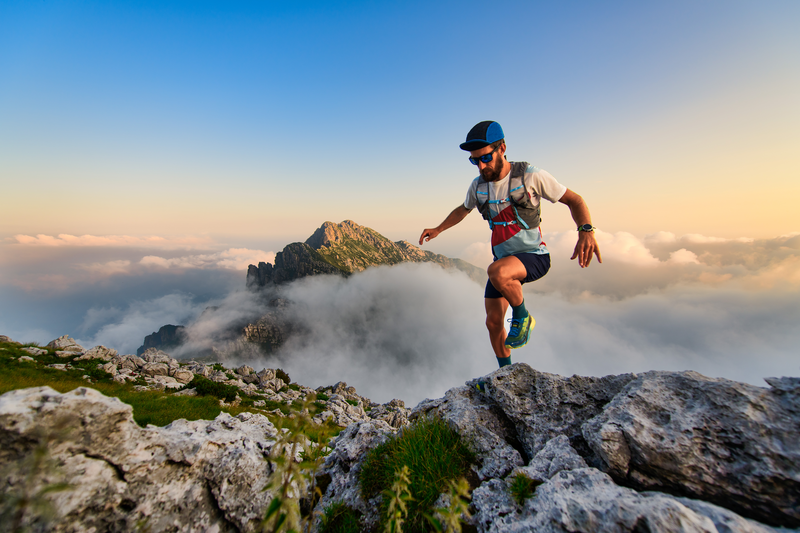
94% of researchers rate our articles as excellent or good
Learn more about the work of our research integrity team to safeguard the quality of each article we publish.
Find out more
ORIGINAL RESEARCH article
Front. Plant Sci. , 28 February 2025
Sec. Aquatic Photosynthetic Organisms
Volume 16 - 2025 | https://doi.org/10.3389/fpls.2025.1540266
This article is part of the Research Topic Harnessing the Potential of Duckweed: Biological Insights and Ecological Applications View all 3 articles
Rare earth elements (REEs) are increasingly utilized in modern technologies but are now recognized as emerging pollutants, with limited understanding of their impact on aquatic ecosystems. In this study, the effects of selected REEs (Ce, Nd, Gd, Dy, Yb, Ho, and Lu) in chloride form were evaluated on Lemna minor L., a plant species widely used as tool for the biomonitoring of the aquatic environments. Under controlled laboratory conditions, growth parameters, pigment content, oxidative stress markers, total antioxidant capacity, and antioxidant enzyme activities were assessed at millimolar concentrations over different exposure periods. L. minor exhibited tolerance to low millimolar concentrations of REEs over short-term exposure. However, prolonged exposure to high concentrations resulted in toxicity, characterized by growth inhibition, chlorophyll degradation, increased lipid peroxidation, and oxidative stress. Particularly, a hormetic response was observed for cerium, with stimulation at low concentrations and inhibition at higher levels, while dysprosium did not significantly affect growth. Other tested REEs induced varying degrees of stress, with holmium and lutetium causing the most severe toxic effects. Changes in antioxidant enzyme activities indicated a differential activation of stress responses depending on the REE type. These findings highlight the necessity for continuous monitoring of REEs in aquatic systems and support the use of L. minor as a valuable tool for environmental risk assessment.
Rare earth elements (REEs) include elements from lanthanum to lutetium that are vital for environmentally friendly energy production, electronics, and military applications (Reisman et al., 2013). Currently, REEs are globally produced at a rate of 124,000 tons per year (U.S. Geological Survey, 2016), two orders of magnitude lower than copper or aluminum (Schüler et al., 2011). However, the production of industrially relevant REEs is anticipated to increase rapidly in the coming decades (Alonso et al., 2012). Coupled with virtually no recycling of REEs (Binnemans et al., 2013) and the difficulty in replacing REEs due to their unique properties (UNCTAD, 2014), their environmental impact is unavoidable and accelerating. The European Commission considers REEs the most problematic group of raw materials, with the highest supply risk (European Commission, 2010).
With China’s decreasing exports of REEs, all other countries in the world are facing a supply risk, and, to address the problem of supply of these rare earth elements, mining companies have been active in the search for new exploitable REE deposits and some old mines have been reopened (Humphries, 2010).
Cerium (Ce) is one of the most abundant elements among REEs, displaying a wide range of human applications. Chloride and nitrate forms of Ce and lanthanum (La) are the main constituents of REE micro-fertilizers used in China since the 1970s to improve crop yield (Hu et al., 2004). In addition, the utilization of REEs in agriculture, animal husbandry, and medicine is well known (Tommasi and d’Aquino, 2017; d’Aquino and Tommasi, 2017). The presence of anthropogenic REEs in aquatic systems was first demonstrated with the release of gadolinium (Gd) contained in contrast agents used in magnetic resonance imaging (MRI), disturbing natural REE biogeochemical cycles (Bau and Dulski, 1996). There are limited data on other REEs, notably on neodymium (Nd), terbium (Tb), and heavy (H)REEs such as thulium (Tm) and ytterbium (Yb), which should thus be studied with priority, particularly light (L)REE that are more abundant and bioaccumulated than HREE (Blinova et al., 2020). Most studies evaluated the individual effects of REEs but not the combined effects of REE mixtures (Gonzalez et al., 2014), although different REEs occur together in the environment. Missing data on the toxicity of some REEs and their mixtures prevents the establishment of a consensus on the uniformity of different REEs (Gonzalez et al., 2014).
Although REEs have received increased attention recently, little is known about their effects on the aquatic biome and aquatic organisms. However, some data indicate that their presence is growing. The presence of anthropogenic REEs in aquatic systems leads to positive anomalies (Song et al., 2017), raising environmental concerns. Despite more studies being available nowadays, REE ecotoxicology is still poorly understood (Arienzo et al., 2022).
In surface waters, REE behaves as trivalent ions, except for Ce3+, which can be oxidized to Ce4+ and precipitate in solution (Cotton et al., 1999), or Europium Eu3+ which is reduced to Eu2+, increasing its solubility (Weltje et al., 2002).
Lanthanum and cerium are the most studied elements in the aquatic ecosystem. As regards toxicity, some studies report that the algae Chlorella vulgaris and Skeletonema costatum, treated with various lanthanides at millimolar concentrations, show signs of toxicity (den Doore de Jong and Roman, 1965; Tai et al., 2010). In Hyalella azteca, ecotoxicity decreases with the increasing atomic number of light lanthanides (Borgmann et al., 2005). The cause of this can be attributed to the variable composition of the culture media used in ecotoxicology and the differences related to the different REEs used. In particular, forming insoluble precipitates with phosphate and carbonate (Barry and Meehan, 2000) in some culture media probably leads to changes in the lanthanide concentration during some tests, resulting in an underestimation of their toxicity (Borgmann et al., 2005). Recent studies show adverse effects on sea urchin Paracentrotus lividus embryos after treatment with Ce4+ and La3+, with decreased mitotic activity and increased mitotic aberrations afterwards. At micromolar concentrations, there were adverse effects on embryogenesis (Oral et al., 2010). Among aquatic plants, the duckweed (Lemna minor L) is an easy-to-culture and handle vascular plant that is relatively sensitive to different pollutants, hence suitable for ecotoxicological testing (Fenske and Raymond, 2006; Moody and Miller, 2005). It is a common, relatively simple structured freshwater plant belonging to the Lemnoideae. Standard guidelines to perform a growth inhibition test on L. minor were published by the Organization for Economic Co-operation and Development (OECD, 2006). In plants, the responses to the abiotic stress induced by toxic agents with harmful overproduction of reactive oxygen species (ROS) comprise a network of antioxidant systems to counteract their toxicity. Between them, the components of the ascorbate-glutathione cycle participate in ROS-scavenging (Paradiso et al., 2016; Hasanuzzaman et al., 2019) in which the ascorbate (ASC) is a key component (Paciolla et al., 2019). Recently it has been reported that Ce affect growth and metabolism of duckweed plants following a biphasic trend, with stimulatory effects at lower concentrations and inhibitory effects at higher concentrations. Greater Ce concentrations also inducing toxicity symptoms and alteration of photosynthetic pigment content as well as alteration of ROS production, lipid peroxidation, unbalance of antioxidant systems, i.e. typical markers for stress conditions, confirming the potential biological risk associated to Ce-accumulation in soil and freshwater (Zicari et al., 2018).
