- 1Facultad de Ciencias, Universidad Católica de la Santísima Concepción, Concepción, Chile
- 2Dirección de Investigación, Vicerrectoría Académica, Universidad de Talca, Talca, Chile
- 3Centro de Ecología Integrativa, Instituto de Ciencias Biológicas, Universidad de Talca, Talca, Chile
- 4Facultad de Ciencias Biológicas, Pontificia Universidad Católica de Chile, Santiago, Chile
Flowering plants produce pollinator rewards such as nectar and pollen, whose quantity and quality usually depend on the whole-plant state under specific environmental conditions. Increasing aridity and temperature linked to climate change may force plants to allocate fewer resources to these traits, potentially disrupting plant-pollinator interactions. In this study, for the first time, both quantitative review (vote-counting procedure) and meta-analytic approach were used to assess the implications of increased temperatures linked to global warming on floral rewards, including nectar (sugar concentration, content, and volume) and pollen (germination and viability), as well as on pollinator visits. Furthermore, we explored whether observed effects of warming are related either to temperature range, plant type (wild vs crop), or study approach (greenhouse vs field experiments). We also assessed the correlations between elevated temperatures and the characteristics that were affected by the temperature range. The results of the vote-counting technique showed that higher temperatures led to a decrease in floral rewards but did not affect the number of pollinator visits. Concurrently, meta-analysis detected adverse effects of warming on pollen germination and viability. Warming effects depended on the plant type for pollen germination and viability, on study approach for nectar sugar concentration and pollen germination, and on temperature range for pollen germination and pollinator visits. Additionally, we found that pollen germination and pollinator visits significantly decreased as temperature range increased. Our results showed that global warming affects floral rewards in both wild and crop plants, providing insights into the effects of changing climatic conditions on plant-pollinator interactions and pollination services.
Introduction
Global surface temperatures have increased by approximately 1.5°C over the last two decades, threatening ecosystem functioning and biodiversity worldwide (IPCC, 2021). Global warming is expected to alter biodiversity and species distributions and disrupt ecological networks, including plant-insect interactions (Parmesan and Yohe, 2003; Kearns et al., 1998; Chen et al., 2011; González-Teuber et al., 2023). Since most flowering plants depend on pollinator assistance for seed set and reproduction, insect pollination emerges as a crucial service for the proper functioning of ecosystems (Ollerton et al., 2011; Brzosko et al., 2021; Sidemo-Holm et al., 2021). Increased temperatures may disrupt this mutualism by modifying floral traits such as morphology, scent, and rewards, affecting pollinator attraction, visits, and behavior (Dormont et al., 2019; Kuppler et al., 2016). Disruption of pollination is likely to have a global impact on the reproductive success of 90% of wild plants and the yields of 85% of major food crops (Klein et al., 2007). While several studies have independently evaluated the impact of increased temperature on the floral traits of some species (see Table 1), there is currently a gap in straightforward and comprehensive multi-species assessments. Conducting such multi-species tests to assess effects of warming on distinct floral traits and pollinator visits can provide valuable insight for more accurate predictions regarding the potential consequences of forecasted climate changes on plant-pollinator interactions, as well as ecosystem pollination service.
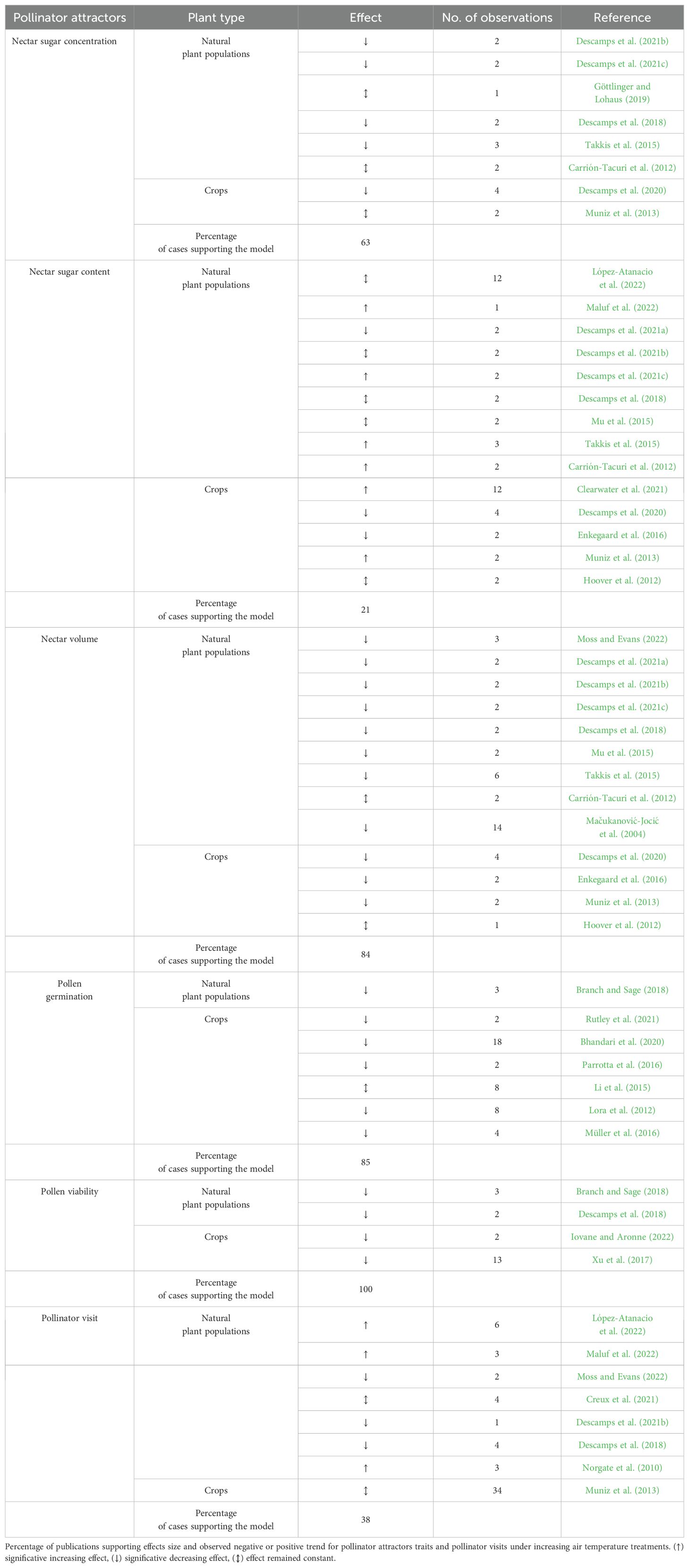
Table 1. Studies used in meta-analysis of response by pollinator attractors and pollinator visits to increasing air temperature including natural plant populations and crops, response trend and number of observations reported in each publication.
Floral rewards such as nectar and pollen play a major role in the acceptance of the flower by pollinators, serving as primary food sources for them (Hegland et al., 2009; Willmer, 2011; Celedón-Neghme et al., 2016; Vaudo et al., 2020, 2024). Nectar is rich in carbohydrates and amino acids (González-Teuber and Heil, 2009a, 2009b), whereas pollen provides essential proteins and lipids (Roulston and Cane, 2000). Higher concentrations of these metabolites usually enhance floral attractiveness of flowers to visiting insects (Somme et al., 2015; Zhao et al., 2016; Descamps et al., 2018). Increased temperatures have been linked to alterations in nectar and pollen (Borghi et al., 2019). Some studies have reported that both nectar volume and sugar content and concentration are negatively impacted by increased temperatures (Descamps et al., 2018, 2020, 2021a, 2021b), although effects appear to be species-dependent (Descamps et al., 2020; Göttlinger and Lohaus, 2019). Similarly, pollen fertilization traits appear to be highly sensitive to elevated temperatures (Raja et al., 2019). Many studies have shown that increased temperatures often lead to pollen abortion and asynchronous pollen and stigma development, negatively affecting pollen viability, germination, and pollen tube growth (Parrotta et al., 2016; Müller and Rieu, 2016). While variations in pollen quality (i.e., nutritional composition) in response to increased temperatures have been little studied, some studies have shown evidence that reductions in protein and starch concentrations contribute to failures in pollen development and viability (Pressman et al., 2002; Sato et al., 2006; Muth et al., 2016), suggesting a link between pollen nutrition and pollen fertilization.
