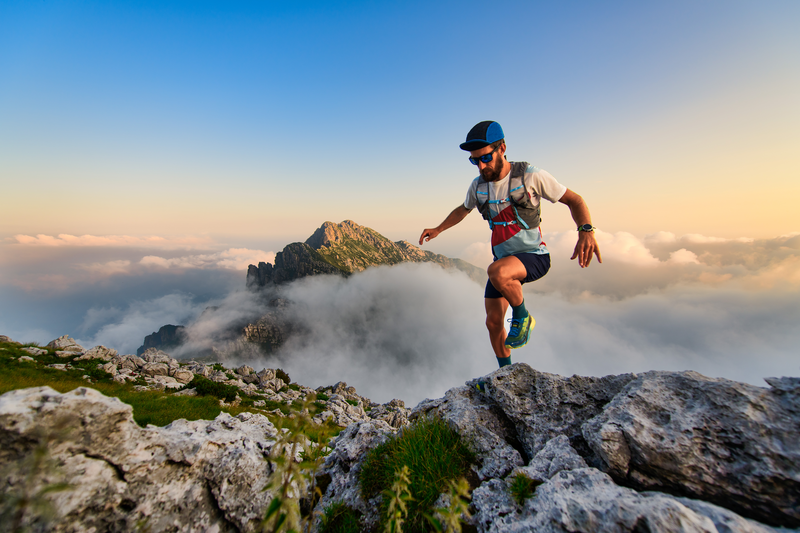
94% of researchers rate our articles as excellent or good
Learn more about the work of our research integrity team to safeguard the quality of each article we publish.
Find out more
ORIGINAL RESEARCH article
Front. Microbiol. , 19 May 2023
Sec. Microbe and Virus Interactions with Plants
Volume 14 - 2023 | https://doi.org/10.3389/fmicb.2023.1176428
This article is part of the Research Topic Next Generation Solutions for Efficient Management of Phytopathogens View all 7 articles
Phytopathogenic fungi are the predominant causal agents of plant diseases. Available fungicides have substantial disadvantages, such as being insufficiently effective owing to intrinsic tolerance and the spread of antifungal resistance accumulating in plant tissues, posing a global threat to public health. Therefore, finding a new broad-spectrum fungicide is a challenge to protect plants. We studied the potency of a novel antimicrobial agent, M451, a 1,6-diaminohexane derivative, against different phytopathogenic fungi of the Ascomycota, Oomycota, and Basidiomycota phyla. M451 exhibited significant antifungal activity with EC50 values from 34–145 μg/mL. The minimal fungicidal concentration against Fusarium oxysporum ranged from 4 to 512 μg/mL depending on the exposure times of 5 min to 24 h. M451 has the highest activity and significantly lower exposure times compared to different polyene, azole, and phenylpyrrole antifungals. The conidial germination assay revealed that M451 induced 99 and 97.8% inhibition against F. oxysporum within 5 min of exposure to 5,000 and 500 μg/mL, respectively. Germ tube elongation, spore production, and spore germination were also significantly inhibited by M451 at concentrations of ≥50 μg/mL. Based on the broad spectrum of antifungal effects across different plant pathogens, M451 could be a new chemical fungicide for plant disease management.
Phytopathogenic fungi are among the predominant causal agents of plant diseases and can severely interrupt the normal growth of crops, fruits, and vegetables (Blackwell, 2011; Fisher et al., 2012; Doehlemann et al., 2017; Jain et al., 2019). Over 19,000 fungal species, primarily representatives of the Ascomycota, Oomycota, and Basidiomycota phyla, cause various plant infections ranging from persisting in and using living plant tissues to killing the plants to extract nutrients (Doehlemann et al., 2017). Along with direct economic effects due to pre- and post-harvest plant losses, fungal pathogens secrete multiple secondary metabolites, including mycotoxins, which can contaminate agricultural products that harm animal and human health (Gurikar et al., 2022). Moreover, some plant fungi can cause opportunistic infections in humans and other animals, which, although relatively rare, are characterized by broad resistance to antifungal agents (Ma et al., 2013).
Representatives of the Fusarium genus are some of the most economically critical fungal phytopathogens, potent mycotoxin producers, and most frequent opportunistic human pathogens (Lazarovits et al., 2014; Doehlemann et al., 2017; Möller and Stukenbrock, 2017). Plant diseases caused by Fusarium species are challenging to prevent and control as the infection may be transmitted through the roots in soil or via air or water in above-ground parts (Ma et al., 2013). Fusarium oxysporum is one of the top 10 fungal plant pathogens, affecting the yield, quality, and storage life of harvested plants. The economic impact of diseases caused by Fusarium determines the relevance of using fungicides in agriculture (McGrath, 2004; Dean et al., 2012; Arie, 2019; Steinberg and Gurr, 2020).
