- 1National Key Laboratory of Cotton Bio-breeding and Integrated Utilization, School of Agricultural Sciences, Zhengzhou University, Zhengzhou, China
- 2Henan Key Laboratory of Ion-Beam Green Agriculture Bioengineering, School of Agricultural Sciences, Zhengzhou University, Zhengzhou, China
With the growth of the global population and the increasing scarcity of resources, the sustainability and efficiency improvement of agricultural production have become urgent needs. The rapid development of nanotechnology provides new solutions to this challenge, especially the application of nanoparticles in agriculture, which is gradually demonstrating its unique advantages and broad prospects. Nonetheless, various nanoparticles can influence plant growth in diverse manners, often through distinct mechanisms of action. Beyond their direct effects on the plant itself, they frequently alter the physicochemical properties of the soil and modulate the structure of microbial communities in the rhizosphere. This review focuses intently on the diverse methods through which nanoparticles can modulate plant growth, delving deeply into the interactions between nanoparticles and plants, as well as nanoparticles with soil and microbial communities. The aim is to offer a comprehensive reference for the utilization of functionalized nanoparticles in the agricultural sector.
1 Introduction
In recent years, with the rapid development of agricultural technology and the increasing awareness of environmental protection, the demand for new materials and technologies in the field of agricultural production has become increasingly urgent (Moretti and Marucci, 2019; Magnabosco et al., 2023). The prolonged use of chemicals, pesticides, and fertilizers can indeed ease the challenges of food security in the long term. However, this practice poses risks such as contamination, soil fertility loss, non-target species impact, disease/insect resistance, biodiversity decline, and harm to humans/animals (Yousef et al., 2023; Chandrasekaran and Paramasivan, 2024). Consequently, there is an urgent need for innovative and efficient agricultural technologies to address the global challenges of food production and security (Thorakkattu et al., 2024).
Nanoparticles, often abbreviated as NPs, are regarded as materials ranging from 1 to 100 nm in size, and have different sizes, geometry, physical shape, mechanical strength and chemical composition, which have attracted people attention due to their wide application prospects (Lowry et al., 2019; Ravichandran et al., 2021; dos Santos et al., 2022; Garg et al., 2024; Sun et al., 2024). Nanotechnology has been widely applied across various sectors, including biomedicine, agriculture, and environmental remediation. The United Nations Food and Agriculture Organization (FAO) and the World Bank are actively encouraging the integration of nanotechnology into agricultural practices, with the development of sustainable agricultural systems being a central goal of current nanotechnological applications (De Chiffre et al., 2003; Mishra et al., 2017; Kah et al., 2019; Ahmad et al., 2022; Wang et al., 2022). Compared to traditional agricultural technology, nano-agricultural technology offers numerous advantages, closely linked to enhancements in production efficiency, reductions in input costs, and diminished ecotoxicity (Servin et al., 2015; Kah et al., 2019; Zhang et al., 2024). For example, zinc oxide, silver oxide nanoparticles had been explored as effective slow-release nanofertilizers, transport carriers, and bacteriostatic agents to provide plants with essential nutrients and inhibit pathogens, thus promoting plant growth and increasing crop yields (Elhaj Baddar and Unrine, 2018; Sun et al., 2018; Shireen Akhter Jahan et al., 2024). The application of nanoparticles to soil can influence its physical and chemical properties, the metabolic richness of plant roots, and the activity of the rhizosphere microbial community (Dimkpa et al., 2013; Sarma et al., 2024). Furthermore, the physical and chemical characteristics of soil, including texture, organic matter content, and pH level, inherently influence the migration and morphology of nanoparticles within the soil, which impact the bioavailability of nanoparticles (Cornelis et al., 2014; Reith and Cornelis, 2017; Gómez-Sagasti et al., 2019). The ecological functions of nanoparticles and their environmental impacts are current research focal points. However, their effects on soil health and agricultural applications are of greater practical significance. In particular, the influence of nanoparticles on rhizosphere microorganisms and plant physiology is crucial for enhancing research into sustainable agricultural development strategies.
Land plants interact with soil microorganisms through their roots, making rhizosphere microbial community crucial for soil health and crop growth (Ding et al., 2019; Zhou et al., 2020; Kusiak et al., 2022). Compared to the perpendicular soil (soil not connected to the roots and soil falling after root shaking) (Ding et al., 2019), rhizosphere soil (the soil within 1 mm of the root surface) is teeming with a multitude of microorganisms exhibiting high biological and chemical activity, which is directly linked to the stability and productivity of agricultural production systems (Kaye et al., 2005; Edwards et al., 2015). Numerous recent studies have demonstrated the enhanced efficacy of nanoagents in regulating the plant rhizosphere microbiome compared to traditional non-nanometer approaches (Ahmed et al., 2022; Ahmed et al., 2023). Moreover, the regulation of microbiomes using nanoagents has the potential to enhance plant growth through a variety of mechanisms (Ahmed et al., 2022; Ahmed et al., 2023). Secondly, nanoparticles can indirectly promote plant nutrient absorption and ultimately promote plant growth by increasing the richness of rhizosphere microbiota (Xu et al., 2023). For example, pepper plants treated with Nano-selenium could significantly enhance the presence of beneficial microorganisms in the rhizosphere soil, including Gammaproteobacteria, Alphaproteobacteria, Bacteroidetes, Gemmatimonadetes, and Deltaproteobacteria, as well as Anaerolineae (Li et al., 2022). These alterations in microbial communities lead to a substantial increase in soil enzyme content, soil metabolites such as fluorescein diacetate, urease, brassinosteroids, and p-hydroxybenzoic acid, and plant metabolites like rutin, luteolin, brassinosteroids, and abscisic acid, which enhanced the contribute to bolstering plant defense mechanisms and improving plant growth (Li et al., 2022). Rhizosphere microorganisms promoted plant growth by providing nutrients and hormones, while the metabolites secreted by plant roots could also change the species and number of rhizosphere microbial communities (Hwangbo et al., 2016; Khoiri et al., 2024). Consequently, the creation and application of suitable nanoparticles in agriculture are anticipated to not only improve but potentially supplant the use of chemical pesticides and fertilizers.
This review delves into the utilization of nanoparticles within agricultural production, examining how these particles can foster plant growth through various mechanisms: (i) enhancing nutrient absorption, (ii) facilitating controlled release of nutrients, (iii) enabling precise delivery of nutrients to targeted locations, and (iv) augmenting the population of beneficial microorganisms in the rhizosphere while suppressing those that are pathogenic and detrimental to plant development. Nanoparticles can stimulate plant growth both directly, by enhancing physiological processes, and indirectly, by modulating the beneficial microorganisms in the rhizosphere and altering soil conditions. Consequently, strategies driven by nanotechnology offer a promising and sustainable approach to boost crop growth and bolster crop resilience against stress factors.
2 Nanoparticles enhance nutrient absorption
Nanoparticles hold great potential for enhancing nutrient uptake, and certain nanoparticles can improve plant nutrient utilization efficiency by employing mechanisms such as directional delivery, sustained or controlled release (Solanki et al., 2015; Dutta et al., 2022; Taware et al., 2024) (Figure 1). During the promotion of plant nutrient uptake, nanoparticles can influence nutrient absorption in two ways. On the one hand, they can act as carriers for nutrients, and on the other, they can modulate soil microorganisms to enhance the plant’s nutrient absorption capabilities (Bortoletto-Santos et al., 2020; Khan et al., 2022); Conversely, nutrient elements could be precisely delivered to various regions of plants via nanoparticle carriers, thereby enhancing the efficiency of nutrient utilization (Fincheira et al., 2021).
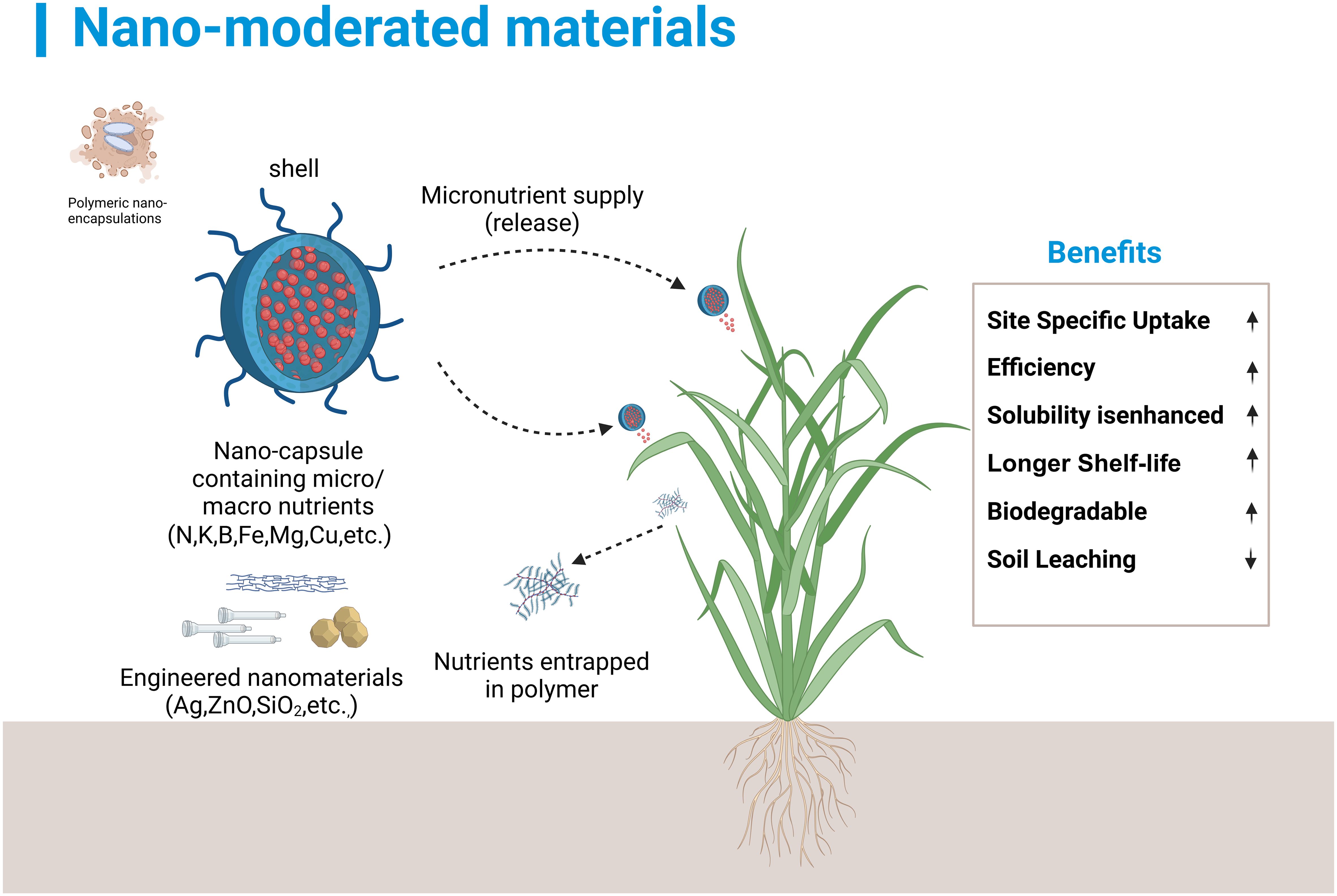
Figure 1. Nanoparticle-based protective agents or carriers are designed to regulate the discharge of active compounds, enhance stability, and control the release rate, thereby achieving sustainable agricultural practices.
2.1 Nanoparticles function as carriers for slow-release fertilizers, enhancing the absorption of nutrients by plant roots.
