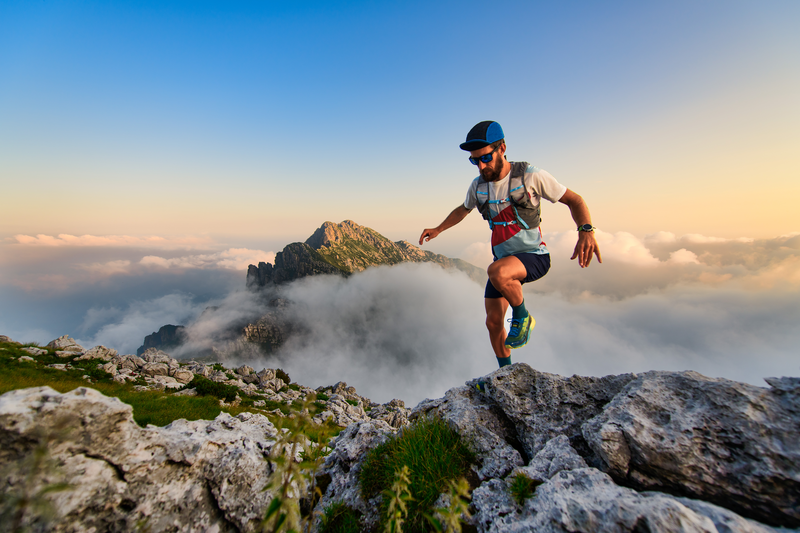
95% of researchers rate our articles as excellent or good
Learn more about the work of our research integrity team to safeguard the quality of each article we publish.
Find out more
ORIGINAL RESEARCH article
Front. Plant Sci. , 26 February 2025
Sec. Plant Abiotic Stress
Volume 16 - 2025 | https://doi.org/10.3389/fpls.2025.1528923
Introduction: Harnessing plant growth-promoting rhizobia presents a sustainable and cost-effective method to enhance crop performance, particularly under drought stress. This study evaluates the variability of plant growth-promoting (PGP) traits among three strains of Rhizobium laguerreae LMR575, LMR571, and LMR655, and two native PGP strains Bacillus LMR698 and Enterobacter aerogenes LMR696. The primary objective was to assess the host range specificity of these strains and their effectiveness in improving drought tolerance in three legume species: Pisum sativum, Vicia faba, and Phaseolus vulgaris.
Methods: In-vitro experiments were conducted to assess the PGP traits of the selected strains, including phosphate solubilization, indole-3-acetic acid (IAA) production, and siderophore production. Greenhouse trials were also performed using a mixed inoculum of performing strains to evaluate their effects on plant physiological and biochemical traits under drought conditions.
Results: Significant variability in PGP traits was observed among the strains. R. laguerreae LMR655 exhibited the highest phosphate solubilization (113.85 mg mL-1 PO42-), while R. laguerreae LMR571 produced the highest IAA concentration (25.37 mg mL-1). E. aerogenes LMR696 demonstrated 82% siderophore production. Symbiotic interactions varied, with R. laguerreae LMR571 and LMR655 forming associations with P. sativum and V. faba, but none establishing compatibility with P. vulgaris. Greenhouse experiments showed that a mixed inoculum of R. laguerreae LMR571, LMR655, and E. aerogenes LMR696 significantly improved proline, total soluble sugars, proteins, and chlorophyll content under drought stress, with V. faba showing the strongest response.
Discussion: These findings highlight the importance of strain selection based on host specificity and PGP potential. The enhanced drought tolerance observed suggests that tailored microbial inoculants can improve legume resilience in water-limited environments. This study provides valuable insights for optimizing bioinoculant formulations to enhance crop performance under drought stress.
Climate change is a pressing global challenge that drives environmental crises, impacting ecosystems, biodiversity, human health, and food security (Abbass et al., 2022). Over the past 65 years, both natural and human-induced factors, including industrial activities and agriculture, have intensified its effects (Wu et al., 2020; Abbass et al., 2022). The agricultural sector, accounting for 30-40% of greenhouse gas (GHG) emissions, significantly contributes to global warming through practices such as excessive use of chemical fertilizers, mechanization, and fossil fuel consumption (Tubiello et al., 2021).
Climate change also disrupts hydrological cycles, leading to erratic rainfall, shrinking water bodies, and frequent droughts, which severely impact crop productivity, especially in water-scarce regions like Morocco. Situated between North Africa’s arid zone and Europe’s temperate climate, Morocco faces rising temperatures, decreasing rainfall, and increasing droughts, which threaten agricultural sustainability (Simonneaux et al., 2015; Woillez, 2019). Drought stress, in particular, hampers legume production in arid and semi-arid regions (Jagadish et al., 2016). Leguminous crops, vital for human nutrition and soil health, are especially vulnerable to such conditions. These crops form symbiotic relationships with N2-fixing bacteria, such as rhizobia, which enhance biological N2 fixation and improve soil fertility (Sprent et al., 2017).
The application of rhizobia as biofertilizers has proven effective in improving N2 fixation and soil fertility, particularly under stress conditions. Recent studies suggest that co-inoculation with native rhizobia and plant growth-promoting rhizobacteria (PGPR) significantly enhances drought tolerance in legumes. Notably, Rhizobium laguerreae has demonstrated remarkable potential in promoting plant growth under osmotic stress. Isolated from various leguminous crops across different countries (Saïdi et al., 2014; Taha et al., 2018), this species has shown beneficial effects on lentil growth under drought conditions when co-inoculated with native Bacillus sp. and Enterobacter aerogenes (Taha et al., 2022). Additionally, R. laguerreae has been shown to enhance productivity and phenolic composition in lettuce under saline conditions (Ayuso-Calles et al., 2020). These findings highlight the potential of R. laguerreae as a biofertilizer to enhance legume resilience and productivity in challenging environmental conditions.
The objective of this study is to evaluate the drought tolerance of three R. laguerreae strains, previously isolated from Lens culinaris root nodules (Taha et al., 2018), under controlled osmotic stress conditions. Specifically, the study aims to: a) Characterize the plant growth-promoting (PGP) traits of these strains, including auxin production, phosphate solubilization, and siderophore production, b) Assess their host range specificity with Pisum sativus, Phaseolus vulgaris, and Vicia faba, and c) Investigate their potential to form effective microbial mixed inoculum with other PGP rhizobacteria, such as Bacillus sp. and Enterobacter aerogenes, and evaluate their impact on drought resistance and legume productivity in water-limited environments.
Three strains of Rhizobium laguerreae LMR 575, LMR 597, and LMR 655, along with two rhizosphere bacteria, Bacillus sp. LMR 698 and Enterobacter aerogenesaer. LMR 696 were used in this study. These strains were isolated from lentil (Lens culinaris Medik) root nodules and rhizosphere, respectively, grown in various regions in Morocco (Taha et al., 2018). They were selected based on their performance in improving lentil growth, in single or combined inoculations, under drought stress conditions, as well as their ability to withstand osmotic stress in-vitro using PEG6000 as a stressor agent (Taha et al., 2022).
Auxin production of the selected strains was determined following Sarwar method (Sarwar et al., 1992). Freshly prepared bacterial cultures were inoculated in 15 ml of YEM medium amended with 50 μg mL-1 of L-tryptophane as a precursor for auxin production. Different concentrations of PEG6000 (5%, 10%, 20%, 30%, 35%) were added to induce osmotic stress. Bacterial cultures were then incubated at 28 ± 2 °C for three days at a shaking speed of 120 rpm. Two milliliters of bacterial cultures were collected every 24 hours to measure bacterial growth and auxin production. The amount of IAA production in each isolate was measured using the Salkowski reagent (Ehmann, 1977). After incubation, the cultures were centrifuged at 8000 rpm for 15 min at 4 °C, and the supernatant was mixed with Salkowski reagent then incubated in the dark for 30 min for color development. Absorbance was measured at 530 nm and the IAA concentration was estimated by using a standard IAA curve with pure IAA concentrations ranging from 0 to 100 µg mL-1 (Gordon and Weber, 1951).
