- Department of Biophysics, N. I. Lobachevsky State University of Nizhny Novgorod, Nizhny Novgorod, Russia
1 Introduction
Terrestrial plants are affected by action of numerous stressors (e.g., increased or decreased temperatures, excess light, mechanic damages, and others) which can be spatially heterogenous. Induction of physiological responses in non-irritated parts of plant can be based on generation and propagation of electrical signals (ESs) including action potentials, variation potentials, and system potentials (Gallé et al., 2015; Li et al., 2021; Pachú et al., 2021; Sukhova et al., 2023). It is known (Gallé et al., 2015; Farmer et al., 2020; Li et al., 2021) that ESs influence expression of defense genes, production of phytohormones, respiration, phloem mass-flow, transpiration, and many other processes and, thereby, increase plant tolerance to action of adverse factors (Szechyńska-Hebda et al., 2017; Zandalinas et al., 2020).
Photosynthesis is an important target of ESs because these signals can change the CO2 assimilation (Ahv), quantum yields of photosystem I and II (γPSI and γPSII), non-photochemical quenching of chlorophyll fluorescence (NPQ), linear electron flow (LEF), and cyclic electron flow around photosystem I (CEF) (Gallé et al., 2015; Szechyńska-Hebda et al., 2017; Sukhova et al., 2023). It is traditionally considered that ESs suppress photosynthetic processes decreasing Ahv, γPSI, γPSII, and LEF and increasing NPQ and CEF (see, e.g., Lautner et al., 2005; Grams et al., 2009; Pavlovič et al., 2011; Gallé et al., 2013; Sukhov et al., 2015; Białasek et al., 2017; Krausko et al., 2017; Szechyńska-Hebda et al., 2022). However, there are works which show that electrical signals can activate photosynthesis including increasing Ahv and γPSII (Grams et al., 2007; Vuralhan-Eckert et al., 2018; Yudina et al., 2022; Grinberg et al., 2023). Considering an important role of the ESs-induced photosynthetic inactivation in stimulation of the plant tolerance to adverse factors (Sukhov, 2016; Sukhova et al., 2023), potential mechanisms of the positive influence of electrical signals on photosynthesis require additional discussion.
2 Mechanisms of ESs-induced photosynthetic inactivation
Mechanisms of the ESs-induced photosynthetic inactivation are relatively investigated now (Sukhov, 2016; Sukhova et al., 2023). There are at least two groups of potential targets of electrical signals: photosynthetic dark and light reactions (Pavlovič et al., 2011; Gallé et al., 2013; Sukhov et al., 2015). The ESs influence on both targets is related to transient inactivation of H+-ATPase in the plasma membrane, which accompanies induction of all types of ESs, increases pH in the apoplast, and decreases pH in the cytoplasm, stroma and lumen of chloroplasts (Sukhova et al., 2023).
Decreasing pH in the chloroplast lumen inactivates photosynthetic light reactions through the direct NPQ stimulation by the PsbS protonation (Ruban, 2015, 2016) and LEF suppression by slowing the plastoquinol oxidation (Tikhonov, 2013, 2014); the last process activates CEF (Sukhov et al., 2015). In contrast, the ESs-induced inactivation of photosynthetic dark reactions is caused by disruption of the CO2 flux into the chloroplast stroma through decreasing the mesophyll CO2 conductance (gm) (Gallé et al., 2013). This response is probable to be related to pH-dependent increase of HCO3-: CO2 ratio in the apoplast (Sukhova et al., 2023) because HCO3- is weakly transported through the plasma membrane (Tholen and Zhu, 2011). Alternative hypotheses explain influence of pH shifts on gm through changes in activity of carbonic anhydrases (Grams et al., 2009) or aquaporins (Gallé et al., 2013). It should be noted that the ESs-induced inactivation of photosynthetic dark reactions also suppresses photosynthetic light reactions providing additional mechanism of changes in NPQ, γPSI, γPSII, LEF, and CEF (Pavlovič et al., 2011; Sukhov et al., 2015; Sukhov, 2016).
