- 1College of Life Science, Jilin Agricultural University, Changchun, Jilin, China
- 2College of Agronomy, Jilin Agricultural University, Changchun, China
With the rapid advances in next-generation sequencing technology, numerous non-protein-coding transcripts have been identified, including long noncoding RNAs (lncRNAs), which are functional RNAs comprising more than 200 nucleotides. Although lncRNA-mediated regulatory processes have been extensively investigated in animals, there has been considerably less research on plant lncRNAs. Nevertheless, multiple studies on major crops showed lncRNAs are involved in crucial processes, including growth and development, reproduction, and stress responses. This review summarizes the progress in the research on lncRNA roles in several major crops, presents key strategies for exploring lncRNAs in crops, and discusses current challenges and future prospects. The insights provided in this review will enhance our comprehension of lncRNA functions in crops, with potential implications for improving crop genetics and breeding.
1 Introduction
Noncoding RNAs (ncRNAs), which do not encode proteins and were originally considered to be “transcriptional noise,” account for most of the total RNA in cells (Nojima and Proudfoot, 2022). With the development and application of transcriptomic technology, the importance of an increasing number of ncRNAs for genomic organization and function has been revealed (Jha et al., 2023). In fact, ncRNAs have gradually become a major focus of life sciences research (Waititu et al., 2022; Cui, 2023). The two types of ncRNAs are distinguished by their mechanism of action. Specifically, housekeeping ncRNAs include transfer RNAs (tRNAs), small nuclear RNAs (snRNAs), small nucleolar RNAs (snoRNAs), and ribosomal RNAs (rRNAs), whereas regulatory ncRNAs include short interfering RNAs (siRNAs), microRNAs (miRNAs), PIWI-interacting RNAs (piRNAs), and long noncoding RNAs (lncRNAs) (Duan X. et al., 2020; Virciglio et al., 2021). Among these ncRNAs, lncRNAs affect gene expression through a wide range of mechanisms and are essential regulators of many important biological processes (Qin et al., 2017; Ahmed et al., 2020).
The first stage of research on lncRNAs was from 1980 to 2000, during which lncRNAs were first identified using traditional gene mapping methods, with H19 being one of the first reported lncRNAs (Yoshimura et al., 2018; Yang et al., 2021). Additionally, XIST, the main regulator of X-chromosome inactivation, was also discovered in this period (Hierholzer et al., 2022). In the second stage, which involved a shift from the noncoding genome to the noncoding transcriptome, thousands of lncRNAs were identified in plants. In the third stage, microarrays, tiled arrays, and next-generation sequencing technologies were used to identify regulatory lncRNAs and clarify their involvement in many processes, such as development and pathogenesis, in numerous plant species (Jarroux et al., 2017; Wu et al., 2020). The increasing functional characterization of lncRNAs has been accompanied by an increase in the number of studies on lncRNAs over the last decade. The mechanisms of action of lncRNAs in animals have been extensively studied (Zhang et al., 2020; Zhang X. et al., 2023). Moreover, there has been a steady increase in the research on lncRNAs in both animals and plants over the years. The resulting published articles reflect the growing interest, funding, and research on crop lncRNAs. However, plant lncRNA studies lag behind those on animal lncRNAs, likely because of the delayed initiation of plant research. Nevertheless, lncRNAs in major crops, such as rice, maize, and cotton, have been identified and characterized. Technological advances may be exploited to further expand the research on plant lncRNAs. A comprehensive overview of the progress in crop lncRNA research may be relevant to future investigations on lncRNA mechanisms and their potential applications for crop improvement.
2 Progress in the research on lncRNAs in common crops
lncRNAs play a crucial role in regulating many biological processes in crops. Crops can be classified in different ways, one of which is the botanical classification method used in agriculture. However, since the same crop often serves multiple purposes, it is generally divided based on its primary use. Here, we introduce the research progress of lncRNA by dividing common crops into grain crops (wheat, corn, and rice), oil crops (soybean, peanut, and rapeseed), sugar crops (sugarcane and beet), fiber crops (cotton and hemp), beverage crops (tea and coffee), and vegetables (tomato) (Figure 1).
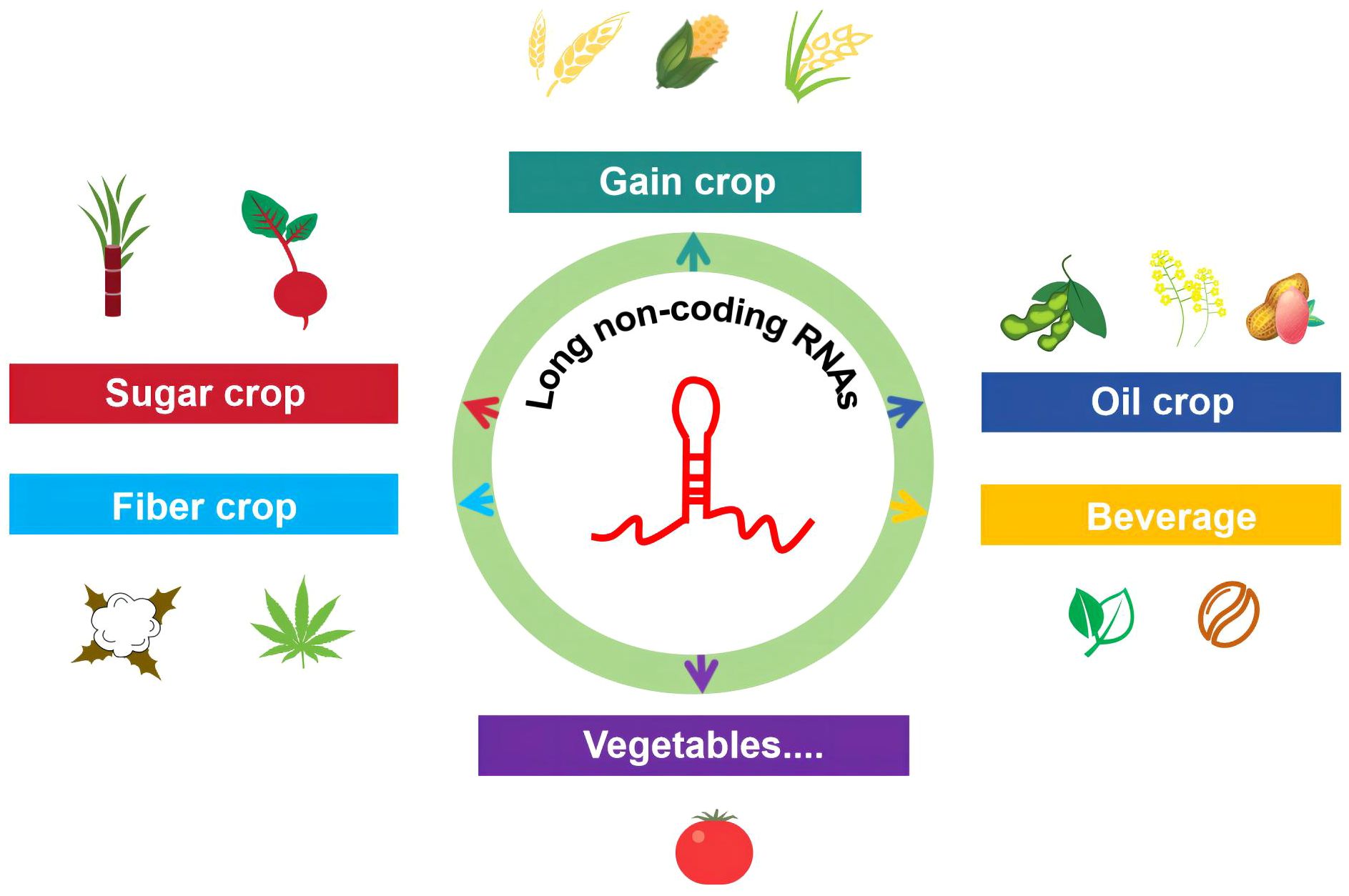
Figure 1 Progress in the research on lncRNAs in common crop species. This review comprehensively summarizes the functions of lncRNAs in major food crops (wheat, corn, and rice), oil crops (soybean, peanut, and rapeseed), sugar crops (sugarcane and beet), fiber crops (cotton and hemp), beverage crops (tea and coffee), and vegetables (tomato).
2.1 Grain crops
In rice, TWISTEDLEAF (TL), is transcribed from the opposite strand of the R2R3 MYB transcription factor gene locus (OsMYB60). Silencing TL via RNA interference reportedly results in abnormal leaves (Liu et al., 2018) (Figure 2A). In terms of disease resistance-related lncRNAs, an RNA sequencing-based analysis of rice leaves infected with Xanthomonas oryzae pv. oryzae (Xoo) revealed the interactions between 39 jasmonate (JA)-related protein-coding genes and 73 lncRNAs. The overexpression of ALEX1 enhances the resistance to Xoo and activates JA signaling (Yu et al., 2020) (Figure 2B). Research on anther and ovary meiosis in autotetraploid rice showed lncRNA57811 overexpression significantly decreases fertility and the seed setting rate, which reflects the critical roles of lncRNAs affecting polyploid rice pollen development (Li X. et al., 2020) (Figure 2C). In addition, MSTRG.28732.3, which is a lncRNA associated with drought resistance, interacts with miR171 to modulate the chlorophyll biosynthesis pathway, thereby influencing drought resistance through Os02g0662700, Os02g0663100, and Os06g0105350 in rice (Yang et al., 2022) (Figure 2D).