The strains’ capacity to produce siderophores was tested using the CAS-shuttle assay method developed by Schwyn and Neilands (1987). Bacterial cultures were grown in iron-deficient minimal medium (El Attar et al., 2019), supplemented with the same PEG600 concentrations as mentioned above. After seven days of incubation, the bacterial cultures were centrifuged to remove the pellet. An equal volume of supernatant and CAS reagent was then mixed, and the optical absorption at 630 nm was measured. Siderophore production was estimated using the formula of Payne (1993).
The inorganic phosphate (P) solubilization capacity was determined by using Pikovskaya’s medium (PVK) (Pikovskaya, 1948) supplemented with 0.05 g of Moroccan rock phosphate as a sole source of P. PEG6000 was added to the medium using the same concentrations mentioned above. Bacterial cultures were incubated at 28 °C for 3 days with shaking. After incubation, the cultures were centrifuged at 12 000 rpm for 15 min to collect the supernatant. Soluble P content in the supernatant was estimated following the Vanadate-molybdate method (Tandon et al., 1968). Absorbance was measured at 405 nm and soluble phosphorus in the medium was calculated from the standard curve of P concentration using KH2PO4.
To determine the host range of R. laguerreae strains, four grain legume hosts were used: Lentil (Lens culinars), Pea (Pisum sativum), faba bean (Vicia faba), and common bean (Phaseolus vulgaris). The seeds were kindly provided by INRA (Institut National de Recherche pour l’Agriculture) Institute of Rabat, Morocco.
Seeds of P. sativum, V. faba, and Phaseolus vulgaris were surface sterilized by immersion in 70% ethanol for 2 minutes, followed by thorough rinsing with sterile distilled water to remove any ethanol residues. The seeds were then immersed in a 20% sodium hypochlorite solution for 20 minutes and rinsed again with sterile distilled water to eliminate any remaining traces of salt. Lentil seed sterilization was done following the same protocol described by Taha et al. (2018). After surface sterilization, all seeds were germinated in 0.7% agar plates at 28 °C in the dark for 2 days.
Five germinated seeds were transferred to plastic pots containing a mixture of an equal quantities of sterilized sand and surface-applied beads (Haweison and dilworth, 2016), then thinned to 3 after successful seedling establishment.
R. laguerreae strains were grown in liquid YEM medium at 28°C for 24h and bacterial density was standardized to 108 CFU mL-1. Seedlings in each pot were inoculated with 1 mL of rhizobia culture.
Two sets of control plants were settled: non-inoculated non-fertilized seedlings (Control N0) and non-inoculated fertilized seedling supplied with mineral nitrogen (0.5 g L–1 KNO3) (Control N+). The experiment was carried out with three replicates using a Randomized Complete Block (RCB) design and all pots were watered twice a week with an N-free nutrient solution.
Based on the results of host range experiment and the evaluation of PGP traits under osmotic stress, R. laguerreae LMR 597 and LMR 655 and E. aerogenes LMR696 strains were selected based on their performance. The three strains were used as a mixed inoculum to further evaluate their effect of plant growth and development under water deficiency stress. The leguminous plants P. sativum and V. faba that had established a symbiosis with R. laguerreae strains were used in this experiment.
To ensure compatibility between rhizobial and PGPR strains intended for mixed inoculation, potential antagonistic interactions were assessed in-vitro following the procedure outlined by Mikiciński et al. (2016). The strains were co-cultured on nutrient agar plates, and zones of inhibition were monitored to detect antagonistic effects. Experiments were performed in triplicate to ensure reproducibility.
The soil used in this experiment was non-sterilized agricultural soil collected from a local site. The soil’s pH was 7.64 ± 0.05, the cation exchange capacity (CEC) was found to be 0.21 ± 0.02 mS/cm, the potassium content was 120.02 ± 12.50 ppm, while phosphorus was present at 58.45 ± 0.71 ppm. Nitrogen was measured at 0.038 ± 0.004%, and the organic matter content was 2.88 ± 0.91%. The dry matter content was 91.13 ± 0.26%, and magnesium was present at 7.25 ± 0.04%. The carbon content in the soil was measured at 1.81 ± 0.005%, with sodium present at 1.29 ± 0.06 m eq 100 g-1.
A preliminary irrigation test was conducted to determine the soil’s maximum water retention capacity, from which 30% and 70% moisture levels were selected to represent drought stress and well-watered conditions, respectively. The experimental setup included a total of 72 pots, randomly distributed across three blocks, with six replicates per treatment. The six treatments comprised: inoculated plants under drought stress, inoculated plants with normal irrigation, non-inoculated plants under drought stress, non-inoculated plants with normal irrigation, and two non-inoculated controls, negative (N0) and positive (N+), that was supplemented with N2 fertilizer (0.5 g L-1 KNO3-). Both were subjected to the same irrigation regime. Non-inoculated controls received no microbial treatment, while inoculated plants were treated with the bacterial mixed inoculum.
The plants were initially watered to meet their growth requirements, and drought stress was applied starting in the third week of growth, continuing until the flowering stage. After 8 weeks of growth, all plants were harvested for further analysis of growth parameters, including root and shoot biomass, chlorophyll content, and drought tolerance indicators.
The multi-strain mixed inoculum was prepared by combining equal proportions of fresh cultures of each strain, which were separately grown in liquid YEM medium. The mixed inoculum suspension was then adjusted to a final concentration of 108 cfu mL–1 and applied immediately after transplantation, with a follow-up application after 15 weeks. The experiment was conducted following the RCB design, with six replicates per treatment.
Six weeks into the experiment, the plants were harvested, and the measurements of nodule number, shoot, and root system lengths were measured. Plants shoot and root parts were oven-dried at 70°C for 48 hours and dry weights were recorded. For chlorophyll (a), (b), and carotenoid measurements, leaf segments of each treatment were immersed in 4 ml of 80% v/v acetone solution and incubated for 48 hours at 4°C (Amine-Khodja et al., 2022). Photosynthetic pigments were quantified according to Lichtenthaler and Wellburn’s (1983) equation.
The proline content of all tested plants was extracted by using a cold extraction procedure by mixing plant samples with ethanol and left to incubate overnight at 4°C, then they were centrifuged for 5 min at 8000 rpm to retrieve the pellet and optical density of the supernatant was measured at 520 nm (Gibon et al., 2000). The detection of proline was related to a standard curve prepared as described by Carillo et al. (2011).
Anthocyanin biosynthesis was measured following the protocol of Mustilli et al., 1999. Fresh shoot biomass was incubated in a 1% HCl methanol solution in the dark for two nights at +4°C. After incubation, chloroform and distilled water were added to the supernatant retrieved by centrifugation. The absorbance of the supernatant was measured at 535 and 650 nm, using the extraction solution as the blank. Anthocyanin content was calculated as the difference in absorbance (A535 - A650) per mg of fresh material.
Total soluble sugar content was estimated using the method described by DuBois et al. (1951). Plant material was homogenized with 80% ethanol and incubated for two nights. After centrifugation, the collected supernatant was added to a reaction mixture containing 5% phenol and 96% sulfuric acid. The mixture was then incubated at 30°C for 20 minutes. The optical density of the resulting solution was measured at 490 nm, and the total soluble sugar content was estimated per gram of fresh weight, referring to a standard curve prepared with varying concentrations of glucose.
Protein production was calculated by grinding fresh leaves with a cooled phosphate buffer (pH=7.8). The supernatant obtained after centrifugation and pellet removal was incubated with Bradford reactant at 37°C for 5 min. The mixture absorbance was measured at 595 nm after incubation, and the concentration of soluble protein was assessed by using the Bovine serum albumin standard curve following the method described by Bradford (1976).
