- 1Centro de Investigaciones Biotecnológicas del Ecuador, ESPOL, Polytechnic University, ESPOL, Guayaquil, Ecuador
- 2Herbario GUAY, Departamento de Botánica, Facultad de Ciencias Naturales, Universidad de Guayaquil, Guayaquil, Ecuador
- 3Instituto de Farmacia, Facultad de Ciencias, Universidad Austral de Chile, Valdivia, Chile
Wigandia ecuadoriensis, a member of the Namaceae family, is a source of metabolites and has been traditionally used as an anti-inflammatory. This work aimed to determine the total phenolic content (TPC), total flavonoid content (TFC), antioxidant effect, inhibition of α-glucosidase and cholinesterase enzymes (AChE, BChE), and antibacterial activity of the methanolic extract (ME) and subfractions of Wigandia ecuadoriensis. The findings revealed that ME and its subfractions exhibited significant antioxidant capacity, with the ethyl acetate fraction being the most active, displaying an IC50 of 17.66 µg/mL against the 1,1-diphenyl-2-picrylhydrazyl (DPPH) radical and 10.31 µg/mL against 2,2′-azinobis-(3-ethylbenzothiazoline-6-sulfonic acid) diammonium salt (ABTS). This activity was attributed to its high total phenolic content (357.47 mg GAE/g). Furthermore, W. ecuadoriensis fractions showed marked antimicrobial properties against human pathogen strains with Minimum Bactericidal Concentration (MBC) values of 1.56–6.25 mg/mL for S. aureus, E. faecalis and E. coli. Furthermore, aqueous fraction exhibited slight inhibition of acetylcholinesterase (IC50: 915.98 µg/mL) and butyrylcholinesterase (IC50: 380.42 µg/mL). Interestingly, EF showed the greatest inhibitory effect of α-glucosidase (IC50: 38.44 µg/mL) which is more potent than the control used, acarbose (IC50: 179.07 µg/mL). UHPLC-QTOF-MS analysis identified forty compounds, including phenolic acids, flavonoids, saponins, terpenes, and fatty acyls. As far as we know, this is the first study to evaluate the chemical composition and biological potential of W. ecuadoriensis. Our results provide the first evidence to the chemical knowledge of the species W. ecuadoriensis and demonstrate its bioactive potential as an interesting source of secondary metabolites with possible beneficial properties for health.
1 Introduction
In recent years, there has been significant interest in using plant extracts. Indeed, it is recognized that plants harbor valuable bioactive compounds that can be beneficial both for promoting human well-being and for the formulation of supplements or nutraceuticals containing these enriching substances.
Antioxidant activity is essential to neutralize free radicals involved in aging and various chronic diseases, including cancer and cardiovascular diseases (Farhat et al., 2013). The study of inhibitors of enzymes such as α-glucosidase and cholinesterase is crucial in the search for valuable treatments for various diseases (Anand and Singh, 2013). Inhibition of α-glucosidase is an effective strategy in the management of type 2 diabetes mellitus, as it reduces blood glucose levels by slowing down carbohydrate digestion (Mustikasari et al., 2024). On the other hand, cholinesterase inhibitors play an important role in the treatment of Alzheimer’s disease, improving the transmission of nerve signals by preventing the breakdown of acetylcholine (Anand and Singh, 2013). Together, these studies provide a solid foundation for the development of therapies that combat metabolic and neurodegenerative diseases.
In this sense, the Ecuadorian flora is known for its amazing diversity, hosting a unique wealth of plant species that arouse the interest of the scientific community. In this context, we focus on Wigandia ecuadoriensis (Figure 1), a new plant species botanically described in 2006 and which has captured the attention of botanists and ecologists due to its colonizing capacity and remarkable tolerance to reduced levels of precipitation, which could be used in the restoration of native vegetation in regions characterized by very dry tropical forests (Cornejo, 2006) and an interesting source of bioactive molecules. Although this species has been recognized locally, its detailed scientific study is still incipient.
Wigandia ecuadorensis is a shrub or small tree, up to 4 m tall, with large, shovel-shaped leaves and a terminal, branched inflorescence with pink flowers. It belongs to the Namaceae family and is endemic to the subtropical and tropical regions of the Ecuadorian coast (Cornejo, 2006; Vasile et al., 2020). In Ecuador, the Kichwa people used the leaves of the Wigandia Kunth genus as an anti-inflammatory (Torre et al., 2008). Other traditional uses of the Wigandia urens species are abortifacient, infections, epilepsy, psychological, skin, and immunological problems (Hitziger, 2016). The anti-inflammatory activity of different extracts of Wigandia urens has also been reported (Zavala-Sánchez et al., 2009). Some flavonoids and phacelioids have been isolated in the genus Wigandia (Gómez et al., 1980; Reynolds et al., 1989; Cao et al., 2003).
So far, there are no scientific studies in the literature on the plant species W. ecuadorensis, therefore, it represents an intriguing opportunity to discover new medicinal properties, unique chemical compounds, and possibly significant contributions to chemotaxonomy and natural medicine. This work aims to measure the content of phytochemicals (phenols and flavonoids) and evaluate the antioxidant, antibacterial, and enzymatic inhibition properties on cholinesterases and α-glucosidase of the methanolic extract and its subfractions from the leaves of W. ecuadorensis; and to analyze the metabolomic chemical profile by UHPLC-QTOF-MS of the fractions that presented the highest activity.
2 Materials and methods
2.1 Plant collection
The plant species W. ecuadoriensis was collected in Guayaquil, Guayas province, Ecuador (2°08’42.7”S 79°56’49.6”W) in October 2023. The species was identified by biologist Xavier Cornejo from the Faculty of Natural Sciences of the University of Guayaquil. A reference specimen (CIBE057) was deposited in the Herbarium of the Centro de Investigaciones Biotecnológicas del Ecuador, Guayaquil-Ecuador.
2.2 Extraction procedure and liquid–liquid fractionation
10 g of dried and powdered leaves of W. ecuadoriensis were macerated with methanol (three times with 100 mL each) under constant shaking using an orbital shaker (150 rpm) at 25°C for 24 h. Each extract was filtered through Whatman No. 1 filter paper and the solutions were concentrated under reduced pressure at 40°C to obtain the methanolic extract. Then, 2.9 g of the methanolic extract was suspended in 50 mL of a methanol-water mixture (1:3) and partitioned with n-hexane (HF), dichloromethane (DMF), ethyl acetate (EF), and a residual aqueous fraction (AqF). The extracts and subfractions were stored at -20°C until required for analysis.
2.3 Total phenolic content
Total phenolic content was determined according to the Folin-Ciocalteu (FC) assay (Ghareeb et al., 2017). 20 µL of the sample was mixed with 100 µL FC reagent (1:10 v/v) and 80 µL of a Na2CO3 solution (7.5%), incubated for 60 minutes at room temperature, and the absorbance of the resulting blue solution was measured at 760 nm in a microplate reader (Biotek Synergy HTX, Vermont, USA). The results of the total phenol content are expressed in mg of gallic acid equivalent per gram of dry extract (mg GAE/g DE). All measurements were carried out in triplicate.
2.4 Total flavonoid content
Total flavonoids were determined using the aluminum chloride method (Woster, 2003). 100 µL of sample was mixed with 100 µL of 2% AlCl3 solution in ethanol. After 60 minutes at room temperature, absorbance at 420 nm was measured using a microplate reader (Biotek Synergy HTX, Vermont, USA). The results of the flavonoid content expressed in mg of quercetin equivalent per gram of dry extract (mg QE/g DE).
2.5 Antioxidant assays
2.5.1 DPPH radical scavenging assay
The antioxidant activity of extracts and subfractions was determined by the procedure described by (Thaipong et al., 2006). 50 µL of the sample was mixed with 150 µL of a DPPH solution (0.15 mM) dissolved in methanol in the dark for 30 minutes. Subsequently, the absorbance was measured at 517 nm in a microplate reader (Biotek Synergy HTX, Vermont, USA). A calibration curve with Trolox was used and the results were expressed in mg equivalent to Trolox/g DE.
2.5.2 ABTS
The antioxidant capacity was measured through the iron reduction method described by (van den Berg et al., 1999). The radical ABTS stock solution is diluted to a final concentration of 156 µM to obtain a final absorbance of 0.70 ± 0.02 at 732 nm. The radical discoloration was initiated by adding 50 µL of the sample solution with 150 µL of the ABTS solution. After 30 minutes of incubation in the dark, the absorbance was measured at 732 nm using a microplate reader (Biotek Synergy HTX, Vermont, USA). The calibration curve was constructed with Trolox, and the results were expressed in mg equivalent to Trolox/g DE.
2.5.3 Ferric-reducing antioxidant potential assay
The ferric reducing antioxidant power assay (FRAP) was determined according to the procedure described by (Ghareeb et al., 2017). In a 96-well microplate, 20 µl of each extract was mixed with 180 µl of FRAP reagent. The mixture remained for 30 minutes in the dark and the absorbance at 595 nm was measured in a microplate reader (BioTek Instrument, Inc., Winooski, VT, EE. UU.). The calibration curve was constructed with ferrous sulfate heptahydrate (FeSO4.7H2O), and the results were expressed in mmol Fe/g DE.