3 Participation of changes in stomatal CO2 conductance in forming ESs-induced photosynthetic activation and inactivation
It is known (Flexas et al., 2008, 2012) that the CO2 flux from air to the chloroplast stroma is dependent on gm and the stomatal CO2 conductance (gs). Potentially, it means that changes in gs can also participate in induction of photosynthetic responses by electrical signals. However, ESs-induced decreasing the CO2 assimilation is accompanied by increasing the stomatal CO2 conductance in many investigations (Koziolek et al., 2004; Grams et al., 2009; Gallé et al., 2013); therefore, the changes in gs can weakly influence Ahv in some cases.
Assuming that gm and gs are series connected (Flexas et al., 2008), (Equation 1) can be used for description of the total CO2 conductance (g):
Equation 1 shows that g≈gm at gs>>gm; i.e., ESs-induced changes in gs should not influence the total CO2 conductance and, therefore, assimilation in this case. This point is in a good accordance with works noted above. In contrast, ESs-induced changes in gs can influence g and, thereby, Ahv at gs≈gm or gm>>gs; in the last case (gm>>gs), decreasing gm should not strongly influence the photosynthetic CO2 assimilation.
It is known that electrical signals can induce both initial increasing and decreasing gs (Koziolek et al., 2004; Kaiser and Grams, 2006; Grams et al., 2007, 2009; Gallé et al., 2013). Increasing gs can be observed under specific conditions; e.g., under the high air humidity (Yudina et al., 2019) or strong soil drought (Yudina et al., 2022). It is also probable that ESs induce this increasing in some plant species including, e.g., maize (Grams et al., 2009), mimosa (Kaiser and Grams, 2006), and soybean (Gallé et al., 2013). In accordance with Yudina et al. (2019), final ESs-induced changes in stomatal conductance can be caused by combination of two opposite processes: decreasing turgor of guard cells, which contributes the stomata closure, and decreasing turgor of epidermal cells, which contributes the stomata opening. Therefore, different contributions of these mechanisms can provide both opening and closure of stomata. The turgor decreasing is related to fluxes of ions and protons from the cytoplasm to apoplast, which accompany the transient inactivation of H+-ATPase and changes in activity of ion channels during the ESs generation (Sukhov, 2016; Sukhova et al., 2023).
As a result, it can be hypothesized that the positive influence of ESs on photosynthesis requires increasing gs and substantial contribution of gs to g (gm>>gs). Despite absence of works, which directly investigated influence of ESs-induced increasing gs on Ahv under the low gs:gm ratio, there are points supporting this hypothesis. First, the ESs-induced activation of the photosynthetic CO2 assimilation has dynamics being similar to dynamics of increasing gs in all investigations (Peña-Cortés et al., 1995; Grams et al., 2007; Vuralhan-Eckert et al., 2018; Yudina et al., 2022; Grinberg et al., 2023); in contrast, dynamics of the Ahv inactivation can be similar (Hlavácková et al., 2006; Hlavinka et al., 2012) or different (Koziolek et al., 2004; Kaiser and Grams, 2006; Grams et al., 2009; Gallé et al., 2013) with dynamics of decreasing gs in various works.
Second, decreasing the initial gs (under the strong water deficit), which increases contribution of gs to g in accordance with (Equation 1), transforms the negative influence of electrical signals on photosynthesis into the positive influence (Yudina et al., 2022). In this case, magnitude of the ESs-induced increasing Ahv is positively dependent on the magnitude of the ESs-induced gs increasing. In contrast, decreasing the initial gm (under the high CO2 concentration) increases magnitude of the ESs-induced inactivation of photosynthetic CO2 assimilation (Gallé et al., 2013). It should be additionally noted that pea seedlings, which mainly demonstrate unrelated dynamics of changes in gs and Ahv under favorable conditions (Yudina et al., 2019, 2022), have the high gs:gm ratio under these conditions (Sukhov et al., 2017); i.e., the total CO2 flux into the chloroplast stroma is limited by gm in this plant.