All statistical analyses were done using R version 4.3.2. A Tukey Honest Significant Differences (TukeyHSD) test was used to provide confidence intervals for the various pairwise comparisons after an Analysis of Variance (ANOVA) was completed. The ggplot2 package version 3.5.1 was used to create all the plots, and multcompLetters4() from the multcompView package version 0.1.10 was used to add the letters of significance.
Bacterial growth was assessed simultaneously with the measurement of auxin production. The results presented in Figure 1 revealed the negative effect of water deficiency on bacterial growth, which showed a graduate decrease as PEG6000 concentrations increased. R. laguerreae LMR575, LMR597, and Bacillus sp. LMR 698, strains exhibited moderate growth compared the control, at a concentration of 30% maintaining an optical density above 0.5 at 620 nm. In contrast, R. laguerreae LMR 655 was able to tolerate up at 20% PEG6000. Meanwhile, E. aerogenes LMR 696 was highly sensitive to osmotic stress. Notably, all strains were found to be vulnerable to the high osmotic pressure applied by PEG6000 (35%).
Figure 1. Effect of osmotic stress (PEG6000 concentrations) on growth of R. laguerreae strains LMR575, LMR597, and LMR655, Enterobacter aerogenes LMR696, and Bacillus sp. LMR698. Different letters represent significant statistical differences using Tukey-HSD tests at (p < 0.05).
The maximum amount of auxin produced by bacteria in YEM medium supplemented with 50 μg mL-1 of L-tryptophan, was reached after 72 hours of incubation (Figure 2). The bacterial strains exhibited a greater capacity to produce auxin under normal conditions compared to stressful ones. For R.laguerreae LMR 597, the auxin production dropped from 24.22 µg/ml in normal conditions to 12.03 µg mL-1 at PEG6000 (35%). In contrast, auxin production by Bacillus sp. LMR 698 and R. laguerreae LMR 655 was only observed under normal conditions, with concentrations of 25.24 and 12.87 µg mL-1, respectively. Additionally, R. laguerreae LMR 575 exhibited the highest auxin synthesis after 72 hours of incubation, while E. aerogenes LMR 696 showed a gradual decrease in auxin synthesis as the PEG concentration increased. It is noteworthy, that except for R. laguerreae LMR 597, the activity of all strains, was significantly inhibited at PEG6000 (35%), with auxin synthesis falling below 5 µg mL-1.
Figure 2. Effect of osmotic stress (PEG6000 concentrations) on auxin production of R. laguerreae strains LMR575, LMR597, and LMR655, Enterobacter aerogenes LMR696, and Bacillus sp LMR698. Different letters represent significant statistical differences using Tukey-HSD tests at (p < 0.05).
Under normal conditions, E.aerogenes LMR 696 exhibited the highest level of siderophore production of 82%, followed by R. laguerreae LMR 597 with 27%, Bacillus sp. LMR 698 with 18%, and finally R. laguerreae LMR 655 with 17%. However, these strains showed no siderophore activity under varying water potential levels. Conversely, R. laguerreae LMR 575 showed a high level of siderophores production up to 88% at 5% PEG6000, but this production declined significantly with the increase in PEG6000 concentrations, reaching 16.78% at 35% of PEG6000 (Figure 3).
Figure 3. Effect of osmotic stress (PEG6000 concentrations) on siderophore production of R. laguerreae strains LMR575, LMR597, and LMR655, Enterobacter aerogenes LMR696, and Bacillus sp LMR698. Different letters represent significant statistical differences using Tukey-HSD tests at (p < 0.05).
All the bacterial strains tested demonstrated the ability to solubilize inorganic phosphate (Figure 4). The highest level of production was recorded by R. laguerreae LMR 655, which produced 113.85 µg mL-1. In comparison, R. laguerreae LMR 575 and LMR 597 showed moderate production levels of 48.5 and 61.57 µg mL-1, respectively. Conversely, E.aerogenes LMR 696 and Bacillus sp. LMR 698 exhibited lower solubilization rates, producing 22.5 and 20.5 µg mL-1, respectively. The ability of the studied strains to solubilize phosphate was significantly diminished compared to the control, and this decrease was correlated with increasing PEG concentrations. While R. laguerreae LMR 597 and LMR 655 could produce PO4²- at a lower level of 30 µg mL-1 at 20% and 30% PEG6000, respectively. In contrast, R. laguerreae LMR 575, E. aerogenes LMR 696, and Bacillus sp. LMR 698 showed no phosphate solubilization capacity starting at 10% PEG6000.
Figure 4. Effect of osmotic stress (PEG6000 concentrations) on phosphate solubilization of R. laguerreae strains LMR575, LMR597, and LMR655, Enterobacter aerogenes LMR696, and Bacillus sp. LMR698. Different letters represent significant statistical differences using Tukey-HSD tests at (p < 0.05).
The studied R. laguerreae strains, LMR 575, LMR 597, and LMR 655, were individually inoculated into L. culinaris, P. sativum, V. faba, and Phaseolus vulgaris seeds to determine their host range. The results indicated that all strains were capable of nodulating their host plant: L. culinaris. R. laguerreae LMR 597 and LMR 655 strains were also able to establish symbiosis with P. sativum and V. faba while no strain was able to form nodules in P. vulgaris (Supplementary Figure S1).
The in-vitro compatibility test revealed that there are no antagonistic interactions between the selected strains.
Under non-stress conditions with inoculation, P. sativum and V. faba demonstrated high nodulation, with P. sativum producing over 80 nodules per plant and V. faba yielding an average of 67 nodules with minimal variation (SD = 0.52), indicating consistent nodule formation. In contrast, both species showed no nodulation under non-inoculated or KNO3-supplemented treatments, regardless of stress conditions. Under stress conditions, however, inoculated plants of P. sativum and V. faba still formed nodules, though in reduced numbers (22.67 and 41.33 nodules per plant, with SD values of 0.90 and 0.45 respectively) (Table 1).
As shown in Figure 5A, B, V. faba and P. sativum exhibited distinct responses in root and shoot length under various treatments. In V. faba, the non-stressed, inoculated plants showed the highest shoot length (84.33 cm), which was significantly greater than the non-stressed plants supplemented with KNO3- and the stressed, non-inoculated plants, both of which did not differ significantly from the other treatments. Root length in V. faba remained relatively consistent across all treatments. In P. sativum, root length was significantly higher in stressed, inoculated plants (28 cm) compared to the stressed and non-stressed plants treated with KNO3- (19 cm and 22 cm, respectively). The shoot length of stressed, non-inoculated P. sativum plants was notably lower (72 cm), differing significantly from stressed, inoculated plants and non-stressed plants supplemented with KNO3- or inoculated, which all showed higher shoot lengths averaging around 113 cm.
Figure 5. Shoot and root length under different treatments for two leguminous species, (A) Vicia faba and (B) Pisum sativum. Different letters represent significant statistical differences using Tukey-HSD tests at (p < 0.05).
The chlorophyll and carotenoid content in V. faba and P. sativum demonstrated a clear response to inoculation and stress treatments. In both species, the non-stressed, inoculated plants exhibited the highest concentrations of chlorophyll (a), chlorophyll (b), total chlorophyll, and carotenoids, significantly surpassing non-inoculated and KNO3-supplemented plants. Specifically, non-stressed inoculated plants had a chlorophyll (a) concentration of 144.39 mg g-1, which was significantly higher than both non-inoculated and KNO3-supplemented plants. Regarding chlorophyll (b), inoculated plants under non-stressed conditions showed a concentration of 35.12 mg g-1, again surpassing non-inoculated and stressed non-inoculated plants. Under drought stress, inoculation mitigated the reduction in chlorophyll and carotenoid content more effectively than KNO3- supplementation, with stressed inoculated plants in V. faba maintaining higher chlorophyll (a) and total chlorophyll levels (Figure 6A). In P. sativum, stressed inoculated plants similarly retained relatively higher chlorophyll (a) and carotenoid concentrations compared to KNO3- treated plants. For total chlorophyll content, non-stressed inoculated plants displayed a significantly high concentration of 179.51 mg g-1, while under stress, inoculation increased total chlorophyll concentration to 84.90 mg g-1 compared to KNO3-supplemented plants. Interestingly, stressed non-inoculated plants retained relatively high chlorophyll (a) concentrations (115.85 mg g-1) compared to KNO3-treated plants (Figure 6B).