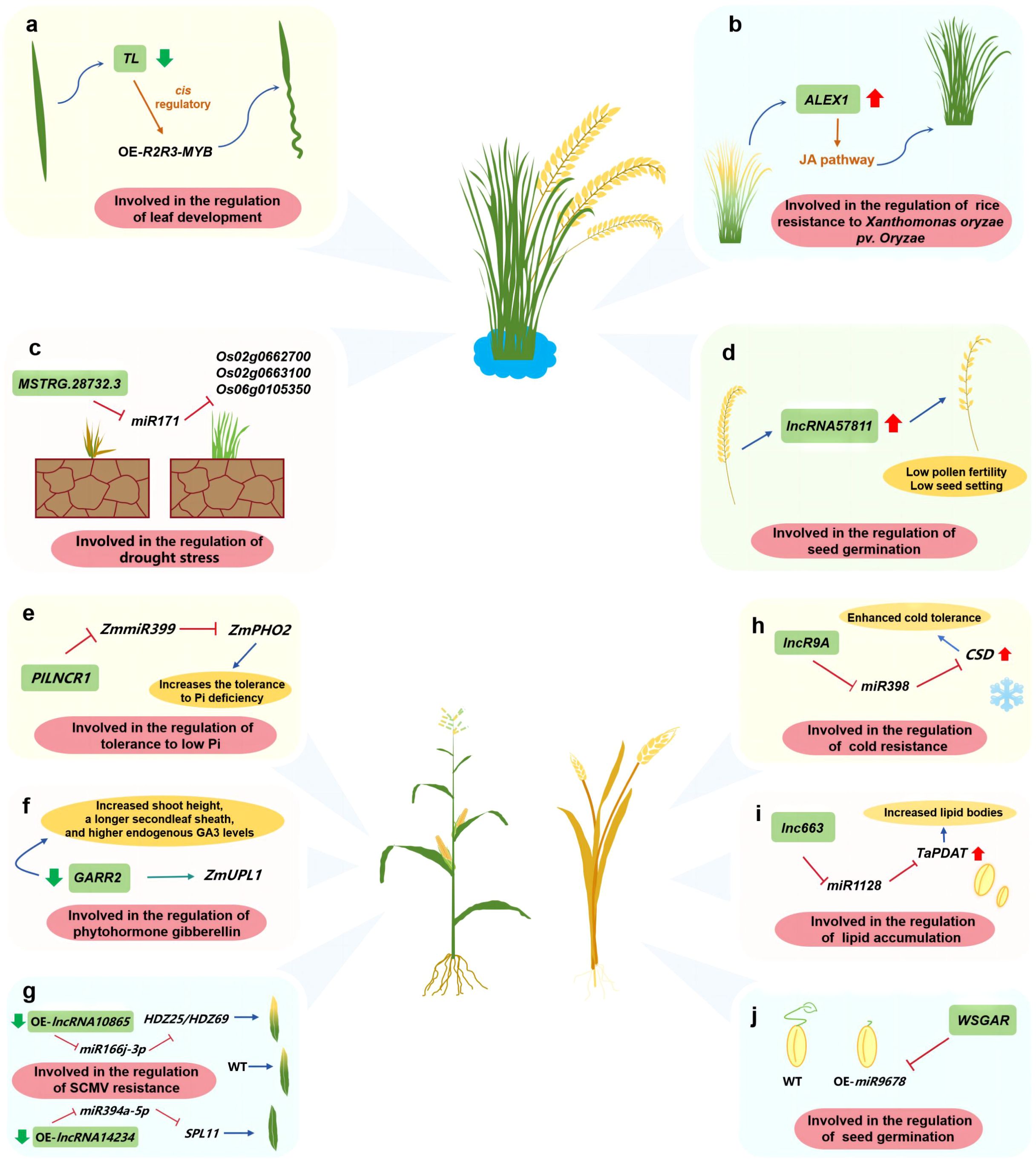
Figure 2 Investigating the function of lncRNAs in gain crops such as maize, rice, and wheat. (A) lncRNA involved in the regulation of leaf development; (B) lncRNA involved in the regulation of rice resistance to Xanthomonas oryzae pv. Oryzae; (C) lncRNA involved in the regulation of drought stress; (D) lncRNA involved in the regulation of seed germination; (E) Involved in the regulation of tolerance to low Pi; (F) lncRNA involved in the regulation of phytohormone gibberellin; (G) lncRNA involved in the regulation of SCMV resistance; (H) lncRNA involved in the regulation of cold resistance; (I) lncRNA involved in the regulation of lipid accumulation; (J) lncRNA involved in the regulation of seed germination.
In maize, lncRNAs contribute to several growth and developmental processes. For example, Pi-deficiency-induced long-noncoding RNA1 (PILNCR1), which was identified following an analysis of strand-specific RNA libraries, can inhibit ZmmiR399-guided cleavage of ZmPHO2, ultimately affecting the ability of maize to tolerate low-Pi conditions (Du et al., 2018) (Figure 2E). The CRISPR/Cas9-based editing of the lncRNA GARR2 in the GARR2KO line leads to increases in bud height, second leaf sheath length, and endogenous GA3 levels. Additionally, according to RNA pull-down assays, GARR2 can influence the abundance of its target (ZmUPL1) during the gibberellin (GA) response (Li W. et al., 2022) (Figure 2F). Furthermore, sugarcane mosaic virus (SCMV)-responsive lncRNA–miRNA–mRNA networks have been established. The lncRNA10865-miR166j-3p-HDZ25/HDZ69 and lncRNA14234-miR394a-5p-SPL11 modules played roles in maize resistance to SCMV infection. Among them, after lncRNA10865 and lncRNA14234 were silenced, SCMV symptoms were aggravated and alleviated, respectively (Gao et al., 2023) (Figure 2G).
In a previous study on the mechanism mediating the cold resistance of winter wheat, lncR9A was revealed to function as a competing endogenous RNA (ceRNA) that regulates the cooperative interaction between tae-miR398 and TaCSD1 under cold conditions (Lu et al., 2020) (Figure 2H). An investigation on wheat grain fat biosynthesis detected a lncRNA that serves as a ceRNA modulating lipid accumulation through TaPDAT. More specifically, on the basis of a GFP reporter assay, lnc663 can sequester miR1128 through complementary interactions to up-regulate TaPDAT expression in tobacco (Madhawan et al., 2023) (Figure 2I). In addition, lncRNAs may also regulate abscisic acid/GA signaling to affect seed germination. The overexpression of miR9678 delays wheat seed germination by decreasing the bioactive GA content. Interestingly, miR9678 targeted the lncRNA WSGAR (Guo et al., 2018) (Figure 2J).
In summary, lncRNAs modulate the growth, development, and biotic and abiotic stress responses of major grain crops, including rice, maize, and wheat. While these biological processes may affect grain crop yields, the molecular mechanisms underlying lncRNA functions in grain crops must be more precisely deciphered to improve grain crop production.
2.2 Oil crops
Soybean is one of the main oil crops. In the study of soybean salt response stress, the interaction between Gmax_MSTRG.35921.1 and miR166i was verified by LAMP assay followed by RT-PCR, which indicated the potential regulatory role of lncRNA under salinity stress (Li C. et al., 2022) (Figure 3A). In addition, overexpressing lncRNA77580 in soybean could increase the drought tolerance and seed yield by increasing the number of seeds per plant (Chen X. et al., 2023) (Figure 3B). The lncRNA43234-miRNA10420-XM_014775781.1 network related to lipid synthesis was screened out by full-length transcriptome sequencing for Wild type (WT) soybean “JN18” (Jishendou 2006) and low linolenic acid mutant “MT72”. Overexpression of lncRNA43234 resulted in increased protein content and decreased oleic acid content in Arabidopsis thaliana seeds (Ma et al., 2021; Zhang A. et al., 2023) (Figure 3C).
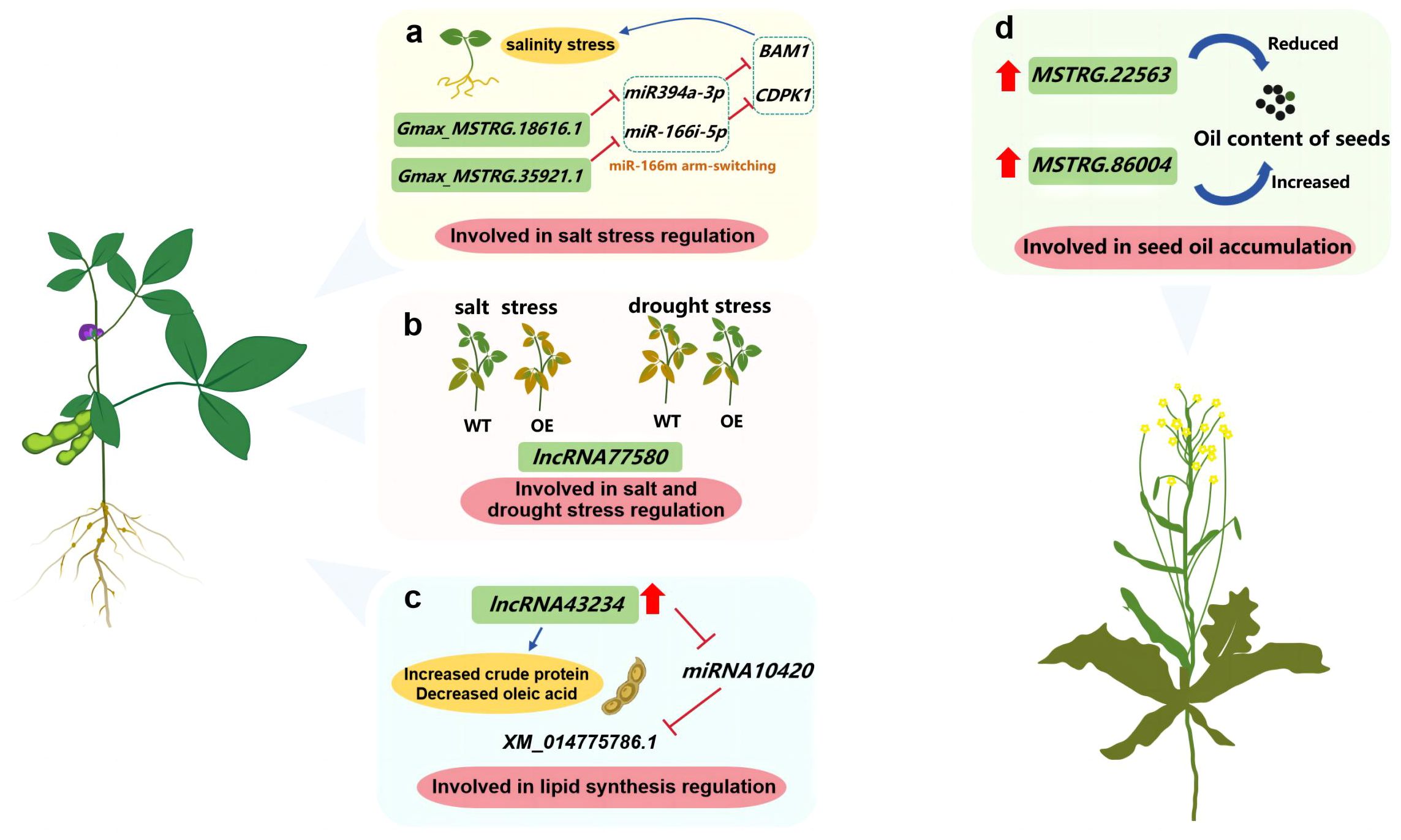
Figure 3 Investigating the function of lncRNAs in oil crops such as soybean and rapeseed. (A) lncRNA involved in salt stress regulation; (B) lncRNA involved in salt and drought stress regulation; (C) lncRNA involved in lipid synthesis regulation; (D) lncRNA involved in seed oil accumulation.