2.6 Cholinesterase inhibition
Acetylcholinesterase (AChE) and butyrylcholinesterase (BChE) inhibitions were performed in vitro according to the method of (Barrientos et al., 2023). The enzymes were dissolved in Tris-HCl buffer (50 mM, pH 8.0) and 5-dithiol-bis(2-nitrobenzoic) acid (DTNB) was prepared in buffer. W. ecuadoriensis fractions were prepared at a concentration of 20 mg per milliliter in buffer. 25 µL of the sample was mixed with 125 µL of DTNB and 25 µL of the enzyme (AChE 0.3 U/mL), incubated for 15 min at 37°C, then the substrates acetyl thiocholine iodide (15 mM) and butyryl thiocholine chloride (15 mM) were added, as appropriate, and the absorbance was measured at 412 nm at 37°C in a microplate reader (BioTek Instrument, Inc., Winooski, VT, USA). The results were expressed as IC50 values (μg/mL). Galantamine was used as a positive control.
2.7 α-glucosidase inhibition assay
To determine the inhibitory activity of α-glucosidase, 600 μL of phosphate buffer (100 mM pH 6.9) was added with 250 μL of p-nitrophenyl-α-d-glucopyranoside (5 mM), 100 μL of sample (EF/AqF from W. ecuadoriensis) and incubated at 37°C for 5 min. After that, 50 μL of 0.5 U/mL α-glucosidase enzyme solution was added to start the reaction. After 15 min at 37°C, 1000 μL of Na2CO3 (200 mM) was added. The absorbance was measured at 400 nm (Biotek Synergy HTX, Vermont, USA). The results were expressed as IC50 values (μg/mL). Acarbose was used as a positive control (Coral Caycho et al., 2020).
2.8 Determination of the minimum bactericidal concentration
A widely accepted sensitive serial dilution microplate method was used to determine the minimum inhibitory concentration (MBC) (Elisha et al., 2017). Overnight bacterial cultures were adjusted to McFarland standard 1, equivalent to 3.0 x 108 CFU/mL (Staphylococcus aureus, Enterococcus faecalis, Escherichia coli, and Pseudomonas aeruginosa). The dried extract and subfractions were dissolved in 12.5% DMSO at a concentration of 25 mg/mL and 100 µL was added to the first well of a 96-well microtiter plate and serially diluted 1:1 with water. Bacterial cultures (100 µL) were added to each well. Starting with an extract concentration of 25 mg/mL, bacteria were therefore subjected to final concentrations of 6.25 to 0.05 mg/mL. Ampicillin was used as a positive control and DMSO (12.5%) as a solvent control. The highest concentration to which the bacteria were exposed was 12.5% DMSO in the first well and decreased two-fold in each subsequent well. Microplates were incubated overnight at 37°C. Finally, the solutions of the 96-well plates were subcultured in Petri dishes with 25 mL of soy agar. The MBC was defined as the lowest concentration of the extracts at which there was no sign of bacterial growth. The results are reported as mg/mL (Viteri et al., 2021). The antibiotic ampicillin was used as a positive control. For each bacteria and extract analyzed, a positive control (without plant extract) and a blank (without bacteria) were prepared.
2.9 UHPLC-QTOF-MS analysis
The separation and identification of the compounds present in the W. ecuadoriensis fractions was performed on a Compact QTOF MS + Elute UHPLC system, with the software Data Analysis 4.0 (all Bruker Daltonik GmbH, Bremen, Germany). Approximately 5 mg/mL of the fraction was dissolved in methanol and filtered through a 0.2 μm PTFE membrane and 3 µL was injected into the equipment. They were then measured in the chromatographic system consisting of a column temperature of 40°C; flow rate of 0.4 mL/min, mobile phase H2O + 0.1% formic acid (A) and acetonitrile + 0.1% formic acid (B), and elution gradient, 0–0.5 min (12% B), 0.5–11 min (1–99% B), 11–14 min (99% B), and 14–16 min (12% B). Mass spectrometry conditions: electrospray ionization (ESI) source, scanning range 50–1300 m/z, the fragmentation pattern was obtained using the spectral libraries of the MassBank of North America (MoNA), obtained from https://mona.fiehnlab.ucdavis.edu/downloads.
2.10 Statistical analysis
All the assays were performed in triplicate and represented as median ± standard deviation (SD) using Microsoft Excel software (Microsoft 365, Microsoft Corporation, Redmond, WA, USA). Statistical significance between groups was set at p < 0.05 and determined by one-way ANOVA with Tukey’s post hoc test using the commercial software Minitab 19.
3 Results and discussion
3.1 Extraction procedure
The total yield of the subfractions was performed after methanolic extraction and fractionation with n-hexane, dichloromethane, and ethyl acetate, resulting in four subfractions (hexane fraction, dichloromethane fraction, ethyl acetate fraction, and aqueous fraction). The results indicated that the methanolic extract (ME) yielded of 29.0%, followed by the aqueous fraction (AqF) which reached 15.5%. The dichloromethane fraction (DMF) had an intermediate yield of 8.5%, while the hexane fraction (HF) showed a much lower yield of 3.8%. Finally, the ethyl acetate fraction (EF) presented the lowest yield with only 1.0%.
3.2 Total phenolic and flavonoid content
The TPC and TFC of the extract and leaf fractions of W. ecuadoriensis were examined, and the results are presented in Table 1. The phenolic content was highest for the ethyl acetate fraction with 357.47 ± 12.78 mg GAE/g of dry extract. The flavonoid content was highest for the ethyl acetate fraction with 48.93 ± 6.32 mg QE/g of dry extract. Although this is the first report on W. ecuadoriensis, scientific information on the genus Wigandia and family Namaceae is scarce. However, there are some previous studies on other plants of the order Boraginales. The content of phenols and flavonoids in the ethanolic extract of Eriodictyon californicum leaves has been reported (Richards and Chaurasia, 2020). The authors report this species as a promising nutraceutical due to its healing properties against oxidative stress. Our results were superior to those reported for the species Symphytum officinale and Anchusa ochroleuca, with phenolic and flavonoid contents between 5.39-125.50 mg GAE/g of extract and 0.11-36.58 mg QE/g of extract, respectively (Trifan et al., 2021). In other species such as Symphytum anatolicum and Cynoglottis barrelieri the phenolic content was 32.7 and 52.8 mg GAE/g extract, respectively (Varvouni et al., 2020). In another study, it was determined that the phenolic and flavonoid content in the methanol extract of the Onosma ambigens species was lower with values of 51.19 mg GAE/g of extract and 45.39 mg QEs/g of extract, respectively (Sarikurkcu et al., 2020).
3.3 Antioxidant activity
The antioxidant activity of the extract and subfractions was analyzed by DPPH, ABTS, and FRAP methods and are presented in Table 2. These methods are widely used due to their simplicity, sensitivity, and ability to provide comparative antioxidant capacities of various extracts and compounds (Tabart et al., 2009).
The DPPH (2,2-diphenyl-1-picrylhydrazyl) assay measures the ability of antioxidants to scavenge free radicals by monitoring the color change from purple to yellow as the DPPH radical is reduced (Baliyan et al., 2022). The results of this assay ranged from 157.54 ± 0.49 to 187.32 ± 0.56 µmol TE/g of dry extract. W. ecuadoriensis ethyl acetate fraction scavenged DPPH in a concentration-dependent way with an IC50 of 17.66 µg/mL (Figure 2A). The hexane fraction had the highest value (IC50: 97.30 µg/mL). The antioxidant activity (IC50) decreased in descending order: EF > ME > AqF > DMF > HF. According to (Setha et al., 2013) IC50 values < 50 µg/mL are considered potent antioxidants. A study reported that the extract of the Eriodictyon californicum species showed a 93.39% inhibition of DPPH radicals at a concentration of 1.0 mg/mL (Richards and Chaurasia, 2020), similar to those obtained in our study evaluated at the same concentration (ME: 85.97%, EF: 76.12%, DMF: 81.78%, EF: 88.63%, AqF: 89.30%). Likewise, a study reported that the extract of the polar aerial part of S. officinale showed a DPPH radical scavenging activity similar to our study (138.41 µmol TE/g) (Trifan et al., 2021). In Cordia gilletii, an IC50 between 3.2 - 83.5 µg/mL is reported in different extracts (Okusa et al., 2007).
