- 1Sorbonne Université, CNRS UMR7622, Institut de Biologie Paris-Seine-Laboratoire de Biologie du Développement (IBPS-LBD), Paris, France
- 2Muséum National d’Histoire Naturelle, Unité Molécules de Communication et Adaptation des Micro-organismes, UMR 7245, Paris, France
- 3Sorbonne Université, Faculté des Sciences et Ingénierie, UFR 927, Paris, France
- 4Seedlab, Novalliance, Zone Anjou Actiparc, Longué-Jumelles, France
Seed germination is a major determinant of plant development and final yield establishment but strongly reliant on the plant’s abiotic and biotic environment. In the context of global climate change, classical approaches to improve seed germination under challenging environments through selection and use of synthetic pesticides reached their limits. A currently underexplored way is to exploit the beneficial impact of the microorganisms associated with plants. Among plant microbiota, endophytes, which are micro-organisms living inside host plant tissues without causing any visible symptoms, are promising candidates for improving plant fitness. They possibly establish a mutualistic relationship with their host, leading to enhanced plant yield and improved tolerance to abiotic threats and pathogen attacks. The current view is that such beneficial association relies on chemical mediations using the large variety of molecules produced by endophytes. In contrast to leaf and root endophytes, seed-borne fungal endophytes have been poorly studied although they constitute the early-life plant microbiota. Moreover, seed-borne fungal microbiota and its metabolites appear as a pertinent lever for seed quality improvement. This review summarizes the recent advances in the identification of seed fungal endophytes and metabolites and their benefits for seed biology, especially under stress. It also addresses the mechanisms underlying fungal effects on seed physiology and their potential use to improve crop seed performance.’
Introduction
Endophytic microorganisms are part of the plant microbiome and reside transiently or permanently within plant tissues without causing disease symptoms (Wilson, 1995). Endophytic fungi mainly belong to the Ascomycota or Basidiomycota (Rashmi, 2019) and, together with bacteria, constitute the most abundant, diverse and ubiquitous group of endophytes. Their association with plants is attested since Devonian (Krings et al., 2007) and they have been detected in most plants studied so far and in a variety of plant organs including leaves, stems, roots, flowers, fruits and seeds (Stone et al., 2004). The classification of endophytic fungi integrates their phylogeny, their host range, extent of tissue colonization and transmission mode (Rodriguez et al., 2009). Their transmission is particularly important for the building of endophytic communities and their maintenance over space and time. Indeed, horizontal transmission assures constant supply of endophytes for (re)inoculation from plant environment whereas vertical transmission allows the transfer of endophytic populations from mother plants to their progeny via the seeds and possibly the maintenance of endophytic microbiome composition across generations (Bright and Bulgheresi, 2010; Abdelfattah et al., 2022).
A particular attention has been paid to endophytic fungi considering the numerous services they can provide to plants and their high potential for application in agriculture. Indeed abundant literature reports the capacity of fungal endophytes to promote plant growth and to improve their tolerance towards abiotic and biotic stresses, in exchange of nutriment supply and shelter (Baron and Rigobelo, 2022; Verma et al., 2022). As for seed-borne endophytic fungi, evidence also points to their ability to promote seed germination and early seedling growth, and possibly impact the whole plant development and response to environmental cues (Li et al., 2019). Interestingly, the positive effects of fungal endophytes largely rely on bioactive molecules they produce and that stimulate plant growth, and participate in adaptive responses or immunity (Prado et al., 2012; Lugtenberg et al., 2016). Indeed, the chemical repertoire of fungal endophytes is not only exceptionally diverse in itself, but also shaped by their environment within the plant, which makes these fungi a unique reservoir of bioactive molecules.
Seeds are unique structures found in Gymnosperms and Angiosperms that ensure their sustainability and dissemination, which is critical for species survival and spreading in ecosystems. They also constitute the income and outcome of major crop productions, and seed performance, including high germination rate, vigor or longevity, is crucial for plantlet establishment and final yield (Ellis, 1992). Due to their sensitivity towards environmental stresses, seed germination and possibly other seed traits are strongly jeopardized by ongoing climate change (Finch-Savage and Bassel, 2016). Moreover, global warming will enhance plant diseases, lowering yields and impairing food safety (Raza and Bebber, 2022). Because of on-going environmental policies and increasing concern for sustainable development, environmental-safe strategies to improve seed performance and resistance to pathogens are urgently required. In this view, the valorization of bio-sourced molecules as biostimulants, i.e. to promote plant growth and plant stress tolerance, or for the biocontrol of pathogens, is a promising strategy. Fungal endophytes, especially those naturally hosted in seeds, therefore appear as a valuable and original source of bioactive molecules. This review will bring an update on seed-borne fungal endophytes and their potential valorization to improve seed traits, i.e. dormancy, germination, vigor and longevity, under optimal or stress conditions, and seed tolerance towards pathogens and pests.
Seed-borne endophytic fungi: population diversity and dynamics
Embodying bridges between successive generations, seeds have a central role in the conservation and transmission of the endophytic microbiome to the next generation. Recent studies have described the microbiome of crop and non-crop seeds using metagenomics approaches (Wassermann et al., 2019; Bintarti et al., 2022; Simonin et al., 2022). They highlight a high proportion of fungi in seed microbiota compared to other tissues, possibly reflecting the high capacity of fungal species to adapt to seed environment (Simonin et al., 2022). They also point out a strong diversity among plant species, between seeds of the same plant and even within the same fruit (Bintarti et al., 2022; Simonin et al., 2022). Indeed, seed endophytic fungal communities are not only dependent on host genetics, but are further shaped by environmental factors (Klaedtke et al., 2016; Wassermann et al., 2019; Franić et al., 2020; Bintarti et al., 2022; Philpott et al., 2023). Despite this variability, a handful of genera, e.g. Alternaria, Phoma, Cladosporium, Fusarium, Xylaria, Penicillium or Aspergillus, that are abundant and ubiquitously found in crops and wild species, emerge as the core endophytic community of seeds (Samreen et al., 2021; Simonin et al., 2022). In addition to these, Epichloe genus is widely present among Poacea (grass) seeds (Stone et al., 2004). Beside this core population, a diversity of additional endophytic fungal species have also been detected in seeds, that represent a minority and are highly flexible among plant species (Kluger et al., 2008; Klaedtke et al., 2016; Billingsley Tobias et al., 2017; Hill et al., 2021; Mertin et al., 2022; Simonin et al., 2022). Endophytic fungi from both core and flexible populations can improve seed performance (Table 1), which leaves open the relative contribution and functions of these fungal subgroups.
