- 1State Key Laboratory for Biology of Plant Diseases and Insect Pests, Institute of Plant Protection, Chinese Academy of Agricultural Science, Beijing, China
- 2School of Life Sciences, Hebei University, Baoding, China
- 3Western Agricultural Research Center, Chinese Academy of Agricultural Sciences, Changji, China
Introduction: The establishment of invasive alien plants (IAPs) is primarily driven by climate warming and human activities, and their populations have a negative impact on agricultural economics, ecological systems, and human health. Lolium temulentum and Aegilops tauschii are critical IAPs in China because they reduce the quality of cereal grains and decrease wheat yields. Lolium temulentum is a winter-temperate weed that spreads easily and is poisonous to humans and animals. Aegilops tauschii is resistant to herbicides, has a high reproductive rate, and frequently grows in wheat. Both species have been listed in the Ministry of Agriculture and Rural Affairs of the People’s Republic of China’s management catalog since 2006.
Methods: In the present study, the historical occurrence and invasion of each species were collected and reconstructed, which showed that the population outbreak of L. temulentum began in 2010, whereas that of A. tauschii began in 2000. Using the optimal MaxEnt model, the geographical distributions of L. temulentum and A. tauschii were predicted based on screened species occurrences and environmental variables under the current and three future scenarios in the 2030s and 2050s (i.e., SSP1-2.6, SSP2-4.5, and SSP5-8.5).
Results: The mean AUC values were 0.867 and 0.931 for L. temulentum and A. tauschii, respectively. Human influence index (HII), mean temperature of coldest quarter (bio11), and precipitation of coldest quarter (bio19) were the most significant variables for L. temulentum, whereas human influence index, temperature seasonality (standard deviation×100) (bio4), and annual mean temperature (bio1) were the critical environmental variables for A. tauschi. Suitable habitat areas in China for L. temulentum and A. tauschii currently covered total areas of 125 × 104 and 235 × 104 km2, respectively. Future suitable areas of L. temulentum reached the maximum under SSP2-4.5, from 2021 to 2060, whereas for A. tauschii they reached the maximum under SSP5-8.5, from 2021 to 2060. Furthermore, the overlap area under the current climate conditions for L. temulentum and A. tauschii was approximately 90 × 104 km2, mainly located in Hubei, Anhui, Jiangsu, Shandong, Henan, Shaanxi, Shanxi, and Hebei. The overlap areas decreased in the 2030s, increased in the 2050s, and reached a maximum under SSP1-2.6 (or SSP2-4.5) with an approximate area of 104 × 104 km2. The centroid of L. temulentum in Henan was transferred to the southwest, whereas for A. tauschii it transferred to higher latitudes in the northeast.
Discussion: Our findings provide a practical reference for the early warning, control, and management of these two destructive IAP populations in China.
1. Introduction
Global trade, climate warming, and human-mediated transportation are the primary driving factors for invasive alien plants (IAPs), and these pose a threat to the economy, biodiversity, and human livelihood (Dukes and Mooney, 1999; Pejchar and Mooney, 2009; Diagne et al., 2021). Furthermore, climate warming can alter the geographical distribution of IAPs and increase their establishment rates, thereby facilitating the global spread of IAPs (Petitpierre et al., 2012; Bellard et al., 2013). The Intergovernmental Panel on Climate Change (IPCC) issued the sixth assessment report (AR6), indicating that temperature and global carbon dioxide (CO2) emissions will gradually increase under five Shared Socioeconomic Pathways (SSPs), namely SSP1-1.9, SSP1-2.6, SSP2-4.5, SSP3-7.0, and SSP5-8.5. Under the most optimistic scenarios, the global temperature would rise by 1.5 °C from 2050 to 2100, whereas under the worst-case emission scenario, the temperature would rise by 4.4 °C during this period; in either case, the warming would expand the geographical distribution of IAPs (Bellard et al., 2013; IPCC, 2021). Human activities, such as land use, urbanization, and trade transport, promote the invasion of IAPs through seed dispersal and disruption to native plant habitats (Vilà and Ibáñez, 2011; Rew et al., 2018). Furthermore, when improving trade contacts and construction economics, humans inevitably try to establish better dispersal corridors to transport plants or others items; however, these new pathways may detrimentally facilitate the spread of non-native plants to a new region as some seeds may be attached to the items being transported (Kueffer, 2017). Population growth and agricultural development are drivers of land use expansion and urbanization, which disturb native ecosystems, and inherently complement the wide niche of invasive plant establishment and colonization (Le Maitre et al., 2014). Therefore, estimating the distribution patterns of IAPs that are driven primarily by climate warming and human activities could improve the understanding of the spread of IAPs and help in the implementation of advanced control and management strategies worldwide.
As the third most plant-diverse country in the world, China has reported 402 IAPs, which threaten China’s rich biodiversity, disrupt ecosystem function, and even cause huge economic losses (Yan et al., 2012; Ma, 2020). Most IAPs with high reproduction and adaptation rates compete for light and nutrients and crowd out native species, leading to decreased biodiversity and genetic diversity (Keller and Shea, 2021). Previous studies have shown that invasive plant populations that outbreak in new regions of China are difficult to control, decrease the value of native species, and create economic losses in the order of 4.52–67.71 billion on field and wetland ecosystems and more than US$14.5 billion on pulling and pesticide development per year (Xu et al., 2006; Yan et al., 2012). Poaceae comprise the third most populated IAP in China, with half of the Poaceae family transported through foraging, and the remainder through cereal trade, including Lolium temulentum and Aegilops tauschii (Zhang et al., 2021a).
Lolium temulentum, known as darnel, is an annual C3 winter weed that is native to the Mediterranean and is widely distributed throughout North America, Asia, Europe, South America, Africa, and Oceania, in over 60 countries and 120 regions (Lush, 1976; Sarno et al., 1986; CABI, 2022). It was first recorded in China in 1954 in a shipment of wheat imported from Bulgaria, and has since spread to Southwest and Eastern China. Lolium temulentum has strong environmental adaptability under low temperature and high nitrogen (N), kalium (K), phosphorus (P) conditions, a high reproductive rate with a fibrous root system, and 6–30 terminal spikes that each spike with 7–9 nerved, mainly reproducing via seed (Terreix, 1968; Thomas et al., 2011). Darnel typically grows amongst crops of wheat and other winter cereals and has a similar size and weight to wheat, which makes it difficult to separate. Therefore, its presence leads to a decline in crop quality and causes yield loss (Ratera, 1983). The mix rate of darnel to wheat has been positively correlated with a reduction in wheat yield (Lin et al., 2004). In addition, darnel has three poisonous compounds, namely temulin and loline in the seeds and perloline in the stem (Swallen, 1961; Dannhardt and Steindl, 1985). When consumed with mixed wheat, these may cause dizziness, vomiting, and even death in humans and livestock (Liu et al., 2010). Previous studies have reported that, in addition to wheat losses, human poisoning has occurred in every province of China, including Shaanxi, Jiangsu, Heilongjiang, and Hubei, since the invasion of L. temulentum in the 1900s (Lin et al., 2004; Zhou et al., 2007).
Aegilops tauschii, is a noxious weed native to Eastern Europe and Western Asia that has subsequently spread in France, Italy, Turkey, the United States, and Mexico (Donald and Ogg, 1991; Fang et al., 2015). In 1955, it was found in China’s Henan Province, while now it is located in Shandong, Shanxi, Hebei, Henan, and Anhui. Aegilops tauschii typically grows with wheat, has a high reproductive rate with 5–13 terminal spikes, where each spike has 7–9 nerved via seed reproduction. Wheat and A. tauschii have similar seed sizes and germination and emergence conditions, which make it difficult to completely eradicate. The presence of A. tauschii causes wheat yield loss and a decrease in quality (Gong, 2022). This species has pesticide resistance, and the lack of new pesticides to control or destroy A. tauschii has facilitated the reckless spread of this IAP among wheat crops (Wang et al., 2021). One study showed a wheat yield decrease of 15–20%, whereas the worst situation attained a loss of 50% when A. tauschii was mixed with wheat crops, which mainly affected Henan, Shandong, and Shaanxi (Gao et al., 2021a; Wang et al., 2021).
Lolium temulentum and A. tauschii have continued to spread to new environments in provinces throughout China, and poses a potential threat to crops, primarily cereals (Wu et al., 2022). Both L. temulentum and A. tauschii are considered the most prolific weeds in wheat production industry and are listed in the “List of imported plant quarantine pests of the People’s Republic of China”, “National List of Invasive Alien Species under Key Management (First Batch)”, and “List of administrative regions where agricultural phytosanitary pests are distributed nationwide” by the Ministry of Agriculture and Rural Affairs in 2007, 2013, and 2016, respectively.
Species distribution models (SDMs), such as maximum entropy (MaxEnt), the genetic algorithm for rule-set prediction (GARP), and biomood2, have become important tools to predict spatial species distributions through mathematical algorithms consisting of species occurrence (or presence-absence) data and environmental variables (Peterson et al., 2011; Sillero, 2011). MaxEnt uses presence data alone, and provides efficient algorithms with optimal performance, toilless analysis, and avoids overfitting on other SDMs (Elith et al., 2006; Phillips et al., 2006; Phillips and Dudík, 2008). Previous studies on IAPs, including Prosopis juliflora, Ageratina adenophora, and Cenchrus spinifex, used the MaxEnt model to predict geographical distribution patterns (Cao et al., 2021; Singh et al., 2021; Xian et al., 2022). However, the default MaxEnt model has some methodological issues in balancing goodness-of-fit with model complexity, which leads to overfitting and poor transferability when projected in a novel environment (Warren and Seifert, 2011; Guevara et al., 2018). There are some ways to optimal MaxEnt parameters, such as ENMeval, SDMtune, and kuenm. Compared with other ways, ENMeval is widely used to avoid model overfitting via automatic matching of model parameter combinations (Muscarella et al., 2014). Therefore, based on Rstudio in the R software, ENMeval was developed to address the noise in the data and reduce biased sampling or evaluation methods in the default algorithm via tuning the settings for specific species and calibrating the data sets (Merow et al., 2014; Muscarella et al., 2014; Kass et al., 2021).