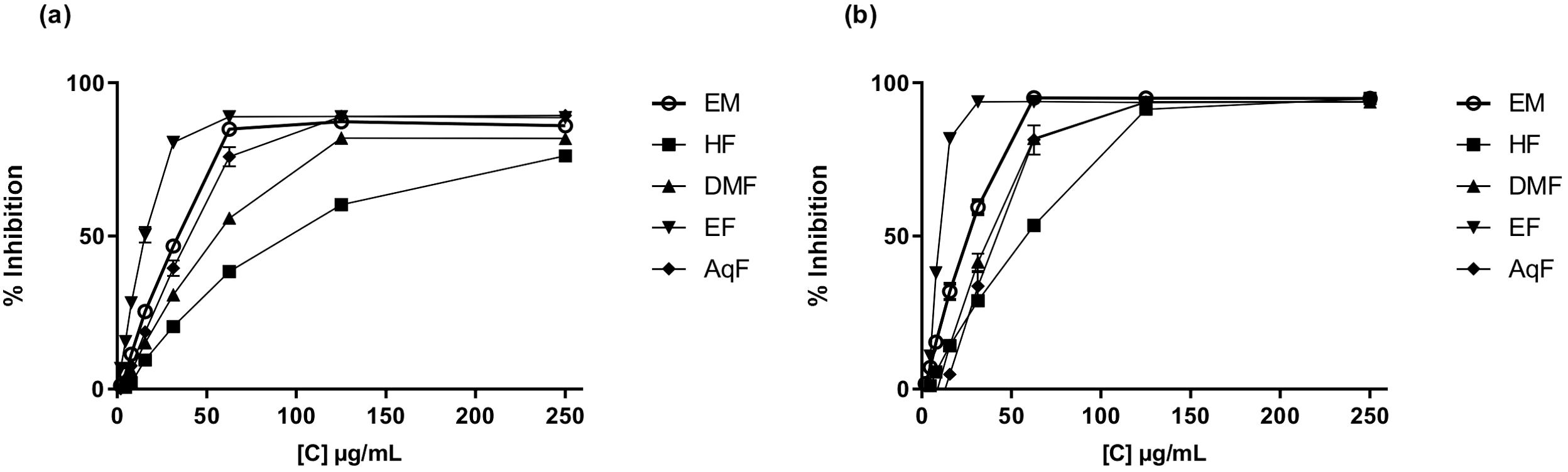
Figure 2. Antioxidant activity of W. ecuadoriensis extract and subfractions against DPPH (A) and ABTS (B). Each point represents the average of three measurements.
The ABTS, 2,2’-azino-bis(3-ethylbenzothiazoline-6-sulfonic acid) assay involves the generation of a blue-green ABTS radical cation, which is reduced by the antioxidants present in the sample, resulting in a decrease in absorbance. Similarly, the antioxidant activity evaluated against the ABTS radical, the extract, and all fractions had similar values in the range of 183.80 ± 0.37 and 184.12 ± 0.00 µmol TE/g extract. W. ecuadoriensis ethyl acetate fraction scavenged DPPH in a concentration-dependent way with an IC50 of 10.31 µg/mL (Figure 2B). The antioxidant activity (IC50) decreased in descending order: EF > ME > DMF > AqF > HF. Likewise, the extract of the polar aerial part of S. officinale showed ABTS radical scavenging activity similar to our study (205.82 µmol TE/g extract) (Trifan et al., 2021). In another work it was observed that the Trolox equivalent antioxidant capacity (TEAC= IC50 Trolox/IC50 of the sample) ratio was 0.013, while in our study it was 0.18, concluding the antioxidant power of the methanolic extract of W. ecuadoriensis (Sarikurkcu et al., 2020).
The FRAP (ferric reducing antioxidant power) assay quantifies the antioxidant effect by evaluating the reduction of the ferric-tripyridyltriazine complex to its ferrous form, which has an intense blue color. FRAP values ranged from 0.65 ± 0.01 to 2.87 ± 0.01 mmol Fe/g of dry extract. The species of the genus Eriodictyon presented antioxidant activity with a concentration-dependent behavior comparable to the ascorbic acid standard (Richards and Chaurasia, 2022).
All these results demonstrate that the species W. ecuadoriensis and especially the ethyl acetate fraction present the highest antioxidant activity evaluated by the DPPH, ABTS, and FRAP methods, probably due to the higher total phenolic and flavonoid content compared to the other fractions tested (Table 1). In fact, the main compounds found in EF and AqF of W. ecuadoriensis, namely caffeic acids, rosmarinic acid, cirsimaritin, luteolin-7-glycoside and apigenin-8-C-(6”acetyl)-β-D-glucopyranoside (Table 3), have already been reported as DPPH and ABTS scavengers. In this sense, these results indicate that the compounds present in the EF and DMF fractions have a strong capacity to neutralize free radicals, suggesting a high bioactive potential. This antioxidant activity highlights the W. ecuadoriensis species as a promising source of natural antioxidants, which may have therapeutic and preventive applications in diseases related to oxidative stress.
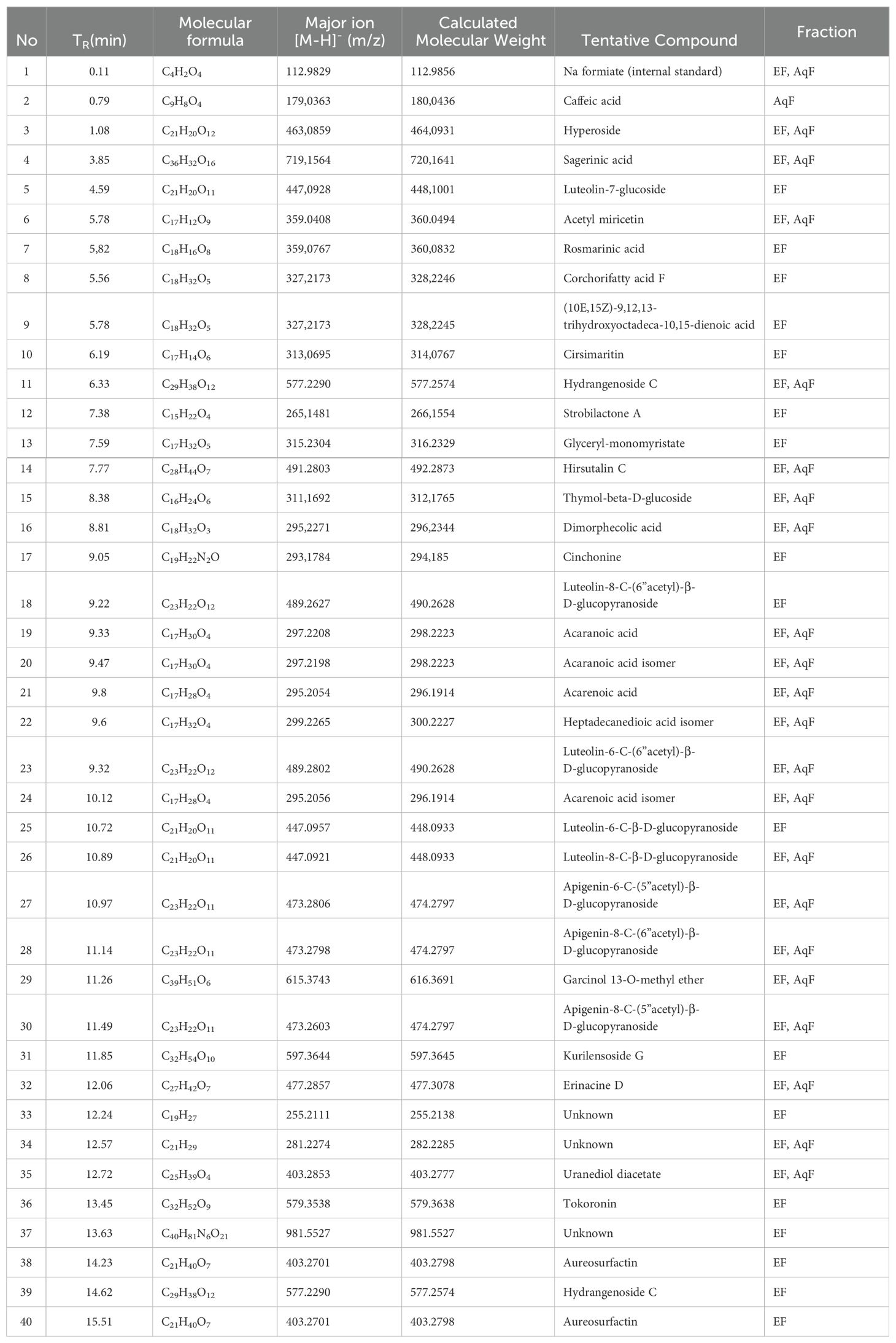
Table 3. UHPLC-QTOF-MS identification of ethyl acetate (a) and aqueous fraction (b) from W. ecuadoriensis leaves.