Floral trait modifications may lead to bottom-up effects on flower visitation by pollinators, affecting their foraging behavior, pollen transfer, and, ultimately, plant reproductive success. Although pollinator flower preferences are unequivocally based on nectar and pollen taste, how variations in these floral rewards may alter pollinator behavior in a warming climate remains uncertain (Descamps et al., 2018, 2021a, 2021b). Here, we assembled a global dataset of distinct floral traits (nectar volume, nectar sugar concentration, nectar sugar content, pollen germination, and pollen viability) and pollinator visitation to test how these traits change under increased temperatures in a global warming context. We provide a comprehensive quantitative synthesis and conduct a meta-analysis based on available data in the published literature, examining the effects of experimentally increased temperature on multi-trait displays of flowers in both natural plant populations and crop species. Since floral traits attract pollinators, we hypothesize that reductions in floral rewards (nectar and pollen) linked to climate warming should be accompanied by decreased pollinator visits. Specifically, we initially tested, using the available literature, whether experimentally increased temperatures harm floral rewards and pollinator visits. Subsequently, we assessed how the increase in air temperature influences floral rewards and pollinator visits, considering various explanatory variables (plant type, study approach, and temperature range). Furthermore, we explored relationships between increased air temperature and all evaluated traits impacted by global warming. Relevant implications for pollinator services and recommendations for future research directions are additionally discussed. While there is evidence of floral trait responses to climate change drivers such as drought (see Kuppler and Kotowska, 2021; Jaworski et al., 2022), to our knowledge, this study represents the first direct, comprehensive assessment of the potential effects of global warming on floral rewards and floral-visitor interactions.
Materials and methods
Study selection and data collection
We conducted a search in the Web of Science and Scopus using multiple search term combinations with no restriction on publication years for one of the following flower rewards traits keywords: nectar sugar concentration ‘OR’ nectar sugar content ‘OR’ nectar volume ‘OR’ pollen viability ‘OR’ pollen germination ‘OR’ pollinator visit ‘OR’ plant-pollinator interaction; AND all of following global warming keywords: climate change ‘OR’ global warming ‘OR’ heat stress ‘OR’ high air temperature. Our initial search yielded 1561 publications, which were reviewed to assess their suitability. To be included in the analysis, studies had to inform measurements of floral rewards and/or pollinator visits (i.e., mean value, standard deviation or standard error, and sample size) in control and observational or experimental air temperature increase treatments. To ensure the comparability of studies, we established a maximum range of increased temperature treatment of 12°C. Despite both relating to nectar quality, we distinguished nectar sugar concentration and nectar sugar content. The former refers to amount per gram of tissue, while the latter exclusively refers to amount per tissue (Brzosko et al., 2021; Descamps et al., 2021a; Nicolson, 2022). Studies regarding variations in the nutritional composition of pollen as a consequence of increased temperatures could not be included in the meta-analysis, because of the low number of available studies (Egger et al., 1997). A total of 28 publications met these criteria, and we estimated the percentage of studies verifying the effect of increased air temperature on floral reward traits and pollinator visits (vote-counting procedure; Table 1).
Statistical analysis
To examine the mean effects of experimentally increased temperature (warming) on floral traits and pollinator visits, we calculated Hedge’s effect size (g) using the scalc function from the metafor library (Viechtbauer, 2010) in R environment (R Core Team, 2024). Hedge’s g represents the standardized difference in means between floral traits (and pollinator visits) under increased and non-increased or control temperature conditions. Positive values of g indicate an increase in floral reward traits (or pollinator visit) following warming, whereas negative values signify a decrease. We conducted a random-effects meta-analysis using the rma.mv function for each floral trait, incorporating “species” and “study” as random factors. The former was included to account for variability across plant responses to temperature increases, and the latter to address heterogeneity among study cases (Gurevitch and Hedges, 1999):
M1<–rma.mv(EffectSize, Vi, random = list(~1|study, ~1|species), data = Data)
Heterogeneity tests (QT and QM) were performed to assess effect size homogeneity in each of the six analyses (nectar sugar concentration, nectar sugar content, nectar volume, pollen germination, pollen viability, and pollinator visit). All analyses showed significant heterogeneity (Table 2). To examine sources of variation, we included explanatory variables (i.e., moderators): plant type (wild vs crop), study approach (greenhouse vs field experiments), and increased temperature ranges ([0.99; 4°C], [4.1; 8°C], and [8.1; 12°C]). Categorical random-effects models tested the effects of each moderator on floral reward traits and pollinator visits.
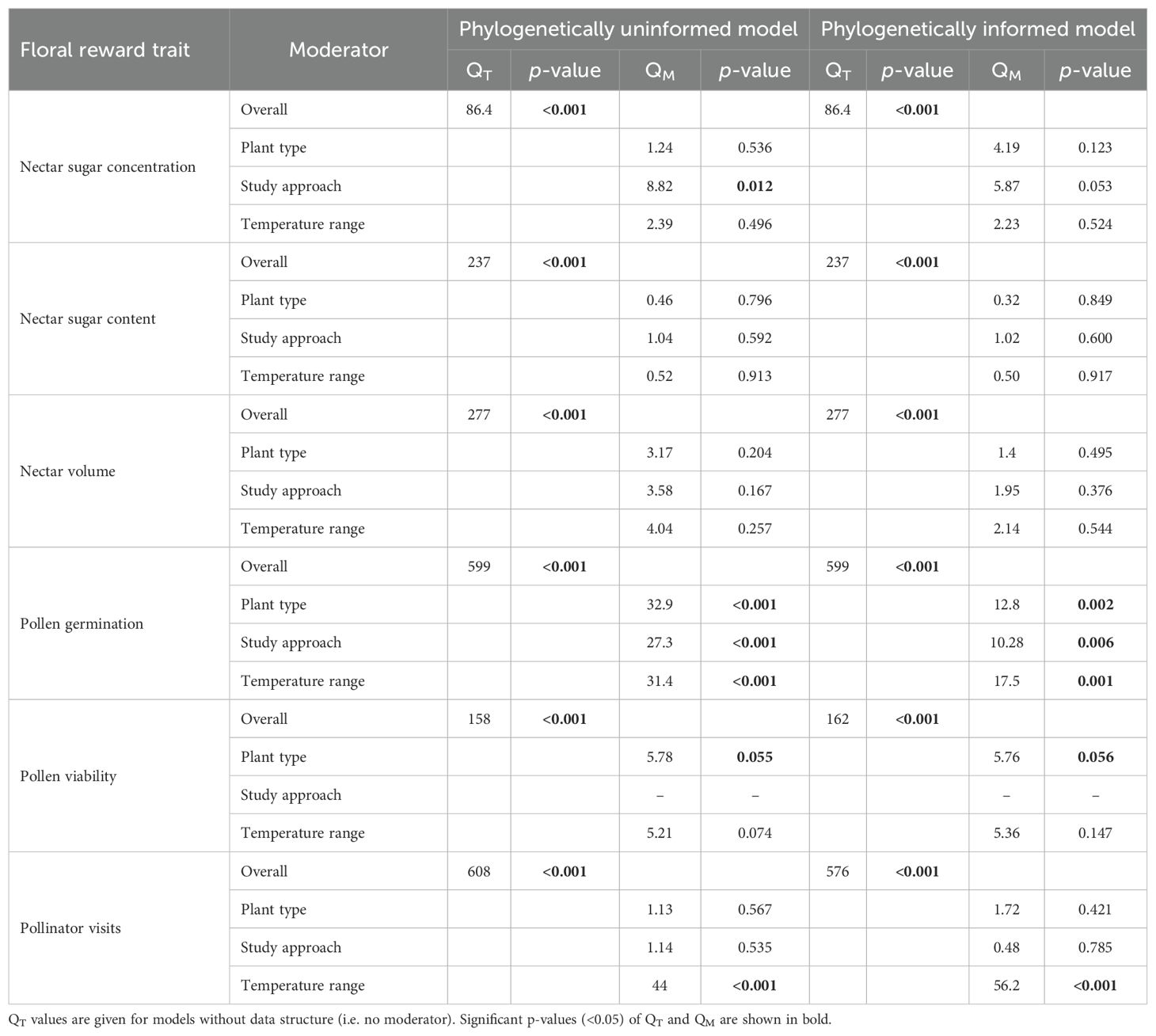
Table 2. Total heterogeneity (QT) and between-group heterogeneity (QM) of man effect sizes (Hedges’ g) in studies comparing floral reward traits in response to increasing air temperature in phylogenetically uninformed and informed models.