Lolium temulentum and A. tauschii are critical quarantine weeds in China, causing wheat yield reduction. However, the suitable and overlapping areas of two species are still unkown. Therefore, predicting the potential geographical distribution of L. temulentum and A. tauschii to provide a theoretical basis for future surveillance and control. In this study, we used the optimal MaxEnt to predict the geographical distribution of L. temulentum and A. tauschii throughout China under current and future climate scenarios. The steps included (1) reconstructing the historical invasion of L. temulentum and A. tauschii using ArcGIS software; and (2) predicting potential geographical distribution under the current, 2030s, and 2050s climate scenarios using the optimal MaxEnt model; (3) calculating future special changes and centroid transfer; (4) analyzing the overlap between L. temulentum and A. tauschii under the current and future climate scenarios; and (5) identifying significant environmental variables. Our results provide a foundation for the development of early warning, prevention, control measures, and co-management for L. temulentum and A. tauschii in China under predicted future climate change scenarios.
2. Material and methods
2.1. Occurrence records
Lolium temulentum occurrence records were collected from the Global Biodiversity Information Facility (GBIF.org, 2022a), the Chinese Virtual Herbarium (CVH; https://www.cvh.ac.cn/, accessed May 2022), the Ministry of Agriculture and Rural Affairs of the People′s Republic of China (http://www.moa.gov.cn/, accessed May 2022), and China National Knowledge Infrastructure (CNKI; https://www.cnki.net/). Aegilops tauschii occurrence records were downloaded from GBIF (GBIF.org, 2022b), CVH in August 2022, and CNKI. For non-longitude and latitude records, Google Earth was used to obtain precise coordinates. To decrease the effect of duplicate coordinates and sampling bias, all occurrence records for both species were filtered using ENMTools to ensure that only one record was retained in per 5 km × 5 km grid square (Figure 1). Ultimately, 4578 and 1966 occurrence records of L. temulentum and A. tauschii were retained worldwide, respectively.
2.2. Invasive historical reconstruction
Lolium temulentum and A. tauschii occurrence records were collected from CNKI and CVH. The year in which the earliest published articles or specimens were published was regarded as the invasive time in China. There were 217 for L. temulentum and 694 points for A. tauschii in China from 1950 to 2022. We used ArcGIS 10.5 was used with the GCS_Beijing_1954 geographic coordinate system, to map the invasive historical process of L. temulentum and A. tauschii over a 20-years interval in China.
2.3. Environmental variables
Current climate data that comprised 19 bioclimatic variables at 2.5 arc-min resolution from 1979 to 2013 were downloaded from PaleoClim v1.2 (http://www.paleoclim.org/) (Brown et al., 2018). Future climate and elevation data with 2.5 arc-min resolution were obtained from WorldClim v. 2.0 (https://www.worldclim.org/), and these included two time periods (2021–2040 and 2041–2060) and three climate scenarios (SSP1-2.6, SSP2-4.5, and SSP5-8.5) based on the BCC-CSM2-MR model (Eyring et al., 2016; Fick and Hijmans, 2017). Slope and Aspect were extracted from elevation data using ArcGIS software. Soil data (AWC_CLASS, T_OC, T_PH_H2O, and T_SAND) were downloaded from the Harmonized World Soil Database version 1.2 (https://www.fao.org/) (Fischer et al., 2008). The Global Human Influence Index (HII) from 1995 to 2004 was downloaded from the Socioeconomic Data and Applications Center (https://sedac.ciesin.columbia.edu/) (Wildlife Conservation Society and Center for International Earth Science Information Network, 2005). A total of 27 environmental variables are listed in Table S1.
The collinearity of environmental variables can influence parameter optimization during MaxEnt model calibration (Dormann et al., 2013). To address this issue, the “band collection statistics” in ArcGIS was used to estimate the linear correlations and eliminate the highly linear correlations of 27 environmental variables (Figure S1). All species occurrences of L. temulentum and A. tauschii and related environmental variables were entered into MaxEnt 3.4.4 to establish initial SDMs that provide percent contributions of each environmental variable. Comparing the correlation between two environmental variables (|r| > 0.8), the lower percent contribution was removed. Finally, 11 and 12 environmental variables screened for L. temulentum and A. tauschii are shown in Figure S2.
2.4. Model calibration, settings, and evaluation
SDMs, such as the MaxEnt model, face the challenge of balancing goodness-of-fit with model complexity because of their over-reliance on default settings (Warren and Seifert, 2011). Therefore, we used the R package (ENMeval) to calibrate the MaxEnt model to address these issues (Muscarella et al., 2014; Kass et al., 2021). Feature combinations (FCs) and regularization multipliers (RMs) are regarded as critical parameters for optimal settings in model calibration (Muscarella et al., 2014). RMs act synergistically in all FCs, which can adjust the level of regularization through each RM to protect against overfitting (Phillips, 2008). Based on the occurrence records and environmental variables of L. temulentum and A. tauschii, RM was set from 0.5 to 4, with a 0.5 interval, and six different FC combinations, including L, LQ, LQP, LQH, LQHP, and LQHPT (L = linear, H = hinge, Q = quadratic, P = product, and T= threshold) (Phillips and Dudík, 2008). The Akaike Information Criterion corrected (AICc) reflects the goodness-of-fit and model complexity, and the minimum AICc (i.e., Δ AICc = 0) was regarded as the best model parameter (Warren and Seifert, 2011). Therefore, for 48 combinations of FCs and RM values, FCs were set as LQHPT and RM was set to 0.5.
Optimized occurrence records and environmental variables of L. temulentum and A. tauschii were entered into MaxEnt 3.3.4; 25% of occurrence records were selected as training data, whereas the other 75% were considered testing data. The optimal MaxEnt model was set to replicate 10 times, and the replicated run type was set to “Bootstrap”. The maximum number of background points was set to 10000, and the output format was set to “Cloglog”, “Random seed” was chosen to improve the model’s randomness, and other parameters were left as default settings.
The area under the curve (AUC) of the receiver operator characteristic (ROC) is a metric for model performance (Roca et al., 2004). The AUC value is closely related to the rates of negative and positive values. The values range from 0 to 1, and those that are lower than 0.6 indicate that the model is unreliable. AUC values have been classified into four indices: poor (0.6 ≤ P < 0.7), moderate (0.7 ≤ P < 0.8), good (0.8 ≤ P < 0.9), and excellent (0.9 ≤ P < 1) (Swets, 1988).
2.5. Centroid transfer and threshold chosen
The centroid of change trend in geographical distribution from the current climate to future scenarios was calculated using the ArcGIS software. In this study, we focused on the centroid transfer of L. temulentum and A. tauschii in the 2030s and 2050s, for example, SSP1-2.6, SSP2-4.5, and SSP5-8.5. The geographical distributions of L. temulentum and A. tauschii were reclassified and transferred to a vector file that dissolved a single central point to describe the direction (longitude and latitude) of the predicted change from the current climate to future scenarios in the 2030s and 2050s. This allows the model to depict shifts in habitat by tracking the centroid transfer and transfer distance.
The potential geographical distribution of L. temulentum and A. tauschii in a particular raster was based on MaxEnt model output. The maximum test sensitivity plus specificity was chosen as the threshold (Cantor et al., 1999; Manel et al., 2001). The potential geographical distribution of L. temulentum was classified into the following four categories under current and future climates: unsuitable (P < 0.35), poor (0.35 ≤ P < 0.5), moderate (0.5 ≤ P < 0.7), and high (0.7 ≤ P < 1). Similarly, A. tauschii was also classified into the following categories: unsuitable (P < 0.25), poor (0.25 ≤ P < 0.4), moderate (0.4 ≤ P < 0.6), and high (0.6 ≤ P < 1). Furthermore, ArcGIS was used to calculate the niche overlap of L. temulentum and A. tauschii under the current climate and three future scenarios in the 2030s and 2050s. The threshold of binary classification was based on the maximum test sensitivity plus specificity, for L. temulentum: unsuitable (P < 0.35) and suitable (0.35 ≤ P < 1), and for A. tauschii: unsuitable (P < 0.25) and suitable (0.25 ≤ P < 1).
2.6. Niche breadth and niche overlap
Niche breadth is the range and variety of living conditions in a species’ niche, such as temperature range and food variety (Sexton et al., 2017). The value of niche breadth was calculated using ENMTools, Levins’ B1 and B2, that is, inverse concentration and uncertainty, respectively (Levins, 1968; Warren et al., 2010). The values of Levins’ B1 and B2 ranged from 0 to 1, indicating narrow to wide niche breadth (Levins, 1968). Furthermore, based on the ENMTools, there was value in evaluating the range of niche overlap in different species (Schoener’s D) (Schoener, 1968; Warren et al., 2008). The value of Schoener’s D ranged from 0 to 1, indicating that no niche overlapped to niche overlap identically (Warren et al., 2008). This could be directly compared to the traditional ecological assessment of niche overlap, including the overlap of microhabitat and diet.
3. Results
3.1. Model performance
For L. temulentum and A. tauschii, Δ AICc was calculated 400.24 and 254.95 in default MaxEnt model (FC = LQHPT and RM = 1). The optimal MaxEnt parameter settings for predicting the potential geographical distribution of L. temulentum and A. tauschii were when RM was set to 0.5, and FC was set as LQHPT via model calibration, Δ AICc was calculated 0. The mean AUC of L. temulentum and A. tauschii was 0.867 and 0.931, indicating the MaxEnt performance was “good” and “excellent”, respectively (Figure S3).
