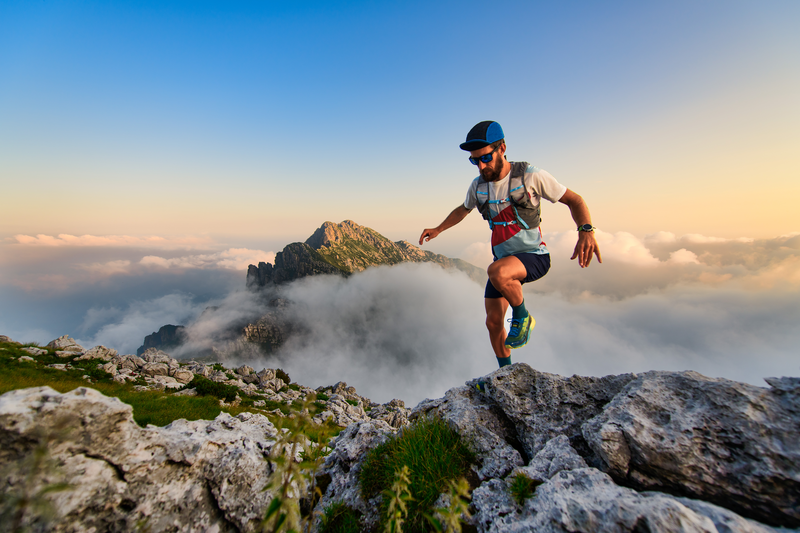
94% of researchers rate our articles as excellent or good
Learn more about the work of our research integrity team to safeguard the quality of each article we publish.
Find out more
ORIGINAL RESEARCH article
Front. Plant Sci. , 07 February 2025
Sec. Plant Metabolism and Chemodiversity
Volume 16 - 2025 | https://doi.org/10.3389/fpls.2025.1521990
Pyrus calleryana fruit (bean pear) is processed into fruit wine and used in traditional Chinese medicine. The present study reported phytochemical constituents, antioxidant, anti-inflammatory, and enzyme inhibitory activities of P. calleryana fruit water extract (WE) and ethanol extract (EE). In the P. calleryana fruit WE and EE, 63 compounds were identified using UHPLC-Q-Orbitrap-MS analysis, including 23 phenols, 13 flavonoids, 14 terpenoids, and 13 other types of compounds. In the antioxidant activity, WE and EE showed marked free radical scavenging effects on both ABTS (2.33 ± 0.15 μg/mL and 2.23 ± 0.15 μg/mL, respectively) and DPPH (5.93 ± 0.55 μg/mL and 7.07 ± 0.23 μg/mL, respectively), especially, their scavenging effects on DPPH free radicals were superior or equivalent to that of BHT (7.47 ± 0.47 μg/mL). In LPS-induced RAW264.7 cells, P. calleryana fruit WE and EE remarkably inhibited the secretion of inflammatory factors, and the inhibitory effect of WE on the release of IL-6, NO, and PGE2 was superior or equivalent to that of EE. Interestingly, P. calleryana fruit WE and EE exhibited potent inhibition on α-glucosidase (0.60 ± 0.09 μg/mL and 0.48 ± 0.09 μg/mL, respectively) and tyrosinase (210.11 ± 2.59 μg/mL and 45.35 ± 0.96 μg/mL, respectively), which were superior to their respective positive controls acarbose (302.57 ± 22.09 μg/mL) and arbutin (243.07 ± 15.91 μg/mL). Our findings suggested that P. calleryana fruit WE and EE possess significant antioxidant, anti-inflammatory, α-glucosidase, and tyrosinase inhibitory properties. Thus, P. calleryana fruit has great potential for application in functional food products.
Pyrus calleryana Decne., a deciduous tree of the genus Pyrus in the Rosaceae family, is a native tree species of China (Sapkota et al., 2022) and primarily distributed in Zhejiang, Jiangsu, Jiangxi, Hubei, Guangdong, Guangxi, Yunnan, and Guizhou provinces in China (Jiang et al., 2016). P. calleryana fruit, commonly known as bean pear, is rich in polysaccharides (15-20%) (Liu et al., 2011). It is often eaten after cooking, primarily due to its sour and astringent when raw, and cooking not only eliminates its sour and astringent taste but also makes it very sweet. Besides, it is also a common raw material for brewing fruit wine (Wu and Wu, 2012).
In addition, P. calleryana is often used as a rootstock for pear (Pyrus pyrifolia), and its wood is employed to make furniture (Li et al., 2015). It is also cultivated as an ornamental tree due to its bright white flowers in early spring (Culley and Hardiman, 2007; Santamour and Demuth, 1980; Sapkota et al., 2022). As a folk medicine, various parts of P. calleryana, including its fruit, branches, leaf, and root, are used medicinally to treat stomach pain, cough, indigestion, acute conjunctivitis, and dysentery (China Medical Information Platform, 2024). Past studies of phytochemical constituents and pharmacological activity of P. calleryana have shown that its leaf ethanol extract exhibited strong antioxidant effects (Nassar et al., 2011), and the volatile oil of its fruit possessed antibacterial and anti-inflammatory effects (Tian et al., 2023).
P. calleryana fruit possesses both edible and medicinal values and has great development potential in functional foods. Nevertheless, there are fewer studies on its chemical composition and bioactivities. Hence, in this study, we aimed to analyze the phytochemical composition of P. calleryana fruit WE and EE, explore their antioxidant, anti-inflammatory effects, and enzyme inhibitory activities, and offer a theoretical basis for exploiting P. calleryana fruit in the field of functional foods.
RAW264.7 cells were purchased at the Kunming Cell Bank (Kunming, China). Folin-Ciocalteu reagent, rutin, arbutin, lipopolysaccharide (LPS), 3-[4,5-Dimethylthiazol-2-yl]-2, 5-diphenyltetrazolium bromide (MTT), dexamethasone (DXM), galanthamine, dimethyl sulfoxide (DMSO), acarbose and gallic acid were supplied from Solarbio Sciences & Technology (Beijing, China). Mouse TNF-α and IL-6 ELISA kits were obtained from Multi Sciences (Lianke) Biotech, Co., Ltd. (Hangzhou, China). Abnova Corporation (Taiwan, China) provided a prostaglandin E2 (PGE2) ELISA kit. Ascorbic acid, 5,5’dithiobis-(2-nitrobenzoic acid), tyrosinase, p-nitrophenyl-α-D-glucopyranoside (p-NPG), L-tyrosine, acetylthiocholine iodide (ATCI), acetylcholinesterase (AChE), butyrylthiocholine chloride (BTCl), butyrylcholinesterase (BChE), butylated hydroxytoluene (BHT), 2,2-azino-bis-3-ethylbenzthiazoline-6-sulphonic acid (ABTS), α-glucosidase, and 1,1-Diphenyl-2-picrylhydrazyl (DPPH) were supplied by Sigma-Aldrich (Germany). Beyotime Biotechnology offered the NO detection kit (Shanghai, China).
P. calleryana fruit was bought from Shansen Reservoir, Yudu County, Ganzhou City, Jiangxi Province, and was identified by Prof. Guoxiong Hu, and preserved in the College of Life Sciences, Guizhou University (herbarium number: PC20210907).
Fresh P. calleryana fruit was chopped, placed in a round-bottomed flask, and extracted with ultrapure water or 70% ethanol solution at a material-liquid ratio of 1:3 (w/v) for 2 h. Afterward, the filtrate was gathered, and the residue was re-extracted according to the above conditions. Subsequently, the two filtrates were merged and condensed by rotary evaporation to obtain the concentrated solution. Finally, the concentrated solution was dried by a freeze-dryer to obtain the WE and EE. The samples were packed into brown bottles and sealed storage at 4°C.
