- 1College of Horticulture and Landscape Architecture, Southwest University, Chongqing, China
- 2Chongqing Key Laboratory of Olericulture, Chongqing, China
- 3The Institute of Vegetables and Flowers, Chongqing Academy of Agricultural Sciences, Chongqing, China
Male sterility is a highly attractive agronomic trait as it effectively prevents self-fertilization and facilitates the production of high-quality hybrid seeds in plants. Timely release of mature pollen following anther dehiscence is essential for stamen development in flowering plants. Although several theories have been proposed regarding this, the specific mechanism of anther development in eggplant remains elusive. In this study, we selected an R2R3-MYB transcription factor gene, SmMYB108, that encodes a protein localized primarily in the nucleus by comparing the transcriptomics of different floral bud developmental stages of the eggplant fertile line, F142. Quantitative reverse transcription polymerase chain reaction revealed that SmMYB108 was preferentially expressed in flowers, and its expression increased significantly on the day of flowering. Overexpression of SmMYB108 in tobacco caused anther dehiscence. In addition, we found that SmMYB108 primarily functions as a transcriptional activator via C-terminal activation (amino acid 262–317). Yeast one-hybrid and dual-luciferase reporter assays revealed that genes (SmMYB21, SmARF6, and SmARF8) related to anther development targeted the SmMYB108 promoter. Overall, our results provide insights into the molecular mechanisms involved in the regulation of anther development by SmMYB108.
Highlights
• Excavating the differentially expressed gene SmMYB108 of eggplant fertile line F142 in anther different developmental stages.
• Elucidating that SmMYB108 functioned as a transcriptional activator to regulate anther development.
• Finding some genes related to anther development directly targeted SmMYB108 promoter.
• The research results are of great significance to understand the molecular mechanism of SmMYB108 regulating anther development.
Introduction
Eggplant (Solanum melongena), an important vegetable crop, cultivated in tropical, subtropical, and temperate regions, exhibits heterosis and is mainly produced by hybrids. The use of a strict pollination control system is a prerequisite to avoid self-fertilization in plants (Kempe and Gils, 2011). Male sterility is generally used to produce hybrid seeds that can effectively avoid self-fertilization and solve the difficulties of traditional seed production. Molecular mechanisms of male infertility have been reported in previous studies (Ma et al., 2015; Singh et al., 2015). RNA-sequencing (RNA-seq) technology helps in understanding the gene expression patterns and inferring the candidate genes related to male infertility. Many studies have conducted RNA-seq analyses of the male sterile floral organs of tomatoes (Jeong et al., 2014), cotton (Wei et al., 2013), peppers (Liu et al., 2013), Brassica napus (Yan et al., 2013), and watermelons (Rhee et al., 2015).
Stamen is the male reproductive organ of flowering plants, which consists of an anther, a space for pollen development, and a filament that provides the anther with structural support and nutrients. Development of anther, an important component of stamens, is mainly divided into two stages. In the first stage, the initial stamen primordium forms a complete pollen sac wall after tissue specialization and meiosis, which is followed by the formation of the outer epidermis, endothecium, middle layer, and tapetum from the outside to the inside. Tetrad structure of the microspores is located at the center of the anther chamber. In the second stage, the anther continues to expand, filament elongates, microspores develop into pollen grains from the tetrad structure, pollens sac cracks and releases pollens, and pollination and fertilization are completed, after which, the anther tissue gradually ages and degenerates (Goldberg et al., 1993; Wilson et al., 2011).
An anther dehisces in a timely manner to release mature pollen for complete pollination and fertilization. Transcription factors (TFs) containing the MYB domain in plants are known as MYB TFs. MYB domain consists of one to four amino acid (aa) sequences and forms a helix-turn-helix motif (Dubos et al., 2010). On the basis of the number of adjacent repeats, MYB proteins are divided into four categories: 1R-MYB (MYB-related), 2R-MYB (R2R3-MYB), 3R-MYB (R1R2R3-MYB), and 4R-MYB (Katiyar et al., 2012). Among them, R2R3-MYB TFs are one of the most abundant gene families in plants (Stracke et al., 2001) that are involved in plant cell development and morphogenesis, biological and non-biological stress responses, hormone signal transduction, and secondary metabolism regulation (Wang et al., 2016; Kocábek et al., 2018; Guan et al., 2019).
Secondary thickening of the inner wall of the anther chamber is essential for anther dehiscence (Zhao and Dong, 2015). AtMYB26 is vital for secondary thickening, directly inducing the expression of AtNST1 and AtNST2 in the anthers. Both myb26 and nst1 nst2 mutants failed to exhibit anther dehiscence (Yang et al., 2017). Previous studies have reported that AtMYB103, AtMYB83, and AtMYB46 are direct targets of AtNST1 and AtNST2, regulating secondary wall biosynthesis to affect anther dehiscence and pollen development. Another study reported that AtMYB83 acts redundantly with AtMYB46 as a key molecular in the regulating of secondary wall biosynthesis (Zhong et al., 2008; McCarthy et al., 2009; Zhong et al., 2007). Li et al. (2019) revealed that RUS4 affects the synthesis of the secondary wall of the inner wall of the drug chamber by indirectly activating the expression of the TFs, AtMYB103 and AtMYB85 (Li et al., 2019). Because of the defects in filament elongation, anther dehiscence, and pollen viability, myb21 myb24 double-mutant plants are male sterile. R2R3-MYB TFs, MYB21 and MYB24, are essential for filament elongation, anther dehiscence, and pollen maturation, jasmonic acid (JA) regulation of male fertility in Arabidopsis thaliana (Song et al., 2011). Overexpression of GhMYB24 in Arabidopsis severely decreases the fertility by inhibiting filament elongation and anther dehiscence and decreasing the pollen viability. atmyb21 atmyb24 double-mutant plants have been reported to be severely male sterile, and the expression of GhMYB24 in the atmyb21 atmyb24 double-mutant has been reported to partially rescued the sterile phenotype (Li et al., 2013). Several studies have investigated the role of the TF, MYB108, in anther development. AtMYB108 and AtMYB24 have been reported to coordinate the JA pathway during anther dehiscence (Mandaokar and Browse, 2009). Sun et al. reported that a JA-induced R2R3-MYB TF, CaMYB108, is involved in the regulation of capsaicinoids, cap biosynthesis, and stamen development. Silencing of CaMYB108 has been reported to delay anther dehiscence and decrease the pollen viability (Sun et al., 2019). arf17 mutant anther is indehiscent, and the auxin response factor, ARF17, can directly bind to the AtMYB108 promoter and induce its expression in arf17 mutant to rescue its anther dehiscence phenotype (Xu et al., 2019).
Molecular mechanism by which TF MYB108 regulates the development of anthers remains poorly understood. In this study, we identified differentially expressed gene (DEG), SmMYB108, in eggplant, whose expression was highest on the day of flowering (FD). We verified that this TF was involved in stamen development. In addition, we found that proteins related to anther development (SmMYB21, SmARF6, and SmARF8) directly bound to the promoter of SmMYB108. Our findings indicate that SmMYB108 plays a central role in anther development.
Results
Identification of DEGs
We determined the mRNA (messenger Ribonucleic Acid) expression levels in the floral buds of the eggplant fertile line, F142, at different developmental stages. The FPKM (fragments per kilobase of exon per million mapped fragments) method was used to identify the DEGs. In total, 5,549 DEGs were identified via pairwise comparisons. Comparison of 8 days before flowering (DBF) and 5 DBF revealed the lowest number, 269 DEGs, of which 203 were upregulated and 66 were downregulated. The maximum number of DEGs was between 8 DBF and the day of flowering (FD), including 2,756 upregulated and 1,750 downregulated genes (Figure 1A). Notably, 2,552 common DEGs (1,739 upregulated and 816 downregulated) were shared between the 8 DBF and FD and between 5 DBF and FD groups (Supplementary Figures S1A–C).