At present, nanoparticles find extensive application in the realms of energy, electronics, and architecture, yet their utilization in agriculture-related domains remains comparatively limited (de Silva et al., 2020). It possesses the potential for the slow release of fertilizers, owing to its diminutive size, substantial surface area, robust adsorption capacity, and the capability to control the release kinetics at the target site (Ghormade et al., 2011; Zulfiqar et al., 2019; Al-Mamun et al., 2021). The nanoparticles’ diminutive scale and elevated reactivity allow them to penetrate the plant cell wall with greater ease, thereby enhancing the transport and absorption of nutrients throughout the plant (Chhipa, 2017). Furthermore, it enhances fertilizer adsorption efficiency and stability, allowing slow, sustained nutrient release. This fosters plant growth, preserves beneficial microbiota diversity, mitigates eutrophication runoff, and prevents pollution (Kalwani et al., 2022).
Research conducted on nanofertilizers in aqueous environments revealed that a 40% urea-hydroxyapatite formulation demonstrated a controlled release of nitrogen, capable of sustaining the process for up to one week., while pure urea depleted within just a few minutes (Kottegoda et al., 2017). Likewise, the gradual and continuous release of urea from urea-silica nanohybrids prevents premature depletion of urea, ensuring effective and precise delivery of nitrogen and silica to the plant (de Silva et al., 2020).
Nanoparticles can also exert their slow-release function within soil media, markedly enhancing the efficiency of nutrient utilization. For instance, in the study of indigenous wheat irrigated nanohybrid, it was found that urea molecules were slowly released in doped Zn and Mg hydroxyapatite nanohybrids for up to two weeks, and this nanocompound containing only a 50% nitrogen dose maintained wheat crop yield and nitrogen nutrient uptake (Sharma et al., 2022). The recent research had revealed that a nanocarrier with a core and shell structure, composed of urea-loaded Metal-Organic Frameworks (MOFs) and silica, can facilitate the sustained release of nitrogen to crops. When utilizing urea/MIL-100(Fe)/silica heterojunction nanomaterials to treat potted rice, the nitrogen use efficiency of the rice was found to be 34.7% greater than that achieved with traditional urea treatment (Wu et al., 2024).
During the growth cycle of rice, urea-coated hydroxyapatite nanoparticles (urea coated hydroxyapatite nanoparticles, UHA) were released more slowly than conventional urea (Bhavani et al., 2020). Nano U-NPK(containing Ca, P, K, NO3 and urea multi-nutrient nanofertilizer) not only ensures the slow release of the most crucial plant macronutrients(N, P, K), but also has the potential to reduce the nitrogen supply to plants by 40% (Ramírez-Rodríguez et al., 2020). Potassium-based nanoparticles (K2SiO3-NP, K18Mo8O33-NPs) compared with traditional potassium fertilizer, its utilization efficiency was about 40% higher in soybean growth, mainly due to the slow release effect of the nanoparticles, which can provide potassium ions for a longer time, effectively avoiding the toxic effect of large dose rapid delivery (Wang et al., 2024). The montmorillonite nano-hybrid composite was capable of decelerating the release rate of nitrogen across various pH conditions, extending its duration to foster plant growth (Madusanka et al., 2017). The latest study found that a special slow-release fertilizer: milk, could be made into high-fertilizer fertilizer rich in fulvic acid and potassium. The pot experiment results showed that the slow-release fertilizer was slow and more significant fertilizer effect than the traditional organic fertilizer and potassium fertilizer, and had good acid soil restoration effect (Zhu et al., 2024). In conclusion, tailored nanofertilizers have the potential to not only enhance plant growth but also to improve the soil’s physiochemical properties, playing a pivotal role in the advancement of sustainable agriculture.
2.2 Nanoparticles are capable of precisely delivering nutrients
Nanoparticles had improved the nutrient uptake in plants through the precise application of chemical fertilizers and plant growth regulators, thereby fostering innovation within the agricultural sector and offering a novel strategic approach for precision agriculture (Ghosh et al., 2023; Saberi Riseh et al., 2024; Singh et al., 2024). Research had indicated that a seed coating composed of a zinc and urea hydroxyapatite nanohybrid can more precisely deliver plant nutrients and enhance the efficiency of nutrient utilization (Abeywardana et al., 2021). Under acidic soil conditions, innovative phosphate hydroxyapatite nanofertilizers, specifically Hydroxyapatite nanoparticles (HA-NPs), were administered to sunflower crops, demonstrating a significantly faster and more efficient phosphate uptake compared to conventional phosphate and triphosphate fertilizers (Xiong et al., 2018). The design of nanocarriers represents a pivotal avenue for future research into the precise delivery of nanoparticles. The strategic development of nanocarriers that can target tissues and organs within plants and organic matter will facilitate precise control over plant absorption, decrease input requirements, and minimize energy wastage.
3 The Impact of nanoparticles on rhizosphere microbial communities
Nanoparticles engage in a range of physical, chemical, and biological processes, such as vulcanization, flocculation, precipitation, and adsorption, which enable them to interact with soil organic matter, plants, and root microorganisms (Chang et al., 2024). The study indicated that parameters including soil texture, pH, redox potential, organic matter content, and cation exchange capacity influence the chemical properties of nanoparticles (Ben-Moshe et al., 2013; Gao et al., 2019). When nanoparticles enter the soil system, they start to regulate the physiological, biochemical and genetic mechanisms of soil microorganisms; after entering the plant system, they were transferred to xylem tissue and then transported to other tissues to regulate the adaptability of the plant environment (Ahmed et al., 2023). In this section, we primarily explore the function and potential applications of nanoparticles in enhancing the rhizosphere soil microenvironment and fostering interactions among rhizosphere microbes.
3.1 Nanoparticles for enhancing the soil microenvironment in the rhizosphere
Research indicated that nanoparticles could alter soil structure by decreasing the surface energy and enhancing the water repellency of soil particles, while also increasing soil water conductivity and aggregate stability (Chen et al., 2022). These changes could impact the soil water cycle, influencing aspects such as water retention and evaporation rates. Concurrently, alterations in soil structure could also modulate the distribution of soil pH levels and nutrient elements, subsequently influencing the beneficial microbial community within the rhizosphere (Wang et al., 2020). For example, the newly developed biochar-enriched phosphorus-doped aqueous solutions, in conjunction with iron ore nanoparticles, had the capability to alter the soil pH balance, concurrently enhancing the soil’s organic matter and phosphorus concentrations (Li et al., 2022). Nanoparticles alter the structure, content, and diversity of advantageous microbial communities within plants, influencing the interaction within the plant-soil-microbial community system, and a robust soil structure further enhances the activity and nutrient cycling of soil microorganisms (Schjønning et al., 2011; Das et al., 2023; You et al., 2023; Zhang et al., 2023). Research had indicated that the application of carbon nanoparticles (CNPs) to soil could improve its water retention capacity, but also positively influence the functionality of soil microorganisms, thereby indirectly supporting plant growth (Xin et al., 2022). Concurrently, CNPs, which infiltrate plant roots via stomatal penetration or adhere to the epidermis, can stimulate soil microorganisms and enhance enzyme activities (Xin et al., 2022). This, in turn, can improve soil fertility and quality (Figure 2).
Nanoparticles have the potential to foster the proliferation of microbial community diversity by altering the elemental composition of the soil (Karimian and Samiei, 2023). CNPs can significantly enhance the soil’s nitrogen and phosphorus content, thereby fostering a thriving environment for microbial growth-related enzymes, which in turn promotes soil health and fertility (Zhao et al., 2021). Biologically synthesized nanoparticles exert a beneficial influence on organic carbon and microorganisms within soils used for corn cultivation, thereby accelerating plant growth (Haider et al., 2015). Innovative semi-polymer nanocomposite particles possess the capability to significantly augment the concentrations of both organic carbon and active organic carbon, thereby fostering the vigorous growth and development of plants (Zhao et al., 2019). The aforementioned studies indicate that nanoparticles have the potential to enhance soil structure, pH levels, and various other properties, which in turn can influence the interactions among plants, soil, and microbes. However, the precise manner in which these soil properties impact the interplay between these three elements warrants further investigation.
3.2 Nanoparticles promote the interaction between rhizosphere microorganisms and plants
The rhizosphere is regarded as the soil’s most abundant reservoir of organic matter, serving as the primary zone for microbial proliferation and activity (Reinhold-Hurek et al., 2015). The rhizosphere microbial communities play a pivotal role in various beneficial plant growth processes, including nitrogen fixation, nutrient decomposition, and the synthesis of bioactive metabolites (Bi et al., 2021; Chauhan et al., 2023). Owing to their minute particle dimensions, surface functionalization, and unique chemical properties, nanoparticles are employed to enhance plant nutrient absorption and stress tolerance (Lv et al., 2019). Presently, the majority of research into the utilization of nanoparticles is centered on the plants alone, overlooking the impact of nanoparticles on soil microorganisms and their metabolites during this process.
The interaction between rhizosphere secretions and rhizosphere microorganisms is bidirectional, indicating that the metabolites excreted by plant roots play a role in shaping rhizosphere microbial communities; conversely, rhizosphere microorganisms are intimately connected to plant growth and health (Staley et al., 2017; Hu et al., 2018; Trivedi et al., 2020). Nevertheless, a considerable amount of uncertainty persists regarding the interplay between secretions and microbes in the rhizosphere.
The interaction between nanoparticles and plants can significantly promote the generation of metabolites in plant cells and the increase of rhizosphere secretions (Francis et al., 2024). These bioactive substances, once in contact with nanoparticles, indirectly have a profound impact on their key properties such as dispersion stability, aggregation state, and solubility, which has been confirmed in relevant research (McManus et al., 2018; Cervantes-Avilés et al., 2021). Specifically, biomolecules in plant cell metabolites and rhizosphere secretions (amino acids, sugars, phenolic compounds, and other secondary metabolites), Its functional groups have high reactivity and can quickly adsorb onto the surface of nanoparticles through competitive interactions, forming a surface ecological corona (eco-corona) (Nasser and Lynch, 2016; Wheeler et al., 2021). This ecological corona not only changes the physical and chemical properties of nanoparticles, but also further affects their migration, transformation, and biological effects in the soil environment, thus forming a complex ecological interaction network (Kang et al., 2024). This ecological corona phenomenon has a significant impact on the bioavailability of nanoparticles by crops, and its effect exhibits duality. In certain specific contexts, it may have a positive impact, promoting crop uptake and utilization of nanoparticle nutrients; However, in other cases, it may also have adverse effects, interfering with the normal physiological processes of crops. When ecological corona enhances the dispersion and stability of nanoparticles in soil or rhizosphere environment, it helps crop roots to more effectively contact and uptake these nanoparticles, thereby improving bioavailability and promoting crop growth and development. The organic acids in soybean rhizosphere secretions bind to the surface of nanoparticles (CeO2, Mn3O4, Cu(OH)2, MoO3), greatly reducing the bioavailability and delivery efficiency of these agricultural nano chemicals (Cervantes-Avilés et al., 2021). However, if ecological corona leads to an increase in the aggregation degree or a decrease in the solubility of nanoparticles, it may hinder the effective absorption of nanoparticles by crops. For example, the rhizosphere secretion of wheat increases the solubility of CuO nanoparticles in alkaline soil, thereby enhancing their bioavailability by crops (Hortin et al., 2020). Therefore, when using nanoparticles for agricultural production, it is necessary to fully consider the impact of ecological corona phenomenon on crop bioavailability. Through scientific and reasonable nanoparticle design and application strategies, the positive effects can be maximized while minimizing potential risks, in order to achieve sustainable development of agricultural production.