3.4 Antibacterial activity of W. ecuadoriensis
In the study of the antibacterial activity of the extract and subfractions of leaves of W. ecuadoriensis against Staphylococcus aureus, Enterococcus faecalis, Escherichia coli, and Pseudomonas aeruginosa, it was found that the crude extract and the different fractions presented varied effectiveness (Table 4). The crude extract showed remarkable activity against S. aureus (1.56 mg/mL) and E. faecalis (3.13 mg/mL), being less effective against E. coli. Curiously, the hexane fraction was the most effective against S. aureus and E. faecalis (1.56 mg/ml), while the dichloromethane and ethyl acetate fractions presented limited activity. No activity was detected in the aqueous fraction. Ampicillin was used as a control, showing high effectiveness against S. aureus and E. coli. The genus Wigandia has been reported to have antimicrobial activities. The activity of three extracts (n-hexane, ethanol, and acetone) of W. caracasana leaves has been reported against the strains Streptococcus pneumoniae, S. pyogenes, E. coli, Salmonella typhi and Shigella flexneri with zones of inhibition between 6 and 12 mm (Cáceresa et al., 1993). Another species, Wigandia urens, has reported the antimicrobial activity of the ethanolic extract of leaves against strains S. aureus, E. coli, and P. aeruginosa with diameters between 13-19 mm (Rojas et al., 2003). Another study reported the antimicrobial activity of Cordia oncocalyx (Boraginaceae) with MIC values <512 µg/mL (Thyalisson da Costa Silva et al., 2024). Our results were like those reported for the species Echium humile, who reported MCB values between 1.56 and 12.5 mg/mL against S. aureus, 0.19 and 12.5 against E. faecalis, 1.56 and 3.12 against E. coli, using different extraction solvents (Aouadi et al., 2022). Although the extract and subfractions showed low antibacterial activity, these results are interesting, considering that these come directly from a leaf extract.
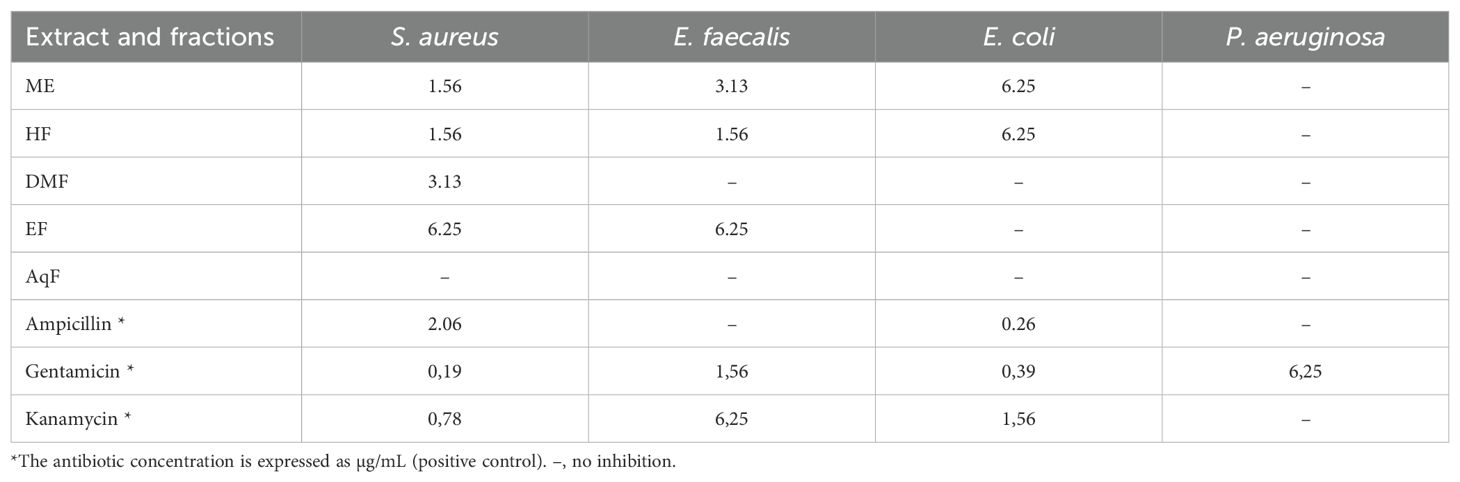
Table 4. Minimum bactericidal concentration (mg/mL) of leaf extracts against 4 pathogenic bacteria by microdilution assay.
3.5 Enzyme inhibitory activity
The inhibitory activity of the most polar fractions (EF and AqF) of W. ecuadoriensis leaves was determined by spectrophotometric assays against α-glucosidase and cholinesterases (AChE, BChE) (Table 5). Inhibition of α-glucosidase is seen as an effective strategy for the control of obesity and diabetes (Zengin et al., 2018). This enzyme, located at the edge of the small intestine, breaks down complex carbohydrates into glucose. By inhibiting α-glucosidase, the metabolism of complex carbohydrates is slowed down, which lowers blood glucose levels (Mustikasari et al., 2024). Figure 3 shows the effect of EF and acarbose on α-glucosidase enzyme activity. Figure 3 shows that increasing the concentration of EF (10–100 µg/mL) and acarbose (10–200 µg/mL) increased the inhibition of α-glucosidase activity. At the highest concentrations, EF and acarbose (100 and 200 µg/mL) achieved inhibitions of 73.58 ± 0.36% and 59.39 ± 0.45%, respectively. The IC50 values for α-glucosidase inhibition were 38.44 ± 0.75 µg/mL for EF (Figure 3A), which is approximately five times higher than the commercial standard, acarbose (179.07 ± 1.18 µg/mL, Figure 3B). These results indicate that the ethyl acetate (EF) fraction is more effective than acarbose in inhibiting α-glucosidase. According to (Benjamin et al., 2024) IC50 values lower than 50 μg/mL are indicators of a strong potential as an inhibitor of α-glucosidase activity. In this sense, it is important to highlight that our result was obtained from a fraction and not from an isolated compound, which highlights a promising bioactive potential for the species. The presence of several unpurified compounds within the fraction suggests that upon further purification, the inhibitory activity could even be enhanced, revealing individual compounds with even stronger properties. This observed enzyme inhibition could be related to the presence of phenolic compounds described in Table 1. On the other hand, the aqueous fraction showed low inhibition, reaching only 4.75 ± 0.16% at a concentration of 2 mg/mL, therefore, its IC50 value could not be determined. Several studies have investigated the inhibitory potential of α-glucosidase in species of the order Boraginales, showing promising results. Another medicinal herb that has been used for centuries to treat diabetes is Symphytum. The inhibitory effect of the whole plant extract of Symphytum anatolicum showed a potent inhibitory activity (IC50: 18.28 ± 0.31 μg/mL), comparable to that exerted by acarbose (IC50: 17.05 ± 0.25 μg/mL), used as a control (Kılınc et al., 2023). The methanolic extract of Echium humile presented a value of 60 µg/mL, indicating a strong effectiveness (Aouadi et al., 2022). An in vitro antidiabetic study revealed that ethyl acetate extract exhibits 60% inhibition, at a concentration of 500 μg/mL, with an IC50 value of 380 μg/mL and the IC50 value of standard acarbose was 250 μg/mL (Syed Akbar et al., 2023). The aqueous extract of Glandora diffusa showed a potent inhibitory effect on a-glucosidase with an IC50 value of 33.3 µg/mL, almost ten times lower than that described for acarbose 300 µg/mL. These authors attribute this activity to the compounds caffeic acid and rosmarinic acid, which have been reported as inhibitors of α-glucosidase, and which are also present in our study (Table 3) (Ferreres et al., 2013). According to these IC50 values, the ethyl acetate fraction studied in this work seems to show an enzyme inhibition capacity comparable to that reported in previous studies.
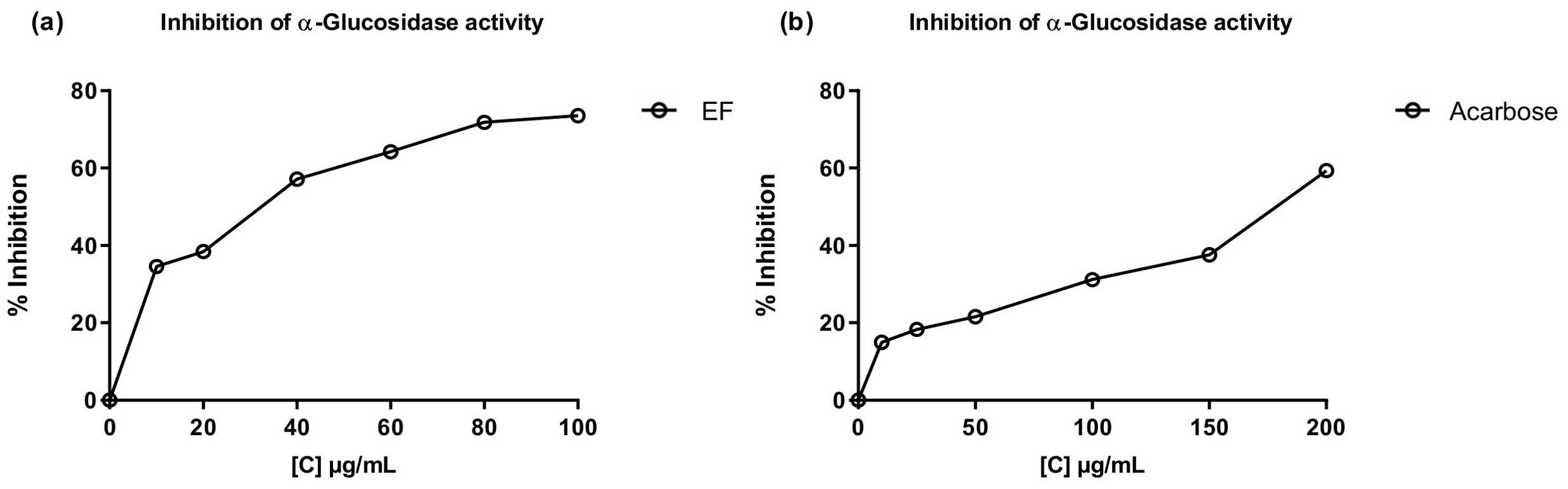
Figure 3. Inhibitory effects of EF from W. ecuadoriensis (A) and galantamine (B) on the enzyme α-glucosidase. Each point represents the average of three measurements.