Additionally, we carried out linear meta-analysis random models to evaluate the association between the reported increased temperature (as a continuous moderator) and warming-influenced traits. Models included studies as a random factor again, and heterogeneity tests were also implemented. Despite the small number of plant species included in the analysis, we implemented a phylogenetic correction for the overall model by specifying a species phylogenetic correlation matrix in the R argument of the rma.mv function. We constructed a phylogenetic tree of the species in this study based on the megaphylogeny of plants (Jin and Qian, 2023) using the S.Phylomaker function (Jin and Qian, 2022) and phytools package (Revell, 2012). Then, we extracted the correlation matrix for all species using the vcv function from the ape package (Paradis and Schliep, 2019), which applies a Brownian-motion evolution model. In this matrix, closely related species exhibit higher correlations, reflecting their expected similarities. The Egger et al. (1997) test was used to detect publication bias, and a weighted method was applied to calculate the fail-safe number to evaluate whether unpublished data may have affected our conclusions (Rosenberg, 2005).
Results
The literature survey (i.e., vote-counting) found that warming (increased temperature) had detrimental effects on floral reward traits and pollinator visits (65% of cases) (Table 1), considering 31 plant species (Supplementary Table S1). Information about origin of plants species is indicated in Supplementary Table S1. Specifically, warming decreased nectar sugar concentration (63% of cases), nectar sugar content (21% of cases), nectar volume (84% of cases), pollen germination (85% of cases), pollen viability (all cases), and pollinator visit (38% of cases). The meta-analyses showed that warming significantly decreased pollen germination (g = -2.19, p = 0.000) and viability (g = -2.41, p = 0.047), whereas nectar sugar concentration, nectar volume, and even pollinator visits tended to non-significantly reduce (Figure 1). Conversely, nectar sugar content tended to increase due to warming, though not significantly (g = 0.25, p = 0.572). These results were consistent for the phylogenetically uninformed-informed models (Supplementary Figure S1). We did not detect trait phylogenetic signals, which indicate that study species are less related than expected by chance, suggesting that species can be considered as independent samples. Alternatively, the lack of phylogenetic signal can be due to the small size of the phylogeny (Chamberlain et al., 2012). Phylogenetic corrections were found to result in more conservative estimators, likely influenced by the phylogenetic relationships among species, despite the inclusion of 16 distinct plant families in the study.
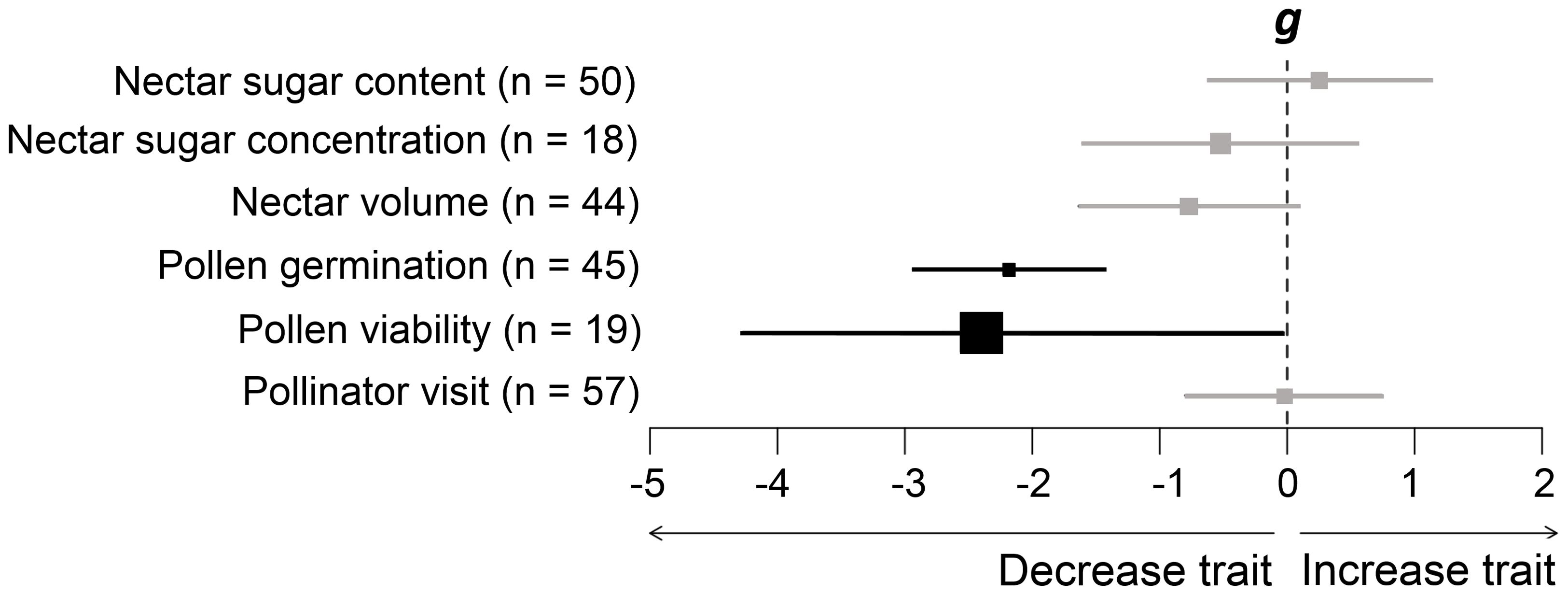
Figure 1. Mean effect sizes (Hedges’ g) of differences in floral reward traits in response to increasing air temperature in phylogenetically uninformed models. Error bars depict 95% confidence intervals (CIs). A mean effect size is significantly different from zero when CIs do not overlap zero. Significant results are shown in black. Negative (or positive) effect sizes indicate a decrease (or increase) in floral reward traits due to warming.
We found that for only some studied traits moderators explained a significant proportion of their variation in Hedges’ effect sizes (g) (Table 2 and Figure 2). While effect sizes of nectar volume and sugar content did not differ significantly by any moderator, we found a significant effect of between-group heterogeneity (QMs) for nectar sugar concentration, pollen germination, pollen viability, and pollinator visits (Table 2). Specifically, nectar sugar concentration was influenced by study approach moderator, showing a significant decrease and increase in g values under greenhouse and field experiments, respectively (Figure 2A). Pollen germination and viability were influenced by temperature range moderator, where g values in crop species consistently decreased, but in wild plant type, they did not change (Figures 2B, C). Although g values in pollinator visits are being influenced by temperature range moderator (Table 2), no significant decrease was observed for any temperature range category (Figure 2D). The g values for pollen germination (z = -4.470, p = < 0.05) and pollinator visits (z = -5.976, p = < 0.05) were observed to significantly decrease as experimental temperature increases (Figures 3A, B).