Brassica napus L., which is one of three types of oilseed rape, has the highest grain yield among all oilseed rape varieties. The seed oil content decreases by 3.1%–3.9% following the overexpression of MSTRG.22563, but increases by approximately 2% if MSTRG.86004 is overexpressed (Li Y. et al., 2023) (Figure 3D). However, clubroot disease causes significant Brassica yield losses. A total of 464 differentially expressed lncRNAs were identified in the roots of resistant plants challenged with Plasmodiophora brassicae, with most of the genes targeted by these lncRNAs associated with plant–pathogen interactions and hormone signaling pathways (Summanwar et al., 2021). Furthermore, the positive effects of lncRNAs on B. napus drought tolerance have been elucidated. Certain lncRNAs affecting plant hormone signaling and defense mechanisms are co-expressed with protein-coding genes (Tan et al., 2020).
In 2019, a weighted correlation network analysis established a co-expression network comprising 4,713 lncRNAs, which enabled the identification of lncRNAs associated with the growth and development of various peanut tissues (Zhao et al., 2019). Concurrently, seeds from two peanut recombinant inbred lines (RIL8) with differing seed sizes were subjected to strand-specific whole transcriptome sequencing at 15 and 35 days after flowering (DAF). An examination of differentially expressed genes and qPCR data revealed the importance of 11 lncRNAs and their cis-acting target genes for peanut seed development (Ma et al., 2020). Furthermore, 10 lncRNAs functioned as ceRNAs involved in oxidation–reduction processes and other metabolic pathways during a root-knot nematode infection of peanut (Xu et al., 2022).
The findings of previous studies indicate lncRNAs modulate the oil content and quality of oil crops (e.g., soybean and rapeseed). Clarifying the gene regulatory network governing lipid metabolism is crucial for enhancing oil crop yield and quality. A thorough examination of the key lncRNAs associated with oil metabolism will provide relevant insights into the molecular mechanisms underlying lipid metabolism in oil crops.
2.3 Sugar crops
The main sugar crops include sugarcane (Saccharum officinarum L.) and sugar beet (Beta vulgaris). In a study exploring sugarcane tiller development, 310 conserved lncRNAs were screened on the basis of a PacBio Iso-Seq analysis of leaf and tiller bud samples (Yan et al., 2021). Previous studies had shown that miR408 is important for the interaction between sugarcane and microorganisms. A long intergenic noncoding RNA (lincRNA) with significant complementarity to miR408 was predicted to act as miRNA bait, with inhibitory effects on the regulation of canonical miR408 targets (Thiebaut et al., 2017). Other studies showed that miRNAs influence sugarcane growth and development, stress resistance, and other processes (Li A. M. et al., 2023; Gao et al., 2022). The research conducted to date on sugarcane lncRNAs has primarily relied on predictions, which will need to be experimentally verified. In particular, the regulatory effects of lncRNAs on sweetness-related genes should be characterized.
Changes in gene expression during sugar beet responses to salt stress have been elucidated via whole transcriptome RNA-seq and degradome sequencing analyses, which identified 61 differentially expressed lncRNAs in roots and 55 target genes (Li J. et al., 2020). In another study, sugar beet responses to drought stress were examined, resulting in the detection of 386 differentially expressed lncRNAs; the expression of the most significantly up-regulated lncRNA increased more than 6,000-fold, whereas the expression of the most significantly down-regulated lncRNA decreased more than 18,000-fold (Zou et al., 2023). In sugar beet, the gene (Bv8_189980_mizi.t1) targeted by the lncRNA MSTRG.26204.1 encodes a B3 domain-containing transcriptional repressor (VAL1-like), suggesting this gene may be associated with vernalization. Hence, lncRNAs may be involved in the sugar beet vernalization process (Liang et al., 2022).
Although there is evidence indicating lncRNAs affect the drought resistance as well as the growth and development of sugar crops, their contribution to sugar biosynthesis and the underlying molecular mechanism remain unclear. Exploring the effects of lncRNAs on plant sugar biosynthesis pathways may provide insights relevant to regulating key sugar crop traits.
2.4 Beverage crops
Cocoa, coffee, and tea are the main beverage crops worldwide. To date, there has been limited research on cocoa lncRNAs, but coffee and tea lncRNAs have been identified and functionally characterized. In Coffea canephora, 2,384 high-confidence lncRNAs were identified on the basis of a comprehensive genome-wide analysis (Lemos et al., 2020). A total of 10,564 lncRNAs were identified in another coffee species (Coffea arabica L.). Their involvement in important biological processes was predicted by a Gene Ontology (GO) analysis (Abdel-Salam et al., 2021). In tea (Camellia sinensis), lncRNAs are involved in disease resistance-related mechanisms. Additionally, in C. sinensis ‘Baiye No. 1’, differentially expressed lncRNAs participate in responses to periodic albinism through the GAMYB–miR159–lncRNA regulatory network (Xu et al., 2023). A recent study indicated MSTRG.20036, MSTRG.3843, MSTRG.26132, and MSTRG.56701 influence the development of tea leaf spot disease through cis-regulatory mechanisms (Huang et al., 2023). Another lncRNA (MSTRG.139242.1) may modulate the response to salt stress through Ca2+ ATPase 13 in the Ca2+ transport pathway (Wan et al., 2020). In response to daylight-induced withering, lncRNAs alter flavonoid and terpenoid metabolic pathways as well as JA/methyl jasmonate biosynthesis and signal transduction in oolong tea (C. sinensis) (Zhu et al., 2019). Thus, lncRNAs help regulate disease resistance mechanisms and salt stress responses in beverage crops. They also regulate the production of biologically active substances that influence the flavor profile and other characteristics of beverage crops.
2.5 Fiber crops
Cotton seeds produce fiber. Some studies have shown that lncRNAs are involved in the disease resistance of cotton. For example, lncRNA2 and its target gene PG12 negatively regulate cotton resistance to verticillium wilt, while lncRNA7 and its target gene PMEI13 have the opposite effect (Zhang L. et al., 2022) (Figure 4A). Interestingly, lncRNAs are also involved in cotton responses to abiotic stress. More specifically, DAN1, which is a lincRNA associated with drought responses, can regulate AAAG motif-containing genes in the auxin response pathway (Tao et al., 2021) (Figure 4B). Another lincRNA, XH123, was revealed to control the cold stress response of cotton seedlings (Cao et al., 2021) (Figure 4C). The salt-responsive lncRNAs TRABA and lncRNA354 serve as upstream regulators that control the expression of the salt stress response-related genes GhBGLU24 and GhARF, respectively (Zhang X. et al., 2021; Cui et al., 2024) (Figure 4D). In cotton, MSTRG 2723.1 mediates the expression of key genes related to fatty acid metabolism, the MYB25-mediated pathway, and pectin metabolism to regulate fiber synthesis (Zou et al., 2022). In addition to cotton, hemp is another major fiber crop. In ramie (Boehmeria nivea L. Gaud), a MYB gene (BntWG10016451) is targeted by lncRNA00022274. The overexpression of this gene reportedly increases fiber production in A. thaliana (Fu et al., 2023). Considered together, the findings of earlier studies suggest lncRNAs play crucial roles in fiber crop responses to biotic and abiotic stresses, while also influencing fiber formation. These investigations have increased our understanding of how lncRNAs regulate plant fiber development.
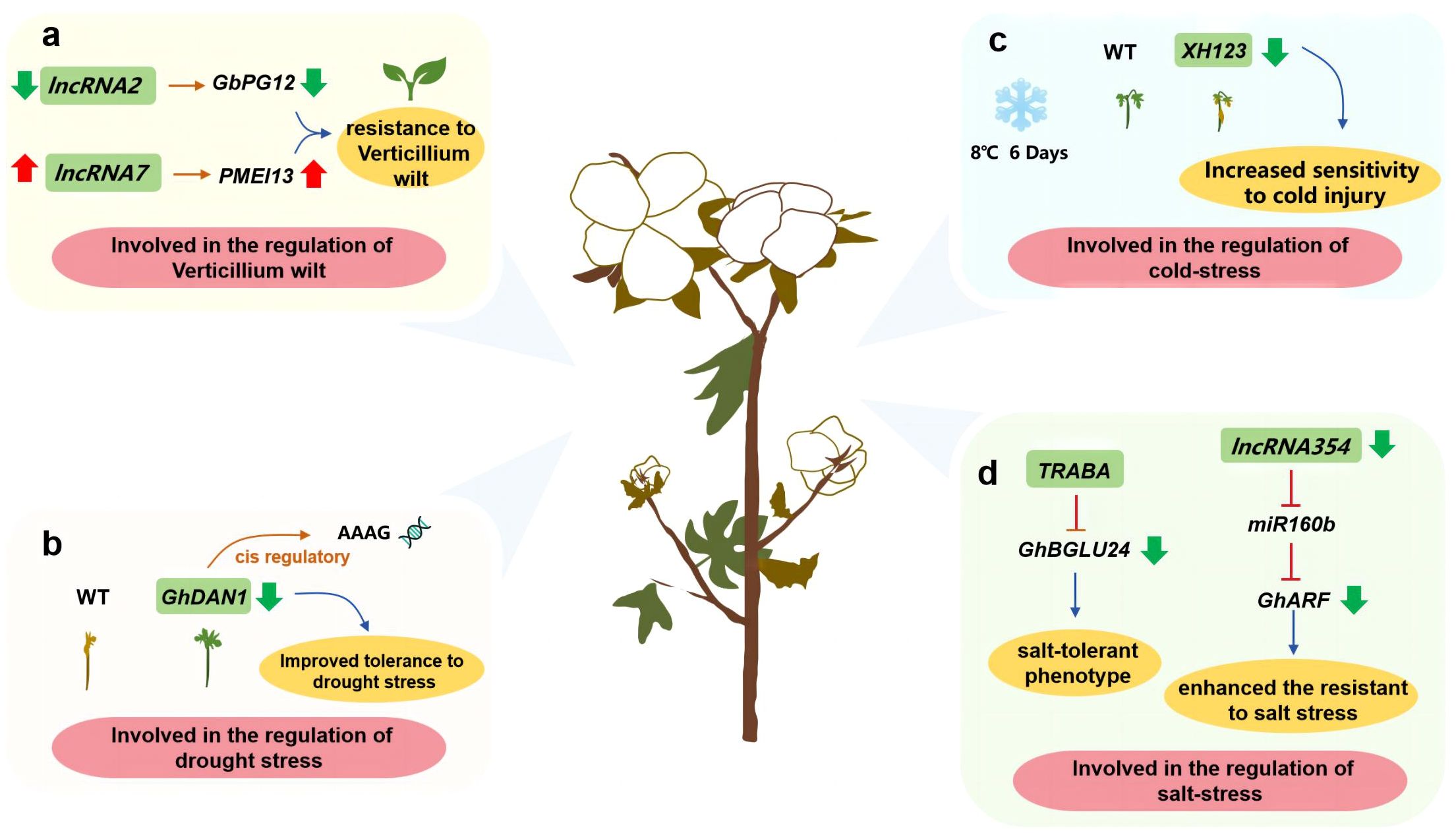
Figure 4 Investigating the function of lncRNAs in fiber crops such as cotton. (A) lncRNA involved in the regulation of Verticillium wilt; (B) lncRNA involved in the regulation of drought stress; (C) lncRNA involved in the regulation of cold-stress; (D) lncRNA involved in the regulation of salt-stress.