Cholinesterase inhibitors play a crucial role in the functioning of the nervous system and are related to the treatment of Alzheimer’s disease. Over the past two decades, the search for natural products related to AChE and BChE inhibition has increased (Ortega de Oliveira et al., 2024). Figure 4 shows the inhibition of AChE and BChE enzymes in the presence of AqF and galantamine at increasing concentrations. The results revealed a dose-dependent behavior for the AChE enzyme with an IC50 value of 915.98 ± 7.25 µg/mL for AqF (Figure 4A). Regarding the effect on BChE, Figure 4C shows that the fraction inhibits the butyrylcholinesterase enzyme depending on the concentration with an IC50 value of 380.42 ± 22.10 µg/mL for AqF (Figure 4C). The ethyl acetate fraction did not show any inhibitory activity. These results were higher compared to the positive control galantamine, which presented an IC50 of 0.53 ± 0.03 µg/mL for AChE and 5.15 ± 0.44 µg/mL for BChE, indicating that the standard drug, galantamine, is more effective in inhibiting these enzymes compared to the aqueous fraction of W. ecuadoriensis. However, this result is still encouraging, since we are evaluating a fraction and not an isolated compound. It is likely that the bioactive compounds responsible for the activity are found in low concentration within the fraction, suggesting that further purification could significantly increase the inhibitory activity. The results obtained for AChE are comparable to that reported for the methanolic extract of Wigandia urens, with an AChE inhibition of 43% evaluated at a concentration of 1 mg/mL (Ortiz et al., 2013). There is information on the traditional use of the Wigandia urens species in Guatemala for epilepsy and psychological problems (Hitziger, 2016). On the other hand, some extracts from the Boraginaceae family (S. anatolicum, S. aintabicum, Cynoglossum creticum, C. barrelieri, and Alkanna sfikasiana) have shown inhibitory effects on AChE, BChE, and α-glucosidase (Varvouni et al., 2020). Our results presented lower IC50 compared to other species of Onosma trapezuntea and Onosma rigidum with IC50 values of 1270 and 1180 µg/mL for AChE and 2550 and 2060 µg/mL for BChE, respectively (Kirkan et al., 2022). Another study reported that hexane, chloroform, ethyl acetate and methanol extracts of Calophyllum gracilentum, at a concentration of 1.0 mg/mL, inhibited AChE by 3.02 ± 0.998%, 12.30 ± 5.641%, 31.62 ± 2.057% and 4.61 ± 2.129%, respectively (Seruji et al., 2024). In our study, we evaluated at the same concentration reported an inhibition of 51.20 ± 0.60%. Based on these values, the fraction investigated in this study seems to be more effective in inhibiting this enzyme compared to previously reported results.
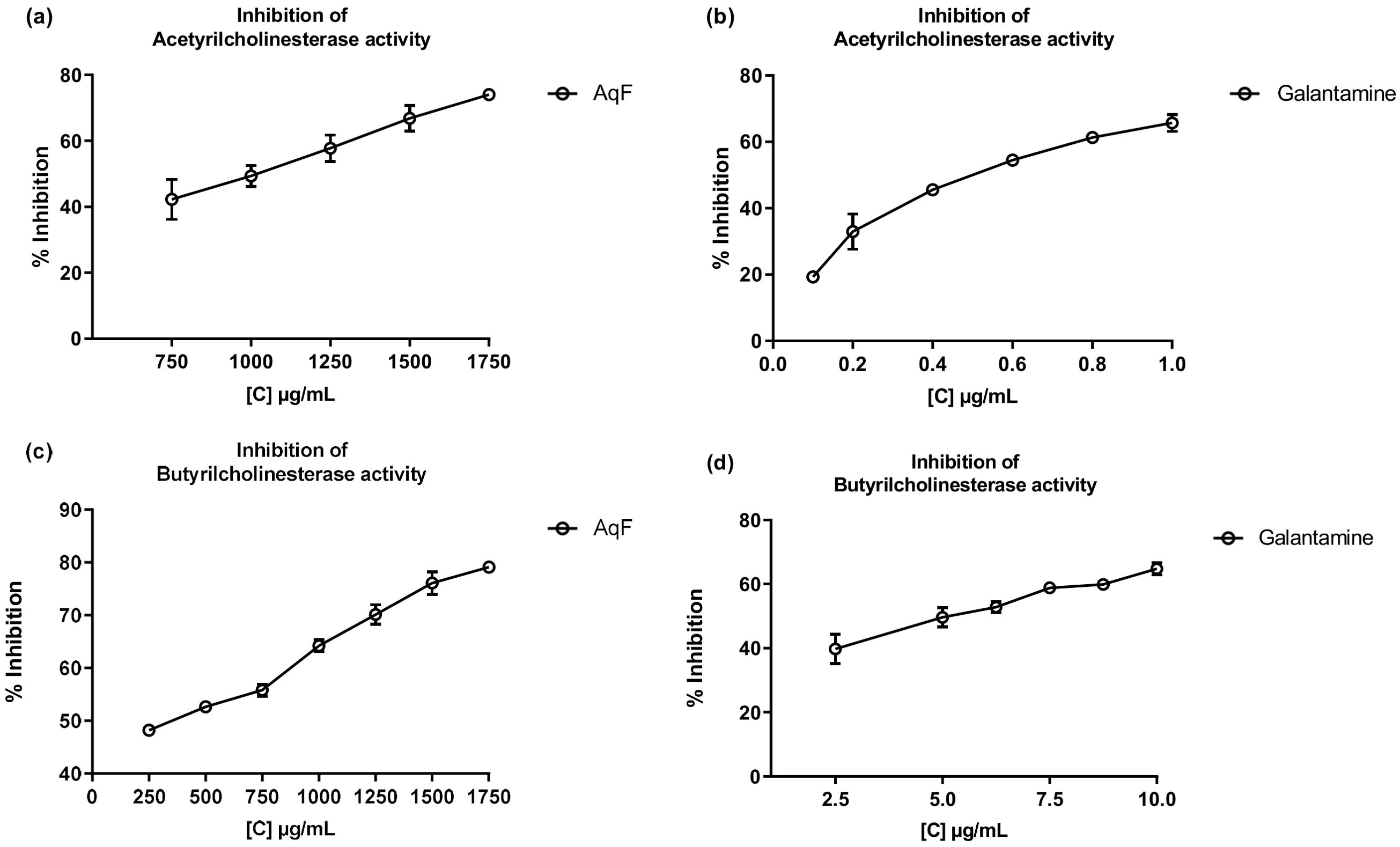
Figure 4. Inhibitory effects of AqF from W. ecuadoriensis and galantamine on cholinesterase enzymes. (A, B) Effect on AChE; (C, D) Effect on BChE. Each point represents the average of three measurements.
The results obtained in this study reveal that certain fractions of W. ecuadoriensis possess a strong antioxidant potential and a remarkable inhibitory activity of the enzyme α-glucosidase, suggesting that this species could be a promising source of bioactive compounds. However, extensive research should be carried out to isolate the main compounds and determine their activity, in order to understand their mechanism of action better. Furthermore, these observations provide a valuable scientific contribution to the chemical knowledge and biological properties of the plant species Wigandia ecuadoriensis.
3.6 UHPLC-QTOF-MS analysis
The compounds from the ethyl acetate and aqueous fractions of the methanolic extract of W. ecuadoriensis leaves were analyzed by UHPLC-QTOF-MS. The total ion current chromatogram in negative ESI mode is shown in Figure 5, and the tentatively detected compounds are summarized in Table 3. The UHPLC-QTOF-MS profile revealed the presence of 39 metabolites, belonging to the classes of phenolic acids, flavonoids, fatty acyls, naphthofuran, glycerolipids, terpene, alkaloid, prenol lipids. The tentative identification was performed in Metaboscape software, a proprietary Bruker software that allows the identification of metabolites based on their mass, fragmentation pattern, and isotopic pattern, subsequently compared with the MassBank of North America (MoNA) database. These compounds include two phenolic acids (peaks 2 and 7), eleven flavonoids (peaks 3, 5, 6, 10, 18, 23, 25, 26, 27, 28 and 30), one lignan (peak 4), four fatty acyls (peaks 8, 9, 16, 22 and 38), four prenol lipids (peaks 11, 29, 32 and 39), one naphthofuran (peak 12), one glycerolipids (peak 13), two terpenes (peaks 14 and 15), and one alkaloid (peak 17), four lactones (peaks 19, 20, 21 and 24), three steroidal (peaks 31, 35 and 36), three unknown compounds (peaks 33, 34 and 37).