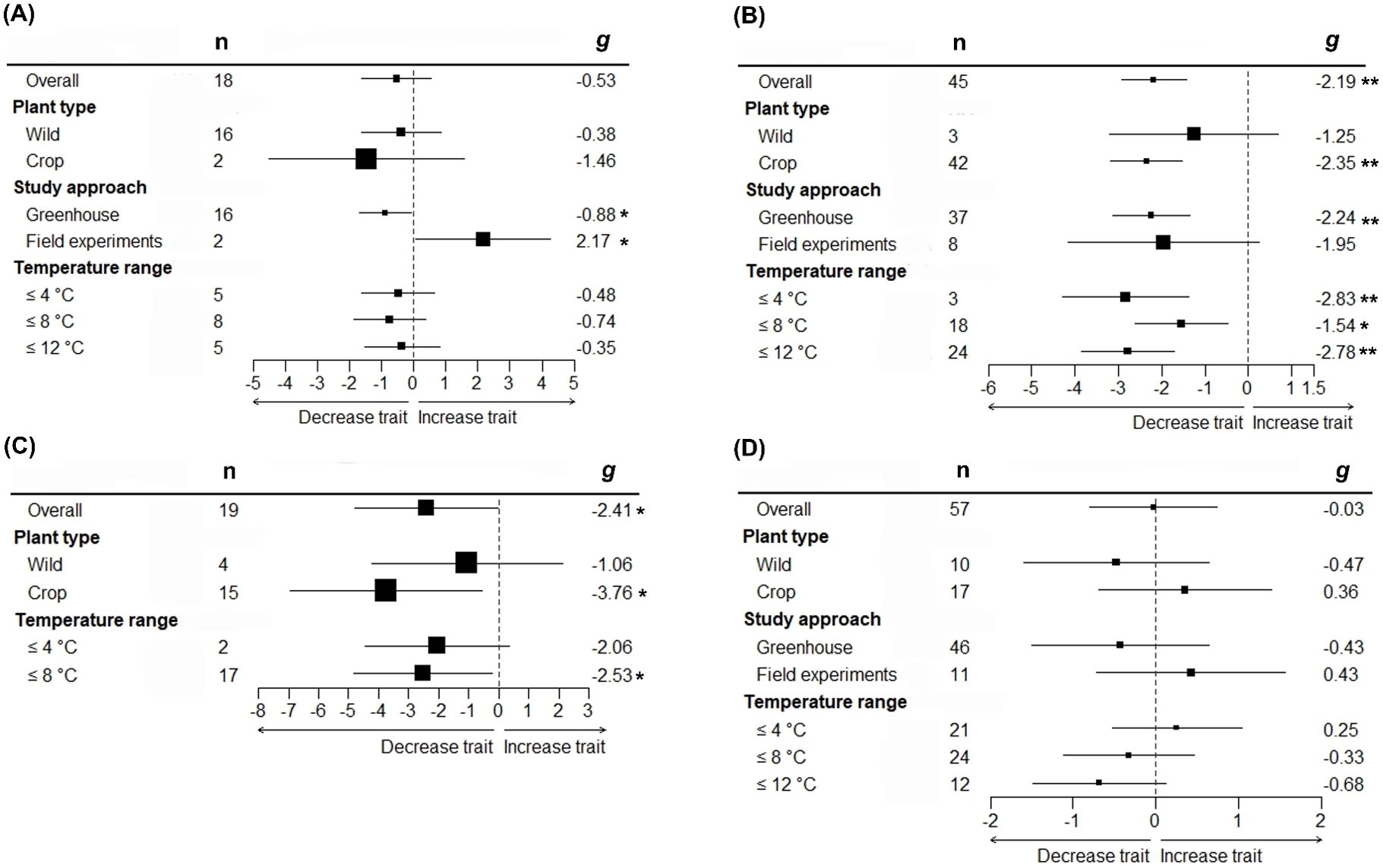
Figure 2. Group-specific mean effect sizes (Hedges’ g) for floral rewards traits in response to increasing air temperature. Nectar sugar concentration (A), Pollen germination (B), pollen viability (C) and pollinator visit (D) phylogenetically uninformed models. Effect size g have been grouped according to plant type, study approach and temperature range. Mean effect size, their 95% confidence interval (CI) and the number of effect sizes (n) for phylogenetically uninformed overall and with moderator models are shown. *P < 0.05; **P < 0.01.
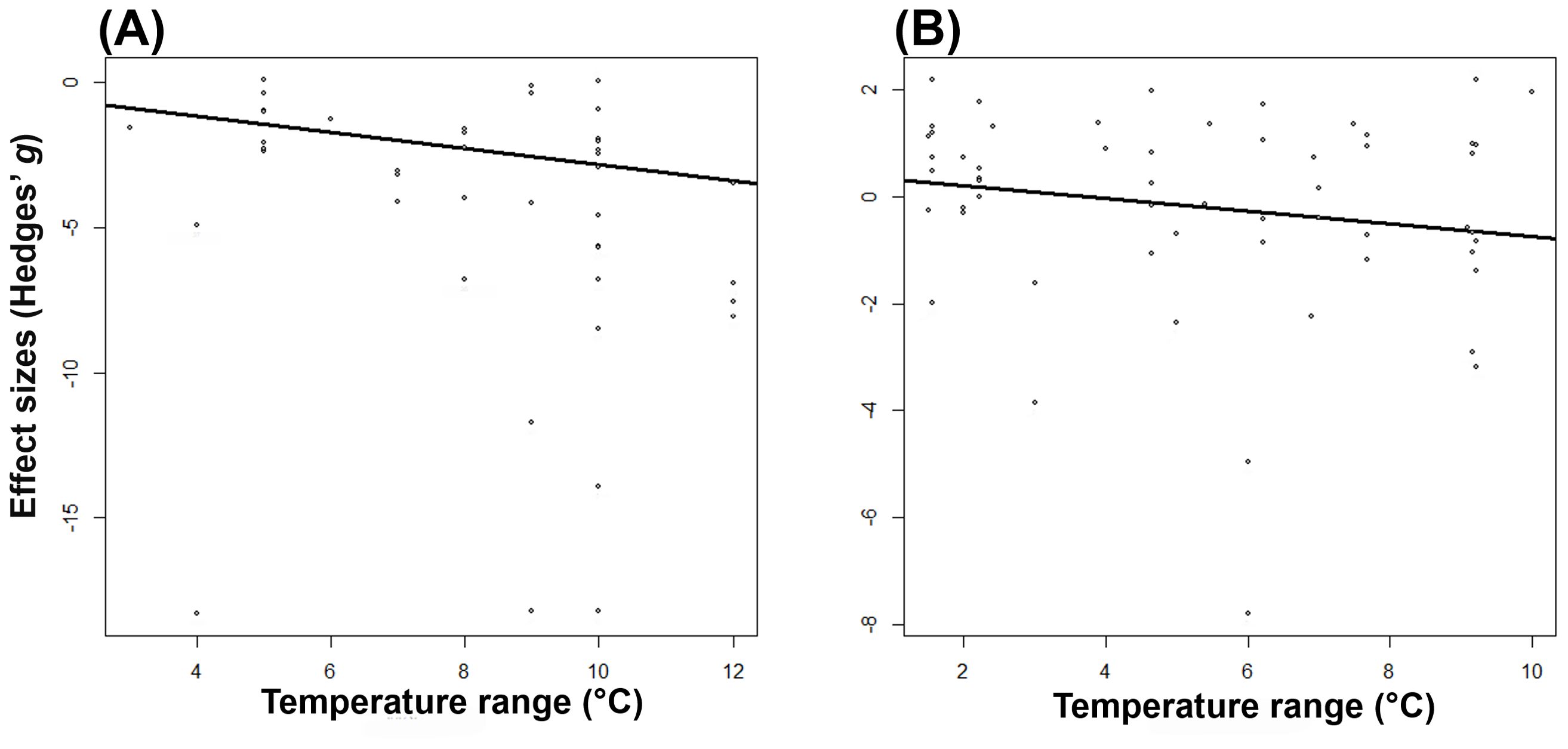
Figure 3. The relationship between effect sizes (Hedges’ g) and increased air temperature of pollen germination (P < 0.05) (A) and pollinator visit (P < 0.05) (B).
Discussion
This study represents the first comprehensive, multi-species examination of the impact of global warming (i.e., increased temperature experiments) on floral rewards, encompassing nectar and pollen-associated traits, and plant-pollinator interactions. A quantitative literature survey, including 31 plant species from tropical and temperate climates, supported detrimental effects of increased temperature on floral reward traits and pollinator visits in 65% of cases. Our meta-analysis revealed that pollen-associated traits were highly sensitive to increased temperature, whereas negative yet non-significant effects of increased temperature were reported on nectar traits (except nectar sugar content) and pollinator visits. Significant heterogeneity in effect size was detected across all analyses, with the primary explanatory factor being the experimental temperature range, followed by plant type (wild vs crop), and lastly, study approach (greenhouse vs field experiment).