Research has indicated that nanoparticles frequently influence rhizosphere microorganisms by stimulating the secretion of root metabolites. For instance, the adsorption of zinc oxide nanoparticles (ZnO NPs) and their aged counterpart, s-ZnO NPs, onto the epidermis of legume roots triggers a stress response that results in the production of numerous root metabolites, including amino acids and terpenoids (Liu et al., 2023). These metabolites could directly impact soil organic matter or activate microbial decomposition of organic carbon, thereby enhancing the release and breakdown of organic carbon within the rhizosphere (Liu et al., 2023). Selenium nanoparticles (Se NPs) enhanced organic acid biosynthesis and transport genes in plants, directly enhance malate and citric acid secretion in rice roots, and then recruit sphingomonas and Streptomyces, to enhance their interaction with rice and promote the growth of rice (Jiao et al., 2023). The application of Silica dioxide nanoparticles (SiO2 NPs) stimulated the synthesis, transport, and secretion of organic acids in rice roots, which provided a rich carbon source for rhizosphere microorganisms, increases the abundance of beneficial microorganisms such as Proproteobacteria and Actinobacteria in the rhizosphere by 15.2-80.5%, promotes the optimization of the bacterial community, and facilitates the absorption and growth of nitrogen in plants (Yue et al., 2023). It has been reported that compounds released by root exudates and rhizosphere microorganisms can form complexes with metal ions, thereby influencing their bioavailability to plants and microorganisms (Chen et al., 2017). Fe3O4 nanoparticles encapsulated in citrate (CA) release a higher solubility of iron and interact with root exudates, which modulate plant hormones to stimulate root elongation, thereby enhancing plant growth (Sun et al., 2023). Consequently, nanoparticles can be employed to modulate rhizosphere secretions, thereby influencing the metabolic processes and community dynamics of rhizosphere microorganisms, which in turn can facilitate the emission of plant root exudates (Steinauer et al., 2016). The studies conducted clearly indicate that nanoparticles have the capacity to modify the rhizosphere microbiome, thereby enhancing the population of beneficial microbes. To fully harness the agricultural benefits, it is imperative to gain a more profound and holistic comprehension of how nanoparticles influence the interplay between root exudates and the rhizosphere microbiome.
3.3 Nanoparticles suppress plant pathogenic microorganisms
Plant diseases can pose a formidable threat to the productivity and quality of plants. Certain nanoparticles, harnessing their exceptional cell penetration abilities and unique surface properties, demonstrated a promising potential for inhibiting a diverse array of pathogenic microorganisms, thereby ultimately fulfilling the objective of managing and controlling plant diseases effectively (Gordienko et al., 2019; Gao et al., 2023; Rahimizadeh et al., 2023; Scandolera et al., 2024). The most widely studied nanoparticles currently are those of silver, copper, zinc, silicon, etc. These particles usually possess special physical and chemical properties that enable them to interact effectively with plant pathogens (directly destroying the cell membranes of plant pathogenic bacteria, causing lysis of the pathogenic cells and a decrease in pathogenic activity) and inhibit their growth and spread, thereby effectively reducing the incidence and severity of plant diseases (Andleeb et al., 2021; Jaithon et al., 2022). Figure 3 enumerated the application of nanoparticles in five common plant diseases.
Research has indicated that nanoparticles predominantly contribute to the management of plant diseases by disrupting the morphological structure, sporulation capacity, and adhesion behavior of pathogens (Table 1). Silver-associated nanoparticles currently stand out as the most effective in combating pathogenic microorganisms, with their antibacterial properties being particularly evident in organisms such as Ustilaginoidea virens, Ralstonia solanacearum, and Xanthomonas perforans (Pisárčik et al., 2021). The antimicrobial efficacy of these silver nanoparticles was intricately linked to their unique physicochemical characteristics, including concentration, particle size, pH, and other factors (Pisárčik et al., 2021). For example, the growth inhibition of rice false smut fungus by nanosilver was concentration-dependent, and nanosilver at a median effective concentration could significantly inhibit the sporulation and pathogenicity of the fungus. In addition, Ag NPs reduced the H3K27me3 modification mediated by UvKmt6, leading to the upregulation of genes involved in biosynthesis of oryzalide, and the decrease in H3K27me3 levels is associated with the inhibition of mycelial growth (Wen et al., 2023). In addition to combating fungal and bacterial pathogens, silver nanoparticles could also diminish the concentration of Bean Yellow Mosaic Virus (BYMV) and alleviate disease severity in broad beans (Abdelkhalek et al., 2023). Nano biotechnology has revealed that copper nanoparticles, particularly tobacco-derived copper oxide nanoparticles (CuO NPs), exhibit a pronounced antibacterial effect that is concentration-dependent. Scanning electron microscopy (SEM) and transmission electron microscopy (TEM) observations have shown that CuO NPs can disrupt the hyphal cell wall, resulting in a rough and convex surface (Chen et al., 2022). Additionally, there is evidence of significant partial collapse and bending of the hyphae (Chen et al., 2022). This phenomenon occurs because CuO NPs aggregate and adhere to the hyphal cell wall, and may even penetrate the cell membrane (Chen et al., 2022). The direct contact between nanoparticles and the hyphae leads to the accumulation of reactive oxygen species (ROS) and a corresponding increase in hyphal superoxide dismutase (SOD) enzyme activity (Chen et al., 2022). A recent study showed that copper nanoparticles (Cu NPs) also play an important role in the inhibition of bacterial fruit spot disease in watermelon, also by inducing oxidative stress and destroying cell integrity (Noman et al., 2023). Table 1 offers a comprehensive overview of the utilization of nanoparticles as antimicrobial agents.
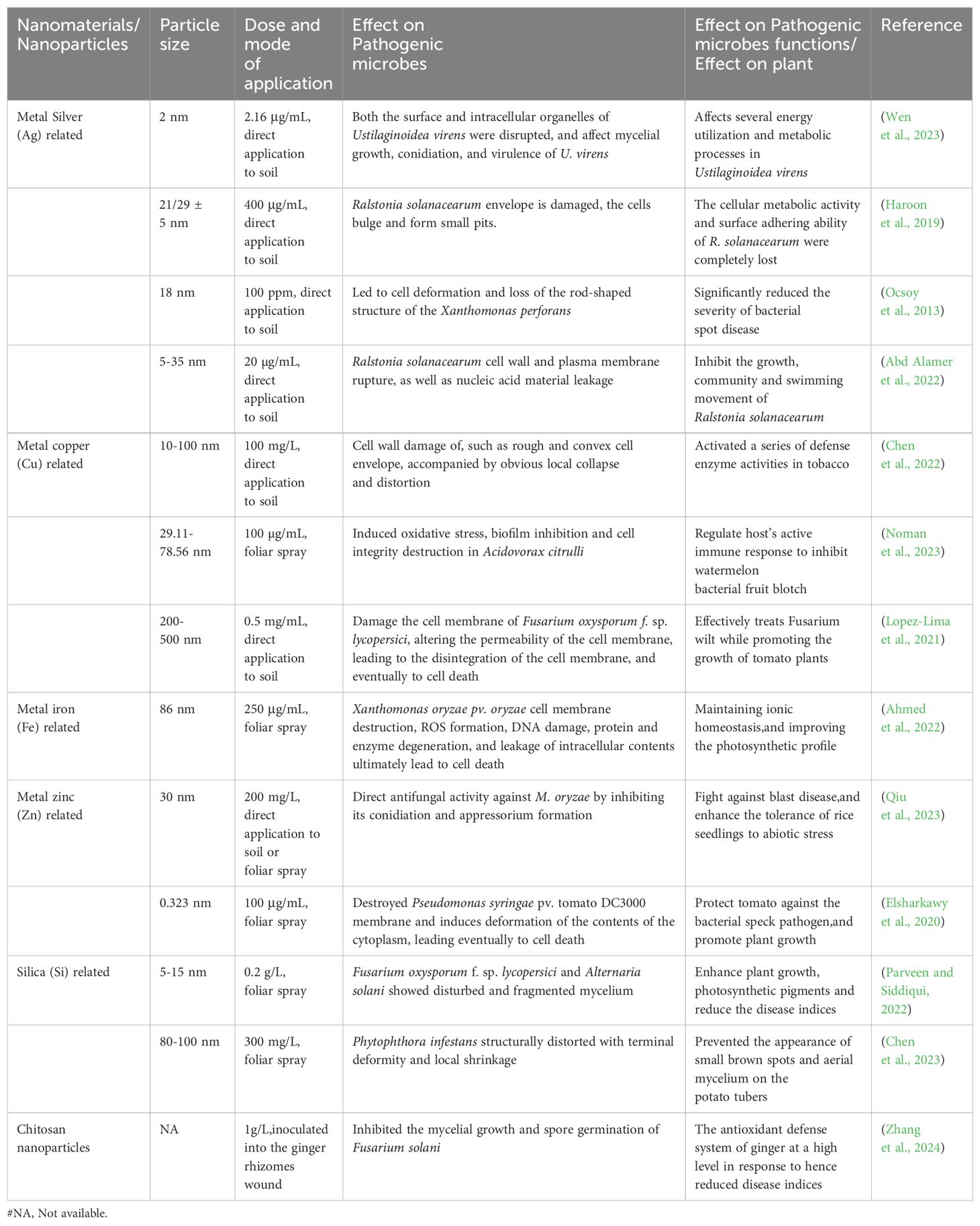
Table 1. Effect of various nanofertilizer/nanoparticles on pathogenic microbes and microbial functions.
In conclusion, the antimicrobial properties of nanoparticles are derived from their unique physical structure and chemical reactivity. Nonetheless, despite their demonstrated efficacy in inhibiting pathogenic microorganisms, practical applications must take into account their stability, biocompatibility, environmental impact, and cost-effectiveness.
3.4 Nanoparticles boost the efficacy of probiotic microorganisms
Numerous nanoparticles have the potential to improve nutrient transport and soil fertility by modulating the function, species diversity, or population size of the microbial community within the rhizosphere (Wei et al., 2020). Foliar application or direct application of nanoparticle solutions could influence the function of rhizosphere microbial communities in plant root nutrient uptake, nitrogen regulation, and the regulation of related enzyme activities, thereby impacting plant growth and development (Figure 4) (Wu et al., 2023).