As presented in Figure 1, seed endophytic communities are partly inherited from the microbiota of the mother plant via vertical transmission, through asexual (vascular tissues, intercellular spaces) and possibly sexual (gametophytes) routes (Abdelfattah et al., 2022). Endophytic fungi can also be acquired from seed environment by horizontal transmission, from air and rain during seed development and from soil after seed dispersal (U’Ren et al., 2009; Nelson, 2018). In this last case, the seed coat that protects seeds and prevents the penetration of pathogens represents a barrier and a harsh environment for endophyte penetration and survival. Moreover, the low diversity of endophytic fungi observed in seeds compared to other plant tissues may result from interactions among seed-transmitted microorganisms including endophytes so as plant defense mechanisms (Newcombe et al., 2018). After dispersal, endophytic fungal populations further evolve depending on seed conservation. On the one hand, soil seed banks can be infected by soil-borne microbes and an increased fungal diversity is observed over time (Gallery et al., 2007; U’Ren et al., 2009). On the other hand, the viability of fungal endophytes can be reduced during post-harvest storage of dry seeds depending on the storage temperature and humidity (Rolston et al., 1986; Gundel et al., 2009; Lane et al., 2018). A recent study carried out on seeds from wild banana relatives conserved in seed banks also suggests that loss of seed viability correlates with specific modifications of the fungal endophyte community (Hill et al., 2021). Moreover, post-harvest treatments with fungicides (Hill and Brown, 2000; Leyronas et al., 2006) but also insecticides (Nettles et al., 2016; Solanki et al., 2019) reduce seed endophytic fungi populations. When germination occurs, the growth of endophytes is reinitiated and they are mobilized to colonize plantlets (Johnston-Monje et al., 2021). A further reduction of seed-borne endophyte diversity is therefore observed in the seedlings, due to differences in the growth rate among endophytic fungi (Ganley and Newcombe, 2006; Barret et al., 2015). As recently suggested, seed-borne endophytes may subsequently colonize specific organs (root, stem) through selective mechanisms currently unknown (Abdelfattah et al., 2022). Beside their importance for seed biology, seed endophytes could therefore also play critical functions in early plantlet establishment.
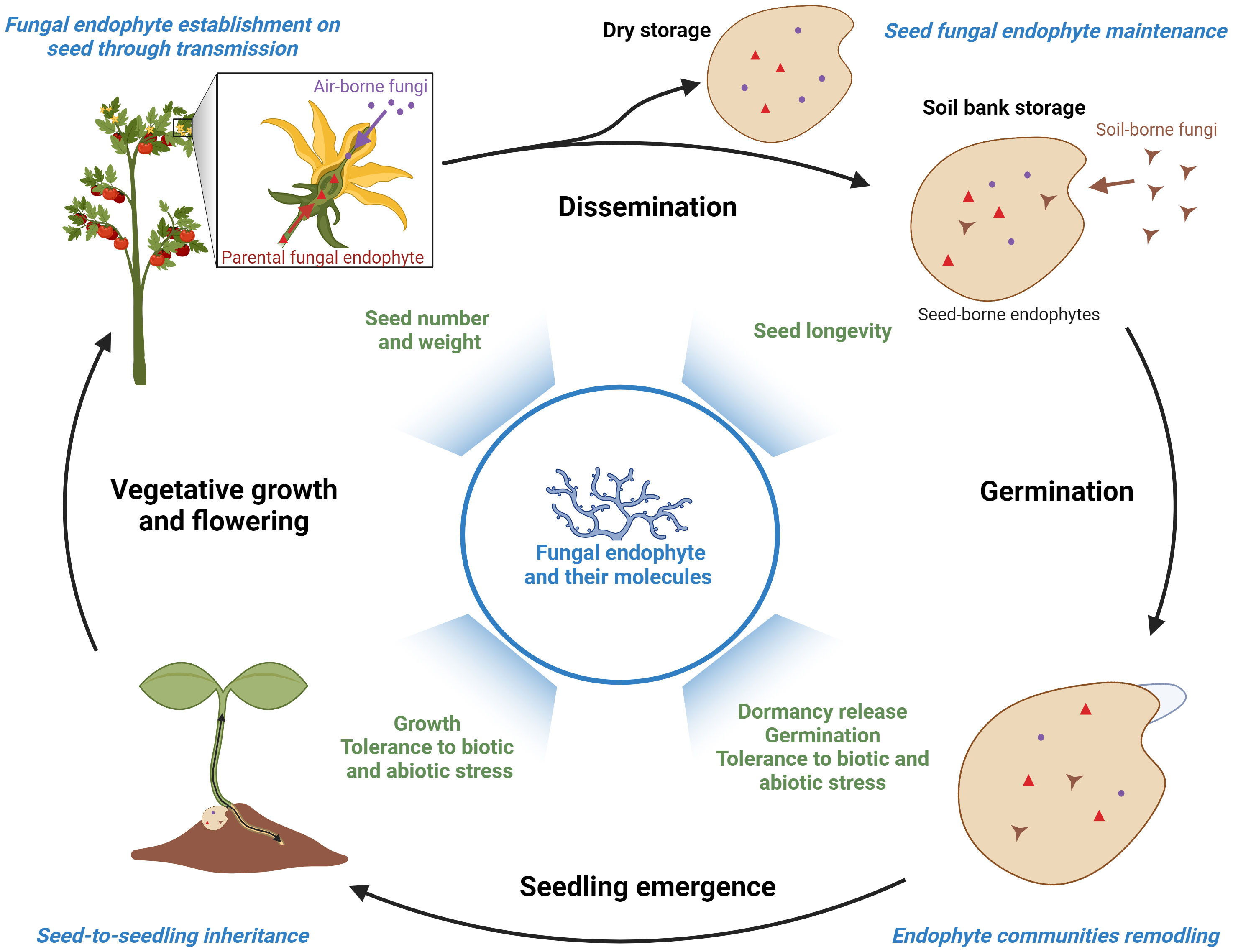
Figure 1 Origins and biological functions of fungal endophytes during seed-to-seed cycle. Seed fungal endophytes can be inherited from the mother plant (red labels) by vertical transmission during the early stages of seed development or acquired from air-borne (purple labels) and soil-borne (brown labels) populations before, during or after seed dispersal. During germination and early seedling development, a portion of the seed-borne endophytes migrate towards the emerging plantlets (vertical transmission). Major stages of endophytic community acquisition, maintenance and dynamics are highlighted in blue. The benefits of endophytes and their bioactive molecules for seed and seedling performance are indicated in green. Created with BioRender.com.