Contrary to our expectations, nectar rewards (i.e., nectar sugar content, nectar sugar concentration, and nectar volume) showed no consistent pattern in response to temperature treatments. Significant heterogeneity in effect size, however, was observed for nectar sugar concentration; some of which was explained by the study approach (greenhouse vs field experiments). Nectar sugar concentration showed a significant inverse tendency depending on this moderator, declining in response to temperature increases under controlled conditions, while increasing under field conditions. An earlier meta-analysis of floral trait responses showed similar divergence between controlled- and field experiments, with declines in nectar volume in response to water deficit observed in indoor conditions, but not outdoor conditions (Kuppler and Kotowska, 2021). Even when mechanisms involved in these opposite tendencies between indoor and outdoor conditions are not fully understood, some factors might help to explain this phenomenon. For example, in natural conditions (i.e., field experiments) plants are compelled to invest in nectar production to maintain pollinator interactions, despite potential costs it may involve in stressful environments (Willmer, 2011). This investment is likely unnecessary in indoor conditions in the case that no pollinators consume the nectar. Nectar is a plastic trait, whose secretion may be adjusted according to the intensity of consumption, or, it can be even reabsorbed in the case of non-consumption (Pacini et al., 2003). Furthermore, nectar volume and concentration are highly influenced by environmental factors such as air temperature and humidity. Many of the studies, covered in this meta-analysis, quantified either the nectar volume or its concentration, but not both. The latter might lead to high variations in nectar secretion measurements, since both must be considered together in order to effectively calculate the realistic amounts of secreted soluble solids (Heil, 2011). While nectar variation might be an inherent limitation across studies, the use of a rigorous statistical meta-analytic framework has allowed us to have a reliable estimation of the effects of increased temperatures on nectar traits.
Our results supported the detrimental effects of increased temperature on both pollen germination and viability; nevertheless, declines in pollen germination and viability were only observed for crop species, but not for wild species (Figure 2). Numerous studies have shown that crops are highly sensitive to temperature increases, particularly during reproductive phases (Driedonks et al., 2016). Crop plants are more sensitive to abiotic stresses than their wild-type relatives because breeding selection for yield is not necessarily linked to an adaptive stress tolerance strategy (Villalobos-López et al., 2022).
Pollen germination and viability usually have a direct influence on seed set and fruit set ratio. Even when impacts of increased temperatures on seed and/or fruit set were not included in this meta-analysis, numerous studies have reported that negative effects on pollen viability and germination due to higher temperatures are accompanied by declines in seed set (Powell et al., 2012; Tolessa and Heuvelink, 2018; Yang et al., 2019; Shenoda et al., 2021). Given that 75% of all food crop species rely on insect pollination for seed set (Klein et al., 2007), higher temperatures potentially represent a threat to yields and food security. Pollen germination and viability were significantly reduced by temperature range (Figures 2B, C; 3A), with the former more sensitive to temperature increases than the latter. Whereas pollen germination was negatively affected by all temperature increments (Figure 2B), pollen viability was only negatively affected by temperatures over 8°C (Figure 2C). Development and functioning of the male gametophyte (or pollen) are known to be the most temperature-sensitive processes within the plant life cycle (Zinn et al., 2010). Furthermore, both pollen germination and viability are highly sensitive at even short periods of high temperature exposure (Müller et al., 2016). Thus, differences observed in the temperature-sensitive range between pollen germination and viability might be explained by genetic variation for thermotolerance among plant species included in the current study.
The results from our meta-analysis and literature survey did not align with the prediction of detrimental effects on pollinator visits due to warming. Specifically, the meta-analysis showed that temperature increases did not significantly affect pollinator visits. Likewise, the literature survey only recorded 38% support for our prediction. Although the high heterogeneity of effect sizes could certainly be a contributing factor, results might be at least partially explained by the temperature range (Table 1; Figure 3B). There was a clear trend of decreasing pollinator visitation with increasing temperature (Figure 3B). It is known that elevated temperatures can influence pollinator activity both directly and indirectly (Descamps et al., 2018). Whereas direct effects are mainly associated with changes in pollinator activity and flower-visiting behavior (Descamps et al., 2018), indirect ones (i.e., those mediated via at least one other interacting species) may result from changes in floral signals and rewards (Knauer and Schiestl, 2015; Descamps et al., 2018). Insect pollinators are typically only active within certain temperature limits, becoming inactive at temperatures above and below these thresholds (Corbet et al., 1993; Kühsel and Blüthgen, 2015; Plos et al., 2023). Moreover, since pollinators rely exclusively on floral rewards for food (Hegland et al., 2009; Willmer, 2011), variations in the quantity and quality of nectar and pollen are expected to lead to changes in pollinator-visiting behavior; and thereby, may influence plant reproduction (Robertson et al., 1999; Konzmann and Lunau, 2014; Descamps et al., 2018; Plos et al., 2023). In our study, temperature effects on pollinator visits were not differentiated based on either direct or indirect effects (information not provided in the studies). Since both types of effects may interact (Kharouba et al., 2018; Kharouba and Yang, 2021; Freimuth et al., 2022), future research should consider both in order to determine the overall outcome of climate change impacts on species interactions.
Our meta-analysis revealed that increased temperatures affect floral rewards, particularly pollen traits, which likely scale up to pollinator interactions. Climate models predict that the global mean temperature will increase by 1-4°C by the end of the twenty-first century (IPCC, 2021). Nevertheless, most detrimental effects on plants seem to be caused by heat waves or extreme temperature events (López et al., 2022), which are projected to increase in both intensity and frequency (Meehl et al., 2007). Heat waves are predicted to have short-term durations of a few days, but with an increase in temperatures of over 5°C (IPCC, 2021). Heat-induced pollen damage is related to reductions in seed and fruit set. Therefore, understanding how floral traits, particularly pollen development, respond to extreme weather events is key to predicting how reproduction in natural plant populations and agricultural systems may be affected by climate change. Moreover, if heat-related shifts in pollen traits are likely associated with a decline in pollinator visits, this scenario may be much more serious. A recent meta-analysis reported that without pollinators, half of all the flowering plants would suffer a decline in fertility of over 80%, while a third would not produce seeds at all (Rodger et al., 2021). While ecological consequences of other climate change-associated factors such as drought on floral traits and plant-pollinator interactions are relatively well established, to our knowledge, this is the first meta-analytic approach to assess potential effects of global warming on floral rewards and floral-visitor interactions. Elevated temperatures, however, are usually accompanied by increased risk of a range of other abiotic stresses (such as drought and light intensity); therefore, future studies should explore combinations of these factors to improve understanding of climate change effects on floral metabolism and plant-pollinator interactions.
Data availability statement
The raw data supporting the conclusions of this article will be made available by the authors, without undue reservation.
Author contributions
SA-R: Investigation, Methodology, Writing – original draft. VE: Methodology, Writing – original draft. MG-T: Supervision, Writing – review & editing.
Funding
The author(s) declare financial support was received for the research, authorship, and/or publication of this article. SA-R was funded by ANID-Subdirección de Capital Humano/Doctorado Nacional/2021-21210677.
Acknowledgments
SA-R is grateful to ANID-Subdirección de Capital Humano/Doctorado Nacional/2021- 21210677. MG-T acknowledges Fondecyt Regular grant N° 1230282.
Conflict of interest
The authors declare that the research was conducted in the absence of any commercial or financial relationships that could be construed as a potential conflict of interest.
Publisher’s note
All claims expressed in this article are solely those of the authors and do not necessarily represent those of their affiliated organizations, or those of the publisher, the editors and the reviewers. Any product that may be evaluated in this article, or claim that may be made by its manufacturer, is not guaranteed or endorsed by the publisher.