Figure 6. Production of photosynthetic pigments under different treatments for two leguminous species, (A) Vicia faba and (B) Pisum sativum. Different letters represent significant statistical differences using Tukey-HSD tests at (p < 0.05).
Proline production in P. sativum and V. faba under drought stress is shown in Figure 7. In both leguminous species, the level of proline produced under drought stress in plants treated with a mixed inoculum is higher with values of 48 and 38 mg g-1 for P. sativum and V. faba respectively, differing significantly with the other treatment. In V. faba (Figure 7A), no proline production was detected in plants under other treatment; Meanwhile the production of proline in P. sativum (Figure 7B) was not only related to the stress response but also to inoculation and KNO3 addition, where there was no significant difference in the amount of proline produced in stressed and non-stressed plant amended with KNO3 and non-stressed plant inoculated with the mixed inoculum. Whilst the lowest proline production was observed in non-stressed non-inoculated plants (6.5 mg g-1) and stressed non-inoculated plants (13.5 mg g-1).
Figure 7. Proline production under different treatments for two leguminous species, (A) Vicia faba and (B) Pisum sativum. Different letters represent significant statistical differences using Tukey-HSD tests at (p < 0.05).
Anthocyanins, pigments produced in response to stress, were also measured in our study (Figure 8). The results showed that concentrations did not exceed 1 mg g-1. In V. faba, anthocyanin synthesis did not appear to be associated with either stress response or the effect of inoculation (Figure 8A). Conversely, in P. sativum, the highest anthocyanin concentration was observed in the non-inoculated, stressed plants, reaching 0.65 mg g-1 which is significantly higher than in the inoculated plants and those supplemented with KNO3, where concentrations decreased to 0.176 and 0.116 mg g-1, respectively (Figure 8B).
Figure 8. Anthocyanin biosynthesis under different treatments for two leguminous species, (A) Vicia faba and (B) Pisum sativum. Different letters represent significant statistical differences using Tukey-HSD tests at (p < 0.05).
The effect of inoculation on plant physiological responses varies between leguminous species. Examining the behavior of V. faba (Figure 9A), we observed a highly significant accumulation of soluble sugar in stressed inoculated plants, reaching 3.29 mg g-1, compared to 1.57 mg g-1 in stressed non-inoculated and 1.60 mg g-1 in the non-inoculated non stressed plants. Broadly speaking, both KNO3 treatment and inoculation helped maintain soluble sugar levels in stressed plants similarly to those under normal condition treated with a mixed inoculum and KNO3. Regarding P. sativum (Figure 9B), the highest significant concentration of soluble sugar was observed in the stressed non-inoculated plants reaching 3.44 mg g-1, compared to the other treatments. Simultaneously no significant difference was detected between the plants irrigated with KNO3 and those inoculated under the same stress condition, where the sugar concentration decreased to a range of 2.14 and 1.98 mg g-1 respectively.
Figure 9. Total soluble sugar production under different treatments for two leguminous species, (A) Vicia faba and (B) Pisum sativum. Different letters represent significant statistical differences using Tukey-HSD tests at (p < 0.05).
The protein content in V. faba plants (Figure 10A) was impacted by the applied stress. The non-inoculated plants exhibited the lowest protein content of 25.74 mg g-1, which differed significantly from the plants amended with KNO3, reaching a concentration of 49.03 mg g-1, whilst under normal conditions, no significant difference was observed among the applied treatments. Conversely, in P. sativum plants (Figure 10B), a significant effect of the inoculation was observed in the non-stressed plants achieving a concentration of 66.79 mg g-1, differing significantly from the plants supplemented with KNO3 (52.70 mg g-1) and the control (34.71 mg g-1). Meanwhile, the inoculated plants and those irrigated with KNO3 under drought stress displayed protein concentrations of 40.52 and 43.23 mg g-1 respectively, which were significantly higher than the stressed non-inoculated plants.
Figure 10. Proteins production under different treatments for two leguminous species, (A) Vicia faba and (B) Pisum sativum. Different letters represent significant statistical differences using Tukey-HSD tests at (p < 0.05).
This study builds upon previous research that focused on the isolation and identification of Rhizobium laguerreae as the primary nitrogen-fixing symbiont of Lens culinaris (lentil) across various regions in Morocco (Taha et al., 2018). Subsequently, the highest-performing strains were selected based on phenotypic screening (including pH, temperature, salinity, and osmotic stress tolerance), PGP potential, and symbiotic effectiveness. Settling on the final collection, which included three R. laguerreae strains LMR 575, LMR 597, and LMR 655 and two native PGPRs, Bacillus sp. LMR 698 and Enterobacter aerogenes LMR 696, these strains were then used in combinations for co-inoculation experiments with L. culinaris under stress conditions, generating promising results (Taha et al., 2022). These outcomes led us to question the ability of these bacteria to maintain their PGP traits under osmotic stress applied in-vitro using PEG6000.
Primarily, we assessed their growth and IAA production simultaneously, as several studies have been conducted in this area. The research conducted by Dhull et al., (2018) on Rhizobium strains isolated from the nodules of Cymopsis tetragonoloba in semi-arid regions of India revealed that all the strains tolerated up to 10% of PEG6000 with some able to grow at concentrations of 20% and 30%. Similarly, the isolated strain Rhizobium ‘QHCD11’ from (Vicia faba L.) in the arid region of China, that showed improved performance at 20% (Li et al., 2023). Moreover, out of a rhizobacteria collection isolated from a degraded soil of the north zone in Ethiopia, 10 isolates were able to tolerate an increased concentration of 40% PEG. However, bacteria that exhibit growth above 0.4 OD (Optical Density) are considered to be at the threshold for drought tolerance (Alikhani and Mohamadi, 2010). Likewise, our tested rhizobacteria could be considered drought-tolerant, with a high capacity to withstand water deficit, especially as R. laguerreae strains LMR575, LMR597 and Bacillus sp. LMR698 maintained stable population sizes at a PEG6000 concentration of 30%.
Previous studies have shown that bacteria tolerant to drought stress are able to display other PGP activities such as phytohormone production, nutrient availability in soil through phosphate solubilization and siderophore synthesis (El Attar et al., 2019; Zia et al., 2021, Taha et al., 2022). Corroborating our results, all the tested bacteria exhibited moderate performance in their PGP traits, which were influenced by the concentrations of PEG6000 applied, as obtained from the study of Sijilmassi et al. (2020). In their study, it was found that the production of IAA and phosphate solubilization ability of Rhizobium strains under drought stress decreased sharply with the severe application of osmotic stress. Furthermore, a study carried out on the behavior of three bacterial strains, Proteus mirabilis R2, Pseudomonas balearica RF-2, and Cronobacter sakazakii RF-4, under drought stress, revealed that none of the strains exhibited PGP activity at the 10% PEG6000 stress level.
According to the results of our bacterial kinetics and their PGP potential, we observed that their activities are strongly dependent on their growth, both of which are negatively affected by elevated concentrations of PEG6000. This correlation can be explained by the disruption of the genetic regulatory mechanism, Quorum Sensing, which controls many bacterial functions, all of which are closely linked to bacterial population size (Williams, 2007).
This variability in PGP responses of each R. laguerreae strain was also reflected in their distinct symbiotic effectiveness with the tested leguminous species. However, our findings do not align with most studies, which isolated several species belonging to the genera Rhizobium, Sinorhizobium, Mesorhizobium, Bradyrhizobium, Azorhizobium, and Agrobacterium from the nodules of Phaseolus vulgaris (Martínez-Romero, 2003; El Attar et al., 2019). Furthermore, Flores-Félix et al. (2020) were the first to isolate R. laguerreae from P. vulgaris in a region where lentils were traditionally cultivated. These insights, and the unexpected loyalty of R. laguerreae LMR 575 to Lens culinaris, brought up several questions about the infection mechanism, the specificity of nodulation genes, and the whole-genome characteristics of these strains.