The limited number of existing fungicides and the high resistance to available antifungal compounds contribute to the challenges in controlling fungal plant diseases (Doehlemann et al., 2017). Moreover, many environmental antibiotics, such as azoles or polyenes, used in agriculture are closely related to those used to treat human fungal infections resulting in cross-resistance to the drugs in humans (Lucas et al., 2015; Dalhoff, 2018; Brauer et al., 2019; Matsuzaki et al., 2020; Bastos et al., 2021; Jørgensen and Heick, 2021). Therefore, highly efficient novel antifungal agents not used in human medicine to control agricultural fungal diseases are needed.
Our group previously developed a novel antimicrobial agent, Mul-1867, which possesses a broad spectrum of antimicrobial activity by nonspecifically attacking the fungal cell wall (Tetz et al., 2017; Tetz and Tetz, 2022b). Mul-1867 is effective against Candida spp. and Aspergillus spp. isolated from patients with fungal lung infections. Mul-1867 was highly effective against resistant clinical isolates, and low minimum fungicidal concentrations were also effective against pre-formed yeast and mold biofilms.
In the present study, we studied the derivative of Mul-1867 developed by our group, TGV-28 (poly-(N-carboxamido-1,6-diaminohexane) particularly N′-aminated) for the first time, which had more potent antifungal activity against different unrelated phytopathogens including Fusarium spp., Blumeria spp., Claviceps spp., Alternaria spp., and Phytophthora spp. We also studied the potentiation of the antifungal activity of TGV-28 against phytopathogenic fungi by using M451, a is a phosphate salt of TGV-28 and the permeation enhancer 0.01% NaH2PO4. (Dahlgren et al., 2020).
The in vitro antifungal activity was determined against the following plant pathogenic fungi: F. oxysporum VT-23, F. culmorum VT-45, F. graminearum VT-60, F. sporotrichioides VT-102, F. solani VT-60, F. verticillioides VT-125 (all from Human Microbiology Institute collection, NY, United States), F. proliferatum KSU 4853 (Kansas State University, KS, United States), F. dimerum KSU 14971 (Kansas State University KS, United States), Blumeria graminis, Claviceps purpurea, Alternaria alternata, and Phytophtora infestans (all from the Human Microbiology Institute collection). All tested isolates were identified using classic cultivation methods and coupled with 16S ribosomal RNA sequencing for isolate identification and preserved as pure cultures in the collection of the Human Microbiology Institute (New York, NY, United States) (Camele et al., 2021). The fungi were maintained as pure cultures at 4°C and subcultured on potato dextrose agar (PDA; CM0139; Thermo Fisher Scientific Inc., Waltham, MA United States) (Camele et al., 2021).
The experimental compound M451 (Human Microbiology Institute) is a phosphate salt of TGV-28 (poly-(N-carboxamido-1,6-diaminohexane) particularly N′-aminated) and the permeation enhancer 0.01% NaH2PO4 (Figure 1). TGV-28, a derivative of Mul-186, has more polymeric chains than its predecessor, rendering higher antifungal activity (Winiwarter et al., 2007).
We used agriculture-purpose antifungals as positive controls: prothioconazole and tebuconazole (both azole derivatives), natamycin (a polyene derivative), fludioxonil, NaH2PO4 (all from Sigma–Aldrich, St. Louis, MO, United States), and Maxim® XL (Syngenta Canada Inc., Canada).
Mycelial growth inhibition was evaluated as previously described (Baggio et al., 2014; Chen et al., 2014). For subculturing, varying concentrations of fungicidal agents were added to 90-mm Petri dishes filled with PDA (cooled to ~50°C) before it solidified to obtain M451 0.1%, M451 0.05%, NaH2PO4 0.1%, or fludioxonil 0.25% plates. The control plate contained untreated PDA medium. To test the 13 fungal strains, 3 mm diameter mycelium plugs of each fungal strain were cut from the edge of a 5 day-old colony grown on stock PDA plates, transferred to the control and experimental Petri dishes, and incubated at 25°C for 120 h. The diameter of the colonies was measured, and the growth inhibition percentage was calculated.
Spore production was determined as previously described (Baggio et al., 2014). To test F. oxysporum spore production, 3 mm diameter mycelial plugs were taken from the edge of a five-day-old colony and transferred into the control and test tubes. The control tubes contained 10 mL dH20, 10 μL Tween 20, and 30 μL lactoglycerol as spore germination preventers. The test tubes contained the same mixture of fungicides at various concentrations. The spores were counted using a Malassez counting chamber (Thermo Fisher Scientific, United States) by transferring 0.2 mL from each tube.