3.2. Invasive historical reconstruction
The reconstruction of the historical expansion of L. temulentum and A. tauschii is shown in Figure 2. The impact on the cereal trade from the spread of L. temulentum into China’s Heilongjiang province was first documented in 1956. Subsequently, L. temulentum gradually spread to most provinces, primarily in northwestern Xinjiang, the border of Gansu, Shaanxi, and Sichuan, and with a scattered distribution in Jilin, Shandong, Zhejiang, Anhui, Shanghai, Hubei, Jiangxi, Guangxi, Guangdong, and Tibet. Until 1990, the spread of L. temulentum increased in Qinghai, Jiangsu, Henan, Fujian, and Inner Mongolia, with the most notable increases in Hubei and Shaanxi. In the 2010s, the spread of L. temulentum increased in Hebei and Yunnan, with more increases in Yunnan and Jiangsu. To date, Liaoning, Tianjin, Beijing, Chongqing, Guizhou, and Ningxia are the most invaded regions of L. temulentum, which is primarily located in Gansu, Xinjiang, Yunnan, Hubei, Henan, Anhui, and Shandong. The historical spreading trend of L. temulentum began in Northwest China, spread to the Central China surrounding that region, then spread to North China, and sporadically throughout the Southwest and South China.
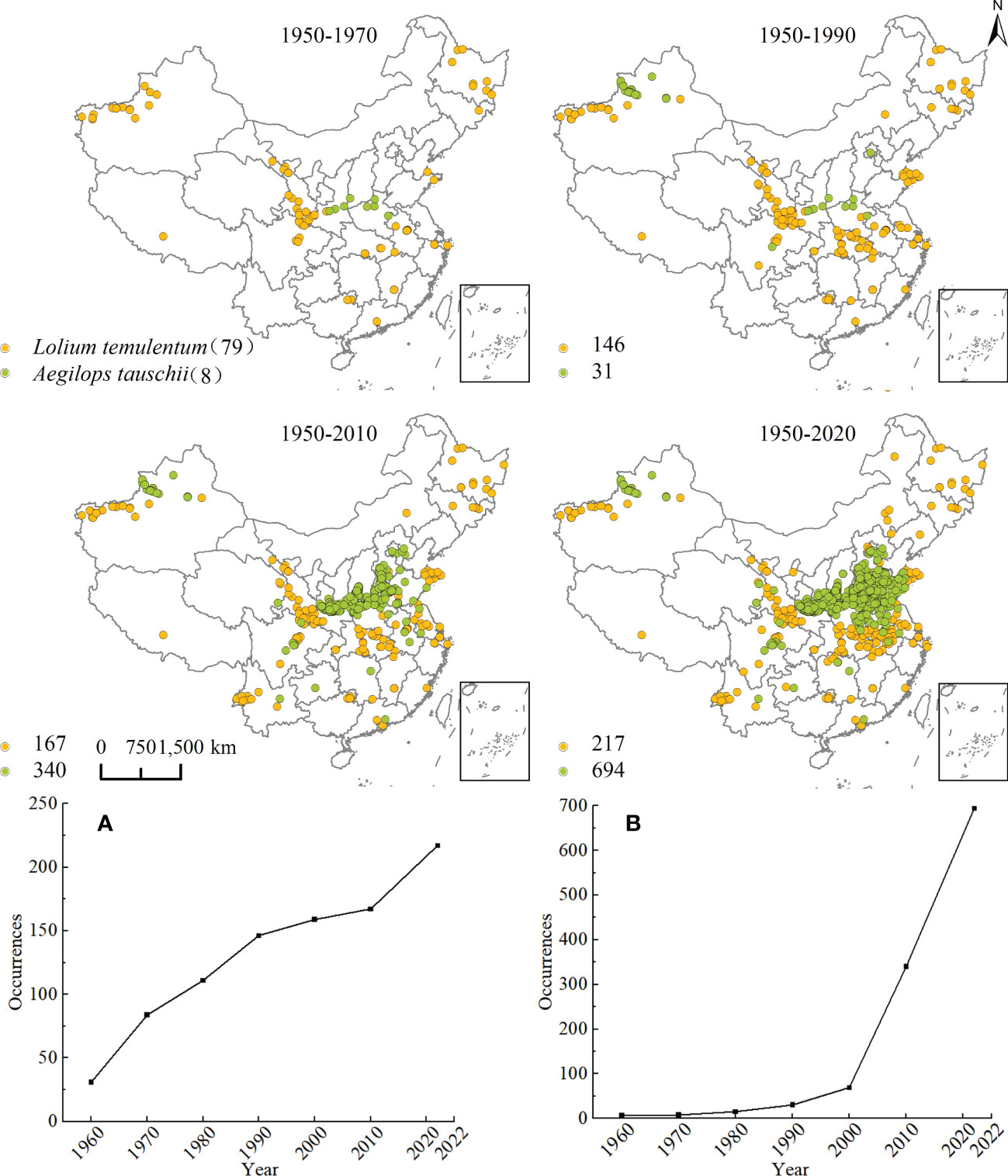
Figure 2 Historical reconstruction of the expansion process and increased species occurrence of (A) Lolium temulentum and (B) Aegilops tauschii from 1950 to 2022.
In 1950, it was reported that A. tauschii spread in Henan as the source of the D-gene in wheat, and spread sporadically in Shanxi and Shaanxi. Until 1990, A. tauschii had spread gradually in Xinjiang, Sichuan, and Beijing; however, by the 2010s, A. tauschii had spread to Jiangsu, Shaanxi, Guizhou, Hubei, Henan, Yunnan, Shandong, Shanxi, Guangdong, Anhui, and Gansu, primarily located on the border of Hubei, Henan, Shandong, and Shaanxi. Until now, Qinghai and Tianjin had become increasingly invaded regions, primarily in the Shaanxi, Shanxi, Hubei, Henan, Shandong, and Anhui regions. The historical trend shows the spread of A. tauschii originating in the southeast and northwest areas of Northwest China and spreading to the border of North China and down to Central and Eastern China. The spread continued constantly to the surrounding provinces but occurred more sporadically in areas in Southwest China.
The trends associated with species occurrence for L. temulentum and A. tauschii for the period from 1950 to 2022 are shown in Figure 2. The number of L. temulentum increased rapidly from 1950 to 1990, but then declined until 2010 because of global control strategies. In 2010, the rate of increase for L. temulentum recovered, and ultimately exceeded the rate from the initial period. The number of A. tauschii increased slowly after the introduction of the species; however, in the 2000s, its occurrence increased significantly.
3.3. Potential geographical distribution under the current and future climate scenarios
The potential geographical distribution of L. temulentum and A. tauschii for the current climate conditions in China is shown in Figure 3, and under the 2030s and 2050s three climate scenarios, namely SSP1-2.6, SSP2-4.5, and SSP5-8.5, are presented in Figures 4 and 5. The mainly suitable habitats under the current and future climate scenarios for L. temulentum were mainly located in northern Shanghai, southwestern and northeastern Sichuan, central Anhui, western Jiangsu, southern Gansu, central and southern Shaanxi, southwestern Shanxi, northern Henan, southern Hebei, and southern Beijing, Hubei, Tianjin, and Xinjiang.
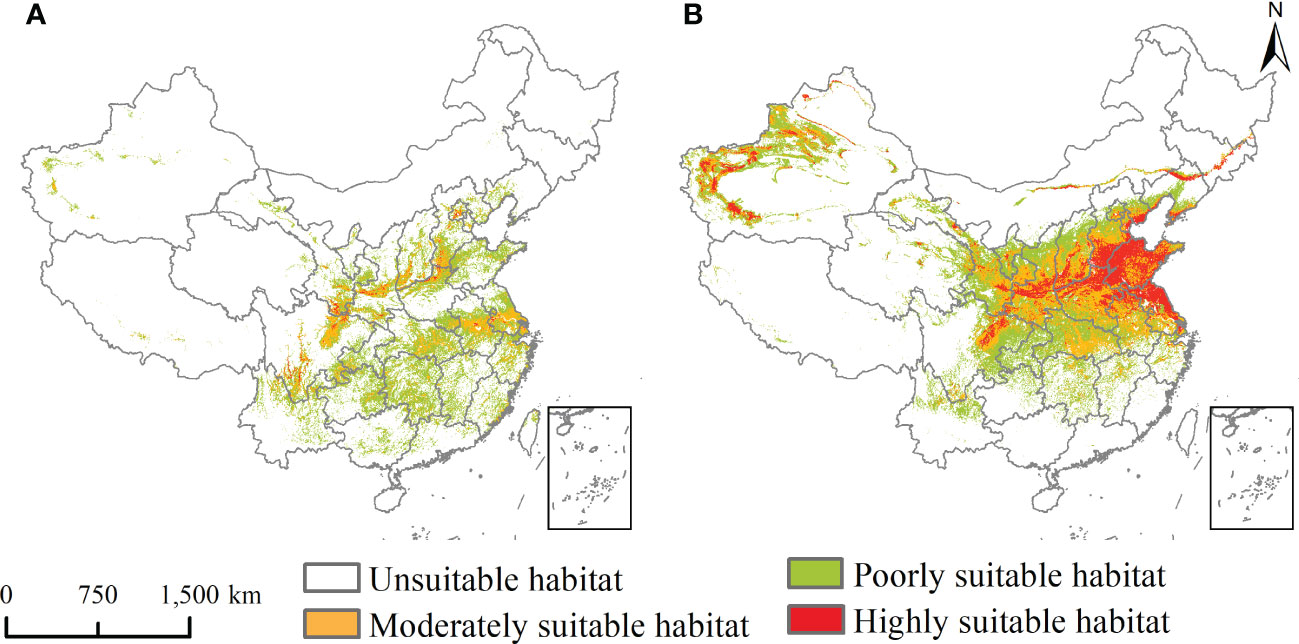
Figure 3 Potential geographical distribution of (A) Lolium temulentum and (B) Aegilops tauschii under the current climate.