Combining the results mentioned above, the choice of mixed inoculum strains as inoculum was based on the idea of maximizing the benefits of the synergistic effects established by non-symbiotic PGPR and Rhizobium. In our case, this was a combination of R. laguerreae LMR 597 and LMR 655, which demonstrated the ability to produce auxin and solubilize inorganic phosphate, respectively, while establishing symbiosis with P. sativum and V. faba. Additionally, E. aerogenes LMR 696 was chosen for its siderophore production. This integration of symbiotic and non-symbiotic bacteria was outlined by various research works, which suggest that non-rhizobial strains could strengthen the effectiveness and efficiency of rhizobium with their plant host (Ben Gaied et al., 2024). These studies also highlight its potential as a promising biofertilizer that reduces the excessive use of chemical inputs, assists in mitigating challenging abiotic stresses such as drought through the induction of systemic tolerance (IST), and establishes beneficial interactions with leguminous plants (Yang et al., 2009; Khan et al., 2018).
In addition to its damaging effects on plant growth and nodule formation, drought stress also alters the physico-chemical properties of the soil, leading to infertility, susceptibility to disaggregation and deficiencies in essential minerals, such as nitrogen and phosphorus, that play a crucial role in plants physiological processes, including pigments synthesis, respiration and energy transfer (Wang et al., 2014; Dutta et al., 2018; Stavi et al., 2021). Thus, the application of symbiotic nitrogen fixers, phosphate solubilizers, IAA and siderophore producers, aimed to alleviate drought stress by promoting root elongation, regulating physiological and biochemical processes, enhancing nutrient availability in the soil, and improving biomass production (Deinum et al., 2016; Jabborova et al., 2021; Uzma et al., 2022).
The reduction in nodule numbers was the first clear sign of the severe stress applied to the inoculated plants. The absence of nodules in the non-inoculated plants and non-inoculated + KNO3 treatments in both leguminous species could be attributed to the absence of rhizobia in the soil or their inability to establish symbiosis with P. sativum and V. faba due to lower population sizes and effectiveness (Allito et al., 2021). Interestingly, the presence or absence of nodules did not correlate with the observed response in shoot and root growth of V. faba, nor did it indicate the effects of water deficiency and availability. Similarly to as shown by Ulzen (2018), where an increase in shoot dry weight following rhizobia inoculation in soybean was not noticed. This finding suggests that root morphology changes under water stress are species-specific (Mishra et al., 2020). On the other hand, the beneficial effect of the mixed inoculum was observed in the roots of P. sativum, where plants under stress exhibited the same performance as non-stressed, inoculated plants and the supplied with KNO3. This supports the role of the mixed inoculum in modulating root growth and architecture via IAA production, as observed in Rhodobacter sphaeroides KE149, a drought-tolerant, IAA-releasing bacterium that enhanced the growth of Vigna angularis var. nipponensis cv. Arari (Kang et al., 2020).
Chlorophyll (a) and (b) are the primary photosynthetic pigments in plants, directly facilitating photosynthesis, while carotenoid pigments are involved in dissipating excess excitation light energy (Khatun et al., 2021). Under drought stress, photosynthesis is one of the first physiological parameters to be affected, leading to a reduction in leaf water content and stomatal closure, which in turn causes photo-oxidation and degradation of chlorophyll pigments (Anjum et al., 2011; Yang et al., 2021). In V. faba, the mixed inoculum exhibited a promising result in enhancing chlorophyll content under normal condition as well as the application of KNO3, suggesting that application of this inoculum in normal irrigation conditions as biofertilizers rather than exogenous nitrogen could increase the absorption efficiency of water and nutrients in V. faba which are important for photosynthesis, which is an imperative process that improve yield trait (Mansour et al., 2021). Interestingly, stressed V. faba plants that were not inoculated produced higher amounts of photosynthetic pigments compared to those under stress, inoculated, and treated with KNO3. This observation aligns with findings from several experiments conducted under drought stress, where some cultivars of black gram (Vigna mungo L. Hepper) increased their chlorophyll content in response to water stress (Ashraf and Karim, 1991). Similarly, a chickpea variety (ILC482) exhibited higher chlorophyll (a) levels compared to other varieties during the vegetative stage (Mafakheri et al., 2010). For P. sativum, the observed non-significant differences in chlorophyll pigment production align with the findings of Lucas et al. (2023), who reported no chlorophyll degradation and an increase in all pigments in inoculated plants under stress. This variability in chlorophyll synthesis under osmotic stress can be attributed to the duration and severity of drought stress, along with differences in crop type, where some tolerant genotype of Phaseolus vulgaris, Glycine max Merr., Hordeum vulgare, and Zea mays can maintain their chlorophyll content in high level under drought stress (Monteoliva et al., 2021; Khatun et al., 2021). Chlorophyll synthesis in P. sativum and V. faba may be associated with several mechanisms that enable these drought-tolerant genotypes to preserve chlorophyll under such conditions, involving a combination of transcriptional regulation, enzymatic activity, and the stability of photosystem components. The study by Avramova et al. (2015) on maize leaf response to drought stress demonstrated a transcriptional up-regulation of the photosynthetic machinery throughout the entire leaf growth zone, with increased mRNA levels of photosynthesis-related transcripts and genes encoding enzymes involved in the synthesis of tetrapyrrole, which likely contributes to the maintenance or even enhancement of chlorophyll content. Additionally, it has been shown that certain components of the photosynthetic apparatus, particularly PSI and PSII, demonstrate notable resistance to water stress, further supporting the preservation of chlorophyll and the overall resilience of the photosynthetic system under water-deficient conditions (Nikolaeva et al., 2010).
Proline, soluble sugars, and proteins are important plant cellular osmolytes produced under stress conditions, they maintain normal cellular morphology, protect cell membrane stability, eliminate reactive oxygen species (ROS), and enhance plant adaptation (Etesami and Maheshwari, 2018). When plants are subjected to drought stress, these metabolites accumulate to reduce osmotic pressure (Ghosh et al., 2021; Jing et al., 2023). For proline activity, both plant species (P.sativum and V.faba) accumulated high concentrations of this osmolyte under stress when inoculated with the mixed inoculum. This finding aligns with results from two separate studies on maize cultivated under water deficiency, where the application of Pseudomonas putida strain GAP-P45 and Bacillus thuringiensis enhanced proline accumulation (Sandhya et al., 2010; Armada et al., 2014). Similarly, Paenibacillus polymyxa promoted proline production in tomato cultivars (Ghosh et al., 2019), and Mesorhizobium ciceri spp. increased proline accumulation in chickpea under drought stress (Abdela et al., 2020). Co-inoculation with R. leguminosarum and P. putida was also associated with proline and soluble sugar accumulation compared to the control (Mansour et al., 2021), and a mixed inoculum applied to maize under stress significantly enhanced proline and soluble sugar levels in the seedlings (Wang et al., 2018). Likewise, inoculated V. faba plants under stress showed increased accumulation of proline, soluble sugars, and protein. However, P. sativum plants exhibited high accumulation of only protein and proline, with no significant increase in soluble sugars under stress. These differences in osmolyte accumulation responses constitute a debate among researchers. Some consider that higher proline accumulation is associated with less-tolerant species, while others have reported that increased proline concentrations are a sign of greater drought tolerance mechanisms (Arteaga et al., 2020).
Globally, the distinction between stress responses and stress tolerance is often blurred, leading to misunderstandings in the explanation and interpretation of results related to osmolyte production under stress. Not all responses are directly linked to tolerance, even though stress tolerance mechanisms are grounded in specific stress responses. The accumulation of osmolytes can be viewed either as a response mechanism or as part of the stress tolerance strategy, depending on the species and possibly the specific plant parts, such as shoots and roots.