Spore germination was analyzed using a previously described method (Benslim et al., 2017), in which 1 mL of spore suspension (107 spores/mL) was placed in a series of microtubes, and 20 μL of M451 was added. The tubes were prepared in triplicate and incubated for 24 h at 25°C. After incubation, spore germination inhibition was observed under an AxioStar Plus light microscope (Carl Zeiss, Germany; objective lens: A-Plan 100х/1.25) using a Malassez cell counting chamber. The percentage of non-germinated spores was calculated using Equation 1:
%SNG: percentage of non-germinated spores.
SG: number of germinated spores.
SNG: number of non-germinated spores.
The minimal fungicidal concentration (MFC) of the tested products was assessed using the standard broth microdilution method, according to the recommendations of the Clinical and Laboratory Standards Institute with RPMI 1640 (Thermo Fisher Scientific, United States) (Clinical and Laboratory Standards Institute, 2008). The standard inoculum for fungal testing was a fungal suspension of 0.5 McFarland. MFC was defined as the lowest concentration of the tested compound resulting in no visual growth after 48 h of incubation at 25°C. After the experiment, the microdilution plates were examined for visible Fusarium spp. growth.
We performed a time-kill test in vitro to determine the minimum concentration and exposure time required for the tested compounds to kill the fungi. We assessed the activity of serially diluted compounds against F. oxysporum as described by Ernst et al. (1998). All fungal cultures were grown for 120 h as liquid cultures, and 150 μL of the inoculum (5 × 105 colony-forming units (CFU)/mL) for each strain was transferred to 96-well microtiter plates (Sarstedt, Numbrecht, Germany). The plates were incubated for 120 h at 25°C. Then, 50 μL of the tested compounds at different concentrations were added for 5 min, 30 min, 1 h, 3 h, 6 h, and 24 h. Untreated probes were used as negative controls. After exposure, the fungal suspension was centrifuged at 4000 × g for 15 min and washed with deionized water. Centrifugation and washing were repeated twice. The probes were diluted in 11 mL phosphate-buffered saline, and the total CFU number was determined through serial dilution and plating on PDA. All assays included at least two replicates and were repeated in three independent experiments.
The germ tube elongation was performed following a previously reported procedure (Chen et al., 2014). To test the inhibition of germ tube elongation, we prepared a spore suspension of F. oxysporum (107 spores/mL). One hundred microliters of this suspension were placed on control and experimental plates supplemented with M451 at various concentrations. The plates were incubated for 10 h at room temperature. Germ tube length was measured for 100 germinated spores at 400× magnification (Axiostar plus (Carl Zeiss, Germany)) using Fiji software (Schindelin et al., 2012).
The conidial germination assay was conducted as previously reported (Oukhouia et al., 2017). M451 (measured for TGV-28) and fludioxonil were diluted in 0.9% NaCl, mixed with 0.2% agar, and added to 5 day-old F. oxysporum conidia suspension (106 conidia/mL) to a final concentration of 5,000, 500, 100, 50, 5, 1, or 0.5 μg/mL. After 1, 3, 6, and 24 h of incubation at 25°C, the number of germinated conidia was determined using the Malassez counting chamber in 10 fields with standard techniques (Niemann and Baayen, 1989).
All data were analyzed using GraphPad Prism version 9.3.1. Two-way analysis of variance (ANOVA) was used for multiple comparisons at a 95% confidence level (p < 0.05). Data are presented as the mean ± standard deviation (SD) with three independent replicates. All experiments were performed in triplicate.
We tested TGV-28 and NaH2PO4, alone and in combination (M451), against different species from the Ascomycota, Oomycota, and Basidiomycota phyla. TGV-28 was highly effective against all strains, with the MFC 64.3–96.3 μg/mL (Table 1). As expected, NaH2PO4 at a 100 μg/mL concentration did not exhibit antifungal activity (data not shown). Surprisingly, the TGV-28 and NaH2PO4 combination increased the antifungal activity of TGV-28 against F. oxysporum by 49% (p < 0.05). Therefore, in subsequent experiments, we used a complex of TGV-28 and NaH2PO4 named M451.
M451 exhibited significant activity against all tested plant-pathogenic fungal isolates and inhibited the in vitro mycelial growth of all Ascomycota, Oomycota, and Basidiomycota strains (Table 2), with an EC50 of 34–145 μg/mL and a high correlation coefficient. The EC50 of M451 against Fusarium spp. (phylum Ascomycota) varied from 66 μg/mL to 145 μg/mL. The activity against other non-Fusarium representatives of the Ascomycota phylum was higher, with EC50 values ranging from 34 to 52 μg/mL. The EC50 values for Phytophtora infestans (phylum Oomycota) and Rhizoctonia solani (phylum Basidiomycota) were 58 and 53 g/mL, respectively.