Seed-borne endophytic fungi: a high potential to improve seed performance
Numerous studies have reported beneficial effects of fungal endophytes on different aspects of seed biology, i.e. seed development, germination (including dormancy release) under optimal or stress conditions and longevity (Table 1 and references therein). These studies have been carried out on both crops, e.g. tomato, wheat or soybean, and non-crop, e.g. Achnatherum inebrians seeds, using core or flexible endophytic fungi. Although fragmented, they provide elements to understand how endophytic fungi can modify seed physiology and improve seed performance. The best examples come from studies performed on Epichloe spp. (anamorph genus Neotyphodium spp., family Clavicipitaceae), which are obligate symbionts of cool-season grasses and strictly seed transmitted (Zhang et al., 2010; Ma et al., 2015; Chen et al., 2016; Bao et al., 2019; Ahmad et al., 2020; Chen et al., 2021). They interact mutualistically with their hosts, promoting growth, reproduction and resistance to pests, mainly by producing alkaloids (Kuldau and Bacon, 2008). Epichloë infection enhances Achnatherum inebrians germination rate under optimal and stress, e.g. extreme temperature, salt stress, extreme pH or heavy metals, conditions (Zhang et al., 2010; Chen et al., 2016; Bao et al., 2019; Ahmad et al., 2020; Chen et al., 2021). It also promotes seed dormancy release (Chen et al., 2021). Dormancy is a critical parameter for seed survival in nature, avoiding seed germination under stress, and homogeneity and germination speed in agriculture. A general mechanism through which Epichloë, and other fungal endophytes such as Penicilium sp. (Hubbard et al., 2012; Vujanovic et al., 2016; Shearin et al., 2018), Cladosporium cladosporioides (Qin et al., 2016) or Fusarium verticillioides (Radhakrishnan et al., 2013), promote seed germination and dormancy release in these different contexts is likely the modification of hormonal equilibrium. Indeed, higher and lower content of hormones promoting [gibberellins (GA), auxin] or inhibiting germination [Abscissic acid (ABA)], are respectively measured in endophytic seeds upon imbibition (Radhakrishnan et al., 2013; Vujanovic et al., 2016; Chen et al, 2021). The ABA/GA balance is critical for seed germination and essentially regulated at the transcriptional level (Carrera-Castaño et al., 2020). Endophytic fungi might therefore modulate hormone-related gene expression in seeds, as reported for Epichloë in Achnatherum inebrians plants (Zhao et al., 2021). Alternatively, Epichloë endophytes could interact with hormonal balance through loline alkaloids production, which have been suggested to participate in promoting growth either directly or indirectly via the modulation of hormones, such as polyamines, with which they share precursor amino acids (Schardl et al., 2007). However, no results demonstrate the direct role of alkaloid on seed biology so far. Epichloë infection also triggers important modifications of the seed metabolome (Zhang et al, 2019; Liang et al, 2023). Beside the accumulation of alkaloids, changes in the contents of purine and amino acid derivatives, lipids and sugars have been reported (Zhang et al., 2019; Chen et al., 2021; Liang et al., 2023). The comparison of metabolomic and transcriptomic data suggest that Epichloë infection affects seed metabolism at least partly through transcriptional regulation (Chen et al., 2016; Rahnama et al., 2023). As previously shown, metabolic resumption is critical for efficient germination and fungal endophytes could participate in this process (Rosental et al., 2014).
A major outcome of seed infection by endophytic fungi is the improvement of seed germination under abiotic stress conditions (Hubbard et al., 2012; Radhakrishnan et al., 2013). In this context, metabolites accumulated in Epichloë-infected seeds could participate in a better tolerance to stress at the germination stage, as recently proposed in root and leaves (Hou et al., 2021; Liu et al., 2021). For instance, Li et al. (2020) reported that seeds infected with Epichloë accumulated higher contents of proline and soluble sugars when conserved in sub-optimal conditions, leading to a prolonged longevity. In addition, various seed species infected with endophytic fungi present a higher level of antioxidant defense (Zhang et al., 2010; Zhang et al., 2012; Ma et al., 2015; Li et al., 2020; Wang et al., 2020). Reactive oxygen species (ROS) content in seeds is a key factor for seed capacity to germinate (Bailly, 2019). As shown by Li et al. (2020), under unfavorable conditions, seeds infected with Epichloë exhibited a higher level of antioxidant activities, e.g. superoxide dismutase, ascorbate peroxidase, correlated with a lower ROS content and limited oxidative damages. Further evidence of ROS scavenging by Epichloë is the correlation between loline produced by the fungal endophyte and the production of the antioxidant molecule tocochromanol in Lolium multiflorum seeds (Gundel et al., 2018). From the numerous evidences obtained in vegetative organs (reviewed by Chen et al, 2022), it is expected that endophytic fungi modulate seed tolerance to stress through the modification of gene expression. So far, transcriptomic analyses have only been performed in cold-stressed Achnatherum inebrians seeds infected with Epichloë (Chen et al., 2016). In this context, seed infection impacts the expression of more than 150 genes, including stress response genes involved in protein folding, ROS scavenging and membrane lipid remodeling, that participate in cold tolerance (Chen et al., 2016). A generalization of such approach to multiple seed and endophyte species and stress conditions are now needed to identify common and specific transcriptomic signatures and associate them to stress tolerance at the germination stage.
Although less investigated, seed formation and yield are stimulated by endophytic fungi (Rice et al., 1990). This might reflect the improvement of nutrient translocation from the mother plant to the developing seeds by endophytic fungi. The positive effect of endophytes on seed number and weight is particularly significant for plants exposed to abiotic stresses (Hubbard et al., 2014; Parmar et al., 2022). Strikingly, the infection during seed formation on the mother plant also imprints seed tolerance to stress after release, at the germination stage (Hubbard et al., 2014). The transgenerational transmission of stress tolerance is associated with specific epigenetic regulations. Forte et al., 2023 recently evidenced that Epichloë infection triggers specific modifications of Lolium perenne DNA methylation. Whether it participates to the maintenance of stress tolerance in seeds over generation is currently unknown but provides a new angle to tackle seed-borne endophytic fungi functions.
