MacArthur Health and Behavior Questionnaire (HBQ 1.0). Chicago: MacArthur Foundation Research Network on Psychopathology and Development Kupfer, D.J. University of Pittsburgh.
Ashburner, J., and Friston, K. J. (2000). Voxel-based morphometry – the methods. NeuroImage 11, 805–821.
Bergman, K., Sarkar, P., Glover, V., and O’Connor, T. G. (2010). Maternal prenatal cortisol and infant cognitive development: moderation by infant-mother attachment. Biol. Psychiatry 67, 1026–1032.
Billiards, S. S., Pierson, C. R., Haynes, R. L., Folkerth, R. D., and Kinney, H. C. (2006). Is the late preterm infant more vulnerable to gray matter injury than the term infant? Clin. Perinatol. 33, 915–933; abstract x–xi.
Binder, J. R., Desai, R. H., Graves, W. W., and Conant, L. L. (2009). Where is the semantic system? A critical review and meta-analysis of 120 functional neuroimaging studies. Cereb. Cortex 19, 2767–2796.
Binder, J. R., Frost, J. A., Hammeke, T. A., Bellgowan, P. S., Springer, J. A., Kaufman, J. N., and Possing, E. T. (2000). Human temporal lobe activation by speech and nonspeech sounds. Cereb. Cortex 10, 512–528.
Bourgeois, J. P. (1997). Synaptogenesis, heterochrony and epigenesis in the mammalian neocortex. Acta Paediatr. Suppl. 422, 27–33.
Brett, M., Anton, J. L., Romain, V., and Poline, J. (2002). Region of interest analysis using an SPM toolbox (abstract). Presented at the 8th International Conference on Functional Mapping of the Human Brain. NeuroImage, Sendai, Japan.
Craig, A. D. (2009). How do you feel–now? The anterior insula and human awareness. Nat. Rev. Neurosci. 10, 59–70.
Crossley, N. A., Mechelli, A., Fusar-Poli, P., Broome, M. R., Matthiasson, P., Johns, L. C., Bramon, E., Valmaggia, L., Williams, S. C., and McGuire, P. K. (2009). Superior temporal lobe dysfunction and frontotemporal dysconnectivity in subjects at risk of psychosis and in first-episode psychosis. Hum. Brain Mapp. 30, 4129–4137.
Davis, E. P., and Sandman, C. A. (2010). The timing of prenatal exposure to maternal cortisol and psychosocial stress is associated with human infant cognitive development. Child Dev. 81, 131–148.
De Haan, M., Bauer, P. J., Georgieff, M. K., and Nelson, C.A. (2000). Explicit memory in low-risk infants aged 19 months born between 27 and 42 weeks of gestation. Dev. Med. Child Neurol. 42, 304–312.
Delobel-Ayoub, M., Arnaud, C., White-Koning, M., Casper, C., Pierrat, V., Garel, M., Burguet, A., Roze, J. C., Matis, J., Picaud, J. C., Kaminski, M., and Larroque, B. (2009). Behavioral problems and cognitive performance at 5 years of age after very preterm birth: the EPIPAGE study. Pediatrics 123, 1485–1492.
Genovese, C., Lazar, N., and Nichols, T. (2002). Thresholding of statistical maps in functional neuroimaging using the false discovery rate. NeuroImage 15, 870–878.
Goldstein, K. E., Hazlett, E. A., New, A. S., Haznedar, M. M., Newmark, R. E., Zelmanova, Y., Passarelli, V., Weinstein, S. R., Canfield, E. L., Meyerson, D. A., Tang, C. Y., Buchsbaum, M. S., and Siever, L. J. (2009). Smaller superior temporal gyrus volume specificity in schizotypal personality disorder. Schizophr. Res. 112, 14–23.
Gozzo, Y., Vohr, B., Lacadie, C., Hampson, M., Katz, K. H., Maller-Kesselman, J., Schneider, K. C., Peterson, B. S., Rajeevan, N., Makuch, R. W., Constable, R. T., and Ment, L. R. (2009). Alterations in neural connectivity in preterm children at school age. Neuroimage 48, 458–463.
Gurvits, T. V., Metzger, L. J., Lasko, N. B., Cannistraro, P. A., Tarhan, A. S., Gilbertson, M. W., Orr, S. P., Charbonneau, A. M., Wedig, M. M., and Pitman, R. K., (2006). Subtle neurologic compromise as a vulnerability factor for combat-related posttraumatic stress disorder: results of a twin study. Arch. Gen. Psychiatry 63, 571–576.