Our study highlights that selecting strains based on their growth and PGP traits under drought stress is a crucial factor, alongside the choice of host plant. Interspecific interactions between the mixed inoculum and P. sativum and V. faba were evaluated through various biochemical parameters. Inoculation enhanced drought tolerance in V. faba through multiple mechanisms, while most responses in P. sativum were more closely related to the plant’s metabolic characteristics. Under normal conditions, this inoculum also showed promising results in boosting plant growth and yield. Our findings contribute to a deeper understanding of how combinations of symbiotic strains and non-rhizobia influence leguminous symbiosis and drought response, supporting the potential of inoculant applications as an effective strategy to combat drought, improve tolerance, and enhance yields in the context of climate change.
The original contributions presented in the study are included in the article/Supplementary Material. Further inquiries can be directed to the corresponding authors.
AH: Conceptualization, Writing – original draft, Data curation, Investigation, Methodology. IE: Conceptualization, Writing – review & editing, Supervision. NM: Data curation, Formal Analysis, Writing – review & editing, Software. SE: Investigation, Writing – review & editing. EA-O: Data curation, Investigation, Writing – review & editing. MB: Conceptualization, Data curation, Writing – review & editing. SO: Validation, Writing – review & editing. BB: Validation, Writing – review & editing. JA: Supervision, Writing – review & editing. LS: Funding acquisition, Project administration, Resources, Supervision, Writing – review & editing. KT: Conceptualization, Funding acquisition, Project administration, Resources, Supervision, Validation, Visualization, Writing – review & editing, Methodology.
The author(s) declare that no financial support was received for the research, authorship, and/or publication of this article.
The authors thank the partnership for research and innovation in the Mediterranean area (PRIMA) program for its research support (ECOBOOST project).
The authors declare that the research was conducted in the absence of any commercial or financial relationships that could be construed as a potential conflict of interest.
The author(s) declare that no Generative AI was used in the creation of this manuscript.
All claims expressed in this article are solely those of the authors and do not necessarily represent those of their affiliated organizations, or those of the publisher, the editors and the reviewers. Any product that may be evaluated in this article, or claim that may be made by its manufacturer, is not guaranteed or endorsed by the publisher.
The Supplementary Material for this article can be found online at: https://www.frontiersin.org/articles/10.3389/fpls.2025.1528923/full#supplementary-material
Abbass, K., Qasim, M. Z., Song, H., Murshed, M., Mahmood, H., Younis, I. (2022). A review of the global climate change impacts, adaptation, and sustainable mitigation measures. Environ. Sci. pollut. Res. 29, 42539–42559. doi: 10.1007/s11356-022-19718-6
Abdela, A. A., Barka, G. D., Degefu, T. (2020). Co-inoculation effect of Mesorhizobium ciceri and Pseudomonas fluorescens on physiological and biochemical responses of Kabuli chickpea (Cicer arietinum L.) during drought stress. Plant Physiol. Rep. 25, 359–369. doi: 10.1007/s40502-020-00511-x
Allito, B. B., Ewusi-Mensah, N., Logah, V., Hunegnaw, D. K. (2021). Legume-rhizobium specificity effect on nodulation, biomass production and partitioning of faba bean (Vicia faba L.). Sci. Rep. 11 (1), 3678. doi: 10.1038/s41598-021-83235-8
Alikhani, H. A., Mohamadi, L. (2010). Assessing tolerance of rhizobial lentil symbiosis isolates to salinity and drought in dry land farming conditions. J. Agric. Sci. 10:165. doi: 10.35248/2329-6682.21.10.165
Amine-Khodja, I. R., Boscari, A., Riah, N., Kechid, M., Maougal, R. T., Belbekri, N., et al. (2022). Impact of two strains of Rhizobium leguminosarum on the adaptation to terminal water deficit of two cultivars Vicia faba. Plants 11, 515. doi: 10.3390/plants11040515
Anjum, S. A., Xie, X. Y., Wang, L. C., Saleem, M. F., Man, C., Lei, W. (2011). Morphological, physiological and biochemical responses of plants to drought stress. Afr. J. Agric. Res. 6 (9), 2026–2032. doi: 10.5897/AJAR10.027
Armada, E., Roldán, A., Azcon, R. (2014). Differential activity of autochthonous bacteria in controlling drought stress in native Lavandula and Salvia plants species under drought conditions in natural arid soil. Microb. Ecol. 67, 410–420. doi: 10.1007/s00248-013-0326-9
Arteaga, S., Yabor, L., Díez, M. J., Prohens, J., Boscaiu, M., Vicente, O. (2020). The use of proline in screening for tolerance to drought and salinity in common bean (Phaseolus vulgaris L.) genotypes. Agronomy 10, 817. doi: 10.3390/agronomy10060817
Ashraf, M., Karim, F. (1991). Screening of some cultivars/lines of blackgram (Vigna mungo (L.) Hepper) for resistance to water stress. 68 (1), 57–62.
Avramova, V., AbdElgawad, H., Zhang, Z., Fotschki, B., Casadevall, R., Vergauwen, L., et al. (2015). Drought induces distinct growth response, protection, and recovery mechanisms in the maize leaf growth zone. Plant Physiol. 169, 1382–1396. doi: 10.1104/pp.15.00276
Ayuso-Calles, M., García-Estévez, I., Jiménez-Gómez, A., Flores-Félix, J. D., Escribano-Bailón, M. T., Rivas, R. (2020). Rhizobium laguerreae improves productivity and phenolic compound content of lettuce (Lactuca sativa L.) under saline stress conditions. Foods 9, 1166. doi: 10.3390/foods9091166
Ben Gaied, R., Sbissi, I., Tarhouni, M., Brígido, C. (2024). Bacterial endophytes from Legumes native to arid environments are promising tools to improve Mesorhizobium–chickpea symbiosis under salinity. Biology 13 (2), 96. doi: 10.3390/biology13020096
Bradford, M. M. (1976). A rapid and sensitive method for the quantitation of microgram quantities of protein utilizing the principle of protein-dye binding. Anal. Biochem. 72, 248–254. doi: 10.1016/0003-2697(76)90527-3
Carillo, P., Parisi, D., Woodrow, P., Giovanni, P., Giuseppina, M., Maria, G. A., et al. (2011). Salt-induced accumulation of glycine betaine is inhibited by high light in durum wheat. Funct. Plant Biol. 38 (2), 139–150. doi: 10.1071/FP10177
Deinum, E. E., Kohlen, W., Geurts, R. (2016). Quantitative modelling of legume root nodule primordium induction by a diffusive signal of epidermal origin that inhibits auxin efflux. BMC Plant Biol. 16, 1–14. doi: 10.1186/s12870-016-0935-9
Dhull, S. D., Sheoran, H. S., Kakar, R., Gera, R. (2018). Selection of temperature stress tolerance of Rhizobium isolated from root nodules of clusterbean [Cyamopsis tetragonoloba (L.) Taub.] growing in arid and semi-arid regions of Haryana, India. Ann. Biol. 34 (1), 24–27.
