- Department of Systems Biology, Human Microbiology Institute, New York, NY, United States
Fungal plant infections result in substantial losses to the agricultural sector. A range of fungicide seed dressings are available to control seed-borne fungal diseases; however, they lack sufficient efficacy because of intrinsic tolerance and acquired resistance. Moreover, many fungicide seed dressings can also penetrate plants, negatively affecting plant growth owing to their toxic effects on endophytes, as well as contributing to the spread of antibiotic resistance. Here, we evaluated the efficacy of M451, a member of a new class of antimicrobial agents that are not relevant to human healthcare. As a seed dressing for wheat seeds, M451 exhibited significant antifungal activity against one of the most devastating plant fungal pathogens, Fusarium spp. Furthermore, M451 was more active than the commercially used fungicide Maxim XL against both seed-borne and soil-borne F. oxysporum infection. Importantly, and unlike other antifungals, M451 seed dressing did not inhibit any of the major characteristics of wheat grains and seedlings, such as germination percentage, germination time, grain vigor, shoot- and root weight and length, but rather improved some of these parameters. The results also demonstrated that M451 had no negative impacts on endophytes and did not accumulate in grains. Thus, M451 may have potential applications as an antifungal agent in wheat cultivation.
1 Introduction
Fungal wheat diseases, cause substantial losses in food production, being responsible for over 15% of global losses in wheat production (Kaur, 2016; Zaller et al., 2016; Simón et al., 2021). In wheat cultivation seed-borne fungal diseases are responsible for grain death, poor crop establishment, and reduced germination rates, while soil-borne root fungal diseases also cause massive destruction and crop loss, playing a vital role in reducing the profitability, quality, and quantity of production (Rajput et al., 2005). Therefore, fungicidal seed dressings are widely used to protect crop grains from fungal diseases. Seed application of antifungal compounds has unique benefits over other methods used to treat fungal plant infections, and it can be used to prevent and overcome those diseases that cannot be controlled after planting.
However, existing antifungal seed dressing agents show limited effectiveness in reducing fungal diseases in crops. This is partly because seed and root pathogenic fungi belong to diverse genera, and only a few fungicides have broad-spectrum activities against a variety of fungal pathogens, being able to lessen the severity of the infection (Fan et al., 2017; McGee, 1995; Tunali et al., 2008; Coker et al., 2010). Another relevant issue is the emergence of resistance to available fungicides, which—along with high intrinsic antifungal resistance—dramatically reduces the efficacy of available therapeutic options (Conner et al., 1996; Janmajay et al., 2011; Thind, 2012; Lucas et al., 2015). Therefore, several commercially available agricultural seed dressing fungicides contain two or more antifungal agents that target different fungal pathogens (e.g., Maxim XL contains fludioxonil and mefenoxam). However, even these combined products have limited efficacy. Moreover, they show increased toxicity in plants, resulting in lower seed germination rates and decreases in shoot and root lengths and relative water content (Kookana et al., 1998; Komárek et al., 2010; Sykes et al., 2018; Yang et al., 2019; Wen et al., 2022; Serra et al., 2015; Singh and Sahota, 2018). In addition to having a direct toxic effect on plant seeds, many agricultural fungicides inhibit plant endophytes, which are important contributors to plant growth (Baibakova et al., 2019). Indeed, many plant endophytes that belong to Aspergillus spp., Penicillium spp., and Staphylococcus spp. are sensitive to fungicide seed dressings and their loss disrupts mutualist endophyte-host interactions negatively affecting different plant characteristics (Javed et al., 2019; Błaszczyk et al., 2021).
Another concern related to many existing agricultural fungicides is that they have a chemical structure similar to that of fungicides used in human healthcare, including derivatives of azoles and polyene antibiotics (Takahashi et al., 2020). These environmental fungicides can become potential sources of resistance for invasive human mycoses owing to cross-resistance with medical antifungal agents (Takahashi et al., 2020; Bastos et al., 2021). This can happen through fungicide-exposed soil driving the selection of acquired fungal-resistant clones and/or the accumulation of these environmental fungicides inside plants, which are then consumed by humans and other animals (Bastos et al., 2021; Li et al., 2022; Elfikrie et al., 2020). As a result, there is a significant unmet need for a broad-spectrum antifungal seed dressing agent that is active against resistant fungal species, does not penetrate plants, is not toxic to endophytes, and is not a derivative of those used for the treatment of human diseases (Brauer et al., 2019).
Recently, we described the high fungicidal activity of M451, a broad-spectrum antifungal agent. M451 is a combinatorial product of TGV-28 [poly-(N-carboxamido-1,6-diaminohexane), particularly N’-aminated], which is a derivative of the antimicrobial agent Mul-1867, and NaH2PO4 (0.01%). M451 has been shown to exhibit fungicidal activity against different strains of Ascomycota, Oomycota, and Basidiomycota (Tetz and Tetz, 2015; Tetz et al., 2017; Tetz et al., 2023). In this study, we assessed the efficacy of M451 as a seed dressing against seed- and root-borne fungal infection in wheat. We also evaluated its effects on endosymbionts and its extent of penetration and absorption into wheat plants.
2 Materials and methods
2.1 Seed dressing preparation
Triticale grains (Triticum aestivum L. emend. Fiori et Paol) were obtained from local commercial suppliers. Wheat grains with known seed-borne Fusarium infections were obtained from the Human Microbiology Institute (New York, NY, USA). Potato dextrose agar (PDA; Thermo Fisher Scientific Inc., Waltham, MA, USA) was used as a growth medium for seed germination.
Grains in the control group were sterilized according to Htwe et al. (2011), with minor modifications. Briefly, uniformly sized grains were washed in a solution of 4.5% (w/v) NaOCl:water (1:1 ratio) for 20 min. For the experimental groups, uniformly sized grains were treated with different concentrations of three different solutions: 1) 0.1% and 0.05% M451 (a water-based solution of TGV-28 [Figure 1]; Human Microbiology Institute) in combination with 0.01% NaH2PO4 (Sigma Aldrich, St. Louis, MO, USA) for 30 min, 2) 0.01% NaH2PO4 for 30 min (Sigma Aldrich), and 3) Maxim XL v/v1:10 dilution in water for 12 h according to the manufacturer’s instructions (Syngenta Canada Inc., Guelph, ON, Canada) (Costa et al., 2019; Pfeiffer et al., 2021). Subsequently, the grains from control and M451-treated groups were washed three times with autoclaved distilled water and then air-dried on filter paper. The grains treated with Maxim XL were left unwashed.

Figure 1 Chemical structure of TGV-28 [poly-(N-carboxamido-1,6-diaminohexane), particularly N’-aminated].
2.2 Analysis of growth characteristics
Grains were treated with different concentrations of test compounds, as described above, or the control solution (4.5% (w/v) NaOCl:water (1:1 ratio), and the number of grains that sprouted and germinated were counted daily for up to 7 days. Sprouted grains were defined as those that produced at least one noticeable plumule or radicle (Rahman et al., 2001). The grains were considered to have germinated when at least 2 mm of radicle emerged from the grain coat. On the seventh day of germination, the following parameters were recorded: water uptake percentage, germination percentage, germination index, grain vigor, mean germination time, and biomass (Tsegay and Gebreslassie, 2014). Seedling height, shoot length, and root length of three randomly chosen seedlings were measured in each treatment and the control groups (Zhang et al., 2014).
2.3 Measurement of water uptake percentage
Prior to sterilizing the grains, the dry weight of each selected grain was measured using a scale (MKE-5, Arlyn Series, Arlyn Scales, East Rockaway, NY, USA). Thereafter, the grains were treated with M451 (0.1% and 0.05%), NaH2PO4 (0.01%), or Maxim XL, as described in 2.1. The grains were then transferred to Petri dishes (n =? per dish) lined with Whatman No. 1 filter paper, and 10 ml of sterile distilled water was added to each dish. The grains were incubated for 24 h at the room temperature. The fresh weight of the grains in each treatment group was recorded to determine the water uptake (Gairola et al., 2011) using the equation:
2.4 Measurement of the germination percentage
Germination percentage (GP) was calculated based on the total number of grains in each group that germinated using the equation:
2.5 Measurement of the germination index
The germination index (GI) was calculated as the sum of germinated grains on a given day (Gt) divided by the number of germination days (Dt) using the following equation:
2.6 Measurement of the mean germination time
The mean germination time (MGT) was determined as the average amount of time needed for a grain to initiate and terminate the germination process (Ellis and Roberts, 1981). A grain was considered to have germinated once its radicle had reached 2 mm in length (Montaña et al., 2014). MGT was calculated using the following equation:
where n is the number of germinated grains that germinated on day D, and D is the number of days counted from the beginning of germination.