It should be additionally noted that combination of the ESs-induced increasing gs and similar initial values of gm and gs (gs≈gm) can potentially contribute to intricate dynamics of changes in photosynthetic activity because decreasing gm should suppress the CO2 assimilation; in contrast, increasing gs should stimulate this assimilation. Peña-Cortés et al. (1995) showed intricate dynamics of photosynthetic changes (including increasing and decreasing Ahv) after local action of various stressors (the electrical current, mechanical damage, heating); however, these changes were rather related to the intricate dynamics of gs after irritations.
Thus, simultaneous presence of both noted properties (the ESs-induced increasing gs and substantial contribution of gs to g) seems to be possible; at least, in some plants and under specific conditions. It can explain variety of direction of ESs-induced photosynthetic responses (activation or inactivation) which were shown in various works.
4 Discussion
The current analysis shows that ESs-induced photosynthetic responses in terrestrial plants can be result of combination of three groups of processes including suppressing photosynthetic light reactions by acidification of the chloroplast lumen, decreasing gm, and increasing/decreasing gs (Figure 1). The pH-dependent suppression of light reactions is not probable to be strongly related to changes in gm and gs. In contrast, changes in the mesophyll and stomatal CO2 conductance should strongly influence the CO2 flux from air to the chloroplast stroma and, thereby, photosynthesis.
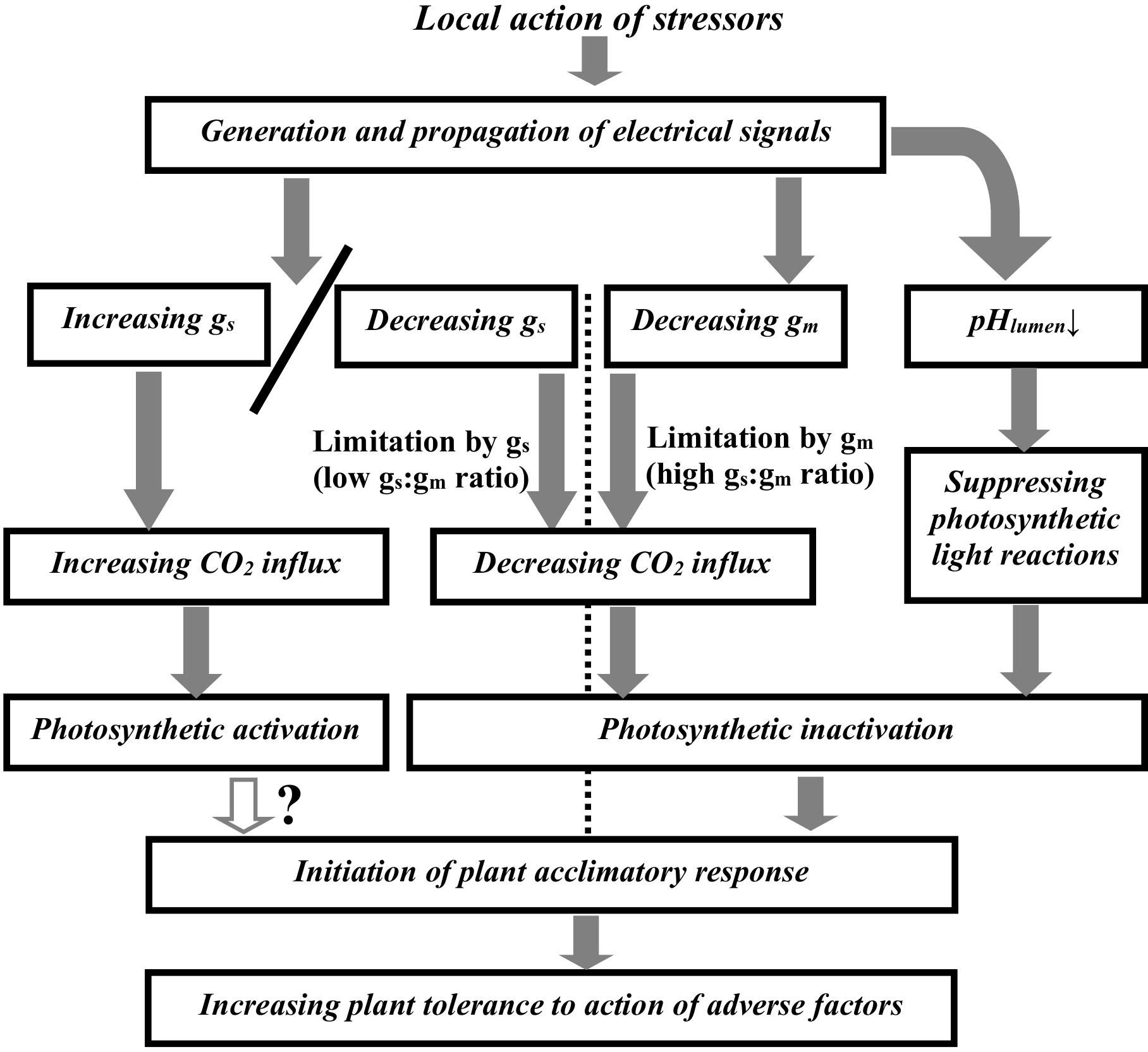
Figure 1. Potential ways of influence of electrical signals on photosynthetic activity. gs is the stomatal CO2 conductance, gm is the mesophyll CO2 conductance. pHlumen is pH in the chloroplast lumen. In accordance with Equation 1, the low ratio of the initial gs to the initial gm corresponds to limitation of the CO2 flux from air to the chloroplast stroma by the stomatal CO2 conductance; the high ratio of the initial gs to the initial gm corresponds to limitation of this CO2 flux by the mesophyll CO2 conductance. Initial conductance is conductance before induction of electrical signals.