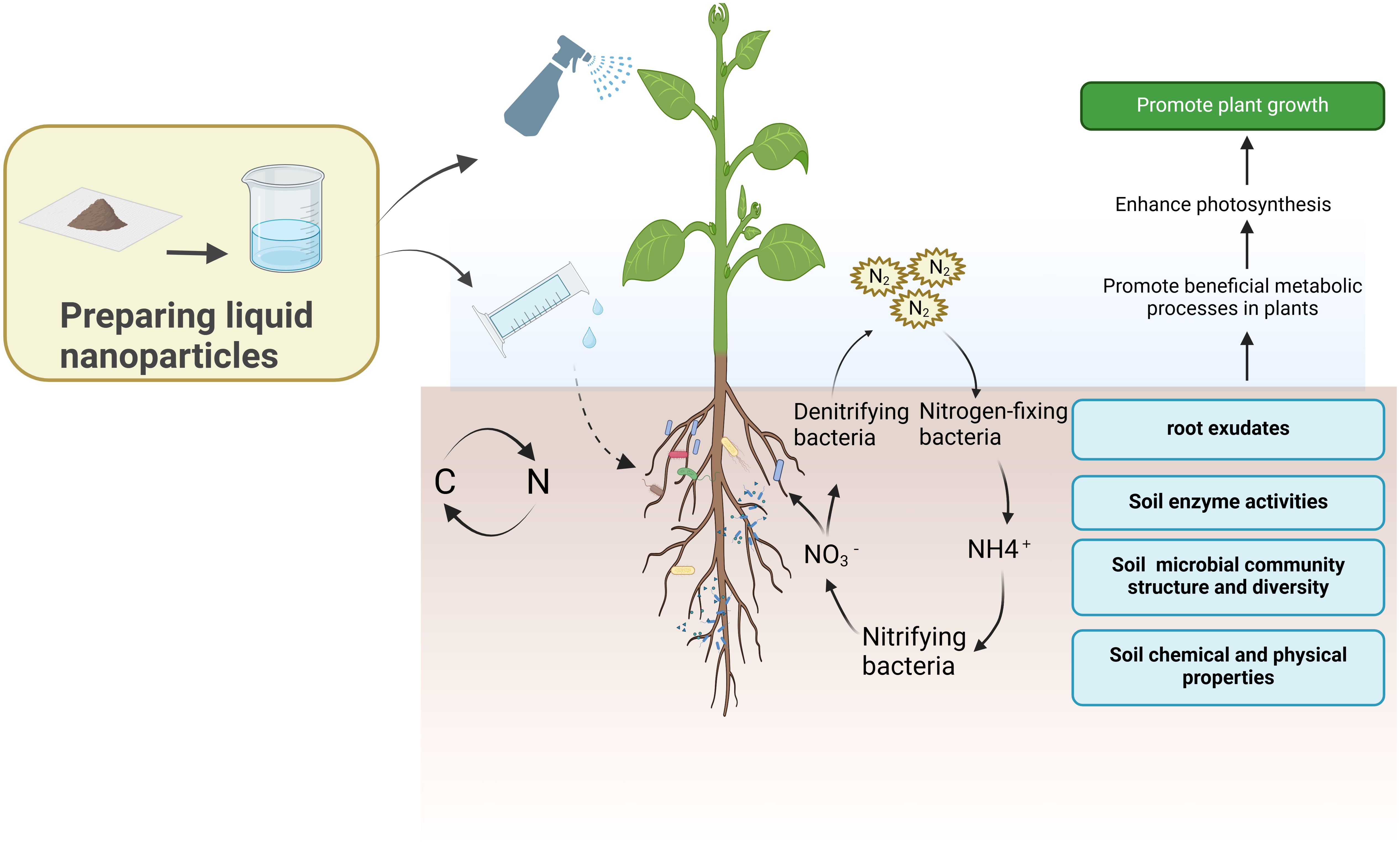
Figure 4. Nanoparticles enhance the activity of beneficial microbes within the rhizosphere, modulate soil metabolic processes, and subsequently foster plant growth and development.
Throughout the phase of accelerated plant growth, the high nutrient demand entices a larger concentration of rhizosphere microorganisms, which play a crucial role in the plants’ nutrient absorption (Chaparro et al., 2014). Simultaneously, the interplay between nanoparticles and rhizosphere microorganisms could enhance plant growth and improve soil health (Tripathi et al., 2024). The research indicated that in the developmental phase of rice seedlings, selenium nanoparticles (Se NPs) enhance the abundance of sphingomonas and various other bacterial species, while also encouraging the secretion of root exudates (Jiao et al., 2023). These combined influenced synergistically modulate nutrient uptake and foster the growth of rice plants (Jiao et al., 2023).In Brassica chinensis L., there exists a comparable regulatory function throughout the growth process (Wang et al., 2022). The symbiotic relationship between rhizosphere microorganisms and plants enhanced the bioavailability of nutrients within the rhizosphere soil, thereby augmenting the plants’ nutrient uptake capabilities (Xu et al., 2023). The application of zinc oxide quantum dots (ZnO QDs) during the growth phase of pumpkins aids in enhancing beneficial microorganisms within the endophytic and rhizosphere environments, thus promoting nutrient uptake and plant growth (Xu et al., 2023). It has been shown that the 50 mg/kg of Fe7(PO4)6 nanoparticle treatment of tomato will increase the relative abundance of beneficial microorganisms associated with nutrient accumulation, which will accelerate nutrient accumulation (Jiao et al., 2024).
Numerous studies have demonstrated that the availability of soil nitrogen and the uptake of nitrogen by plants play a crucial role in determining crop yield. Concurrently, the absorption, excretion, and transformation of soil nitrogen are largely contingent upon the interactions within the rhizosphere microbial communities (Yang et al., 2019; Yu et al., 2019). The effective and sustainable supply of nitrogen in the rhizosphere soil mainly depends on specific rhizosphere microbial communities that convert inert nitrogen into nitrogen compounds. Therefore, nitrogen transformation driven by rhizosphere microbial communities was a significant determinant of plant nitrogen uptake (Moreau et al., 2019). For example, the application of FeNPs to alfalfa increased the diversity of the rhizosphere microbial community, further enhancing the nitrogen-fixing ability of the roots (Zhang et al., 2024). Silver nanoparticles (AgNPs) enhanced the population of rhizosphere bacteria, including Saccharimonadia (also known as Plant Growth Promoting Rhizobacteria, or PGPR) (Sellstedt et al., 2013; Francioli et al., 2021). Certain strains of these bacteria facilitate atmospheric nitrogen fixation, while others contribute to plant growth and aid in nutrient transformation (Sellstedt et al., 2013; Francioli et al., 2021). Table 2 offers a comprehensive overview of the enhancement of diverse nanoparticles on various beneficial microbes within the rhizosphere, along with their affirmative impacts on plant growth.
Although numerous studies have extensively reported the positive effects of nanoparticles on plant and rhizosphere microbial community diversity, there are still some studies suggesting that the effects of nanoparticles on plants and microorganisms may exhibit dose-dependent effects, especially under high concentration conditions, where they may have inhibitory effects on plant growth and some rhizosphere microbial communities (Saghaï et al., 2022; Ren et al., 2024). For example, in plant growth experiments, low concentrations of ZnO nanoparticles promoted the growth of Vigna radiata and Cicer arietinum seedlings. Treatment concentrations of nanoparticles higher than 20 ppm and 1 ppm, respectively, would inhibit plant growth (Mahajan et al., 2011). At the same time, 1.2 mM low concentration ZnO nanoparticles can also promote the germination and metabolic activity of Solanum lycopersicum, while the germination and metabolic activity of plants above this concentration are inhibited (Singh et al., 2016). High concentrations of nanoparticles not only affect seed germination and plant growth rate, but also affect the level of edible nutrients. Low concentrations of TiO2 nanoparticles (50 mg/L) can increase the level of nutrients in Coriandrum sativum L., while high concentrations can reduce the decrease in edible nutrient content and inhibit growth. In terms of microbial activity, it usually follows a dose-dependent pattern, which is also related to the type of nanoparticles (Yang et al., 2021; Lin et al., 2022). Low dose nanoparticles may have beneficial effects on soil rhizosphere microorganisms by promoting metabolism and energy conversion. For example, treating Medicago truncatula with 5 mg/kg of Ag and 50 mg/kg of Zn, and Ti nanoparticles significantly increased the types and total amount of soil rhizosphere microorganisms (Chen et al., 2017). Low dose (10 mg/kg) ZnO nanoparticles promote the proliferation of Cyanobacteria in the rhizosphere of Lactuca sativa L., but 100 mg/kg ZnO has no significant effect on this colony under the same treatment (Xu et al., 2018). Therefore, excessive application of nanoparticles is likely to disrupt the balance of soil ecosystems. In order to fully utilize the benefits of nanoparticles and reduce their potential risks, it is necessary to conduct in-depth research on the interaction mechanism between nanoparticles and microorganisms, and explore reasonable usage methods and dosages. At the same time, it is necessary to strengthen the environmental risk assessment and supervision of nanoparticles to ensure their safe application.
There are also specific effects between microbes and soil enzymes (Philippot et al., 2024). A variety of soil enzymes play a crucial role in biochemical processes, facilitating the breakdown of complex organic matter and the mobilization of nutrients, while also influencing the functionality of certain microorganisms (Wen et al., 2024). In soils rich in organic matter, a greater abundance of microorganisms correlates with heightened enzyme activity, thereby enhancing the interaction between these two components (Donald et al., 2018). The utilization of ZnO nanoparticle to mung bean plants increased the diversity of soil phosphatase and phytase activities and microorganisms, and improved the level of phosphorus acquisition, while also enhancing soil health and nutrient cycle (Raliya et al., 2016). Studies have shown that the absorption of nitrogen, phosphorus and potassium in 200 mg/kg CNPs was significantly increased by 185%, 30.4% and 193%, respectively (Xin et al., 2022). The increase of plant roots was higher than that of branches, and carbon nanoparticles enhanced the activity of most soil enzymes, thus affecting the soil microbial function, thus indirectly regulating plant growth (Xin et al., 2022). These studies enable us to gain deeper insights into the ways in which nanoparticles can enhance plant nutrient absorption. However, the mechanisms underlying the complex interactions among nanoparticles, plant roots, and soil have yet to be fully investigated.
4 Conclusion
The above research results indicate that nanoparticles play an important role in promoting plant nutrient absorption and rhizosphere microbial communities. There is a close connection and synergistic effect between the two, jointly affecting the growth and development of plants, as well as the stability and sustainability of ecosystems. Nanoparticles can promote plant nutrient absorption by loading nutrients and accurately deliver nutrients to different parts of the plant, thereby improving nutrient utilization efficiency. Concurrently, nanoparticles also play a pivotal role in modulating rhizosphere microorganisms. They not only foster plant growth and enhance yield by combating pathogenic microorganisms that are detrimental to plants, but also alter the composition of the rhizosphere microbial community by modifying soil physical and chemical properties and root exudates, ultimately influencing the growth and development of plants.
Nanoparticles enhance plant growth and development by facilitating nutrient uptake, modulating rhizosphere microorganisms, and enhancing soil physiochemical properties, among other benefits, with a focus on their positive effects on plants. Further research into the interactions between roots and microbes in the rhizosphere influenced by nanoparticles will elucidate the mechanisms behind plant phenotypic alterations induced by nanoparticles. This will also shed light on the manipulation of the plant rhizosphere microbiome by nanoparticles, paving the way for more sustainable agricultural practices.
Various types of nanoparticles have played important roles in plant growth, but metal nanoparticles have the widest application prospects in agricultural production. They can serve as pesticide carriers, improve pesticide utilization, and reduce environmental pollution; At the same time, it can also serve as a trace element or plant growth regulator, promoting plant growth and improving yield and quality. In addition, metal nanoparticles have shown great potential in environmental monitoring and biosensing, providing timely and accurate environmental data for agricultural production. Although there are still some challenges in practical applications, such as assessing environmental effects and biosafety, as well as optimizing production costs, with the continuous development and improvement of nanotechnology, the application of metal nanoparticles in agriculture will become more extensive and in-depth, making important contributions to agricultural production and sustainable development.
Nanoparticles have the potential to directly influence plant growth and indirectly affect the surrounding ecological environment, thereby ensuring the sustainable development of agriculture and fostering the green revolution. Moving forward, it is imperative to conduct additional research to uncover the effects of nanoparticles (NPs) on higher plants, particularly crops and vegetables, when applied to soils with varying properties. This should include an exploration of the molecular mechanisms of NPs uptake, transformation, and its impact on growth parameters, as well as the interaction mechanisms between NPs and rhizosphere microbes. Concurrently, greater focus should be placed on the interplay among rhizosphere microbial communities, soil, NPs, and plants. Further studies are necessary to ascertain the beneficial effects of NPs. Moreover, long-term investigations into cereal crops and other key crops are essential to establish correlations between NP dosage, soil type, and ecological impacts. Such research is crucial for reducing reliance on chemical pesticides and fertilizers and for securing the future sustainable development of agriculture.
In summary, this review encapsulates the outcomes of nanoparticles in enhancing plant growth and modulating the rhizosphere microbiome via various mechanisms, offering a foundation for the synergistic integration of future research endeavors in the realms of nanoscience, sustainable agriculture, and environmental science.
Author contributions
HZ: Writing – review & editing, Writing – original draft. TZ: Writing – original draft. YW: Writing – original draft, Visualization, Data curation. TL: Writing – review & editing. QC: Writing – review & editing.