This work aimed to clarify the potential toxicity of different single REEs and the differences between each one of them in aquatic environments using duckweed as a free-floating macrophyte. The effect of REEs, including Ce, Nd, Gd, Dy, Yb, holmium (Ho) and lutetium (Lu) on development (growth parameters), pigment content and redox status including oxidation level, total antioxidant capacity and antioxidant systems, were investigated under controlled conditions up to millimolar concentrations, considered as threshold levels for polluted water sites (Zhu et al., 2012).
A strain of L. minor, registered at the Herbarium Horti Barensis (BI) n. 41980, RDSC clone 5617, was obtained from the collection of the Botanical Garden of the University Aldo Moro in Bari (Apulia, Italy). Botanical identification was confirmed on a phenotypic basis following Pignatti et al. (2017). The plants were surface sterilized by immersion in a 0.5% (v/v) sodium hypochlorite solution for 1 minute. The plant material was then rinsed with sterile water and transferred, as a number of batches, into culture vessels containing fresh modified Hoagland medium under white light at PFFD of 90 μmol m-2 s-1 with 16 h/8 h light/dark photoperiod at 24 ± 2°C for 7 - 10 days. For each experiment, representative samples of at least 40 plants with 2 visible fronds (Zicari et al., 2018) were incubated in Petri dishes of 9 cm of diameter containing 15 mL of solutions with REE chloride at concentrations 0 (control), 0.1, and 1 mM with pH 5.5. The material was harvested after 3, 7 and 12 days. The concentrations utilized in the trials were applied on the basis of the previous studies (Zicari et al., 2018) and are in accordance with the values reported in some environmental analyses (Zhu et al., 2012).
In order to find a suitable medium maximizing L. minor growth and limiting REE-precipitation, the Hoagland medium was used for the stock culture only. The tests were conducted using water solutions of REE (since phosphate can precipitate with lanthanides (Firsching and Brune, 1991), putting REE in the growth media with phosphates may easily mask the actual toxicity of these elements, resulting in unrealistically low toxicity. REE stock solutions were prepared by dissolution in MilliQ water (cerium chloride (CeCl3•6H2O), neodymium chloride (NdCl3•6H2O), gadolinium chloride (GdCl3•6H2O), dysprosium chloride (DyCl3•6H2O), holmium chloride (HoCl3•6H2O), ytterbium chloride (YbCl3•6H2O), lutetium chloride (LuCl3•6H2O); all purity > 99%; Sigma Aldrich, Milan, Italy). Plants were cultivated in containers filled with modified Hoagland solution containing 252 mg L-1 KNO3; 472 mg L-1 Ca (NO3)2 •4H2O; 246 mg L-1 MgSO4•7H2O; 66 mg L-1 KH2PO4; 25.4 mg L-1 micronutrients; pH 5.5 and semi-closed with a square Petri dish.
Plant growth was determined by relative growth rate (RGR) measurement, a parameter as suggested by the ISO/DIS 20079 (2005) protocol and calculated as follows:
in which N0 is the number of fronds at the beginning of the experiment, Nt is the number of fronds at the selected exposure time and t is the exposure time (3, 7 and 12 days).
Untreated and treated fresh plant samples (1 g) were homogenized at 4°C with 80% acetone with a ratio of 1:15 (w/v) and the homogenates were centrifuged at 20,000 × g for 15 min. With a Beckmann DU-800 spectrophotometer, the absorbance at λ of 663.2, 646.8, and 470 nm was determined on the supernatant, respectively for chlorophyll a (chl a), chlorophyll b, (chl b) and carotenoids (car) as reported by Lichtenthaler (1987).
The oxidation level of the material was monitored by measuring the end product malondialdehyde (MDA), which indicates the level of lipid peroxidation and sugar and amino acid oxidation, according to Lanubile et al. (2022). Briefly, plant material was homogenized with 0.1% trichloroacetic acid (TCA) with a ratio of 1:4 (w/v). After centrifugation (12,000 × g for 10 min), the supernatant was diluted 1:1 with a solution containing 20% TCA and 0.5% thiobarbituric acid (TBA) and incubated for 30 min at 90°C. The reaction was stopped in ice and the samples centrifuged at 12,000 × g for 10 min. The resulting supernatant was used for the determination of MDA-TBA complex by spectrophotometric measurement at 532 nm (extinction coefficient 155 mM−1 cm−1). The obtained absorbance was corrected subtracting the value of unspecific turbidity at 600 nm.
ABTS (2,2′-azino-bis-(3-ethylbenzothiazoline-6-sulfonic) acid) radical-scavenging activity of the hydrophilic fractions was determined by a procedure reported by Teow et al. (2007). Plant samples (1 g) were homogenized with 85% ethanol with a ratio of 1:6 (w/v) and the homogenates were centrifuged at 20,000 × g for 15 min. The total antioxidant activity was determined using ABTS as a radical reacting with the different antioxidant molecules. The ABTS absorbance at λ 730 nm was determined after 1 min reaction.
Treated and control plants (0.5 g) were homogenized with 5% metaphosphoric acid in a 1:4 ratio in a porcelain mortar. The homogenate was centrifuged for 15 min at 20,000 × g (4°C) and the supernatant was collected and immediately assayed for ASC and dehydroascorbate (DHA), according to Zhang and Kirkham (1996).
Samples (0.6 g) were homogenized with 5 mL of ethanol and vortexed for 1 min. The mixture was centrifuged at 6,000 × g for 10 min at 4 °C; 50 μL of the supernatant was added to 950 μL of distilled water and 50 μL of a 1:1 water diluted Folin-Ciocalteu reagent (Sigma Aldrich, Milan, Italy). After 3 min, 100 μL of 0.1 M NaOH solution containing 20% (w/v) Na2CO3 was added, and the resulting solution was incubated at 25 °C for 90 min (Hasperué et al., 2016). The total phenolic content was determined spectrophotometrically at 760 nm using as standard gallic acid (GA) and the results were expressed as GA equivalents (μEq GA) for g–1 fresh weight (FW).
Samples (0.5 g) were ground with four volumes of 50 mM Tris–HCl buffer, pH 8.0, containing 0.3 M mannitol, 1 mM EDTA, and 0.05% (w/v) cysteine. The homogenate was centrifuged at 20,000 × g for 20 min at 4 °C. The supernatant was used for spectrophotometric analysis of the total proteins and enzymatic activities. The total protein content of samples was measured with bovine serum albumin as standard, according to Bradford (1976). The enzymatic spectrophotometric assays of ascorbate peroxidase (APX), catalase (CAT) and total peroxidases (POD) activities were performed according to the method described by Paciolla et al. (2008), with slight modifications. APX activity was carried out following the H2O2-dependent oxidation of ASC at 265 nm in a reaction mixture containing 50 μg of total proteins, 50 μM ASC, 90 μM H2O2 and 50 mM phosphate buffer, pH 6.5. The non-enzymatic H2O2-dependent oxidation of ASC was subtracted. CAT activity assay was evaluated by following H2O2 dismutation at 240 nm in a reaction mixture containing 50 μg of total proteins, 0.1 M phosphate buffer, pH 7.0, and 0.88 μM H2O2. POD activity was measured using 4-methoxy-1-naphthol (4-MN) as substrate. The reaction mixture contained 50 μg of total proteins, 0.1 M Tris Acetate buffer, pH 5.0, 0.1 M 4-MN, and 10 mM H2O2 in a total volume of 1 mL. The decrease in absorbance due to the oxidation of 4-MN was measured at 593 nm.