2.6 Vegetables
Tomato (Solanum lycopersicum L.) is one of the most important vegetable crops. The silencing of lncRNA1459 reportedly decreases ethylene accumulation and carotenoid biosynthesis in tomato, with detrimental effects on fruit ripening (Li et al., 2018) (Figure 5A). During carotenoid biosynthesis, octahydro-lycopene synthase (PSY) catalyzes the formation of two GGPP molecules. Additionally, trans-splicing between SlPsy1 and the lncRNA ACoS-AS1 leads to the formation of yellow tomato fruit (Xiao et al., 2020) (Figure 5B). Overexpressing Solyc10g006360 decreases the formation of type I trichomes. An earlier study showed lncRNA000170, which is transcribed from the complementary strand of Solyc10g006360, may affect multicellular trichome formation by inducing target gene expression (Liao et al., 2020) (Figure 5C). In the study of tomato against Phytophthora infestans, overexpression of Sl-lncRNA47980 up-regulated the expression of SlGA2ox4, while overexpression of lncRNA39026 down-regulated the expression of miR168a and increases the expression of SlAGO1. In tomato, the overexpression of lncRNA23468 and lncRNA08489 significantly decreases the expression of miR482b and miR482e-3p, respectively, but the expression of target genes encoding NBS-LRR proteins increases significantly. These lncRNAs positively regulate the resistance of tomato plants to P. infestans. Conversely, Sl-lncRNA39896 negatively regulates tomato resistance to P. infestans; this lncRNA functions as an endogenous target mimic of Sl-miR166b that controls HDZ expression (Jiang et al., 2019; Hou et al., 2020; Su et al., 2023; Liu et al., 2022; Hong et al., 2022) (Figure 5D).
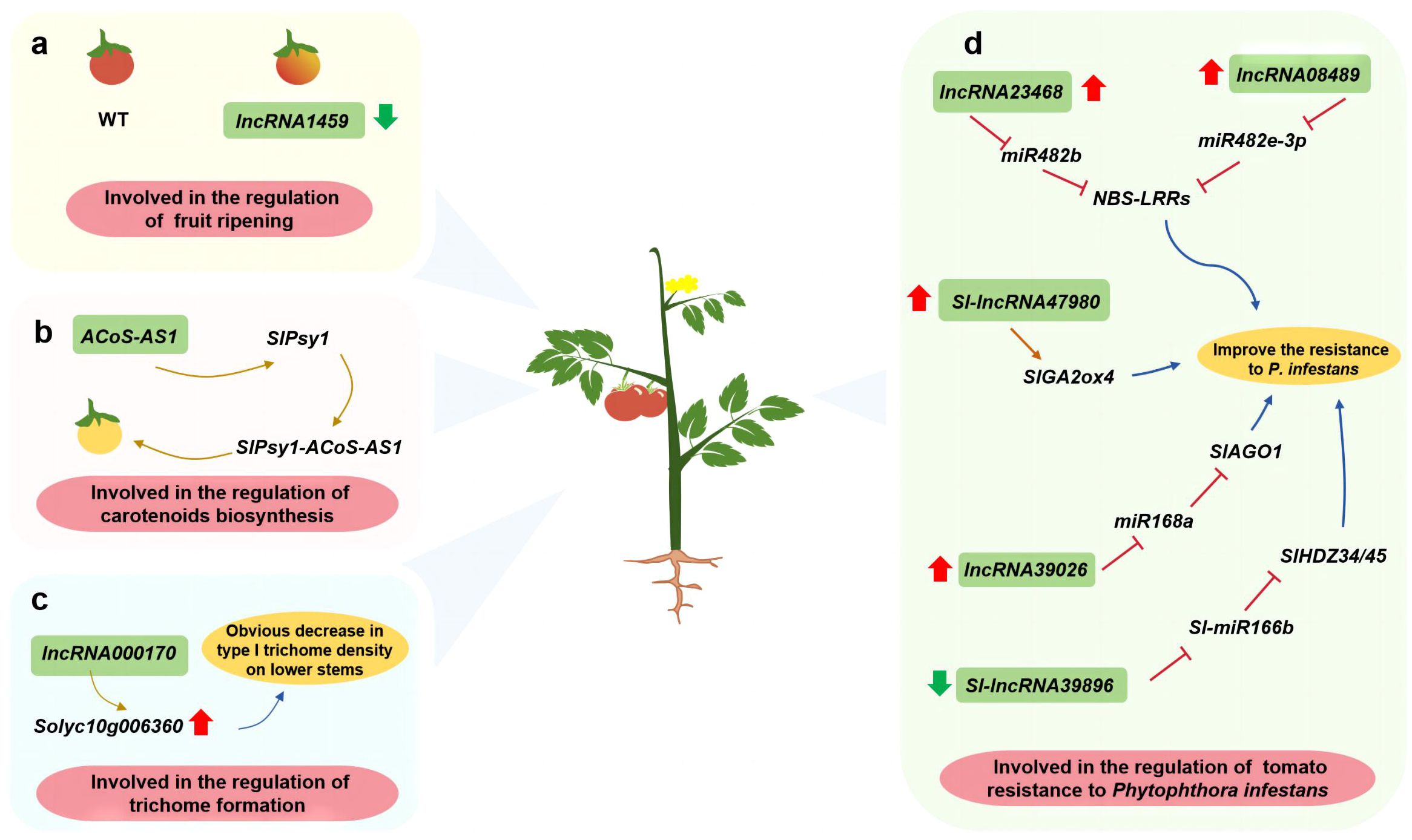
Figure 5 Investigating the function of lncRNAs in vegetable such as tomato. (A) lncRNA involved in the regulation of fruit ripening; (B) lncRNA involved in the regulation of carotenoids biosynthesis; (C) lncRNA involved in the regulation of trichome formation; (D) lncRNA involved in the regulation of tomato resistance to Phytophthora infestans.
3 Main research strategies for crop lncRNA
In this section, we highlight the primary methods used to investigate lncRNAs in common crops (Figure 6). Novel lncRNAs are generally identified in studies involving high-throughput sequencing (e.g., after various treatments, at selected time points, or in specific tissues) followed by transcript splicing and assembly. The expression levels of candidate lncRNAs and mRNAs are then analyzed to screen for differential expression. Plant studies focused on lncRNA functions mainly involve the application of second-generation sequencing technologies, despite the increasing popularity of third-generation sequencing. Although third-generation sequencing technology may be better than earlier sequencing technologies for sequencing genomes and transcriptomes, its widespread application may be restricted by its high costs. Because of its advantages (e.g., short reads, high throughput, and high accuracy), second-generation sequencing technology is still commonly used for plant research. However, third-generation full-length transcriptome sequencing has generated high-quality complete transcriptomes (Zhu et al., 2013; Rhoads and Au, 2015; van Dijk et al., 2023).
Sequenced transcripts may be screened for lncRNAs using diverse methods (e.g., CPC, CNCI, CPAT, and pfam protein domain analysis) (Zhang J. et al., 2022; Liu X. Q. et al., 2019; Duan Y. et al., 2020). The PmlIPM model was recently used to predict plant miRNA–lncRNA associations (Tang and Ji, 2023). By integrating a paired sgRNA design with an off-target analysis, CRISPRlnc can be used to design CRISPR/Cas9 sgRNAs for ncRNAs (Yang et al., 2024). The identified lncRNAs, including lincRNAs, intronic lncRNAs, antisense RNAs, NATs, bidirectional lncRNAs, and eRNAs, may be classified according to their genomic locations relative to protein-coding genes. This classification is useful for future studies on lncRNA functions (Chekanova, 2015; Wierzbicki et al., 2021). The expression and functional significance of lncRNAs and protein-coding genes must be clarified. Differentially expressed genes and consistently highly expressed genes may play crucial roles in key metabolic pathways and biological processes. Several databases (e.g., KEGG, GO, GreeNC, PlncRNADB, LncTar, NONCODE, and other lncRNA-related databases) have been used to predict lncRNA functions and select lncRNAs for further analyses (Di Marsico et al., 2022; Li et al., 2015; Quek et al., 2015; He et al., 2008).