We then compared the antifungal activity of M451 and fludioxonil against the same fungal isolates. M451 revealed dose-dependent activity: 0.1% M451 displayed 91–97% mycelial growth inhibition, and 0.05% M451 resulted in a slightly lower decrease in mycelial growth with 82–86.7% inhibition among different fungal pathogens (Figure 2A). Notably, M451 at a concentration of 0.05 and 0.1% was more effective against all tested fungi than fludioxonil (the active ingredient in Maxim XL), which, when used at a fixed concentration of 0.25% according to the manufacturer’s recommendations, inhibited mycelial growth by only 32–47% (p < 0.05 for the control, M451 0.1%, and M451 0.05%). Representative images of the mycelial growth inhibition of F. oxysporum by M451 are shown in Figures 2A–E.
Figure 2. Effect of tested compounds on fungal growth. (A) Mycelial growth inhibition of plant phytopathogens. (B–E) Antifungal activity against F. oxysporum. Representative images from three independent experiments of fungi cultivated for five days at 25°C after treatment with the tested compounds. (B) Negative control, (C) M451 0.1%, (D) M451 0.05%, (E) Fludioxonil 0.25%. #p < 0.05 compared to the control, ‡p < 0.05 compared to fludioxonil.
Table 3 shows the MFCs of M451 and antifungal antibiotics used in agriculture representing polyene and azole classes and stand-alone Maxim XL (titrated depending on the fludioxonil concentration) against F. oxysporum. The time-kill study against planktonic fungi demonstrated a higher antifungal activity of M451 at all tested time points. At the end of 24 h of exposure, the antifungal activity of M451 was 5–50 times higher than that of other antifungal agents. Notably, M451 was the only tested antifungal agent that showed antifungal activity from 5 min of exposure.
Next, we studied the effect of different M451 concentrations corresponding to 5,000–5 μg/mL TGV-28 compared to Maxim XL on F. oxysporum conidial germination (Figure 3). M451 treatment at 5000 and 500 μg/mL inhibited conidial germination by 99 and 97.8%, respectively, after 5 min of exposure. We could see the dose- and time-dependent effects of M451 at lower concentrations. Thirty minutes of incubation with M451 at 100 μg/mL resulted in 53.8% inhibition; increasing the incubation time to 3 h resulted in 94.4% inhibition. Under the same conditions, Maxim XL had a much lower level of anti-Fusarium activity, which at a 5,000 μg/mL concentration only reduced germination by 3.6 and 67.8% after 5 min and 24 h, respectively.
Figure 3. Heatmap of the effect of different M451 and fludioxonil concentrations on F. oxysporum conidia. Growth inhibition is represented by a color scale from white (minimal) to blue (maximum). The data are representative of three independent experiments.
Finally, we determined the in vitro effects of M451 on F. oxysporum germ tube elongation, spore production, and germination (Figure 4). Germ tube elongation was highly sensitive to M451 treatment, with >80% inhibition, even when M451 was applied at a sub-inhibitory concentration of 50 μg/mL and over 95% inhibition starting from 100 μg/mL (Figure 4A). Notably, M451 significantly inhibited spore production at all tested concentrations. Even at the lowest tested concentration of 50 μg/mL, M451 inhibited spore production by over 2-fold. This inhibition might be explained by the quick action of M451 when the compound kills fungi faster than the sporulation process starts (Figure 4B). Finally, M451 had a dose-dependent inhibitory effect on Fusarium spore germination (Figure 4C). Thus, M451 at 50 and 100 μg/mL reduced spore germination from 73 to 83% compared to the control, respectively, and from concentrations of 500 μg/mL, M451 almost completely inhibited this process.
Figure 4. Antifungal effects of M451 on F. oxysporum. The bars represent the effects of M541 at concentrations from 50 to 5,000 μg/mL on (A) germ tube elongation, (B) spore production, and (C) spore germination. **p < 0.01, ***p < 0.001, ****p < 0.0001 compared to the control. Compiled data are from three independent experiments.
Plant fungal diseases caused by Ascomycota, Oomycota, and Basidiomycota spp. contributes to severe crop losses and a negative economic effect on essential agricultural products (Gisi and Cohen, 1996; Tudzynski and Scheffer, 2004; Haverkort et al., 2016). The primary difficulties in preventing and treating agricultural fungal diseases are the resistance of phytopathogenic fungi to existing fungicides, tolerant spore development, and insufficient studies on fungal regulation strategies (Gisi and Cohen, 1996; Avenot and Michailides, 2007; Al-Hatmi et al., 2019; Meyers et al., 2019; Jindo et al., 2021; Tetz and Tetz, 2022a,b).