The data presented are the mean of at least three different replicates of three independent experiments. All analyses were run separately for each REE evaluated. One-way ANOVA followed by Dunnett’s multiple comparisons test was performed using GraphPad Prism version 9.0 (GraphPad Software, San Diego, CA, United States). Statistical significance was accepted at p < 0.01 (*), p < 0.001 (**). A Pearson correlation test was used to determine the correlations between antioxidant capacity (AC) results, total phenolics and ascorbate content. Differences of p < 0.05 were considered significant. The Scheffé test was performed to analyze the significant differences between data (p < 0.05).
Plant growth was monitored by the RGR index (Table 1). A significant increase in RGR was observed only in plants treated with Ce 0.1 mM after 7 and 12 days compared to the control. A decrease was measured at 1 mM after 7 and 12 days of treatment. Nd induced a significant inhibition of the growth at 0.1 mM after 7 and 12 days of treatment. Plants treated with Gd showed a decrease in the RGR at the highest concentration after 3, 7 and 12 days. After treatment with Dy, Yb and Lu there was significant inhibition of the growth compared to the control at all concentrations except for Yb at 0.1 mM after 3 days. Plants treated with Gd showed a decrease in the RGR at the highest concentration after 3, 7 and 12 days. After treatment with Ho, a decrease in RGR was measured after 7 and 12 days of treatment for both concentrations. The appearance of marked chlorosis was observed after 7 days in plants treated with Ce, Dy, Ho, and Lu and after 12 days in plants treated with Ce, Nd, Dy and Lu at 1 mM. The plants treated with Ho at 1 mM after 12 days were in necrosis (Figure 1).
Figure 1. L. minor plants in water (control) and treated with (REE)Cl3•6H2O at concentrations 0.1 and 1 mM for 3, 7 and 12 days.
Chl a and chl b (Figure 2), and car contents (Figure 3) were affected by different REEs and concentrations. When treated with Ce, no significant changes were observed after 3 days for both concentrations. Still, after 12 days there was a significant decrease in chl a and car contents at all concentrations and after 7 days there was a significant decrease at 1 mM, compared to the control. Chl b content decreased significantly after 12 days at the highest concentrations. Treatments with 0.1 mM Nd did not cause significant changes in the content of photosynthetic pigments, except for day 7 of treatment, where total chlorophyll content (chl a + chl b) and carotenoids increased compared to the control. Nd 1 mM caused a significant decrease in the chl a content at all days of treatments; in contrast, chl b and car content decreased after 12 days. For Gd treatment, chl a decreased at both concentrations for the whole test duration, compared to the control, with the most significant decrease after 12 days at 0.1 mM and 1 mM. For chl b a significant decrease was measured after 7 and 12 days and for car over the whole duration of the test at the highest concentration as compared to the control. After treatment with Dy, the chl a and chl b contents decreased significantly at 1 mM compared to the control for the whole duration of the test (Figure 2); the same trend was observed for the carotenoid’s content when the seedlings were treated with 1 mM (Figure 3). Similar to 1mM Dy, a decrease at all time points was recorded in plants treated with Ho for chl a for both concentrations, except after 3 days at 0.1 mM, while for carotenoids, a decrease was measured at 7 and 12 days at the highest concentration. Chl b content was significantly lower after 12 days for both concentrations. As regards Yb, it induced a significant decrease in both chl content at 7 and 12 days for 0.1 mM and during all days of treatment for 1 mM except for chl b at 3 days. Carotenoid content decreased significantly after treatment for 7 days with 0.1 mM and after both 7 and 12 days of treatment with 1 mM. Chl a content was significantly lower than the control in plants treated with Lu at 1 mM for the whole test duration and after 7 days at 0.1 mM; for chl b and carotenoids a significant decrease was recorded in plants treated at the highest concentration after 7 and 12 days.
Figure 2. Effects of different REEs at 0 (control), 0.1 and 1 mM on the contents of chlorophyll a (chl a) and chlorophyll b (chl b) after 3, 7 and 12 days of treatment in L. minor. Vertical bars indicate the SD of three replicates in each treatment group. Statistical significance at p < 0.01 (*) and p < 0.001 (**); FW, fresh weight.
Figure 3. Effects of different REEs at 0 (control), 0.1 and 1 mM on the contents of carotenoids after 3, 7 and 12 days of treatment in L. minor. Vertical bars indicate the SD of three replicates in each treatment group. Statistical significance at p < 0.01 (*) and p < 0.001 (**); FW, fresh weight.
The effect of different REEs on the ascorbate and dehydroascorbate level is reported in Figure 4. Exposure to Ce at 0.1 mM increased the ASC and total ascorbate (ASC+DHA), with the increase being significant after 3 and 7 days of treatment. At 12 days a significant increase was measured for DHA and ASC+DHA. When treated with the highest concentration, ASC, DHA and total ASC+DHA increased significantly in all days of exposure. Plants treated with Nd had a significant increase of ASC+DHA when treated with 0.1 mM after 12 days, due to DHA increase; a significant increase for the ASC content was measured when the seedlings were treated with 1 mM at 3 days, and as a consequence of that, of the ASC+DHA also. Exposure to Gd at 1 mM showed an increase of both ASC and DHA after 3 days whereas for the other days, DHA increased at the highest concentration, but it did not induce a significant change in the total ASC+DHA. Treatment with Dy at 1 mM showed a significant increase in ascorbate and total ASC+DHA levels after 3 and 7 days. After treatment with Ho at 0.1 mM, there was a significant increase of ASC and ASC+DHA after 7 and 12 days of treatment, while the highest concentration induced a significant increment of ASC and ASC+DHA after 3 days and of ASC, DHA and consequently total ASC+DHA after 7 days. A significant increase was shown for ASC content for plants treated with Yb at the highest concentrations for all days of treatment. No significant changes in ASC and DHA levels were measured in plants treated with Lu for the whole duration of the test, for both concentrations.
Figure 4. Ascorbate pool (ASC, ascorbate; DHA, dehydroascorbate; TOT, ascorbate plus dehydroascorbate) in L. minor treated with different REEs at 0.1 and 1 mM after 3, 7 and 12 days. Statistical significance at p < 0.01 (*) and p < 0.001 (**); FW, fresh weight.
The analysis of total antioxidants, which enables our work to evaluate the activity of both hydrophilic and lipophilic antioxidants as a whole, is reported in Figure 5.
Figure 5. Effects of different concentrations of different REEs on the total antioxidant content after 3, 7 and 12 days of treatment in Lemna minor. Vertical bars indicate the SD of three replicates in each treatment group. Statistical significance at p < 0.01 (*) and p < 0.001 (**); FW, fresh weight.