The ratio between initial gs and gm is probable to be the first key criterion to determine parameters of ESs-induced photosynthetic responses. The high gs:gm ratio contributes to induction of typical fast photosynthetic inactivation (Sukhov, 2016) which is based on the ESs-induced decreasing gm (Gallé et al., 2013). In this case, dynamics of changes in Ahv and other photosynthetic parameters can be weakly related to changes in the stomatal conductance (Koziolek et al., 2004; Kaiser and Grams, 2006; Grams et al., 2009; Gallé et al., 2013).
In contrast, the low gs:gm ratio can contribute induction of different types of photosynthetic responses. This type is dependent on the second key criterion which is direction of changes in gs. In this case, the ESs-induced decreasing gs should also cause the photosynthetic inactivation; however, dynamics of this inactivation should be strongly related to dynamics of the stomata closure. Particularly, this response was shown in tobacco (Hlavácková et al., 2006) and tomato (Hlavinka et al., 2012) after the local burning and the ESs propagation. The ESs-induced increasing gs should cause the photosynthetic activation with dynamics strongly related to dynamics of stomata opening. This relation is observed in works showing the ESs-induced photosynthetic activation (Grams et al., 2007; Vuralhan-Eckert et al., 2018; Yudina et al., 2022; Grinberg et al., 2023).
It should be additionally noted that proposed mechanism explains the ESs-induced Ahv activation, However, increasing the quantum yield of photosystem II can be also observed after the ESs propagation (Grinberg et al., 2023). This effect is probable to be caused by stimulation of ATP consumption and following decrease of electrochemical H+ gradient across the thylakoid membrane; this decreasing can stimulate electron flows and increase the quantum yield of photosystem II (Tikhonov, 2013, 2014).