Ultra-high-performance liquid chromatography coupled to quadrupole-Orbitrap high-resolution mass spectrometry (UHPLC-Q-Orbitrap-MS) was used to identify phytochemical components of P. calleryana fruit WE and EE. Dionex Ultimate 3000 RSLC liquid phase conditions were as follows: injection volume (5 μL), column (Thermo Fisher Hypersil GOLD aQ, 2.1 mm × 100 mm, 1.9 μm), column temperature (40°C), mobile phase (A: 0.1% formic acid aqueous solution and B: 0.1% formic acid acetonitrile), and flow rate (0.3 mL/min). The gradient elution conditions were as follows: 0~2 min (5% B), 2~42 min (5~95% B), 42~47 min (95% B), 47~47.1 min (5% B), 47.1~50 min (5% B).
Thermo Fisher Scientific’s Q Exactive Focus hybrid quadrupole-Orbitrap high-resolution mass spectrometry (Q-Orbitrap-MS) with heated electrospray ionization (HESI-II) was used to acquire MS data. The HESI-II settings were as follows: sheath gas (35 arb), auxiliary gas (10 arb), S-Lens (60), probe heater temperature (350°C), spray voltages (-2.5/+3.0 kV), capillary temperature (320°C), sweep gas (0 arb). The mass spectrometry scanning parameters were as follows: full MS scan range (100 to 1500 m/z), loop count (3), intensity threshold (1.6 e5), stepped NCE (20, 40, 60 eV), maximum injection time (full MS: 100 ms, MS/MS: 50 ms), resolution (full MS: 70,000, MS/MS: 17,500), AGC target (full MS: 1 e6, MS/MS: 2 e5). Thermo Fisher Scientific Xcalibur 4.1 (Waltham, MA, USA) was utilized for the processing of mass spectrometry data on chemical compositions. Phytochemicals were identified by comparing the mzVault and mzCloud databases and references within a 10 ppm threshold.
The Folin-Ciocalteu method was utilized to determine the TPC in the P. calleryana fruit WE and EE, with gallic acid as the standard. WE or EE were dissolved in ultrapure water to prepare sample solution. Folin-Ciocalteu solution (2.5 mL) and sample solution (0.5 mL) were accurately pipetted into a 5 mL tube and reacted for 4 min. Afterward, 7.5% Na2CO3 solution (2 mL) was added, mixed, and reacted for 60 min at room temperature. After recording the absorbance at 760 nm, we used linear fitting to create a standard curve with the absorbance values as the vertical coordinate and the concentration of gallic acid as the horizontal coordinate. The TPC of P. calleryana fruit WE and EE was calculated according to the standard curve and expressed as milligrams of gallic acid equivalents per gram of sample (mg GAEs/g sample).
The TFC in the WE and EE of P. calleryana fruit was estimated by the NaNO2-Al(NO3)3-NaOH colorimetric method and rutin was used as a standard. The WE or EE dissolved in 70% ethanol (5 mL) and NaNO2 solution (5%, 0.4 mL) were mixed and reacted for 6 min. Next, we added Al(NO3)3 solution (10%, 0.4 mL) and let it react for 6 min. Subsequently, NaOH solution (4%, 4 mL) and ultrapure water (0.2 mL) were added and reacted for 15 min. Then, the absorbance at 510 nm was measured. The standard curve was established using rutin concentration as the horizontal coordinate and absorbance as the vertical coordinate. The standard curve was used to calculate the TFC, which was then expressed as milligrams of rutin equivalents per gram of sample (mg REs/g sample).
The ability of P. calleryana fruit WE and EE to scavenge free radicals was determined by ABTS and DPPH assay. Ascorbic acid and BHT were used as positive controls.
In the ABTS free radical scavenging capacity assay, ABTS solution (0.7 mM) was prepared by dissolving ABTS (19.2 mg) with dehydrated ethanol (50 mL), and K2S2O4 solution (2.4 mM) was obtained by dissolving K2S2O4 (33 mg) with ultrapure water (50 mL). Subsequently, equal volumes of ABTS and K2S2O4 solution were mixed, protected from light, and incubated at 37°C for 12 h to prepare ABTS•+ solution. The ABTS assay included a sample group, a control group, and a blank group. Sample solution (18 μL) was combined with ABTS•+ solution (180 μL) in the sample group; in the control group, sample solution (18 μL) was combined with solvent of sample solution (180 μL); in the blank group, 18 μL of the solvent of sample solution and 180 μL of ABTS•+ solution were added. Then, all groups were incubated for 10 min and sheltered from light. The absorbance (A) was detected at 734 nm. The findings were displayed as milligrams of ascorbic acid equivalents per gram of sample (mg AEs/g sample) and IC50 values. The following formula was used to determine the scavenging rate:
In the free radical clearance test for DPPH, DPPH (0.08 mM) solution was prepared by dissolving DPPH (3.15 mg) in dehydrated ethanol (100 mL). The sample group, control group, and blank group were set up. In the sample group, the sample solution (100 μL) was mixed with an equal amount of DPPH solution. Similarly, equal volumes of sample solution and solvent of sample solution were mixed as the control group. In the blank group, the DPPH solution and solvent of the sample solution were mixed in a 1:1 ratio (v/v). Next, these groups were placed under room temperature and light-avoidance conditions for 0.5 h, and their absorbance values were detected at 517 nm. The free radical scavenging capacity was expressed as milligrams of ascorbic acid equivalents per gram of sample (mg AEs/g sample) and IC50 values. The aforementioned formula was used to determine the scavenging rate.
MTT test was utilized to test the toxicity of P. calleryana fruit WE and EE on RAW264.7 cells (Zhao et al., 2020a). The samples were dissolved in DMSO and diluted half-fold with culture medium (DMSO content was less than 0.05%). Cells (2×105 cells/well) were inoculated and cultured in 96-well plates. Then, the old culture medium was aspirated out and discarded after 24 h incubation. Afterward, the sample solution was pipetted into 96 well plates and incubated for 24 h at 37°C. Next, MTT solution (10 μL, 5 mg/mL) was pipetted into and reacted for 4 h. Subsequently, the old supernatant was discarded, and 150 μL of DMSO was added to each well. Finally, the 96-well plate was shaken at room temperature for 10 min, and the absorbance value was determined at 490 nm.
In the assay of inflammatory factors release, 100 μL of cell suspensions (2×106 cells/mL) were inoculated in 96-well plates and cultured for 24 h. The sample group, blank group, positive group (DXM), and model group (LPS) were set up. Then, the old supernatants were aspirated out and discarded. In the sample group, 100 μL of sample solution (62.5, 125, and 250 μg/mL) was added; 100 μL of DXM (20 μg/mL) was added in the positive group; 100 μL of culture medium was added in blank and model groups. After 2 h of incubation, the supernatants were discarded; in the sample group, equal volumes of sample solution (100 μL) and LPS (100 μL) were mixed, and the sample solution’s ultimate concentrations were 62.5, 125, and 250 μg/mL; DXM (100 μL) and LPS (100 μL) were added to the positive group, and the ultimate concentration of DXM was 20 μg/mL. In the model group, culture medium (100 μL) and LPS (100 μL) were mixed, and the three groups mentioned above had a final concentration of 1 μg/mL of LPS. In addition, culture medium (200 μL) was added to the blank group. Next, all the groups were placed at a constant temperature of 37°C for 24 h. Then, all the groups were photographed under an inverted fluorescence microscope to observe cell morphological changes. Subsequently, the cell supernatants were collected. Determination of NO release was performed referring to the instructions of the NO detection kit, and the levels of IL-6, TNF-α, and PGE2 were measured by ELISA kits.