Moreover, developing fungus-specific targets is complicated because fungal and mammalian cells share many of these targets. Therefore, only a few classes of antifungal agents are available, and some are used in agriculture and human health. This everyday use of antifungal agents causes the spread of antibiotic resistance in human medicine in several ways, varying from direct infection with resistant bacteria to the transfer of antibiotic resistance genes from plant to human pathogens (Chang et al., 2015). Therefore, identifying novel antifungal agents that can be used only in agriculture and not in humans is crucial for agriculture and human health.
The novel fungicide candidate TGV-28, a derivative of the broad-spectrum antimicrobial agent Mul-1867, exhibits high antifungal activity against several emergent plant pathogens, including representative Fusarium spp., Erysiphe spp., Phytophthora spp., and Rhizoctonia spp. Moreover, its antimicrobial activity against specific fungal plant pathogens was potentiated by the permeation enhancer NaH2PO4. Therefore, we also studied a complex of TGV-28 and NaH2PO4 named M451. However, the detailed mechanisms underlying this selective potentiation against only a particular type of fungi (F. oxysporum) are a subject for future research and are outside the scope of this study. M451 exhibited significant antifungal activity against Fusarium spp. as a seed dressing for wheat seeds, which exceeded the commercially used fungicide Maxim XL against both seed- and soil-borne F. oxysporum infections (Kardava et al., 2023).
We were particularly interested in evaluating the antifungal effect of M451 against F. oxysporum owing to its importance as a devastating plant pathogen (Ma et al., 2013; De Corato et al., 2020). We compared the activity of M451 with that of different agriculture-purpose antifungal agents, including azole and polyene derivatives and fludioxonil. M451 had lower MFCs at all measured time points and could inhibit the fungal growth even at concentrations substantially lower than the anticipated M451 concentrations (0.05–0.1%); therefore, we envision that M451 can be used in real-world agricultural settings. Moreover, unlike other tested antifungal agents, M451-induced rapid killing of F. oxysporum after 5 min of exposure. The fast-acting antifungal effect was expected because TGV-28 and its predecessor Mul-1867 exhibit a topical mechanism of antifungal action without metabolizing it (Tetz et al., 2017). One of the pathways through which fungi overcome antibiotic challenges is to develop highly resistant spores. The results confirmed that M451 prevents fungal survival by negatively affecting sporulation even after a short exposure time.
We compared the activity of M451 and fludioxonil against F. oxysporum conidia, as conidial stress resistance is higher than that of vegetative fungal cells (Wyatt et al., 2013). Whereas M451 exhibited a high antifungal activity from 5 min of exposure, fludioxonil required considerably more time to show antifungal activity. At the end of the observation period, fludioxonil could not inhibit fungal growth even at the highest concentration tested, which exceeded two-fold that recommended by the manufacturer. This finding was most likely due to the experimental settings of the conidial germination assay, which enabled fungi to escape from the antifungal effects by asexual sporulation. These results are consistent with recently published data that fludioxonil has limited activity in field studies owing to limited activity towards conidia and sexual spores, although it is broadly used in agriculture to treat and prevent Fusarium infection (Masiello et al., 2019).
As fungi are present in the outer environment at different life cycle stages in real-world conditions, we also compared the effect of M451 and fludioxonil on germ tube elongation, spore production, and spore germination. This experiment was conducted considering that F. oxysporum overcomes the killing effect of antifungal agents by forming highly tolerant spores. M451 exhibited a high efficacy against F. oxysporum at all developmental stages, inhibiting F. oxysporum spore germination and even possessing sporicidal activity. This class of compounds does not have significant cytotoxicity; therefore, the observed sporicidal effect could not be addressed owing to corrosive effects and is planned to be studied in the future (Tetz et al., 2017; Tetz and Tetz, 2022b). In conclusion, this study revealed high in vitro antifungal activities of M451 against difficult-to-treat plant pathogens. Fungi at different developmental stages frequently characterize real-life plant fungal infections. Therefore, one of the unique M451 features was that it inhibited vegetative proliferation and conidial and spore germination. Finally, M451 represents a novel class of chemical compounds unrelated to antifungal antibiotics used to treat human diseases. M451 does not share structural characteristics with azoles of polyene antibiotics; therefore, it is unlikely to contribute to the developing antibiotic resistance frequently seen across antifungals used in medicine and agriculture (Verweij et al., 2022). Further development of M451 can be done following the local regulatory authorities and include relevant in vivo studies.