Supplementary material
The Supplementary Material for this article can be found online at: https://www.frontiersin.org/articles/10.3389/fpls.2024.1448070/full#supplementary-material
References
Bhandari, K., Sita, K., Sehgal, A., Bhardwaj, A., Gaur, P., Kumar, S., et al. (2020). Differential heat sensitivity of two cool-season legumes, chickpea and lentil, at the reproductive stage, is associated with responses in pollen function, photosynthetic ability and oxidative damage. J. Agron. Crop Sci. 206, 734–758. doi: 10.1111/jac.12433
Borghi, M., Perez de Souza, L., Yoshida, T., Fernie, A. R. (2019). Flowers and climate change: a metabolic perspective. New Phytol. 224, 1425–1441. doi: 10.1111/nph.16031
Branch, H. A., Sage, R. F. (2018). Reproductive heat tolerance in a Mojave Desert annual plant, Trianthema portulacastrum. Am. J. Bot. 105, 2018–2024. doi: 10.1002/ajb2.1199
Brzosko, E., Bajguz, A., Burzyńska, J., Chmur, M. (2021). Nectar chemistry or flower morphology—What is more important for the reproductive success of generalist Orchid Epipactis palustris in natural and anthropogenic populations? Int. J. Mol. Sci. 22, 12164. doi: 10.3390/ijms222212164
Carrión-Tacuri, J., Berjano, R., Guerrero, G., Figueroa, M. E., Tye, A., Castillo, J. M. (2012). Nectar production by invasive Lantana camara and endemic L. peduncularis in the Galápagos Islands. Pac. Sci. 66, 435–445. doi: 10.2984/66.4.2
Celedón-Neghme, C., Santamaría, L., González-Teuber, M. (2016). The role of pollination drops in animal pollination in the Mediterranean gymnosperm Ephedra fragilis (Gnetales). Plant Ecol. 217, 1545–1552. doi: 10.1007/s11258-016-0667-9
Chamberlain, S. A., Hovick, S. M., Dibble, C. J., Rasmussen, N. L., Van Allen, B. G., Maitner, B. S., et al. (2012). Does phylogeny matter? Assessing the impact of phylogenetic information in ecological meta-analysis. Ecol. Lett. 15, 627–636. doi: 10.1111/j.1461-0248.2012.01776.x
Chen, I. C., Hill, J. K., Ohlemüller, R., Roy, D. B., Thomas, C. D. (2011). Rapid range shifts of species associated with high levels of climate warming. Science 333, 1024–1026. doi: 10.1126/science.1206432
Clearwater, M. J., Noe, S. T., Manley-Harris, M., Truman, G., Gardyne, S., Murray, J., et al. (2021). Nectary photosynthesis contributes to the production of mānuka (Leptospermum scoparium) floral nectar. New Phytol. 232, 1703–1717. doi: 10.1111/nph.17632
Corbet, S. A., Fussell, M., Ake, R., Fraser, A., Gunson, C., Savage, A., et al. (1993). Temperature and the pollinating activity of social bees. Ecol. Entomol 18, 17–30. doi: 10.1111/j.1365-2311.1993.tb01075.x
Creux, N. M., Brown, E. A., Garner, A. G., Saeed, S., Scher, C. L., Holalu, S. V., et al. (2021). Flower orientation influences floral temperature, pollinator visits and plant fitness. New Phytol. 232, 868–879. doi: 10.1111/nph.17627
Descamps, C., Boubnan, N., Jacquemart, A. L., Quinet, M. (2021a). Growing and flowering in a changing climate: effects of higher temperatures and drought stress on the bee-pollinated species Impatiens glandulifera Royle. Plants 10, 988. doi: 10.3390/plants10050988
Descamps, C., Jambrek, A., Quinet, M., Jacquemart, A. L. (2021b). Warm temperatures reduce flower attractiveness and bumblebee foraging. Insects 12, 493. doi: 10.3390/insects12060493
Descamps, C., Marée, S., Hugon, S., Quinet, M., Jacquemart, A. L. (2020). Species-specific responses to combined water stress and increasing temperatures in two bee-pollinated congeners (Echium, Boraginaceae). Ecol. Evol. 10, 6549–6561. doi: 10.1002/ece3.6389
Descamps, C., Quinet, M., Baijot, A., Jacquemart, A. (2018). Temperature and water stress affect plant-pollinator interactions in Borago officinalis (Boraginaceae). Ecol. Evol. 8, 3443–3456. doi: 10.1002/ece3.3914
Descamps, C., Quinet, M., Jacquemart, A. L. (2021c). Climate change-induced stress reduce quantity and alter composition of nectar and pollen from a bee-pollinated species (Borago officinalis, Boraginaceae). Front. Plant Sci. 12. doi: 10.3389/fpls.2021.755843
Dormont, L., Joffard, N., Schatz, B. (2019). Intraspecific variation in floral color and odor in Orchids. Int. J. Plant Sci. 180, 1036–1058. doi: 10.1086/705589
Driedonks, N., Rieu, I., Vriezen, W. H. (2016). Breeding for plant heat tolerance at vegetative and reproductive stages. Plant Reprod. 29, 67–79. doi: 10.1007/s00497-016-0275-9
Egger, M., Davey Smith, G., Schneider, M., Minder, C. (1997). Bias in meta-analysis detected by a simple, graphical test. Br. Med. J. 315, 629–634. doi: 10.1136/bmj.315.7109.629
Enkegaard, A., Kryger, P., Boelt, B. (2016). Determinants of nectar production in oilseed rape. J. Apic. Res. 55, 89–99. doi: 10.1080/00218839.2016.1192341
Freimuth, J., Bossdorf, O., Scheepens, J. F., Willems, F. M. (2022). Climate warming changes synchrony of plants and pollinators. Proc. R. Soc B 289, 20212142. doi: 10.1098/rspb.2021.2142
González-Teuber, M., Heil, M. (2009a). Nectar chemistry is tailored for both attraction of mutualists and protection from exploiters. Plant Signal. Behav. 4, 809–813. doi: 10.4161/psb.4.9.9393
González-Teuber, M., Heil, M. (2009b). The role of extrafloral nectar amino acids for the preferences of facultative and obligate ant mutualists. J. Chem. Ecol. 35, 459–468. doi: 10.1007/s10886-009-9618-4
González-Teuber, M., Palma-Onetto, V., Aguirre, C., Ibáñez, A. J., Mithöfer, A. (2023). Climate change-related warming-induced shifts in leaf chemical traits favor nutrition of the specialist herbivore Battus polydamas archidamas. Front. Ecol. Evol. 11. doi: 10.3389/fevo.2023.1152489
Göttlinger, T., Lohaus, G. (2019). Influence of light, dark, temperature and drought on metabolite and ion composition in nectar and nectaries of an epiphytic bromeliad species (Aechmea fasciata). Plant Biol. 22, 781–793. doi: 10.1111/plb.13150
Gurevitch, J., Hedges, L. V. (1999). Statistical issues in ecological meta-analyses. Ecology 80, 1142–1149. doi: 10.1890/0012-9658(1999)080[1142:siiema]2.0.co;2
Hegland, S. J., Nielsen, A., Lázaro, A., Bjerknes, A. L., Totland, Ø. (2009). How does climate warming affect plant-pollinator interactions? Ecol. Lett 12, 184–195. doi: 10.1111/j.1461-0248.2008.01269.x
Heil, M. (2011). Nectar: generation, regulation and ecological functions. Trends Plant Sci. 16, 191–200. doi: 10.1016/j.tplants.2011.01.003
Hoover, S. E. R., Ladley, J. J., Shchepetkina, A. A., Tisch, M., Gieseg, S. P., Tylianakis, J. M. (2012). Warming, CO2, and nitrogen deposition interactively affect a plant-pollinator mutualism. Ecol. Lett. 15, 227–234. doi: 10.1111/j.1461-0248.2011.01729.x
Iovane, M., Aronne, G. (2022). High temperatures during microsporogenesis fatally shorten pollen lifespan. Plant Reprod. 35, 9–17. doi: 10.1007/s00497-021-00425-0
IPCC (2021). Climate Change 2021: The Physical Science Basis. Contribution of Working Group I to the Sixth Assessment Report of the Intergovernmental Panel on Climate Change. Eds. Masson-Delmotte, V., Zhai, P., Pirani, A., Connors, S. L., Péan, C., Berger, S., Caud, N., Chen, Y., Goldfarb, L., Gomis, M. I., Huang, M., Leitzell, K., Lonnoy, E., Matthews, J. B. R., Maycock, T. K., Waterfield, T., Yelekçi, O., Yu, R., Zhou, B. (New York, USA: Cambridge Univ. Press, Cambridge, United Kingdom). doi: 10.1017/9781009157896
Jaworski, C. C., Geslin, B., Zakardjian, M., Lecareux, C., Caillault, P., Nève, G., et al. (2022). Long-term experimental drought alters floral scent and pollinator visits in a Mediterranean plant community despite overall limited impacts on plant phenotype and reproduction. J. Ecol. 110, 2628–2648. doi: 10.1111/1365-2745.13974
Jin, Y., Qian, H. (2022). V.PhyloMaker2: An updated and enlarged R package that can generate very large phylogenies for vascular plants. Plant Divers. 44, 335–339. doi: 10.1016/j.pld.2022.05.005