2.7 Measurement of grain vigor
Grain vigor was determined to evaluate the activity level and performance of a grain during germination using a previously described formula (Abdul-Baki and Anderson, 1973) as follows:
where GP is the germination percentage.
2.8 Measurement of dry weight
The dry weight of seedlings before and after treatment was determined by drying freshly collected seedling samples at 80°C for 24 h. Dry weight was measured in mg using a scale (MKE-5, Arlyn Series) (Carpýcý et al., 2009).
2.9 Measurement of shoot and root length
After 7 d of germination in a plastic pot, the length of normal seedlings and the corresponding shoot and root length were recorded in cm (Oukhouia et al., 2017).
2.10 Isolation of endophytic bacteria and fungi
Grains sterilized with 4.5% NaOCl:water (1:1) or treated with 0.1% M451 were cut in half and plated on PDA medium, and then incubated for 120 h at 25°C. Fungi were identified based on the morphological parameters of the colonies, such as colony shape, pigmentation, the presence of septa, and the shape of the conidia. For morphological identification, fungal fragments were placed on a glass slide, stained with methylene blue, and observed under light microscopy (Axiostar plus, Carl Zeiss, Germany; objective lens: A-Plan 100х/1.25). Bacteria were identified based on proteome analysis using matrix-assisted laser desorption/ionization-time of flight (MALDI-TOF) mass spectrometry (MS) with sample preparation on AnchorChip microtiter plates (Bruker Corporation, Billerica, MA, USA). The bacteria were identified using the MALDI Biotyper Database (Bruker Taxonomy Tree, Bruker Corporation).
2.11 Germination of soil-borne Fusarium grains
Fusarium oxysporum conidia, confirmed by molecular identification, were harvested from 5-d-old cultures grown on PDA medium, mixed with sterile water, and then mixed with soil at a spore density of 2 × 105 g–1 dry weight soil, as previously described (Oukhouia et al., 2017). Grains in the control and fungicide-treated groups were seeded in a single row (length, 20 cm; 10 grains per row; depth, 2 cm) and completely covered with soil. A total of seven rows were planted per container, spaced 5 cm apart. After planting, the containers were placed in growth chambers at a constant temperature with a 12-h light cycle.
2.12 Analysis of M451 accumulation in plants
For the quantitative analysis of M451 accumulation in plants, we used untreated seedlings as a negative control, seedlings from seeds treated with 0.1% sulfamethoxazole (Sigma Aldrich) as a positive seed dressing control, and seedlings from seeds treated with 0.1% M451 seed dressing as the test group. The samples were freeze-dried for 24 h for this analysis (Jones-Lepp et al., 2010). The extraction and cleanup of the samples were performed as previously described by Pan et al. (2014). The extracted samples were examined using ultra-high-performance liquid chromatography coupled with quadrupole-linear ion-trap tandem MS (UPLC-MS/MS; LC-20 Prominence HPLC system coupled with a LCMS-8050 triple quadrupole mass spectrometer; Shimadzu, Tokyo, Japan) as described in Gros et al. (2013). The chromatographic and MS conditions for the analysis of M451 accumulation are described in Supplementary Table 1.
2.13 Biochemical composition of wheat seedlings
For the phytochemical profiling of wheat seedlings, a 100 g sample was collected from each group in triplicate. Dry matter was measured gravimetrically (AOAC 931.04), Bertrand’s method was used to determine the sugar content (Badger, 2002), and ascorbic acid levels were measured spectrophotometrically (SmartSpec Plus, Bio-Rad Laboratories, Hercules, CA, USA) (Salachna et al., 2018). Carotenoid and chlorophyll content was evaluated at different wavelengths according to Schüler et al. (2020) (Supplementary Table 2).
2.14 Statistical analysis
For all data, appropriate descriptive statistics are presented in the supplementary tables. Between-group differences were evaluated using one-way analysis of variance (ANOVA) in the GraphPad Prism software (version 9.3.1). Differences were considered statistically significant at P<0.05. All experiments were performed in triplicate and the results are presented as the mean ± standard deviation (SD).
3 Results
3.1 Effect of M451 seed dressing on seed-borne pathogens
We evaluated the effects of the following seed dressings on wheat grains with known Fusarium spp. infection: control (4.5% NaOCl:water [1:1 ratio]), 0.05% and 0.1% M451, and Maxim XL (at a fixed concentration corresponding to 0.25% fludioxonil and 0.01% for mefenoxam) (https://www.syngenta-us.com/seed-treatment/maxim-xl; Figure 2). Although all tested compounds inhibited microbial growth, 0.1% M451 exhibited the most effective antimicrobial effect. Maxim XL was less effective than 0.1% M451, and 4.5% NaOCl was least effective as an antifungal agent.
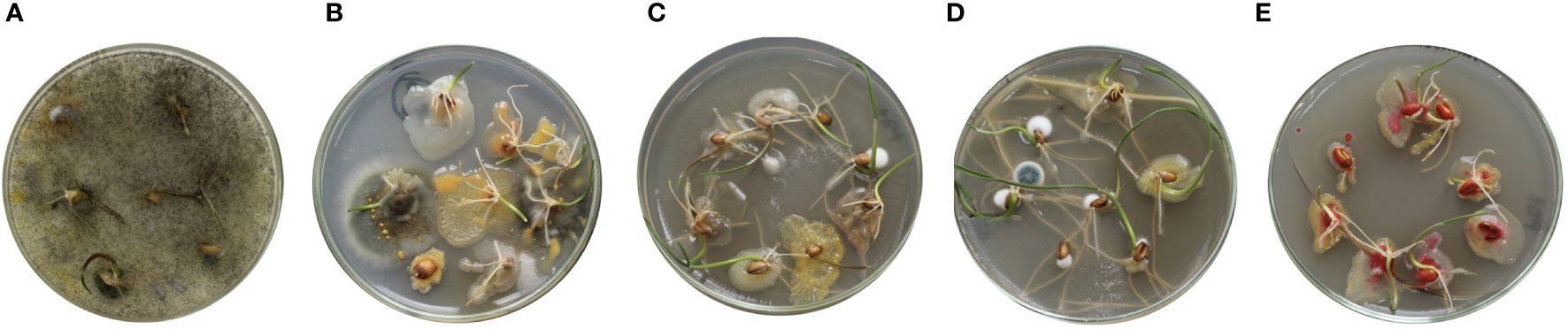
Figure 2 Triticum aestivum L. grains with known Fusarium spp. infection were pretreated with the test compounds and germinated in a Petri dish. (A) Non-treated grains with seed-borne infection. Grains treated with (B) 4.5% sodium hypochlorite:water (1:1), (C) 0.05% M451, (D) 0.1% M451, and (E) Maxim XL (0.25% fludioxonil and 0.01% for mefenoxam).
3.2 Effect of M451 on the major characteristics of wheat grains and seedlings
Next, we examined how seed dressings with the tested compounds affected different features of early plant development (Figure 3). Treatment of wheat grains with 0.1% and 0.05% M451 did not inhibit water uptake within the first 24 h (Figures 3A, B). Indeed, treatment with either 0.1% or 0.05% M451 significantly increased the GP and GI by 26% and 21%, respectively (P<0.01 and P<0.001, respectively (Figures 3C, D). Treatment with Maxim XL also increased the GP compared with the control (P<0.01); however, this improvement was statistically lower than that induced by treatment with 0.1% M451 (P<0.05). Treatment with Maxim XL did not affect the GI. Treatment with 0.05% and 0.1% M451 substantially reduced the MGT by 40% and 63%, respectively; however, the difference was statistically significant only for treatment with 0.1% M451 (Figure 3E). Similarly, treatment with 0.1% M451 increased the grain vigor by 35% (P<0.0001), although this parameter was not changed by the other treatments (Figure 3F). Finally, on day 7, treatment with 0.1% M451 significantly increased shoot and root length and dry weight of seedlings by 44–50% (P-values ranging from<0.05 to<0.0001) compared with those of the untreated control. These parameters were not affected by treatment with Maxim XL (Figures 3G–J).