The inhibitory activities of P. calleryana fruit WE and EE on tyrosinase, α-glucosidase, and cholinesterase were determined by using arbutin, acarbose, and galanthamine as positive controls, respectively.
In the assay of tyrosinase inhibitory activity, we set up four groups: sample group, sample blank group, negative group, and blank group. First, sample solution (70 μL) was added to the sample and sample blank groups, and PBS buffer (70 μL, pH=6.8) was added in the negative and blank groups. Subsequently, tyrosinase (100 μL, 100 U/mL) was added in the sample and negative groups, while PBS buffer (100 μL, pH=6.8) was spiked into the sample blank and blank groups. The above four groups were incubated at a constant temperature of 37°C for 5 min. Then, L-tyrosine (80 μL, 5.5 mM) was added in all groups. After 37°C incubation for 30 min, their absorbance (A) was determined at 492 nm. The following formula was used to get the tyrosinase inhibition rate, and IC50 values were utilized to express the inhibitory activity of tyrosinase.
In the α-glucosidase inhibitory activity assay, the experimental procedure was divided into four groups: sample group, sample blank group, negative group, and blank group. First, 90 μL of sample solution was added into the sample and sample blank group, whereas 90 μL of PBS buffer (pH=6.8) was utilized in the negative and blank groups. Next, 10 μL of α-glucosidase (0.8 U/mL) was added to the sample and negative groups, while 10 μL of PBS buffer (pH=6.8) was added to the sample blank and blank groups. The four groups were placed in a constant temperature incubator of 37°C for 15 min. Then, 10 μL of the p-NPG (1 mM) was added to all groups and reacted at 37°C for 15 min. Finally, the reaction was terminated by adding 80 μL of sodium carbonate solution (0.2 mM). The absorbance values of each group were measured at 405 nm. The α-glucosidase inhibition rate was calculated as above, and IC50 values represented the inhibitory activity of α-glucosidase.
In the determination of cholinesterase inhibitory activity, we also set up sample, sample blank, negative, and blank groups. First, the sample and sample blank groups were given a 50 μL sample solution, while 50 μL of PBS buffer (pH=8) was added to the negative and blank groups. Subsequently, 10 μL of AChE or BChE (0.5 U/mL) was pipetted into the sample and negative groups, and 10 μL of PBS buffer (pH=8) was added to the sample blank and blank groups, which were kept at 4°C for 5 min. Then, 20 μL of ATCI (2 mM) or BTCI (2 mM) and 5,5’dithiobis-(2-nitrobenzoic acid) solution (2 mM) was added to all groups and maintained at 37°C for 30 min. Absorbance values were determined at 405 nm, and the inhibition rate was calculated as above. Finally, the inhibitory activity of the WE and EE on cholinesterase were expressed as IC50 values.
In this study, three independent replications of each experiment were carried out, and their results were represented using mean ± standard deviation (SD). The significant difference was analyzed by one-way analysis of variance (ANOVA) with Fischer’s LSD post-hoc test or two-tailed unpaired t-test using IBM SPSS Statistics 25 software (p < 0.05 indicates a significant difference).
Based on fresh weight, the extraction rates of P. calleryana fruit WE and EE were 8.3% (w/w) and 11% (w/w), respectively. The Folin-Ciocalteu method and the NaNO2-Al(NO3)3-NaOH colorimetric method were used to determine the TPC and TFC of WE and EE, and the results are shown in Figure 1. The TPC of WE and EE were 120.74 ± 0.32 mg GAEs/g sample and 95.37 ± 0.28 mg GAEs/g sample, respectively, and WE exhibited more TPC compared to EE (p < 0.001). The TFC of WE and EE separately were 347.05 ± 6.27 mg REs/g sample and 562.51 ± 10.71 mg REs/g sample, and the TFC in EE was significantly higher than WE (p < 0.001).
Based on UHPLC-Q-Orbitrap-MS analysis, 63 compounds were characterized in the P. calleryana fruit WE and EE (detailed analysis of fragmentation patterns in Supplementary Material), of which 40 compounds were identified in WE, and 41 compounds were identified in EE (Table 1, Supplementary Figure S1 in Supplementary Materials). A total of 23 phenolic compounds were identified as neochlorogenic acid (6), 3,5-dimethoxy-4-hydroxybenzaldehyde (8), gentisic acid (11), cryptochlorogenic acid (12), aloenin (14), chlorogenic acid (15), vanillic acid (16), 1-caffeoylquinic acid (17), caffeic acid (18), 7-hydroxycoumarin (21), vanillin (23), 2-hydroxy-4-methoxybenzaldehyde (24), protocatechualdehyde (28), ellagic acid (34), sinapyl aldehyde (36), dehydrodiisoeugenol (38), 3,5-dicaffeoylquinic acid (39), isochlorogenic acid B (40), isochlorogenic acid C (44, ethyl caffeate (49), astringin (51), 6-gingerol (53), and (+)-usniacin (60) (Supplementary Figure S2, Supplementary Material). Thirteen flavonoid compounds were identified, including (+)-catechin hydrate (22), procyanidin B1 (26), taxifolin 7-rhamnoside (29), medicarpin (31), hesperetin (32), morin (33), cynaroside (35), isorhamnetin (41), diosmetin-7-O-β-D-glucopyranoside (43), hyperoside (45), isoquercitrin (46), kaempferol-7-O-β-D-glucopyranoside (47), and luteolin (50) (Supplementary Figure S3, Supplementary Material). Fourteen terpenoid compounds were identified as ailanthone (20), sarracenin (25), perillene (37), germacrone (48), glabrolide (52), medicagenic acid (54), quillaic acid (55), 18β-glycyrrhetintic acid (56), ursonic acid (57), echinocystic acid (58), ursolic acid (59), acetyl-11-keto-β-boswellic acid (61), lupenone (62), and roburic acid (63) (Supplementary Figure S4, Supplementary Material). Besides, 13 other types of compounds were identified, including sucrose (1), quinic acid (2), γ-aminobutyric acid (3), L-tryptophan (4), scopolin (5), citric acid (7), o-veratraldehyde (9), mannitol (10), 2-isopropylmalic acid (13), homoveratrumic acid (19), ethyl 4-methoxycinnamate (27), androsin (30), and benzoic acid (42) (Supplementary Figure S5, Supplementary Material). Except for chlorogenic acid (15), vanillic acid (16), and caffeic acid (18), which were previously identified from P. calleryana leaf (Nassar et al., 2011), the remaining 60 compounds were identified for the first time in P. calleryana. The results showed that there were plenty of phenolic, flavonoid, and terpenoid compounds in P. calleryana fruit WE and EE.
Table 1. Chemical composition of P. calleryana fruit WE and EE were authenticated using UHPLC-Q-Orbitrap-MS in positive and negative ion modes.
ABTS and DPPH methods were utilized to determine the antioxidant capacity of P. calleryana fruit WE and EE. As shown in Table 2, both WE (2.33 ± 0.15 μg/mL) and EE (2.23 ± 0.15 μg/mL) exhibited significant ABTS radical scavenging effects. Moreover, WE (5.93 ± 0.55 μg/mL) and EE (7.07 ± 0.23 μg/mL) displayed strong DPPH radical scavenging abilities, which was superior or equivalent to that of BHT (7.47 ± 0.47 μg/mL). The DPPH and ABTS free radical scavenging abilities of WE (601.75 ± 55.63 mg AEs/g and 630.35 ± 40.50 mg AEs/g, respectively) were superior or equivalent to those of EE (503.07 ± 17.34 mg AEs/g and 658.75 ± 44.19 mg AEs/g, respectively).