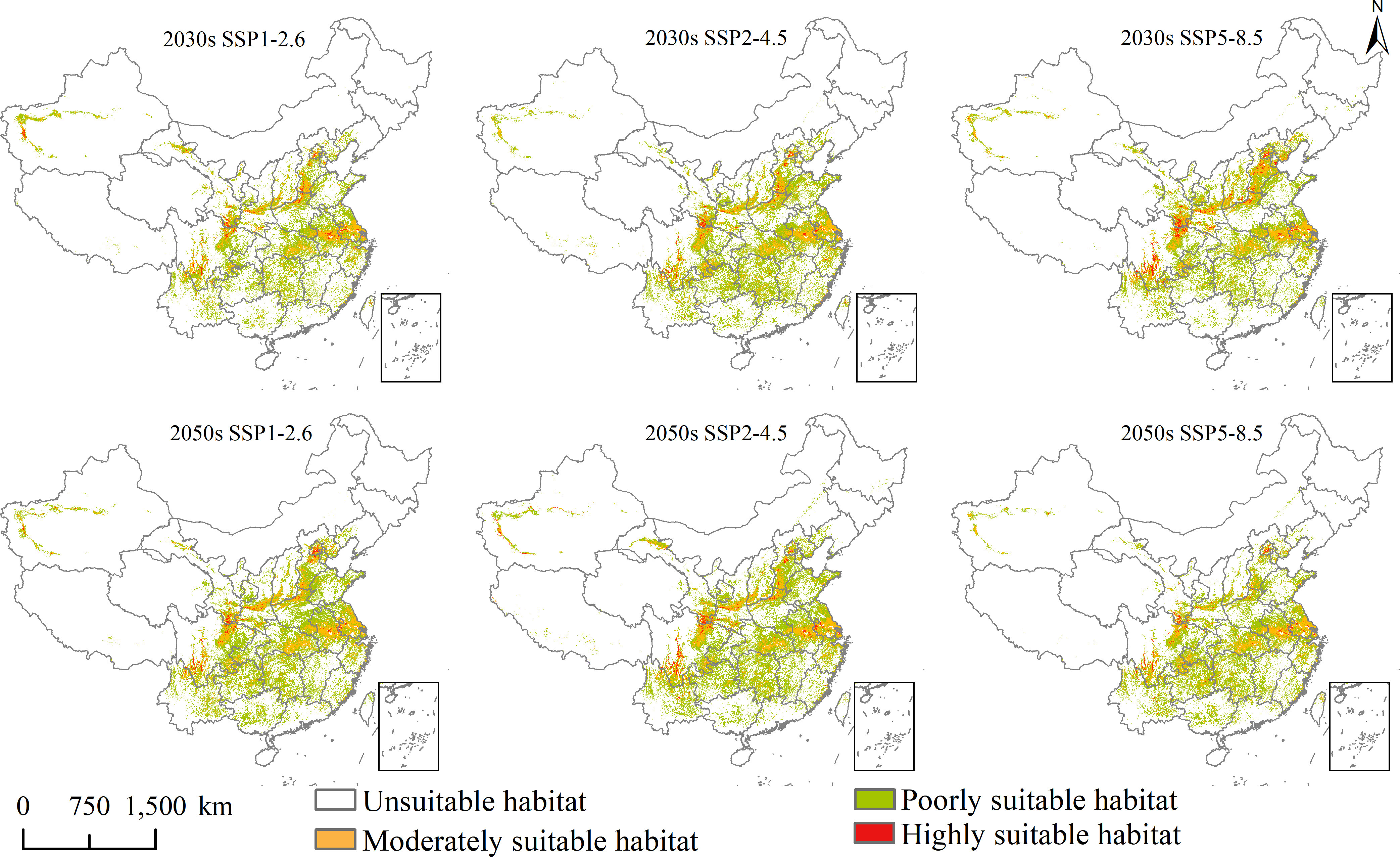
Figure 4 Future potential geographical distribution of Lolium temulentum under three scenarios in the 2030s and 2050s.
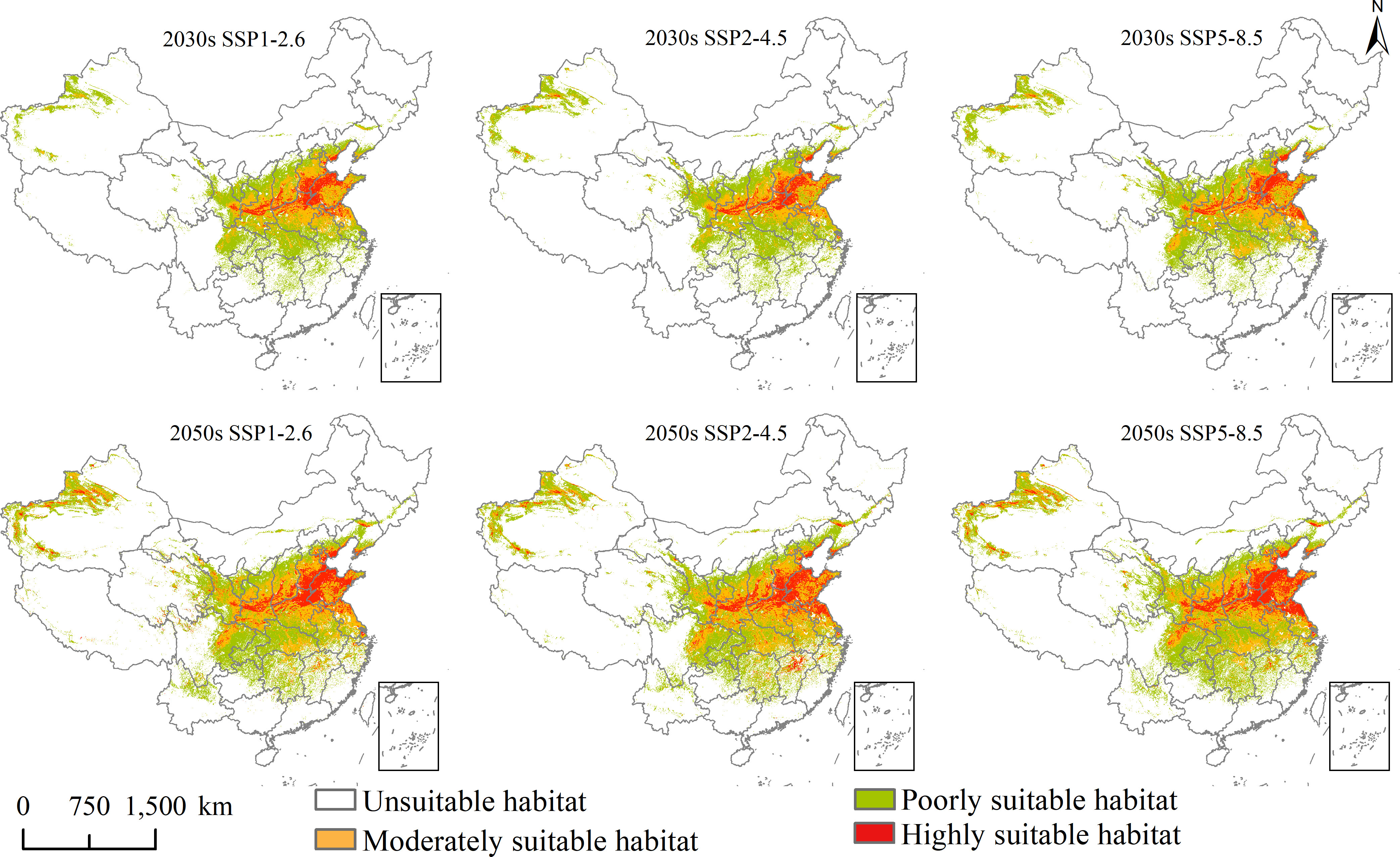
Figure 5 Future potential geographical distribution of Aegilops tauschii under three scenarios in the 2030s and 2050s.
The mainly suitable habitats under the current and future climate scenarios for A. tauschii were located primarily in northeastern Sichuan, central and southeastern Gansu, central Ningxia, central Shaanxi, northern and eastern Henan, northern Anhui, northern Shanghai, northern Jiangsu, Shandong, southern Hebei, southwestern Shanxi, southern Tianjin, central Beijing, northern and southern Liaoning, central Jilin, central Inner Mongolia, northwestern and southwestern Xinjiang, and Hubei.
In the current climate, the current total, highly, and moderately suitable habitat areas of L. temulentum were up to 125 × 104, 2 × 104, and 29 × 104 km2, accounting for 13.02, 0.21, and 3.02% of the total area of China, respectively. For A. tauschii, the current total, highly, and moderately suitable habitat areas were up to 235 × 104, 47 × 104, and 72 × 104 km2, accounting for 24.48, 4.90, and 7.50% of the total area of China, respectively.
Under SSP1-2.6, SSP2-4.5, and SSP5-8.5 in the 2030s, for L. temulentum, the overall trend in total, highly, and moderately suitable habitat areas of L. temulentum increased. The total and highly suitable habitat areas of L. temulentum reached the maximum under SSP2-4.5 and SSP5-8.5, up to 133 × 104 and 5 × 104 km2, accounting for 13.85 and 0.52% of the total area of China, respectively. For A. tauschii, the overall trend in total and highly suitable habitat areas of A. tauschii decreased. The total and highly suitable habitat areas reached the maximum under SSP5-8.5, up to 168 × 104 and 19 × 104 km2, accounting for 13.85 and 0.52% of the total area of China, respectively.