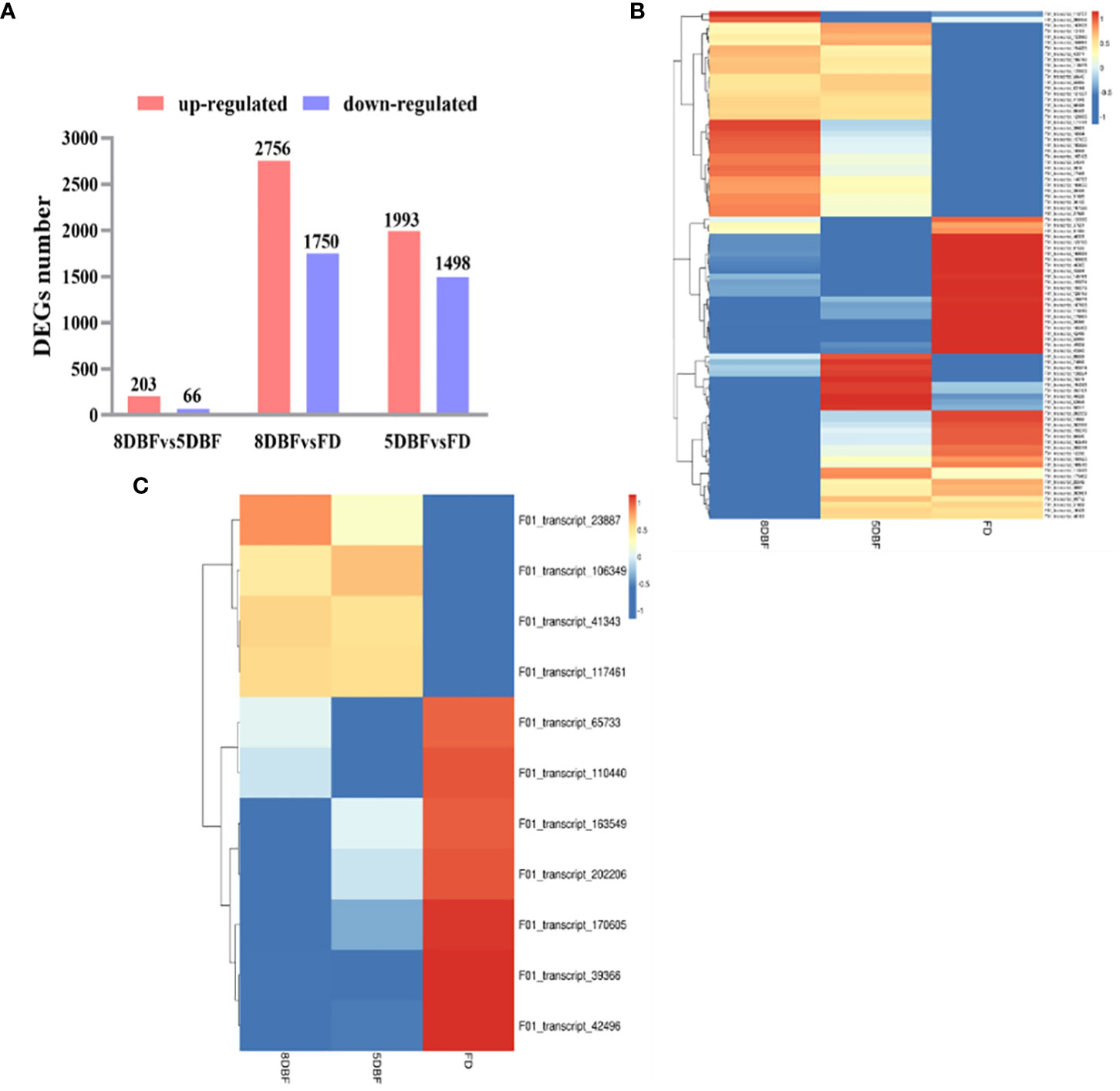
Figure 1 Analysis of DEGs between the eggplant fertile line F142 in different stages of flower buds. (A) The total number of upregulated and downregulated DEGs. (B) Heat map of differentially expressed transcription factors. (C) Heat map analysis of 11 common differentially expressed genes.
TFs play an important role in anther development. Eighty-nine TFs were identified among all DEGs, which could be classified into 29 families. The important families were bHLH (basic helix-loop-helix) (19.10%), MYB(v-myb avian myeloblastosis viral oncogene homolog)-related (13.48%), AP2 (APETALA2) (11.24%), NAC (NAM、ATAF1/2、CUC1/2) (1.12%), WRKY (7.87%), TCP ((TEOSINTE BRANCHED1/CYCLOIDEA/PROLIFERATING CELL FACTORS) (7.87%), and the MADS (2.25%) families (Supplementary Figure S2A). On the basis of the similarity in gene expression, hierarchical clustering was performed using the 89 differentially expressed TFs in samples (Figure 1B; Supplementary Table 2). Hierarchical clustering of the gene expression profiles in the three stages revealed different expression patterns in these samples, providing an overall understanding of the changes in gene expression. Forty-two MYB TFs were identified among 89 TFs, of which 31 were upregulated and 11 were downregulated. Moreover, 11 common MYB TFs between 8 DBF and FD and between 5 DBF and FD were identified, and the expression pattern was visualized using a heat map (Supplementary Figure S2B; Supplementary Table 3). Interestingly, we found that the expression levels of gene F01_transcript_42496 (referred as SmMYB108 in this study) were significantly higher in FD than that in 8 and 5 DBF (Figure 1C). These results suggest the involvement of an extremely intricate transcriptional network in anther development.
SmMYB108 is highly expressed during the flowering period
To verify the RNA-seq data, quantitative reverse transcription polymerase chain reaction (qRT-PCR) was conducted on nine randomly selected genes, and the results confirmed the accuracy of transcriptome analysis (Supplementary Figure S3). We found that SmMYB108 was predominantly expressed in the flowers, followed by roots, but its transcript levels were low in the stems, leaves, anthers, petals, and sepals through qRT-PCR (Figure 2A). Moreover, we quantitatively analyzed the expression levels of SmMYB108 at different developmental stages. Within the floral buds, SmMYB108 transcript level was stable from 8 to 3 DBF, increased slightly 2 DBF, and reached the maximum level at flowering (Figure 2B). Taken together, our results indicate SmMYB108 as a candidate regulator of anther development. Moreover, SmMYB108 may have roles other than anther development regulation in plants.
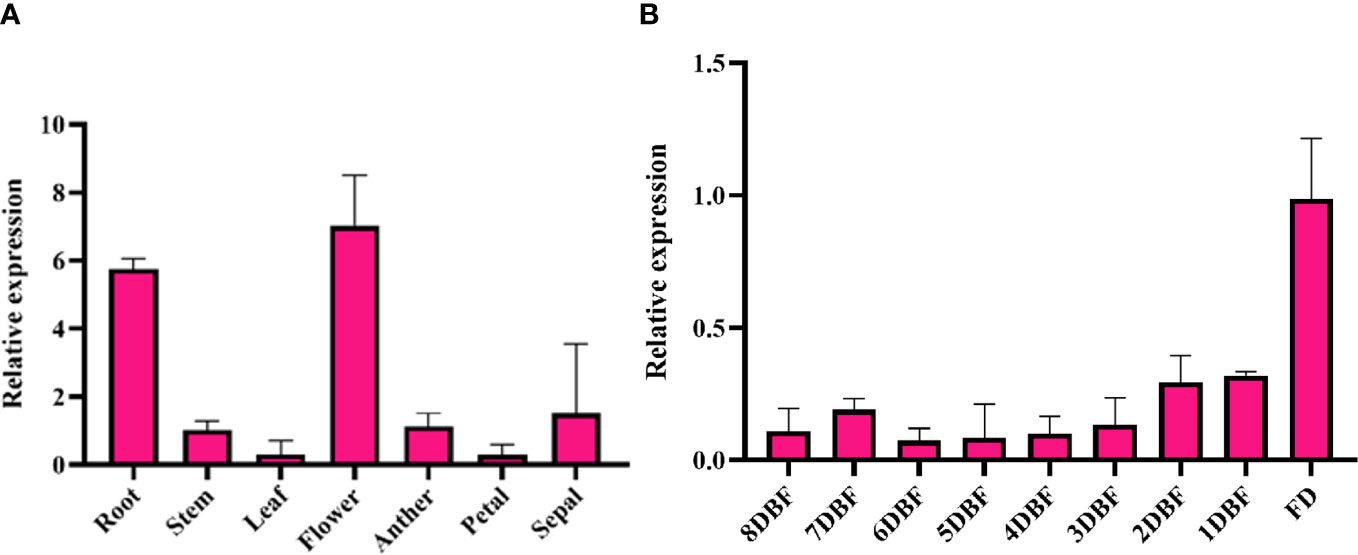
Figure 2 Expression analysis of SmMYB108. (A) Expression of SmMYB108 in different tissues. (B) Expression of SmMYB108 in different developmental stages of flower buds.
To visualize the detailed expression patterns of SmMYB108, its promoter was cloned approximately 2-kb 5′ upstream of the sequence included in gSmMYB108 in this study. We generated prSmMYB108:GUS Arabidopsis transgenic lines expressing GUS (Β-glucuronidase) fused with the promoter sequence of SmMYB108 to detect SmMYB108 expression patterns. Consistent with the gene expression pattern, blue staining was high in the roots, flowers, and leaves of mature plants (Figures 3B–I) and mainly observed in the mature anthers in flowers (Figures 3E, J). GUS staining was observed in all organs in approximately 2-week-old seedlings (Figures 3A, F). These findings highlight the potential role of SmMYB108 in anther development.