The datasets presented in this study can be found in online repositories. The names of the repository/repositories and accession number(s) can be found at: https://www.biorxiv.org/content/10.1101/2023.02.04.525039v1.
VT, KKa, and GT conceived and supervised the research and edited and helped to draft the final manuscript. KKa and MV conducted experiments. KKa and KKr analyzed the data and wrote the manuscript. All authors contributed to the article and approved the submitted version.
The authors declare that the research was conducted without any commercial or financial relationships that could be construed as a potential conflict of interest.
All claims expressed in this article are solely those of the authors and do not necessarily represent those of their affiliated organizations, or those of the publisher, the editors and the reviewers. Any product that may be evaluated in this article, or claim that may be made by its manufacturer, is not guaranteed or endorsed by the publisher.
Al-Hatmi, A. M., de Hoog, G. S., and Meis, J. F. (2019). Multiresistant Fusarium pathogens on plants and humans: solutions in (from) the antifungal pipeline? Infect. Drug Resist. 12, 3727–3737. doi: 10.2147/IDR.S180912
Arie, T. (2019). Fusarium diseases of cultivated plants, control, diagnosis, and molecular and genetic studies. J. Pestic. Sci. 44, 275–281. doi: 10.1584/jpestics.J19-03
Avenot, H. F., and Michailides, T. J. (2007). Resistance to Boscalid fungicide in Alternaria alternata isolates from pistachio in California. Plant Dis. 91, 1345–1350. doi: 10.1094/PDIS-91-10-1345
Baggio, J. S., Lourenço, S. D. A., and Amorim, L. (2014). Eradicant and curative treatments of hexanal against peach brown rot. Sci. Agric. 71, 72–76. doi: 10.1590/S0103-90162014000100010
Bastos, R. W., Rossato, L., Goldman, G. H., and Santos, D. A. (2021). Fungicide effects on human fungal pathogens: cross-resistance to medical drugs and beyond. PLoS Pathog. 17:e1010073. doi: 10.1371/journal.ppat.1010073
Benslim, A., Mezaache-Aichour, S., Haichour, N., Chebel, S., and Zerroug, M. M. (2017). Evaluation of inhibition of fungal spore germination by rhizospheric bacterial extracts. Ann. Res. Rev. Biol. 11, 1–7. doi: 10.9734/ARRB/2016/31228
Blackwell, M. (2011). The fungi: 1, 2, 3 5.1 million species? Am. J. Bot. 98, 426–438. doi: 10.3732/ajb.1000298
Brauer, V. S., Rezende, C. P., Pessoni, A. M., De Paula, R. G., Rangappa, K. S., Nayaka, S. C., et al. (2019). Antifungal agents in agriculture: friends and foes of public health. Biomol. Ther. 9:521. doi: 10.3390/biom9100521
Camele, I., Gruľová, D., and Elshafie, H. S. (2021). Chemical composition and antimicrobial properties of Mentha × piperita cv ‘Kristinka’essential oil. Plants 10:1567. doi: 10.3390/plants10081567
Chang, Q., Wang, W., Regev-Yochay, G., Lipsitch, M., and Hanage, W. P. (2015). Antibiotics in agriculture and the risk to human health: how worried should we be? Evol. Appl. 8, 240–247. doi: 10.1111/eva.12185
Chen, F., Han, P., Liu, P., Si, N., Liu, J., and Liu, X. (2014). Activity of the novel fungicide SYP-Z048 against plant pathogens. Sci. Rep. 4:6473. doi: 10.1038/srep06473
Clinical and Laboratory Standards Institute (2008). Reference method for broth dilution antifungal susceptibility testing of filamentous fungi: second edition. Document M38-A2. Wayne, PA: CLSI.