Funding
The author(s) declare that financial support was received for the research, authorship, and/or publication of this article. This research was funded by National Natural Science Foundation of China (32101661); Natural Science Foundation of Henan Province, China (Grant No. 212300410276); Open Project of State Key Laboratory of Cotton Biology (CB2024A35).
Conflict of interest
The authors declare that the research was conducted in the absence of any commercial or financial relationships that could be construed as a potential conflict of interest.
Publisher’s note
All claims expressed in this article are solely those of the authors and do not necessarily represent those of their affiliated organizations, or those of the publisher, the editors and the reviewers. Any product that may be evaluated in this article, or claim that may be made by its manufacturer, is not guaranteed or endorsed by the publisher.
References
Abd Alamer, I. S., Tomah, A. A., Ahmed, T., Li, B., Zhang, J. (2022). Biosynthesis of Silver Chloride Nanoparticles by Rhizospheric Bacteria and Their Antibacterial Activity against Phytopathogenic Bacterium Ralstonia solanacearum. Molecules 27 (1), 224. doi: 10.3390/molecules27010224
Abdelkhalek, A., Yassin, Y., Abdel-Megeed, A., Abd-Elsalam, K.A., Moawad, H., Behiry, S.I. (2023). Rhizobium leguminosarum bv. viciae-Mediated Silver Nanoparticles for Controlling Bean Yellow Mosaic Virus (BYMV) Infection in Faba Bean Plants. Plants 12 (1), 45. doi: 10.3390/plants12010045
Abeywardana, L., de Silva, M., Sandaruwan, C., Dahanayake, D., Priyadarshana, G., Chathurika, S., et al. (2021). Zinc-doped hydroxyapatite–urea nanoseed coating as an efficient macro–micro plant nutrient delivery agent. ACS Agric. Sci. Technol. 1, 230–239. doi: 10.1021/acsagscitech.1c00033
Ahmad, Z., Tahseen, S., Wasi, A., Ganie, I.B., Shahzad, A., Emamverdian, A., et al. (2022). Nanotechnological interventions in agriculture. Nanomaterials 12 (15), 2667. doi: 10.3390/nano12152667
Ahmed, T., Noman, M., Gardea-Torresdey, J. L., White, J. C., Li, B. (2023). Dynamic interplay between nano-enabled agrochemicals and the plant-associated microbiome. Trends Plant Sci. 28, 1310–1325. doi: 10.1016/j.tplants.2023.06.001
Ahmed, T., Noman, M., Jiang, H., Shahid, M., Ma, C., Wu, Z., et al. (2022). Bioengineered chitosan-iron nanocomposite controls bacterial leaf blight disease by modulating plant defense response and nutritional status of rice (Oryza sativa L.). Nano Today 45, 101547. doi: 10.1016/j.nantod.2022.101547
Al-Mamun, M. R., Hasan, M. R., Ahommed, M. S., Bacchu, M. S., Ali, M. R., Khan, M. Z. H. (2021). Nanofertilizers towards sustainable agriculture and environment. Environ. Technol. Innovation 23, 101658. doi: 10.1016/j.eti.2021.101658
Andleeb, A., Andleeb, A., Asghar, S., Zaman, G., Tariq, M., Mehmood, A., et al. (2021). A systematic review of biosynthesized metallic nanoparticles as a promising anti-cancer-strategy. Cancers 13 (11), 2818. doi: 10.3390/cancers13112818
Ben-Moshe, T., Frenk, S., Dror, I., Minz, D., Berkowitz, B. (2013). Effects of metal oxide nanoparticles on soil properties. Chemosphere 90, 640–646. doi: 10.1016/j.chemosphere.2012.09.018
Bhavani, P., Prakash, S. S., Harinikumar, K. M., Thimmegowda, M. N., Benherlal, P. S., Yoganand, S. B. (2020). Performance of slow release hydroxyapatite coated urea nanofertilizer on aerobic paddy. Int. J. Curr. Microbiol. Appl. Sci. 9, 1320–1330. doi: 10.20546/ijcmas.2020.911.155
Bi, B., Wang, K., Zhang, H., Wang, Y., Fei, H., Pan, R., et al. (2021). Plants use rhizosphere metabolites to regulate soil microbial diversity. Land Degrad. Dev. 32, 5267–5280. doi: 10.1002/ldr.v32.18
Bortoletto-Santos, R., Plotegher, F., Majaron, V. F., da Silva, M. G., Polito, W. L., Ribeiro, C. (2020). Polyurethane nanocomposites can increase the release control in granulated fertilizers by controlling nutrient diffusion. Appl. Clay Sci. 199, 105874. doi: 10.1016/j.clay.2020.105874
Cao, X., Yue, L., Wang, C., Luo, X., Zhang, C., Zhao, X., et al. (2022). Foliar application with iron oxide nanomaterials stimulate nitrogen fixation, yield, and nutritional quality of soybean. ACS Nano 16, 1170–1181. doi: 10.1021/acsnano.1c08977
Cervantes-Avilés, P., Huang, X., Keller, A. A. (2021). Dissolution and aggregation of metal oxide nanoparticles in root exudates and soil leachate: implications for nanoagrochemical application. Environ. Sci. Technol. 55, 13443–13451. doi: 10.1021/acs.est.1c00767
Chandrasekaran, M., Paramasivan, M. (2024). Plant growth-promoting bacterial (PGPB) mediated degradation of hazardous pesticides: A review. Int. Biodeterior. Biodegrad. 190, 105769. doi: 10.1016/j.ibiod.2024.105769
Chang, M., Liu, Y., Xu, M., Li, H., Li, S. W. (2024). Particle morphology and soil properties affect the retention of copper oxide nanoparticles in agricultural soils. Environ. Geochem. Health 46, 281. doi: 10.1007/s10653-024-02057-5
Chaparro, J. M., Badri, D. V., Vivanco, J. M. (2014). Rhizosphere microbiome assemblage is affected by plant development. ISME J. 8, 790–803. doi: 10.1038/ismej.2013.196
Chauhan, P., Sharma, N., Tapwal, A., Kumar, A., Verma, G. S., Meena, M., et al. (2023). Soil microbiome: diversity, benefits and interactions with plants. Sustainability 15 (19), 14643. doi: 10.3390/su151914643
Chen, C., Tsyusko, O. V., McNear, D. H., Judy, J., Lewis, R. W., Unrine, J. M.. (2017a). Effects of biosolids from a wastewater treatment plant receiving manufactured nanomaterials on Medicago truncatula and associated soil microbial communities at low nanomaterial concentrations. Sci. Total Environ. 609, 799–806. doi: 10.1016/j.scitotenv.2017.07.188
Chen, J.-n., Wu, L.-t., Song, K., Zhu, Y.-S., Ding, W. (2022a). Nonphytotoxic copper oxide nanoparticles are powerful “nanoweapons” that trigger resistance in tobacco against the soil-borne fungal pathogen Phytophthora nicotianae. J. Integr. Agric. 21, 3245–3262. doi: 10.1016/j.jia.2022.08.086
Chen, S., Guo, X., Zhang, B., Nie, D., Rao, W., Zhang, D., et al. (2023). Mesoporous silica nanoparticles induce intracellular peroxidation damage of phytophthora infestans: A new type of green fungicide for late blight control. Environ. Sci. Technol. 57, 3980–3989. doi: 10.1021/acs.est.2c07182
Chen, X., Duan, M., Zhou, B., Cui, L. (2022b). Effects of biochar nanoparticles as a soil amendment on the structure and hydraulic characteristics of a sandy loam soil. Soil Use Manage. 38, 836–849. doi: 10.1111/sum.12740
Chen, Y.-T., Wang, Y., Yeh, K.-C. (2017). Role of root exudates in metal acquisition and tolerance. Curr. Opin. Plant Biol. 39, 66–72. doi: 10.1016/j.pbi.2017.06.004
Chhipa, H. (2017). Nanofertilizers and nanopesticides for agriculture. Environ. Chem. Lett. 15, 15–22. doi: 10.1007/s10311-016-0600-4
Christian, O. D., Upendra, S., Prem, S. B., Wade, H. E., Jorge, L. G.-T., Jason, C. W. (2019). Zinc oxide nanoparticles alleviate drought-induced alterations in sorghum performance, nutrient acquisition, and grain fortification. Sci. Total Environ. 688, 926–934. doi: 10.1016/j.scitotenv.2019.06.392
Cornelis, G., Hund-Rinke, K., Kuhlbusch, T., van den Brink, N., Nickel, C. (2014). Fate and bioavailability of engineered nanoparticles in soils: A review. Crit. Rev. Environ. Sci. Technol. 44, 2720–2764. doi: 10.1080/10643389.2013.829767
Das, P., Penton, C. R., Westerhoff, P., Perreault, F. (2023). Prospects of 2D graphene nanomaterials in plant-based agriculture and their fate in terrestrial soil: a critical review. Environ. Sci.: Nano 10, 2936–2956. doi: 10.1039/d3en00511a
De Chiffre, L., Kunzmann, H., Peggs, G. N., Lucca, D. A. (2003). Surfaces in precision engineering, microengineering and nanotechnology. CIRP Ann. - Manufact. Technol. 52, 561–577. doi: 10.1016/S0007-8506(07)60204-2
de Silva, M., Siriwardena, D. P., Sandaruwan, C., Priyadarshana, G., Karunaratne, V., Kottegoda, N. (2020). Urea-silica nanohybrids with potential applications for slow and precise release of nitrogen. Mater. Lett. 272, 127839. doi: 10.1016/j.matlet.2020.127839
Dimkpa, C. O., Latta, D. E., McLean, J. E., Britt, D. W., Boyanov, M. I., Anderson, A. J. (2013). Fate of cuO and znO nano- and microparticles in the plant environment. Environ. Sci. Technol. 47, 4734–4742. doi: 10.1021/es304736y
Ding, L.-J., Cui, H.-L., Nie, S.-A., Long, X.-E., Duan, G.-L., Zhu, Y.-G. (2019a). Microbiomes inhabiting rice roots and rhizosphere. FEMS Microbiol. Ecol. 95, fiz040. doi: 10.1093/femsec/fiz040
Ding, Z., Tang, M., Chen, X., Yin, L., Gui, H., Zhu, B. (2019b). Measuring rhizosphere effects of two tree species in a temperate forest: A comprehensive method comparison. Rhizosphere 10, 100153. doi: 10.1016/j.rhisph.2019.100153
Donald, J., Weir, I., Bonnett, S., Maxfield, P., Ellwood, M. D. F. (2018). The relative importance of invertebrate and microbial decomposition in a rainforest restoration project. Restor. Ecol. 26, 220–226. doi: 10.1111/rec.2018.26.issue-2
dos Santos, O. A. L., Pizzorno Backx, B., Abumousa, R. A., Bououdina, M. (2022). Environmental implications associated with the development of nanotechnology: from synthesis to disposal. Nanomaterials 12, 4319. doi: 10.3390/nano12234319