Full-length lncRNAs may be amplified via rapid amplification of cDNA ends (RACE) for in-depth analyses when only transcript fragments are available (Zhou et al., 2023). The mechanisms mediating the regulatory effects of lncRNAs in plants vary because of the diversity in the cellular locations of lncRNAs. The localization of lncRNAs in cells can be determined by conducting a qPCR analysis of lncRNA expression in the isolated nucleus and cytoplasm or a fluorescence in situ hybridization assay (Qin and Xiong, 2019; Yang et al., 2020). Nuclear lncRNAs interact with DNA, RNA, proteins, and other molecules to regulate chromosome structure and function, while also controlling gene transcription (cis- or trans-regulation). In contrast, cytoplasm-localized lncRNAs have post-transcriptional regulatory effects (Zhang M. et al., 2021; Dietrich et al., 2015; Hu et al., 2023). The interaction between lncRNAs and proteins may be confirmed using various approaches, including pull-down assays, RNA-binding protein immunoprecipitation (RIP), cross-linking immunoprecipitation (CLIP), and chromatin isolation by RNA purification (ChIRP) (Ferrè et al., 2016; Jiang et al., 2023). The ceRNA mechanism is currently a major topic of interest among researchers. Various methods, including 5′ RLM RACE, ChIRP, and binding site prediction, are useful for investigating the interaction between lncRNAs and miRNAs or circRNAs (Zhang L. et al., 2023; Rao et al., 2022). After the initial verification, lncRNA functionality must be confirmed. This involves constructing overexpression vectors that are subsequently inserted into plants for an analysis of the effects of lncRNA overexpression. Additionally, RNAi, CRISPR/Cas9, VIGS, and other technologies were utilized to suppress target genes or induce mutations, ultimately confirming the function of the target lncRNA (Aydinoglu and Kuloglu, 2023; Bravo-Vázquez et al., 2023).
4 Discussion and prospects
In addition to conventional breeding techniques, technological advances (e.g., transgenic technology) have resulted in several alternative methods for improving crop traits (Kumar et al., 2020). Third-generation sequencing technologies have facilitated the detection and characterization of functional genes beyond protein-coding genes, with the identified lncRNAs potentially useful for enhancing crop traits (Xu et al., 2020; Chen T. et al., 2023). We herein reviewed the effects of lncRNAs on the growth and development of key crops. Crops have been classified according to their uses as well as their botanical characteristics. Field crops are frequently divided into three categories: edible crops, industrial raw materials, and feed crops. However, because of the multifunctionality of many crops, in this review, we classified them according to their primary use. We specifically focused on lncRNAs with confirmed regulatory functions in crops, rather than those that are merely predicted to be associated with crop growth and development. We also summarized the major findings of studies on lncRNA functions in various plant species. The importance of lncRNAs for regulating crop growth was emphasized (Table 1).
Research on lncRNAs in crops lags behind the corresponding research in animals. Hence, there are numerous gaps in our knowledge that will need to be addressed. Nevertheless, numerous functional lncRNAs had been identified and functionally annotated in various model plants (Jampala et al., 2021). Further research is needed to elucidate the functions of lncRNAs in crop species as well as the underlying mechanisms. Unlike the extensively annotated protein-coding genes, lncRNAs are frequently inadequately annotated. Crop lncRNAs may be annotated and classified using the methods that were employed for annotating animal lncRNAs (Ballarino et al., 2023; Park and Kim, 2023). However, in addition to RNA-seq technology, animal lncRNAs can be identified using gene chip technology. Although gene chips are widely used for animal and pharmaceutical research, they are too expensive for most agricultural scientific research institutions. Therefore, the application of gene chip technology for annotating plant lncRNAs may depend on a decrease in the associated costs (Morohashi et al., 2009; Verma et al., 2022; Song et al., 2023). The precise genome locations and functional significance of numerous lncRNAs remain unknown. The biological functions of lncRNAs are intricately linked to their secondary structure. Unfortunately, existing programs and tools for lncRNAs often prioritize the complete secondary structure, while overlooking local structures crucial for biological functions (Herman et al., 2022; Sanbonmatsu, 2022). To further annotate lncRNAs, their secondary structures will need to be explored at a higher resolution. New sources of lncRNAs were continually being identified and classified (Chorostecki et al., 2023; Li C. et al., 2023; Mattick et al., 2023).
Further advances in related technologies may lead to a more comprehensive elucidation of lncRNA functions and the associated mechanisms. The development of more efficient programs and tools has enabled researchers to acquire increasingly accurate insights into lncRNAs in crops (Sheng et al., 2023). Moreover, CRISPR technology, which was initially used for plant genome editing in 2013, has been exploited to improve crop traits. Progress in the related research has resulted in enhanced breeding practices, but it has also simplified the classification of lncRNA functions, thereby enabling researchers to functionally validate lncRNAs in crops (Atia et al., 2024; Chovatiya et al., 2024). In this context, lncRNAs are also expected to play a more essential role in the genetic breeding of crops, the development of biological resource, the engineering of plant cells, and other areas. Improved living standards, farming system changes, research on plant diseases and pest infestations, and the development of specialized crops have necessitated the generation of new crop varieties. Furthermore, varietal replacement rates have increased. Hence, transgenic breeding can no longer be reserved for exploring protein-coding genes. Functional lncRNAs will need to be identified and analyzed regarding their utility for promoting crop production. This may increase crop yields, enhance crop stress resistance, and optimize the contents of beneficial substances, thereby increasing the efficiency of agricultural production (Palos et al., 2023; Yang et al., 2023). Furthermore, lncRNAs may be considered as key factors influencing cellular architecture. By culturing and proliferating cells or modifying specific plant cell characteristics, breeders can generate economically valuable crop products (Gonzales et al., 2024). Although research on crop lncRNAs is in its nascent stages, studies conducted to date have highlighted the importance of lncRNAs as well as the need for additional research to more precisely determine their roles in crops.
Author contributions
AZ: Writing – original draft. WP: Writing – review & editing. YW: Writing – review & editing. YL: Writing – review & editing. JW: Writing – review & editing. SL: Writing – review & editing. XC: Writing – review & editing. HL: Writing – original draft. DY: Writing – original draft. RZ: Writing – original draft.
Funding
The author(s) declare financial support was received for the research, authorship, and/or publication of this article. This research was funded by Jilin Province science and technology development plan project (20240601083RC), the Science and technology research project of Education Department of Jilin Province (JJKH20230394KJ), and the Key Research and Development Program of Science and Technology of Jilin Province (No. 20210202006NC).
Acknowledgments
We appreciate the support of the National Engineering Research Center for food crops.
Conflict of interest
The authors declare that the research was conducted in the absence of any commercial or financial relationships that could be construed as a potential conflict of interest.
Publisher’s note
All claims expressed in this article are solely those of the authors and do not necessarily represent those of their affiliated organizations, or those of the publisher, the editors and the reviewers. Any product that may be evaluated in this article, or claim that may be made by its manufacturer, is not guaranteed or endorsed by the publisher.
References
Abdel-Salam, E. M., Qahtan, A. A., Gaafar, A. Z., Zein El-Abedein, A. I., Alshameri, A. M., Alhamdan, A. M. (2021). Tissue-specific analysis of Coffea arabica L. transcriptome revealed potential regulatory roles of lncRNAs. Saudi J. Biol. Sci. 28, 6023–6029. doi: 10.1016/j.sjbs.2021.07.006
Ahmed, W., Xia, Y., Li, R., Bai, G., Guo, P. (2020). Non-coding RNAs: functional roles in the regulation of stress response in brassica crops. Genomics. 112, 1419–1424. doi: 10.1016/j.ygeno.2019.08.011