BHT is widely utilized as a common antioxidant and preservative in the cosmetic and food industries (Yehye et al., 2015). However, toxic effects on the liver, kidneys, and lungs have been reported from prolonged exposure to BHT, raising concerns among consumers (Ghosh et al., 2020). In this study, WE and EE exhibited DPPH radical scavenging abilities that were superior to or comparable to BHT. Therefore, P. calleryana fruit WE and EE can be used as antioxidant substitutes for BHT. The determination of TPC and TFC indicated that phenolic and flavonoid compounds were abundant in P. calleryana fruit WE and EE. Plant-derived phenolic and flavonoid compounds have been shown to have potent antioxidant activity (Diaz et al., 2012; Mustafa et al., 2010). In our study, a total of 23 phenols and 13 flavonoids were identified through UHPLC-Q-Orbitrap-MS analysis, among which luteolin (IC50: 0.59 ± 0.02 μg/mL and 2.10 ± 0.06 μg/mL, respectively) has been shown to be superior to that of BHT (IC50: 1.45 ± 0.02 μg/mL and 10.5 ± 0.08 μg/mL, respectively) and ascorbic acid (IC50: 2.16 ± 0.03 μg/mL and 3.03 ± 0.12 μg/mL, respectively) in ABTS and DPPH scavenging effects (Tian et al., 2021a). In the ABTS radical clearance test, vanillin exhibited greater activity than ascorbic acid (Tai et al., 2011). Moreover, ursolic acid (Do Nascimento et al., 2014), vanillic acid (Szwajgier et al., 2005; Tai et al., 2012), isorhamnetin (Gong et al., 2020), morin (Hsu et al., 2022), dehydrodiisoeugenol (Li et al., 2020), 6-gingerol (Mi et al., 2016), caffeic acid (Gülçin, 2006), 3,5-dicaffeoylquinic acid (Hong et al., 2015), ellagic acid (Han et al., 2006), and cynaroside (Zou et al., 2018) have been found to have high ABTS and/or DPPH free radical scavenging capacity. Thus, the strong antioxidant activity of P. calleryana fruit WE and EE may be explained by their high content of flavonoids and phenolic compounds. Our results showed that P. calleryana fruit could be used as a potential antioxidant in functional foods.
The MTT assay was used to assess the cytotoxicity of WE and EE against RAW264.7 cells. As shown in Figure 2, both WE and EE were non-cytotoxic to RAW264.7 cells in the dose range of 15.625 to 250 mg/mL compared with untreated cells (p > 0.05). Therefore, the concentrations of 62.5, 125, and 250 μg/mL were selected for subsequent experiments.
Figure 2. Cytotoxic activity of P. calleryana fruit WE and EE on RAW264.7 macrophages. ***p < 0.001 versus the untreated cells.
The pathogenesis of inflammation is closely related to the excessive secretion of pro-inflammatory factors (IL-6 and TNF-α) and mediators (NO and PGE2). Inhibiting their production is one of the most important means for treating inflammatory diseases (Wang et al., 2020; Zhao et al., 2021). As shown in Figure 3A, the morphology of RAW264.7 cells changed from round to stretched after LPS stimulation compared with control, while the stretched cells gradually decreased with the gradual increase of WE and EE concentrations, especially at high concentrations (250 μg/mL) there were no stretched cells. As shown in Figures 3B–E, LPS induction significantly increased the release of NO, PGE2, TNF-α, and IL-6. Compared to the LPS group, different concentrations of P. calleryana fruit WE and EE (62.5, 125, and 250 μg/mL) markedly suppressed the release of NO, PGE2, and IL-6 in RAW264.7 cells induced by LPS, and the high concentration (250 μg/mL) of WE and EE effectively suppressed the level of TNF-α. Moreover, WE (62.5, 125, and 250 μg/mL) and EE (125 and 250 μg/mL) showed better inhibition on PGE2 and IL-6 release compared with the positive control DXM (20 μg/mL), and the inhibitory effects of WE on NO, PGE2, and IL-6 was superior to or equivalent to that of EE at the same concentrations. These results showed that the P. calleryana fruit WE and EE effectively inhibited the level of NO, PGE2, TNF-α, and IL-6 in RAW264.7 cells induced by LPS.
Figure 3. The influence of P. calleryana fruit WE and EE on LPS-induced RAW264.7 macrophage morphological alterations and pro-inflammatory mediator and cytokine level. (A) P. calleryana fruit WE and EE’s effect on alterations in cellular morphology. (B–E) The NO release was determined by the NO detection kit, while the levels of PGE2, TNF-α, and IL-6 were measured by ELISA kits. The mean ± SD is used to express data. Significant differences are represented by different alphabets (p < 0.05).
Plant-derived flavonoids, terpenoids, and phenolic compounds have been widely demonstrated to be associated with anti-inflammatory effects (Diaz et al., 2012; Faisal et al., 2022; Jaroš et al., 2022; Maleki et al., 2019; Rathee et al., 2009). Phenols, flavonoids, and terpenoids identified in the P. calleryana fruit, like ellagic acid, caffeic acid, ursolic acid, and luteolin, reduced the levels of IL-6 and TNF-α in mouse models (Aziz et al., 2018; Chao et al., 2010; Zhao et al., 2023). Besides, 18β-glycyrrhetintic acid significantly reduced TNF-α level in serum and NO level in the kidney of MTX-induced rats (Abd El-Twab et al., 2016). Additionally, in the past in vitro studies, vanillin (Zhao et al., 2019), chlorogenic acid (Huang et al., 2023; Hwang et al., 2014), cryptochlorogenic acid (Zhao et al., 2020b), medicarpin (Chern et al., 2021; Mansoori et al., 2020), ethyl caffeate (Chiang et al., 2005), cynaroside (Zou et al., 2018), roburic acid (Islam, 2017), ursonic acid (Son and Lee, 2020), echinocystic acid (Hyam et al., 2013), ursolic acid (Zhao et al., 2023), and 3,5-dicaffeoylquinic acid (Hong et al., 2015) significantly inhibited the release of inflammatory factors, such as TNF-α, IL-6, NO, and PGE2. Therefore, the strong anti-inflammatory effects of P. calleryana fruit WE and EE may be associated with these flavonoids, terpenoids, and phenolic compounds. Our results suggested that P. calleryana fruit could be exploited greatly as an anti-inflammatory agent in functional foods.
Inhibiting the activity of α-glucosidase can suppress oligosaccharide hydrolysis and reduce the absorption of carbohydrates after meals, which can effectively help lower blood glucose (Gao et al., 2022). As shown in Table 3, WE (0.60 ± 0.09 μg/mL) and EE (0.48 ± 0.09 μg/mL) exhibited extremely strong α-glucosidase inhibition, which was stronger than that of acarbose (302.57 ± 22.09 μg/mL) (p < 0.05). Plant-derived flavonoids and phenolic compounds can serve as natural α-glucosidase inhibitors (Goncçalves and Romano, 2017; Şöhretoğlu and Sari, 2020). Phenolic and flavonoid compounds identified in WE and EE of P. calleryana fruit, such as vanillic acid (Singh et al., 2022), vanillin (Zabad et al., 2019), morin (Vanitha et al., 2014), ellagic acid (Chao et al., 2010), isorhamnetin (Alqudah et al., 2023), and luteolin (Zang et al., 2016) have been shown to markedly improved blood glucose levels in a diabetic mouse model. Furthermore, the inhibitory activities of protocatechualdehyde and 6-gingerol on α-glucosidase were superior to those of the positive control acarbose in vitro (Mohammed et al., 2017; Tian et al., 2021b). The above findings indicated that P. calleryana fruit can be utilized in functional foods as an α-glucosidase inhibitor.