Dutta, S., Pal, S., Panwar, P., Sharma, R. K., Bhutia, P. L. (2022). Biopolymeric nanocarriers for nutrient delivery and crop biofortification. ACS Omega 7, 25909–25920. doi: 10.1021/acsomega.2c02494
Edwards, J., Johnson, C., Santos-Medellín, C., Lurie, E., Podishetty, N. K., Bhatnagar, S., et al. (2015). Structure, variation, and assembly of the root-associated microbiomes of rice. Proc. Natl. Acad. Sci. 112, E911–E920. doi: 10.1073/pnas.1414592112
Elhaj Baddar, Z., Unrine, J. M. (2018). Functionalized-znO-nanoparticle seed treatments to enhance growth and zn content of wheat (Triticum aestivum) seedlings. J. Agric. Food Chem. 66, 12166–12178. doi: 10.1021/acs.jafc.8b03277
Elsharkawy, M., Derbalah, A., Hamza, A., El-Shaer, A. (2020). Zinc oxide nanostructures as a control strategy of bacterial speck of tomato caused by Pseudomonas syringae in Egypt. Environ. Sci. pollut. Res. 27, 19049–19057. doi: 10.1007/s11356-018-3806-0
Fincheira, P., Tortella, G., Seabra, A. B., Quiroz, A., Diez, M. C., Rubilar, O. (2021). Nanotechnology advances for sustainable agriculture: current knowledge and prospects in plant growth modulation and nutrition. Planta 254, 66. doi: 10.1007/s00425-021-03714-0
Francioli, D., Cid, G., Kanukollu, S., Ulrich, A., Hajirezaei, M.-R., Kolb, S. (2021). Flooding causes dramatic compositional shifts and depletion of putative beneficial bacteria on the spring wheat microbiota. Front. Microbiol. 12. doi: 10.3389/fmicb.2021.773116
Francis, D. V., Abdalla, A. K., Mahakham, W., Sarmah, A. K., Ahmed, Z. F. R. (2024). Interaction of plants and metal nanoparticles: Exploring its molecular mechanisms for sustainable agriculture and crop improvement. Environ. Int. 190, 108859. doi: 10.1016/j.envint.2024.108859
Gao, X., Rodrigues, S. M., Spielman-Sun, E., Lopes, S., Rodrigues, S., Zhang, Y., et al. (2019). Effect of soil organic matter, soil pH, and moisture content on solubility and dissolution rate of cuO NPs in soil. Environ. Sci. Technol. 53, 4959–4967. doi: 10.1021/acs.est.8b07243
Gao, Q., Wang, Z., Rao, Y., Zhao, Y., Cao, J., Ho, K. -F., et al. (2023). Oxygen vacancy mediated α-MoO3 bactericidal nanocatalyst in the dark: Surface structure dependent superoxide generation and antibacterial mechanisms. J. Hazard. Mater. 443, 130275. doi: 10.1016/j.jhazmat.2022.130275
Garg, J., Chiu, M. N., Krishnan, S., Kumar, R., Rifah, M., Ahlawat, P., et al. (2024). Emerging trends in zinc ferrite nanoparticles for biomedical and environmental applications. Appl. Biochem. Biotechnol. 196, 1008–1043. doi: 10.1007/s12010-023-04570-2
Ghormade, V., Deshpande, M. V., Paknikar, K. M. (2011). Perspectives for nano-biotechnology enabled protection and nutrition of plants. Biotechnol. Adv. 29, 792–803. doi: 10.1016/j.biotechadv.2011.06.007
Ghosh, S., Yadav, P., Sankaranarayanan, S., Bhatia, D. (2023). Plant-derived nanomaterials for targeted biological applications and smart agriculture. ChemistrySelect 8, e202303495. doi: 10.1002/slct.202303495
Gómez-Sagasti, M. T., Epelde, L., Anza, M., Urra, J., Alkorta, I., Garbisu, C. (2019). The impact of nanoscale zero-valent iron particles on soil microbial communities is soil dependent. J. Hazard. Mater. 364, 591–599. doi: 10.1016/j.jhazmat.2018.10.034
Gordienko, M. G., Palchikova, V. V., Kalenov, S. V., Belov, A. A., Lyasnikova, V. N., Poberezhniy, D. Y., et al. (2019). Antimicrobial activity of silver salt and silver nanoparticles in different forms against microorganisms of different taxonomic groups. J. Hazard. Mater. 378, 120754. doi: 10.1016/j.jhazmat.2019.120754
Guan, X., Gao, X., Avellan, A., Spielman-Sun, E., Xu, J., Laughton, S., et al. (2020). CuO nanoparticles alter the rhizospheric bacterial community and local nitrogen cycling for wheat grown in a calcareous soil. Environ. Sci. Technol. 54, 8699–8709. doi: 10.1021/acs.est.0c00036
Haider, G., Koyro, H. W., Azam, F., Steffens, D., Müller, C., Kammann, C. (2015). Biochar but not humic acid product amendment affected maize yields via improving plant-soil moisture relations. Plant Soil 395, 141–157. doi: 10.1007/s11104-014-2294-3
Haroon, M., Zaidi, A., Ahmed, B., Rizvi, A., Khan, M. S., Musarrat, J. (2019). Effective inhibition of phytopathogenic microbes by eco-friendly leaf extract mediated silver nanoparticles (AgNPs). Indian J. Microbiol. 59, 273–287. doi: 10.1007/s12088-019-00801-5
Hortin, J. M., Anderson, A. J., Britt, D. W., Jacobson, A. R., McLean, J. E. (2020). Copper oxide nanoparticle dissolution at alkaline pH is controlled by dissolved organic matter: influence of soil-derived organic matter, wheat, bacteria, and nanoparticle coating. Environ. Sci.: Nano 7, 2618–2631. doi: 10.1039/d0en00574f
Hu, L., Robert, C. A. M., Cadot, S., Zhang, X., Ye, M., Li, B., et al. (2018). Root exudate metabolites drive plant-soil feedbacks on growth and defense by shaping the rhizosphere microbiota. Nat. Commun. 9, 2738. doi: 10.1038/s41467-018-05122-7
Hwangbo, K., Um, Y., Kim, K. Y., Madhaiyan, M., Sa, T. M., Lee, Y. (2016). Complete genome sequence of bacillus velezensis CBMB205, a phosphate-solubilizing bacterium isolated from the rhizoplane of rice in the Republic of Korea. Genome Announc. 4, e00654-16. doi: 10.1128/genomeA.00654-16
Jaithon, T., Ruangtong, J., T-Thienprasert, J., T-Thienprasert, N. P. (2022). Effects of waste-derived znO nanoparticles against growth of plant pathogenic bacteria and epidermoid carcinoma cells. Crystals 12 (6), 779. doi: 10.3390/cryst12060779
Jiao, L., Cao, X., Wang, C., Chen, F., Zou, H., Yue, L., et al. (2023). Crosstalk between in situ root exudates and rhizobacteria to promote rice growth by selenium nanomaterials. Sci. Total Environ. 878, 163175. doi: 10.1016/j.scitotenv.2023.163175
Jiao, L., Yue, L., Le, X., Cao, X., Chen, F., Wang, C., et al. (2024). Dynamic microbial regulation of triiron tetrairon phosphate nanomaterials in the tomato rhizosphere. Environ. Sci.: Nano 11, 1157–1169. doi: 10.1039/D3EN00797A
Kah, M., Tufenkji, N., White, J. C. (2019). Nano-enabled strategies to enhance crop nutrition and protection. Nat. Nanotechnol. 14, 532–540. doi: 10.1038/s41565-019-0439-5
Kalwani, M., Chakdar, H., Srivastava, A., Pabbi, S., Shukla, P. (2022). Effects of nanofertilizers on soil and plant-associated microbial communities: Emerging trends and perspectives. Chemosphere 287, 132107. doi: 10.1016/j.chemosphere.2021.132107
Kang, M., Bai, X., Liu, Y., Weng, Y., Wang, H., Ye, Z. (2024). Driving role of zinc oxide nanoparticles with different sizes and hydrophobicity in metabolic response and eco-corona formation in sprouts (Vigna radiata). Environ. Sci. Technol. 58, 9875–9886. doi: 10.1021/acs.est.4c01731
Karimian, Z., Samiei, L. (2023). ZnO nanoparticles efficiently enhance drought tolerance in Dracocephalum kotschyi through altering physiological, biochemical and elemental contents. Front. Plant Sci. 14. doi: 10.3389/fpls.2023.1063618
Kaye, J. P., McCulley, R. L., Burke, I. C. (2005). Carbon fluxes, nitrogen cycling, and soil microbial communities in adjacent urban, native and agricultural ecosystems. Global Change Biol. 11, 575–587. doi: 10.1111/j.1365-2486.2005.00921.x
Khan, S. T., Adil, S. F., Shaik, M. R., Alkhathlan, H. Z., Khan, M., Khan, M. (2022). Engineered nanomaterials in soil: their impact on soil microbiome and plant health. Plants 11 (1), 109. doi: 10.3390/plants11010109
Khoiri, A. N., Wattanachaisaereekul, S., Jirakkakul, J., Sutheeworapong, S., Kusonmano, K., Cheevadhanarak, S., et al. (2024). Co-transplantation of phyllosphere and rhizosphere microbes promotes microbial colonization and enhances sugarcane growth. Appl. Soil Ecol. 201, 105469. doi: 10.1016/j.apsoil.2024.105469
Kottegoda, N., Sandaruwan, C., Priyadarshana, G., Siriwardhana, A., Rathnayake, U. A., Berugoda Arachchige, D. M., et al. (2017). Urea-hydroxyapatite nanohybrids for slow release of nitrogen. ACS Nano 11, 1214–1221. doi: 10.1021/acsnano.6b07781
Kusiak, M., Oleszczuk, P., Jośko, I. (2022). Cross-examination of engineered nanomaterials in crop production: Application and related implications. J. Hazard. Mater. 424, 127374. doi: 10.1016/j.jhazmat.2021.127374
Li, H., Jiang, Q., Li, R., Zhang, B., Zhang, J., Zhang, Y. (2022). Passivation of lead and cerium in soil facilitated by biochar-supported phosphate-doped ferrihydrite: Mechanisms and microbial community evolution. J. Hazard. Mater. 436, 129090. doi: 10.1016/j.jhazmat.2022.129090
Li, D., Zhou, C., Wu, Y., An, Q., Zhang, J., Fang, Y., et al. (2022). Nanoselenium integrates soil-pepper plant homeostasis by recruiting rhizosphere-beneficial microbiomes and allocating signaling molecule levels under Cd stress. J. Hazard. Mater. 432, 128763. doi: 10.1016/j.jhazmat.2022.128763
Lin, J., Ma, K., Chen, H., Chen, Z., Xing, B. (2022). Influence of different types of nanomaterials on soil enzyme activity: A global meta-analysis. Nano Today 42, 101345. doi: 10.1016/j.nantod.2021.101345
Liu, L., Tsyusko, O. V., Unrine, J. M., Liu, S., Liu, Y., Guo, L., et al. (2023). Pristine and sulfidized zinc oxide nanoparticles promote the release and decomposition of organic carbon in the legume rhizosphere. Environ. Sci. Technol. 57, 8943–8953. doi: 10.1021/acs.est.3c02071