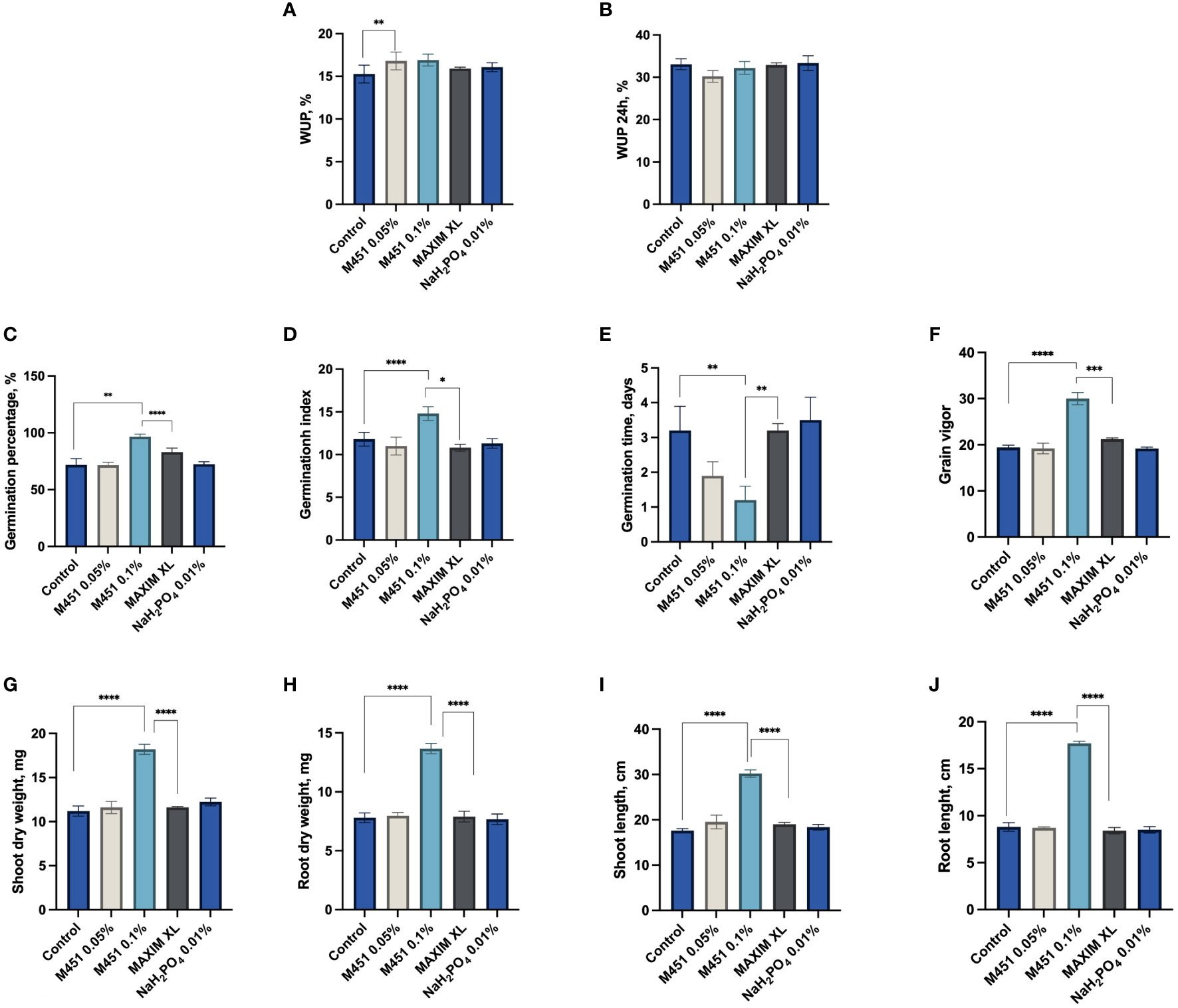
Figure 3 Effects of test compounds on the functional and physical parameters of wheat grains and seedlings. Effects on (A, B) water uptake, (C) germination percentage, (D) mean germination time, (E) germination index, (F) grain vigor, (G) shoot dry weight, (H) root dry weight, (I) shoot length, and (J) root length. *P<0.05, **P<0.01, ***P<0.001, ****P<0.0001.
3.3 Effect of M451 on endophytic bacteria and fungi
Since M451 is a broad-spectrum antimicrobial agent, we examined whether it would negatively affect endophytic bacteria and fungi in wheat seedlings through seed dressing with 0.1% M451 for 30 min. The results were compared with those of grains sterilized with 4.5% NaOCl:water (1:1) for 20 min, which is known not to inhibit endophytic microorganisms (Figure 4) (Sahu et al., 2022). MALDI-TOF analysis of the microbial species revealed that neither treatment inhibited the growth of well-known endophytes, such as Aspergillus spp., Penicillium spp., and Staphylococcus spp Toghueo and Boyom, 2020; Patel at al., 2021; Pang at al., 2022.
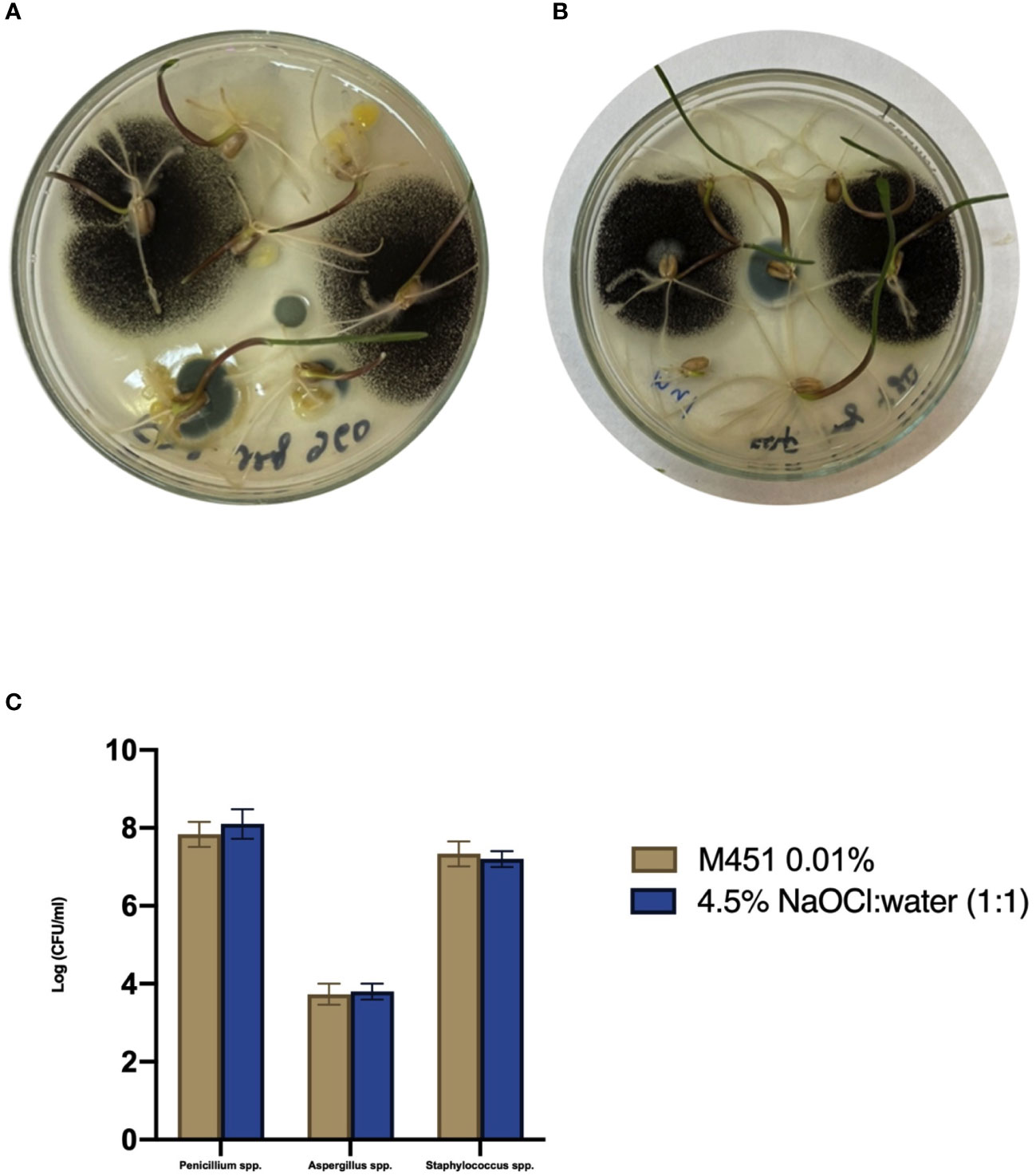
Figure 4 Effect of the M451 seed dressing on the growth of endophytic microorganisms. Wheat grains pretreated with the test compounds were germinated in a Petri dish. (A) Grains treated with 4.5% NaOCl:water (1:1). (B) Grains treated with 0.1% M451. (C) Effects of the test compounds on the growth of endophytes.