When treated with Ce, the total antioxidant content increased with respect to the control; the plants showed a significant increase in total antioxidant level which is concentration-dependent after 3 and 7 days, while at 12 days, no significant changes were measured. Plants treated with 0.1 mM Nd showed no significant difference in antioxidant levels from the respective controls, whereas at the highest concentration this parameter was significantly lower at days 7 and 12 (about -25%). Plants treated with the highest Gd concentration showed a significant increase in ABTS after 7 and 12 days. Treatment with Dy at 0.1 and 1 mM does not induce significant changes in the total antioxidant content except at 12 days where a decrease for the highest concentration was measured. As regards to the treatment with Ho, low concentration does not cause variations in the levels of total antioxidants; in contrast, a significant decrease was observed with the 1 mM after 12 days of treatment, if compared to the control. In plants treated with Yb at 0.1 mM, a significant decrease in total antioxidants at all time points compared to the relative controls was observed; when treated with 1 mM at day 7 a decrease of about 44% reaching 68% at day 12 compared to the relative control. In plants treated with Lu, a significant decreasing effect was measured after 12 days of treatment at the highest concentration with no significant difference at other time points and lower concentration respect to the control.
The measure of the end product malondialdehyde (MDA), which indicates the level of lipid peroxidation of biological membranes, and sugar and amino acid oxidation significantly increased in plants treated with Ce, Nd and Ho at the highest concentration after 3, 7 and 12 days and when treated with Ce at 0.1 mM after 12 days and Nd at 0.1 mM for the whole duration of the test Figure 6. In plants treated with Gd, MDA content significantly increased for both concentrations after 3 days, whereas it significantly decreased after 12 days at the highest concentration. In Dy treatments, an increase was induced at both treatments after 3 and 7 days, while after 12 days they were not significantly different. After being treated with Ho at the highest concentration, the duckweed plant showed a significant increase in the MDA content at all time points. Treatment with Yb induced an increase after 3 and 7 days for the highest concentration; no significant changes were measured after 12 days for both concentrations. Treatments with Lu at 1 mM induced a significant increase after 3 and 7 days, while after 12 days the MDA levels were significantly lower if compared to the control.
Figure 6. Effects of the concentrations for different REEs on the MDA content after 3, 7 and 12 days of treatment in Lemna minor. Vertical bars indicate the SD of three replicates in each treatment group. Statistical significance at p < 0.01 (*), p < 0.001 (**). FW, fresh weight.
The effect of different REEs at different concentrations and times of treatment on the total phenolic content in duckweed is shown in Figure 7. The longest exposure time for Nd, Dy, Ho, Lu, Yb and Lu at the highest concentration showed a significant decrease in the phenolic content. The same result was measured on plants treated with Nd and Ho at 0.1 mM after 12 days. Plants treated with Yb and Lu showed a significant decrease in the phenolic content when treated at the 0.1 mM concentration after 7 days. When treated with Lu there is a decrease after 7 and 12 days at the highest concentration.
Figure 7. Effects of the concentrations for different REEs on the phenolic content after 3, 7 and 12 days of treatment in Lemna minor. Vertical bars indicate the SD of three replicates in each treatment group. Statistical significance at p < 0.01 (*) and p < 0.001 (**); FW, fresh weight.
For this endpoint were evaluated how some of the light and heavy REEs utilized in this experimental design could affect the activity of antioxidant enzymes as CAT, APX and POD (Figure 8). Ce and Nd were specifically chosen due to their status as two of the most in demand and produced REEs (de Boer and Lammertsma, 2013), reflecting their ecological significance and widespread presence in environmental systems, as light REEs. Additionally, Ho was selected as a representative of heavy REEs, providing insight into the potential differential effects between light and heavy REE exposures on Lemna minor. The results after 7 days of treatment are shown in Figure 8. All treatments showed a significant increase in APX activity at all concentrations. Total peroxidase activity was different after treatment with REEs, as compared with the control. Specifically, all treatments induced a significant increase in POD activity except for Ce at the lowest concentration. Catalase activity in plants treated with Ho significantly increased at the highest concentration whereas no difference was recorded after Nd and Ce treatment.
Figure 8. Effects of 0.1 and 1 mM of Ce, Nd and Ho on the activity of ascorbate peroxidase (APX), total peroxidase (POD) and catalase (CAT) enzymes after 7 days of treatment in Lemna minor. Vertical bars indicate the SD of three replicates in each treatment group. Statistical significance at p < 0.01 (*) and p < 0.001 (**); FW, fresh weight.
To define the contribution of ascorbate to the total antioxidant capacity in duckweed in the various REE treatments, the relationship between these two parameters detected was analyzed (Table 2).
Table 2. Pearson’s correlation coefficients of the antioxidant capacity and ascorbate content of Lemna minor after treatment for 3, 7 and 12 days with single REE.
This test showed that there was a strong positive correlation between the two variables for plants treated with Ce that was significant during time treatments. On the other hand, when treated with Nd, Gd and Dy, ASC content was negatively correlated with the antioxidant capacity, being significant after 7 and 12 days and the latter at 3 days, too. The correlation was significantly positive for treatments with Ho after 3 and 12 days. Treatment with Yb induced a positive, medium correlation at 7 days and a negative, very high correlation at 12 days. When treated with Lu, a significant negative correlation was found at 3 and 7 days.
The increasing demand for REE in modern technology has led to a steady rise in global REE mining and extraction activities. However, little is known about these elements’ potential environmental impacts and toxicities on terrestrial and aquatic plants growing in contaminated soils (Carpenter et al., 2015) and the concentration of these elements in water and soil. Lemna minor is a common model organism used to study the toxicity of these elements in aquatic systems (Weltje et al., 2002). Although no negative effects were reported on Lemna growth at nanomolar concentrations, it is important to consider the bioaccumulation factor and monitor lanthanide emissions in water (Weltje et al., 2002). On the other hand, some data reported presence and concentration patterns of REEs in rivers in China suggesting an emerging risk of contamination (Ma et al., 2019). Data of this work confirms the potential toxicity of REEs correlated with concentration. The effects on the growth of L. minor plants treated with Ce chloride follow a dose-depending response inducing stimulation at 0.1 mM and inhibition at the highest concentration. This biphasic effect resembles the hormetic effect (Zicari et al., 2018) and is consistent with data reported for Arabidopsis thaliana (Wang et al., 2012) treated with Ce. REEs have been reported as having positive effects at low concentrations, thus suggesting a hormetic trend, implying a concentration-related shift from stimulation to inhibition and toxicity, when the concentration passes a threshold different for each species (Tommasi et al., 2023). The other REEs used in this study induced growth inhibition at both concentrations. Growth inhibition was also associated with chlorotic symptoms and, after more prolonged treatment in the case for some REEs, with necrosis, following ISO/DIS 20079 (2005) guidelines.
The accentuated leaf chlorosis under holmium treatment indicated an iron deficiency probably due to interference of this REE with the iron absorption process. Indeed, chlorosis is a characteristic symptom for plants grown in conditions of low iron levels. It manifests in decreased photosynthetic units, stromal lamellae of the chloroplast and thylakoids per granum (Spiller and Terry, 1980; Terry, 1980).
However, some REEs, such as CeCl3 at lower concentrations, could improve the reaction of changing yellowing seedlings into green seedlings, which suggested that Ce3+ may enhance the conversion of protochlorophyll into chlorophyll (Ni, 1995). While a previous study on zebrafish embryos suggested LREE to be more toxic than HREE (Lin et al., 2022), our findings show that HREEs have a much stronger and more significant effect on all endpoints measured. The same trend was reported by Alp et al. (2023), where an excess of terbium (HREE) caused toxicity and reduced plant growth in L. minor. On the other hand, the preferential translocation to leaves of HREEs in the five Phytolacca species with consequent altered growth goes along with this result (Grosjean et al., 2019).