Tyrosinase is the key enzyme that produces melanin and causes skin pigmentation, and excessive melanin leads to many skin diseases, such as age spots, malignant melanoma, and freckles (Abdul Karim et al., 2014; Brenner and Hearing, 2008). Besides, tyrosinase causes enzymatic browning of beverages, fruits, and vegetables (Loizzo et al., 2012). Therefore, inhibition of tyrosinase activity is useful for achieving skin whitening effects and preventing enzymatic browning of food products. As shown in Table 3, the inhibitory effects of WE (210.11 ± 2.59 μg/mL) and EE (45.35 ± 0.96 μg/mL) on tyrosinase were significantly superior to the positive control arbutin (243.07 ± 15.91 μg/mL) (p < 0.05). Past studies have shown that phenolic and flavonoid compounds can serve as important sources of tyrosinase inhibitors (Goncçalves and Romano, 2017). Luteolin, a compound identified from the fruits of P. calleryana, dose-dependently inhibited tyrosinase activity and melanogenesis in α-melanocyte-stimulating hormone (α-MSH)-stimulated B16 melanoma cells (An et al., 2008; Choi et al., 2008). In addition, benzoic acid (Sima et al., 2011), vanillic acid (Smeriglio et al., 2019), ellagic acid (Pillaiyar et al., 2017), and 2-hydroxy-4-methoxybenzaldehyde (Rafiee and Javaheri, 2015) have been well demonstrated to have good tyrosinase inhibitory effects. Several chemical components identified in P. calleryana fruit EE but not detected in WE were proven to have tyrosinase inhibitory effects. For example, ursolic acid exhibited significant anti-tyrosinase effort and inhibited α-MSH-stimulated melanin synthesis in B16F1 cells (Neimkhum et al., 2021; Park et al., 2020). Hesperetin observably inhibited tyrosinase in a competitive manner with IC50 = 11.25 ± 1.73 mM (Si et al., 2012). Besides, isorhamnetin (Yu et al., 2019) and morin (Wang et al., 2014) have been demonstrated to serve as tyrosinase inhibitors. These compounds identified only from P. calleryana fruit EE may be the reason why EE was significantly superior to WE on tyrosinase inhibition. Hence, the strong tyrosinase inhibition activity of P. calleryana fruit WE and EE may be closely related to the presence of these phenolic and flavonoid compounds. The results demonstrated that P. calleryana fruit can be developed as a potential tyrosinase inhibitor for skin whitening and prevention of food browning.
Low levels of acetylcholine in the human brain play a crucial role in the pathogenesis of Alzheimer’s disease, and reducing the catabolism of acetylcholine by inhibiting the activities of acetylcholinesterase and butyrylcholinesterase is an effective treatment for Alzheimer’s disease (Adewusi et al., 2010). As shown in Table 3, EE (40.47 ± 5.22 μg/mL and 26.77 ± 0.89 μg/mL, respectively) and WE (60.75 ± 5.05 μg/mL and 34.31 ± 1.97 μg/mL, respectively) showed moderate inhibitory effects on acetylcholinesterase and butyrylcholinesterase. Past studies have shown that vanillin and vanillic acid inhibited acetylcholinesterase and butyrylcholinesterase activities (Salau et al., 2020). Besides, 2-hydroxy-4-methoxybenzaldehyde and protocatechualdehyde had potential acetylcholinesterase inhibitory activity (Aderogba et al., 2013; Kundu and Mitra, 2013). These compounds may contribute to the moderate cholinesterase inhibitory activity observed in P. calleryana fruit.
The present study reports the chemical composition, antioxidant, anti-inflammatory, and enzyme inhibitory activities of P. calleryana fruit WE and EE for the first time. The chemical composition of P. calleryana fruit WE and EE were analyzed by UHPLC-Q-Orbitrap-MS, and the results showed that P. calleryana fruit was rich in phenolic, flavonoid, and terpenoid compounds. Both WE and EE exhibited significant free radical scavenging effects on ABTS and DPPH, and their DPPH radical scavenging effects were superior or equivalent to that of BHT. In addition, WE and EE exhibited remarkable anti-inflammatory activity with significant inhibition on the release of pro-inflammatory cytokines (IL-6 and TNF-α) and mediators (NO and PGE2) in LPS-stimulated RAW264.7 cells. In enzyme inhibitory activity, P. calleryana fruit WE and EE had significant inhibitory effects on tyrosinase and α-glucosidase, whose inhibitory effect was considerably superior to that of the positive control arbutin and acarbose. Our findings showed that P. calleryana fruit WE and EE possessed significant antioxidant, anti-inflammatory, α-glucosidase and tyrosinase inhibitory properties. Thus, P. calleryana fruit can act as a natural source of antioxidants, anti-inflammatory agents, and tyrosinase and α-glucosidase inhibitors, which has a great potential for application in the field of functional foods. Interestingly, P. calleryana fruit exhibited potent inhibition of tyrosinase and α-glucosidase, indicating that it possesses whitening and hypoglycemic efficacy. Further studies should be conducted on its whitening and hypoglycemic effects in vitro and in vivo to promote its development and utilization in the whitening cosmetics and health food industries.
The original contributions presented in the study are included in the article/Supplementary Material. Further inquiries can be directed to the corresponding author/s.
HZ: Investigation, Methodology, Writing – original draft. QW: Investigation, Validation, Writing – original draft. LY: Investigation, Validation, Writing – original draft. YR: Investigation, Validation, Writing – original draft. QH: Formal analysis, Writing – original draft. YH: Conceptualization, Funding acquisition, Methodology, Supervision, Validation, Writing – original draft, Writing – review & editing. MT: Conceptualization, Funding acquisition, Methodology, Supervision, Validation, Writing – original draft, Writing – review & editing.
The author(s) declare financial support was received for the research, authorship, and/or publication of this article. This work was supported by the National Natural Science Foundation of China (82360834 and 32260747) and the Science and Technology Program of Guizhou province, China (Qian Ke He Zhi Cheng (2020) 1Y133, Qian Ke He Ji Chu-ZK (2021)).
The authors declare that the research was conducted in the absence of any commercial or financial relationships that could be construed as a potential conflict of interest.
The author(s) declare that no Generative AI was used in the creation of this manuscript.
All claims expressed in this article are solely those of the authors and do not necessarily represent those of their affiliated organizations, or those of the publisher, the editors and the reviewers. Any product that may be evaluated in this article, or claim that may be made by its manufacturer, is not guaranteed or endorsed by the publisher.