Pathogen and feeder attacks are major threats for seed germination and seed endophytic fungi provide protection against a wide range of bio-aggressors (Ma et al., 2015; Li et al., 2017). Interestingly, protection by seed-borne endophytes frequently extends to later development stages following plantlet emergence (Ma et al., 2015). Protective mechanisms include the production of antimicrobial compounds, e.g. alkaloids, terpenoids or cell wall-degrading enzymes, by the endophytes, so as the activation of plant defense mechanisms through the stimulation of plant salicylic or jasmonic acid production (Schmid et al., 2017; Kou et al., 2021). Epichloë endophytes have been highlighted for their antifungal activity provided by the constitutive production of antifungal molecules (Niones and Takemoto, 2014; Fernando et al., 2020). Beside Epichloë, several seed-borne fungal endophytes, e.g. Penicillium crustosum, Sarocladium zeae, Sarocladium strictum or Lecanicillium lecanii have been reported to produce antimicrobial compounds (Valente et al., 2013; Shen et al., 2014; Błaszczyk et al., 2021; Kim et al., 2022). Nevertheless, their role in mitigating pathogen attacks in seeds and their mode of action is still not fully known. Molecules identified in seeds include loline (Justus et al., 1997; Gundel et al., 2018) and ergot alkaloids (Ahimsa-Müller et al., 2007), peramine (Ball et al., 1997a) and lolitrem B (Ball et al., 1997b). Although unknown in seeds, their role can likely be extrapolated from that in vegetative organs. They could participate in plant defense against herbivores as reported for Epichloë lolines and ergot alkaloids, indole diterpenoids (lolitrems) and pyrrolopyrazines (peramine) (Bush et al., 1997). The neurotropic activities of lolines, and the activity of peramine as a feeding deterrent, can significantly enhance competitiveness of grasses housing such alkaloid-producing endophytes (Bush et al., 1997). However, loline alkaloids exhibit a broader range and more overt toxicity to insects than peramine (Schardl et al., 2004). Apart from Epichloë, the seed endophyte Undifilum oxytropsis produces swainsonine, an alkaloid bioactive on neurological functions, and that protects host plant from herbivores (Oldrup et al., 2010; Cook et al., 2011). A major limitation for the use of such endophytes to control feeder attacks is the anti-vertebrate activities of some of their metabolites, i.e. indole diterpene and ergot alkaloids, that are responsible for livestock intoxication (Bacon, 1995). Epichloë strains altered in alkaloid production that retain protection potential, with minimal negative effects on livestock, have therefore been selected. These strains produce neither lolitrem B nor ergovaline and the sole production of peramine provides a defense against major pest insects. They are now commercially available and commonly used by farmers to improve pasture performance in agrosystems (Eady, 2021).
Future developments towards endophyte-based solutions in seed treatments
Recent progress based on metagenomics uncovered the huge diversity of endophytic fungal communities in seeds. This knowledge paves the way for engineering seed endophytic microbiota to improve seed performance, in particular under stress conditions. In this view, endophytic fungal strains selected from tolerant seeds may be used to improve the germination of sensitive seed varieties under stress, a strategy that has been successful for promoting plant growth under stress (Sampangi-Ramaiah et al., 2020). Beyond, endophytic populations from wild or tolerant relatives of selected crops might represent a valuable source to build synthetic communities (SynCom) for seed improvement. Nevertheless, this strategy remains challenging (de Souza et al., 2020). On the one hand, the design of efficient SynComs will require a better understanding of the individual, synergistic and cumulative effects of identified seed-borne endophytes on seed performance. On the other hand, their stability following seed inoculation has to be assessed.
An alternative strategy to the inoculation of seeds with endophytes themselves is the application of bioactive molecules produced by fungal endophytes. In this view, seed-borne endophytes are likely candidates to produce biostimulants active on seed biology. So far, only a handful of seed-borne endophytic fungi have been studied in this respect, and the potential value of their metabolites has essentially been considered for pest and pathogen management. As the benefits of fungal endophytes during seed cycle go far beyond the sole protection against biotic stresses, much gain can be anticipated from the discovery and use of the chemical mediators that underlie such services. The extraction and purification of bioactive molecules require the cultivation of fungal species and a major bottleneck of this approach is the gap between the number of identified seed-borne endophytic fungi and those cultivable. Moreover, culture conditions differ from the natural seed environment and will modify fungal metabolomes. Optimized approaches to isolate and cultivate seed-borne endophytes, so as to purify and test the bioactivity of their metabolic extracts, will therefore be required to identify new and robust seed biostimulants. Aside from providing potential solutions for agriculture, the study of the mode of action of these extracts will bring important information on the regulation of seed development, germination and/or longevity.
Author contributions
FR: Writing – original draft, Writing – review & editing. CK: Writing – review & editing. KC: Writing – original draft, Writing – review & editing. CD: Writing – review & editing. SP: Writing – original draft, Writing – review & editing. CB: Writing – review & editing. EB: Writing – original draft, Writing – review & editing.
Funding
The author(s) declare financial support was received for the research, authorship, and/or publication of this article. This work was supported by the Centre National de la Recherche Scientifique (CNRS), Sorbonne University, the National Museum of Natural History (MNHN) and the Agence Nationale de la Recherche (PRCE “Biostim”, grant ANR-21-CE43-0020). FR is supported by a PhD fellowship from ANR.
Conflict of interest
The authors declare that the research was conducted in the absence of any commercial or financial relationships that could be construed as a potential conflict of interest.
Publisher’s note
All claims expressed in this article are solely those of the authors and do not necessarily represent those of their affiliated organizations, or those of the publisher, the editors and the reviewers. Any product that may be evaluated in this article, or claim that may be made by its manufacturer, is not guaranteed or endorsed by the publisher.