Dubois, M., Gilles, K. A., Hamilton, J. K., Rebers, P. A., Smith, F. A. J. N. (1951). A colorimetric method for the determination of sugars. Nature 168 (4265), 167–167. doi: 10.1038/168167a0
Dutta, T., Neelapu, N. R. R., Wani, S. H., Challa, S. (2018). Response of pulses to drought and salinity stress response: A physiological perspective. Pulse Improve.: Physiol. Mol. Genet. Perspect., 77–98. doi: 10.1007/978-3-030-01743-9_4
Ehmann, A. (1977). The Van Urk-Salkowski reagent—a sensitive and specific chromogenic reagent for silica gel thin-layer chromatographic detection and identification of indole derivatives. J. Chromatogr. A 132 (2), 267–276. doi: 10.1016/S0021-9673(00)89300-0
El Attar, I., Taha, K., El Bekkay, B., El Khadir, M., Alami, I. T., Aurag, J. (2019). Screening of stress tolerant bacterial strains possessing interesting multi-plant growth promoting traits isolated from root nodules of Phaseolus vulgaris L. Biocatal. Agric. Biotechnol. 20, 101225. doi: 10.1016/j.bcab.2019.101225
Etesami, H., Maheshwari, D. K. (2018). Use of plant growth promoting rhizobacteria (PGPRs) with multiple plant growth promoting traits in stress agriculture: Action mechanisms and future prospects. Ecotoxicol. Environ. Saf. 156, 225–246. doi: 10.1016/j.ecoenv.2018.03.013
Flores-Félix, J. D., Carro, L., Cerda-Castillo, E., Squartini, A., Rivas, R., Velázquez, E. (2020). Analysis of the Interaction between Pisum sativum L. Rhizobium laguerreae Strains Nodulating This Legume Northwest Spain. Plants 9 (12), 1755. doi: 10.3390/plants9121755
Ghosh, D., Gupta, A., Mohapatra, S. (2019). A comparative analysis of exopolysaccharide and phytohormone secretions by four drought-tolerant rhizobacterial strains and their impact on osmotic-stress mitigation in Arabidopsis thaliana. World J. Microbiol. Biotechnol. 35, 1–15. doi: 10.1007/s11274-019-2659-0
Ghosh, U. K., Islam, M. N., Siddiqui, M. N., Khan, M. A. R. (2021). Understanding the roles of osmolytes for acclimatizing plants to changing environment: a review of potential mechanism. Plant Signaling Behav. 16 (8), 1913306. doi: 10.1080/15592324.2021.1913306
Gibon, Y., Sulpice, R., Larher, F. (2000). Proline accumulation in canola leaf discs subjected to osmotic stress is related to the loss of chlorophylls and to the decrease of mitochondrial activity. Physiologia Plantarum 110 (4), 469–476. doi: 10.1111/j.1399-3054.2000.1100407.x
Gordon, S. A., Weber, R. P. (1951). Colorimetric estimation of indoleacetic acid. Plant Physiol. 26 (1), 192. doi: 10.1104/pp.26.1.192
Howieson, J. G., Dilworth, M. J. (2016). Working with rhizobia Vol. p (Canberra: Australian Centre for International Agricultural Research), 173). doi: 10.1139/cjm-2016-0776
Jabborova, D., KanNepalli, A., Davranov, K., Narimanov, A., Enakiev, Y., Syed, A., et al. (2021). Co-inoculation of rhizobacteria promotes growth, yield, and nutrient contents in soybean and improves soil enzymes and nutrients under drought conditions. Sci. Rep. 11(1), 22081. doi: 10.1038/s41598-021-01337-9
Jagadish, S. V. K., Bahuguna, R. N., Djanaguiraman, M., Gamuyao, R., Prasad, P. V. V., Craufurd, P. Q. (2016). Implications of High Temperature and Elevated CO2 on Flowering Time in Plants. Front. Plant Sci. 7. doi: 10.3389/fpls.2016.00913
Jing, D., Liu, B., Ma, H., Liu, F., Liu, X., Ren, L. (2023). Effects of inoculation with different plant growth-promoting rhizobacteria on the eco-physiological and stomatal characteristics of walnut seedlings under drought stress. Agronomy 13 (6), 1486. doi: 10.3390/agronomy13061486
Kang, S. M., Adhikari, A., Lee, K. E., Khan, M. A., Khan, A. L., Shahzad, R., et al. (2020). Inoculation with Indole-3-acetic acid-producing rhizospheric Rhodobacter sphaeroides KE149 augments growth of adzuki bean plants under water stress. J. Microbiol. Biotechnol. 30, 717. doi: 10.4014/jmb.1911.11063
Khan, N., Bano, A., Zandi, P. (2018). Effects of exogenously applied plant growth regulators in combination with PGPR on the physiology and root growth of chickpea (Cicer arietinum) and their role in drought tolerance. J. Plant Interact. 13 (1), 239–247. doi: 10.1080/17429145.2018.1471527
Khatun, M., Sarkar, S., Era, F. M., Islam, A. M., Anwar, M. P., Fahad, S., et al. (2021). Drought stress in grain legumes: Effects, tolerance mechanisms and management. Agronomy 11 (12), 2374. doi: 10.3390/agronomy11122374
Li, P., Teng, C., Zhang, J., Liu, Y., Wu, X., He, T. (2023). Characterization of drought stress-mitigating Rhizobium from faba bean (Vicia faba L.) in the Chinese Qinghai-Tibet Plateau. Front. Microbiol. 14, 1212996. doi: 10.3389/fmicb.2023.1212996
Lichtenthaler, H. K., Wellburn, A. R. (1983). Determinations of total carotenoids and chlorophylls a and b of leaf extracts in different solvents. doi: 10.1042/bst0110591
Lucas, J. A., Garcia-Villaraco, A., Montero-Palmero, M. B., Montalban, B., Ramos Solano, B., Gutierrez-Mañero, F. J. (2023). Physiological and genetic modifications induced by plant-growth-promoting rhizobacteria (PGPR) in tomato plants under moderate water stress. Biology 12, 901. doi: 10.3390/biology12070901
Mafakheri, A., Siosemardeh, A., Bahramnejad, B., Struik, P. C., Sohrabi, Y., Struik, P., et al. (2010). Effect of drought stress on yield, proline and chlorophyll contents in three chickpea cultivars. Aust. J. Crop Sci. 4.8, 580–585.
Mansour, E., Mahgoub, H. A., Mahgoub, S. A., El-Sobky, E. S. E., Abdul-Hamid, M. I., Kamara, M. M., et al. (2021). Enhancement of drought tolerance in diverse Vicia faba cultivars by inoculation with plant growth-promoting rhizobacteria under newly reclaimed soil conditions. Sci. Rep. 11, 24142. doi: 10.1038/s41598-021-02847-2
Martinez-Romero, E. (2003). Diversity of Rhizobium-Phaseolus vulgaris symbiosis: overview and perspectives. Plant Soil 252, 11–23. doi: 10.1023/A:1024199013926
Mikiciński, A., Sobiczewski, P., Puławska, J., Malusa, E. (2016). Antagonistic potential of Pseudomonas graminis 49M against Erwinia amylovora, the causal agent of fire blight. Arch. Microbiol. 198, 531–539. doi: 10.1007/s00203-016-1207-7
Mishra, S. K., Khan, M. H., Misra, S., Dixit, V. K., Gupta, S., Tiwari, S., et al. (2020). Drought tolerant Ochrobactrum sp. inoculation performs multiple roles in maintaining the homeostasis in Zea mays L. subjected to deficit water stress. Plant Physiol. Biochem. 150, 1–14. doi: 10.1016/j.plaphy.2020.02.025
Monteoliva, M. I., Guzzo, M. C., Posada, G. (2021). Breeding for drought tolerance by monitoring chlorophyll content. Gene Technol. 10, 165–95. doi: 10.35248/2329-6682.21.10.165
Mustilli, A. C., Fenzi, F., Ciliento, R., Alfano, F., Bowler, C. (1999). Phenotype of the tomato high pigment-2 mutant is caused by a mutation in the tomato homolog of DEETIOLATED1. Plant Cell 11 (2), 145–157. doi: 10.1105/tpc.11.2.145
Nikolaeva, M. K., Maevskaya, S. N., Shugaev, A. G., Bukhov, N. G. (2010). Effect of drought on chlorophyll content and antioxidant enzyme activities in leaves of three wheat cultivars varying in productivity. Russian J. Plant Physiol. 57, 87–95. doi: 10.1134/S1021443710010127
Payne, S. M. (1993). Iron acquisition in microbial pathogenesis. Trends Microbiol. 1, 66–69. doi: 10.1016/0966-842X(93)90036-Q
Pikovskaya, R. I. (1948). Mobilization of phosphorus in soil connection with the vital activity of some microbial species. Microbiology 17, 362–370.