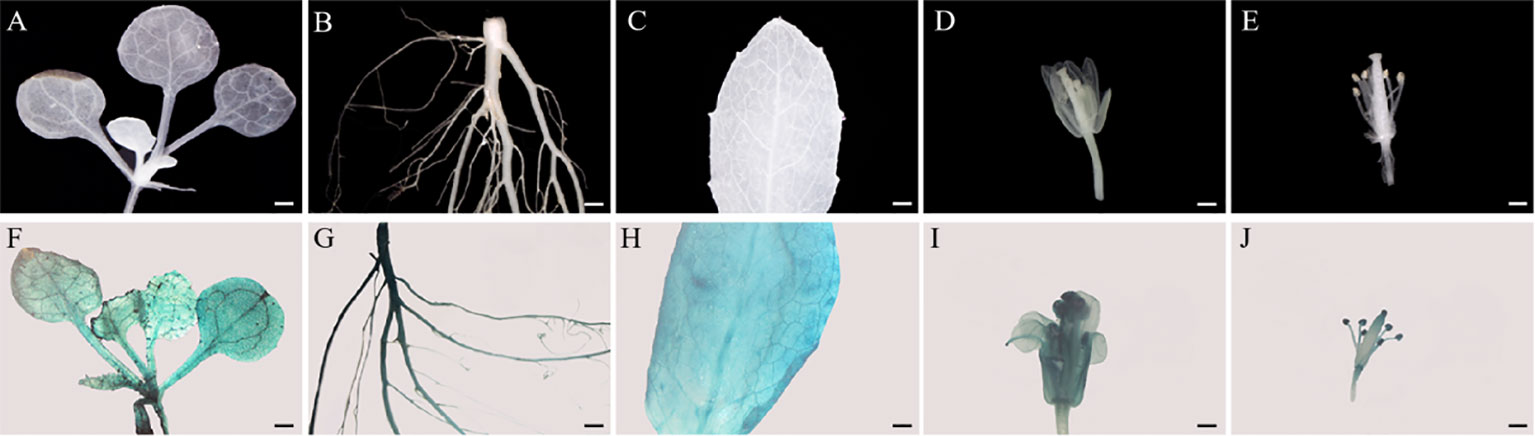
Figure 3 Tissue-specific expression analysis of SmMYB108 in prSmMYB108:GUS transgenic plants. (A–E) Seedling, root, leaf, mature flower, and anther (from left to right) were from wild type. (F–J) Gus-stained seedling, root, leaf, mature flower, and anther (from left to right) were from prSmMYB108:GUS transgenic plants. Bar, 500 µm.
SmMYB108 encodes a nucleus-localized R2R3 MYB TF
Full-length coding sequence (CDS) of SmMYB108 was isolated from the eggplant fertile line F142, and its open reading frame was 954 bp, encoding a protein of 317 amino acids, located on chromosome 5 (Figure 4A). Aa sequence analysis revealed that SmMYB108 contains two motif DNA-binding domains (BDs) (R2-R3 MYB type) next to the N-terminus (Figure 4B). We retrieved SmMYB108 homologs from Solanum lycopersicum, S. tuberosum, Nicotiana attenuata, Capsicum annuum, and A. thaliana. A phylogenetic tree was constructed to identify the orthologs of these genes. We found that the nearest MYB TFs from StMYB108 (S. tuberosum), CaMYB108 (C. annuum), SlMYB78 (S. lycopersicum), and NaMYB108 (N. attenuata), shared sequence identity > 90% (Figure 4C). AtMYB108, which showed only 60% aa sequence identity, was the MYB TF most closely related to A. thaliana. Interestingly, both CaMYB108 and AtMYB108 were induced by JA to participate in anther development (Mandaokar and Browse, 2009; Sun et al., 2019), suggesting that SmMYB108 may also play a role in stamen development.
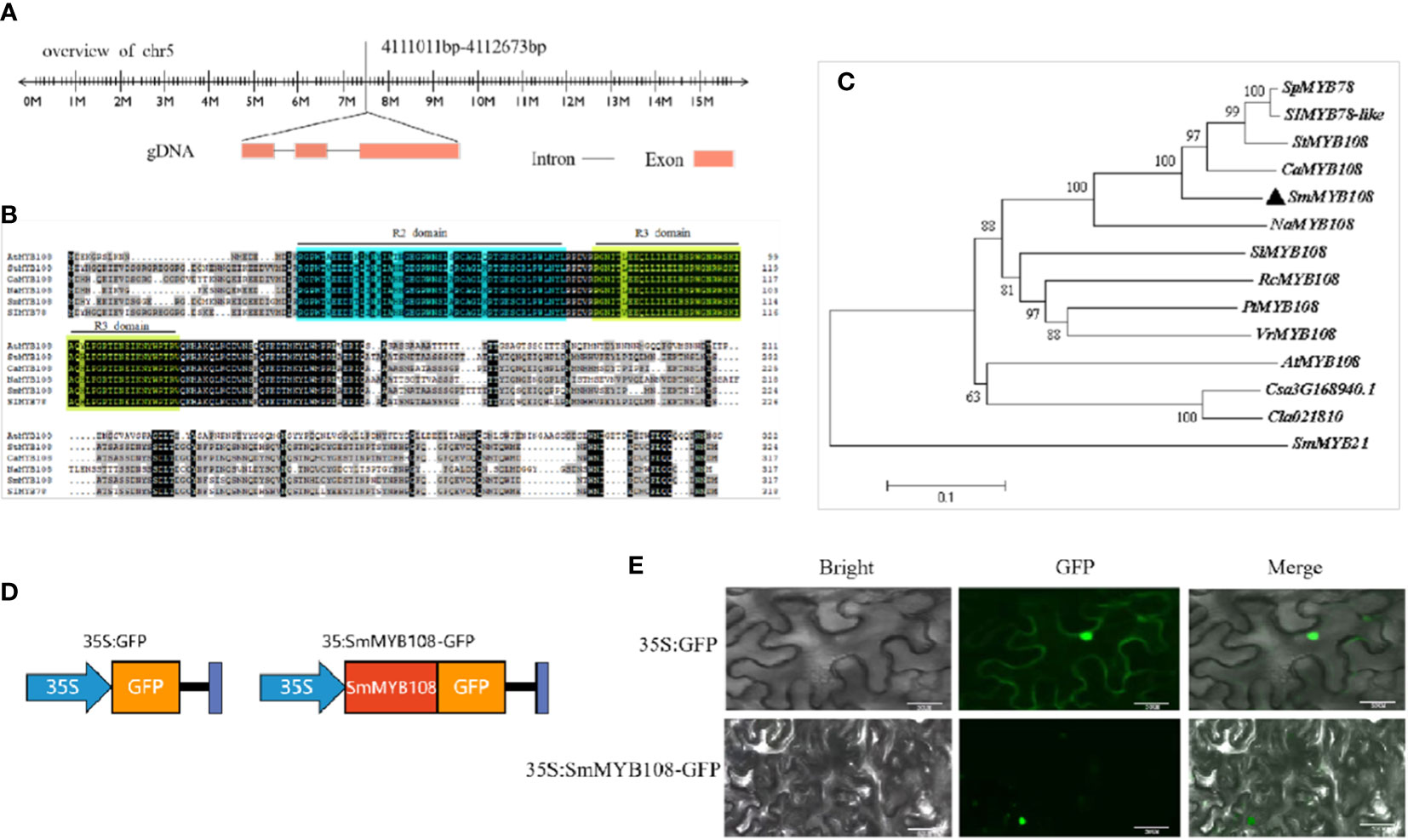
Figure 4 Phylogenetic and subcellular analyses of SmMYB108. (A) The genomic sequence of SmMYB108 that is mapped to eggplant genome. (B) Sequence analysis of SmMYB108. To compare the MYB sequences among different plant species, SmMYB108 was used as a bait gene and searched in National Center for Biotechnology Information (NCBI) database for highly similar sequences MYB TFs in other species. (C) A phylogenetic tree showing aa sequence similarities among SmMYB108 and closely related MYB TFs in other species. (D, E) Subcellular localization of 35S:SmMYB108-GFP fusion protein in Tobacco epidermal cells. 35S::GFP served as a control. Bar, 50 µm.
TFs regulate transcription depending on their localization in the nucleus. To determine the subcellular localization of SmMYB108, the CDS of SmMYB108 was fused in frame with the green fluorescence protein (GFP) to generate a 35Spro::GFP-SmMYB108 fusion protein (Figure 4D). 35Spro::GFP-SmMYB108 was transiently expressed in the nucleus of N. benthamiana leaf epidermal cells. GFP-SmMYB108 was observed exclusively in the nucleus, whereas free GFP (35S::GFP) was observed in both the cytoplasm and nucleus of epidermal cells (Figure 4E), suggesting that SmMYB108 is localized in the nucleus.