Lopez-Lima, D., Mtz-Enriquez, A. I., Carrión, G., Basurto-Cereceda, S., Pariona, N. (2021). The bifunctional role of copper nanoparticles in tomato: Effective treatment for Fusarium wilt and plant growth promoter. Scientia Hortic. 277, 109810. doi: 10.1016/j.scienta.2020.109810
Lowry, G. V., Avellan, A., Gilbertson, L. M. (2019). Opportunities and challenges for nanotechnology in the agri-tech revolution. Nat. Nanotechnol. 14, 517–522. doi: 10.1038/s41565-019-0461-7
Lv, J., Christie, P., Zhang, S. (2019). Uptake, translocation, and transformation of metal-based nanoparticles in plants: recent advances and methodological challenges. Environ. Sci.: Nano 6, 41–59. doi: 10.1039/C8EN00645H
Madusanka, N., Sandaruwan, C., Kottegoda, N., Sirisena, D., Munaweera, I., De Alwis, A., et al. (2017). Urea–hydroxyapatite-montmorillonite nanohybrid composites as slow release nitrogen compositions. Appl. Clay Sci. 150, 303–308. doi: 10.1016/j.clay.2017.09.039
Magnabosco, P., Masi, A., Shukla, R., Bansal, V., Carletti, P. (2023). Advancing the impact of plant biostimulants to sustainable agriculture through nanotechnologies. Chem. Biol. Technol. Agric. 10, 117. doi: 10.1186/s40538-023-00491-8
Mahajan, P., Dhoke, S. K., Khanna, A. S. (2011). Effect of Nano-ZnO Particle Suspension on Growth of Mung (Vigna radiata) and Gram (Cicer arietinum) Seedlings Using Plant Agar Method. J. Nanotechnol. 2011, 696535. doi: 10.1155/2011/696535
McManus, P., Hortin, J., Anderson, A. J., Jacobson, A. R., Britt, D. W., Stewart, J., et al. (2018). Rhizosphere interactions between copper oxide nanoparticles and wheat root exudates in a sand matrix: Influences on copper bioavailability and uptake. Environ. Toxicol. Chem. 37, 2619–2632. doi: 10.1002/etc.v37.10
Mishra, S., Keswani, C., Abhilash, P. C., Fraceto, L. F., Singh, H. B. (2017). Integrated approach of agri-nanotechnology: challenges and future trends. Front. Plant Sci. 8. doi: 10.3389/fpls.2017.00471
Moreau, D., Bardgett, R. D., Finlay, R. D., Jones, D. L., Philippot, L. (2019). A plant perspective on nitrogen cycling in the rhizosphere. Funct. Ecol. 33, 540–552. doi: 10.1111/fec.2019.33.issue-4
Moretti, S., Marucci, A. (2019). A photovoltaic greenhouse with variable shading for the optimization of agricultural and energy production. Energies 12 (13), 2589. doi: 10.3390/en12132589
Nasser, F., Lynch, I. (2016). Secreted protein eco-corona mediates uptake and impacts of polystyrene nanoparticles on Daphnia magna. J. Proteomics 137, 45–51. doi: 10.1016/j.jprot.2015.09.005
Noman, M., Ahmed, T., White, J. C., Nazir, M. M., Azizullah, Li, D., et al. (2023). Bacillus altitudinis-Stabilized Multifarious Copper Nanoparticles Prevent Bacterial Fruit Blotch in Watermelon (Citrullus lanatus L.): Direct Pathogen Inhibition, In Planta Particles Accumulation, and Host Stomatal Immunity Modulation. Small 19, 2207136. doi: 10.1002/smll.202207136
Ocsoy, I., Paret, M. L., Ocsoy, M. A., Kunwar, S., Chen, T., You, M., et al. (2013). Nanotechnology in Plant Disease Management: DNA-Directed Silver Nanoparticles on Graphene Oxide as an Antibacterial against Xanthomonas perforans. ACS Nano 7, 8972–8980. doi: 10.1021/nn4034794
Parveen, A., Siddiqui, Z. A. (2022). Impact of silicon dioxide nanoparticles on growth, photosynthetic pigments, proline, activities of defense enzymes and some bacterial and fungal pathogens of tomato. Vegetos 35, 83–93. doi: 10.1007/s42535-021-00280-4
Philippot, L., Chenu, C., Kappler, A., Rillig, M. C., Fierer, N. (2024). The interplay between microbial communities and soil properties. Nat. Rev. Microbiol. 22, 226–239. doi: 10.1038/s41579-023-00980-5
Pisárčik, M., Lukáč, M., Jampílek, J., Bilka, F., Bilková, A., Pašková, Ľ., et al. (2021). Silver nanoparticles stabilized with phosphorus-containing heterocyclic surfactants: synthesis, physico-chemical properties, and biological activity determination. Nanomaterials 11 (8), 1883. doi: 10.3390/nano11081883
Przemieniecki, S. W., Ruraż, K., Kosewska, O., Oćwieja, M., Gorczyca, A. (2024). The impact of various forms of silver nanoparticles on the rhizosphere of wheat (Triticum aestivum L.) – Shifts in microbiome structure and predicted microbial metabolic functions. Sci. Total Environ. 914, 169824. doi: 10.1016/j.scitotenv.2023.169824
Qiu, J., Chen, Y., Liu, Z., Wen, H., Jiang, N., Shi, H., et al. (2023). The application of zinc oxide nanoparticles: An effective strategy to protect rice from rice blast and abiotic stresses. Environ. pollut. 331, 121925. doi: 10.1016/j.envpol.2023.121925
Rahimizadeh, K., Zahra, Q., Chen, S., Le, B. T., Ullah, I., Veedu, R. N. (2023). Nanoparticles-assisted aptamer biosensing for the detection of environmental pathogens. Environ. Res. 238, 117123. doi: 10.1016/j.envres.2023.117123
Raliya, R., Tarafdar, J. C., Biswas, P. (2016). Enhancing the mobilization of native phosphorus in the mung bean rhizosphere using znO nanoparticles synthesized by soil fungi. J. Agric. Food Chem. 64, 3111–3118. doi: 10.1021/acs.jafc.5b05224
Ramírez-Rodríguez, G. B., Dal Sasso, G., Carmona, F. J., Miguel-Rojas, C., Pérez-de-Luque, A., Masciocchi, N., et al. (2020). Engineering biomimetic calcium phosphate nanoparticles: A green synthesis of slow-release multinutrient (NPK) nanofertilizers. ACS Appl. Bio Mater. 3, 1344–1353. doi: 10.1021/acsabm.9b00937
Ravichandran, D., Xu, W., Jambhulkar, S., Zhu, Y., Kakarla, M., Bawareth, M., et al. (2021). Intrinsic field-induced nanoparticle assembly in three-dimensional (3D) printing polymeric composites. ACS Appl. Mater. Interf. 13, 52274–52294. doi: 10.1021/acsami.1c12763
Reinhold-Hurek, B., Bünger, W., Burbano, C. S., Sabale, M., Hurek, T. (2015). Roots shaping their microbiome: global hotspots for microbial activity. Annu. Rev. Phytopathol. 53, 403–424. doi: 10.1146/annurev-phyto-082712-102342
Reith, F., Cornelis, G. (2017). Effect of soil properties on gold- and platinum nanoparticle mobility. Chem. Geol. 466, 446–453. doi: 10.1016/j.chemgeo.2017.06.033
Ren, Y., Wang, G., Su, Y., Li, J., Zhang, H., Ma, G., et al. (2024). Effect of CeO2, TiO2 and SiO2 nanoparticles on the growth and quality of model medicinal plant Salvia miltiorrhiza by acting on soil microenvironment. Ecotoxicol. Environ. Saf. 280, 116552. doi: 10.1016/j.ecoenv.2024.116552
Saberi Riseh, R., Vatankhah, M., Hassanisaadi, M., Varma, R. S. (2024). A review of chitosan nanoparticles: Nature’s gift for transforming agriculture through smart and effective delivery mechanisms. Int. J. Biol. Macromol. 260, 129522. doi: 10.1016/j.ijbiomac.2024.129522
Saghaï, A., Wittorf, L., Philippot, L., Hallin, S. (2022). Loss in soil microbial diversity constrains microbiome selection and alters the abundance of N-cycling guilds in barley rhizosphere. Appl. Soil Ecol. 169, 104224. doi: 10.1016/j.apsoil.2021.104224
Sarma, H., Shyam, S., Zhang, M., Guerriero, G. (2024). Nano-biochar interactions with contaminants in the rhizosphere and their implications for plant-soil dynamics. Soil Environ. Health 2, 100095. doi: 10.1016/j.seh.2024.100095
Scandolera, T., Teano, G., Naderpour, M., Geffroy, V., Pflieger, S. (2024). Insights into the effects of elevated atmospheric carbon dioxide on plant-virus interactions: A literature review. Environ. Exp. Bot. 221, 105737. doi: 10.1016/j.envexpbot.2024.105737
Schjønning, P., Thomsen, I. K., Petersen, S. O., Kristensen, K., Christensen, B. T. (2011). Relating soil microbial activity to water content and tillage-induced differences in soil structure. Geoderma 163, 256–264. doi: 10.1016/j.geoderma.2011.04.022
Sellstedt, A., Richau, K., Sellstedt, A., Richau, K. H. (2013). Aspects of nitrogen-fixing Actinobacteria, in particular free-living and symbiotic Frankia. FEMS Microbiol. Lett. 342, 179–186. doi: 10.1111/1574-6968.12116
Servin, A., Elmer, W., Mukherjee, A., De la Torre-Roche, R., Hamdi, H., White, J. C., et al. (2015). A review of the use of engineered nanomaterials to suppress plant disease and enhance crop yield. J. Nanopart. Res. 17, 92. doi: 10.1007/s11051-015-2907-7
Sharma, B., Shrivastava, M., Afonso, L. O. B., Soni, U., Cahill, D. M. (2022). Zinc- and magnesium-doped hydroxyapatite nanoparticles modified with urea as smart nitrogen fertilizers. ACS Appl. Nano Mater. 5, 7288–7299. doi: 10.1021/acsanm.2c01192
Shireen Akhter Jahan, Q., Sultana, Z., Ud-Daula, M. A., Shamim, R., Rahman, M. M., et al. (2024). Optimization of green silver nanoparticles as nanofungicides for management of rice bakanae disease. Heliyon 10, e27579. doi: 10.1016/j.heliyon.2024.e27579
Singh, A., Singh, N. B., Hussain, I., Singh, H., Yadav, V., Singh, S. C. (2016). Green synthesis of nano zinc oxide and evaluation of its impact on germination and metabolic activity of Solanum lycopersicum. J. Biotechnol. 233, 84–94. doi: 10.1016/j.jbiotec.2016.07.010
Singh, A., Upadhyay, P., Rami, E., Singh, S. K. (2024). Nanotechnology interventions for sustainable plant nutrition and biosensing. J. Soil Sci. Plant Nutr. 24, 1775–1798. doi: 10.1007/s42729-024-01772-3
Solanki, P., Bhargava, A., Chhipa, H., Jain, N., Panwar, J. (2015). “Nano-Fertilizers and their Smart Delivery System,” in Nanotechnologies in Food and Agriculture. Eds. Rai, M., Ribeiro, C., Mattoso, L., Duran, N. (Springer International Publishing, Cham), 81–101.