3.4 Lack of penetration and accumulation of M451 in wheat plants
For the quantitative analysis of M451 uptake by wheat plants, we analyzed the shoots and roots of wheat seedlings treated with 0.1% M451 or 0.1% sulfamethoxazole (positive control) seed dressings. UPLC-MS/MS analysis indicated that the concentration of sulfamethoxazole in the samples ranged from 10.5 to 20.1 µg·kg−1. However, there was no quantifiable uptake of M451 (Figure 5).
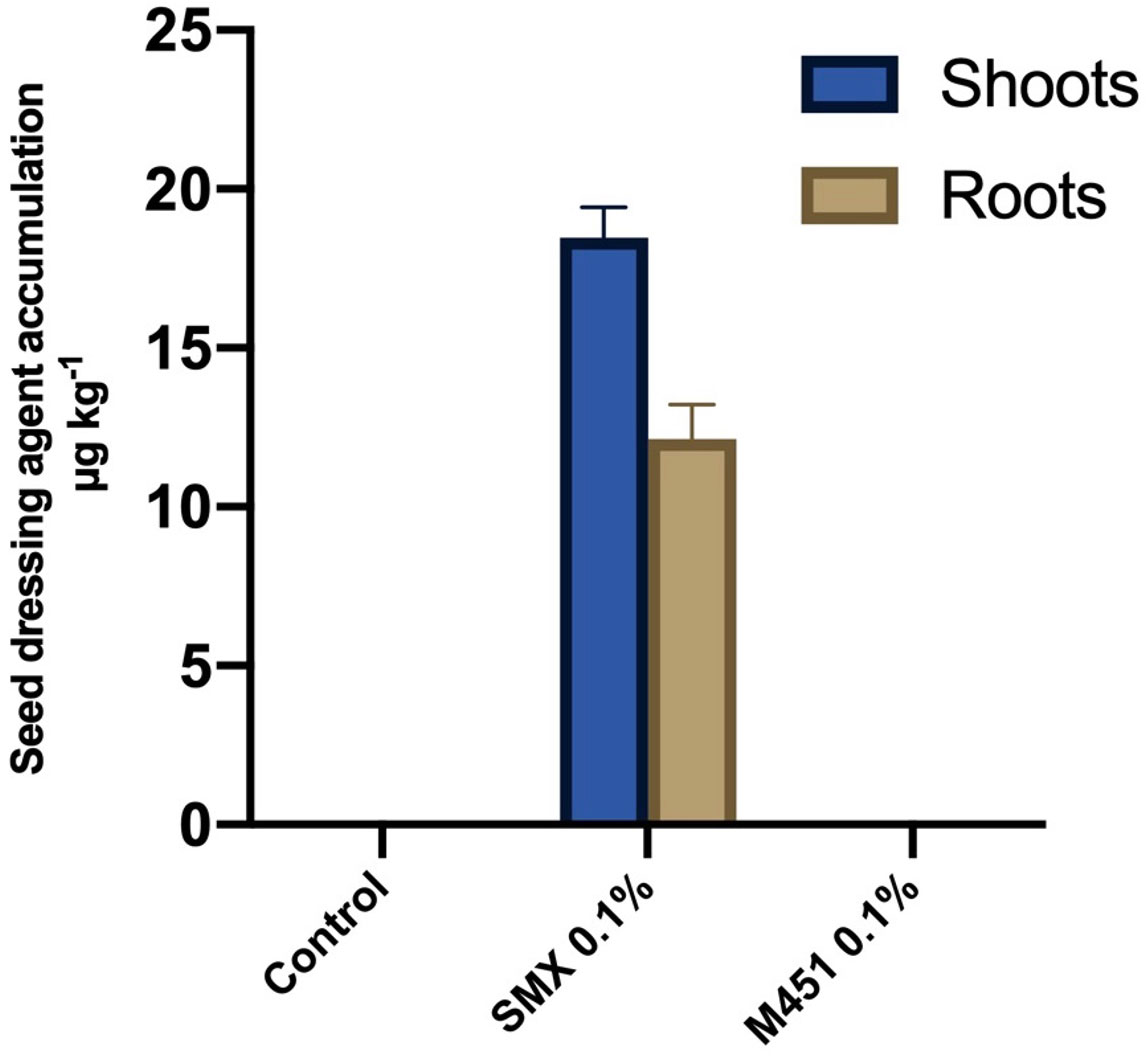
Figure 5 UPLC-MS/MS-based detection of 0.1% M451 and 0.1% sulfamethoxazole (SMX; positive control) in wheat shoots and roots after seed dressing. The data are representative of results from three independent experiments.
3.5 Effect of M451 on plant characteristics in soil infected with Fusarium
Treatment with M451 not only protected the plants from seed-borne infections, but also increased seedling growth in non-infected soil. Therefore, we evaluated the effects of the M451 seed dressing on seedling germination and growth in Fusarium-infected soil. Infection with soil-borne F. oxysporum reduced the GP of untreated control grains by 54.8% (P<0.001) (Figure 6A). The negative impact of fungal infection was slightly alleviated when the wheat grains were pretreated with Maxim XL. However, even in this case, the GP of Maxim XL-treated grains was reduced by >21% (not statistically significant) compared with that of uninfected grains. M451 was the most effective agent in this regard. Seed dressing with 0.05% M451 reduced the GP by 12.5%. As discussed in 3.2, seed dressing with 0.1% M451 significantly increased the GP of wheat grains in non-infected soil. Even in Fusarium-infected soil, the GP of grains treated with 0.1% M451 was 8% higher (not statistically significant) than that of control grains in non-infected soil.