The observed alterations of photosynthetic pigment levels are typical markers of REEs toxicity, as reported in the responses to abiotic stress when high concentrations of them occur (Wang et al., 2014; Zhang et al., 2015). In the leaves of the aquatic plant Hydrocharis dubia treated with lanthanum, it is observed a decrease in pigment content, which could be related either to the disturbance in biochemical synthesis processes or to an enhanced degradation associated with damage to chloroplast ultrastructure (Xu et al., 2012). On the other hand, a number of studies revealed that several REEs may play multiple catalytic roles in chlorophyll formation and indirectly contribute to chlorophyll biosynthesis (Hong et al., 2002). A study with spinach leaves treated with concentrations of cerium at 5 and 10 µg/mL reported that Ce3+ increased Mg2+ absorption and the formation of Mg2+–chlorophyll, the Mg2+ bound to the porphyrin ring more easily than Ce3+, and Ce3+ could primarily play a catalyst role in chlorophyll formation (Hong et al., 2002). Our results align with this finding only for plants treated with Ce at the lowest concentration, where no changes in chlorophyll content were observed.
Regarding the treatment with gadolinium, a lower chlorophyll content compared to the control was observed in Lemna already at 0.1 mM, suggesting that this concentration was toxic for this aquatic plant. In A. thaliana the total chlorophyll content at low concentrations of Gd (heavy metals) (0.05 mM) appeared unaffected, while at higher concentrations (0.2 mM) it decreased significantly (Liu et al., 2021); this underlines the presence of different response between aquatic and terrestrial plants when they are treated with Gd. The effect of REEs at high concentrations on chlorophyll content can be compared to that of heavy metals. Indeed, some studies have indicated that could damage genes regulating chlorophyll in A. thaliana, thus altering chlorophyll content and affecting plant growth (Liu et al., 2021).
Data reported in this work show that a remarkable increase in ascorbate content was evident in duckweed plants when treated at the highest concentration throughout the whole duration of the test for all REEs, with the exceptions of Ho and Nd. Generally, increased ascorbate content highlights the plant’s attempts to counteract the oxidative stress induced by REEs (Gjata et al., 2022). It is known that ASC is a key molecule in the plant antioxidant system and due to its redox potential ranges from +0.40 to +0.50 can directly suppress the reactive species (Paciolla et al., 2019). In shorter times, there is an evident strong and positive correlation between ascorbate and antioxidant capacity, which implies an important involvement of ascorbate in the antioxidant machinery of Lemna after REE treatment. In this case, ASC can be considered an important antioxidant component, being sensitive to abiotic stress like REEs. Our results show however that there are also negative correlations between these two variables in plants treated with Nd, Gd, Dy and Yb after 12 days. In this case, we can assume that ASC was not directly involved or insufficient in counteracting the cellular redox imbalance due to REEs and that, after a longer time, the antioxidant machinery, including ascorbate, was compromised. On the other hand, lower ascorbate content in Lemna seedlings treated with Ho or Nd can be related to the fact that the plants were showing symptoms of chlorosis or were in necrosis.
The present investigation into the effects of REEs on oxidation levels in duckweed revealed significant alterations in MDA content, an indicator for the level of lipid peroxidation as well as sugar and amino acid oxidation. The increase in MDA content at various time points indicates that the REEs induced oxidative stress in the duckweed plants, as also reported by Ippolito et al. (2010). The fact that this response varies over time suggests that the plants’ oxidative stress response is dynamic and potentially influenced by factors such as the duration of exposure and the specific properties of each REE (Gjata et al., 2022). Different REEs have distinct effects on the oxidation level. For instance, Ce and Nd consistently increased MDA levels at high concentrations at all time points, suggesting strong oxidative processes of the components in biological membrane.
In contrast, the initially increased MDA levels but later its decrease in Gd, suggested a possible adaptive response or detoxification mechanism activated by prolonged exposure. On the other hand, this trend can be in part explained by an increase in available ASC which is able to reduce tocopheroxyl radicals with a consequent decrease in lipid peroxidation (Szarka et al., 2012). Further research should explore the underlying mechanisms of these differential responses, including potential adaptive processes that may mitigate oxidative damage over longer exposure periods.
Phenolic compounds play a crucial role in plant defense against various stresses (Lin et al., 2016). The decrease in total phenolic content suggests that the plants’ defense mechanisms are being compromised, potentially making them more susceptible to stressors (Kumar et al., 2020). The reduction in phenolic content is influenced by both the concentration of the REEs and the duration of exposure. Higher concentrations and longer exposure times generally lead to more pronounced decreases in phenolic content, indicating a cumulative negative impact on the plant’s metabolic processes over time (Wang et al., 2014). Yb and Lu show significant effects at both lower and higher concentrations, but the timing of the impact differs. This variability indicates that each REE interacts differently with the plant’s biochemical pathways. It is known that exposure to certain doses of REE induces a rise in antioxidant enzymes and in antioxidant substances such as carotenoids and total phenols of plants (Emmanuel et al., 2010a; 2010b). Such impacts of REEs do not only depend on the dose but are also associated with the duration of exposure (Zhang et al., 2013).
Antioxidant enzymes are important for plant defense against oxidative stress caused by ROS. These enzymes, including APX, POD, and CAT, function synergistically to detoxify ROS, are involved in maintaining redox homeostasis (Zandi and Schnug, 2022) and enhance plant stress tolerance (Hasanuzzaman et al., 2019). APX and CAT synergistically convert H2O2 into H2O and O2 (Ahmad and Tahir, 2016); particularly, APX can remove a low quantity of H2O2 more efficiently than CAT and POD, having higher affinity constant for it (μM range vs mM range) (Asada, 1992). Except for Ho at the highest concentration, treatments with REEs did not induce significant changes in CAT activity, suggesting that CAT was not strongly involved in breaking down H2O2, further reducing oxidative damage. Similar results have been previously detected in L. minor plants treated with Tb (Alp et al., 2023).
On the other hand, there was increase in activity of both APX and POD enzymes in all treatments. The increase in APX activity can also be related to the elevated levels of ASC, as when ASC biosynthesis is experimentally induced, the APX also appears earlier (De Gara et al., 1997). POD catalyzes the oxidation of various reducing agents using H2O2. Higher activity of POD could carry out increased lignification, giving strength and stiffness to plant cell walls (Habibi, 2014) and, therefore, can be considered a response to REE stressors.
Data from this works suggests that REE elements have different effects on the growth and metabolism of duckweed. Cerium showed a positive effect at lower concentrations on the growth, chlorophyll content, and antioxidant systems, which was supported by no change in oxidation level. A negative effect on the growth, chlorophyll and oxidation level was observed at the highest concentration although an increase in antioxidant capacity, including ascorbate, APX and POD suggested a counteraction of Lemna to oxidative stress.
In the presence of neodymium, although a decrease of plant growth at lower concentrations together with higher oxidation levels of the membranes was observed, the heightened antioxidant systems appear to support the plant’s response capacity and thus lower toxicity of this REE.
In the case for gadolinium and ytterbium, the analysis of parameters studied indicated that only at the concentration of 1 mM did they assist in growth inhibition, indicating a higher tolerance of Lemna to these two REEs.
Dysprosium and lutetium appear to be the REEs with the highest toxicity considering the high oxidation level and low chlorophyll content with no antioxidant response.
Although a marked increase of all antioxidant enzymes analyzed was observed in the presence of holmium, this was not an efficient response to oxidative stress thus causing growth inhibition at longer times.