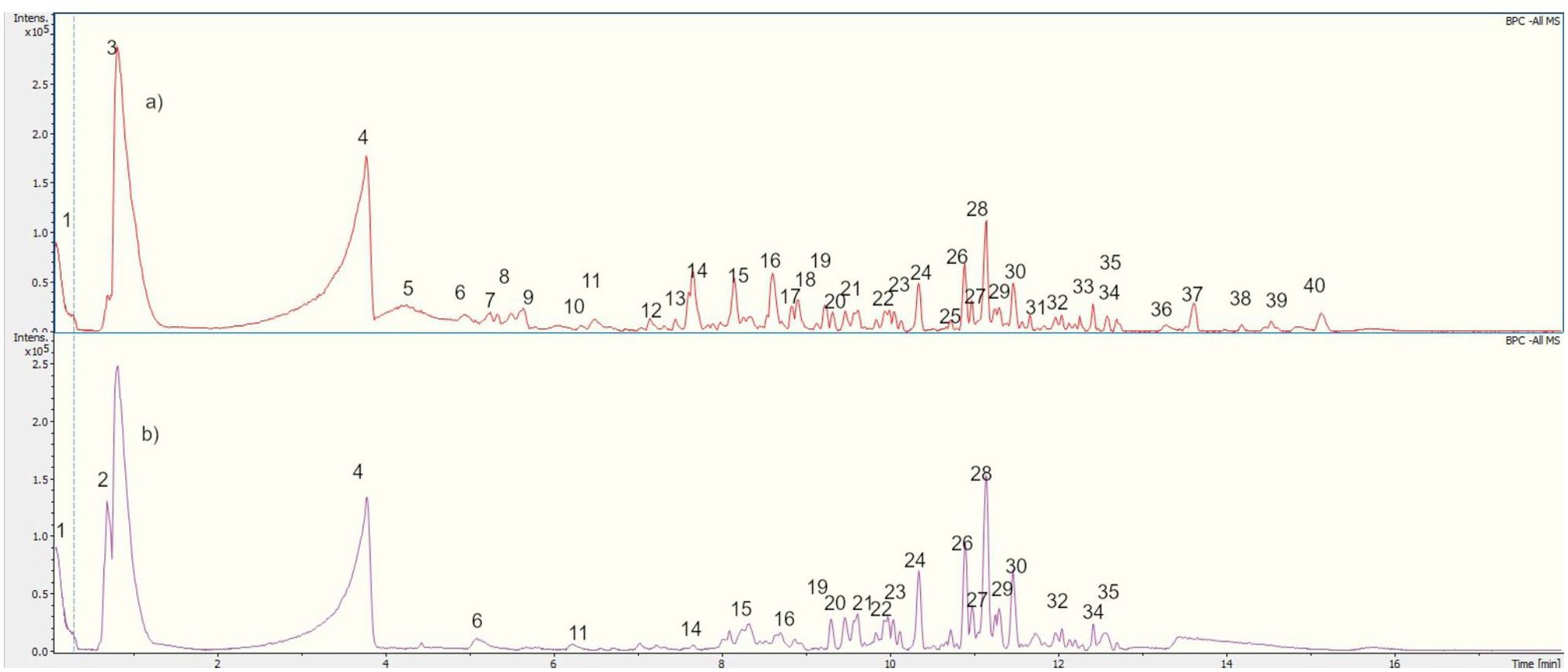
Figure 5. UHPLC-QTOF-MS Chromatogram of (A) EF and (B) AqF leaves W. ecuadoriensis in a negative ion mode.
The compounds identified in the subfractions of W. ecuadoriensis exhibit a wide range of biological activities. Quinic acid shows antioxidant, antidiabetic, anticancer, antimicrobial, antiviral, antiaging, protective, antinociceptive, and analgesic properties (Benali et al., 2022). 3,4-Dihydroxybenzeneacetic and caffeic acids possess antioxidant activity in rat plasma (Raneva et al., 2001). Citric acid, known for its antimicrobial and antioxidant properties (Søltoft-Jensen and Hansen, 2005). Other compounds such as 3-hydroxybenzaldehyde, p-hydroxybenzoic acid, and hyperoside exhibit multiple properties, from antioxidant and anti-inflammatory to anticancer and organ protective (Rohini et al., 2013; Chen et al., 2021; Wang et al., 2022). Luteolin-7-glucoside, rosmarinic acid, and cirsimaritin have antioxidant, antitumor, anti-inflammatory, and protective activities against various diseases (Cai et al., 2020; Silva et al., 2020; De Stefano et al., 2021). Strobilactone A is known for its antifungal activity (Cohen et al., 2011), while thymol-beta-D-glucoside and dimorpholicacid have antibacterial activity (Mundt et al., 2003; Anderson et al., 2021). Cinchonina, in addition to being an antimalarial agent, has anticancer, antiobesity, anti-inflammatory, antiparasitic, antimicrobial, and antiplatelet effects (Parveen et al., 2024). Derivatives of acaranoic acid exhibit potent antifungal action against Botrytis cinerea, Septoria tritici and Pyricularia oryzae (Hussain et al., 2012). Luteolin-8-C-β-D-glucopyranoside and apigenin-8-C-(6”acetyl)-β-D-glucopyranoside stand out for their antioxidant capacity to scavenge free radicals (Simirgiotis et al., 2013). Kurilensoside G shows moderate inhibition in sea urchin sperm tests (Stonik et al., 2008). Erinacine D promotes nerve growth factor synthesis (Kawagishi et al., 1996). Tokoronin inhibits α-MSH-induced melanogenesis with low cytotoxicity (Ukiya et al., 2020). Aureosurfactin, a biosurfactant with comparable activity to rhamnolipid, surfactin and sophorolipid (Kim et al., 2016). Hydrogenoside C enhances cell viability and procollagen type I production in UVB-irradiated Hs68 cells (Shin et al., 2019).
4 Conclusion
This study is the first report of the in vitro activity of W. ecuadoriensis leaves. The ethyl acetate fraction was shown to have the highest content of phenols and flavonoids compared to the other fractions. This result elicited potent antioxidant activity. Furthermore, the ethyl acetate fraction was found to have strong potential as an inhibitor of α-glucosidase activity. On the other hand, the methanolic extract and its hexane fraction revealed antimicrobial activity. According to the UHPLC-MS results, the dominant compounds present in the fractions are caffeic acid, and hyperoside. These findings represent a valuable contribution to the knowledge of the species and suggest that Wigandia could be a promising source of bioactive compounds, creating new opportunities for the development of phytopharmaceuticals. Nevertheless, these initial results underline the need for further research to isolate the main compounds of the ethyl acetate fraction of W. ecuadoriensis to validate its antidiabetic effects.
Data availability statement
The datasets presented in this study can be found in online repositories. The names of the repository/repositories and accession number(s) can be found in the article/supplementary material.
Author contributions
RV: Conceptualization, Project administration, Visualization, Writing – original draft, Investigation. FE: Investigation, Methodology, Writing – review & editing. XC: Data curation, Methodology, Writing – review & editing. MS: Supervision, Writing – review & editing. PM: Writing – review & editing.
Funding
The author(s) declare financial support was received for the research, authorship, and/or publication of this article. The authors acknowledge the Biotechnology Research Center of Ecuador (CIBE) for supporting founding, Project CIBE-13-2023 and Fondecyt 1220075.
Acknowledgments
The authors acknowledge the Fondequip EQM170172.
Conflict of interest
The authors declare that the research was conducted in the absence of any commercial or financial relationships that could be construed as a potential conflict of interest.
Publisher’s note
All claims expressed in this article are solely those of the authors and do not necessarily represent those of their affiliated organizations, or those of the publisher, the editors and the reviewers. Any product that may be evaluated in this article, or claim that may be made by its manufacturer, is not guaranteed or endorsed by the publisher.