Under SSP1-2.6, SSP2-4.5, and SSP5-8.5 in the 2050s, for L. temulentum, the overall trend in total, highly, and moderately suitable habitat areas of L. temulentum increased. The total and highly suitable habitat areas of L. temulentum reached the maximum under SSP2-4.5, up to 144 × 104 and 4 × 104 km2, accounting for 15.00 and 0.42% of the total area of China, respectively. For A. tauschii, the overall trend in total and highly suitable habitat areas of A. tauschii increased. The total and highly suitable habitat areas reached the maximum under SSP5-8.5 and SSP2-4.5, up to 239 × 104 and 40 × 104 km2, accounting for 24.89 and 4.17% of the total area of China, respectively.
3.4. Future spatial changes and centroid transfer
Changes in the future potential geographical distribution of L. temulentum and A. tauschii under SSP1-2.6, SSP2-4.5, and SSP5-8.5 in the 2030s and 2050s were shown in Figures 6 and 7. Under the three scenarios in the 2030s, the increased habitat areas of L. temulentum in China were up to 32 × 104, 17 × 104, and 21 × 104 km2, mainly located in eastern Sichuan, northern and western Shandong, eastern Hebei, and northwestern Xinjiang, sporadically distributed in Southwest, Central, and Easter of China, and decreased habitat areas were up to 32 × 104, 17 × 104, and 21 × 104 km2, mainly located in northeastern and southeastern Guizhou, southern Shaanxi, southeastern Gansu, central Shanxi, and northern Shandong, sporadically distributed in Southwest and Central China. In the 2050s, the increased habitat areas of L. temulentum in China were up to 23 × 104, 22 × 104, and 28 × 104 km2, mainly distributed in northwestern and southeastern Yunnan, central Guizhou, eastern Sichuan, northern Anhui, southern Henan, central Jiangsu, and western Xinjiang, with decreased habitat areas of 23 × 104, 26 × 104, and 23 × 104 km2, sporadically distributed in eastern and western Southwest, Central, and southern North China.
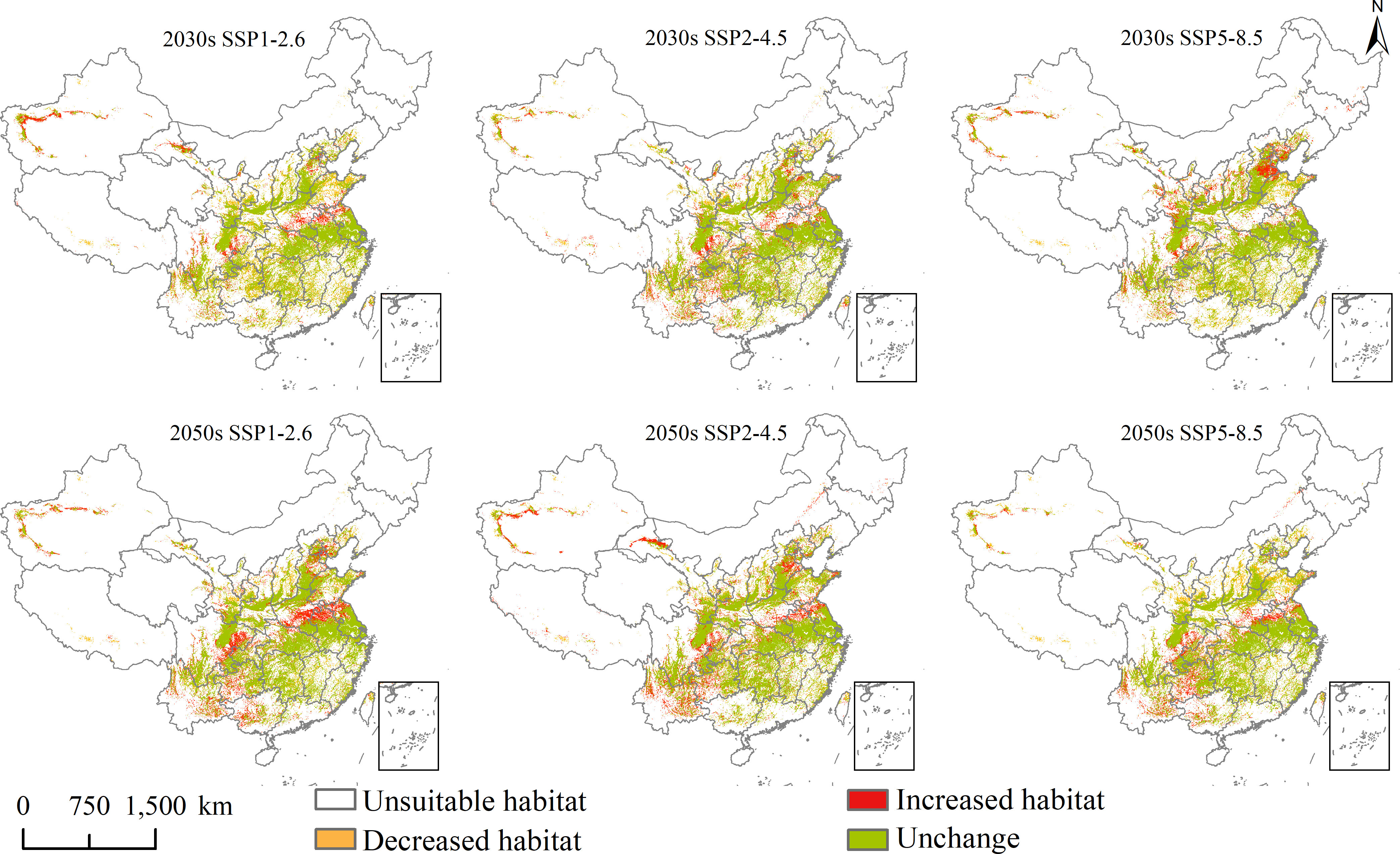
Figure 6 Future potential geographical distribution change of Lolium temulentum under three scenarios in the 2030s and 2050s.
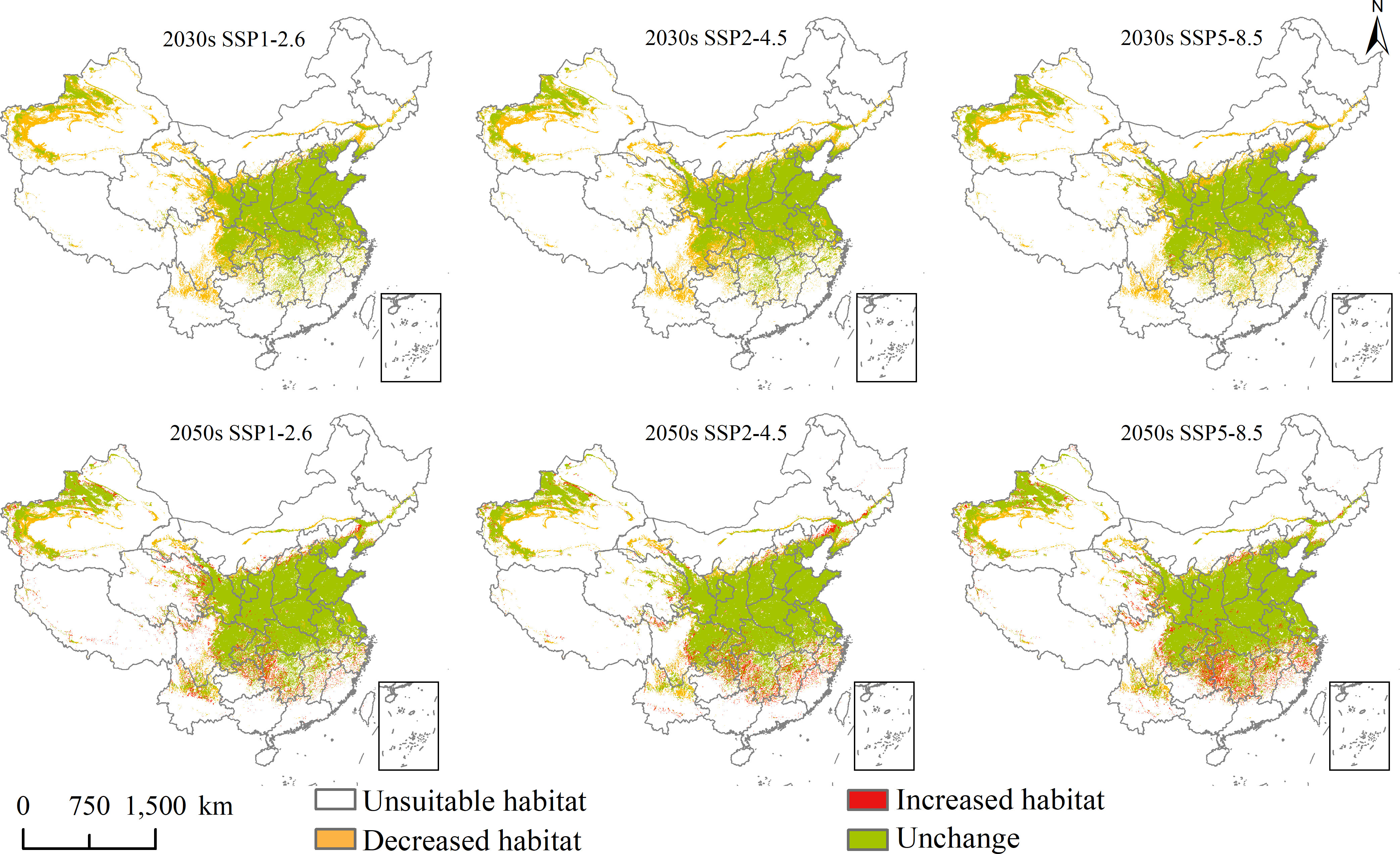
Figure 7 Future potential geographical distribution change of Aegilops tauschii under three scenarios in the 2030s and 2050s.