References
Abdelfattah, A., Tack, A. J. M., Lobato, C., Wassermann, B., Berg, G. (2022). From seed to seed: the role of microbial inheritance in the assembly of the plant microbiome. Trends Microbiol. 31, 346–355. doi: 10.1016/j.tim.2022.10.009
Ahimsa-Müller, M. A., Markert, A., Hellwig, S., Knoop, V., Steiner, U., Drewke, C., et al. (2007). Clavicipitaceous fungi associated with ergoline alkaloid-containing convolvulaceae. J. Nat. Prod. 70, 1955–1960. doi: 10.1021/np070315t
Ahmad, R. Z., Khalid, R., Aqeel, M., Ameen, F., Li, C. J. (2020). Fungal endophytes trigger Achnatherum inebrians germination ability against environmental stresses. S. Afr. J. Bot. 134, 230–236. doi: 10.1016/j.sajb.2020.01.004
Bacon, C. W. (1995). Toxic endophyte-infected tall fescue and range grasses: historic perspectives. J. Anim. Sci. 73, 861–870. doi: 10.2527/1995.733861x
Bailly, C. (2019). The signalling role of ROS in the regulation of seed germination and dormancy. Biochem. J. 476, 3019–3032. doi: 10.1042/BCJ20190159
Ball, O. J.-P., Barker, G. M., Prestidge, R. A., Lauren, D. R. (1997a). Distribution and accumulation of the alkaloid peramine in neotyphodium lolii-infected perennial ryegrass. J. Chem. Ecol. 23, 1419–1434. doi: 10.1023/B:JOEC.0000006473.26175.19
Ball, O. J.-P., Barker, G. M., Prestidge, R. A., Sprosen, J. M. (1997b). Distribution and accumulation of the mycotoxin lolitrem B in Neotyphodium lolii-infected perennial ryegrass. J. Chem. Ecol. 23, 1435–1449. doi: 10.1023/B:JOEC.0000006474.44100.17
Bao, G., Song, M., Wang, Y., Saikkonen, K., Wang, H. (2019). Interactive effects of Epichloë fungal and host origins on the seed germination of Achnatherum inebrians. Symbiosis 79, 49–58. doi: 10.1007/s13199-019-00636-0
Baron, N. C., Rigobelo, E. C. (2022). Endophytic fungi: a tool for plant growth promotion and sustainable agriculture. Mycology 13, 39–55. doi: 10.1080/21501203.2021.1945699
Barret, M., Briand, M., Bonneau, S., Préveaux, A., Valière, S., Bouchez, O., et al. (2015). Emergence shapes the structure of the seed microbiota. Appl. Environ. Microbiol. 81, 1257–1266. doi: 10.1128/AEM.03722-14
Billingsley Tobias, T., Farrer, E. C., Rosales, A., Sinsabaugh, R. L., Suding, K. N., Porras-Alfaro, A. (2017). Seed-associated fungi in the alpine tundra: Both mutualists and pathogens could impact plant recruitment. Fungal Ecol. 30, 10–18. doi: 10.1016/j.funeco.2017.08.001
Bintarti, A. F., Sulesky-Grieb, A., Stopnisek, N., Shade, A. (2022). Endophytic microbiome variation among single plant seeds. Phytobiomes J. 6, 45–55. doi: 10.1094/PBIOMES-04-21-0030-R
Błaszczyk, L., Waśkiewicz, A., Gromadzka, K., Mikołajczak, K., Chełkowski, J. (2021). Sarocladium and Lecanicillium associated with maize seeds and their potential to form selected secondary metabolites. Biomolecules 11, 98. doi: 10.3390/biom11010098
Bright, M., Bulgheresi, S. (2010). A complex journey: transmission of microbial symbionts. Nat. Rev. Microbiol. 8, 218–230. doi: 10.1038/nrmicro2262
Bush, L. P., Wilkinson, H. H., Schardl, C. L. (1997). Bioprotective alkaloids of grass-fungal endophyte symbioses. Plant Physiol. 114, 1–7. doi: 10.1104/pp.114.1.1
Carrera-Castaño, G., Calleja-Cabrera, J., Pernas, M., Gómez, L., Oñate-Sánchez, L. (2020). An updated overview on the regulation of seed germination. Plants 9, 703. doi: 10.3390/plants9060703
Chen, N., He, R., Chai, Q., Li, C., Nan, Z. (2016). Transcriptomic analyses giving insights into molecular regulation mechanisms involved in cold tolerance by Epichloë endophyte in seed germination of Achnatherum inebrians. Plant Growth Regul. 80, 367–375. doi: 10.1007/s10725-016-0177-8
Chen, Y., Su, K., Li, C., White, J. F. (2021). Interactive effects of Epichloë endophyte, dormancy-breaking treatments and geographic origin on seed germination of Achnatherum inebrians. Microorganisms 9 (11), 2183. doi: 10.3390/microorganisms9112183
Chen, X., Sun, M., Chong, S., Si, J., Wu, L. (2022). Transcriptomic and metabolomic approaches deepen our knowledge of plant–endophyte interactions. Front. Plant Sci. 12. doi: 10.3389/fpls.2021.700200
Cheplick, G. P., Clay, K. (1988). Acquired chemical defences in grasses: the role of fungal endophytes. Oikos 52, 309–318. doi: 10.2307/3565204
Clay, K. (1987). Effects of fungal endophytes on the seed and seedling biology of Lolium perenne and Festuca arundinacea. Oecologia 73, 358–362. doi: 10.1007/BF00385251
Cook, D., Gardner, D. R., Grum, D., Pfister, J. A., Ralphs, M. H., Welch, K. D., et al. (2011). Swainsonine and endophyte relationships in Astragalus mollissimus and Astragalus lentiginosus. J. Agric. Food Chem. 59, 1281–1287. doi: 10.1021/jf103551t
de Souza, R. S. C., Armanhi, J. S., L and Arruda, P. (2020). From microbiome to traits: designing synthetic microbial communities for improved crop resiliency. Front. Plant Sci. 11. doi: 10.3389/fpls.2020.01179
Eady, C. (2021). The impact of alkaloid-producing Epichloë endophyte on forage ryegrass breeding: A New Zealand perspective. Toxins 13, 158. doi: 10.3390/toxins13020158
Ellis, R. H. (1992). Seed and seedling vigour in relation to crop growth and yield. Plant Growth Regul. 11, 249–255. doi: 10.1007/BF00024563
Fernando, K., Reddy, P., Hettiarachchige, I. K., Spangenberg, G. C., Rochfort, S. J. (2020). and guthridge, K Novel antifungal activity of lolium-associated Epichloë endophytes. M. Microorganisms 8, 955. doi: 10.3390/microorganisms8060955
Finch-Savage, W. E., Bassel, G. W. (2016). Seed vigour and crop establishment: extending performance beyond adaptation. J. Exp. Bot. 67, 567–591. doi: 10.1093/jxb/erv490
Forte, F. P., Malinowska, M., Nagy, I., Schmid, J., Dijkwel, P., Hume, D. E., et al. (2023). Methylome changes in Lolium perenne associated with long-term colonisation by the endophytic fungus Epichloë sp. LpTG-3 strain AR37. Front. Plant Sci. 14. doi: 10.3389/fpls.2023.1258100