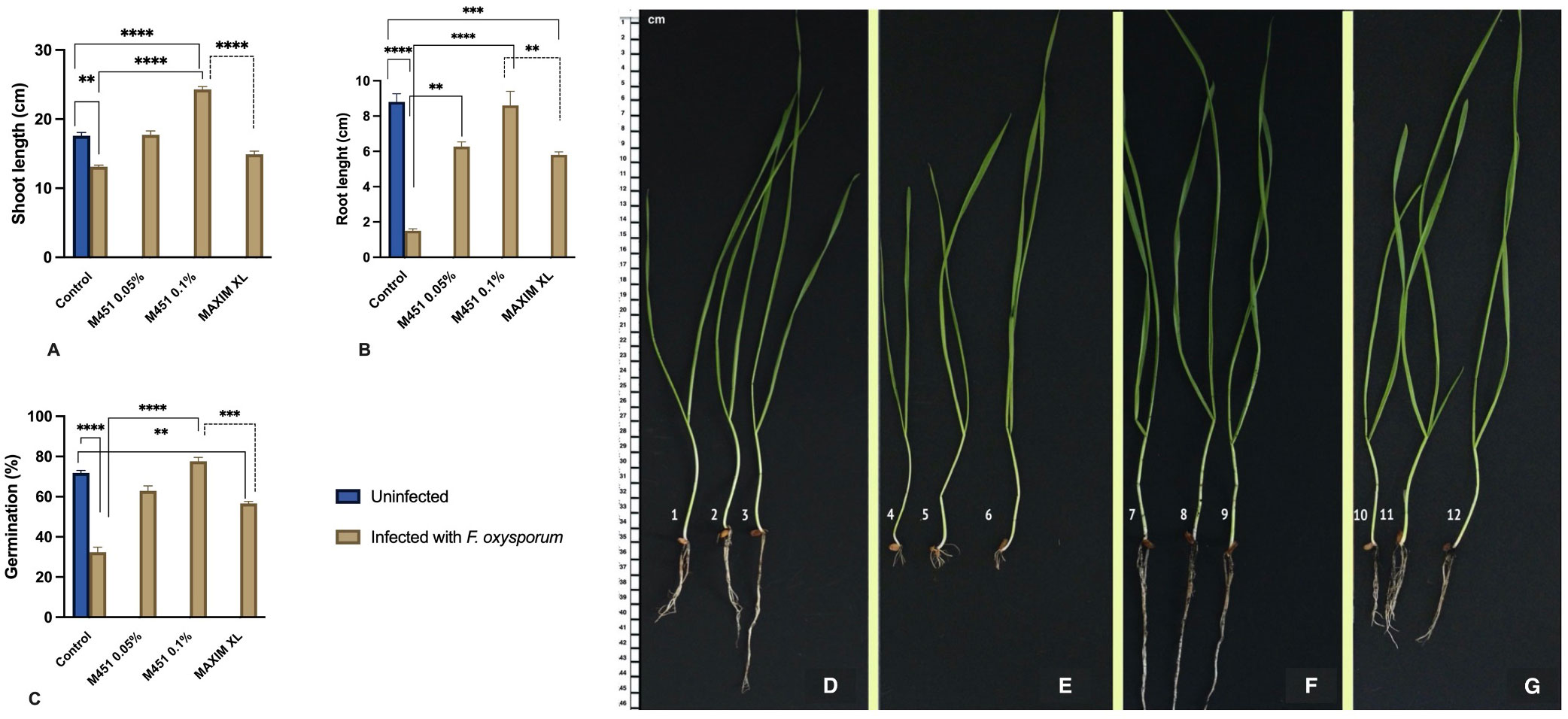
Figure 6 Effect of M451 on plant characteristics in a soil-borne Fusarium model of wheat plants. (A–C) Effects of M451 and Maxim XL on major characteristics of wheat grains grown in uninfected and Fusarium-infected soil. (A) Shoot length, (B) root length, and (C) germination percentage. (D–G) Representative images of Triticum aestivum L. seedlings germinated from untreated grains or grains treated with M451 and grown in uninfected or Fusarium-infected soil. (D) Untreated healthy seedling, (E) Untreated seedling with soil-borne Fusarium, (F) healthy seedling treated with 0.1% M451, and (G) M451-treated seedling grown in Fusarium-infected soil. **P<0.01, ***P<0.001, ****P<0.0001.
We also evaluated the effect of soil-borne infection on seedling characteristics. As expected, soil-borne Fusarium reduced the shoot and root lengths of seedlings sprouting from untreated grains by 25.5% and 88%, respectively (Figures 6B, C). Under the same conditions, pretreatment with Maxim XL only reduced the negative impact of Fusarium infection on the shoot and root length, which were reduced by 15.3% and 34%, respectively, compared with the untreated control in uninfected soil. M451 was significantly more effective in alleviating the negative effects of soil-borne Fusarium infection on seedling growth. In wheat seedlings that sprouted from grains treated with 0.05% M451, the shoot length was 1% higher and the root length was 24% lower than that of the untreated control in uninfected soil (Figures 6B, C). Treatment with 0.1% M451 had the greatest protective effect, inducing an 11% increase in shoot length and only a 1.2% decrease in root length compared with the seedlings of untreated grains in uninfected soil (Figures 6B, C). Image analysis of the root structure showed that seed dressing with 0.1% M451 improved the characteristics of the whole root system, including root length, root volume, and root number (Figures 6D–G).
3.6 Effect of M451 on the biochemical characteristics of wheat seedlings
Finally, we examined the effects of M451 treatment on 15-d-old Triticum aestivum L. seedlings grown from M451-dressed grains (Figure 7). The concentrations of chlorophyll a, chlorophyll b, and total chlorophyll increased in seedlings from M451-treated grains by 42.7%, 20.5%, and 36.1.%, respectively (all P<0.01) Moreover, pretreatment of seeds with M451 increased the levels of carotenoids, carotens, and β-carotene by 32.9%, 32.4%, and 95.2%, respectively (all P<0.01). These results clearly indicated that treating wheat seeds—even non-infected seeds—with M451 changed their specific phytochemical characteristics.
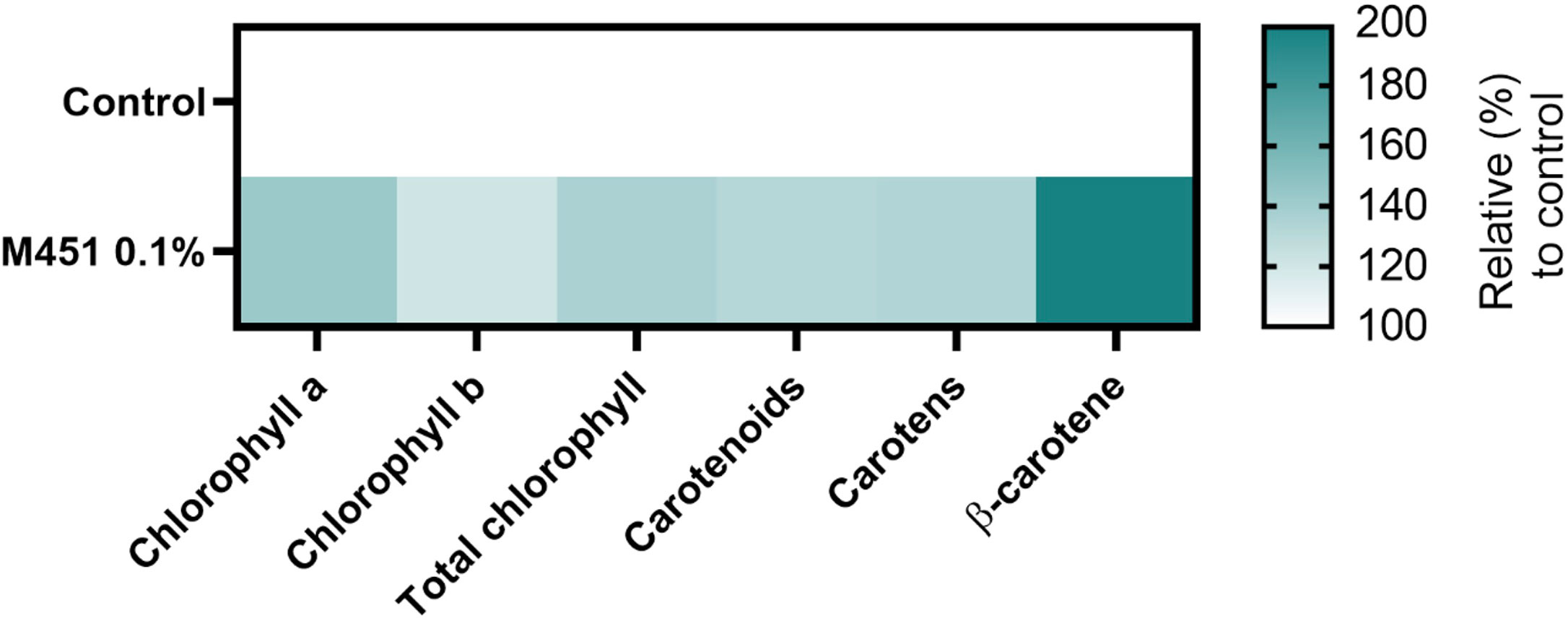
Figure 7 Phytochemical profile of 15-d-old wheat seedlings. The heatmap demonstrates an increase or decrease in the mean value of each parameter (%). The value of each parameter was normalized to the value of the same parameter in the control group. The color scale ranges from white (minimum) to dark green (maximum). The data are representative of results from three independent experiments.
4 Discussion
New fungicides with a broad spectrum of antifungal activity can be used to treat different infections in wheat. There is an urgent need to develop novel broad-spectrum fungicides for agricultural use; however, antifungal drug discovery has always been challenging owing to the difficulties in identifying unique targets for antifungal antibiotics (Tetz and Tetz, 2022; Kitchen et al., 2016; Lugtenberg et al., 2016). In addition, there is an insufficient level of contemporary understanding of affected regulatory pathways, which are shared across pathogenic fungi and other eukaryotic organisms. As a result, today, many antifungals, such as azoles or polyenes that are used in agriculture, are the same as those used in human medicine (Fisher et al., 2022). This represents a particular problem and is a key driver of environmental drug resistance in contaminated soil and crops, resulting in a cross-resistance to medicinal drugs (Jauregi et al., 2021). Previous studies confirmed that the use of environmental azole antifungals triggers the acquisition of the cyp51A gene in Aspergillus spp., rendering them resistant to medicinal azoles, including voriconazole (Ren et al., 2017). Therefore, the development of agriculture- vs. medicinal-specific fungicides is essential.