Overexpression of SmMYB108 promotes stamen development
To further study the function of SmMYB108, we constructed an overexpression vector used for the Agrobacterium-mediated transformation of tobacco. We successfully developed 14 transgenic tobacco plants overexpressing SmMYB108. In T0 plants, SmMYB108-OE-2, SmMYB108-OE-3, and SmMYB108-OE-5 were selected for further analysis (Figure 5A). Floral organ morphology and pollen vitality were not significantly different between the wild-type and SmMYB108-OE lines as they exhibited normal anther dehiscence and released viable pollen (Figure 5B). However, the anthers of transgenic tobacco were completely dehiscent and covered by pollen, whereas those of wild-type tobacco were partially dehiscent and covered by a small amount of pollen. These results revealed that the degree of anther dehiscence in transgenic tobacco was greater than that in wild-type tabacco. Moreover, flower buds immediately at the top of tobacco developed earlier than those in transgenic tobacco and wild-type plants. Taken together, these results suggest that SmMYB108 overexpression promotes the early dehiscence of anthers (Figure 5C).
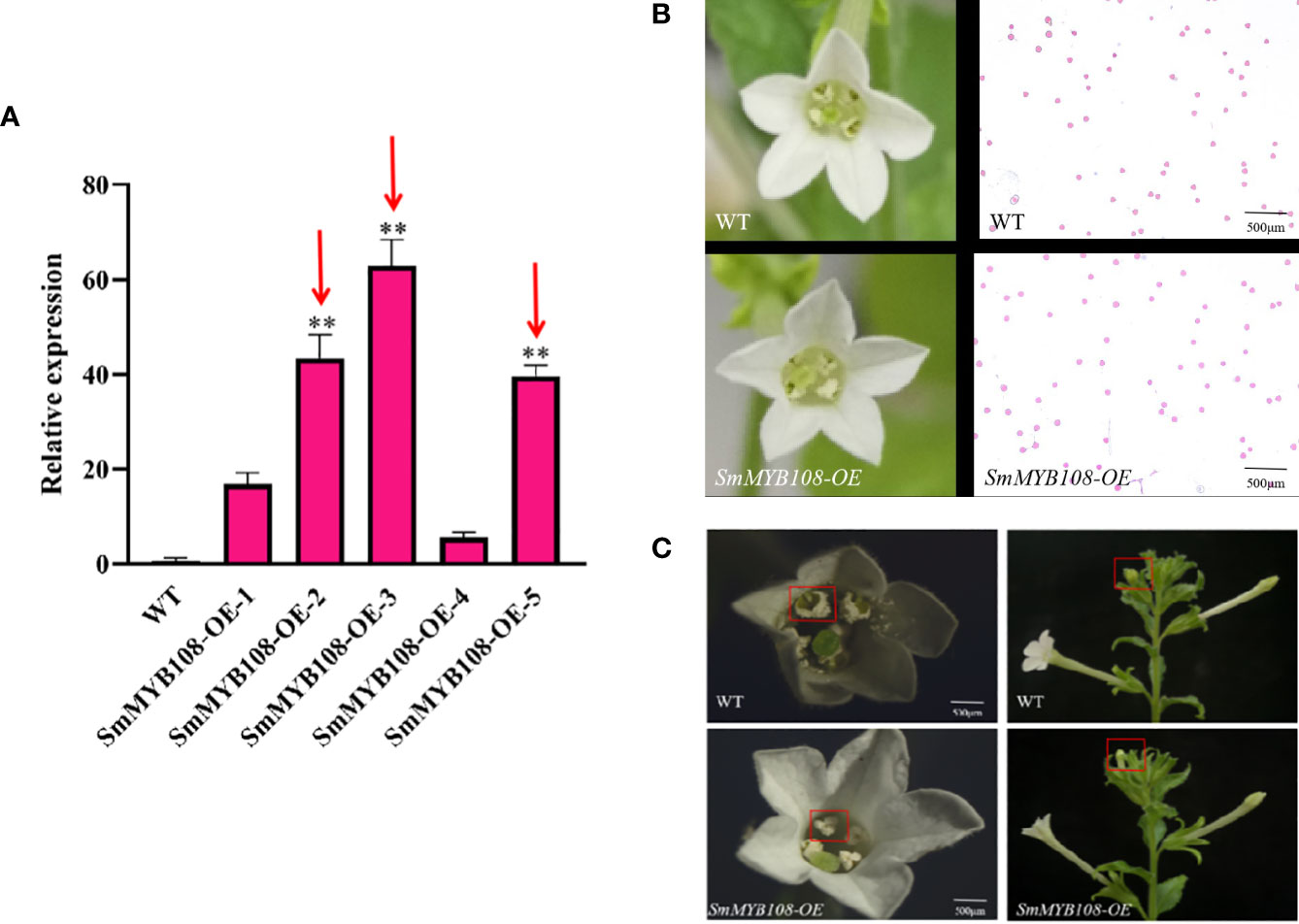
Figure 5 Morphological characterization of SmMYB108-OE plants floral organ. (A) RT-qPCR analysis of SmMYB108 transcripts present in wild-type (WT) and SmMYB108-OE lines. Error bars, 6 SEM (n = 3). Three lines were selected for further research (red arrows). (B) Morphological features of flowers in the same period in wild type and SmMYB108-OE lines. (C) The degree of antherin the same period in wild type and SmMYB108-OE lines.
SmMYB108 functions as a transcriptional activator
SmMYB108 contains an N-terminal R2R3 repeat domain, responsible for DNA binding, and a C-terminal domain. Transcriptional activation domain of the R2-R3 type MYB TF, which encodes the aa, was located at the C-terminus, as previously reported (Song et al., 2011). We performed a transactivation activity assay in yeast to test the transcriptional activation activity of SmMYB108. On the basis of the presence of conserved domains, full-length and a series of truncations were fused in-frame with the GAL4 DNA-binding domain (BD) in the pGBKT7 (BD) vector, and the constructs were transformed into the yeast two-hybrid (Y2H) gold yeast strain. Yeast carrying BDSmMYB108 grew and turned blue on SD/−Trp selective medium along with X-α-gal, indicating that TF SmMYB108 acts as a transcriptional activator (Figure 6). To determine the specific domain influencing the activation activity of SmMYB108, the constructed truncated SmMYB108 was transformed into the Y2H gold yeast strain (Figure 6). The results showed reduced transcriptional activation of SmMYB108 (275–317 aa) and SmMYB108 (262–274 aa), indicating destruction of the self-activating domain. Therefore, the C-terminus (262–317 aa) was essential for the activation activity of SmMYB108 (Figure 6).
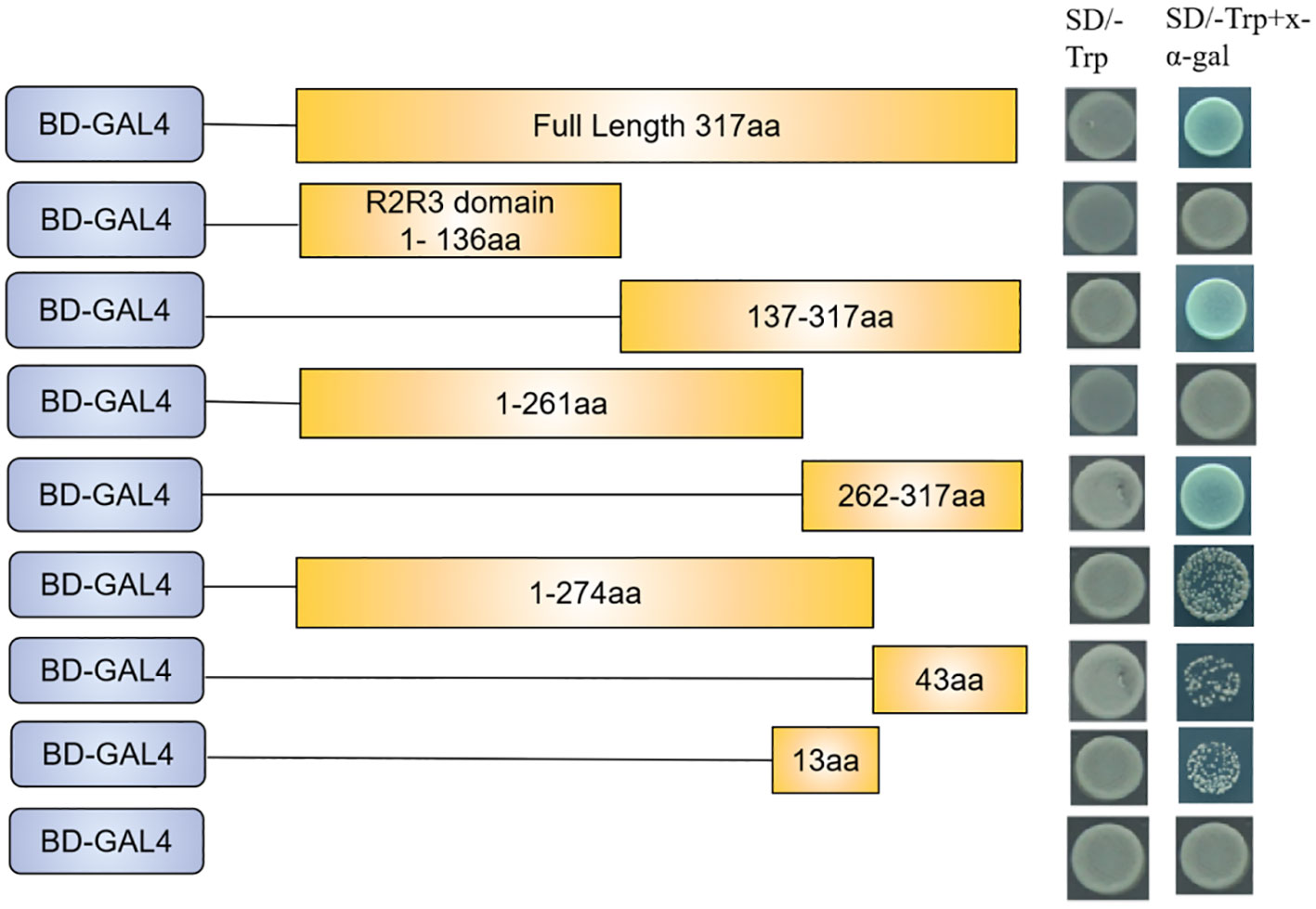
Figure 6 SmMYB108 is a transcription activator. Activation analysis of SmMYB108 in yeast. Full-length and truncated SmMYB108 was used for activation analysis. The number shown on the right indicates the protein region used for activation analysis. Auxotroph plates of SD/–Trp(left) and SD/–Trp–x-α-gal(right) showing transcriptional activation of protein.