Under the three scenarios in the 2030s, the increased habitat areas of A. tauschii in China were up to 1 × 104, 0 × 104, and 1 × 104 km2, sporadically located in eastern Southwest and southern North China, and decreased habitat areas were up to 74 × 104, 73 × 104, and 68 × 104 km2, mainly located in northeastern Yunnan, southern and eastern Sichuan, southeastern Qinghai, southern and central Gansu, northwestern Ningxia, southwestern Liaoning, eastern Jilin, central Inner Mongolia, and northwestern and southwestern Xinjiang. In the 2050s, the increased habitat areas of A. tauschii in China were up to 23 × 104, 22 × 104, and 28 × 104 km2, and sporadically located in Yunnan, Sichuan, Chongqing, Guizhou, Hunan, Hunan, Jiangxi, Zhejiang, Anhui, Hubei, Qinghai, Shanxi, and Xinjiang, and the decreased habitat areas were up to 23 × 104, 26 × 104, and 23 × 104 km2, mainly located in northeastern Yunnan, southern Sichuan, northern Gansu, northern Ningxia, and northwestern and southwestern Xinjiang, and sporadically distributed in Tibet, Qinghai, Inner Mongolia and Liaoning.
The trend of centroid transfer of L. temulentum and A. tauschii in China from the current climate to the three scenarios (SSP 2.6, SSP 4.5, and SSP 8.5) in the 2030s and 2050s is shown in Figure S4. For L. temulentum, the centroid transferred to northeastern and northwestern in China from current to 2030s in the three scenarios, and moved to southeastern and southwestern under the 2050s in the three scenarios (Figure 3B). The longest transfer distance of L. temulentum centroid from the current climate to all future scenarios in the 2030s and 2050s was approximately 766.54 km and occurred under SSP5-8.5 in the 2030s. For A. tauschii, the centroid transferred to northeastern in China from current to 2030s in the three scenarios, and moved to southeastern and southern under the 2050s in the three scenarios (Figure 3A). The longest transfer distance of the centroid of A. tauschii from the current climate to the all future scenarios in the 2030s and 2050s was approximately 780.87 km under SSP1-2.6 in the 2050s.
3.5. Niche breadth and niche overlap
Using ENMTools, the niche breadths of L. temulentum and A. tauschii were determined. The B2 niche breadth value of L. temulentum was 0.9308, which was similar to the value of A. tauschii (B2 = 0.9006). These values indicate that L. temulentum and A. tauschii are likely to fit a broad niche. However, the D value was 0.4742, suggesting only moderate niche overlap between L. temulentum and A. tauschii.
Under the current climate, there are four scenarios, namely unsuitable habitat, the geographical distribution of either L. temulentum or A. tauschii, and the overlap of the two (Figure 8). The overlap area was 90 × 104 km2, and was primarily located in northern Yunnan, southern and northeastern Sichuan, northern Guizhou, southern Chongqing, Hunan, Jiangxi, northern Zhejiang, Shanghai, southeastern Hubei, central Anhui, southern Jiangsu, northern Shandong, northern and southern Henan, central and southwestern Shaanxi, southern and northeastern Gansu, southwestern and central Shanxi, southern Hebei, Beijing, southwestern Liaoning, and southern Xinjiang. The remaining areas of L. temulentum and A. tauschii were 35 × 104 and 145 × 104 km2, respectively.
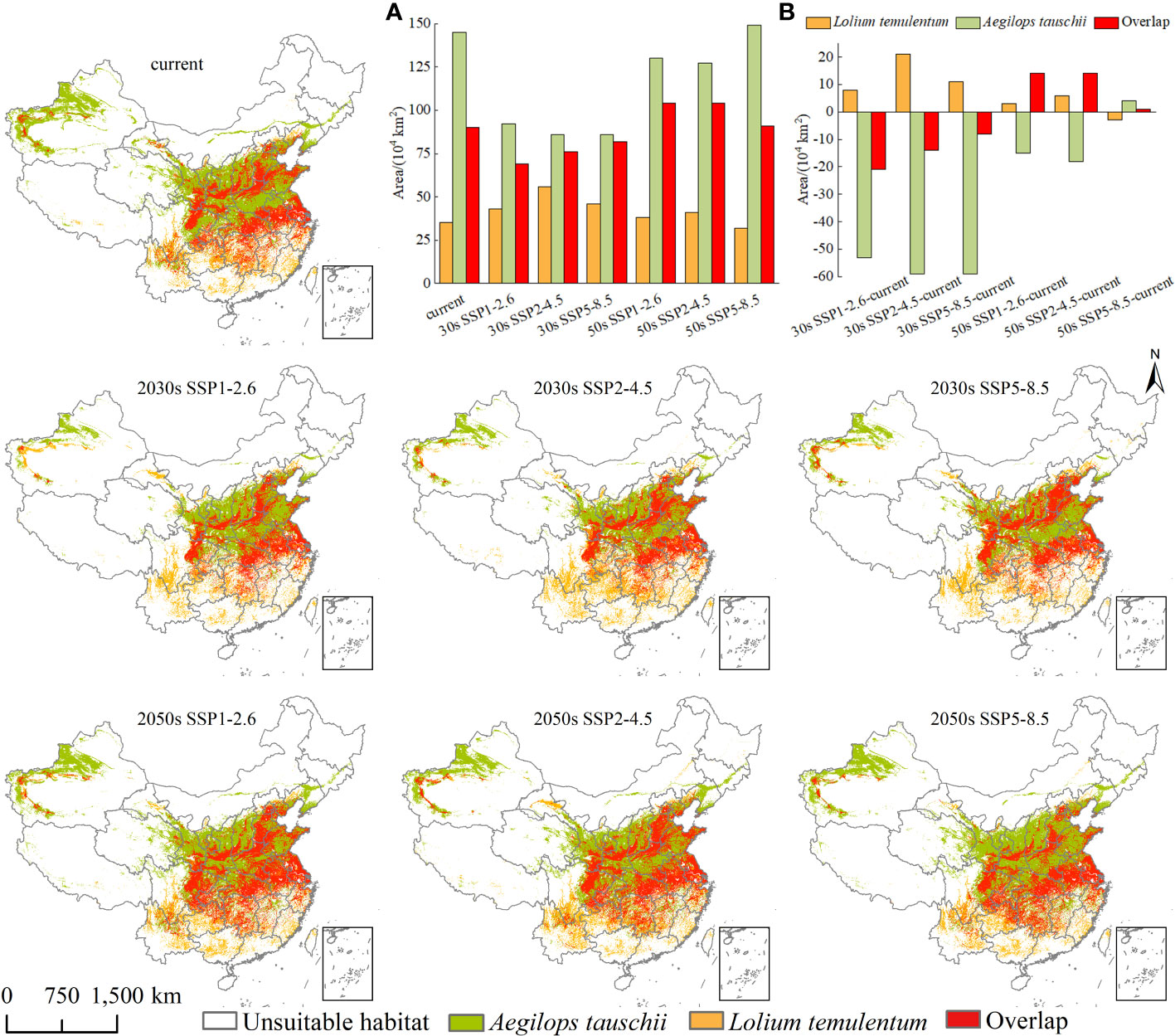
Figure 8 The niche overlap of Lolium temulentum and Aegilops tauschii under the current climate and future scenarios in the 2030s and 2050s, (A) the single plant and overlap areas, (B) the change of single plant and overlap areas from the current climate to the future scenarios.
The future overlap between L. temulentum and A. tauschii is shown in Figure 8. Compared with the current overlap area, the overlap area of both under the three scenarios in the 2030s declined, achieving a maximum area with approximately 82 × 104 km2 under SSP5-8.5-2030s, and increased in all scenarios in the 2050s, achieving a maximum of approximately 104 × 104 km2 under SSP1-2.6-2050s or SSP2-4.5-2050s. The overlap distribution of L. temulentum and A. tauschii in the 2030s and 2050s was similar to the current distribution, mainly distributed in East, Central, and eastern Southwest China. The remaining area of L. temulentum increased in the 2030s and 2050s in all scenarios except SSP5-8.5-2050s, achieving a maximum under SSP2-4.5-2030s (56 × 104 km2) and SSP2-4.5-2050s (41 × 104 km2), whereas A. tauschii decreased in the 2030s and 2050s in all scenarios except SSP 5-8.5-2050s, achieving a maximum under SSP1-2.6-2030s (92 × 104 km2) and SSP5-8.5-2050s (149 × 104 km2). The overlap change of L. temulentum and A. tauschii decreased by 21 × 104, 14 × 104,8 × 104 km2 in the 2030s under SSP1-2.6, SSP2-4.5, and SSP5-8.5, respectively, and increased in the 2050s by 14 × 104, 14 × 104,1 × 104 km2 for the same scenarios.
3.6. Environmental variables
We screened most significant environmental variables of L. temulentum and A. tauschii, through percent contribution and “Jackknife method”. For L. temulentum, human influence index (HII, 43.6%), mean temperature of coldest quarter (bio11, 27.7%), and precipitation of coldest quarter (bio19, 19.8%) were screened (Figure S2 and S5). For A. tauschii, human influence index (HII, 33.8%), temperature seasonality (standard deviation×100) (bio4, 30.8%) and annual mean temperature (bio1, 14.7%) were screened (Figures S2 and S6).
The probability of presence of significant environmental factors in L. temulentum and A. tauschii is shown in the response curve (Figure S7). This value is greater than 0.5, which indicates that the range of environmental variables is more suitable for the development of L. temulentum and A. tauschii. For L. temulentum, mean temperature for the coldest quarter was −8.52–16.53 °C, precipitation of coldest quarter was 72.64–914.52 mm, and the influence of human influence index on L. temulentum growth was increasing. For A. tauschii, the influence of human influence index was increasing, temperature seasonality was 7.68–10.57 °C, and annual mean temperature was 8.88–26.44 °C.