The Supplementary Material for this article can be found online at: https://www.frontiersin.org/articles/10.3389/fpls.2025.1521990/full#supplementary-material
Abd El-Twab, S. M., Hozayen, W. G., Hussein, O. E., Mahmoud, A. M. (2016). 18β-Glycyrrhetinic acid protects against methotrexate-induced kidney injury by up-regulating the Nrf2/ARE/HO-1 pathway and endogenous antioxidants. Ren. Fail. 38, 1516–1527. doi: 10.1080/0886022X.2016.1216722
Abdul Karim, A., Azlan, A., Ismail, A., Hashim, P., Abd Gani, S. S., Zainudin, B. H., et al. (2014). Phenolic composition, antioxidant, anti-wrinkles and tyrosinase inhibitory activities of cocoa pod extract. BMC Complement. Altern. Med. 14, 381. doi: 10.1186/1472-6882-14-381
Aderogba, M. A., Ndhlala, A. R., Van Staden, J. (2013). Acetylcholinesterase inhibitory activity and mutagenic effects of Croton penduliflorus leaf extract constituents. South Afr. J. Bot. 87, 48–51. doi: 10.1016/j.sajb.2013.03.013
Adewusi, E. A., Moodley, N., Steenkamp, V. (2010). Medicinal plants with cholinesterase inhibitory activity: a review. Afr. J. Biotechnol. 9, 8257–8276. doi: 10.5897/AJB10.1129
Alqudah, A., Qnais, E. Y., Wedyan, M. A., Altaber, S., Bseiso, Y., Oqal, M., et al. (2023). Isorhamnetin reduces glucose level, inflammation, and oxidative stress in high-fat diet/streptozotocin diabetic mice model. Molecules 28, 502. doi: 10.3390/molecules28020502
An, S. M., Kim, H. J., Kim, J. E., Boo, Y. C. (2008). Flavonoids, taxifolin and luteolin attenuate cellular melanogenesis despite increasing tyrosinase protein levels. Phytother. Res. 22, 1200–1207. doi: 10.1002/ptr.2435
Aziz, N., Kim, M. Y., Cho, J. Y. (2018). Anti-inflammatory effects of luteolin: a review of in vitro, in vivo, and in silico studies. J. Ethnopharmacol. 225, 342–358. doi: 10.1016/j.jep.2018.05.019
Brenner, M., Hearing, V. J. (2008). The protective role of melanin against UV damage in human skin. Photochem. Photobiol. 84, 539–549. doi: 10.1111/j.1751-1097.2007.00226.x
Chao, C. Y., Mong, M. C., Chan, K. C., Yin, M. C. (2010). Anti-glycative and anti-inflammatory effects of caffeic acid and ellagic acid in kidney of diabetic mice. Mol. Nutr. Food Res. 54, 388–395. doi: 10.1002/mnfr.200900087
Chern, C. M., Lu, C. K., Liou, K. T., Wang, Y. H., Tsai, K. C., Chang, C. L., et al. (2021). Medicarpin isolated from Radix Hedysari ameliorates brain injury in a murine model of cerebral ischemia. J. Food Drug Anal. 29, 581–605. doi: 10.38212/2224-6614.3377
Chiang, Y. M., Lo, C. P., Chen, Y. P., Wang, S. Y., Yang, N. S., Kuo, Y. H., et al. (2005). Ethyl caffeate suppresses NF-κB activation and its downstream inflammatory mediators, iNOS, COX-2, and PGE2 in vitro or in mouse skin. Br. J. Pharmacol. 146, 352–363. doi: 10.1038/sj.bjp.0706343
China Medical Information Platform (2024). Pyrus calleryana. Available online at: https://www.dayi.org.cn/cmedical/1116678 (Accessed 6 September 2024).
Choi, M. Y., Song, H. S., Hur, H. S., Sim, S. S. (2008). Whitening activity of luteolin related to the inhibition of cAMP pathway in α-MSH-stimulated B16 melanoma cells. Arch. Pharm. Res. 31, 1166–1171. doi: 10.1007/s12272-001-1284-4
Culley, T. M., Hardiman, N. A. (2007). The beginning of a new invasive plant: a history of the ornamental Callery pear in the United States. BioScience 57, 956–964. doi: 10.1641/B571108
Diaz, P., Jeong, S. C., Lee, S., Khoo, C., Koyyalamudi, S. R. (2012). Antioxidant and anti-inflammatory activities of selected medicinal plants and fungi containing phenolic and flavonoid compounds. Chin. Med. 7, 1–9. doi: 10.1186/1749-8546-7-26
Do Nascimento, P. G., Lemos, T. L., Bizerra, A. M., Arriaga, Â.M., Ferreira, D. A., Santiago, G. M., et al. (2014). Antibacterial and antioxidant activities of ursolic acid and derivatives. Molecules 19, 1317–1327. doi: 10.3390/molecules19011317
Faisal, S., Jan, H., Abdullah, Alam, I., Rizwan, M., Hussain, Z., et al. (2022). In vivo analgesic, anti-inflammatory, and anti-diabetic screening of Bacopa monnieri-synthesized copper oxide nanoparticles. ACS Omega 7, 4071–4082. doi: 10.1021/acsomega.1c05410
Gao, Y., Bian, W., Fang, Y., Du, P., Liu, X., Zhao, X., et al. (2022). [amp]]alpha;-Glucosidase inhibitory activity of fermented okara broth started with the strain Bacillus amyloliquefaciens SY07. Molecules 27, 1127. doi: 10.3390/molecules27031127
Ghosh, C., Singh, V., Grandy, J., Pawliszyn, J. (2020). Development and validation of a headspace needle-trap method for rapid quantitative estimation of butylated hydroxytoluene from cosmetics by hand-portable GC-MS. RSC Adv. 10, 6671–6677. doi: 10.1039/C9RA08676E
Goncçalves, S., Romano, A. (2017). “Inhibitory properties of phenolic compounds against enzymes linked with human diseases,” in Phenolic Compounds-Biological Activity. Eds. Soto-Hernández, M., Palma-Tenango, M., Garcia-Mateos, R. (InTech, London), 99–118.
Gong, G., Guan, Y. Y., Zhang, Z. L., Rahman, K., Wang, S. J., Zhou, S., et al. (2020). Isorhamnetin: a review of pharmacological effects. Biomed. Pharmacother. 128, 110301. doi: 10.1016/j.biopha.2020.110301
Gülçin, I. (2006). Antioxidant activity of caffeic acid (3,4-dihydroxycinnamic acid). Toxicology 217, 213–220. doi: 10.1016/j.tox.2005.09.011
Han, D. H., Lee, M. J., Kim, J. H. (2006). Antioxidant and apoptosis-inducing activities of ellagic acid. Anticancer Res. 26, 3601–3606.