M451 is a combination product of a novel antimicrobial component, TGV28, which was specifically developed for agriculture and not being used for the treatment of human diseases, and NaH2PO4 (0.01%). Tetz et al. (2023) previously reported the inhibitory effects of M451 on multiple plant pathogens. However, this is the first study to evaluate the effects of M451 seed dressing on seedling growth in wheat plants.
We observed an apparent beneficial effect of M451 seed dressing applied to uninfected grains on wheat seedlings, including stimulated germination and the promotion of other critical properties. These included an improved germination percentage, germination index, and mean germination time, as well as a significant increase in shoot and root lengths. Under the same general treatment conditions, none of these properties were improved by treatment with Maxim XL. M451 also did not have any negative effects on water uptake by wheat seedlings, which is known to be a frequent negative effect of fungicides (Biswas and Haynes, 1970; Zhang et al., 2010; Hadas, 1976; Wolny et al., 2018).
We were particularly interested in evaluating the effects of M451 seed dressing on F. oxysporum since it is one of the top ten fungal plant pathogens that affects the yield of various plants and possesses a high level of resistance to different antifungals (Doehlemann et al., 2017). Because M451 has been shown to possess broad-range antimicrobial activity, we expected it to be highly protective against seed- and soil-borne F. oxysporum infection when used as a seed dressing (Tetz et al., 2023). Consistent with this, wheat seedlings grown from M451-treated grains were significantly less affected by Fusarium infection (which partially inhibited the growth of the control seedlings). We also found clear evidence of a dose-dependent effect for M451; treatment with 0.1% M451 conferred a higher level of protection than treatment with 0.05% M451. Under the same conditions, treatment with Maxim XL conferred a significantly lower level of protection against Fusarium infection.
We speculate that under conditions of seed- or soil-borne Fusarium spp. infection, the seedlings became less sensitive to the fungi, not only because of the direct antifungal activity of M451, but also because M451 enabled the seeds to “outrun” pathogen infection by reducing the time to germination. Indeed, M451 reduced the germination time of uninfected grains, most likely by reducing the duration of dormancy and promoting the transition to the seedling stage. Furthermore, since seedlings are known to have a higher resistance to fungal proliferation, this could lead to a lower sensitivity of the M451 coated grains to Fusarium disease.
Another important desirable feature of the agricultural fungicide M451 is that it does not accumulate in plant parts that are consumed by agricultural animals or humans. Various plants, such as tomato, peppers, and rice take up certain fungicides (Matadha et al., 2019; Li et al., 2022; Tao et al., 2022). Here we confirmed that M451 does not penetrate wheat plants and it does not accumulate in wheat seedling tissues. Thus, this means that M451 will not contribute to residual toxicity, making it an attractive antifungal agent for use in edible plants.
Finally, we analyzed the impact of M451 on the microbiome of wheat grains by focusing on the continued presence of fungal endophytes, which are believed to play a crucial role in the habitat adaptability of plants (Ayesha et al., 2021). Despite its broad-spectrum antimicrobial activity, M451 did not inhibit bacterial or fungal endophytes. One potential explanation for this is that because of its high level of activity, M451 is effective even when applied for a short period of time. In this study, we applied M451 to wheat grains for only 30 min. This time period was sufficient for the eradication of pathogenic bacteria and fungi, but was short enough that M451 did not penetrate the seeds deep enough to inhibit the growth of endophytes. These results are particularly interesting considering our previous findings that M451 was effective in eradication of a broad range of agricultural pathogens, including members of the Ascomycota, Oomycota, and Basidiomycota phyla, within 5 min of exposure (Tetz et al., 2023). This suggests that the 30-min exposure time used in this study could be shortened, further decreasing the potential for nontarget effects of M451 on endophytes. This will be evaluated in follow up studies.
The current study evaluated the potentiation effects of M451 on wheat grain germination and seedling growth under normal and Fusarium-infected conditions. Our findings indicate the possibility of developing M451 further into an agriculture-specific antifungal drug candidate through additional in vivo research.
Data availability statement
The original contributions presented in the study are included in the article/Supplementary Material. Further inquiries can be directed to the corresponding author.
Author contributions
VT, KK, and GT conceived and supervised the research. KK and MV conducted experiments. VT, KK, GT, and MV analyzed the data and wrote the manuscript. KK, GT, and VT edited and helped to draft the final manuscript. All authors contributed to the article and approved the submitted version.
Conflict of interest
The authors declare that the research was conducted in the absence of any commercial or financial relationships that could be construed as a potential conflict of interest.
Publisher’s note
All claims expressed in this article are solely those of the authors and do not necessarily represent those of their affiliated organizations, or those of the publisher, the editors and the reviewers. Any product that may be evaluated in this article, or claim that may be made by its manufacturer, is not guaranteed or endorsed by the publisher.
Supplementary material
The Supplementary Material for this article can be found online at: https://www.frontiersin.org/articles/10.3389/fpls.2023.1176553/full#supplementary-material
References
Abdul-Baki, A. A., Anderson, J. D. (1973). Vigor determination in soybean seed by multiple criteria 1. Crop Sci. 13 (6), 630–633. doi: 10.2135/cropsci1973.0011183X001300060013x
Ayesha, M. S., Suryanarayanan, T. S., Nataraja, K. N., Prasad, S. R., Shaanker, R. U. (2021). Seed treatment with systemic fungicides: time for review. Front. Plant Sci. 1581. doi: 10.3389/fpls.2021.654512
Badger, P. C. (2002). Ethanol from cellulose: a general review. Trends New Crops New Uses. 14, 17–21.
Baibakova, E. V., Nefedjeva, E. E., Suska-Malawska, M., Wilk, M., Sevriukova, G. A., Zheltobriukhov, V. F. (2019). Modern fungicides: mechanisms of action, fungal resistance and phytotoxic effects. Annu. Res. Rev. Biol., 1–16. doi: 10.9734/arrb/2019/v32i330083
Bastos, R. W., Rossato, L., Goldman, G. H., Santos, D. A. (2021). Fungicide effects on human fungal pathogens: cross-resistance to medical drugs and beyond. PloS Pathog. 7 (12), e1010073. doi: 10.1371/journal.ppat.1010073
Biswas, P. K., Haynes, R. L. (1970). Herbicidal effects on water uptake of seeds of selected weed species. Physiol. Plant. 23 (3), 583–590. doi: 10.1111/j.1399-3054.1970.tb06450.x
Błaszczyk, L., Salamon, S., Mikołajczak, K. (2021). Fungi inhabiting the wheat endosphere. Pathogens 10 (10), 1288. doi: 10.3390/pathogens10101288
Brauer, V. S., Rezende, C. P., Pessoni, A. M., De Paula, R. G., Rangappa, K. S., Chandra, S., et al. (2019). Antifungal agents in agriculture: friends and foes of public health. Biomolecules 9, 521. doi: 10.3390/biom9100521
Carpýcý, E. B., Celýk, N., Bayram, G. (2009). Effects of salt stress on germination of some maize (Zea mays l.) cultivars. Afr. J. Biotechnol. 8 (19).
Coker, C., Rupe, J. C., Monfort, S., Kirkpatrick, T. L. (2010). Arkansas Plant disease control products guide. In: USA: Little Rock; 2010.
Conner, R. L., Hwang, S. F., Stevens, R. R. (1996). Fusarium proliferation: a new causal agent of black point in wheat. Can. J. Plant Pathol. 18 (4), 419–423. doi: 10.1080/07060669609500598
Costa, E. M., Nunes, B. M., Ventura, M. V., Mortate, R. K., Vilarinho, M. S., Da Silva, R. M., et al. (2019). Physiological effects of insecticides and fungicide, applied in the treatment of seeds, on the germination and vigor of soybean seeds. J. Agric. Sci. 11, 318. doi: 10.5539/jas.v11n4p318
Doehlemann, G., Ökmen, B., Zhu, W., Sharon, A. (2017). Plant pathogenic fungi. Microbiol. Spectr. 5 (1), 5-10. doi: 10.1128/microbiolspec.FUNK-0023-2016
Elfikrie, N., Ho, Y. B., Zaidon, S. Z., Juahir, H., Tan, E. S. S. (2020). Occurrence of pesticides in surface water, pesticides removal efficiency in drinking water treatment plant and potential health risk to consumers in tengi river basin, Malaysia. Sci. Total. Environ. 712, 136540. doi: 10.1016/j.scitotenv.2020.136540
Ellis, R. H., Roberts, E. H. (1981). The quantification of ageing and survival in orthodox seeds (Netherlands: Seed Science and Technology).