Franić, I., Eschen, R., Allan, E., Hartmann, M., Schneider, S., Prospero, S. (2020). Drivers of richness and community composition of fungal endophytes of tree seeds. FEMS Microbiol. Ecol. 96, 1–10. doi: 10.1093/femsec/fiaa166
Gallery, R. E., Dalling, J. W., Arnold, A. E. (2007). Diversity, Host Affinity, and Distribution of seed-infecting fungi: a case study with Cecropia. Ecology 88, 582–588. doi: 10.1890/05-1207
Ganley, R. J., Newcombe, G. (2006). Fungal endophytes in seeds and needles of Pinus monticola. Mycol. Res. 110, 318–327. doi: 10.1016/j.mycres.2005.10.005
Gundel, P. E., Martínez-Ghersa, M. A., Garibaldi, L. A. (2009). and ghersa, C Viability of Neotyphodium endophytic fungus and endophyte-infected and noninfected Lolium multiflorum seeds. M. Botany 87, 88–96. doi: 10.1139/B08-119
Gundel, P. E., Seal, C. E., Biganzoli, F., Molina-Montenegro, M. A., Vázquez-de-Aldana, B. R., Zabalgogeazcoa, I., et al. (2018). Occurrence of alkaloids in grass seeds symbiotic with vertically-transmitted Epichloë fungal endophytes and its relationship with antioxidants. Front. Ecol. Evol. 6. doi: 10.3389/fevo.2018.00211
Hill, N. S., Brown, E. (2000). Endophyte viability in seedling tall fescue treated with fungicides. Crop Sci. 40, 1490–1491. doi: 10.2135/cropsci2000.4051490x
Hill, R., Llewellyn, T., Downes, E., Oddy, J., MacIntosh, C., Kallow, S., et al. (2021). Seed banks as incidental fungi banks: fungal endophyte diversity in stored seeds of banana wild relatives. Front. Microbiol. 12. doi: 10.3389/fmicb.2021.643731
Hou, W., Wang, J., Christensen, M. J., Liu, J., Zhang, Y., Liu, Y., et al. (2021). Metabolomics insights into the mechanism by which Epichloë gansuensis endophyte increased Achnatherum inebrians tolerance to low nitrogen stress. Plant Soil 463, 487–508. doi: 10.1007/s11104-021-04930-z
Hubbard, M., Germida, J., Vujanovic, V. (2012). Fungal endophytes improve wheat seed germination under heat and drought stress. Botany 90, 137–149. doi: 10.1139/b11-091
Hubbard, M., Germida, J. J., Vujanovic, V. (2014). Fungal endophytes enhance wheat heat and drought tolerance in terms of grain yield and second-generation seed viability. J. Appl. Microbiol. 116, 109–122. doi: 10.1111/jam.12311
Johnston-Monje, D., Gutiérrez, J. P., Lopez-Lavalle, L. A. B. (2021). Seed-transmitted bacteria and fungi dominate juvenile plant microbiomes. Front. Microbiol. 12. doi: 10.3389/fmicb.2021.737616
Justus, M., Witte, L., Hartmann, T. (1997). Levels and tissue distribution of loline alkaloids in endophyte-infected Festuca pratensis. Phytochemistry 44, 51–57. doi: 10.1016/S0031-9422(96)00535-3
Kim, J., Roy, M., Ahn, S.-H., Shanmugam, G., Yang, J. S., Jung, H. W., et al. (2022). Culturable endophytes associated with soybean seeds and their potential for suppressing seed-borne pathogens. Plant Pathol. J. 38, 313–322. doi: 10.5423/PPJ.OA.05.2022.0064
Klaedtke, S., Jacques, M.-A., Raggi, L., Préveaux, A., Bonneau, S., Negri, V., et al. (2016). Terroir is a key driver of seed-associated microbial assemblages. Environ. Microbiol. 18, 1792–1804. doi: 10.1111/1462-2920.12977
Kluger, C. G., Dalling, J. W., Gallery, R. E., Sanchez, E., Weeks-Galindo, C., Arnold, A. E. (2008). Host generalists dominate fungal communities associated with seeds of four neotropical pioneer species. J. Trop. Ecol. 24, 351–354. doi: 10.1017/S0266467408005026
Knoch, T. R., Faeth, S. H., Arnott, D. L. (1993). Endophytic fungi alter foraging and dispersal by desert seed-harvesting ants. Oecologia 95, 470–473. doi: 10.1007/BF00317429
Kou, M.-Z., Bastías, D. A., Christensen, M. J., Zhong, R., Nan, Z.-B., Zhang, X.-X. (2021). The plant salicylic acid signalling pathway regulates the infection of a biotrophic pathogen in grasses associated with an Epichloë endophyte. J. Fungi 7, 633. doi: 10.3390/jof7080633
Krings, M., Taylor, T. N., Hass, H., Kerp, H., Dotzler, N. (2007). and hermsen, E Fungal endophytes in a 400-million-yr-old land plant: infection pathways, spatial distribution, and host responses. J. New Phytol. 174, 648–657. doi: 10.1111/j.1469-8137.2007.02008.x
Kuldau, G., Bacon, C. (2008). Clavicipitaceous endophytes: Their ability to enhance resistance of grasses to multiple stresses. Biol. Control 46, 57–71. doi: 10.1016/j.biocontrol.2008.01.023
Lane, B., Sharma, S., Niu, C., Maina, A. W., Wagacha, J. M., Bluhm, B. H., et al. (2018). Changes in the fungal microbiome of maize during hermetic storage in the United States and Kenya. Front. Microbiol. 9. doi: 10.3389/fmicb.2018.02336
Leyronas, C., Mériaux, B., Raynal, G. (2006). Chemical control of Neotyphodium spp. endophytes in perennial ryegrass and tall fescue seeds. Crop Sci. 46, 98–104. doi: 10.1016/j.sajb.2020.03.022
Li, H., Parmar, S., Sharma, V. K., White, J. F. (2019). “Seed endophytes and their potential applications,” in Seed endophytes: biology and biotechnology. Eds. Verma, S. K., Francis, J.W. J. (Cham: Springer International Publishing), 35–54. doi: 10.1007/978-3-030-10504-4_3
Li, X.-Z., Song, M.-L., Yao, X., Chai, Q., Simpson, W. R., Li, C.-J., et al. (2017). The effect of seed-borne fungi and Epichloë endophyte on seed germination and biomass of Elymus sibiricus. Front.Microbiol. 8. doi: 10.3389/fmicb.2017.02488
Li, X. Z., Simpson, W. R., Song, M. L., Bao, G. S., Niu, X. L., Zhang, Z. H., et al. (2020). Effects of seed moisture content and Epichloe endophyte on germination and physiology of Achnatherum inebrians. S. Afr. J. Bot. 134, 407–414. doi: 10.1016/j.sajb.2020.03.022
Liang, J., Gao, G., Zhong, R., Liu, B., Christensen, M. J., Ju, Y., et al. (2023). Effect of Epichloë gansuensis endophyte on seed-borne microbes and seed metabolites in Achnatherum inebrians. Microbiol. Spectr. 11, e01350-22. doi: 10.1128/spectrum.01350-22