Anther development-related genes directly target SmMYB108
AtMYB21, AtMYB26, AtARF6, and AtARF8 participated in anther development in A. thaliana, as previously reported (Song et al., 2011; Yang et al., 2017; Nagpal et al., 2005). To determine the direct target genes of SmMYB108, we selected SmMYB21, SmMYB26, SmARF6, and SmARF8 as candidate target genes for screening via Y1H assay. SmMYB108 promoters were ligated into the pAbAi vector to construct bait vectors (pAbAi-ProSmMYB108). SmMYB21, SmMYB26, SmARF6, and SmARF8 were ligated into the pGADT7 vector to construct a prey vector. Subsequently, bait and prey vectors were transformed into the Y1H gold yeast strain. As shown in Figure 7A, the negative control (pGADT7 + ProSmMYB108) and transformant (pGADT7-SmMYB26 + ProSmMYB108) did not grow well on the medium containing aureobasidin A (AbA; 600 ng ml−1). However, the positive control (p53 + Prop53) and transformants (pGADT7-SmMYB21 + ProSmMYB108, pGADT7-SmARF6 + ProSmMYB108, and pGADT7-SmARF8 + ProSmMYB108) grew well on the medium containing AbA. These findings indicate that SmMYB21, SmARF6, and SmARF8 are associated with the SmMYB108 promoters in vitro.
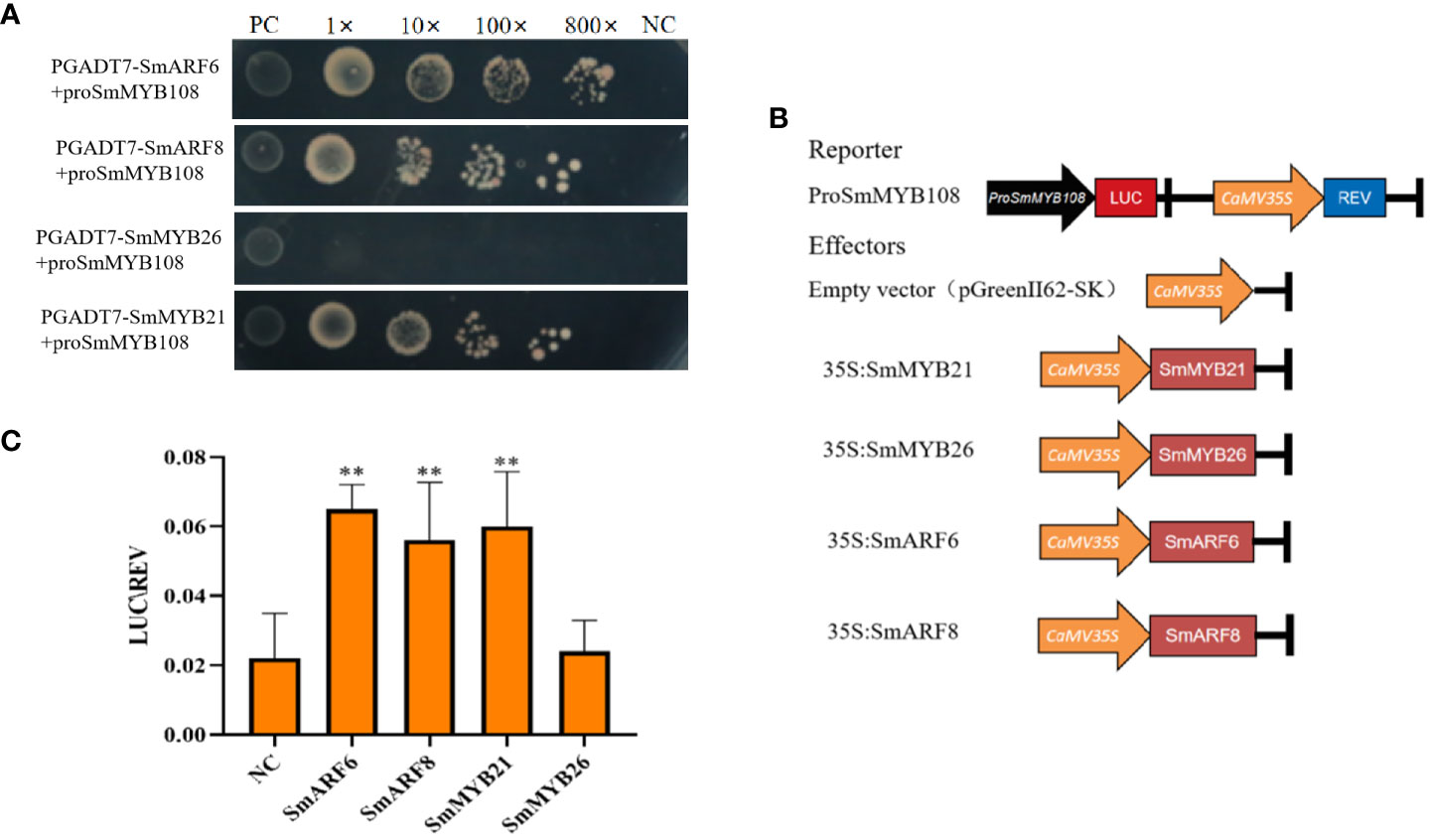
Figure 7 Anther development-related genes targets the promoters of SmMYB108. (A) Y1H analysis of SmMYB21, SmARF6, and SmARF8 associated with the promoters of SmMYB108. (B) ProSmMYB108, empty vector (pGreenII62-SK), 35S:SmMYB21, 35S:SmMYB26, 35S:SmARF6, and 35S:SmARF8 were infected into N. benthamiana cells. (C) Dual-luciferase reporter assay of SmMYB21, SmARF6, and SmARF8 activated the transcription of SmMYB108 promoters. Values are the mean ± SD of six replicates. One-way ANOVA was used to identify significant differences (**p < 0.01).
Next, we used a dual-luciferase (LUC) reporter assay to confirm whether SmMYB108 promoter transcription could be activated by SmMYB21, SmMYB26, SmARF6, and SmARF8 in vivo. SmMYB108 promoter fragment was fused to a firefly LUC reporter sequence and co-transformed into tobacco leaves either with 35S:SmMYB21, 35S:SmMYB26, 35S:SmARF6, and 35S:SmARF8 effector constructs or with an empty vector before determining the relative LUC activity using the LUC/Renilla (REN) ratio (Figure 7B). We found that SmMYB21, SmARF6, and SmARF8 activated the transcription of SmMYB108 promoter in a gene-dependent manner. SmMYB108 promoter transcription was strongly activated by SmMYB21, SmARF6, and SmARF8 in N. benthamiana leaves. However, no difference was observed between SmMYB108 promoter-driven and empty vector LUC activity (Figure 7C). Taken together, these results indicate that SmMYB21, SmARF6, and SmARF8 are the transcriptional activators of SmMYB108.