Dahlgren, D., Sjöblom, M., Hedeland, M., and Lennernäs, H. (2020). The in vivo effect of transcellular permeation enhancers on the intestinal permeability of two peptide drugs enalaprilat and hexarelin. Pharmaceutics 12:99. doi: 10.3390/pharmaceutics12020099
Dalhoff, A. (2018). Does the use of antifungal agents in agriculture and food foster polyene resistance development? A reason for concern. J. Global Antimicrob. Resist. 13, 40–48. doi: 10.1016/j.jgar.2017.10.024
De Corato, U., Patruno, L., Avella, N., Salimbeni, R., Lacolla, G., Cucci, G., et al. (2020). Soil management under tomato-wheat rotation increases the suppressive response against Fusarium wilt and tomato shoot growth by changing the microbial composition and chemical parameters. Appl. Soil Ecol. 154:103601. doi: 10.1016/j.apsoil.2020.103601
Dean, R., Van Kan, J. A., Pretorius, Z. A., Hammond-Kosack, K. E., Di Pietro, A., Spanu, P. D., et al. (2012). The top 10 fungal pathogens in molecular plant pathology. Mol. Plant Pathol. 13, 414–430. doi: 10.1111/j.1364-3703.2011.00783.x
Doehlemann, G., Ökmen, B., Zhu, W., and Sharon, A. (2017). Plant pathogenic Fungi. Microbiol. Spectr. 5, 5–10. doi: 10.1128/microbiolspec.FUNK-0023-2016
Ernst, E. J., Klepser, M. E., and Pfaller, M. A. (1998). In vitro interaction of fluconazole and amphotericin B administered sequentially against Candida albicans: effect of concentration and exposure time. Diagn. Microbiol. Infect. Dis. 32, 205–210. doi: 10.1016/s0732-8893(98)00099-6
Fisher, M. C., Henk, D. A., Briggs, C. J., Brownstein, J. S., Madoff, L. C., McCraw, S. L., et al. (2012). Emerging fungal threats to animal, plant and ecosystem health. Nature 484, 186–194. doi: 10.1038/nature10947
Gisi, U., and Cohen, Y. (1996). Resistance to phenylamide fungicides: a case study with Phytophthora infestans involving mating type and race structure. Annu. Rev. Phytopathol. 34, 549–572. doi: 10.1146/annurev.phyto.34.1.549
Gurikar, C., Shivaprasad, D. P., Sabillón, L., Gowda, N. N., and Siliveru, K. (2022). Impact of mycotoxins and their metabolites associated with food grains. Grain & Oil Science and Technology. 6, 1–9, doi: 10.1016/j.gaost.2022.10.001
Haverkort, A. J., Boonekamp, P. M., Hutten, R., Jacobsen, E., Lotz, L. A. P., Kessel, G. J. T., et al. (2016). Durable late blight resistance in potato through dynamic varieties obtained by cisgenesis: scientific and societal advances in the DuRPh project. Potato Res. 59, 35–66. doi: 10.1007/s11540-015-9312-6
Jain, A., Sarsaiya, S., Wu, Q., Lu, Y., and Shi, J. (2019). A review of plant leaf fungal diseases and its environment speciation. Bioengineered 10, 409–424. doi: 10.1080/21655979.2019.1649520
Jindo, K., Evenhuis, A., Kempenaar, C., Pombo Sudré, C., Zhan, X., Goitom Teklu, M., et al. (2021). Review: holistic pest management against early blight disease towards sustainable agriculture. Pest Manag. Sci. 77, 3871–3880. doi: 10.1002/ps.6320
Jørgensen, L. N., and Heick, T. M. (2021). Azole use in agriculture, horticulture, and wood preservation—is it indispensable? Front. Cell. Infect. Microbiol. 11:730297. doi: 10.3389/fcimb.2021.730297
Kardava, K., Tetz, V., Vecherkovskaya, M., and Tetz, G. (2023). Seed dressing with M451 promotes seedling growth in wheat and reduces root phytopathogenic fungi without affecting endophytes. Front. Plant Sci. 14:1546. doi: 10.3389/fpls.2023.1176553
Lazarovits, G., Turnbull, A., and Johnston-Monje, D. (2014). Plant health management: biological control of plant pathogens. In: N. K. Van Alfen, editor. Encyclopedia of agriculture and food systems. New York, NY: Academic Press. 388–399
Lucas, J. A., Hawkins, N. J., and Fraaije, B. A. (2015). The evolution of fungicide resistance. Adv. Appl. Microbiol. 90, 29–92. doi: 10.1016/bs.aambs.2014.091
Ma, L. J., Geiser, D. M., Proctor, R. H., Rooney, A. P., O’Donnell, K., Trail, F., et al. (2013). Fusarium pathogenomics. Annu. Rev. Microbiol. 67, 399–416. doi: 10.1146/annurev-micro-092412-155650
Masiello, M., Somma, S., Ghionna, V., Logrieco, A. F., and Moretti, A. (2019). In vitro and in field response of different fungicides against Aspergillus flavus and Fusarium species causing ear rot disease of maize. Toxins 11:11. doi: 10.3390/toxins11010011
Matsuzaki, Y., Kiguchi, S., Suemoto, H., and Iwahashi, F. (2020). Antifungal activity of metyltetraprole against the existing QoI-resistant isolates of various plant pathogenic fungi: metyltetraprole against QoI-R isolates. Pest Manag. Sci. 76, 1743–1750. doi: 10.1002/ps.5697
McGrath, M. T. (2004). What are fungicides? Plant Health Instruct. 10:2016. doi: 10.1094/PHI-I-2004-0825-01.Updated
Meyers, E., Arellano, C., and Cowger, C. (2019). Sensitivity of the U.S. Blumeria graminis f. sp. tritici population to Demethylation inhibitor fungicides. Plant Dis. 103, 3108–3116. doi: 10.1094/PDIS-04-19-0715-RE
Möller, M., and Stukenbrock, E. H. (2017). Evolution and genome architecture in fungal plant pathogens. Nat. Rev. Microbiol. 15, 756–771. doi: 10.1038/nrmicro.2017.76
Niemann, G. J., and Baayen, R. P. (1989). Inhibitory effects of phenylserine and salicylic acid on phytoalexin accumulation in carnations infected by Fusarium oxysporum f. sp. dianthi [Fod]. Rijksuniversiteit Faculteit Landbouwwetenschappen, Gent.