REEs are present in soil, stream water, and sewage discharge channels with different and consistent concentrations, while few data are available about the presence and mobility of REEs in these ecosystems. This study shows that Lemna minor can tolerate low concentrations (0.1mM) of REE chlorides, whereas toxic effects occur after treatment at the highest concentrations tested (1 mM) and/or after more prolonged exposures. Data from this work suggests that REEs differently affect the growth and induce metabolic perturbations in duckweed. The decrease in chlorophyll content and the increase in peroxidation levels are indexes of toxicity as well as the alterations of antioxidant systems although of different type and consistence depending on the REE. Cerium, neodymium, gadolinium and ytterbium appeared less toxic than dysprosium, holmium and lutetium, suggesting an increase in toxicity correlated to the molecular mass. The toxicity of REEs at millimolar concentrations observed in this study could represent a potential risk for using REE fertilizers in water cultures. The REEs are less harmful than other elements like cadmium and mercury that induce stress responses at micromolar concentrations and are also less toxic than lead, which duckweed plants tolerate at concentrations up to 0.25 mM. However, our data indicate that these rare elements represent a potential risk for aquatic ecosystems with free-floating macrophytes such as Lemna minor. This plant, therefore, could be a useful toxicity assessment tool in aquatic environments that may be contaminated with millimolar concentrations of REEs, although more data about the presence and mobility of REEs in these ecosystems are necessary to propose warning levels of REEs.
The datasets presented in this study can be found in online repositories. The names of the repository/repositories and accession number(s) can be found in the article/Supplementary Material.
IG: Data curation, Formal Analysis, Investigation, Methodology, Software, Validation, Visualization, Writing – original draft. FT: Data curation, Formal Analysis, Investigation, Methodology, Software, Validation, Visualization, Writing – original draft, Conceptualization, Funding acquisition, Project administration, Resources, Supervision, Writing – review & editing. SD: Data curation, Formal Analysis, Investigation, Methodology, Software, Visualization, Writing – review & editing. CP: Data curation, Formal Analysis, Investigation, Methodology, Software, Visualization, Conceptualization, Funding acquisition, Project administration, Resources, Supervision, Validation, Writing – original draft, Writing – review & editing.
The author(s) declare that financial support was received for the research, authorship, and/or publication of this article. This project has received funding from European Union’s Horizon 2020 research and innovation program under the Marie Sklodowska-Curie Grant Agreement N°857989 and from the project “Tredimpostrale terre rare, elementi geochimici di importanza strategica: presenza nel suolo e sottosuolo ed effetti in organismi modello” (CUP H91I21001690006 -Avviso Horizon Europe Seeds D.R. 1940 del 04/06/2021).
The authors want to thank the Botanical Garden of the University Aldo Moro in Bari for providing the strain of Lemna minor used for this research.
The authors declare that the research was conducted in the absence of any commercial or financial relationships that could be construed as a potential conflict of interest.
The author(s) declared that they were an editorial board member of Frontiers, at the time of submission. This had no impact on the peer review process and the final decision.
The author(s) declare that no Generative AI was used in the creation of this manuscript.
All claims expressed in this article are solely those of the authors and do not necessarily represent those of their affiliated organizations, or those of the publisher, the editors and the reviewers. Any product that may be evaluated in this article, or claim that may be made by its manufacturer, is not guaranteed or endorsed by the publisher.
Ahmad, S. S., Tahir, I. (2016). Increased oxidative stress, lipid peroxidation and protein degradation trigger senescence in Iris versicolor L. flowers. Physiol. Mol. Biol. Plants 22, 507–514. doi: 10.1007/s12298-016-0392-9
Alonso, E., Sherman, A. M., Wallington, T. J., Everson, M. P., Field, F. R., Roth, R., et al. (2012). Evaluating rare earth element availability: a case with revolutionary demand from clean technologies. Environ. Sci. Technol. 46, 3406–3414. doi: 10.1021/es203518d
Alp, F. N., Arikan, B., Ozfidan-Konakci, C., Gulenturk, C., Yildiztugay, E., Turan, M., et al. (2023). Hormetic activation of nano-sized rare earth element terbium on growth, PSII photochemistry, antioxidant status and phytohormone regulation in Lemna minor. Plant Physiol. Biochem. 194, 361–373. doi: 10.1016/j.plaphy.2022.11.031
Arienzo, M., Ferrara, L., Trifuoggi, M., Toscanesi, M. (2022). Advances in the fate of rare earth elements, REE, in transitional environments: coasts and estuaries. Water 14, 401. doi: 10.3390/w14030401
Asada, K. (1992). Ascorbate peroxidase – a hydrogen peroxide-scavenging enzyme in plants. Physiol. Plant 85, 235–241. doi: 10.1111/j.1399-3054.1992.tb04728.x
Barry, M. J., Meehan, B. J. (2000). The acute and chronic toxicity of lanthanum to Daphnia carinata. Chemosphere 41, 1669–1674. doi: 10.1016/S0045-6535(00)00091-6
Bau, M., Dulski, P. (1996). Distribution of yttrium and rare-earth elements in the Penge and Kuruman iron-formations, Transvaal Supergroup, South Africa. Precambrian Res. 79, 37–55. doi: 10.1016/0301-9268(95)00087-9
Binnemans, K., Jones, P. T., Blanpain, B., Van Gerven, T., Yang, Y., Walton, A., et al. (2013). Recycling of rare earths: A critical review. J. Clean. Prod. 51, 1–22. doi: 10.1016/j.jclepro.2012.12.037
Blinova, I., Muna, M., Heinlaan, M., Lukjanova, A., Kahru, A. (2020). Potential hazard of lanthanides and lanthanide-based nanoparticles to aquatic ecosystems: data gaps, challenges and future research needs derived from bibliometric analysis. Nanomaterials (Basel) 10, 328. doi: 10.3390/nano10020328
Borgmann, U., Couillard, Y., Doyle, P., Dixon, D. G. (2005). Toxicity of sixty-three metals and metalloids to Hyalella azteca at two levels of water hardness. ET&C/24 3), 641–652. doi: 10.1897/04-177R.1
Bradford, M. M. (1976). A rapid and sensitive method for the quantitation of microgram quantities of protein utilizing the principle of protein-dye binding. Anal. Biochem. 72, 248–254. doi: 10.1016/0003-2697(76)90527-3
Carpenter, D., Boutin, C., Allison, J. E., Parsons, J. L., Ellis, D. M. (2015). Uptake and effects of six rare earth elements (REEs) on selected native and crop species growing in contaminated soils. PloS One 10, e0129936. doi: 10.1371/journal.pone.0129936
Cotton, F. A., Wilkinson, G., Murillo, C. A., Bochmann, M. (1999). Advanced inorganic chemistry (New York: John Wiley & Sons, INC), ISBN: ISBN: 978-0-471-19957-1.
d’Aquino, L., Tommasi, F. (2017). Rare earth elements and microorganisms in Rare earth elements in human and environmental health: at crossroads between toxicity and safety. Ed. Pagano, G. (Singapore: Pan Stanford Publishing Pte. Ltd), 127–141.
de Boer, M. A., Lammertsma, K. (2013). Scarcity of rare earth elements. ChemSusChem 6, 2023–2197. doi: 10.1002/cssc.201200794
De Gara, L., de Pinto, M. C., Arrigoni, O. (1997). Ascorbate synthesis and ascorbate peroxidase activity during the early stage of wheat germination. Physiol. Plant 100, 894–900. doi: 10.1111/j.1399-3054.1997.tb00015.x
den Doore de Jong, L. E., Roman, W. B. (1965). Tolerance of Chlorella vulgaris for metallic and non-metallic ions. Antonie van Leeuwenhock 31, 301–313. doi: 10.1007/BF02045910
Emmanuel, E. S., Anandkumar, B., Natesan, M., Maruthamuthu, S. (2010a). Efficacy of rare earth elements on the physiological and biochemical characteristics of Zea mays L. Aust. J. Crop Sci. 4, 289–294. Available at: https://api.semanticscholar.org/CorpusID:11086561 (Sccessed December 11, 2024).