4. Discussion
Owing to the high reproductive rates, the toxicity of L. temulentum, and the pesticide resistance of A. tauschii, both species are regarded as significant IAPs in China. They threaten to decrease crop yield and wheat quality by robbing the soil of nutrients and competing with crops and hazard human health (Zhou et al., 2007; Thomas et al., 2011; Fang et al., 2015; Gao et al., 2021b). In this study, we identified the potential geographical distribution and niche overlap of L. temulentum and A. tauschii under the current climate and future scenarios in the 2030s and 2050s using the optimal MaxEnt model, which provides a basis for early warning and combined management.
4.1. Significant environmental variables
The most critical environmental variable for the distribution patterns of L. temulentum and A. tauschii was HII. Previous studies have confirmed that the established and spread of IAP populations in a new environment are closely linked to human activities, such as trade, tourism, transport, and human population density (McKinney, 2001; Pyšek et al., 2010). Another study indicated that the niche width of non-native plants increases with human activities (Irl et al., 2021). Non-native plants are carried by humans through travel and trade transportation, leaving their native region to novel environment (Boivin et al., 2016; Kueffer, 2017). Once, these invasive plants establish themselves and the populations spread, they integrate into ecosystems and ultimately have a negative influence on native species in the region (Kueffer, 2017). In our results, the value of HII increased concomitantly with increases in the probability of the species presence, revealing that HII played an important role and possibly influenced the geographical distribution of L. temulentum and A. tauschii.
In addition, for L. temulentum, bio11 and bio19 were the greatest contributions among 14 environmental variables. Low temperatures and high soil moisture are the conditions needed for the germination and growth of L. temulentum (Sagar et al., 1979). Vernalization can accelerate flowering, although L. temulentum seeds are dormant at 2 °C, but this did not reduce the seeds’ viability (Steiner and Ruckenbauer, 1995). When the temperature is higher than 5 °C, Lolium spp. develop a ‘stored growth’ potential that accelerate the extension of leaves (Peacock, 1975; Fournier et al., 2005). Our results showed that bio11 was −8.52–16.53 °C, which was consistent with the characteristic of low-temperature growth. Temperature, precipitation, and HII were vital environmental variables for L. temulentum growth. One study indicated that a mean annual precipitation of 400 to 1200 mm was an optimal development condition (CABI, 2022). Furthermore, we showed that approximately 900 mm of bio19, was optimum to establish populations for L. temulentum. For A. tauschii, and the greatest contributions among 17 environmental variables were bio4 and bio1, which were selected to predict the geographical distribution of A. tauschii in the optimal MaxEnt model. Previous studies have shown that the germination temperatures of A. tauschii range from 5 to 35 °C (Fang et al., 2015). Our results showed that the optimal range of temperature seasonality and annual mean temperature was consistent with this characteristic. The climatic characteristics of the living species were the dominant factors for population regeneration and establishment (Hansen et al., 2018).
4.2. Invasive historical reconstruction
Non-native plants that establish populations in novel environments undergo a two-stage process (Aikio et al., 2010). The first phase is the lag phase, when the occurrence of the invasive plants increase slowly or do not change at all, and the second is increase phase, species occurrence increase markedly (Crooks and Soulé, 1999). The lag phase is speculated to be closely linked to an adaption period invasive genotypes, abrupt invasion mechanisms, and environmental switches (Aikio et al., 2010). The invasive historical reconstruction of A. tauschii, showed that species occurrence increased slowly over 30 to 40 years after it invaded China, followed by population outbreak in 2000. This is exemplary of the two-stage process shown by the initial lag phase followed by a state of rapid growth. Alternatively, for L. temulentum, there was no obvious pattern, just a moderate increase. It has been suggested that this may have been influenced by sampling bias, such as incomplete survey techniques and monitoring (Delisle et al., 2003). All occurrences of L. temulentum and A. tauschii increase as awareness of IAPs increase and this is attributed to the progressive increases in the search and documentation of species occurrence (Cousens and Mortimer, 1995). Lolium temulentum and A. tauschii are critical IAPs in China, and their occurrence could continue to increase in the future.
4.3. Geographical distribution and ecological niche
The potential geographical distribution of some invasive plants will shrink, whereas others will expand with climate change (Tu et al., 2021; Zhang et al., 2021b). The suitable areas of Ageratina adenophora in China would decrease under the 2050s, whereas increased in the 2090s (Zhang et al., 2022). In this study, the total trend in suitable areas for L. temulentum increased from the current climate to future scenarios (SSP1-2.6, SSP2-4.5, and SSP5-8.5) in the 2030s and 2050s. This result is similar to other studies on the potential geographical distribution of non-invasive plants; for example, suitable areas of Cenchrus ciliaris increased under SSP1-2.6 and SSP2-4.5-2050s, and for Spartina anglica the suitable areas increase under SSP5-8.5-2100 in Northern Europe (Borges et al., 2021; Siller-Clavel et al., 2022). IPCC has indicated that global warming is likely to increase by 1.5 °C from 2030 to 2052 at the current rate (IPCC, 2021). The radiative forcing gradually increased in SSP1-2.6, SSP2-4.5, and SSP5-8.5, reaching 2.6, 4.5, and 8.5 W/m2, respectively, indicating that global CO2 concentrations are rising. Lolium temulentum is a C3 plant that strengthens photosynthesis to advance nutrient absorption, facilitating the development of a rise in CO2 concentrations, so the predicted suitable areas of L. temulentum will likely increase in future scenarios (Lush, 1976). Previous studies have shown that the suitable areas for some invasive plants shrank but still had a significant invasion risk, such as Xanthium italicum (Zhang et al., 2021b). This result is consistent similar with the predicted suitable areas for A. tauschii in the 2030s in this study, but it ultimately ended up increasing in the 2050s. Furthermore, the IPCC report indicated that the yield of some crops that grow at different latitudes, such as wheat, maize, and sugar beet, have been influenced by climate change in recent years (IPCC, 2021). We hypothesize that the change in the potential geographical distribution of L. temulentum and A. tauschii is related to this.
Plants can change their physiological characteristics to migrate to a new habitat or respond more suitably to climate change (Davis and Shaw, 2001).However, not all species have the ability to evolve adaptations that respond to rapid climate change; therefore, migration is considered a critical pathway to address this issue (Etterson and Shaw, 2001; Corlett and Westcott, 2013). some non-native plants, such as Alternanthera philoxeroides and Ambrosia artemisiifolia, are extremely adaptable and can migrate whereas others cannot with climate change (Tu et al., 2021). Previous studies have shown that climate change plays a critical role in range-shifting invasive plants, which may be geographically stable with climate warming, or shifting to different directions in future scenarios (Allen and Bradley, 2016; Rockwell-Postel et al., 2020). Our data show that the centroid of A. tauschii is primarily located in Henan from the current climate and in future scenarios, and it is unable to keep pace with climate change. However, L. temulentum transfers to the north and to different provinces under future scenarios, which indicates high adaptability to climate change.
Niche overlap is deemed to be ecological species that exhibit biological characteristics and ecological adaptability, including similarities in resource utilization and species competition (Jing et al., 2015). When the value of niche width is greater, it indicates greater adaptability to the environment and niche overlap (Wang et al., 2019). Our results demonstrate that L. temulentum and A. tauschii have a lot of similarities in resource utilization and geographical distribution, which may be due to the synergistic reduction in wheat quality. By calculating B1 and B2, it is evident that L. temulentum is more adaptable to the habitat of A. tauschii. The toxicity of L. temulentum threatens human and animal health, and the high reproduction rate and pesticide resistance of A. tauschii pose a significant threat of its spread. Therefore, some control measures have been considered and are proposed herein.
4.4. Control measures
We demonstrated that the current overlap of L. temulentum and A. tauschii is primarily distributed in Yunnan, Sichuan, Guizhou, Chongqing, Hunan, Jiangxi, Zhejiang, Shanghai, Hubei, Anhui, Jiangsu, Shandong, Henan, Shaanxi, Gansu, Shanxi, Hebei, Beijing, Liaoning, and Xinjiang, and this is consistent with previous study (Fang et al., 2013; CABI, 2022). Yunnan, Zhejiang, and province regions were predicted to be novel environments that exist at high invasive risk for L. temulentum and A. tauschii. Spreading occurs primarily from seed dispersal during wheat trade transport to other provinces in China, therefore it is quintessential to secure wheat breeding bases without L. temulentum and A. tauschii seeds to increase wheat quality and purity, and these acceptance are considered effective control measures (Terreix, 1968; Fang et al., 2013; Gong, 2022).
Furthermore, other studies have suggested that cultural control and chemical control are effective means for controlling L. temulentum and A. tauschii. Cultural control includes establishing long rotations periods that disturb the soil environment or using wheat seed cleaning screens (Ferrari et al., 1984). Another study demonstrated that sowing wheat 30 and 60 days later and hand weeding twice was effective in controlling L. temulentum (Angiras and Rana, 1990). Strengthening seed quarantine, crop rotation that changes the environment of weed populations, and artificially pulling weeds are effective ways to control A. tauschii (Yu et al., 2018). However, pulling weeds can be difficult because of the similarities between L. temulentum and wheat. Therefore, individuals should take measures to control L. temulentum in combination with pesticides. The application of triallate and metoxuron to the soil can effectively control L. temulentum development before sowing wheat (Stevens and Meyes, 1976). In addition, the pre-emergence of wheat applications of metribuzin or pendimethalin show substantial governance (Cairns et al., 1979). As for A. tauschii, mesosulfuron-methyl is considered an effective herbicide, whereas excessive spraying could restrain wheat development such that seedlings become yellow or weak (Wu et al., 2022). Therefore, the dose, proportion of inert ingredients, and spraying time of mesosulfuron-methyl are the important issues to consider to avoid herbicide pollution. Recent studies have shown that mesosulfuron-methyl is not a permanent control of A. tauschii growth, which have developed resistance in parts of China, including the northwest plain, central mountains, and northern coastal areas in Shandong (Gao, 2021).