Fan, F., Hamada, M. S., Li, N., Li, G. Q., Luo, C. X. (2017). Multiple fungicide resistance in botrytis cinerea from greenhouse strawberries in hubei province, China. Plant Dis. 101 (4), 601–606. doi: 10.1094/PDIS-09-16-1227-RE
Fisher, M. C., Alastruey-Izquierdo, A., Berman, J., Bicanic, T., Bignell, E. M., Bowyer, P., et al. (2022). Tackling the emerging threat of antifungal resistance to human health. Nat. Rev. Microbiol. 20 (9), 557–571. doi: 10.1038/s41579-022-00720-1
Gairola, K. C., Nautiyal, A. R., Dwivedi, A. K. (2011). Effect of temperatures and germination media on seed germination of jatropha curcas Linn. Adv. Biores. 2 (2), 66–71.
Gros, M., Rodríguez-Mozaz, S., Barceló, D. (2013). Rapid analysis of multiclass antibiotic residues and some of their metabolites in hospital, urban wastewater and river water by ultra-high-performance liquid chromatography coupled to quadrupole-linear ion trap tandem mass spectrometry. J. Chromatogr. A. 1292, 173–188. doi: 10.1016/j.chroma.2012.12.072
Hadas, A. (1976). Water uptake and germination of leguniinous seeds under changing external water potential in osmotic solutions. J. Exp. Bot. 27 (3), 480–489. doi: 10.1093/jxb/27.3.480
Htwe, N. M. P. S., Yuasa, T., Ishibashi, Y., Tanigawa, H., Okuda, M., Zheng, S. H., et al. (2011). Leaf senescence of soybean at reproductive stage is associated with induction of autophagy-related genes, GmATG8c, GmATG8i and GmATG4. Plant Production. Sci. 14 (2), 141–147. doi: 10.1626/pps.14.14
Janmajay, S., Seweta, S., Asha, S., Bandana, B. (2011). Studies on seed mycoflora of wheat (Triticum aestivum l.) treated with potassium nitrate and its effect on germination during storage. Res. J. Seed. Sci. 4 (3), 148–156.
Jauregi, L., Epelde, L., Alkorta, I., Garbisu, C. (2021). Antibiotic resistance in agricultural soil and crops associated to the application of cow manure-derived amendments from conventional and organic livestock farms. Front. Vet. Sci. 23 (8), 633858. doi: 10.3389/fvets.2021.633858
Javed, A., Shah, A. H., Hussain, A., Khan, S. A., Khan, S. A., Hamayun, M., et al. (2019). Identification and characterization of penicillium chrysogenum T8 as potent plant growth promoting endophytic fungi. FEB-FRESENIUS. Environ. Bull. 4896.
Jones-Lepp, T. L., Sanchez, C. A., Moy, T., Kazemi, R. (2010). Method development and application to determine potential plant uptake of antibiotics and other drugs in irrigated crop production systems. J. Agric. Food Chem. 58 (22), 11568–11573. doi: 10.1021/jf1028152
Kaur, N. (2016). Root rot pathogens of wheat in south Dakota and their effect on seed germination and seedling blight in spring wheat cultivars. Electronic. Theses. Dissertations. 1117.
Kitchen, J. L., van den Bosch, F., Paveley, N. D., Helps, J., van den Berg, F. (2016). The evolution of fungicide resistance resulting from combinations of foliar-acting systemic seed treatments and foliar-applied fungicides: a modeling analysis. PloS One 11 (8), e0161887. doi: 10.1371/journal.pone.0161887
Komárek, M., Čadková, E., Chrastný, V., Bordas, F., Bollinger, J. C. (2010). Contamination of vineyard soils with fungicides: a review of environmental and toxicological aspects. Environ. Int. 36 (1), 138–151. doi: 10.1016/j.envint.2009.10.005
Kookana, R. S., Baskaran, S. N. R. S. N. R., Naidu, R. (1998). Pesticide fate and behaviour in Australian soils in relation to contamination and management of soil and water: a review. Soil Res. 36 (5), 715–764. doi: 10.1071/S97109
Li, H., Li, Y., Wang, W., Wan, Q., Yu, X., Sun, W. (2022). Uptake, translocation, and subcellular distribution of three triazole pesticides in rice. Environ. Sci. pollut. Res. 1, 1–10. doi: 10.1007/s11356-021-17467-6
Lucas, J. A., Hawkins, N. J., Fraaije, B. A. (2015). The evolution of fungicide resistance. Adv. Appl. Microbiol. 90, 29–92. doi: 10.1016/bs.aambs.2014.09.001
Lugtenberg, B. J., Caradus, J. R., Johnson, L. J. (2016). Fungal endophytes for sustainable crop production. FEMS Microbiol. Ecol. 92 (12). doi: 10.1093/femsec/fiw194
Matadha, N. Y., Mohapatra, S., Siddamallaiah, L., Udupi, V. R., Gadigeppa, S., Raja, D. P. (2019). Uptake and distribution of fluopyram and tebuconazole residues in tomato and bell pepper plant tissues. Environ. Sci. pollut. Res. 28 (26), 6077–6086. doi: 10.1007/s11356-018-04071-4
McGee, D. C. (1995). Epidemiological approach to disease management through seed technology. Annu. Rev. Phytopathol. 33 (1), 445–466. doi: 10.1146/annurev.py.33.090195.002305
Montaña, L. A., Fischer, G., Magnitskiy, S., Zuluaga, G. (2014). Effect of NaCl salinity on seed germination and seedling emergence of purple passion fruit (Passiflora edulis sims). Agronomía. Colombiana. 32 (2), 188–195. doi: 10.15446/agron.colomb.v32n2.38287
North Dakota State University (2009) Use fungicide treated seed to prevent diseases. Available at: https://www.ag.ndsu.edu/news/newsreleases/2009/april-20-2009/use-fungicide-treated-seed-to-prevent-diseases (Accessed April 20, 2009).
Oukhouia, M., Hamdani, H., Jabeur, I., Sennouni, C., Remmal, A. (2017). In-vitro Study of anti-fusarium effect of thymol, carvacrol, eugenol and menthol. J. Plant Pathol. Microbiol. 8 (10), 1000423. doi: 10.4172/2157-7471.1000423
Pan, M., Wong, C. K., Chu, L. M. (2014). Distribution of antibiotics in wastewater-irrigated soils and their accumulation in vegetable crops in the pearl river delta, southern China. J. Agric. Food Chem. 62 (46), 11062–11069. doi: 10.1021/jf503850v
Pang, F., Tao, A., Ayra-Pardo, C., Wang, T., Yu, Z., Huang, S. (2022). Plant organ-and growth stage-diversity of endophytic bacteria with potential as biofertilisers isolated from wheat (Triticum aestivum l.). BMC Plant Biol. 22 (1), 1–16. doi: 10.1186/s12870-022-03615-8
Patel, J. K., Gohel, K., Patel, H., Solanki, T. (2021). Wheat growth dependent succession of culturable endophytic bacteria and their plant growth promoting traits. Curr. Microbiol. 78 (12), 4103–4114. doi: 10.1007/s00284-021-02668-6
Pfeiffer, T., von Galen, A., Zink, P., Hübner, S., Linkies, A., Felgentreu, D., et al. (2021). Selection of bacteria and fungi for control of soilborne seedling diseases of maize. J. Plant Dis. Prot. 128 (5), 1227–1241. doi: 10.1007/s41348-021-00498-z
Rahman, M. S., Miyake, H., Takeoka, Y. (2001). Effect of sodium chloride salinity on seed germination and early seedling growth of rice (Oryza sativa l.). Pak. J. Bot 1 (4), 304–309. doi: 10.3923/pjbs.2001.351.355
Rajput, M. A., Pathan, M. A., Lodhi, A. M., Shah, G. S., Khanzada, K. A. (2005). Studies on seed-borne fungi of wheat in sindh province and their effect on seed germination. Pakistan J. Bot. 37 (1), 181–185.