Saïdi, S., Ramírez-Bahena, M. H., Santillana, N., Zúñiga, D., Álvarez-Martínez, E., Peix, A., et al. (2014). Rhizobium laguerreae sp. nov. nodulates Vicia faba on several continents. Int. J. Syst. Evol. Microbiol. 64, 242–247. doi: 10.1099/ijs.0.052191-0
Sandhya, V. S. K. Z., Ali, S. Z., Grover, M., Reddy, G., Venkateswarlu, B. (2010). Effect of plant growth promoting Pseudomonas spp. on compatible solutes, antioxidant status and plant growth of maize under drought stress. Plant Growth Regul. 62, 21–30. doi: 10.1007/s10725-010-9479-4
Sarwar, M., Arshad, M., Martens, D. A., Frankenberger, W. T. (1992). Tryptophan-dependent biosynthesis of auxins in soil. Plant Soil 147, 207–215. doi: 10.1007/BF00029072
Schwyn, B., Neilands, J. (1987). Universal chemical assay for the detection and determination of siderophores. Analytical Biochem. 160 (1), 47–56. doi: 10.1016/0003-2697(87)90612-9
Sijilmassi, B., Filali-Maltouf, A., Boulahyaoui, H., Kricha, A., Boubekri, K., Udupa, S., et al. (2020). Assessment of genetic diversity and symbiotic efficiency of selected Rhizobia strains nodulating lentil (Lens culinaris Medik.). Plants 10, 15. doi: 10.3390/plants10010015
Simonneaux, V., Cheggour, A., Deschamps, C., Mouillot, F., Cerdan, O., Le Bissonnais, Y. (2015). Land use and climate change effects on soil erosion in a semi-arid mountainous watershed (High Atlas, Morocco). J. Arid Environ. 122, 64–75. doi: 10.1016/j.jaridenv.2015.06.002
Sprent, J. I., Ardley, J., James, E. K. (2017). Biogeography of nodulated legumes and their nitrogen-fixing symbionts. New Phytol. 215 (1), 40–56. doi: 10.1111/nph.14474
Stavi, I., Thevs, N., Priori, S. (2021). Soil salinity and sodicity in drylands: A review of causes, effects, monitoring, and restoration measures. Front. Environ. Sci. 9, 712831. doi: 10.3389/fenvs.2021.712831
Taha, K., El Attar, I., Dekkiche, S., Aurag, J., Béna, G. (2018). Rhizobium laguerreae is the main nitrogen-fixing symbiont of cultivated lentil (Lens culinaris) in Morocco. Systematic Appl. Microbiol. 41 (2), 113–121. doi: 10.1016/j.syapm.2017.09.008
Taha, K., El Attar, I., Hnini, M., Raif, A., Béna, G., Aurag, J. (2022). Beneficial effect of Rhizobium laguerreae co-inoculated with native Bacillus sp. Enterobacter aerogenes lentil Growth under drought stress. Rhizosphere 22, 100523. doi: 10.1016/j.rhisph.2022.100523
Tandon, H. L. S., Cescas, M. P., Tyner, E. H. (1968). An acid-free vanadate-molybdate reagent for the determination of total phosphorus in soils. Soil Sci. Soc. America J. 32 (1), 48–51. doi: 10.2136/sssaj1968.03615995003200010012x
Tubiello, F. N., Rosenzweig, C., Conchedda, G., Karl, K., Gütschow, J., Xueyao, P., et al. (2021). Greenhouse gas emissions from food systems: building the evidence base. Environ. Res. Lett. 16 (6), 065007. doi: 10.1088/1748-9326/ac018e
Ulzen, J., Abaidoo, R. C., Ewusi-Mensah, N., Masso, C. (2018). On-farm evaluation and determination of sources of variability of soybean response to Bradyrhizobium inoculation and phosphorus fertilizer in northern Ghana. Agriculture Ecosyst. Environ. 267, 23–32. doi: 10.1016/j.agee.2018.08.007
Uzma, M., Iqbal, A., Hasnain, S. (2022). Drought tolerance induction and growth promotion by indole acetic acid producing Pseudomonas aeruginosa in Vigna radiata. PloS One 17 (2), e0262932. doi: 10.1371/journal.pone.0262932
Wang, Q., Wu, J., Lei, T., He, B., Wu, Z., Liu, M., et al. (2014). Temporal-spatial characteristics of severe drought events and their impact on agriculture on a global scale. Quaternary Int. 349, 10–21. doi: 10.1016/j.quaint.2014.06.021
Wang, C., Li, G., Xie, Y. S., Gao, Y. L., Guo, J. H., Xu, Q. (2018). Enhanced drought tolerance in maize conferred by a consortium of plant growth-promoting rhizobacterium strains. Agric. Res. Arid Areas 36, 126–132. doi: 10.7606/j.issn.1000-7601.2018.01.20
Williams, P. (2007). "Quorum sensing, communication and cross-kingdom signalling in the bacterial world. Microbiology 153.12, 3923–3938. doi: 10.1099/mic.0.2007/012856-0
Woillez, M. N. (2019). Revue de littérature sur le changement climatique au Maroc: observations, projections et impacts. Papiers Recherche, 1–33. doi: 10.3917/afd.woill.2019.01.0001
Wu, D., Piao, S., Zhu, D., Wang, X., Ciais, P., Bastos, A., et al. (2020). Accelerated terrestrial ecosystem carbon turnover and its drivers. Glob Chang Biol. 26 (9), 5052–5062. doi: 10.1111/gcb.15224
Yang, J., Kloepper, J. W., R yu, C. M. (2009). Rhizosphere bacteria help plants tolerate abiotic stress. Trends Plant Sci. 14 (1), 1–4. doi: 10.1016/j.tplants.2008.10.004
Yang, X., Lu, M., Wang, Y., Wang, Y., Liu, Z., Chen, S. (2021). Response Mechanism of Plants to Drought Stress. Horticulturae. 7, 50. doi: 10.3390/horticulturae7030050
Keywords: Rhizobium laguerreae, PGPR, Pisum sativum, Vicia faba, legumes, drought stress
Citation: Hami A, El Attar I, Mghazli N, Ennajeh S, Ait-Ouakrim EH, Bennis M, Oulghazi S, Badaoui B, Aurag J, Sbabou L and Taha K (2025) Enhancing drought tolerance in Pisum sativum and Vicia faba through interspecific interactions with a mixed inoculum of Rhizobium laguerreae and non-host beneficial rhizobacteria. Front. Plant Sci. 16:1528923. doi: 10.3389/fpls.2025.1528923
Received: 22 November 2024; Accepted: 06 February 2025;
Published: 26 February 2025.
Edited by:
Maurizio Ruzzi, University of Tuscia, ItalyReviewed by:
Mariela I. Monteoliva, National Institute of Agricultural Technology (INTA), ArgentinaCopyright © 2025 Hami, El Attar, Mghazli, Ennajeh, Ait-Ouakrim, Bennis, Oulghazi, Badaoui, Aurag, Sbabou and Taha. This is an open-access article distributed under the terms of the Creative Commons Attribution License (CC BY). The use, distribution or reproduction in other forums is permitted, provided the original author(s) and the copyright owner(s) are credited and that the original publication in this journal is cited, in accordance with accepted academic practice. No use, distribution or reproduction is permitted which does not comply with these terms.
*Correspondence: Asma Hami, asma.hami@um5r.ac.ma; Kaoutar Taha, k.taha@um5r.ac.ma
Disclaimer: All claims expressed in this article are solely those of the authors and do not necessarily represent those of their affiliated organizations, or those of the publisher, the editors and the reviewers. Any product that may be evaluated in this article or claim that may be made by its manufacturer is not guaranteed or endorsed by the publisher.
Research integrity at Frontiers
Learn more about the work of our research integrity team to safeguard the quality of each article we publish.