Atia, M., Jiang, W., Sedeek, K., Butt, H., Mahfouz, M. (2024). Crop bioengineering via gene editing: reshaping the future of agriculture. Plant Cell Rep. 43, 98–117. doi: 10.1007/s00299-024-03183-1
Aydinoglu, F., Kuloglu, A. (2023). icotiana benthamiana as a model plant host for Fusarium verticillioides to investigate RNA interference, cross-kingdom RNA exchange, and competitive endogenous RNA (ceRNA) network. Mol. Biol. Rep. 50, 8061–8072. doi: 10.1007/s11033-023-08698-6
Ballarino, M., Pepe, G., Helmer-Citterich, M., Palma, A. (2023). Exploring the landscape of tools and resources for the analysis of long non-coding RNAs. Comput. Struct. Biotechnol. J. 21, 4706–4716. doi: 10.1016/j.csbj.2023.09.041
Bravo-Vázquez, L. A., Méndez-García, A., Chamu-García, V., Rodríguez, A. L., Bandyopadhyay, A., Paul, S. (2023). The applications of CRISPR/Cas-mediated microRNA and lncRNA editing in plant biology: shaping the future of plant non-coding RNA research. Planta. 259, 32–52. doi: 10.1007/s00425-023-04303-z
Cao, Z., Zhao, T., Wang, L., Han, J., Chen, J., Hao, Y. (2021). The lincRNA XH123 is involved in cotton cold-stress regulation. Plant Mol. Biol. 106, 521–531. doi: 10.1007/s11103-021-01169-1
Chekanova, J. A. (2015). Long non-coding RNAs and their functions in plants. Curr. Opin. Plant Biol. 27, 207–216. doi: 10.1016/j.pbi.2015.08.003
Chen, P., Song, Y., Liu, X., Xiao, L., Bu, C., Liu, P. (2022). LncRNA PMAT-PtoMYB46 module represses PtoMATE and PtoARF2 promoting Pb2+ uptake and plant growth in poplar. J. Hazard Mater. 433, 128769. doi: 10.1016/j.jhazmat.2022.128769
Chen, T., Zuo, D., Yu, J., Hou, Y., Wang, H., Gu, L., et al. (2023). Full-length transcriptome sequencing analysis and characterization of WRKY transcription factors responsive to cadmium stress in arabis paniculata. Plants (Basel). 12, 3779–3795. doi: 10.3390/plants12213779
Chen, W., Zhu, T., Shi, Y., Chen, Y., Li., W. J., Chan, R. J. (2023). An antisense intragenic lncRNA SEAIRa mediates transcriptional and epigenetic repression of SERRATE in Arabidopsis. Proc. Natl. Acad. Sci. U.S.A. 120, e2216062120. doi: 10.1073/pnas.2216062120
Chen, X., Jiang, X., Niu, F., Sun, X., Hu, Z., Gao, F. (2023). Overexpression of lncRNA77580 regulates drought and salinity stress responses in soybean. Plants (Basel). 12, 181–192. doi: 10.3390/plants12010181
Chorostecki, U., Bologna, N. G., Ariel, F. (2023). The plant noncoding transcriptome: a versatile environmental sensor. EMBO J. 16, 42–62. doi: 10.15252/embj.2023114400
Chovatiya, A., Rajyaguru, R., Tomar, R. S., Joshi, P. (2024). Revolutionizing agriculture: harnessing CRISPR/cas9 for crop enhancement. Indian J. Microbiol. 64, 59–69. doi: 10.1007/s12088-023-01154-w
Cui, C., Wan, H., Li, Z., Ai, N., Zhou, B. (2024). Long noncoding RNA TRABA suppresses β-glucosidase-encoding BGLU24 to promote salt tolerance in cotton. Plant Physiol. 194 (2), 1120–1138. doi: 10.1093/plphys/kiad530
Cui, J. (2023). Editorial: lncRNA in plants: function, mechanisms and applications. Front. Plant Sci. 14. doi: 10.3389/fpls.2023.1238185
Dietrich, A., Wallet, C., Iqbal, R. K., Gualberto, J. M., Lotfi, F. (2015). Organellar non-coding RNAs: emerging regulation mechanisms. Biochimie. 117, 48–62. doi: 10.1016/j.biochi.2015.06.027
Di Marsico, M., Paytuvi Gallart, A., Sanseverino, W., Aiese Cigliano, R. (2022). GreeNC 2.0: a comprehensive database of plant long non-coding RNAs. Nucleic Acids Res. 50, 1442–1447. doi: 10.1093/nar/gkab1014
Du, Q., Wang, K., Zou, C., Xu, C., Li, W. X. (2018). The PILNCR1-miR399 regulatory module is important for low phosphate tolerance in maize. Plant Physiol. 177, 1743–1753. doi: 10.1104/pp.18.00034
Duan, X., Song, X., Wang, J., Zhou, M. (2020). Genome-wide identification and characterization of Fusarium graminearum-responsive lncRNAs in Triticum aestivum. Genes. 11, 1135–1150. doi: 10.3390/genes11101135
Duan, Y., Zhang, W., Cheng, Y., Shi, M., Xia, X. Q. (2020). A systematic evaluation of bioinformatics tools for identification of long noncoding RNAs. RNA. 27, 80–98. doi: 10.1261/rna.074724.120
Ferrè, F., Colantoni, A., Helmer-Citterich, M. (2016). Revealing protein-lncRNA interaction. Brief Bioinform. 17, 106–116. doi: 10.1093/bib/bbv031
Fu, Y., Yi, L., Li, F., Rao, J., Yang, X., Wang, Y. (2023). Integrated microRNA and whole-transcriptome sequencing reveals the involvement of small and long non-coding RNAs in the fiber growth of ramie plant. BMC Genomics 24, 599–609. doi: 10.1186/s12864-023-09711-9
Gao, S., Yang, Y., Yang, Y., Zhang, X., Su, Y., Guo, J. (2022). Identification of Low-Nitrogen-Related miRNAs and Their Target Genes in Sugarcane and the Role of miR156 in Nitrogen Assimilation. Int. J. Mol. Sci. 23, 13187–13202. doi: 10.3390/ijms232113187
Gao, X., Hao, K., Du, Z., Zhang, S., Guo, J., Li, J. (2023). Whole-transcriptome characterization and functional analysis of lncRNA-miRNA-mRNA regulatory networks responsive to sugarcane mosaic virus in maize resistant and susceptible inbred lines. Int. J. Biol. Macromol. 257, 128685. doi: 10.1016/j.ijbiomac
Gonzales, L. R., Blom, S., Henriques, R., Bachem, C. W. B., Immink, R. G. H. (2024). LncRNAs: the art of being influential without protein. Trends Plant Sci. 16, S1360–S1385. doi: 10.1016/j.tplants.2024.01.006
Guo, G., Liu, X., Sun, F., Cao, J., Huo, N., Wuda, B. (2018). Wheat miR9678 Affects Seed Germination by Generating Phased siRNAs and Modulating Abscisic Acid/Gibberellin Signaling. Plant Cell. 30, 796–814. doi: 10.1105/tpc.17.00842
He, S., Liu, C., Skogerbø, G., Zhao, H., Wang, J., Liu, T. (2008). NONCODE v2.0: decoding the non-coding. Nucleic Acids Res. 36, 170–172. doi: 10.1093/nar/gkm1011
Herman, A. B., Tsitsipatis, D., Gorospe, M. (2022). Integrated lncRNA function upon genomic and epigenomic regulation. Mol. Cell. 82, 2252–2266. doi: 10.1016/j.molcel.2022.05.027
Hierholzer, A., Chureau, C., Liverziani, A., Ruiz, N. B., Cattanach, B. M., Young, A. N. (2022). long noncoding RNA influences the choice of the X chromosome to be inactivated. Proc. Natl. Acad. Sci. U.S.A. 28, 119–129. doi: 10.1073/pnas.2118182119
Hong, Y., Zhang, Y., Cui, J., Meng, J., Chen, Y., Zhang, C. (2022). The lncRNA39896-miR166b-HDZs module affects tomato resistance to Phytophthora infestans. J. Integr. Plant Biol. 64, 1979–1993. doi: 10.1111/jipb.13339
Hou, X., Cui, J., Liu, W., Jiang, N., Zhou, X., Qi, H. (2020). LncRNA39026 Enhances Tomato Resistance to Phytophthora infestans by Decoying miR168a and Inducing PR Gene Expression. Phytopathology. 110, 873–880. doi: 10.1094/PHYTO-12-19-0445-R
Hu, X., Yin, G., Zhang, Y., Zhu, L., Huang, H., Lv, K. (2023). Recent advances in the functional explorations of nuclear microRNAs. Front. Immunol. 14. doi: 10.3389/fimmu.2023.1097491
Huang, H., Huang, H., Xia, Z., Yang, Y., Jiang, X., Huang, C. (2023). Sequencing, Functional Annotation, and Interaction Prediction of mRNAs and Candidate Long Noncoding RNAs Originating from Tea Leaves During Infection by the Fungal Pathogen Causing Tea Leaf Spot, Didymella bellidis. Plant Dis. 107, 2830–2834. doi: 10.1094/PDIS-05-22-1240-A
Jampala, P., Garhewal, A., Lodha, M. (2021). Functions of long non-coding RNA in Arabidopsis thaliana. Plant Signal Behav. 16, 9–21. doi: 10.1080/15592324.2021.1925440
Jarroux, J., Morillon, A., Pinskaya, M. (2017). History, discovery, and classification of lncRNAs. Adv. Exp. Med. Biol. 1008, 1–46. doi: 10.1007/978-981-10-5203-3_1
Jha, U. C., Nayyar, H., Roychowdhury, R., Prasad, P. V. V., Parida, S. K., Siddique, K. H. M. (2023). Non-coding RNAs (ncRNAs) in plant: Master regulators for adapting to extreme temperature conditions. Plant Physiol. Biochem. 205, 108–164. doi: 10.1016/j.plaphy.2023.108164
Jiang, L., Yue, M., Liu, Y., Zhang, N., Lin, Y., Zhang, Y. (2023). A novel R2R3-MYB transcription factor FaMYB5 positively regulates anthocyanin and proanthocyanidin biosynthesis in cultivated strawberries (Fragaria×ananassa). Plant Biotechnol. J. 21, 1140–1158. doi: 10.1111/pbi.14024
Jiang, N., Cui, J., Shi, Y., Yang, G., Zhou, X., Hou, X. (2019). Tomato lncRNA23468 functions as a competing endogenous RNA to modulate NBS-LRR genes by decoying miR482b in the tomato-Phytophthora infestans interaction. Hortic. Res. 6, 28–38. doi: 10.1038/s41438-018-0096-0
Jin, Y., Ivanov, M., Dittrich, A. N., Nelson, A. D., Marquardt, S. (2023). LncRNA FLAIL affects alternative splicing and represses flowering in Arabidopsis. EMBO J. 42, e110921. doi: 10.15252/embj.2022110921
Kumar, K., Gambhir, G., Dass, A., Tripathi, A. K., Singh, A., Jha, A. K. (2020). Genetically modified crops: current status and future prospects. Planta. 251, 91–117. doi: 10.1007/s00425-020-03372-8
Lemos, S. M. C., Fonçatti, L. F. C., Guyot, R., Paschoal, A. R., Domingues, D. S. (2020). Genome-wide screening and characterization of non-coding RNAs in coffea canephora. Noncoding RNA. 6, 39–53. doi: 10.3390/ncrna6030039
Li, A. M., Liao, F., Wang, M., Chen, Z. L., Qin, C. X., Huang, R. Q. (2023). Transcriptomic and proteomic landscape of sugarcane response to biotic and abiotic stressors. Int. J. Mol. Sci. 24, 8913–8929. doi: 10.3390/ijms24108913