Thus, the current analysis preliminary explains potential mechanisms of the ESs-induced photosynthetic activation which was observed in some works (see above). Further checking this explanation requires experimental and model-based investigations; however, there are other questions related to the ESs-induced photosynthetic activation. Particularly, the ESs-induced photosynthetic inactivation is considered to play an important role in increasing tolerance of plants to action of adverse factors (Sukhov, 2016; Sukhova et al., 2023). Considering this point, analysis of influence of the photosynthetic activation on the plant tolerance is an additional important task of future investigations.
Author contributions
VS: Conceptualization, Writing – original draft, Writing – review & editing.
Funding
The author(s) declare that no financial support was received for the research, authorship, and/or publication of this article.
Conflict of interest
The author declares that the research was conducted in the absence of any commercial or financial relationships that could be construed as a potential conflict of interest.
Publisher’s note
All claims expressed in this article are solely those of the authors and do not necessarily represent those of their affiliated organizations, or those of the publisher, the editors and the reviewers. Any product that may be evaluated in this article, or claim that may be made by its manufacturer, is not guaranteed or endorsed by the publisher.
References
Białasek, M., Górecka, M., Mittler, R., Karpiński, S. (2017). Evidence for the Involvement of electrical, calcium and ROS signaling in the systemic regulation of non-photochemical quenching and photosynthesis. Plant Cell Physiol. 58, 207–215. doi: 10.1093/pcp/pcw232
Farmer, E. E., Gao, Y. Q., Lenzoni, G., Wolfender, J. L., Wu, Q. (2020). Wound- and mechanostimulated electrical signals control hormone responses. New Phytol. 227, 1037–1050. doi: 10.1111/nph.v227.4
Flexas, J., Barbour, M. M., Brendel, O., Cabrera, H. M., Carriquí, M., Díaz-Espejo, A., et al. (2012). Mesophyll diffusion conductance to CO2: an unappreciated central player in photosynthesis. Plant Sci. 193-194, 70–84. doi: 10.1016/j.plantsci.2012.05.009
Flexas, J., Ribas-Carbó, M., Diaz-Espejo, A., Galmés, J., Medrano, H. (2008). Mesophyll conductance to CO2: current knowledge and future prospects. Plant Cell Environ. 31, 602–621. doi: 10.1111/j.1365-3040.2007.01757.x
Gallé, A., Lautner, S., Flexas, J., Fromm, J. (2015). Environmental stimuli and physiological responses: The current view on electrical signaling. Environ. Exp. Bot. 114, 15–21. doi: 10.1016/j.envexpbot.2014.06.013
Gallé, A., Lautner, S., Flexas, J., Ribas-Carbo, M., Hanson, D., Roesgen, J., et al. (2013). Photosynthetic responses of soybean (Glycine max L.) to heat-induced electrical signalling are predominantly governed by modifications of mesophyll conductance for CO2. Plant Cell Environ. 36, 542–552. doi: 10.1111/j.1365-3040.2012.02594.x
Grams, T. E., Koziolek, C., Lautner, S., Matyssek, R., Fromm, J. (2007). Distinct roles of electric and hydraulic signals on the reaction of leaf gas exchange upon re-irrigation in Zea mays L. Plant Cell Environ. 30, 79–84. doi: 10.1111/j.1365-3040.2006.01607.x
Grams, T. E., Lautner, S., Felle, H. H., Matyssek, R., Fromm, J. (2009). Heat-induced electrical signals affect cytoplasmic and apoplastic pH as well as photosynthesis during propagation through the maize leaf. Plant Cell Environ. 32, 319–326. doi: 10.1111/j.1365-3040.2008.01922.x