Discussion
RNA-seq is used to determine the gene expression patterns and analyze DEGs responsible for genetic variation. Transcriptome analysis is often used to identify the DEGs involved in male sterility in various species (Jeong et al., 2014; Wang et al., 2021; Yuan et al., 2021). In this study, we conducted RNA-seq to determine the gene expression changes at different developmental stages (8 DBF, 5 DBF, and FD) in eggplant floral buds. Overall, 5,549 genes were identified via pairwise comparisons (Figure 1A). The anther is not only an important part of the stamens but also the place of pollen development. Normal dehiscence of anthers and timely release of pollen are essential for the completion of pollination and fertilization in plants (Goldberg et al., 1993; Wilson et al., 2011). Various TFs, including MYB TFs, play an important role in anther development (Veenstra and Wolffe, 2001). We identified 11 common MYB TFs in 8 DBF vs. FD and 5 DBF vs. FD groups. The expression of SmMYB108 (F01_transcript_42496) was significantly increase on the FD (Figure 1C). Nine genes among all DEGs were randomly selected, and their expression levels were of consistent in both platforms (Supplementary Figure S3). qRT-PCR analysis revealed that the expression of SmMYB108 was highest on the FD (Figure 2). pSmMYB108:GUS Arabidopsis transgenic lines also exhibited consistent gene expression patterns (Figure 3).
N-terminal of MYB TFs has a conserved MYB domain, which is divided into four types: 4R-MYB, 2R-MYB (R2R3-MYB), 3R-MYB (R1R2R3-MYB), and 1R-MYB (MYB-related) (Katiyar et al., 2012). We identified a novel MYB TF, SmMYB108, in the nucleus that had a length of 317 aa. It encoded a R2R3-MYB protein in the N-terminus and was involved in anther development. (Figure 4E). Sequence analysis of SmMYB108 and its orthologs revealed that CaMYB108, StMYB108, SlMYB78, and NaMYB108 shared a fairly high sequence identity with SmMYB108 (>90% aa sequence identity), whereas AtMYB108, which is most closely related to SmMYB108, shared only 60% sequence identify. JA-inducible AtMYB108 has been reported to mediate stamen and pollen maturation as well as response to pathogens. Silencing the JA-inducible CaMYB108 resulted in delayed anther dehiscence, decreased pollen viability, and promoted the synthesis of capsaicin. In our study, phenotypic analysis of transgenic tobacco revealed that the overexpression of SmMYB108 promoted anther dehiscence (Figure 5C). Our results indicated an intriguingly convergent evolution of MYB108 gene function among different plant species (Mandaokar and Browse, 2009; Sun et al., 2019); however, MYB108 gene function is known to differ among different plant species. Zhang et al. (2019) reported that RhMYB108 participates in the interaction between ethylene and JA signals and is strongly expressed in rose petals. Silencing RhMYB108 promoted the expression of senescence associated genes, delaying the petal senescence (Zhang et al., 2019). Both PsnMYB108 and MdMYB108L mediate plant stress resistance and can improve plant salt tolerance under abiotic stress (Wang et al., 2019; Zhao et al., 2020). However, the specific roles of SmMYB108 in different species require further investigation.
In addition to the conserved R2R3 DNA-BD, the C-terminus performs several vital functions (Dubos et al., 2010). Transcriptional activation domain of CaMYB31 is at the C-terminus (213–234 aa) (Zhu et al., 2019). CaMYB48 has a motif that is rich in amino acids at the C-terminus (168–191 aa) that functions as a transcriptional activation domain (Sun et al., 2020). In this study, transcriptional activation analysis of yeast showed that C-terminus (262–317 aa) was crucial for the activation activity of SmMYB108 (Figure 6). Multiple transcriptional binding sites were identified in the myb108 promoter sequence. Y1H and dual-LUC reporter assays revealed that SmMYB21, SmARF6, and SmARF8, but not SmMYB26, could activate the SmMYB108 promoter (Figure 7). AtARF6 and AtARF8 promote anther dehiscence in floral buds (Nagpal et al., 2005). JA and auxin are important hormones in plant development and stress response, which are also involved in the regulation of anther dehiscence. AtARF6 and AtARF8 regulate anther dehiscence by activating DAD1, a key gene in JA biosynthesis, to mediate JA synthesis (Tabata et al., 2010). Moreover, AtMYB21 mediates the JA pathway during anther dehiscence (Song et al., 2011). Whether SmMYB108 also regulates anther development via the hormone pathway needs to be investigated further in future studies.
Conclusion
In summary, we found that the promoter of SmMYB108 directly combines with the targets genes related to anther development—SmMYB21, SmARF6, and SmARF8, but not SmMYB26. We also found that SmMYB108 functions as a transcriptional activator to regulate anther development. Our findings provide mechanistic insights into the evolution of male sterility in plants and can aid in future investigation of the transcriptional regulatory networks involved in anther development in eggplant.
Materials and methods
Plant materials
F142, a fertile eggplant (Solanum melongena) line, was provided by the Institute of Vegetables and Flowers, Chongqing Academy of Agricultural Sciences (Chongqing, China). All plants were cultivated in a controlled chamber (16/8-h light/night photoperiod and 28/22°C light/night temperature with 60%~65% relative humidity). Floral buds were collected respectively at 8 DBF, 5 DBF, and the FD for transcriptome sequencing. Various tissues (roots, stems, leaves, anthers, sepal, petals, and flower buds at different periods) were collected for qRT-PCR. Every sample was set three biological replicates. N. benthamiana is kept and supplied by our laboratory. Wild-type Arabidopsis thaliana is provided by our laboratory.
RNA-seq analysis
The transcriptome data of the fertile line F142 were retrieved from the previous studies (Wang et al., 2021; Yuan et al., 2021). These data included transcription data of genes from different developmental stages of floral buds. Raw reads were first filtered to obtain clean reads, which were aligned to the eggplant HQ-1315 genome using Hisat2 (version 2.1.0). Only reads with a perfect match or one mismatch were further analyzed and annotated based on the reference genome. Each gene expression level was calculated as FPKM. Differential expression analysis (three biological replicates at different developmental stages) was performed using the DESeq. Genes with an adjusted P-value <0.01, as found by DESeq, were considered as differentially expressed (Anders and Huber, 2010).
Quantitative reverse transcription polymerase chain reaction analysis
For the extraction of total RNA from the samples, the RNAprep Pure Plant Kit polysaccharide and polyphenol total RNA extraction kit (Tianmo, China) were used. cDNA was synthesized from RNA (1 µg per sample) using the Prime Script RT Kit (Takara, China) according to the manufacturer’s instructions. Using real-time qRT-PCR validated the RNA-seq data and performed the expression of gene in different tissues. qRT-PCR primers were designed using Primer Premier 5.0 (Supplementary Table 1). The reactions of qRT-PCR were performed with the SYBR Green qPCR Mix (Takara, Japan) on the Bio-Rad CFX96 Touch Real Time PCR detection System (Bio-Rad Laboratories). In addition, reactions were performed three biological replicates and three technical replicates. The GAPDH (Glyceraldehyde -3-phosphate dehydrogenase) gene was used as the reference to quantify transcript levels. The 2−△△CT approach was applied to calculate the relative expression levels of target genes (Livak and Schmittgen, 2001; Vandesompele et al., 2002).
Histochemical analysis of GUS expression
A section (2 kb) of the SmMYB108 promoter was cloned into the pGreen vector harboring the GUS reporter to construct pSmMYB108:GUS. Tissues for GUS staining were first infiltrated with staining solution [50 mM sodium phosphate buffer (pH 7.0), 0.5 mM potassium ferrocyanide, 0.5 mM potassium ferricyanide, and X-Gluc (0.5 mg ml−1)] in a vacuum chamber and, subsequently, incubated with the same solution at 37°C for 4 h.
Sequence alignment and phylogenetic tree construction
To understand the location of the TFs on chromosome, the protein sequence of SmMYB108 was obtained from eggplant reference genome HQ-1315. Other MYB TF sequences that showed the higher sequence identity with SmMYB108 was retrieved from the public database National Center for Biotechnology Information (NCBI; https://www.ncbi.nlm.nih.gov/) and Sol Genomics Network (http://solgenomics.net/) and used for phylogenetic tree construction. The MYB TF sequences were aligned by CLUSTALW with the default parameters, and the phylogenetic tree was constructed by MEGA 7.0 using the neighbor-joining method with 1,000 bootstrap replications.
Subcellular localization
The full-length SmMYB108 cDNA was cloned from fertile line F142, which was inserted into the pCAMBIA1300 vector without the stop codon and fused with GFP-generating SmMYB108-GFP fusion proteins under the control of the CaMV35S promoter. The SmMYB108-GFP fusion proteins were introduced into Agrobacterium tumefaciens strain GV3101, which was infiltrated into tobacco leaves epidermal cells along with empty plasmids. After 48 h of inoculation, the GFP signal was visualized by confocal fluorescence microscopy (Carl Zeiss, Germany). The primers used in this study were listed in Supplementary Table 1.