Staley, C., Ferrieri, A. P., Tfaily, M. M., Cui, Y., Chu, R. K., Wang, P., et al. (2017). Diurnal cycling of rhizosphere bacterial communities is associated with shifts in carbon metabolism. Microbiome 5, 65. doi: 10.1186/s40168-017-0287-1
Steinauer, K., Chatzinotas, A., Eisenhauer, N. (2016). Root exudate cocktails: the link between plant diversity and soil microorganisms? Ecol. Evol. 6, 7387–7396. doi: 10.1002/ece3.2016.6.issue-20
Sun, Y., Jiang, Y., Li, Y., Wang, Q., Zhu, G., Yi, T., et al. (2024). Unlocking the potential of nanoscale sulfur in sustainable agriculture. Chem. Sci. 15, 4709–4722. doi: 10.1039/D3SC06122A
Sun, Q., Li, J., Le, T. (2018). Zinc oxide nanoparticle as a novel class of antifungal agents: current advances and future perspectives. J. Agric. Food Chem. 66, 11209–11220. doi: 10.1021/acs.jafc.8b03210
Sun, X.-D., Ma, J.-Y., Feng, L.-J., Duan, J.-L., Xie, X.-M., Zhang, X.-H., et al. (2023). Magnetite nanoparticle coating chemistry regulates root uptake pathways and iron chlorosis in plants. Proc. Natl. Acad. Sci. 120, e2304306120. doi: 10.1073/pnas.2304306120
Taware, A. S., Rathod, P. B., Katariya, A. P., Tagad, C. K., Wagh, P. S., Sonar, J. P., et al. (2024). +Technological advancement in the development of nano fertilizers for sustainable agriculture. J. Soil Sci. Plant Nutr. 24, 1592–1608. doi: 10.1007/s42729-024-01674-4
Thorakkattu, P., Awasti, N., Sajith Babu, K., Khanashyam, A. C., Deliephan, A., Shah, K., et al. (2024). 3D printing: trends and approaches toward achieving long-term sustainability in the food industry. Crit. Rev. Biotechnol., 1–21. doi: 10.1080/07388551.2024.2344577
Tian, L., Shen, J., Sun, G., Wang, B., Ji, R., Zhao, L. (2020). Foliar application of siO2 nanoparticles alters soil metabolite profiles and microbial community composition in the pakchoi (Brassica chinensis L.) rhizosphere grown in contaminated mine soil. Environ. Sci. Technol. 54, 13137–13146. doi: 10.1021/acs.est.0c03767
Tripathi, S., Tiwari, K., Mahra, S., Victoria, J., Rana, S., Tripathi, D. K., et al. (2024). Nanoparticles and root traits: mineral nutrition, stress tolerance and interaction with rhizosphere microbiota. Planta 260, 34. doi: 10.1007/s00425-024-04409-y
Trivedi, P., Leach, J. E., Tringe, S. G., Sa, T., Singh, B. K. (2020). Plant–microbiome interactions: from community assembly to plant health. Nat. Rev. Microbiol. 18, 607–621. doi: 10.1038/s41579-020-0412-1
Wang, Q., Liao, Y., Zhao, W., Yi, T., Jiang, Y., Zhu, G., et al. (2024). Potassium-based nanomaterials significantly enhance nutrient utilization efficiency and promote high crop yields. Environ. Sci.: Nano. 11 (7), 2906–2922. doi: 10.1039/d4en00148f
Wang, D., Saleh, N. B., Byro, A., Zepp, R., Sahle-Demessie, E., Luxton, T. P., et al. (2022b). Nano-enabled pesticides for sustainable agriculture and global food security. Nat. Nanotechnol. 17, 347–360. doi: 10.1038/s41565-022-01082-8
Wang, C., Yue, L., Cheng, B., Chen, F., Zhao, X., Wang, Z., et al. (2022a). Mechanisms of growth-promotion and Se-enrichment in Brassica chinensis L. by selenium nanomaterials: beneficial rhizosphere microorganisms, nutrient availability, and photosynthesis. Environ. Sci.: Nano 9, 302–312. doi: 10.1038/s41565-022-01082-8
Wang, F., Zhang, X., Zhang, S., Zhang, S., Sun, Y. (2020a). Interactions of microplastics and cadmium on plant growth and arbuscular mycorrhizal fungal communities in an agricultural soil. Chemosphere 254, 126791. doi: 10.1016/j.chemosphere.2020.126791
Wei, M., Zhang, M., Huang, G., Yuan, Y., Fu, C., Yu, L. (2020). Coculture with two Bacillus velezensis strains enhances the growth of Anoectochilus plants via promoting nutrient assimilation and regulating rhizosphere microbial community. Ind. Crops Products 154, 112697. doi: 10.1016/j.indcrop.2020.112697
Wen, L., Peng, Y., Lin, Y., Zhou, Y., Cai, G., Li, B., et al. (2024). Conservation tillage increases nutrient accumulation by promoting soil enzyme activity: a meta-analysis. Plant Soil., 1–16. doi: 10.1007/s11104-024-06817-1
Wen, H., Shi, H., Jiang, N., Qiu, J., Lin, F., Kou, Y. (2023). Antifungal mechanisms of silver nanoparticles on mycotoxin producing rice false smut fungus. iScience 26, 105763. doi: 10.1016/j.isci.2022.105763
Wheeler, K. E., Chetwynd, A. J., Fahy, K. M., Hong, B. S., Tochihuitl, J. A., Foster, L. A., et al. (2021). Environmental dimensions of the protein corona. Nat. Nanotechnol. 16, 617–629. doi: 10.1038/s41565-021-00924-1
Wu, K., Li, W., Ma, F., Gan, F., Zhou, J., Zeng, H., et al. (2024). Urea-loaded core–shell MOF/silica nanocarriers for continuous nitrogen release to crops. ACS Appl. Nano Mater. 7, 11645–11654. doi: 10.1021/acsanm.4c01187
Wu, L. X., Wang, Y., Lyu, H., Chen, X. D. (2023). Effects of a compoundTrichodermaagent onCoptis chinensisgrowth, nutrients, enzyme activity, and microbial community of rhizosphere soil. PeerJ 11, e15652. doi: 10.7717/peerj.15652
Xin, X., Nepal, J., Wright, A. L., Yang, X., He, Z. (2022). Carbon nanoparticles improve corn (Zea mays L.) growth and soil quality: Comparison of foliar spray and soil drench application. J. Clean. Product. 363, 132630. doi: 10.1016/j.jclepro.2022.132630
Xiong, L., Wang, P., Hunter, M. N., Kopittke, P. M. (2018). Bioavailability and movement of hydroxyapatite nanoparticles (HA-NPs) applied as a phosphorus fertiliser in soils. Environ. Sci.: Nano 5, 2888–2898. doi: 10.1039/C8EN00751A
Xu, Y., Chen, Z., Li, X., Tan, J., Liu, F., Wu, J. (2023b). The mechanism of promoting rhizosphere nutrient turnover for arbuscular mycorrhizal fungi attributes to recruited functional bacterial assembly. Mol. Ecol. 32, 2335–2350. doi: 10.1111/mec.16880
Xu, X., Liang, A., Li, H., Shang, H., Qian, K., Jia, W., et al. (2023a). Foliar applied znO quantum dots boost pumpkin (Cucurbita moschata duch.) growth and positively alter endophytic and rhizosphere microbial communities. ACS Sustain. Chem. Eng. 11, 8503–8516. doi: 10.1021/acssuschemeng.3c00954
Xu, J., Luo, X., Wang, Y., Feng, Y. (2018). Evaluation of zinc oxide nanoparticles on lettuce (Lactuca sativa L.) growth and soil bacterial community. Environ. Sci. pollut. Res. 25, 6026–6035. doi: 10.1007/s11356-017-0953-7
Yan, X., Pan, Z., Chen, S., Shi, N., Bai, T., Dong, L., et al. (2022). Rice exposure to silver nanoparticles in a life cycle study: effect of dose responses on grain metabolomic profile, yield, and soil bacteria. Environ. Sci.: Nano 9, 2195–2206. doi: 10.1039/d2en00211f
Yang, J., Wang, M., Li, W., He, X., Teng, W., Ma, W., et al. (2019). Reducing expression of a nitrate-responsive bZIP transcription factor increases grain yield and N use in wheat. Plant Biotechnol. J. 17, 1823–1833. doi: 10.1111/pbi.13103
Yang, H., Zhang, X., Dang, D., Zheng, L., Yang, Q., Yu, Y. (2021). Effects of iron oxide nanoparticles on CH4 and N2O emissions and microbial communities in two typical paddy soils. Chin. J. Appl. Environ. Biol. 27, 725–733. doi: 10.19675/j.cnki.1006-687x.2020.07031
You, Y., Kerner, P., Shanmugam, S., Khodakovskaya, M. V. (2023). Emerging investigator series: differential effects of carbon nanotubes and graphene on the tomato rhizosphere microbiome. Environ. Sci.: Nano 10, 1570–1584. doi: 10.1039/d2en01026g
Yousef, H. A., Fahmy, H. M., Arafa, F. N., Abd Allah, M. Y., Tawfik, Y. M., El Halwany, K. K., et al. (2023). Nanotechnology in pest management: advantages, applications, and challenges. Int. J. Trop. Insect Sci. 43, 1387–1399. doi: 10.1007/s42690-023-01053-z
Yu, L., Tang, Y., Wang, Z., Gou, Y., Wang, J. (2019). Nitrogen-cycling genes and rhizosphere microbial community with reduced nitrogen application in maize/soybean strip intercropping. Nutrient Cycling Agroecosys. 113, 35–49. doi: 10.1007/s10705-018-9960-4
Yue, L., Wang, J., Cao, X., Wang, C., Ma, C., Chen, F., et al. (2023). Silica nanomaterials promote rice tillering and yield by regulating rhizosphere processes, nitrogen uptake, and hormone pathways. ACS Sustain. Chem. Eng. 11, 16650–16660. doi: 10.1021/acssuschemeng.3c05419
Zhang, P., Jiang, Y., Schwab, F., Monikh, F. A., Grillo, R., White, J. C., et al. (2024c). Strategies for enhancing plant immunity and resilience using nanomaterials for sustainable agriculture. Environ. Sci. Technol. 58, 9051–9060. doi: 10.1021/acs.est.4c03522
Zhang, K., Rengel, Z., Zhang, F., White, P. J., Shen, J. (2023). Rhizosphere engineering for sustainable crop production: entropy-based insights. Trends Plant Sci. 28, 390–398. doi: 10.1016/j.tplants.2022.11.008
Zhang, L., Yang, Y., Zhu, Y., Hu, H., Jia, Q., Sun, C., et al. (2024a). Antifungal activity and mechanism of chitosan against Fusarium solani caused ginger soft rot during postharvest storage. Postharvest Biol. Technol. 208, 112680. doi: 10.1016/j.postharvbio.2023.112680
Zhang, M.-X., Zhao, L.-Y., He, Y.-Y., Hu, J.-P., Hu, G.-W., Zhu, Y., et al. (2024b). Potential roles of iron nanomaterials in enhancing growth and nitrogen fixation and modulating rhizomicrobiome in alfalfa (Medicago sativa L.). Biores. Technol. 391, 129987. doi: 10.1016/j.biortech.2023.129987
Zhao, H., Song, J., Zhao, G., Xiang, Y., Liu, Y. (2019). Novel semi-IPN nanocomposites with functions of both nutrient slow-release and water retention. 2. Effects on soil fertility and tomato quality. J. Agric. Food Chem. 67, 7598–7608. doi: 10.1021/acs.jafc.9b00889
Zhao, F., Xin, X., Cao, Y., Su, D., Ji, P., Zhu, Z., et al. (2021). Use of carbon nanoparticles to improve soil fertility, crop growth and nutrient uptake by corn (Zea mays L.). Nanomaterials 11 (10), 2717. doi: 10.3390/nano11102717
Zhou, Y., Coventry, D. R., Gupta, V. V. S. R., Fuentes, D., Merchant, A., Kaiser, B. N., et al. (2020). The preceding root system drives the composition and function of the rhizosphere microbiome. Genome Biol. 21, 89. doi: 10.1186/s13059-020-01999-0
Zhu, Y., Cao, Y., Fu, B., Wang, C., Shu, S., Zhu, P., et al. (2024). Waste milk humification product can be used as a slow release nano-fertilizer. Nat. Commun. 15, 128. doi: 10.1038/s41467-023-44422-5
Keywords: nanoparticles, plants, nutrient uptake, soil, rhizosphere microbial communities
Citation: Zhang H, Zheng T, Wang Y, Li T and Chi Q (2024) Multifaceted impacts of nanoparticles on plant nutrient absorption and soil microbial communities. Front. Plant Sci. 15:1497006. doi: 10.3389/fpls.2024.1497006
Received: 16 September 2024; Accepted: 22 October 2024;
Published: 13 November 2024.
Edited by:
Libin Zhou, Chinese Academy of Sciences (CAS), ChinaReviewed by:
Yin Li, Huazhong University of Science and Technology, ChinaHaixia Xu, Henan Agricultural University, China
Copyright © 2024 Zhang, Zheng, Wang, Li and Chi. This is an open-access article distributed under the terms of the Creative Commons Attribution License (CC BY). The use, distribution or reproduction in other forums is permitted, provided the original author(s) and the copyright owner(s) are credited and that the original publication in this journal is cited, in accordance with accepted academic practice. No use, distribution or reproduction is permitted which does not comply with these terms.
*Correspondence: Qing Chi, cWNoaUB6enUuZWR1LmNu