Liu, Y., Hou, W., Jin, J., Christensen, M. J., Gu, L., Cheng, C., et al. (2021). Epichloë gansuensis increases the tolerance of Achnatherum inebrians to low-P stress by modulating amino acids metabolism and phosphorus utilization efficiency. J. Fungi 7, 390. doi: 10.3390/jof7050390
Lugtenberg, B. J. J., Caradus, J. R., Johnson, L. J. (2016). Fungal endophytes for sustainable crop production. FEMS Microbiol. Ecol. 92, fiw194. doi: 10.1093/femsec/fiw194
Ma, M., Christensen, M. J., Nan, Z. (2015). Effects of the endophyte Epichloë festucae var. lolii of perennial ryegrass (Lolium perenne) on indicators of oxidative stress from pathogenic fungi during seed germination and seedling growth. Eur. J. Plant Pathol. 141, 571–583. doi: 10.1007/s10658-014-0563-x
Madej, C. W., Clay, K. (1991). Avian seed preference and weight loss experiments: the effect of fungal endophyte-infected tall fescue seeds. Oecologia 88, 296–302. doi: 10.1007/BF00320825
Mertin, A. A., Laurence, M. H., van der Merwe, M., French, K., Liew, E. C. Y. (2022). The culturable seed mycobiome of two Banksia species is dominated by latent saprotrophic and multi-trophic fungi. Fungal Biol. 126, 738–745. doi: 10.1016/j.funbio.2022.09.002
Nelson, E. B. (2018). The seed microbiome: origins, interactions, and impacts. Plant Soil 422, 7–34. doi: 10.1007/s11104-017-3289-7
Nettles, R., Watkins, J., Ricks, K., Boyer, M., Licht, M., Atwood, L. W., et al. (2016). Influence of pesticide seed treatments on rhizosphere fungal and bacterial communities and leaf fungal endophyte communities in maize and soybean. Appl. Soil Ecol. 102, 61–69. doi: 10.1016/j.apsoil.2016.02.008
Newcombe, G., Harding, A., Ridout, M., Busby, P. E. (2018). A hypothetical bottleneck in the plant microbiome. Front. Microbiol. 9. doi: 10.3389/fmicb.2018.01645
Niones, J. T., Takemoto, D. (2014). An isolate of Epichloë festucae, an endophytic fungus of temperate grasses, has growth inhibitory activity against selected grass pathogens. J. Gen. Plant Pathol. 80, 337–347. doi: 10.1007/s10327-014-0521-7
Oldrup, E., McLain-Romero, J., Padilla, A., Moya, A., Gardner, D., Creamer, R. (2010). Localization of endophytic Undifilum fungi in locoweed seed and influence of environmental parameters on a locoweed in vitro culture system. Botany 88, 512–521. doi: 10.1139/B10-026
Parmar, S., Sharma, V. K., Li, T., Tang, W., Li, H. (2022). Fungal seed endophyte FZT214 improves Dysphania ambrosioides Cd tolerance throughout different developmental stages. Front. Microbiol. 12. doi: 10.3389/fmicb.2021.783475
Philpott, M., Liew, E. C. Y., van der Merwe, M. M., Mertin, A., French, K. (2023). The influence of cone age and urbanisation on the diversity and community composition of culturable seed fungal endophytes within native Australian Banksia ericifolia L.f. subsp. ericifolia. J. Fungi 9, 706. doi: 10.3390/jof9070706
Pradhan, J., Sarma, A., Kalita, S., Talukdar, R., Tayung, K. (2023). Plant growth-promoting effect of seed-borne endophytic fungi isolated from Senna Alata (L.) Roxb. Proc. Natl. Acad. Sci. India Sect. B Biol. Sci. doi: 10.1007/s40011-023-01483-2
Prado, S., Li, Y., Nay, B. (2012). “Diversity and ecological significance of fungal endophyte natural products,” in Studies in natural products chemistry (Amsterdam, The Netherlands: Elsevier), 249–296. doi: 10.1016/B978-0-444-53836-9.00025-6
Qin, Y., Pan, X., Yuan, Z. (2016). Seed endophytic microbiota in a coastal plant and phytobeneficial properties of the fungus Cladosporium cladosporioides. Fungal Ecol. 24, 53–60. doi: 10.1016/j.funeco.2016.08.011
Radhakrishnan, R., Khan, A. L., Lee, I.-J. (2013). Endophytic fungal pre-treatments of seeds alleviates salinity stress effects in soybean plants. J. Microbiol. 51, 850–857. doi: 10.1007/s12275-013-3168-8
Rahnama, M., Maclean, P., Fleetwood, D. J., Johnson, R. D. (2023). Comparative transcriptomics profiling of perennial ryegrass infected with wild type or a ΔvelA Epichloë festucae mutant Reveals host processes underlying mutualistic versus antagonistic interactions. J. Fungi 9, 190. doi: 10.3390/jof9020190
Rashmi, M. (2019). A worldwide list of endophytic fungi with notes on ecology and diversity. Mycosphere 10, 798–1079. doi: 10.5943/mycosphere/10/1/19
Raza, M. M., Bebber, D. P. (2022). Climate change and plant pathogens. Curr. Opin. Microbiol. 70, 102233. doi: 10.1016/j.mib.2022.102233
Rice, J. S., Pinkerton, B. W., Stringer, W. C., Undersander, D. J. (1990). Seed production in tall fescue as affected by fungal endophyte. Crop Sci. 30, 1303–1305. doi: 10.2135/cropsci1990.0011183X003000060029x
Rodriguez, R. J., White, J. F., Jr., Arnold, A. E., Redman, R. S. (2009). Fungal endophytes: diversity and functional roles. New Phytol. 182, 314–330. doi: 10.1111/j.1469-8137.2009.02773.x
Rolston, M. P., Hare, M. D., Moore, K. K., Christensen, M. J. (1986). Viability of Lolium endophyte fungus in seed stored at different moisture contents and temperatures. New Z. J. Agric. Res. 14, 297–300. doi: 10.1080/03015521.1986.10423042
Rosental, L., Nonogaki, H., Fait, A. (2014). Activation and regulation of primary metabolism during seed germination. Seed Sci. Res. 24, 1–15. doi: 10.1017/S0960258513000391
Sampangi-Ramaiah, M. H., Jagadheesh, Dey, P., Jambagi, S., Vasantha Kumari, M. M., Oelmüller, R., et al. (2020). An endophyte from salt-adapted Pokkali rice confers salt-tolerance to a salt-sensitive rice variety and targets a unique pattern of genes in its new host. Sci. Rep. 10. doi: 10.1038/s41598-020-59998-x
Samreen, T., Naveed, M., Nazir, M. Z., Asghar, H. N., Khan, M. I., Zahir, Z. A., et al. (2021). Seed associated bacterial and fungal endophytes: Diversity, life cycle, transmission, and application potential. Appl. Soil Ecol. 168, 104191. doi: 10.1016/j.apsoil.2021.104191
Schardl, C. L., Grossman, R. B., Nagabhyru, P., Faulkner, J. R., Mallik, U. P. (2007). Loline alkaloids: Currencies of mutualism. Phytochemistry 68, 980–996. doi: 10.1016/j.phytochem.2007.01.010