Hong, S., Joo, T., Jhoo, J. W. (2015). Antioxidant and anti-inflammatory activities of 3, 5-dicaffeoylquinic acid isolated from Ligularia fischeri leaves. Food Sci. Biotechnol. 24, 257–263. doi: 10.1007/s10068-015-0034-y
Hsu, J. H., Yang, C. S., Chen, J. J. (2022). Antioxidant, anti-α-glucosidase, antityrosinase, and anti-inflammatory activities of bioactive components from Morus alba. Antioxidants 11, 2222. doi: 10.3390/antiox11112222
Huang, J., Xie, M., He, L., Song, X., Cao, T. (2023). Chlorogenic acid: a review on its mechanisms of anti-inflammation, disease treatment, and related delivery systems. Front. Pharmacol. 14. doi: 10.3389/fphar.2023.1218015
Hwang, S. J., Kim, Y. W., Park, Y., Lee, H. J., Kim, K. W. (2014). Anti-inflammatory effects of chlorogenic acid in lipopolysaccharide-stimulated RAW 264.7 cells. Inflamm. Res. 63, 81–90. doi: 10.1007/s00011-013-0674-4
Hyam, S. R., Jang, S. E., Jeong, J. J., Joh, E. H., Han, M. J., Kim, D. H. (2013). Echinocystic acid, a metabolite of lancemaside A, inhibits TNBS-induced colitis in mice. Int. Immunopharmacol. 15, 433–441. doi: 10.1016/j.intimp.2012.12.017
Islam, M. T. (2017). Pharmacological activities of roburic acid: A review of literature. Int. J. Adv. Biochem. Res. 1, 26–28. doi: 10.33545/26174693.2017.v1.i2a.110
Jaroš, P., Vrublevskaya, M., Lokočová, K., Michailidu, J., Kolouchová, I., Demnerová, K. (2022). Boswellia serrata extract as an antibiofilm agent against Candida spp. Microorganisms 10, 171. doi: 10.3390/microorganisms10010171
Jiang, S., Zheng, X., Yu, P., Yue, X., Ahmed, M., Cai, D., et al. (2016). Primitive genepools of Asian pears and their complex hybrid origins inferred from fluorescent sequence-specific amplification polymorphism (SSAP) markers based on LTR retrotransposons. PloS One 11, e0149192. doi: 10.1371/journal.pone.0149192
Kundu, A., Mitra, A. (2013). Flavoring extracts of Hemidesmus indicus roots and Vanilla planifolia pods exhibit in vitro acetylcholinesterase inhibitory activities. Plant Foods Hum. Nutr. 68, 247–253. doi: 10.1007/s11130-013-0363-z
Li, C. W., Chu, Y. C., Huang, C. Y., Fu, S. L., Chen, J. J. (2020). Evaluation of antioxidant and anti-α-glucosidase activities of various solvent extracts and major bioactive components from the seeds of Myristica fragrans. Molecules 25, 5198. doi: 10.3390/molecules25215198
Li, H., Cong, Y., Lin, J., Chang, Y. (2015). Enhanced tolerance and accumulation of heavy metal ions by engineered Escherichia coli expressing Pyrus calleryana phytochelatin synthase. J. Basic Microbiol. 55, 398–405. doi: 10.1002/jobm.201300670
Liu, X. H., Yao, L., Qin, Y. R., Liu, Z. Q., Yu, C. H. (2011). Preliminary on the extraction technics conditions of polysaccharide from Pyrus calleryana Decne. Food Sci. Technol. 36, 159–163. doi: 10.13684/j.cnki.spkj.2011.03.023
Loizzo, M. R., Tundis, R., Menichini, F. (2012). Natural and synthetic tyrosinase inhibitors as antibrowning agents: an update. Compr. Rev. Food Sci. Food Saf. 11, 378–398. doi: 10.1111/j.1541-4337.2012.00191.x
Maleki, S. J., Crespo, J. F., Cabanillas, B. (2019). Anti-inflammatory effects of flavonoids. Food Chem. 299, 125124. doi: 10.1016/j.foodchem.2019.125124
Mansoori, M. N., Raghuvanshi, A., Shukla, P., Awasthi, P., Trivedi, R., Goel, A., et al. (2020). Medicarpin prevents arthritis in post-menopausal conditions by arresting the expansion of TH17 cells and pro-inflammatory cytokines. Int. Immunopharmacol. 82, 106299. doi: 10.1016/j.intimp.2020.106299
Mi, H., Guo, X., Li, J. (2016). Effect of 6-gingerol as natural antioxidant on the lipid oxidation in red drum fillets during refrigerated storage. LWT-Food Sci. Technol. 74, 70–76. doi: 10.1016/j.lwt.2016.07.029
Mohammed, A., Gbonjubola, V. A., Koorbanally, N. A., Islam, M. S. (2017). Inhibition of key enzymes linked to type 2 diabetes by compounds isolated from Aframomum melegueta fruit. Pharm. Biol. 55, 1010–1016. doi: 10.1080/13880209.2017.1286358
Mustafa, R. A., Hamid, A. A., Mohamed, S., Abu Bakar, F. (2010). Total phenolic compounds, flavonoids, and radical scavenging activity of 21 selected tropical plants. J. Food Sci. 75, C28–C35. doi: 10.1111/j.1750-3841.2009.01401.x
Nassar, M. I., Mohamed, T. K., El-Toumy, S. A., Gaara, A. H., El-Kashak, W. A., Brouard, I., et al. (2011). Phenolic metabolites from Pyrus calleryana and evaluation of its free radical scavenging activity. Carbohydr. Res. 346, 64–67. doi: 10.1016/j.carres.2010.11.007
Neimkhum, W., Anuchapreeda, S., Lin, W. C., Lue, S. C., Lee, K. H., Chaiyana, W. (2021). Effects of carissa carandas linn. Fruit, pulp, leaf, and seed on oxidation, inflammation, tyrosinase, matrix metalloproteinase, elastase, and hyaluronidase inhibition. Antioxidants 10, 1345. doi: 10.3390/antiox10091345
Park, H. J., Jo, D. S., Choi, D. S., Bae, J. E., Park, N. Y., Kim, J. B., et al. (2020). Ursolic acid inhibits pigmentation by increasing melanosomal autophagy in B16F1 cells. Biochem. Biophys. Res. Commun. 531, 209–214. doi: 10.1016/j.bbrc.2020.07.125
Pillaiyar, T., Manickam, M., Namasivayam, V. (2017). Skin whitening agents: medicinal chemistry perspective of tyrosinase inhibitors. J. Enzyme Inhib. Med. Chem. 32, 403–425. doi: 10.1080/14756366.2016.1256882
Rafiee, M., Javaheri, M. (2015). A theoretical study of benzaldehyde derivatives as tyrosinase inhibitors using Ab initio calculated NQCC parameters. Mol. Biol. Res. Commun. 4, 151–159. doi: 10.22099/mbrc.2015.3118
Rathee, P., Chaudhary, H., Rathee, S., Rathee, D., Kumar, V., Kanchan, K. (2009). Mechanism of action of flavonoids as anti-inflammatory agents: a review. Inflamm. Allergy-Drug Targets 8, 229–235. doi: 10.2174/187152809788681029
Salau, V. F., Erukainure, O. L., Ibeji, C. U., Olasehinde, T. A., Koorbanally, N. A., Islam, M. S. (2020). Vanillin and vanillic acid modulate antioxidant defense system via amelioration of metabolic complications linked to Fe 2+-induced brain tissues damage. Metab. Brain Dis. 35, 727–738. doi: 10.1007/s11011-020-00545-y
Santamour, F. S., Demuth, P. (1980). Identification of Callery pear cultivars by peroxidase isozyme patterns. J. Hered. 71, 447–449. doi: 10.1093/oxfordjournals.jhered.a109412
Sapkota, S., Boggess, S. L., Trigiano, R. N., Klingeman, W. E., Hadziabdic, D., Coyle, D. R., et al. (2022). Microsatellite loci reveal high genetic diversity, mutation, and migration rates as invasion drivers of Callery pear (Pyrus calleryana) in the Southeastern United States. Front. Genet. 13. doi: 10.3389/fgene.2022.861398
Si, Y. X., Wang, Z. J., Park, D., Chung, H. Y., Wang, S. F., Yan, L., et al. (2012). Effect of hesperetin on tyrosinase: Inhibition kinetics integrated computational simulation study. Int. J. Biol. Macromol. 50, 257–262. doi: 10.1016/j.ijbiomac.2011.11.001
Sima, V. H., Patris, S., Aydogmus, Z., Sarakbi, A., Sandulescu, R., Kauffmann, J. M. (2011). Tyrosinase immobilized magnetic nanobeads for the amperometric assay of enzyme inhibitors: application to the skin whitening agents. Talanta 83, 980–987. doi: 10.1016/j.talanta.2010.11.005
Singh, B., Kumar, A., Singh, H., Kaur, S., Arora, S., Singh, B. (2022). Protective effect of vanillic acid against diabetes and diabetic nephropathy by attenuating oxidative stress and upregulation of NF-κB, TNF-α and COX-2 proteins in rats. Phytother. Res. 36, 1338–1352. doi: 10.1002/ptr.7392
Smeriglio, A., D’Angelo, V., Denaro, M., Trombetta, D., Raimondo, F. M., Germanò, M. P. (2019). Polyphenol characterization, antioxidant and skin whitening properties of Alnus cordata stem bark. Chem. Biodivers. 16, e1900314. doi: 10.1002/cbdv.201900314
Son, J., Lee, S. Y. (2020). Therapeutic potential of ursonic acid: comparison with ursolic acid. Biomolecules 10, 1505. doi: 10.3390/biom10111505
Şöhretoğlu, D., Sari, S. (2020). Flavonoids as alpha-glucosidase inhibitors: mechanistic approaches merged with enzyme kinetics and molecular modelling. Phytochem. Rev. 19, 1081–1092. doi: 10.1007/s11101-019-09610-6
Szwajgier, D., Pielecki, J., Targoński, Z. (2005). Antioxidant activities of cinnamic and benzoic acid derivaties. Acta Sci. Pol. Technol. Aliment. 4, 129–142.