Oukhouia, M., Hamdani, H., Jabeur, I., Sennouni, C., and Remmal, A. (2017). In-vitro study of anti-Fusarium effect of thymol, carvacrol, eugenol and menthol. J. Plant Pathol. Microbiol. 8:1000423. doi: 10.4172/2157-7471.1000423
Schindelin, J., Arganda-Carreras, I., Frise, E., Kaynig, V., Longair, M., Pietzsch, T., et al. (2012). Fiji: an open-source platform for biological-image analysis. Nat. Methods 9, 676–682. doi: 10.1038/nmeth.2019
Steinberg, G., and Gurr, S. J. (2020). Fungi, fungicide discovery and global food security. Fungal Genet. Biol. 144:103476. doi: 10.1016/j.fgb.2020.103476
Tetz, G., Cynamon, M., Hendricks, G., Vikina, D., and Tetz, V. (2017). In vitro activity of a novel compound, Mul-1867, against clinically significant fungi Candida spp. and Aspergillus spp. Int. J. Antimicrob. Agents 50, 47–54. doi: 10.1016/j.ijantimicag.2017.02.011
Tetz, V., and Tetz, G. (2022a). Novel prokaryotic system employing previously unknown nucleic acids-based receptors. Microb. Cell Factories 21. doi: 10.1186/s12934-022-01923-01-33
Tetz, V., and Tetz, G. (2022b). Novel cell receptor system of eukaryotes formed by previously unknown nucleic acid-based receptors. Receptors 1, 13–53. doi: 10.3390/receptors1010003
Tudzynski, P., and Scheffer, J. (2004). Claviceps purpurea: molecular aspects of a unique pathogenic lifestyle. Mol. Plant Pathol. 5, 377–388. doi: 10.1111/j.1364-3703.2004.00237.x
Verweij, P. E., Arendrup, M. C., Alastruey-Izquierdo, A., Gold, J. A., Lockhart, S. R., Chiller, T., et al. (2022). Dual use of antifungals in medicine and agriculture: how do we help prevent resistance developing in human pathogens? Drug Resist. Updat. 65:100885. doi: 10.1016/j.drup.2022.100885
Winiwarter, S., Ridderström, M., Ungell, A.-L., Andersson, T. B., and Zamora, I. (2007). Use of molecular descriptors for absorption, distribution, metabolism, and excretion predictions. 531–554.
Keywords: Ascomycota, Oomycota, Basidiomycota, Fusarium oxysporum, phytopathogenic fungi, plant disease, M451, antifungal
Citation: Tetz V, Kardava K, Krasnov K, Vecherkovskaya M and Tetz G (2023) Antifungal activity of a novel synthetic polymer M451 against phytopathogens. Front. Microbiol. 14:1176428. doi: 10.3389/fmicb.2023.1176428
Received: 28 February 2023; Accepted: 09 May 2023;
Published: 19 May 2023.
Edited by:
Ugo De Corato, Italian National Agency for New Technologies, Energy and Sustainable Economic Development (ENEA), ItalyReviewed by:
Ippolito Camele, University of Basilicata, ItalyCopyright © 2023 Tetz, Kardava, Krasnov, Vecherkovskaya and Tetz. This is an open-access article distributed under the terms of the Creative Commons Attribution License (CC BY). The use, distribution or reproduction in other forums is permitted, provided the original author(s) and the copyright owner(s) are credited and that the original publication in this journal is cited, in accordance with accepted academic practice. No use, distribution or reproduction is permitted which does not comply with these terms.
*Correspondence: George Tetz, Zy50ZXR6QGhtaS11cy5jb20=
Disclaimer: All claims expressed in this article are solely those of the authors and do not necessarily represent those of their affiliated organizations, or those of the publisher, the editors and the reviewers. Any product that may be evaluated in this article or claim that may be made by its manufacturer is not guaranteed or endorsed by the publisher.
Research integrity at Frontiers
Learn more about the work of our research integrity team to safeguard the quality of each article we publish.