Li, C., Lai, X., Yu, X., Xiong, Z., Chen, J., Lang, X. (2023). Plant long noncoding RNAs: Recent progress in understanding their roles in growth, development, and stress responses. Biochem. Biophys. Res. Commun. 671, 270–277. doi: 10.1016/j.bbrc.2023.05.103
Li, C., Nong, W., Zhao, S., Lin, X., Xie, Y., Cheung, M. Y. (2022). Differential microRNA expression, microRNA arm switching, and microRNA:long noncoding RNA interaction in response to salinity stress in soybean. BMC Genomics 23, 65–77. doi: 10.1186/s12864-022-08308-y
Li, J., Cui, J., Dai, C., Liu, T., Cheng, D., Luo, C. (2020). Whole-Transcriptome RNA Sequencing Reveals the Global Molecular Responses and CeRNA Regulatory Network of mRNAs, lncRNAs, miRNAs and circRNAs in Response to Salt Stress in Sugar Beet (Beta vulgaris). Int. J. Mol. Sci. 22, 289–301. doi: 10.3390/ijms22010289
Li, J., Ma, W., Zeng, P., Wang, J., Geng, B., Yang, J. (2015). LncTar: a tool for predicting the RNA targets of long noncoding RNAs. Brief Bioinform. 16, 806–812. doi: 10.1093/bib/bbu048
Li, R., Fu, D., Zhu, B., Luo, Y., Zhu, H. (2018). CRISPR/Cas9-mediated mutagenesis of lncRNA1459 alters tomato fruit ripening. Plant J. 94, 513–524. doi: 10.1111/tpj.13872
Li, W., Chen, Y., Wang, Y., Zhao, J., Wang, Y. (2022). Gypsy retrotransposon-derived maize lncRNA GARR2 modulates gibberellin response. Plant J. 110, 1433–1446. doi: 10.1111/tpj.15748
Li, X., Shahid, M. Q., Wen, M., Chen, S., Yu, H., Jiao, Y. (2020). Global identification and analysis revealed differentially expressed lncRNAs associated with meiosis and low fertility in autotetraploid rice. BMC Plant Biol. 20, 82–100. doi: 10.1186/s12870-020-2290-0
Li, X., Yang, Q., Liao, X., Tian, Y., Zhang, F., Zhang, L. (2022). A natural antisense RNA improves chrysanthemum cold tolerance by regulating the transcription factor DgTCP1. Plant Physiol. 190, 605–620. doi: 10.1093/plphys/kiac267
Li, Y., Tan, Z., Zeng, C., Xiao, M., Lin, S., Yao, W. (2023). Regulation of seed oil accumulation by lncRNAs in Brassica napus. Biotechnol. Biofuels Bioprod. 16, 22–40. doi: 10.1186/s13068-022-02256-1
Liang, N., Cheng, D., Zhao, L., Lu, H., Xu, L., Bi, Y. (2022). Identification of the genes encoding B3 domain-containing proteins related to vernalization of beta vulgaris. Genes (Basel). 13, 2217–2235. doi: 10.3390/genes13122217
Liao, X., Wang, J., Zhu, S., Xie, Q., Wang, L., Yu, H. (2020). Transcriptomic and functional analyses uncover the regulatory role of lncRNA000170 in tomato multicellular trichome formation. Plant J. 104, 18–29. doi: 10.1111/tpj.14902
Liu, F., Xu, Y., Chang, K., Li, S., Liu, Z., Qi, S. (2019). The long noncoding RNA T5120 regulates nitrate response and assimilation in Arabidopsis. New Phytol. 224, 117–131. doi: 10.1111/nph.16038
Liu, W., Cui, J., Luan, Y. (2022). Overexpression of lncRNA08489 enhances tomato immunity against Phytophthora infestans by decoying miR482e-3p. Biochem. Biophys. Res. Commun. 587, 36–41. doi: 10.1016/j.bbrc.2021.11.079
Liu, X. Q., Li, B. X., Zeng, G. R., Liu, Q. Y., Ai, D. M. (2019). Prediction of long non-coding RNAs based on deep learning. Genes (Basel). 10, 273–288. doi: 10.3390/genes10040273
Liu, X., Li, D., Zhang, D., Yin, D., Zhao, Y., Ji, C. (2018). A novel antisense long noncoding RNA, TWISTED LEAF, maintains leaf blade flattening by regulating its associated sense R2R3-MYB gene in rice. New Phytol. 218, 774–788. doi: 10.1111/nph.15023
Lu, Q., Guo, F., Xu, Q., Cang, J. (2020). LncRNA improves cold resistance of winter wheat by interacting with miR398. Funct. Plant Biol. 47, 544–557. doi: 10.1071/FP19267
Ma, B., Zhang, A., Zhao, Q., Li, Z., Lamboro, A., He, H. (2021). Genome-wide identification and analysis of long non-coding RNAs involved in fatty acid biosynthesis in young soybean pods. Sci. Rep. 11, 7603–7619. doi: 10.1038/s41598-021-87048-7
Ma, X., Zhang, X., Traore, S. M., Xin, Z., Ning, L., Li, K. (2020). Genome-wide identification and analysis of long noncoding RNAs (lncRNAs) during seed development in peanut (Arachis hypogaea L.). BMC Plant Biol. 20, 192–205. doi: 10.1186/s12870-020-02405-4
Madhawan, A., Bhunia, R. K., Kumar, P., Sharma, V., Sinha, K., Fandade, V. (2023). Interaction between long noncoding RNA (lnc663) and microRNA (miR1128) regulates PDAT-like gene activity in bread wheat (Triticum aestivum L.). Plant Physiol. Biochem. 203, 108040. doi: 10.1016/j.plaphy.2023.108040
Mattick, J. S., Amaral, P. P., Carninci, P., Carpenter, S., Chang, H. Y., Chen, L. L. (2023). Long non-coding RNAs: definitions, functions, challenges and recommendations. Nat. Rev. Mol. Cell Biol. 24, 430–447. doi: 10.1038/s41580-022-00566-8
Morohashi, K., Xie, Z., Grotewold, E. (2009). Gene-specific and genome-wide ChIP approaches to study plant transcriptional networks. Methods Mol. Biol. 553, 3–12. doi: 10.1007/978-1-60327-563-7_1
Nojima, T., Proudfoot, N. J. (2022). Mechanisms of lncRNA biogenesis as revealed by nascent transcriptomics. Nat. Rev. Mol. Cell Biol. 23, 389–406. doi: 10.1038/s41580-021-00447-6
Palos, K., Yu, L., Railey, C. E., Nelson Dittrich, A. C., Nelson, A. D. L. (2023). Linking discoveries, mechanisms, and technologies to develop a clearer perspective on plant long noncoding RNAs. Plant Cell. 35, 1762–1786. doi: 10.1093/plcell/koad027
Park, Y. B., Kim, J. M. (2023). Identification of long non-coding RNA-mRNA interactions and genome-wide lncRNA annotation in animal transcriptome profiling. J. Anim. Sci. Technol. 65, 293–310. doi: 10.5187/jast.2023.e17
Qin, T., Xiong, L. (2019). Subcellular localization and functions of plant lncRNAs in drought and salt stress tolerance. Methods Mol. Biol. 1933, 173–186. doi: 10.1007/978-1-4939-9045-0_9
Qin, T., Zhao, H., Cui, P., Albesher, N., Xiong, L. (2017). A nucleus-localized long non-coding RNA enhances drought and salt stress tolerance. Plant Physiol. 175, 1321–1336. doi: 10.1104/pp.17.00574
Quek, X. C., Thomson, D. W., Maag, J. L., Bartonicek, N., Signal, B., Clark, M. B. (2015). lncRNAdb v2.0: expanding the reference database for functional long noncoding RNAs. Nucleic Acids Res. 43, 168–173. doi: 10.1093/nar/gku988
Rao, S., Balyan, S., Bansal, C., Mathur, S. (2022). An integrated bioinformatics and functional approach for miRNA validation. Methods Mol. Biol. 2408, 253–281. doi: 10.1007/978-1-0716-1875-2_17
Rhoads, A., Au, K. F. (2015). PacBio sequencing and its applications. Genomics Proteomics Bioinf. 13, 278–289. doi: 10.1016/j.gpb.2015.08.002
Sanbonmatsu, K. (2022). Getting to the bottom of lncRNA mechanism: structure-function relationships. Mamm Genome. 33, 343–353. doi: 10.1007/s00335-021-09924-x
Sheng, N., Huang, L., Gao, L., Cao, Y., Xie, X., Wang, Y. (2023). A survey of computational methods and databases for lncRNA-miRNA interaction prediction. IEEE/ACM Trans. Comput. Biol. Bioinform. 20, 2810–2826. doi: 10.1109/TCBB.2023.3264254
Song, B., Ning, W., Wei, D., Jiang, M., Zhu, K., Wang, X. (2023). Plant genome resequencing and population genomics: Current status and future prospects. Mol. Plant 16, 1252–1268. doi: 10.1016/j.molp.2023.07.009
Su, C., Wang, Z., Cui, J., Wang, Z., Wang, R., Meng, J. (2023). Sl-lncRNA47980, a positive regulator affects tomato resistance to Phytophthora infestans. Int. J. Biol. Macromol. 248, 125824. doi: 10.1016/j.ijbiomac.2023.125824
Summanwar, A., Basu, U., Kav, N. N. V., Rahman, H. (2021). Identification of lncRNAs in response to infection by Plasmodiophora brassicae in Brassica napus and development of lncRNA-based SSR markers. Genome. 64, 547–566. doi: 10.1139/gen-2020-0062
Sun, Y., Hao, P., Lv, X., Tian, J., Wang, Y., Zhang, X. (2020). A long non-coding apple RNA, MSTRG.85814.11, acts as a transcriptional enhancer of SAUR32 and contributes to the Fe-deficiency response. Plant J. 103, 53–67. doi: 10.1111/tpj.14706
Tan, X., Li, S., Hu, L., Zhang, C. (2020). Genome-wide analysis of long non-coding RNAs (lncRNAs) in two contrasting rapeseed (Brassica napus L.) genotypes subjected to drought stress and re-watering. BMC Plant Biol. 20, 81–100. doi: 10.1186/s12870-020-2286-9
Tang, X., Ji, L. (2023). Predicting Plant miRNA-lncRNA Interactions via a Deep Learning Method. IEEE Trans. Nanobioscience. 22, 728–733. doi: 10.1109/TNB.2023.3275178
Tang, Y., Qu, Z., Lei, J., He, R., Adelson, D. L., Zhu, Y. (2021). The long noncoding RNA FRILAIR regulates strawberry fruit ripening by functioning as a noncanonical target mimic. PloS Genet. 17, e1009461. doi: 10.1371/journal.pgen.1009461
Tao, X., Li, M., Zhao, T., Feng, S., Zhang, H., Wang, L. (2021). Neofunctionalization of a polyploidization-activated cotton long intergenic non-coding RNA DAN1 during drought stress regulation. Plant Physiol. 186, 2152–2168. doi: 10.1093/plphys/kiab179
Thiebaut, F., Rojas, C. A., Grativol, C., Calixto, E. P. D. R., Motta, M. R., Ballesteros, H. G. F. (2017). Roles of non-coding RNA in sugarcane-microbe interaction. Noncoding RNA. 3, 25–48. doi: 10.3390/ncrna3040025