References
Anand, P., Singh, B. (2013). A review on cholinesterase inhibitors for Alzheimer’s disease. Arch. Pharm. Res. 36, 375–399. doi: 10.1007/s12272-013-0036-3
Anderson, R. C., Levent, G., Petrujkić, B. T., Harvey, R. B., Hume, M. E., He, H., et al. (2021). Antagonistic effects of lipids against the anti-escherichia coli and anti-salmonella activity of thymol and thymol-β-d-glucopyranoside in porcine gut and fecal cultures in vitro. Front. Vet. Sci. 8. doi: 10.3389/fvets.2021.751266
Aouadi, K., Hajlaoui, H., Arraouadi, S., Ghannay, S., Snoussi, M., Kadri, A. (2022). Phytochemical profiling, antimicrobial and α-glucosidase inhibitory potential of phenolic-enriched extracts of the aerial parts from echium humile desf.: in vitro combined with in silico approach. Plants 11, 1131. doi: 10.3390/plants11091131
Baliyan, S., Mukherjee, R., Priyadarshini, A., Vibhuti, A., Gupta, A., Pandey, R. P., et al. (2022). Determination of antioxidants by DPPH radical scavenging activity and quantitative phytochemical analysis of ficus religiosa. Molecules 27, 1326. doi: 10.3390/molecules27041326
Barrientos, R. E., Romero-Parra, J., Cifuentes, F., Palacios, J., Romero-Jola, N. J., Paredes, A., et al. (2023). Chemical fingerprinting, aorta endothelium relaxation effect, and enzymatic inhibition of canelo (Drimys winteri J. R. Forst. & G. Forst, (D.C) A. Gray, family winteraceae) fruits. Foods 12, 2580. doi: 10.3390/foods12132580
Benali, T., Bakrim, S., Ghchime, R., Benkhaira, N., El Omari, N., Balahbib, A., et al. (2022). Pharmacological insights into the multifaceted biological properties of quinic acid. Biotechnol. Genet. Eng. Rev., 1–30. doi: 10.1080/02648725.2022.2122303
Benjamin, M. A. Z., Mohd Mokhtar, R. A., Iqbal, M., Abdullah, A., Azizah, R., Sulistyorini, L., et al. (2024). Medicinal plants of Southeast Asia with anti-α-glucosidase activity as potential source for type-2 diabetes mellitus treatment. J. Ethnopharmacol 330, 118239. doi: 10.1016/j.jep.2024.118239
Cáceresa, A., Figueroa, L., Taracena, A. M., Samayoa, B. (1993). Plants used in Guatemala for the treatment of respiratory diseases. 2: Evaluation of activity of 16 plants against Gram-positive bacteria. J. Ethnopharmacol 39, 77–82. doi: 10.1016/0378-8741(93)90053-8
Cai, Y., Zheng, Q., Sun, R., Wu, J., Li, X., Liu, R. (2020). Recent progress in the study of Artemisiae Scopariae Herba (Yin Chen), a promising medicinal herb for liver diseases. Biomedicine Pharmacotherapy 130, 110513. doi: 10.1016/j.biopha.2020.110513
Cao, S., Rossant, C., Ng, S., Buss, A. D., Butler, M. S. (2003). Phenolic derivatives from Wigandia urens with weak activity against the chemokine receptor CCR5. Phytochemistry 64, 987–990. doi: 10.1016/S0031-9422(03)00448-5
Chen, K.-Y., Chen, Y.-J., Cheng, C.-J., Jhan, K.-Y., Chiu, C.-H., Wang, L.-C. (2021). 3-Hydroxybenzaldehyde and 4-Hydroxybenzaldehyde enhance survival of mouse astrocytes treated with Angiostrongylus cantonensis young adults excretory/secretory products. BioMed. J. 44, S258–S266. doi: 10.1016/j.bj.2020.11.008
Cohen, E., Koch, L., Thu, K. M., Rahamim, Y., Aluma, Y., Ilan, M., et al. (2011). Novel terpenoids of the fungus Aspergillus insuetus isolated from the Mediterranean sponge Psammocinia sp. collected along the coast of Israel. Bioorg Med. Chem. 19, 6587–6593. doi: 10.1016/j.bmc.2011.05.045
Coral Caycho, E. R., Calixto Cotos, M. R., Soberón Lozano, M. M. (2020). Actividad inhibitoria in vitro de los extractos acuosos de los frutos de Hylocereus megalanthus y Passiflora tripartita var. mollisima sobre las enzimas α-amilasa y α-glucosidasa. Rev. la Sociedad Química del Perú 86, 93–104. doi: 10.37761/rsqp.v86i2.279
Cornejo, X. (2006). Wigandia Ecuadorensis (Hydrophyllaceae), una Nueva Especie del Bosque Muy Seco Tropical al Occidente de Ecuador. Novon 16, 324–327. doi: 10.3417/1055-3177(2006)16[324:WEHUNE]2.0.CO;2
De Stefano, A., Caporali, S., Di Daniele, N., Rovella, V., Cardillo, C., Schinzari, F., et al. (2021). Anti-inflammatory and proliferative properties of luteolin-7-O-glucoside. Int. J. Mol. Sci. 22, 1321. doi: 10.3390/ijms22031321
Elisha, I. L., Botha, F. S., McGaw, L. J., Eloff, J. N. (2017). The antibacterial activity of extracts of nine plant species with good activity against Escherichia coli against five other bacteria and cytotoxicity of extracts. BMC Complement Altern. Med. 17, 133. doi: 10.1186/s12906-017-1645-z
Farhat, M.B., Landoulsi, A., Chaouch-Hamada, R., Sotomayor, J. A., Jordán, M. J. (2013). Characterization and quantification of phenolic compounds and antioxidant properties of Salvia species growing in different habitats. Ind. Crops Prod 49, 904–914. doi: 10.1016/j.indcrop.2013.06.047
Ferreres, F., Vinholes, J., Gil-Izquierdo, A., Valentão, P., Gonçalves, R. F., Andrade, P. B. (2013). In vitro studies of α-glucosidase inhibitors and antiradical constituents of Glandora diffusa (Lag.) D.C. Thomas infusion. Food Chem. 136, 1390–1398. doi: 10.1016/j.foodchem.2012.09.089
Ghareeb, M. A., Mohamed, T., Saad, A. M., Refahy, L. A.-G., Sobeh, M., Wink, M. (2017). HPLC-DAD-ESI-MS/MS analysis of fruits from Firmiana simplex (L.) and evaluation of their antioxidant and antigenotoxic properties. J. Pharm. Pharmacol. 70, 133–142. doi: 10.1111/jphp.12843
Gómez, F., Quijano, L., Calderón, J. S., Ríos, T. (1980). Terpenoids isolated from Wigandia kunthii. Phytochemistry 19, 2202–2203. doi: 10.1016/S0031-9422(00)82225-6
Hitziger, M. (2016). Mayan phytotherapy in Guatemala: a transdisciplinary study for ethnographic documentation and local empowerment.
Hussain, H., Krohn, K., Schulz, B., Draeger, S., Nazir, M., Saleem, M. (2012). Two new antimicrobial metabolites from the endophytic fungus, seimatosporium sp. Nat. Prod Commun. 7, 1934578X1200700. doi: 10.1177/1934578X1200700305
Kawagishi, H., Simada, A., Shizuki, K., Ojima, F., Mori, H., Okamoto, K., et al. (1996). Erinacine D, a stimulator of NGF-synthesis, from the mycelia of Hericium erinaceum. Heterocycl Comm 2. doi: 10.1515/HC.1996.2.1.51
Kim, J.-S., Lee, I.-K., Kim, D.-W., Yun, B.-S. (2016). Aureosurfactin and 3-deoxyaureosurfactin, novel biosurfactants produced by Aureobasidium pullulans L3-GPY. J. Antibiot (Tokyo) 69, 759–761. doi: 10.1038/ja.2015.141
Kirkan, B., Sarikurkcu, C., Zengin, G. (2022). Bioactive constituents, antioxidant effects and enzyme inhibitory properties of two Onosma species (Onosma trapezuntea and O. rigidum). South Afr. J. Bot. 145, 142–148. doi: 10.1016/j.sajb.2021.09.036
Kılınc, H., D’Urso, G., Paolillo, A., Alankus, O., Piacente, S., Masullo, M. (2023). LC-MS and NMR based plant metabolomics: A comprehensive phytochemical investigation of symphytum anatolicum. Metabolites 13, 1051. doi: 10.3390/metabo13101051
Mundt, S., Kreitlow, S., Jansen, R. (2003). Fatty acids with antibacterial activity from the cyanobacterium Oscillatoria redekei HUB 051. J. Appl. Phycol 15, 263–267. doi: 10.1023/A:1023889813697
Mustikasari, K., Santoso, M., Fadzelly Abu Bakar, M., Fatmawati, S. (2024). Antioxidant, α-glucosidase inhibitory, and cytotoxic activities of Mangifera rufocostata extract and identification of its compounds by LC-MS/MS analysis. Arabian J. Chem. 17, 105391. doi: 10.1016/j.arabjc.2023.105391
Okusa, P. N., Penge, O., Devleeschouwer, M., Duez, P. (2007). Direct and indirect antimicrobial effects and antioxidant activity of Cordia gilletii De Wild (Boraginaceae). J. Ethnopharmacol 112, 476–481. doi: 10.1016/j.jep.2007.04.003
Ortega de Oliveira, P. C., da Silva, G. M., Cass, Q. B., de Moraes, M. C., Cardoso, C. L. (2024). Natural products as a source of cholinesterase inhibitors. Pharmacol. Res. - Natural Products, 100099. doi: 10.1016/j.prenap.2024.100099
Ortiz, D., Valdez, A., Cáceres, A. (2013). Actividad inhibitoria de la acetilcolinesterasa por extractos de 18 especies vegetales nativas de Guatemala usadas en el tratamiento de afecciones nerviosas. Rev. Cient. Fac. Cienc. Quim. Farm. 22, 17–25. doi: 10.54495/Rev.Cientifica.v23i1.108
Parveen, S., Maurya, N., Meena, A., Luqman, S. (2024). Cinchonine: A versatile pharmacological agent derived from natural cinchona alkaloids. Curr. Top. Med. Chem. 24, 343–363. doi: 10.2174/0115680266270796231109171808
Raneva, V., Shimasaki, H., Ishida, Y., Ueta, N., Niki, E. (2001). Antioxidative activity of 3,4-dihydroxyphenylacetic acid and caffeic acid in rat plasma. Lipids 36. doi: 10.1007/s11745-001-0821-6
Reynolds, G. W., Gafner, F., Rodriguez, E. (1989). Contact allergens of an urban shrub Wigandia caracasana. Contact Dermatitis 21, 65–68. doi: 10.1111/j.1600-0536.1989.tb04698.x
Richards, A., Chaurasia, S. (2020). “A Promising Nutraceutical Eriodictyon californicum, a “Holy Herb,” with its Healing Abilities against Oxidative Stress,” in The 1st International Electronic Conference on Food Science and Functional Foods (MDPI, Basel Switzerland), 64. doi: 10.3390/foods_2020-07675
Richards, A., Chaurasia, S. (2022). Antioxidant activity and reactive oxygen species (ROS) scavenging mechanism of eriodictyon californium, an edible herb of North America. J. Chem. 2022, 1–8. doi: 10.1155/2022/6980121
Rohini, M., Shikha, S., Akash, J., Jasmine, C. (2013). A comprehensive review on biological activities of P-hydroxy benzoic acid and its derivatives. Int. J. Pharm. Sci. Rev. Res. 22, 109–115.