Emmanuel, E. S., Ramachandran, A. M., Ravindran, A. D., Natesan, M., Maruthamuthu, S. (2010b). Effect of some rare earth elements on dry matter partitioning, nodule formation, and chlorophyll content in Arachis hypogaea L. plants. Aust. J. Crop Sci. 4, 670–675. (Accessed December 11, 2024)
European Commission (2010). Critical raw materials for the EU, Report of the Ad-hoc Working Group on defining critical raw materials. Available online at: https://www.euromines.org/files/what-we-do/sustainable-development-issues/2010-report-critical-raw-materials-eu.pdf.
Fenske, M. J., Raymond, J. E. (2006). Affective influences of selective attention. Curr. Dir. Psychol. Sci. 15, 312–316. doi: 10.1111/j.1467-8721.2006.00459.x
Firsching, F. H., Brune, S. N. (1991). Solubility products of the trivalent rare-earth phosphates. J. Chem. Eng. Data 36, 93–95. doi: 10.1021/je00001a028
Gjata, I., Tommasi, F., De Leonardis, S., Dipierro, N., Paciolla, C. (2022). Cytological alterations and oxidative stress induced by Cerium and Neodymium in lentil seedlings and onion bulbs. Front. Environ. Sci. 10. doi: 10.3389/fenvs.2022.969162
Gonzalez, V., Vignati, D. A. L., Leyval, C., Giamberini, L. (2014). Environmental fate and ecotoxicity of lanthanides: Are they a uniform group beyond chemistry? Environ. Int. 71, 148–157. doi: 10.1016/j.envint.2014.06.019
Grosjean, N., Le Jean, M., Berthelot, C., Chalot, M., Gross, E. M., Blaudez, D. (2019). Accumulation and fractionation of rare earth elements are conserved traits in the Phytolacca genus. Sci. Rep. 9, 18458. doi: 10.1038/s41598-019-54238-3
Habibi, G. (2014). Hydrogen peroxide (H2O2) generation, scavenging and signaling in plants in Oxidative Damage to Plants. Ed. Ahmad, P. (Academic Press: San Diego, CA), 557–584. doi: 10.1016/B978-0-12-799963-0.00019-8 (Accessed December 12, 2024).
Hasanuzzaman, M., Bhuyan, M. H. M. B., Anee, T. I., Parvin, K., Nahar, K., Mahmud, J. A., et al. (2019). Regulation of ascorbate-glutathione pathway in mitigating oxidative damage in plants under abiotic stress. Antioxidants 8, 384. doi: 10.3390/antiox8090384
Hasperué, J. H., Guardianelli, L., Rodoni, L. M., Chaves, A. R., Martínez, G. A. (2016). Continuous white–blue LED light exposition delays postharvest senescence of broccoli. LWT-Food Sci. Technol. 65, 495–502. doi: 10.1016/j.lwt.2015.08.044
Hong, F., Wang, L., Meng, X., Wei, Z., Zhao, G. (2002). The effect of cerium (III) on the chlorophyll formation in spinach. Biol. Trace Elem. Res. 89, 263–276. doi: 10.1385/BTER:89:3:263
Hu, Z., Richter, H., Sparovek, G., Schnug, E. (2004). Physiological and biochemical effects of rare earth elements on plants and their agricultural significance: a review. J. Plant Nutr. 27, 183–220. doi: 10.1081/PLN-120027555
Humphries, M. (2010). Rare Earth Elements: The Global Supply Chain (Diane Publishing: Waschington, D.C.). Available at: https://api.semanticscholar.org/CorpusID:152373948.
Ippolito, M. P., Fasciano, C., d’Aquino, L., Morgana, M., Tommasi, F. (2010). Responses of antioxidant systems after exposition to rare earths and their role in chilling stress in common Duckweed (Lemna minor L.): a defensive weapon or a boomerang? Arch. Environ. Contam. Toxicol. 58, 42–52. doi: 10.1007/s00244-009-9340-9
ISO 20079 (2005). Water quality — Determination of the toxic effect of water constituents and waste water on duckweed (Lemna minor) — Duckweed growth inhibition test (ISO: BSI, Brussels). Available at: https://www.iso.org/standard/34074.html.
Kumar, S. J., Kumar, A., Ramesh, K. V., Singh, C., Agarwal, D. K., Pal, G., et al. (2020). Wall bound phenolics and total antioxidants in stored seeds of soybean (Glycine max) genotypes. Indian J. Agric. Sci. 90, 118–222. doi: 10.56093/ijas.v90i1.98557
Lanubile, A., De Michele, R., Loi, M., Fakhari, S., Marocco, A., Paciolla, C. (2022). Cell death induced by mycotoxin fumonisin B1 is accompanied by oxidative stress and transcriptional modulation in Arabidopsis cell culture. Plant Cell Rep. 41, 1733–1750. doi: 10.1007/s00299-022-02888-5
Lichtenthaler, H. K. (1987). Chlorophylls and carotenoids: Pigments of photosynthetic biomembranes in Methods in Enzymology Vol. 148 (Academic Press: Orlando, FL), 350–382. doi: 10.1016/0076-6879(87)48036-1
Lin, Y. T., Liu, R. X., Audira, G., Suryanto, M. E., Roldan, M. J. M., Lee, J. S., et al. (2022). Lanthanides toxicity in zebrafish embryos are correlated to their atomic number. Toxics 10, 336. doi: 10.3390/toxics10060336
Lin, D., Xiao, M., Zhao, J., Li, Z., Xing, B., Li, X., et al. (2016). An overview of plant phenolic compounds and their importance in human nutrition and management of type 2 diabetes. Molecules 21, 1374. doi: 10.3390/molecules21101374
Liu, Z., Guo, C., Tai, P., Sun, L., Chen, Z. (2021). The exposure of gadolinium at environmental relevant levels induced genotoxic effects in Arabidopsis thaliana (L.). Ecotoxicol. Environ. Saf. 215, 112138. doi: 10.1016/j.ecoenv.2021.112138
Ma, L., Dang, D. H., Wang, W., Wang, W. X. (2019). Rare earth elements in the Pearl River delta of China: Potential impacts of the REE industry on watwer, suspenden particles and oysters. Environ. pollut. 244, 190–201. doi: 10.1016/j.envpol.2018.10.015
Moody, M., Miller, J. (2005). Lemna Minor Growth Inhibition Test in Small-scale Freshwater Toxicity Investigations. Eds. Blaise, C., Férard, J. F. (Dordrecht: Springer), 271–298. doi: 10.1007/1-4020-3120-3_8
OECD (2006). Test No. 221: Lemna sp. Growth Inhibition Test, OECD Guidelines for the Testing of Chemicals, Section 2 (Paris: OECD Publishing). doi: 10.1787/9789264016194-en
Oral, R., Bustamante, P., Warnau, M., D’Ambra, A., Guida, M., Pagano, G. (2010). Cytogenetic and developmental toxicity of cerium and lanthanum to sea urchin embryos. Chemosphere 81, 194–198. doi: 10.1016/j.chemosphere.2010.06.057
Paciolla, C., Fortunato, S., Dipierro, N., Paradiso, A., De Leonardis, S., Mastropasqua, L., et al. (2019). Vitamin C in plants: from functions to biofortification. Antioxidants 8, 519. doi: 10.3390/antiox8110519
Paciolla, C., Ippolito, M. P., Logrieco, A., Dipierro, N., Mulè, G., Dipierro, S. (2008). A different trend of antioxidant defence responses makes tomato plants less susceptible to beauvericin than to T-2 mycotoxin phytotoxicity. Physiol. Mol. Plant Pathol. 72, 3–9. doi: 10.1016/j.pmpp.2008.06.003
Paradiso, A., Caretto, S., Leone, A., Bove, A., Nisi, R., De Gara, L. (2016). ROS production and scavenging under anoxia and re-oxygenation in Arabidopsis cells: a balance between redox signaling and impairment. Front. Plant Sci. 7. doi: 10.3389/fpls.2016.01803
Reisman, D., Weber, R., McKernan, J., Northeim, C. (2013). Rare Earth Elements: A Review of Production, Processing,Recycling, and Associated Environmental Issues; EPA Report EPA/600/R-12/572 (Washington, DC, USA: U.S. Environmental Protection Agency (EPA). Available at: https://nepis.epa.gov/Adobe/PDF/P100EUBC.pdf (Accessed November 12, 2024).