Tai, A., Sawano, T., Ito, H. (2012). Antioxidative properties of vanillic acid esters in multiple antioxidant assays. Biosci. Biotechnol. Biochem. 76, 314–318. doi: 10.1271/bbb.110700
Tai, A., Sawano, T., Yazama, F., Ito, H. (2011). Evaluation of antioxidant activity of vanillin by using multiple antioxidant assays. Biochim. Biophys. Acta 1810, 170–177. doi: 10.1016/j.bbagen.2010.11.004
Tian, T., Chen, G. Y., Zhang, H., Yang, F. Q. (2021b). Personal glucose meter for α-glucosidase inhibitor screening based on the hydrolysis of maltose. Molecules 26, 4638. doi: 10.3390/molecules26154638
Tian, C., Liu, X., Chang, Y., Wang, R., Lv, T., Cui, C., et al. (2021a). Investigation of the anti-inflammatory and antioxidant activities of luteolin, kaempferol, apigenin and quercetin. South Afr. J. Bot. 137, 257–264. doi: 10.1016/j.sajb.2020.10.022
Tian, M., Wang, Q., Jia, X., Tian, Y., Hong, Y., Zhou, Y. (2023). Chemical constituents, antibacterial and anti-inflammatory properties of Pyrus calleryana Dcne. essential oil. Ind. Crop Prod. 204, 117353. doi: 10.1016/j.indcrop.2023.117353
Vanitha, P., Uma, C., Suganya, N., Bhakkiyalakshmi, E., Suriyanarayanan, S., Gunasekaran, P., et al. (2014). Modulatory effects of morin on hyperglycemia by attenuating the hepatic key enzymes of carbohydrate metabolism and β-cell function in streptozotocin-induced diabetic rats. Environ. Toxicol. Pharmacol. 37, 326–335. doi: 10.1016/j.etap.2013.11.017
Wang, Q., Liang, J., Stephen Brennan, C., Ma, L., Li, Y., Lin, X., et al. (2020). Anti-inflammatory effect of alkaloids extracted from Dendrobium aphyllum on macrophage RAW 264.7 cells through NO production and reduced IL-1, IL-6, TNF-α and PGE2 expression. Int. J. Food Sci. Technol. 55, 1255–1264. doi: 10.1111/ijfs.14404
Wang, Y., Zhang, G., Yan, J., Gong, D. (2014). Inhibitory effect of morin on tyrosinase: insights from spectroscopic and molecular docking studies. Food Chem. 163, 226–233. doi: 10.1016/j.foodchem.2014.04.106
Wu, G. M., Wu, F. H. (2012). Processing technology of fermented Pyrus calleryana D. wine. China Brew 31, 162–165.
Yehye, W. A., Rahman, N. A., Ariffin, A., Abd Hamid, S. B., Alhadi, A. A., Kadir, F. A., et al. (2015). Understanding the chemistry behind the antioxidant activities of butylated hydroxytoluene (BHT): a review. Eur. J. Med. Chem. 101, 295–312. doi: 10.1016/j.ejmech.2015.06.026
Yu, Q., Fan, L., Duan, Z. (2019). Five individual polyphenols as tyrosinase inhibitors: inhibitory activity, synergistic effect, action mechanism, and molecular docking. Food Chem. 297, 124910. doi: 10.1016/j.foodchem.2019.05.184
Zabad, I. E. M., Amin, M. N., El-Shishtawy, M. M. (2019). Protective effect of vanillin on diabetic nephropathy by decreasing advanced glycation end products in rats. Life Sci. 239, 117088. doi: 10.1016/j.lfs.2019.117088
Zang, Y., Igarashi, K., Li, Y. (2016). Anti-diabetic effects of luteolin and luteolin-7-O-glucoside on KK-Ay mice. Biosci. Biotechnol. Biochem. 80, 1580–1586. doi: 10.1080/09168451.2015.1116928
Zhao, X., Chen, Q., Lu, T., Wei, F., Yang, Y., Xie, D., et al. (2020a). Chemical composition, antibacterial, anti-inflammatory, and enzyme inhibitory activities of essential oil from Rhynchanthus beesianus rhizome. Molecules 26, 167. doi: 10.3390/molecules26010167
Zhao, D., Jiang, Y., Sun, J., Li, H., Huang, M., Sun, X., et al. (2019). Elucidation of the anti-inflammatory effect of vanillin in LPS-activated THP-1 cells. J. Food Sci. 84, 1920–1928. doi: 10.1111/1750-3841.14693
Zhao, M., Wu, F., Tang, Z., Yang, X., Liu, Y., Wang, F., et al. (2023). Anti-inflammatory and antioxidant activity of ursolic acid: a systematic review and meta-analysis. Front. Pharmacol. 14. doi: 10.3389/fphar.2023.1256946
Zhao, H., Wu, L., Yan, G., Chen, Y., Zhou, M., Wu, Y., et al. (2021). Inflammation and tumor progression: signaling pathways and targeted intervention. Signal Transduction Targeting Ther. 6, 263. doi: 10.1038/s41392-021-00658-5
Zhao, X. L., Yu, L., Zhang, S. D., Ping, K., Ni, H. Y., Qin, X. Y., et al. (2020b). Cryptochlorogenic acid attenuates LPS-induced inflammatory response and oxidative stress via upregulation of the Nrf2/HO-1 signaling pathway in RAW 264.7 macrophages. Int. Immunopharmacol. 83, 106436. doi: 10.1016/j.intimp.2020.106436
Keywords: Pyrus calleryana, chemical components, antioxidant, anti-inflammatory effect, enzyme inhibitory activity
Citation: Zhao H, Wang Q, Yang L, Ran Y, Hu Q, Hong Y and Tian M (2025) Phytochemical analysis, antioxidant, anti-inflammatory and enzyme inhibitory activities of bean pear (Pyrus calleryana fruit). Front. Plant Sci. 16:1521990. doi: 10.3389/fpls.2025.1521990
Received: 03 November 2024; Accepted: 23 January 2025;
Published: 07 February 2025.
Edited by:
Marta Olech, Medical University of Lublin, PolandReviewed by:
Rocio Rodriguez Arcos, Spanish National Research Council (CSIC), SpainCopyright © 2025 Zhao, Wang, Yang, Ran, Hu, Hong and Tian. This is an open-access article distributed under the terms of the Creative Commons Attribution License (CC BY). The use, distribution or reproduction in other forums is permitted, provided the original author(s) and the copyright owner(s) are credited and that the original publication in this journal is cited, in accordance with accepted academic practice. No use, distribution or reproduction is permitted which does not comply with these terms.
*Correspondence: Yi Hong, eWhvbmcxQGd6dS5lZHUuY24=; Minyi Tian, bXl0aWFuQGd6dS5lZHUuY24=
Disclaimer: All claims expressed in this article are solely those of the authors and do not necessarily represent those of their affiliated organizations, or those of the publisher, the editors and the reviewers. Any product that may be evaluated in this article or claim that may be made by its manufacturer is not guaranteed or endorsed by the publisher.
Research integrity at Frontiers
Learn more about the work of our research integrity team to safeguard the quality of each article we publish.