Rojas, R., Bustamante, B., Bauer, J., Fernández, I., Albán, J., Lock, O. (2003). Antimicrobial activity of selected Peruvian medicinal plants. J. Ethnopharmacol 88, 199–204. doi: 10.1016/S0378-8741(03)00212-5
Sarikurkcu, C., Sahinler, S. S., Ceylan, O., Tepe, B. (2020). Onosma ambigens: Phytochemical composition, antioxidant and enzyme inhibitory activity. Ind. Crops Prod 154, 112651. doi: 10.1016/j.indcrop.2020.112651
Seruji, N. M. U., Jong, V. Y. M., Karunakaran, T., Zamakshshari, N. H., Mah, S. H., Gunter, N. V., et al. (2024). Molecular docking studies and in-vitro cholinesterase inhibitory activities of chemical constituents of Calophyllum gracilentum. Phytochem. Lett. 63, 93–100. doi: 10.1016/j.phytol.2024.08.007
Setha, B., Gaspersz, F., Idris, A., Rahman, S., Mailoa, M. (2013). Potential of seaweed padina sp. As A source of antioxidant. Int. J. Sci. \& Technol. Res. 2, 221–224.
Shin, J.-S., Han, H.-S., Lee, S.-B., Myung, D., Lee, K., Lee, S. H., et al. (2019). Chemical constituents from leaves of hydrangea serrata and their anti-photoaging effects on UVB-irradiated human fibroblasts. Biol. Pharm. Bull. 42, 424–431. doi: 10.1248/bpb.b18-00742
Silva, M., Caro, V., Guzmán, C., Perry, G., Areche, C., Cornejo, A. (2020). α-Synuclein and tau, two targets for dementia. 1–25. doi: 10.1016/B978-0-12-819483-6.00001-1
Simirgiotis, M., Schmeda-Hirschmann, G., Bórquez, J., Kennelly, E. (2013). The passiflora tripartita (Banana passion) fruit: A source of bioactive flavonoid C-glycosides isolated by HSCCC and characterized by HPLC–DAD–ESI/MS/MS. Molecules 18, 1672–1692. doi: 10.3390/molecules18021672
Søltoft-Jensen, J., Hansen, F. (2005). “New chemical and biochemical hurdles,” in Emerging Technologies for Food Processing (Elsevier), 387–416. doi: 10.1016/B978-012676757-5/50017-7
Stonik, V. A., IvanChina, N. V., Kicha, A. A. (2008). New polar steroids from starfish. Nat. Prod Commun. 3, 1934578X0800301. doi: 10.1177/1934578X0800301005
Syed Akbar, W. A., Arokiarajan, M. S., John Christopher, J., Zaheer Ahmed, N., Meena, R. (2023). Evaluation of bioactive compounds as antimicrobial and antidiabetic agent from the crude extract of Heliotropium curassavicum L. Biocatal Agric. Biotechnol. 50, 102745. doi: 10.1016/j.bcab.2023.102745
Tabart, J., Kevers, C., Pincemail, J., Defraigne, J.-O., Dommes, J. (2009). Comparative antioxidant capacities of phenolic compounds measured by various tests. Food Chem. 113, 1226–1233. doi: 10.1016/j.foodchem.2008.08.013
Thaipong, K., Boonprakob, U., Crosby, K., Cisneros-Zevallos, L., Hawkins Byrne, D. (2006). Comparison of ABTS, DPPH, FRAP, and ORAC assays for estimating antioxidant activity from guava fruit extracts. J. Food Composition Anal. 19, 669–675. doi: 10.1016/j.jfca.2006.01.003
Thyalisson da Costa Silva, J., Lima Bezerra, J. J., Moura, T. F., Pereira da Cruz, R., Gregorio de Oliveira, M., Costa, A. R., et al. (2024). Phytochemical analysis and evaluation of the antibacterial and antibiotic potentiation activities of the aqueous extract of Cordia oncocalyx Allemão (Boraginaceae). Pharm. Sci. Adv. 2, 100042. doi: 10.1016/j.pscia.2024.100042
Torre, L., Navarrete, H., Muriel M., P., Macía Barco, M. J., Balslev, H. (2008). Enciclopedia de las plantas útiles del Ecuador.
Trifan, A., Zengin, G., Sinan, K. I., Wolfram, E., Skalicka-Woźniak, K., Luca, S. V. (2021). LC-HRMS/MS phytochemical profiling of Symphytum officinale L. and Anchusa ochroleuca M. Bieb. (Boraginaceae): Unveiling their multi-biological potential via an integrated approach. J. Pharm. BioMed. Anal. 204, 114283. doi: 10.1016/j.jpba.2021.114283
Ukiya, M., Sato, D., Kimura, H., Hirai, Y., Nishina, A. (2020). Tokoronin Contained in Dioscorea tokoro Makino ex Miyabe Suppressed α -MSH-Induced Melanogenesis in B16 Cells via Suppression of Classical MAPK Pathway Activation. Chem. Biodivers 17. doi: 10.1002/cbdv.202000077
van den Berg, R., Haenen, G. R. M. M., van den Berg, H., Bast, A. (1999). Applicability of an improved Trolox equivalent antioxidant capacity (TEAC) assay for evaluation of antioxidant capacity measurements of mixtures. Food Chem. 66, 511–517. doi: 10.1016/S0308-8146(99)00089-8
Varvouni, E.-F., Zengin, G., Graikou, K., Ganos, C., Mroczek, T., Chinou, I. (2020). Phytochemical analysis and biological evaluation of the aerial parts from Symphytum anatolicum Boiss. and Cynoglottis barrelieri (All.) Vural & Kit Tan (Boraginaceae). Biochem. Syst. Ecol. 92, 104128. doi: 10.1016/j.bse.2020.104128
Vasile, M.-A., Jeiter, J., Weigend, M., Luebert, F. (2020). Phylogeny and historical biogeography of Hydrophyllaceae and Namaceae, with a special reference to Phacelia and Wigandia. Syst. Biodivers 18, 757–770. doi: 10.1080/14772000.2020.1771471
Viteri, R., Giordano, A., Montenegro, G., Zacconi, F. (2021). Eucryphia cordifolia extracts: Phytochemical screening, antibacterial and antioxidant activities. Nat. Prod Res. 1–5. doi: 10.1080/14786419.2021.1960525
Wang, Q., Wei, H., Zhou, S., Li, Y., Zheng, T., Zhou, C., et al. (2022). Hyperoside: A review on its sources, biological activities, and molecular mechanisms. Phytotherapy Res. 36, 2779–2802. doi: 10.1002/ptr.7478
Zavala-Sánchez, M. A., Pérez-Gonzàlez, C., Arias-García, L., Pérez-Gutiérrez, S. (2009). Anti-inflammatory activity of Wigandia urens and Acalypha alopecuroides. Afr J. Biotechnol. 8, 5901–5905. doi: 10.5897/AJB2009.000-9468
Keywords: Wigandia ecuadoriensis, enzyme inhibition, antioxidant activity, antibacterial, phenols content, flavonoids
Citation: Viteri R, Espinoza F, Cornejo X, Simirgiotis MJ and Manzano P (2024) Phytochemical profiling, antioxidant, enzymatic inhibitory, and antibacterial activities of Wigandia ecuadorensis. Front. Plant Sci. 15:1481447. doi: 10.3389/fpls.2024.1481447
Received: 15 August 2024; Accepted: 14 October 2024;
Published: 07 November 2024.
Edited by:
Eman A. Mahmoud, Damietta University, EgyptReviewed by:
Dong Pei, Chinese Academy of Sciences (CAS), ChinaViviana Maresca, University of Naples Federico II, Italy
Copyright © 2024 Viteri, Espinoza, Cornejo, Simirgiotis and Manzano. This is an open-access article distributed under the terms of the Creative Commons Attribution License (CC BY). The use, distribution or reproduction in other forums is permitted, provided the original author(s) and the copyright owner(s) are credited and that the original publication in this journal is cited, in accordance with accepted academic practice. No use, distribution or reproduction is permitted which does not comply with these terms.
*Correspondence: Rafael Viteri, cmF2aXRlcmlAZXNwb2wuZWR1LmVj