Schüler, D., Buchert, M., Liu, R., Dittrich, S., Merz, C. (2011). Study on rare earths and their recycling Vol. 49 (Darmstadt: Öko-Institut e.V), 30–40. Available at: http://www.oeko.de/publikationen/dok/1192.php.
Song, H., Shin, W. J., Ryu, J. S., Shin, H. S., Chung, H., Lee, K. S. (2017). Anthropogenic rare earth elements and their spatial distributions in the Han River, South Korea. Chemosphere 172, 155–165. doi: 10.1016/j.chemosphere.2016.12.135
Spiller, S., Terry, N. (1980). Limiting factors in photosynthesis. II. Iron stress diminished photochemical capacity by reducing the number of photosyntetic units. Plant Physiol. 65, 121–125. doi: 10.1104/pp.65.1.121
Szarka, A., Bálint, T., Gábor, B. (2012). The ascorbate-glutathione-α-tocopherol triad in abiotic stress response. Int. J. Mol. Sci. 13, 4458–4483. doi: 10.3390/ijms13044458
Tai, P., Zhao, Q., Su, D., Li, P., Stagnitti, F. (2010). Biological toxicity of lanthanide elements on algae. Chemosphere 80, 1031–1035. doi: 10.1016/j.chemosphere.2010.05.030
Teow, C., Truong, V. D., McFeeters, R., Thompson, R., Pecota, K., Yencho, C. (2007). Antioxidant activities, phenolic and beta-carotene contents of sweet potato genotypes with varying flesh colours. Food Chem. 103, 829–838. doi: 10.1016/j.foodchem.2006.09.033
Terry, N. (1980). Limiting factors in photosynthesis I. Use of iron stress to control photochemical capacity in vivo. Plant Physiol. 65, 114–120. doi: 10.1104/pp.65.1.114
Tommasi, F., d’Aquino, L. (2017). Rare earth elements and plants in Rare earth elements in human and environmental health: at the crossroad between toxicity and safety. Ed. Pagano, G. (Singapore: Pan Stanford Publishing Pte. Ltd), 107–125.
Tommasi, F., Thomas, P. J., Lyons, D. M., Pagano, G., Oral, R., Siciliano, A., et al. (2023). Evaluation of rare earth element-associated hormetic effects in candidate fertilizers and livestock feed additives. Biol. Trace Elem. Res. 201, 2573–2581. doi: 10.1007/s12011-022-03331-2
UNCTAD (2014). Commodities at a glance: Special issue on rare earths (N°5 – May 2014). Available online at: https://unctad.org/system/files/official-document/suc2014d1_en.pdf (Accessed November 16, 2024).
U.S. Geological Survey (2016). Mineral commodity summaries 2016 (U.S. Geological Survey: Reston, VA), 202 p. doi: 10.3133/70140094
Wang, L., Li, J., Zhou, Q., Yang, G., Ding, X. L., Li, X., et al. (2014). Rare earth elements activate endocytosis in plant cells. PNAS 111, 12936–12941. doi: 10.1073/pnas.1413376111
Wang, X., Lin, Y., Liu, D., Xu, H., Liu, T., Zhao, F. (2012). Cerium toxicity, uptake and translocation in Arabidopsis thaliana seedlings. J. Rare Earths 30, 579–585. doi: 10.1016/S1002-0721(12)60094-5
Weltje, L., Heidenreich, H., Zhu, W., Wolterbeek, H., Korhammer, S., de Goeij, J. J. M., et al. (2002). Lanthanide concentrations in freshwater plants and molluscs, related to those in surface water, pore water and sediment. A case study in The Netherlands. Sci. Total Environ. 286, 191–214. doi: 10.1016/S0048-9697(01)00978-0
Xu, Q., Fu, Y., Min, H., Cai, S., Sha, S., Cheng, G. (2012). Laboratory assessment of uptake and toxicity of lanthanum (La) in the leaves of Hydrocharis dubia (Bl.) Backer. Environ. Sci. pollut. Res. 19, 3950–3958. doi: 10.1007/s11356-012-0982-1
Zandi, P., Schnug, E. (2022). Reactive oxygen species, antioxidant responses and implications from a microbial modulation perspective. Biology 11, 155. doi: 10.3390/biology11020155
Zhang, J., Kirkham, M. B. (1996). Antioxidant responses to drought in sunflower and sorghum seedlings. New Phytol. 132, 361–373. doi: 10.1111/j.1469-8137.1996.tb01856.x
Zhang, C., Li, Q., Zhang, M., Zhang, N., Li, M. (2013). Effects of rare earth elements on growth and metabolism of medicinal plants. Acta Pharm. Sin. B. 3, 20–24. doi: 10.1016/j.apsb.2012.12.005
Zhang, W., Rezaee, M., Bhagavatula, A., Li, Y., Groppo, J., Honaker, R. (2015). A Review of the occurrence and promising recovery methods of rare earth elements from coal and coal by-products. Int. J. Coal Prep. Util. 35, 295–330. doi: 10.1080/19392699.2015.1033097
Zhu, Z., Wang, Z., Li, J., Li, Y., Zhang, Z., Zhang, P. (2012). Distribution of rare earth elements in sewage-irrigated soil profiles in Tianjin, China. J. Rare Earths 30, 609–613. doi: 10.1016/S1002-0721(12)60099-4
Keywords: antioxidant systems, duckweed, hormesis, oxidative stress, rare earth element, toxicity
Citation: Gjata I, Tommasi F, De Leonardis S and Paciolla C (2025) Rare earth elements affect the growth and fitness of free-floating plant Lemna minor L.. Front. Plant Sci. 16:1540266. doi: 10.3389/fpls.2025.1540266
Received: 05 December 2024; Accepted: 10 February 2025;
Published: 28 February 2025.
Edited by:
Eric Lam, Rutgers, The State University of New Jersey, United StatesCopyright © 2025 Gjata, Tommasi, De Leonardis and Paciolla. This is an open-access article distributed under the terms of the Creative Commons Attribution License (CC BY). The use, distribution or reproduction in other forums is permitted, provided the original author(s) and the copyright owner(s) are credited and that the original publication in this journal is cited, in accordance with accepted academic practice. No use, distribution or reproduction is permitted which does not comply with these terms.
*Correspondence: Franca Tommasi, franca.tommasi@uniba.it; Costantino Paciolla, costantino.paciolla@uniba.it
Disclaimer: All claims expressed in this article are solely those of the authors and do not necessarily represent those of their affiliated organizations, or those of the publisher, the editors and the reviewers. Any product that may be evaluated in this article or claim that may be made by its manufacturer is not guaranteed or endorsed by the publisher.
Research integrity at Frontiers
Learn more about the work of our research integrity team to safeguard the quality of each article we publish.