van Dijk, E. L., Naquin, D., Gorrichon, K., Jaszczyszyn, Y., Ouazahrou, R., Thermes, C. (2023). Hernandez, C. Genomics in the long-read sequencing era. Trends Genet. 39, 649–671. doi: 10.1016/j.tig.2023.04.006
Verma, S., Attuluri, V. P. S., Robert, H. S. (2022). Transcriptional control of Arabidopsis seed development. Planta. 255, 90–108. doi: 10.1007/s00425-022-03870-x
Virciglio, C., Abel, Y., Rederstorff, M. (2021). Regulatory non-coding RNAs: an overview. Methods Mol. Biol. 2300, 3–9. doi: 10.1007/978-1-0716-1386-3_1
Waititu, J. K., Zhang, C., Liu, J., Wang, H. (2022). Plant non-coding rnas: origin, biogenesis, mode of action and their roles in abiotic stress. Int. J. Mol. Sci. 21, 1–22. doi: 10.3390/ijms21218401
Wan, S., Zhang, Y., Duan, M., Huang, L., Wang, W., Xu, Q. (2020). Integrated Analysis of Long Non-coding RNAs (lncRNAs) and mRNAs Reveals the Regulatory Role of lncRNAs Associated With Salt Resistance in Camellia sinensis. Front. Plant Sci. 11. doi: 10.3389/fpls.2020.00218
Wierzbicki, A. T., Blevins, T., Swiezewski, S. (2021). Long noncoding RNAs in plants. Annu. Rev. Plant Biol. 72, 245–271. doi: 10.1146/annurev-arplant-093020-035446
Wu, L., Liu, S., Qi, H., Cai, H., Xu, M. (2020). Research progress on plant long non-coding RNA. Plants (Basel). 9, 408–417. doi: 10.3390/plants9040408
Xiao, Y., Kang, B., Li, M., Xiao, L., Xiao, H., Shen, H. (2020). Transcription of lncRNA ACoS-AS1 is essential to trans-splicing between SlPsy1 and ACoS-AS1 that causes yellow fruit in tomato. RNA Biol. 17, 596–607. doi: 10.1080/15476286.2020.1721095
Xu, C., Li, J., Wang, H., Liu, H., Yu, Z., Zhao, Z. (2023). Whole-Transcriptome Sequencing Reveals a ceRNA Regulatory Network Associated with the Process of Periodic Albinism under Low Temperature in Baiye No. 1 (Camellia sinensis). Int. J. Mol. Sci. 24, 7162–7185. doi: 10.3390/ijms24087162
Xu, J., Fang, M., Li, Z., Zhang, M., Liu, X., Peng, Y. (2020). Third-Generation Sequencing Reveals LncRNA-Regulated HSP Genes in the Populus x canadensis Moench Heat Stress Response. Front. Genet. 11. doi: 10.3389/fgene.2020.00249
Xu, P., Li, H., Wang, X., Zhao, G., Lu., X., Dai, S. (2022). Integrated analysis of the lncRNA/circRNA-miRNA-mRNA expression profiles reveals novel insights into potential mechanisms in response to root-knot nematodes in peanut. BMC Genomics 23, 239–250. doi: 10.1186/s12864-022-08470-3
Yan, H., Zhou, H., Luo, H., Fan, Y., Zhou, Z., Chen, R. (2021). Characterization of full-length transcriptome in Saccharum officinarum and molecular insights into tiller development. BMC Plant Biol. 21, 228–239. doi: 10.1186/s12870-021-02989-5
Yang, J., Ariel, F., Wang, D. (2023). Plant long non-coding RNAs: biologically relevant and mechanistically intriguing. J. Exp. Bot. 74, 2364–2373. doi: 10.1093/jxb/erac482
Yang, W., Schuster, C., Prunet, N., Dong, Q., Landrein, B., Wightman, R. (2020). Visualization of protein coding, long noncoding, and nuclear RNAs by fluorescence in situ hybridization in sections of shoot apical meristems and developing flowers. Plant Physiol. 182, 147–158. doi: 10.1104/pp.19.00980
Yang, X., Liu, C., Niu, X., Wang, L., Li, L., Yuan, Q. (2022). Research on lncRNA related to drought resistance of Shanlan upland rice. BMC Genomics 23, 336–350. doi: 10.1186/s12864-022-08546-0
Yang, Z., Zhang, T., Han, S., Kusumanchi, P., Huda, N., Jiang, Y. (2021). Long noncoding RNA H19 - a new player in the pathogenesis of liver diseases. Transl. Res. 230, 139–150. doi: 10.1016/j.trsl.2020.11.010
Yang, Z., Zhang, Z., Li, J., Chen, W., Liu, C. (2024). CRISPRlnc: a machine learning method for lncRNA-specific single-guide RNA design of CRISPR/Cas9 system. Brief Bioinform. 25, bbae066. doi: 10.1093/bib/bbae066
Yoshimura, H., Matsuda, Y., Yamamoto, M., Kamiya, S., Ishiwata, T. (2018). Expression and role of long non-coding RNA H19 in carcinogenesis. Front. Biosci. (Landmark Ed). 23, 614–625. doi: 10.2741/4608
Yu, Y., Zhou., Y. F., Feng, Y. Z., He, H., Lian, J. P., Yang, Y. W. (2020). Transcriptional landscape of pathogen-responsive lncRNAs in rice unveils the role of ALEX1 in jasmonate pathway and disease resistance. Plant Biotechnol. J. 18, 679–690. doi: 10.1111/pbi.13234
Zhang, X., Shen, J., Xu, Q., Dong, J., Song, L., Wang, W. (2021). Long noncoding RNA lncRNA354 functions as a competing endogenous RNA of miR160b to regulate ARF genes in response to salt stress in upland cotton. Plant Cell Environ. 44, 3302–3321. doi: 10.1111/pce.14133
Zhang, X., Du, M., Yang, Z., Wang, Z., Lim, K. J. (2023). Biogenesis, mode of action and the interactions of plant non-coding RNAs. Int. J. Mol. Sci. 24, 10664–10678. doi: 10.3390/ijms241310664
Zhang, M., Cui, G., Bai, X., Ye, Z., Zhang, S., Xie, K. (2021). Regulatory network of preharvest sprouting resistance revealed by integrative analysis of mRNA, noncoding RNA, and DNA methylation in wheat. J. Agric. Food Chem. 69, 4018–4035. doi: 10.1021/acs.jafc.1c00050
Zhang, L., Liu, J., Cheng, J., Sun, Q., Zhang, Y., Liu, J. (2022). lncRNA7 and lncRNA2 modulate cell wall defense genes to regulate cotton resistance to Verticillium wilt. Plant Physiol. 189, 264–284. doi: 10.1093/plphys/kiac041
Zhang, L., Li, Y., Yang, J., Huang, H., Lu, Q., Zhao, J. (2023). Genome-wide identification of drought-responsive microRNAs and their target genes in Chinese jujube by deep sequencing. Genes Genomics 45, 231–245. doi: 10.1007/s13258-022-01274-5
Zhang, A., Liu, H., Yao, D. (2023). Analysis of lncRNA43234-associated ceRNA network reveals oil metabolism in soybean. J. Agric. Food Chem. 71, 9815–9825. doi: 10.1021/acs.jafc.3c00993
Zhang, J., Gan, L., Sun, P., Wang, J., Li, D., Cao, Y. (2022). The long non-coding RNA LNC_000397 negatively regulates PRRSV replication through induction of interferon-stimulated genes. Virol. J. 19, 40–53. doi: 10.1186/s12985-022-01761-x
Zhang, H., Guo, H., Hu, W., Ji, W. (2020). The emerging role of long non-coding RNAs in plant defense against fungal stress. Int. J. Mol. Sci. 21, 2689–2703. doi: 10.3390/ijms21082659
Zhang, Y., Wang, S., Li, W., Wang, S., Hao, L., Xu, C. (2022). A long noncoding RNA HILinc1 enhances pear thermotolerance by stabilizing PbHILT1 transcripts through complementary base pairing. Commun. Biol. 5, 1134–1147. doi: 10.1038/s42003-022-04010-7
Zhao, X., Gan, L., Yan, C., Li, C., Sun, Q., Wang, J. (2019). Genome-wide identification and characterization of long non-coding RNAs in peanut. Genes (Basel). 10, 536–548. doi: 10.3390/genes10070536
Zhou, D., Zhao, S., Zhou, H., Chen, J., Huang, L. A. (2023). lncRNA bra-miR156HG regulates flowering time and leaf morphology as a precursor of miR156 in Brassica campestris and Arabidopsis thaliana. Plant Sci. 337, 111889. doi: 10.1016/j.plantsci.2023.111889
Zhu, C., Zhang, S., Fu, H., Zhou, C., Chen, L., Li, X. (2019). Transcriptome and phytochemical analyses provide new insights into long non-coding RNAs modulating characteristic secondary metabolites of oolong tea (Camellia sinensis) in solar-withering. Front. Plant Sci. 10. doi: 10.3389/fpls.2019.01638
Zhu, J., Fu., H., Wu, Y., Zheng, X. (2013). Function of lncRNAs and approaches to lncRNA-protein interactions. Sci. China Life Sci. 56, 876–885. doi: 10.1007/s11427-013-4553-6
Zhu, M., Dong, Q., Bing, J., Songbuerbatu, Zheng, L., Dorjee, T. (2023). Combined lncRNA and mRNA Expression Profiles Identified the lncRNA-miRNA-mRNA Modules Regulating the Cold Stress Response in Ammopiptanthus nanus. Int. J. Mol. Sci. 24, 6502. doi: 10.3390/ijms24076502
Zou, C., Guo, Z., Zhao, S., Chen, J., Zhang, C., Han, H. (2023). Genome-wide analysis of long non-coding RNAs in sugar beet (Beta vulgaris L.) under drought stress. Front. Plant Sci. 14. doi: 10.3389/fpls.2023.1118011
Keywords: lncRNAs, plant development, biological function, molecular mechanism, crops
Citation: Zhang A, Pi W, Wang Y, Li Y, Wang J, Liu S, Cui X, Liu H, Yao D and Zhao R (2024) Update on functional analysis of long non-coding RNAs in common crops. Front. Plant Sci. 15:1389154. doi: 10.3389/fpls.2024.1389154
Received: 21 February 2024; Accepted: 08 May 2024;
Published: 30 May 2024.
Edited by:
Agnieszka Ludwików, Adam Mickiewicz University in Poznań, PolandReviewed by:
Mingyang Quan, Beijing Forestry University, ChinaNing Li, Xinjiang Academy of Agricultural Sciences, China
Copyright © 2024 Zhang, Pi, Wang, Li, Wang, Liu, Cui, Liu, Yao and Zhao. This is an open-access article distributed under the terms of the Creative Commons Attribution License (CC BY). The use, distribution or reproduction in other forums is permitted, provided the original author(s) and the copyright owner(s) are credited and that the original publication in this journal is cited, in accordance with accepted academic practice. No use, distribution or reproduction is permitted which does not comply with these terms.
*Correspondence: Rengui Zhao, emhhb3Jlbmd1aUBzaW5hLmNvbQ==; Dan Yao, ZHlhb0BqbGF1LmVkdS5jbg==; Huijing Liu, aHVpamluZ2xpdUBqbGF1LmVkdS5jbg==