Transcriptional activation analysis
The ability of SmMYB108 to activate transcription was verified by a yeast transcriptional activation assay according to the manufacturer’s instructions of the Matchmaker Gold yeast two-hybrid system (Takara, China). Full-length or deleted SmMYB108 was fused in frame with the GAL4 DNA-BD in pGBKT7 vector. The pGBKT7 empty vector that only expressed GAL4 BD was used as a negative control. Recombinant plasmids and empty vector were transformed into yeast strain Y2H Gold. Yeast cells were incubated on SD/-Trp medium at 30°C for 3 days. The positive clones were diluted in 0.9% NaCl solution, and 10 µl of each dilution was inoculated on SD/-Trp +X-α-gal medium at 30°C for 3–5 days; the clones were stained with x-α-Gal (Takara, China). The primers used in this study were listed in Supplementary Table 1.
Y1H assay
The ORF (Open reading frame) of four genes (SmMYB21, SmMYB26, SmARF6, and SmARF8) were inserted into SmaI and XhoI of the pGADT7 vector to generate prey. The promoter fragments of SmMYB108 were ligated into SacI and SmaI of the pAbAi vector as bait. The Y1H experiment was carried out using the Matchmaker Gold yeast one-hybrid system (Takara, China) according to the manufacturer’s protocol. The primers used in this study were listed in Supplementary Table 1.
Dual-LUC assay
The SmMYB108 promoter (2,000 bp) was amplified by PCR from eggplant fertile line F142 and inserted into the SacI and BamHI of pGreenII-0800-LUC vector to serve as the reporters. The full-length CDS of four genes (SmMYB21, SmMYB26, SmARF6, and SmARF8) was cloned into the SacI and BamHI of pGreenII62-SK vector under the CaMV35S promoter to serve as an effector. The recombinant plasmids were transformed to the Agrobacterium tumefaciens strain GV3101 (pSoup-p19). The GV3101 harboring the SmMYB108 reporter and the corresponding effectors were injected into 30-day-old tobacco (Nicotiana tabacum) leaves. After incubation for 48–72 h, the firefly LUC and REN LUC activities were measured with the Dual Luciferase Reporter Gene Assay Kit (Yisheng, Shanghai) according to the manufacturer’s introduction. The Varioskan™LUX (Thermo Fisher Scientific, USA) was used to carry out the assay. Activity was expressed as the ratio of firefly LUC activity to REN LUC activity. The primers for all constructs in this study were listed in Supplementary Table 1.
Construction of a pCAMBIA-2301G-SmMYB108 vector and tobacco transformation
SmMYB108 was generated through PCR amplification by using specific primers containing BamHI and SacI sites. The fragments were inserted into the pCAMBIA-2301G vector (Lü et al., 2017) to form the construct pCAMBIA-2301G-SmMYB108. Agrobacterium-mediated transformation of N. benthamiana was then performed, as previously described (Zhao et al., 2012). The sterile tobacco leaves of 15–20 days were cut into 1-cm2 size into resuspension for 10 min at 25°C dark culture for 2 days and transferred to screening medium for differentiation to induce indefinite shoots; differentiated shoots were transferred to culture medium for rooting screening.
Data availability statement
The datasets presented in this study can be found in online repositories. The names of the repository/repositories and accession number(s) can be found below: NCBI accession PRJNA941250.
Author contributions
ZW, RH, and JW designed the research; RH and JW performed the molecular biology experiments; HY, DW, QT, YY and ST carried out the bioinformatics analysis; RH and JW analyzed the data and wrote the paper manuscript. All authors contributed to the article and approved the submitted version.
Funding
This work was supported by grants from the earmarked fund for CARS (Grant No. CARS-23-A08), Sichuan Chongqing cooperation project (cstc2021jscx-cylhX0002).
Conflict of interest
The authors declare that the research was conducted in the absence of any commercial or financial relationships that could be construed as a potential conflict of interest.
Publisher’s note
All claims expressed in this article are solely those of the authors and do not necessarily represent those of their affiliated organizations, or those of the publisher, the editors and the reviewers. Any product that may be evaluated in this article, or claim that may be made by its manufacturer, is not guaranteed or endorsed by the publisher.
Supplementary material
The Supplementary Material for this article can be found online at: https://www.frontiersin.org/articles/10.3389/fpls.2023.1164467/full#supplementary-material
References
Anders, S., Huber, W. (2010). Differential expression analysis for sequence count data. Genome Biol. 11, R106. doi: 10.1186/gb-2010-11-10-r106
Dubos, C., Stracke, R., Grotewold, E., Weisshaar, B., Martin, C., Lepiniec, L. (2010). MYB transcription factors in Arabidopsis. Trends Plant Sci. 15, 573–581. doi: 10.1016/j.tplants.2010.06.005
Goldberg, R. B., Beals, T. P., Sanders, P. M. (1993). Anther development: basic principles and practical applications. Plant Cell 5, 1217–1229. doi: 10.1105/tpc.5.10.1217
Guan, S. Y., Jiao, P., Jiang, Z. Z., Qi, Z., Xia, H. F., Qu, J., et al. (2019). Research progress of MYB transcription factors in plant abiotic stress. J. Jilin Agric. Univ 41, 253–260. doi: 10.13327/j.jjlau.2019.5023
Jeong, H. J., Kang, J. H., Zhao, M., Kwon, J. K., Choi, H. S., Bae, J. H., et al. (2014). Tomato Male sterile 1035 is essential for pollen development and meiosis in anthers. J. Exp. Bot. 65 (22), 6693–6709. doi: 10.1093/jxb/eru389
Katiyar, A., Smita, S., Lenka, S. K., Rajwanshi, R., Chinnusamy, V., Bansal, K. C. (2012). Genome-wide classification and expression analysis of MYB transcription factor families in rice and arabidopsis. BMC Genomics 13, 544. doi: 10.1186/1471-2164-13-544
Kempe, K., Gils, M. (2011). Pollination control technologies for hybrid breeding. Mol. Breed. 27, 417–437. doi: 10.1007/s11032-011-9555-0
Kocábek, T., Mishra, A. K., Matoušek, J., Patzak, J., Lomnická, A., Khare, M., et al. (2018). The R2R3 transcription factor HlMYB8 and its role in flavonoid biosynthesis in hop (Humulus lupulus l.). Plant Sci. 269, 32–46. doi: 10.1016/j.plantsci.2018.01.004
Li, Y., Jiang, J., Du, M. L., Li, L., Wang, X. L., Li, X. B. (2013). A cotton gene encoding MYB-like transcription factor is specifically expressed in pollen and is involved in regulation of late anther/pollen development. Plant Cell Physiol. 54, 893–906. doi: 10.1093/pcp/pct038
Li, W. C., Zhang, Y., Zhao, S. Q. (2019). Silencing of arabidopsis RUS4 impairs anther endothecium secondary cell wall thickening. Chin. J. Cell Biol. 41, 619–626. doi: 10.11844/cjcb.2019.04.0009
Liu, C., Ma, N., Wang, P. Y., Fu, N., Shen, H. L. (2013). Transcriptome sequencing and DeNovo analysis of a cytoplasmic male sterile line and its near-isogenic restorer line in chili pepper (Capsicum annuum l.). PloS One 8, e65209. doi: 10.1371/journal.pone.0065209
Livak, K. J., Schmittgen, T. D. (2001). Analysis of relative gene expression data using real-time quantitative PCR and the 2(-delta delta C(T)) method. Methods 25, 402–408. doi: 10.1006/meth.2001.1262
Lü, J., Sui, X., Ma, S., Li, X., Liu, H., Zhang, Z. (2017). Suppression of cucumber stachyose synthase gene (CsSTS) inhibits phloem loading and reduces low temperature stress tolerance. Plant Mol. Biol. 95, 1–1. doi: 10.1007/s11103-017-0621-9
Ma, Y., Kang, J., Wu, J., Zhu, Y., Wang, X. (2015). Identification of tapetum-specific genes by comparing global gene expression of four different male sterile lines in Brassica oleracea. Plant Mol. Biol. 87, 541–554. doi: 10.1007/s11103-015-0287-0