Grinberg, M., Nemtsova, Y., Ageyeva, M., Brilkina, A., Vodeneev, V. (2023). Effect of low-dose ionizing radiation on spatiotemporal parameters of functional responses induced by electrical signals in tobacco plants. Photosynth. Res. 157, 119–132. doi: 10.1007/s11120-023-01027-9
Hlavácková, V., Krchnák, P., Naus, J., Novák, O., Spundová, M., Strnad, M. (2006). Electrical and chemical signals involved in short-term systemic photosynthetic responses of tobacco plants to local burning. Planta 225, 235–244. doi: 10.1007/s00425-006-0325-x
Hlavinka, J., Nožková-Hlaváčková, V., Floková, K., Novák, O., Nauš, J. (2012). Jasmonic acid accumulation and systemic photo-synthetic and electrical changes in locally burned wild type tomato, ABA-deficient sitiens mutants and sitiens pre-treated by ABA. Plant Physiol. Biochem. 54, 89–96. doi: 10.1016/j.plaphy.2012.02.014
Kaiser, H., Grams, T. E. (2006). Rapid hydropassive opening and subsequent active stomatal closure follow heat-induced electrical signals in Mimosa pudica. J. Exp. Bot. 57, 2087–2092. doi: 10.1093/jxb/erj165
Koziolek, C., Grams, T. E. E., Schreiber, U., Matyssek, R., Fromm, J. (2004). Transient knockout of photosynthesis mediated by electrical signals. New Phytol. 161, 715–722. doi: 10.1111/j.1469-8137.2004.00985.x
Krausko, M., Perutka, Z., Šebela, M., Šamajová, O., Šamaj, J., Novák, O., et al. (2017). The role of electrical and jasmonate signalling in the recognition of captured prey in the carnivorous sundew plant Drosera capensis. New Phytol. 213, 1818–1835. doi: 10.1111/nph.2017.213.issue-4
Lautner, S., Grams, T. E. E., Matyssek, R., Fromm, J. (2005). Characteristics of electrical signals in poplar and responses in photosynthesis. Plant Physiol. 138, 2200–2209. doi: 10.1104/pp.105.064196
Li, J. H., Fan, L. F., Zhao, D. J., Zhou, Q., Yao, J. P., Wang, Z. Y., et al. (2021). Plant electrical signals: A multidisciplinary challenge. J. Plant Physiol. 261, 153418. doi: 10.1016/j.jplph.2021.153418
Pachú, J. K. S., Macedo, F. C. O., Malaquias, J. B., Ramalho, F. S., Oliveira, R. F., Godoy, W. A. C., et al. (2021). Electrical signalling and plant response to herbivory: A short review. Plant Signal. Behav. 18, 2277578. doi: 15592324.2023.2277578
Pavlovič, A., Slováková, L., Pandolfi, C., Mancuso, S. (2011). On the mechanism underlying photosynthetic limitation upon trigger hair irritation in the carnivorous plant Venus flytrap (Dionaea muscipula Ellis). J. Exp. Bot. 62, 1991–2000. doi: 10.1093/jxb/erq404
Peña-Cortés, H., Fisahn, J., Willmitzer, L. (1995). Signals involved in wound-induced proteinase inhibitor II gene expression in tomato and potato plants. Proc. Natl. Acad. Sci. U.S.A. 92, 4106–4113. doi: 10.1073/pnas.92.10.4106
Ruban, A. V. (2015). Evolution under the sun: Optimizing light harvesting in photosynthesis. J. Exp. Bot. 66, 7–23. doi: 10.1093/jxb/eru400
Ruban, A. V. (2016). Nonphotochemical chlorophyll fluorescence quenching: Mechanism and effectiveness in protecting plants from photodamage. Plant Physiol. 170, 1903–1916. doi: 10.1104/pp.15.01935
Sukhov, V. (2016). Electrical signals as mechanism of photosynthesis regulation in plants. Photosynth. Res. 130, 373–387. doi: 10.1007/s11120-016-0270-x
Sukhov, V. S., Gaspirovich, V. V., Gromova, E. N., Ladeynova, M. M., Sinitsyna, Y., Berezina, E. V., et al. (2017). Decrease of mesophyll conductance to CO2 is a possible mechanism of abscisic acid influence on photosynthesis in seedlings of pea and wheat. Biochem. Moscow Suppl. Ser. A. 11, 237–247. doi: 10.1134/S1990747817030096