Jin, Y., Qian, H. (2023). U.PhyloMaker: An R package that can generate large phylogenetic trees for plants and animals. Plant Divers. 45, 347–352. doi: 10.1016/j.pld.2022.12.007
Kearns, C. A., Inouye, D. W., Waser, N. M. (1998). Endangered mutualisms: the conservation of plant-pollinator interactions. Annu. Rev. Ecol. Evol. Syst. 29, 83–112. doi: 10.1146/annurev.ecolsys.29.1.83
Kharouba, H. M., Ehrlén, J., Gelman, A., Bolmgren, K., Allen, J. M., Travers, S. E., et al. (2018). Global shifts in the phenological synchrony of species interactions over recent decades. Proc. Natl. Acad. Sci. 115, 5211–5216. doi: 10.1073/pnas.1714511115
Kharouba, H. M., Yang, L. H. (2021). Disentangling the direct, indirect, and combined effects of experimental warming on a plant–insect herbivore interaction. Ecosphere 12, e03778. doi: 10.1002/ecs2.3778
Klein, A. M., Vaissière, B. E., Cane, J. H., Steffan-Dewenter, I., Cunningham, S. A., Kremen, C., et al. (2007). Importance of pollinators in changing landscapes for world crops. Proc. R. Soc B 274, 303–313. doi: 10.1098/rspb.2006.3721
Knauer, A. C., Schiestl, F. P. (2015). Bees use honest floral signals as indicators of reward when visiting flowers. Ecol. Lett. 18, 135–143. doi: 10.1111/ele.12386
Konzmann, S., Lunau, K. (2014). Divergent rules for pollen and nectar foraging bumblebees – A laboratory study with artificial flowers offering diluted nectar substitute and pollen surrogate. PloS One 9, e91900. doi: 10.1371/journal.pone.0091900
Kühsel, S., Blüthgen, N. (2015). High diversity stabilizes the thermal resilience of pollinator communities in intensively managed grasslands. Nat. Commun. 6, 7989. doi: 10.1038/ncomms8989
Kuppler, J., Höfers, M. K., Wiesmann, L., Junker, R. R. (2016). Time-invariant differences between plant individuals in interactions with arthropods correlate with intraspecific variation in plant phenology, morphology and floral scent. New Phytol. 210, 1357–1368. doi: 10.1111/nph.13858
Kuppler, J., Kotowska, M. M. (2021). A meta-analysis of responses in floral traits and flower–visitor interactions to water deficit. Glob. Change Biol. 27, 3095–3108. doi: 10.1111/gcb.15621
Li, X., Lawas, M. F., Malo, R., Glaubitz, U., Erban, A., Mauleon, R., et al. (2015). Metabolic and transcriptomic signatures of rice floral organs reveal sugar starvation as a factor in reproductive failure under heat and drought stress. Plant Cell Environ. 38, 2171–2192. doi: 10.1111/pce.12545
López, R., Ramírez-Valiente, J. A., Pita, P. (2022). How plants cope with heatwaves in a drier environment. Flora 295, 152148. doi: 10.1016/j.flora.2022.152148
López-Atanacio, M., Lucas-García, R., Rosas-Guerrero, V., Alemán-Figueroa, L., Kuk-Dzul, J. G., Hernández-Flores, G. (2022). Seasonal variation in the response of a monoecious crop to increased temperature and fertilizers. Front. Plant Sci. 13. doi: 10.3389/fpls.2022.1012859
Lora, J., Herrero, M., Hormaza, J. I. (2012). Pollen performance, cell number, and physiological state in the early-divergent angiosperm Annona cherimola Mill. (Annonaceae) are related to environmental conditions during the final stages of pollen development. Sex Plant Reprod. 25, 157–167. doi: 10.1007/s00497-012-0187-2
Mačukanović-Jocić, M., Duletić-Laušević, S., Jocić, G. (2004). Nectar production in three melliferous species of Lamiaceae in natural and experimental conditions. Acta Vet. 54, 475–487. doi: 10.2298/AVB0406475M
Maluf, R. P., Alzate-Marin, A. L., Silva, C. C., Pansarin, L. M., Bonifácio-Anacleto, F., Schuster, I., et al. (2022). Warming and soil water availability affect plant–flower visitor interactions for Stylosanthes capitata, a tropical forage legume. Sci. Total Environ. 817, 152982. doi: 10.1016/j.scitotenv.2022.152982
Meehl, G., Stocker, T., Collins, W., Friedlingstein, P., Gaye, A., Gregory, J. (2007). Contribution of working group I to the Fourth Assessment Report of the Intergovernmental Panel on Climate Change in: Global climate projections. in: Global climate projections. -In: Climate Change 2007: The Physical Science Basis. (Cambridge Univ. Press, Cambridge, UK), 976 pp.
Moss, E. D., Evans, D. M. (2022). Experimental climate warming reduces floral resources and alters insect visitation and wildflower seed set in a cereal Agro-ecosystem. Front. Plant Sci. 13. doi: 10.3389/fpls.2022.826205
Mu, J., Peng, Y., Xi, X., Wu, X., Li, G., Niklas, K. J., et al. (2015). Artificial asymmetric warming reduces nectar yield in a Tibetan alpine species of Asteraceae. Ann. Bot. 116, 899–906. doi: 10.1093/aob/mcv042
Müller, F., Rieu, I. (2016). Acclimation to high temperature during pollen development. Plant Reprod. 29, 107–118. doi: 10.1007/s00497-016-0282-x
Müller, F., Xu, J., Kristensen, L., Wolters-Arts, M., de Groot, F. M., Jansma, S. Y., et al. (2016). High-temperature-induced defects in Tomato (Solanum lycopersicum) anther and pollen development are associated with reduced expression of B-class floral patterning genes. PloS One 11, e0167614. doi: 10.1371/journal.pone.0167614
Muniz, J. M., Pereira, A. L. C., Valim, J. O. S., Campos, W. G. (2013). Patterns and mechanisms of temporal resource partitioning among bee species visiting basil (Ocimum basilicum) flowers. Arthropod Plant Interac 7, 491–502. doi: 10.1007/s11829-013-9271-2
Muth, F., Francis, J. S., Leonard, A. S. (2016). Bees use the taste of pollen to determine which flowers to visit. Biol. Lett. 12, 20160356. doi: 10.1098/rsbl.2016.0356
Nicolson, S. W. (2022). Sweet solutions: nectar chemistry and quality. Phil. Trans. R. Soc B 377, 20210163. doi: 10.1098/rstb.2021.0163
Norgate, M., Boyd-Gerny, S., Simonov, V., Rosa, M. G. P., Heard, T. A., Dyer, A. G. (2010). Ambient temperature influences Australian native stingless bee (Trigona carbonaria) preference for warm nectar. PloS One 5, e12000. doi: 10.1371/journal.pone.0012000
Ollerton, J., Winfree, R., Tarrant, S. (2011). How many flowering plants are pollinated by animals? Oikos 120, 321–326. doi: 10.1111/j.1600-0706.2010.18644.x
Pacini, E., Nepi, M., Vesprini, J. (2003). Nectar biodiversity: a short review. Pl. Syst. Evol. 238, 7–21. doi: 10.1007/s00606-002-0277-y
Paradis, E., Schliep, K. (2019). ape 5.0: an environment for modern phylogenetics and evolutionary analyses in R. Bioinformatics 35, 526–528. doi: 10.1093/bioinformatics/bty633
Parmesan, C., Yohe, G. (2003). A globally coherent fingerprint of climate change impacts across natural systems. Nature 421, 37–42. doi: 10.1038/nature01286
Parrotta, L., Faleri, C., Cresti, M., Cai, G. (2016). Heat stress affects the cytoskeleton and the delivery of sucrose synthase in tobacco pollen tubes. Planta 243, 43–63. doi: 10.1007/s00425-015-2394-1
Plos, C., Stelbrink, N., Römermann, C., Knight, T. M., Hensen, I. (2023). Abiotic conditions affect nectar properties and flower visitation in four herbaceous plant species. Flora 303, 152279. doi: 10.1016/j.flora.2023.152279
Powell, N., Ji, X., Ravash, R., Edlington, J., Dolferus, R. (2012). Yield stability for cereals in a changing climate. Funct. Plant Biol. 39, 539–552. doi: 10.1071/fp12078
Pressman, E., Peet, M. M., Pharr, D. M. (2002). The effect of heat stress on Tomato pollen characteristics is associated with changes in carbohydrate concentration in the developing anthers. Ann. Bot. 90, 631–636. doi: 10.1093/aob/mcf240
Raja, M. M., Vijayalakshmi, G., Naik, M. L., Basha, P. O., Sergeant, K., Hausman, J. F., et al. (2019). Pollen development and function under heat stress: from effects to responses. Acta Physiol. Plant 41, 47. doi: 10.1007/s11738-019-2835-8
R Core Team (2024). R: A Language and Environment for Statistical Computing (Vienna, Austria: R Foundation for Statistical Computing). Available at: https://www.R-project.org/.