Hill, J., Inder, T., Neil, J., Dierker, D., Harwell, J., and Van Essen, D. (2010). Similar patterns of cortical expansion during human development and evolution. Proc. Natl. Acad. Sci. U.S.A. 107, 13135–13140.
Honea, R., Crow, T. J., Passingham, D., and Mackay, C. E. (2005). Regional deficits in brain volume in schizophrenia: a meta-analysis of voxel-based morphometry studies. Am. J. Psychiatry. 162, 2233–2245.
Huppi, P. S., Warfield, S., Kikinis, R., Barnes, P. D., Zientara, G. P., Jolesz, F. A., Tsuji, M. K., and Volpe, J. J. (1998). Quantitative magnetic resonance imaging of brain development in premature and mature newborns. Ann. Neurol. 43, 224–235.
Huttenlocher, P. R., and Dabholkar, A. S. (1997). Regional differences in synaptogenesis in human cerebral cortex. J. Comp. Neurol. 387, 167–178.
Janssens, A., Uvin, K., Van Impe, H., Laroche, S. M., Van Reempts, P., and Deboutte, D. (2009). Psychopathology among preterm infants using the diagnostic classification zero to three. Acta Paediatr. 98, 1988–1993.
Johnson, S., Hollis, C., Kochhar, P., Hennessy, E., Wolke, D., and Marlow, N. (2010). Psychiatric disorders in extremely preterm children: longitudinal finding at age 11 years in the EPICure study. J. Am. Acad. Child Adolesc. Psychiatry 49, 453–463e1.
Karunanayaka, P. R., Holland, S. K., Schmithorst, V. J., Solodkin, A., Chen, E. E., Szaflarski, J. P., and Plante, E. (2007). Age-related connectivity changes in fMRI data from children listening to stories. Neuroimage 34, 349–360.
Kesler, S. R., Vohr, B., Schneider, K. C., Katz, K. H., Makuch, R. W., Reiss, A. L., and Ment, L. R. (2006). Increased temporal lobe gyrification in preterm children. Neuropsychologia 44, 445–453.
Kinney, H. C. (2006). The near-term (late preterm) human brain and risk for periventricular leukomalacia: a review. Semin. Perinatol. 30, 81–88.
Kober, H., Barrett, L. F., Joseph, J., Bliss-Moreau, E., Lindquist, K., and Wager, T. D. (2008). Functional grouping and cortical-subcortical interactions in emotion: a meta-analysis of neuroimaging studies. Neuroimage 42, 998–1031.
Kramer, M. S. (2009). Late preterm birth: appreciable risks, rising incidence. J. Pediatr. 154, 159–160.
Kyriakopoulos, M., and Frangou, S. (2009). Recent diffusion tensor imaging findings in early stages of schizophrenia. Curr. Opin. Psychiatry 22, 168–176.
McCarton, C. M., Wallace, I. F., Divon, M., and Vaughan, H. G. Jr. (1996). Cognitive and neurologic development of the premature, small for gestational age infant through age 6: comparison by birth weight and gestational age. Pediatrics 98, 1167–1178.
Nathanielsz, P. W. (1999). Life in the Womb: The Origin of Health and Disease. Ithaca, NY: Promethean Press.
Nosarti, C., Al-Asadi, M. H., Frangou, S., Stewart, A. L., Rifkin, L., and Murray, R. M. (2000). Adolescents who were born very preterm have decreased brain volumes. Brain 125, 1616–1623.
Oldfield, R. C. (1971). The assessment and analysis of handedness: the Edinburgh inventory. Neuropsychologia 9, 97–113.
Peterson, B. S., Anderson, A. W., Ehrenkranz, R., Staib, L. H., Tageldin, M., Colson, E., Gore, J. C., Duncan, C. C., Makuch, R., and Ment, L. R. (2003). Regional brain volumes and their later neurodevelopmental correlates in term and preterm infants. Pediatrics 111, 939–948.
Petrini, J. R., Dias, T., McCormick, M. C., Massolo, M. L., Green, N. S., and Escobar, G. J. (2009). Increased risk of adverse neurological development for late preterm infants. J. Pediatr. 154, 169–176.
Salmond, C. H., Ashburner, J., Vargha-Khadem, F., Connelly, A., Gadian, D. G., and Friston, K. J. (2002). Distributional assumptions in voxel-based morphometry. Neuroimage 17, 1027–1030.