Sukhov, V., Surova, L., Sherstneva, O., Katicheva, L., Vodeneev, V. (2015). Variation potential influence on photosynthetic cyclic electron flow in pea. Front. Plant Sci. 5, 766. doi: 10.3389/fpls.2014.00766
Sukhova, E. M., Yudina, L. M., Sukhov, V. S. (2023). Changes in activity of the plasma membrane H+-ATPase as a link between formation of electrical signals and induction of photosynthetic responses in higher plants. Biochem. (Mosc.). 88, 1488–1503. doi: 10.1134/S0006297923100061
Szechyńska-Hebda, M., Lewandowska, M., Karpiński, S. (2017). Electrical signaling, photosynthesis and systemic acquired acclimation. Front. Physiol. 8, 684. doi: 10.3389/fphys.2017.00684
Szechyńska-Hebda, M., Lewandowska, M., Witoń, D., Fichman, Y., Mittler, R., Karpiński, S. M. (2022). Aboveground plant-to-plant electrical signaling mediates network acquired acclimation. Plant Cell. 34, 3047–3065. doi: 10.1093/plcell/koac150
Tholen, D., Zhu, X.-G. (2011). The mechanistic basis of internal conductance: a theoretical analysis of mesophyll cell photosynthesis and CO2 diffusion. Plant Physiol. 156, 90–105. doi: 10.1104/pp.111.172346
Tikhonov, A. N. (2013). pH-dependent regulation of electron transport and ATP synthesis in chloroplasts. Photosynth. Res. 116, 511–534. doi: 10.1007/s11120-013-9845-y
Tikhonov, A. N. (2014). The cytochrome b6f complex at the crossroad of photosynthetic electron transport pathways. Plant Physiol. Biochem. 81, 163–183. doi: 10.1016/j.plaphy.2013.12.011
Vuralhan-Eckert, J., Lautner, S., Fromm, J. (2018). Effect of simultaneously induced environmental stimuli on electrical signalling and gas exchange in maize plants. J. Plant Physiol. 223, 32–36. doi: 10.1016/j.jplph.2018.02.003
Yudina, L., Gromova, E., Grinberg, M., Popova, A., Sukhova, E., Sukhov, V. (2022). Influence of burning-induced electrical signals on photosynthesis in pea can be modified by soil water shortage. Plants 11, 534. doi: 10.3390/plants11040534
Yudina, L. M., Sherstneva, O. N., Mysyagin, S. A., Vodeneev, V. A., Sukhov, V. S. (2019). Impact of local damage on transpiration of pea leaves at various air humidity. Russ. J. Plant Physiol. 66, 87–94. doi: 10.1134/S1021443719010163
Keywords: electrical signals, photosynthetic activation, photosynthetic inactivation, stomatal CO2 conductance, mesophyll CO2 conductance, terrestrial plants
Citation: Sukhov V (2024) Increasing stomatal CO2 conductance as a potential mechanism of photosynthetic activation by electrical signals in terrestrial plants. Front. Plant Sci. 15:1476175. doi: 10.3389/fpls.2024.1476175
Received: 05 August 2024; Accepted: 21 October 2024;
Published: 07 November 2024.
Edited by:
Roland Valcke, University of Hasselt, BelgiumReviewed by:
Maria M. Borisova-Mubarakshina, Institute of Basic Biological Problems (RAS), RussiaFrantisek Baluska, University of Bonn, Germany
Copyright © 2024 Sukhov. This is an open-access article distributed under the terms of the Creative Commons Attribution License (CC BY). The use, distribution or reproduction in other forums is permitted, provided the original author(s) and the copyright owner(s) are credited and that the original publication in this journal is cited, in accordance with accepted academic practice. No use, distribution or reproduction is permitted which does not comply with these terms.
*Correspondence: Vladimir Sukhov, dnNzdWhAbWFpbC5ydQ==