Schardl, C. L., Leuchtmann, A., Spiering, M. J. (2004). Symbioses of grasses with seedborne fungal endophytes. Annu. Rev. Plant Biol. 55, 315–340. doi: 10.1146/annurev.arplant.55.031903.141735
Schmid, J., Day, R., Zhang, N., Dupont, P.-Y., Cox, M. P., Schardl, C. L., et al. (2017). Host tissue environment directs activities of an Epichloë endophyte, while it induces systemic hormone and defense responses in its native perennial ryegrass host. Mol. Plant Microbe Interact. 30, 138–149. doi: 10.1094/MPMI-10-16-0215-R
Shearin, Z. R. C., Filipek, M., Desai, R., Bickford, W. A., Kowalski, K. P., Clay, K. (2018). Fungal endophytes from seeds of invasive, non-native Phragmites australis and their potential role in germination and seedling growth. Plant Soil 422, 183–194. doi: 10.1007/s11104-017-3241-x
Shen, X.-Y., Cheng, Y.-L., Cai, C.-J., Fan, L., Gao, J., Hou, C.-L. (2014). Diversity and antimicrobial activity of culturable endophytic fungi isolated from Moso bamboo seeds. PloS One 9, e95838. doi: 10.1371/journal.pone.0095838
Simonin, M., Briand, M., Chesneau, G., Rochefort, A., Marais, C., Sarniguet, A., et al. (2022). Seed microbiota revealed by a large-scale meta-analysis including 50 plant species. New Phytol. 234, 1448–1463. doi: 10.1111/nph.18037
Solanki, M. K., Abdelfattah, A., Britzi, M., Zakin, V., Wisniewski, M., Droby, S., et al. (2019). Shifts in the composition of the microbiota of stored wheat grains in response to fumigation. Front. Microbiol. 10. doi: 10.3389/fmicb.2019.01098
Stone, Polishook, White (2004). Endophytic fungi. Burlington, MA, USA: Elsevier Academic Press. doi: 10.13140/RG.2.1.2497.0726
U’Ren, J. M., Dalling, J. W., Gallery, R. E., Maddison, D. R., Davis, E. C., Gibson, C. M., et al. (2009). Diversity and evolutionary origins of fungi associated with seeds of a neotropical pioneer tree: a case study for analysing fungal environmental samples. Mycological Res. 113, 432–449. doi: 10.1016/j.mycres.2008.11.015
Valente, A. M. M. P., Ferreira, A. G., Daolio, C., Rodrigues Filho, E., Boffo, E. F., Souza, A. Q. L., et al. (2013). Production of 5-hydroxy-7-methoxy-4-methylphthalide in a culture of Penicillium crustosum. An. Acad. Bras. Ciênc. 85, 487–496. doi: 10.1590/S0001-37652013005000024
Verma, A., Shameem, N., Jatav, H. S., Sathyanarayana, E., Parray, J. A., Poczai, P., et al. (2022). Fungal endophytes to combat biotic and abiotic stresses for climate-smart and sustainable agriculture. Front. Plant Sci. 13. doi: 10.3389/fpls.2022.953836
Vujanovic, V., Yuan, X., Daida, P., Milunovic, B., Germida, J. (2016). Manipulation of cold stratification and endophytic effects on expression patterns of RSG and KAO genes in coleorhiza of wheat seeds. Plant Growth Regul. 79, 219–227. doi: 10.1007/s10725-015-0127-x
Wang, Z., Li, C., White, J. (2020). Effects of Epichloë endophyte infection on growth, physiological properties and seed germination of wild barley under saline conditions. J. Agron. Crop Sci. 206, 43–51. doi: 10.1111/jac.12366
Wassermann, B., Cernava, T., Müller, H., Berg, C., Berg, G. (2019). Seeds of native alpine plants host unique microbial communities embedded in cross-kingdom networks. Microbiome 7, 108. doi: 10.1186/s40168-019-0723-5
Wilson, D. (1995). Endophyte: The evolution of a term, and clarification of its use and definition. Oikos 73, 274–276. doi: 10.2307/3545919
Zhang, X., Fan, X., Li, C., Nan, Z. (2010). Effects of cadmium stress on seed germination, seedling growth and antioxidative enzymes in Achnatherum inebrians plants infected with a Neotyphodium endophyte. Plant Growth Regul. 60, 91–97. doi: 10.1007/s10725-009-9422-8
Zhang, X., Li, C., Nan, Z. (2012). Effects of cadmium stress on seed germination and seedling growth of Elymus dahuricus infected with the Neotyphodium endophyte. Sci. China Life Sci. 55, 793–799. doi: 10.1007/s11427-012-4359-y
Zhang, X. X., Li, C. J., Nan, Z. B., Matthew, C. (2011). Neotyphodium endophyte increases Achnatherum inebrians (drunken horse grass) resistance to herbivores and seed predators. Weed Res. 52, 70–78. doi: 10.1111/j.1365-3180.2011.00887.x
Zhang, W., Mace, W. J., Matthew, C., Card, S. D. (2019). The impact of endophyte infection, seed aging, and imbibition on selected sugar metabolite concentrations in seed. J. Agric. Food Chem. 67, 6921–6929. doi: 10.1021/acs.jafc.9b01618
Zhao, Z., Kou, M., Zhong, R., Xia, C., Christensen, M. J., Zhang, X. (2021). Transcriptome analysis revealed plant hormone biosynthesis and response pathway modification by Epichloë gansuensis in Achnatherum inebrians under different soil moisture availability. J. Fungi 7, 640. doi: 10.3390/jof7080640
Keywords: seeds, fungal endophytes, germination, stress tolerance, bio-stimulant, biocontrol, fungal metabolites
Citation: Rétif F, Kunz C, Calabro K, Duval C, Prado S, Bailly C and Baudouin E (2023) Seed fungal endophytes as biostimulants and biocontrol agents to improve seed performance. Front. Plant Sci. 14:1260292. doi: 10.3389/fpls.2023.1260292
Received: 18 July 2023; Accepted: 12 October 2023;
Published: 24 October 2023.
Edited by:
Spyridon Alexandros Petropoulos, University of Thessaly, GreeceReviewed by:
Han-Bo Zhang, Yunnan University, ChinaCopyright © 2023 Rétif, Kunz, Calabro, Duval, Prado, Bailly and Baudouin. This is an open-access article distributed under the terms of the Creative Commons Attribution License (CC BY). The use, distribution or reproduction in other forums is permitted, provided the original author(s) and the copyright owner(s) are credited and that the original publication in this journal is cited, in accordance with accepted academic practice. No use, distribution or reproduction is permitted which does not comply with these terms.
*Correspondence: Emmanuel Baudouin, emmanuel.baudouin@sorbonne-universite.fr