Sandman, C. A., and Kemp, A. S. (2007). “Neuroscience of developmental disabilities,” in Handbook of Developmental Disabilities, eds S. Odem, R. H. Horner, M. E. Snell, and J. Blacher (New York: Guilford Publications), 224–246.
Schmithorst, V. J., Holland, S. K., and Plante, E. (2006). Cognitive modules utilized for narrative comprehension in children: a functional magnetic resonance imaging study. Neuroimage 29, 254–266.
Schothorst, P. F., Swaab-Barneveld, H., and van Engeland, H. (2007). Psychiatric disorders and MND in non-handicapped preterm children. Prevalence and stability from school age into adolescence. Eur. Child Adolesc. Psychiatry 16, 439–448.
Shaw, P., Eckstrand, K., Sharp, W., Blumenthal, J., Lerch, J. P., Greenstein, D., Clasen, L., Evans, A., Giedd, J., and Rapoport, J. L. (2007). Attention-deficit/hyperactivity disorder is characterized by a delay in cortical maturation. Proc. Natl. Acad. Sci. U.S.A. 104, 19649–19654.
Smith, K. E., Landry, S. H., and Swank, P. R. (2006). The role of early maternal responsiveness in supporting school-aged cognitive development for children who vary in birth status. Pediatrics 117, 1608–1617.
Talairach, J., and Tournoux, P. (1988). Co-planar Stereotaxic at; as of the Human Brain; 3-Dimensional Proportional System: An Approach to Cerebral Imaging. New York: Thieme.
Tita, A. T., Landon, M. B., Spong, C. Y., Lai, Y., Leveno, K. J., Varner, M. W., Moawad, A. H., Caritis, S. N., Meis, P. J., Wapner, R. J., Sorokin, Y., Miodovnik, M., Carpenter, M., Peaceman, A. M., O’Sullivan, M.J., Sibai, B.M., Langer, O., Thorp, J. M., Ramin, S. M., and Mercer, B. M. (2009). Timing of elective repeat cesarean delivery at term and neonatal outcomes. N. Engl. J. Med. 360, 111–120.
Toga, A., and Thompson, P. M. (2005). Genetics of brain structure and intelligence. Annu. Rev. Neurosci. 28, 1–23.
van Baar, A. L., Vermaas, J., Knots, E., de Kleine, M. J., and Soons, P. (2009). Functioning at school age of moderately preterm children born at 32 to 36 weeks’ gestational age. Pediatrics 124, 251–257.
van der Knaap, M. S., van Wezel-Meijler, G., Barth, P. G., Barkhof, F., Ader, H. J., and Valk, J. (1996). Normal gyration and sulcation in preterm and term neonates: appearance on MR images. Radiology 200, 389–396.
Wilke, M., Schmithorst, V. J., and Holland, S. K. (2002). Assessment of spatial normalization of whole-brain magnetic resonance images in children. Hum. Brain Mapp. 17, 48–60.
Wright, I. C., Rabe-Hesketh, S., Woodruff, P. W., David, A. S., Murray, R. M., and Bullmore, E. T. (2000). Meta-analysis of regional brain volumes in schizophrenia. Am. J. Psychiatry 157, 16–25.
Yang, S., Platt, R. W., and Kramer, M. S. (2010). Variation in child cognitive ability by week of gestation among healthy term births. Am. J. Epidemiol. 171, 399–406.
Keywords: brain development, MRI, gestational length, fetal programming, full term, preterm
Citation: Davis EP, Buss C, Muftuler LT, Head K, Hasso A, Wing DA, Hobel C and Sandman CA (2011) Children’s brain development benefits from longer gestation. Front. Psychology 2:1. doi: 10.3389/fpsyg.2011.00001
Received: 28 October 2010;
Accepted: 01 January 2011;
Published online: 09 February 2011.
Edited by:
Frederic Dick, University of California at San Diego, USAReviewed by:
Christine Charvet, Cornell University, USANatacha Akshoomoff, University of California at San Diego, USA
Copyright: © 2011 Davis, Buss, Muftuler, Head, Hasso, Wing, Hobel and Sandman. This is an open-access article subject to an exclusive license agreement between the authors and Frontiers Media SA, which permits unrestricted use, distribution, and reproduction in any medium, provided the original authors and source are credited.
*Correspondence: Elysia Poggi Davis, Department of Psychiatry and Human Behavior, University of California Irvine, 333 The City Boulevard, West Suite 1200, Orange, CA 92868, USA. e-mail: edavis@uci.edu