Mandaokar, A., Browse, J. (2009). MYB108 acts together with MYB24 to regulate jasmonate-mediated stamen maturation in arabidopsis. Plant Physiol. 149, 851–862. doi: 10.1104/pp.108.132597
McCarthy, R. L., Zhong, R., Ye, Z. H. (2009). MYB83 is a direct target of SND1 and acts redundantly with MYB46 in the regulation of secondary cell wall biosynthesis in arabidopsis. Plant Cell Physiol. 50, 1950–1964. doi: 10.1093/pcp/pcp139
Nagpal, P., Ellis, C. M., Weber, H., Ploense, S. E., Barkawi, L. S., Guilfoyle, T. J., et al. (2005). Auxin response factors ARF6 and ARF8 promote jasmonic acid production and flower maturation. Development. 132, 4107–18. doi: 10.1242/dev.01955
Rhee, S. J., Seo, M., Jang, Y. J., Cho, S., Lee, G. P. (2015). Transcriptome profiling of differentially expressed genes in floral buds and flowers of male sterile and fertile lines in watermelon. BMC Genomics 16, 914. doi: 10.1186/s12864-015-2186-9
Singh, S. P., Srivastava, R., Kumar, J. (2015). Male Sterility systems in wheat and opportunities for hybrid wheat development. Acta Physiol. Plant 37, 1713. doi: 10.1007/s11738-014-1713-7
Song, S., Qi, T., Huang, H., Ren, Q., Wu, D., Chang, C., et al. (2011). The jasmonate-ZIM domain proteins interact with the R2R3-MYB transcription factors MYB21 and MYB24 to affect jasmonate-regulated stamen development in Arabidopsis. Plant Cell 23, 1000–1013. doi: 10.1105/tpc.111.083089
Stracke, R., Werber, M., Weisshaar, B. (2001). The R2R3-MYB gene family in Arabidopsis thaliana. Curr. Opin. Plant Biol. 4, 447–456. doi: 10.1016/S1369-5266(00)00199-0
Sun, B., Zhou, X., Chen, C., Chen, C., Chen, K., Chen, M., et al. (2020). Coexpression network analysis reveals an MYB transcriptional activator involved in capsaicinoid biosynthesis in hot peppers. Hortic. Res. 7, 162. doi: 10.1038/s41438-020-00381-2
Sun, B., Zu, Z., Chen, C., Chen, G., Cao, B., Chen, C., et al. (2019). Jasmonate-inducible R2R3-MYB transcription factor regulates capsaicinoid biosynthesis and stamen development in capsicum. J. Agric. Food Chem. 67, 10891–10903. doi: 10.1021/acs.jafc.9b04978
Tabata, R., Ikezaki, M., Fujibe, T., Aida, M., Tian, C. E., Ueno, Y., et al. (2010). Arabidopsis auxin response factor6 and 8 regulate jasmonic acid biosynthesis and floral organ development via repression of class 1 KNOX genes. Plant Cell Physiol. 51, 164–175. doi: 10.1093/pcp/pcp176
Vandesompele, J., De Preter, K., Pattyn, F., Poppe, B., Van Roy, N., De Paepe, A., et al. (2002). Accurate normalization of real-time quantitative RT-PCR data by geometric averaging of multiple internal control genes. Genome Biol. 3, RESEARCH0034. doi: 10.1186/gb-2002-3-7-research0034
Veenstra, G. J., Wolffe, A. P. (2001). Gene-selective developmental roles of general transcription factors. Trends Biochem. Sci. 26, 665–671. doi: 10.1016/S0968-0004(01)01970-3
Wang, H. Q., Guo, X. R., Yang, X. B., Su, J., Cao, X. Y. (2016). Screening of the proteins interacting with SmPAP1 of R2R3-MYB transcription factor from Salvia miltiorrhiza bunge by yeast two-hybrid system. Genom Appl. Biol. 35, 2819–2826. doi: 10.13417/j.gab.035.002819
Wang, Y., Mao, Z., Jiang, H., Zhang, Z., Chen, X. (2019). A feedback loop involving MdMYB108L and MdHY5 controls apple cold tolerance. Biochem. Biophys. Res. Commun. 512, 381–386. doi: 10.1016/j.bbrc.2019.03.101
Wang, Z., Yuan, C., Zhang, S. W., Tian, S. B., Tang, Q. L., Wei, D. Y., et al. (2021). Screening and interaction analysis identify genes related to anther dehiscence in Solanum melongena l. Front. Plant Sci. 12, 648193. doi: 10.3389/fpls.2021.648193
Wei, M. M., Song, M. Z., Fan, S. L., Yu, S. X. (2013). Transcriptomic analysis of differentially expressed genes during anther development in genetic male sterile and wild type cotton by digital gene-expression profiling. BMC Genomics 14, 97. doi: 10.1186/1471-2164-14-97
Wilson, Z. A., Song, J., Taylor, B., Yang, C. (2011). The final split: the regulation of anther dehiscence. J. Exp. Bot. 62, 1633–1649. doi: 10.1093/jxb/err014
Xu, X. F., Wang, B., Feng, Y. F., Xue, J. S., Qian, X. X., Liu, S. Q., et al. (2019). AUXIN RESPONSE FACTOR17 directly regulates MYB108 for anther dehiscence. Plant Physiol. 181, 645–655. doi: 10.1104/pp.19.00576
Yan, X. H., Dong, C. H., Yu, J. Y., Liu, W. H., Jiang, C. H., Liu, J., et al. (2013). Transcriptome profileanalysis of young floral buds of fertile and sterile plants from the self-pollinated offspring of the hybrid between novel restorer line NR1 and nsa CMS line in brassica napus. BMC Genomics 14, 26. doi: 10.1186/1471-2164-14-26
Yang, C., Song, J., Ferguson, A. C., Klisch, D., Simpson, K., Mo, R., et al. (2017). Transcription factor MYB26 is key to spatial specificity in anther secondary thickening formation. Plant Physiol. 175, 333–350. doi: 10.1104/pp.17.00719
Yuan, C., Zhang, S. W., Hu, R. L., Wei, D. Y., Tang, Q. L., Wang, Y. Q., et al. (2021). Comparative transcriptome analysis provides insight into the molecular mechanisms of anther dehiscence in eggplant (Solanum melongena l.). Genomics 113, 497–506. doi: 10.1016/j.ygeno.2020.12.032
Zhang, S., Zhao, Q., Zeng, D., Xu, J., Zhou, H., Wang, F., et al. (2019). RhMYB108, an R2R3-MYB transcription factor, is involved in ethylene- and JA-induced petal senescence in rose plants. Hortic. Res. 6, 131. doi: 10.1038/s41438-019-0221-8
Zhao, K., Cheng, Z., Guo, Q., Yao, W., Liu, H., Zhou, B., et al. (2020). Characterization of the poplar R2R3-MYB gene family and over-expression of PsnMYB108 confers salt tolerance in transgenic tobacco. Front. Plant Sci. 11. doi: 10.3389/fpls.2020.571881
Zhao, S. Q., Dong, J. J. (2015). Genetic regulation of anther and pollen development in Arabidopsis thaliana. J. Shanxi Univ: Nat. Sci. Ed 38, 177–184. doi: 10.13451/j.cnki.shanxi.univ(nat.sci.).2015.01.030
Zhao, Q., Wang, G., Ji J and Jin, C. (2012). Construction of plant expression vector with constitutive activation DREB2A and its genetic transformation to tobacco. China Biotech. 32, 42–48. doi: 10.1007/s11783-011-0280-z
Zhong, R., Lee, C., Zhou, J., McCarthy, R. L., Ye, Z. H. (2008). A battery of transcription factors involved in the regulation of secondary cell wall biosynthesis in arabidopsis. Plant Cell 20, 2763–2782. doi: 10.1105/tpc.108.061325
Zhong, R., Richardson, E. A., Ye, Z. H. (2007). The MYB46 transcription factor is a direct target of SND1 and regulates secondary wall biosynthesis in arabidopsis. Plant Cell 19, 2776–2792. doi: 10.1105/tpc.107.053678
Keywords: eggplant, MYB transcription factor, anther dehiscence, regulation of gene expression, RNA-seq
Citation: Hu R, Wang J, Yang H, Wei D, Tang Q, Yang Y, Tian S and Wang Z (2023) Comparative transcriptome analysis reveals the involvement of an MYB transcriptional activator, SmMYB108, in anther dehiscence in eggplant. Front. Plant Sci. 14:1164467. doi: 10.3389/fpls.2023.1164467
Received: 12 February 2023; Accepted: 16 June 2023;
Published: 11 July 2023.
Edited by:
Weibiao Liao, Gansu Agricultural University, ChinaReviewed by:
Weihua Qiao, Chinese Academy of Agricultural Sciences, ChinaZe Wu, Nanjing Agricultural University, China
Copyright © 2023 Hu, Wang, Yang, Wei, Tang, Yang, Tian and Wang. This is an open-access article distributed under the terms of the Creative Commons Attribution License (CC BY). The use, distribution or reproduction in other forums is permitted, provided the original author(s) and the copyright owner(s) are credited and that the original publication in this journal is cited, in accordance with accepted academic practice. No use, distribution or reproduction is permitted which does not comply with these terms.
*Correspondence: Zhimin Wang, minzniwang_555@163.com; Shibing Tian, 2508436730@qq.com
†These authors have contributed equally to this work