Revell, L. J. (2012). phytools: an R package for phylogenetic comparative biology (and other things). Methods Ecol. Evol. 3, 217–223. doi: 10.1111/j.2041-210X.2011.00169.x
Robertson, A. W., Mountjoy, C., Faulkner, B. E., Roberts, M. V., Macnair, M. R. (1999). Bumble bee selection of Mimulus Guttatus flowers: the effects of pollen quality and reward depletion. Ecology 80, 2594–2606. doi: 10.1890/0012-9658(1999)080[2594:BBSOMG]2.0.CO;2
Rodger, J. G., Bennett, J. M., Razanajatovo, M., Knight, T. M., van Kleunen, M., Ashman, T. L., et al. (2021). Widespread vulnerability of flowering plant seed production to pollinator declines. Sci. Adv. 7, eabd3524. doi: 10.1126/sciadv.abd3524
Rosenberg, M. S. (2005). The file-drawer problem revisited: a general weighted method for calculating fail-safe numbers in meta-analysis. Evolution 59, 464–468. doi: 10.1554/04-602
Roulston, T. H., Cane, J. H. (2000). Pollen nutritional content and digestibility for animals. Plant Syst. Evol. 222, 187–209. doi: 10.1007/BF00984102
Rutley, N., Miller, G., Wang, F., Harper, J. F., Miller, G., Lieberman-Lazarovich, M. (2021). Enhanced reproductive thermotolerance of the tomato high pigment 2 mutant is associated with increased accumulation of flavonols in pollen. Front. Plant Sci. 12. doi: 10.3389/fpls.2021.672368
Sato, S., Kamiyama, M., Iwata, T., Makita, N., Furukawa, H., Ikeda, H. (2006). Moderate increase of mean daily temperature adversely affects fruit set of Lycopersicon esculentum by disrupting specific physiological processes in male reproductive development. Ann. Bot. 97, 731–738. doi: 10.1093/aob/mcl037
Shenoda, J. E., Sanad, M. N. M. E., Rizkalla, A. A., El-Assal, S., Ali, R. T., Hussein, M. H. (2021). Effect of long-term heat stress on grain yield, pollen grain viability and germinability in bread wheat (Triticum aestivum L.) under field conditions. Heliyon 7, e07096. doi: 10.1016/j.heliyon.2021.e07096
Sidemo-Holm, W., Carrié, R., Ekroos, J., Lindström, S. A. M., Smith, H. G. (2021). Reduced crop density increases floral resources to pollinators without affecting crop yield in organic and conventional fields. J. Appl. Ecol. 58, 1421–1430. doi: 10.1111/1365-2664.13887
Somme, L., Vanderplanck, M., Michez, D., Lombaerde, I., Moerman, R., Wathelet, B., et al. (2015). Pollen and nectar quality drive the major and minor floral choices of bumble bees. Apidologie 46, 92–106. doi: 10.1007/s13592-014-0307-0
Takkis, K., Tscheulin, T., Tsalkatis, P., Petanidou, T. (2015). Climate change reduces nectar secretion in two common Mediterranean plants. AoB Plants 7, plv111. doi: 10.1093/aobpla/plv111
Tolessa, K., Heuvelink, E. (2018). Pollen viability and fruit set of tomato introgression lines (Solanum esculentum L. chmielewskii) at moderately high temperature regimes. World Appl. Sci. J. 36, 29–38. doi: 10.5829/idosi.wasj.2018.29.38
Vaudo, A. D., Dyer, L. A., Leonard, A. S. (2024). Pollen nutrition structures bee and plant community interactions. Pro. Natl. Acad. Sci. 121, e2317228120. doi: 10.1073/pnas.2317228120
Vaudo, A. D., Tooker, J. F., Patch, H. M., Biddinger, D. J., Coccia, M., Crone, M. K., et al. (2020). Pollen Protein: lipid macronutrient ratios may guide broad patterns of Bee species floral preferences. Insects 11, 132. doi: 10.3390/insects11020132
Viechtbauer, W. (2010). Conducting meta-analyses in R with the metafor package. J. Stat. Softw 36, 1–48. doi: 10.18637/jss.v036.i03
Villalobos-López, M. A., Arroyo-Becerra, A., Quintero-Jiménez, A., Iturriaga, G. (2022). Biotechnological advances to improve abiotic stress tolerance in crops. Int. J. Mol. Sci. 23, 12053. doi: 10.3390/ijms231912053
Willmer, P. (2011). Pollination and Floral Ecology (Princeton Univer. Press, Princeton, NJ). doi: 10.23943/princeton/9780691128610.001.0001
Xu, J., Wolters-Arts, M., Mariani, C., Huber, H., Rieu, I. (2017). Heat stress affects vegetative and reproductive performance and trait correlations in tomato (Solanum lycopersicum). Euphytica 213, 156. doi: 10.1007/s10681-017-1949-6
Yang, Q., Liu, E., Fu, Y., Yuan, F., Zhang, T., Peng, S. (2019). High temperatures during flowering reduce fruit set in rabbiteye blueberry. J. Amer. Soc Hort 144, 339–351. doi: 10.21273/JASHS04650-19
Zhao, Z., Lu, N., Conner, J. K. (2016). Adaptive pattern of nectar volume within inflorescences: bumblebee foraging behavior and pollinator-mediated natural selection. Sci. Rep. 6, 34499. doi: 10.1038/srep34499
Keywords: climate change, warming, floral rewards, insect pollinators, nectar, pollen
Citation: Alquichire-Rojas S, Escobedo VM and González-Teuber M (2024) Impacts of increased temperatures on floral rewards and pollinator interactions: a meta-analysis. Front. Plant Sci. 15:1448070. doi: 10.3389/fpls.2024.1448070
Received: 12 June 2024; Accepted: 11 October 2024;
Published: 08 November 2024.
Edited by:
Adam P. Karremans, University of Costa Rica, Costa RicaReviewed by:
Junpeng Mu, Mianyang Normal University, ChinaBożena Denisow, University of Life Sciences of Lublin, Poland
Copyright © 2024 Alquichire-Rojas, Escobedo and González-Teuber. This is an open-access article distributed under the terms of the Creative Commons Attribution License (CC BY). The use, distribution or reproduction in other forums is permitted, provided the original author(s) and the copyright owner(s) are credited and that the original publication in this journal is cited, in accordance with accepted academic practice. No use, distribution or reproduction is permitted which does not comply with these terms.
*Correspondence: Marcia González-Teuber, bWdvbnphdEB1Yy5jbA==