Ren, J., Jin, X., Zhang, Q., Zheng, Y., Lin, D., Yu, Y. (2017). Fungicides induced triazole-resistance in aspergillus fumigatus associated with mutations of TR46/Y121F/T289A and its appearance in agricultural fields. J. Hazard. Mater. 326, 54–60. doi: 10.1016/j.jhazmat.2016.12.013
Sahu, P. K., Tilgam, J., Mishra, S., Hamid, S., Gupta, A., Verma, S. K., et al. (2022). Surface sterilization for isolation of endophytes: ensuring what (not) to grow. J. Basic. Microbiol. 62 (6), 647–668. doi: 10.1002/jobm.202100462
Salachna, P., Grzeszczuk, M., Meller, E., Soból, M. (2018). Oligo-alginate with low molecular mass improves growth and physiological activity of eucomis autumnalis under salinity stress. Molecules 23 (4), 812. doi: 10.3390/molecules23040812
Schüler, L. M., Gangadhar, K. N., Duarte, P., Placines, C., Molina-Márquez, A. M., Léon-Bañares, R., et al. (2020). Improvement of carotenoid extraction from a recently isolated, robust microalga, tetraselmis sp. CTP4 (chlorophyta). Bioprocess. Biosyst. Eng. 43 (5), 785–796. doi: 10.1007/s00449-019-02273-9
Serra, A. A., Couée, I., Renault, D., Gouesbet, G., Sulmon, C. (2015). Metabolic profiling of lolium perenne shows functional integration of metabolic responses to diverse subtoxic conditions of chemical stress. J. Exp. Bot. 66 (428), 1801–1816. doi: 10.1093/jxb/eru518
Simón, M. R., Börner, A., Struik, P. C. (2021). Fungal wheat diseases: etiology, breeding, and integrated management. Front. Plant Sci. 12. doi: 10.3389/fpls.2021.671060
Singh, G., Sahota, H. K. (2018). Impact of benzimidazole and dithiocarbamate fungicides on the photosynthetic machinery, sugar content and various antioxidative enzymes in chickpea. Plant Physiol. Biochem. 1 (132), 166–173. doi: 10.1016/j.plaphy.2018.09.001
Sykes, E. M., Sackett, K. E., Severns, P. M., Mundt, C. C. (2018). Sensitivity variation and cross-resistance of zymoseptoria tritici to azole fungicides in north America. Eur. J. Plant Pathol. 151 (1), 269–274. doi: 10.1007/s10658-017-1370-y
Takahashi, J. P. F., Feliciano, L. M., Santos, D. C. S., Ramos, S., Oliveira, R. A., Attili-Angelis, D., et al. (2020). Could fungicides lead to azole drug resistance in a cross-resistance manner among environmental cryptococcus strains? Curr. Fungal Infect. Rep. 14 (1), 9–14. doi: 10.1007/s12281-020-00373-8
Tao, Y., Xing, Y., Jing, J., Yu, P., He, M., Zhang, J., et al. (2022). Insight into the uptake, accumulation, and metabolism of the fungicide phenamacril in lettuce (Lactuca sativa l.) and radish (Raphanus sativus l.). Environ. pollut. 1 (304), 119240.
Tetz, G., Cynamon, M., Hendricks, G., Vikina, D., Tetz, V. (2017). In vitro Activity of a novel compound, mul-1867, against clinically significant fungi candida spp. and aspergillus spp. Int. J. Antimicrob. Agents 50 (1), 47–54. doi: 10.1016/j.ijantimicag.2017.02.011
Tetz, V., Kardava, K., Krasnov, K., Vecherkovskaya, M., Tetz, G. (2023). Antifungal activity of the novel compound M451 against phytopathogens. bioRxiv. doi: 10.1101/2023.02.04.525039
Tetz, G., Tetz, V. (2015). In vitro Antimicrobial activity of a novel compound, mul-1867, against clinically important bacteria. Antimicrob. Resist. Infect. Control. 4 (1), 1–6. doi: 10.1186/s13756-015-0088-x
Tetz, V., Tetz, G. (2022). Novel cell receptor system of eukaryotes formed by previously unknown nucleic acid-based receptors. Receptors 1, 13–53. doi: 10.3390/receptors1010003
Toghueo, R. M. K., Boyom, F. F. (2020). Endophytic penicillium species and their agricultural, biotechnological, and pharmaceutical applications. 3 Biotech. 10 (3), 1–35. doi: 10.1007/s13205-020-2081-1
Tsegay, B. A., Gebreslassie, B. (2014). The effect of salinity (NaCl) on germination and early seedling growth of lathyrus sativus and pisum sativum var. abyssinicum. Afr. J. Plant Sci. 8 (5), 225–231. doi: 10.5897/AJPS2014.1176
Tunali, B., Nicol, J. M., Hodson, D., Uçkun, Z., Büyük, O., Erdurmuş, D., et al. (2008). Root and crown rot fungi associated with spring, facultative, and winter wheat in Turkey. Plant Dis. 92 (9), 1299–1306. doi: 10.1094/PDIS-92-9-1299
Wen, Z., Wang, J., Jiao, C., Shao, W., Ma, Z. (2022). Biological and molecular characterizations of field fludioxonil-resistant isolates of fusarium graminearum. Pesticide. Biochem. Physiol. 105101. doi: 10.1016/j.pestbp.2022.105101
Wolny, E., Betekhtin, A., Rojek, M., Braszewska-Zalewska, A., Lusinska, J., Hasterok, R. (2018). Germination and the early stages of seedling development in brachypodium distachyon. Int. J. Mol. Sci. 19 (10), 2916. doi: 10.3390/ijms19102916
Yang, L. N., He, M. H., Ouyang, H. B., Zhu, W., Pan, Z. C., Sui, Q. J., et al. (2019). Cross-resistance of the pathogenic fungus alternaria alternata to fungicides with different modes of action. BMC Microbiol. 19 (1), 1–10. doi: 10.1186/s12866-019-1574-8
Zaller, J. G., König, N., Tiefenbacher, A., Muraoka, Y., Querner, P., Ratzenböck, A., et al. (2016). Pesticide seed dressings can affect the activity of various soil organisms and reduce decomposition of plant material. BMC Ecol. 16 (1), 1–11. doi: 10.1186/s12898-016-0092-x
Zhang, D., Wang, Z., Wang, N., Gao, Y., Liu, Y., Wu, Y., et al. (2014). Tissue culture-induced heritable genomic variation in rice, and their phenotypic implications. PloS One 9 (5), e96879. doi: 10.1371/journal.pone.0096879
Zhang, Y. J., Zhang, X., Chen, C. J., Zhou, M. G., Wang, H. C. (2010). Effects of fungicides JS399-19, azoxystrobin, tebuconazloe, and carbendazim on the physiological and biochemical indices and grain yield of winter wheat. Pesticide. Biochem. Physiol. 98 (2), 151–157. doi: 10.1016/j.pestbp.2010.04.007
Keywords: Triticum aestivum, fungicide, endophytes, pathogen, M451, pathogen resistance
Citation: Kardava K, Tetz V, Vecherkovskaya M and Tetz G (2023) Seed dressing with M451 promotes seedling growth in wheat and reduces root phytopathogenic fungi without affecting endophytes. Front. Plant Sci. 14:1176553. doi: 10.3389/fpls.2023.1176553
Received: 28 February 2023; Accepted: 17 April 2023;
Published: 17 May 2023.
Edited by:
Sabrina Sarrocco, University of Pisa, ItalyReviewed by:
Praveen Satapute, Karnatak University, IndiaMalkhan Singh Gurjar, Indian Agricultural Research Institute (ICAR), India
Copyright © 2023 Kardava, Tetz, Vecherkovskaya and Tetz. This is an open-access article distributed under the terms of the Creative Commons Attribution License (CC BY). The use, distribution or reproduction in other forums is permitted, provided the original author(s) and the copyright owner(s) are credited and that the original publication in this journal is cited, in accordance with accepted academic practice. No use, distribution or reproduction is permitted which does not comply with these terms.
*Correspondence: G. Tetz, g.tetz@hmi-us.com