5. Conclusions
Lolium temulentum and A. tauschii are the primary contributors to wheat loss in China. Climate warming and human activities have accelerated the invasion of these IAPs and the establishment of L. temulentum and A. tauschii in new habitats throughout China. Reconstructing the historical invasive progress of L. temulentum and A. tauschii in China showed that L. temulentum and A. tauschii spread to new environments that were suitable to their development and potential expansion. Based on the occurrence of the screened species and related environmental variables, the suitable habitat and overlap area of L. temulentum and A. tauschii were predicted using the optimal MaxEnt. Our results showed that the total trend in suitable areas of L. temulentum increased under SSP1-2.6, SSP2-4.5, and SSP5-8.5 in the 2030s and 2050s, whereas that of A. tauschii decreased. Meanwhile, the overlap areas of L. temulentum and A. tauschii decreased in the 2030s but increased in the 2050s, mainly in central and eastern China. The future centroid of L. temulentum transferred to higher latitudes, while A. tauschii transferred to lower latitudes compared to the current climate, which revealed that climate change played a critical role in geographical distribution. The results obtained from optimal MaxEnt provide new insights for long-term detection and management.
Data availability statement
The original contributions presented in the study are included in the article/Supplementary Material. Further inquiries can be directed to the corresponding author.
Author contributions
MY and HZ and: conception and design of the research. MY: acquisition of data, analysis and interpretation of data. MY and HZ: statistical analysis. HZ: drafting the manuscript. XX, RW, NY, LC, and W-XL: manuscript revision. All authors contributed to the article and approved the submitted version.
Funding
This work was supported by the National Key R&D Program of China (grant no. 2021YFC2600400; grant no. 2022YFC2601100), Technology Innovation Program of Chinese Academy of Agricultural Sciences (grant no. caascx-2021-2025-IAS), and Key R&D Program of Yunnan province (grant no. 202103AF140007).
Conflict of interest
The authors declare that the research was conducted in the absence of any commercial or financial relationships that could be construed as a potential conflict of interest.
Publisher’s note
All claims expressed in this article are solely those of the authors and do not necessarily represent those of their affiliated organizations, or those of the publisher, the editors and the reviewers. Any product that may be evaluated in this article, or claim that may be made by its manufacturer, is not guaranteed or endorsed by the publisher.
Supplementary material
The Supplementary Material for this article can be found online at: https://www.frontiersin.org/articles/10.3389/fpls.2023.1113567/full#supplementary-material
References
Aikio, S., Duncan, R. P., Hulme, P. E. (2010). Lag-phases in alien plant invasions: Separating the facts from the artefacts. Oikos 119, 370–378. doi: 10.1111/j.1600-0706.2009.17963.x
Allen, J. M., Bradley, B. A. (2016). Out of the weeds? reduced plant invasion risk with climate change in the continental united states. Biol. Conserv. 203, 306–312. doi: 10.1016/j.biocon.2016.09.015
Angiras, N. N., Rana, M. C. (1990). Weed and phosphorus management in gobhi sarson (Brassica napus) under mid-hill conditions of himachal pradesh. Indian J. Weed Sci. 22, 57–64.
Bellard, C., Thuiller, W., Leroy, B., Genovesi, P., Bakkenes, M., Courchamp, F. (2013). Will climate change promote future invasions? Global Change Biol. 19, 3740–3748. doi: 10.1111/gcb.12344
Boivin, N. L., Zeder, M. A., Fuller, D. Q., Crowther, A., Larson, G., Erlandson, J. M., et al. (2016). Ecological consequences of human niche construction: Examining long-term anthropogenic shaping of global species distributions. P. Natl. Acad. Sci. U.S.A. 113, 6388–6396. doi: 10.1073/pnas.1525200113
Borges, F. O., Santos, C. P., Paula, J. R., Mateos-Naranjo, E., Redondo-Gomez, S., Adams, J. B., et al. (2021). Invasion and extirpation potential of native and invasive Spartina species under climate change. Front. Mar. Sci. 8. doi: 10.3389/fmars.2021.696333
Brown, J. L., Hill, D. J., Dolan, A. M., Carnaval, A. C., Haywood, A. M. (2018). PaleoClim, high spatial resolution paleoclimate surfaces for global land areas. Sci. Data 5, 180254. doi: 10.1038/sdata.2018.254
CABI (2022) Lolium temulentum (darnel). Available at: https://www.cabi.org/isc/datasheet/31169 (Accessed October 19, 2022).
Cairns, A. L. P., Loubser, J. W., Roux, D. (1979). “The use of pre-emergence herbicides on wheat in the Western cape,” in Proceedings of the Third National Weeds Conference of South Africa. 123–129.
Cantor, S. B., Sun, C. C., Tortolero-Luna, G., Richards-Kortum, R., Follen, M. (1999). A comparison of C/B ratios from studies using receiver operating characteristic curve analysis. J. Clin. Epidemiol. 52, 885–892. doi: 10.1016/S0895-4356(99)00075-X
Cao, J., Xu, J., Pan, X., Monaco, T. A., Zhao, K., Wang, D., et al. (2021). Potential impact of climate change on the global geographical distribution of the invasive species, Cenchrus spinifex (Field sandbur, gramineae). Ecol. Indic. 131, 108204. doi: 10.1016/j.ecolind.2021.108204
Corlett, R. T., Westcott, D. A. (2013). Will plant movements keep up with climate change? Trends Ecol. Evol. 28, 482–488. doi: 10.1016/j.tree.2013.04.003
Cousens, R., Mortimer, M. (1995). Dynamics of weed populations (Cambridge: Cambridge University Press).
Crooks, J. A., Soulé, M. E. (1999). “Lag times in population explosions of invasive species: causes and implications,” in Invasive species and biodiversity management. Eds. Sandlund, O. T., Schei, P. J., Viken, A. (Dordrecht: Kluwer Academic Press), 103–125.
Dannhardt, G., Steindl, L. (1985). Alkaloids of Lolium temulentum: isolation, identification and pharmacological activity. Planta Med. 53, 212–214. doi: 10.1055/s-2007-969459
Davis, M. B., Shaw, R. G. (2001). Range shifts and adaptive responses to quaternary climate change. Science 292, 673–679. doi: 10.1126/science.292.5517.673
Delisle, F., Lavoie, C., Jean, M., Lachance, D. (2003). Reconstructing the spread of invasive plants: taking into account biases associated with herbarium specimens. J. Biogeogr. 30, 1033–1042. doi: 10.1046/j.1365-2699.2003.00897.x
Diagne, C., Leroy, B., Vaissière, A.-C., Gozlan, R. E., Roiz, D., Jarić, I., et al. (2021). High and rising economic costs of biological invasions worldwide. Nature 592, 571–576. doi: 10.1038/s41586-021-03405-6
Donald, W. W., Ogg, A. G., Jr. (1991). Biology and control of jointed goatgrass (Aegilops cylindrica), a review. Weed Technol. 5, 3–17. doi: 10.1017/S0890037X00033170
Dormann, C. F., Elith, J., Bacher, S., Buchmann, C., Carl, G., Carré, G., et al. (2013). Collinearity: a review of methods to deal with it and a simulation study evaluating their performance. Ecography 36, 27–46. doi: 10.1111/j.1600-0587.2012.07348.x
Dukes, J. S., Mooney, H. A. (1999). Does global change increase the success of biological invaders? Trends Ecol. Evol. 14, 135–139. doi: 10.1016/S0169-5347(98)01554-7
Elith, J., Graham, C. H., Anderson, R. P., Dudík, M., Ferrier, S., Guisan, A., et al. (2006). Novel methods improve prediction of species’ distributions from occurrence data. Ecography 29, 129–151. doi: 10.1111/j.2006.0906-7590.04596.x
Etterson, J. R., Shaw, R. G. (2001). Constraint to adaptive evolution in response to global warming. Science 294, 151–154. doi: 10.1126/science.1063656
Eyring, V., Bony, S., Meehl, G. A., Senior, C. A., Stevens, B., Stouffer, R. J., et al. (2016). Overview of the coupled model intercomparison project phase 6 (CMIP6) experimental design and organization. Geosci. Model. Dev. 9, 1937–1958. doi: 10.5194/gmd-9-1937-2016
Fang, F., Gao, X., Wei, S., Li, Y., Li, M., Zhang, C. (2015). Occurrence and effects of Aegilops tauschii in China. Acta Pratac. Sin. 24, 194–201. doi: 10.11686/cyxb20150222
Fang, F., Zhang, C., Huang, H., Li, Y., Chen, J., Yang, L., et al. (2013). Potential distribution of tausch's goatgrass (Aegilops tauschii) in both China and the rest of the world as predicted by MaxEnt. Acta Pratac. Sin. 22, 62–70.
Ferrari, C., Speranza, M., Catizone, P. (1984). “Weeds and crop management of wheat in northern Italy,” in COLUMA/EWRS (Paris: COLUMA/EWRS).
Fick, S. E., Hijmans, R. J. (2017). WorldClim 2: new 1-km spatial resolution climate surfaces for global land areas. Int. J. Climatol. 37, 4302–4315. doi: 10.1002/joc.5086
Fischer, G., Nachtergaele, F., Prieler, S., van Velthuizen, H. T., Verelst, L., Wiberg, D. (2008) Data from: Global agro-ecological zones assessment for agriculture (GAEZ 2008). Available at: https://www.fao.org/soils-portal/data-hub/soil-maps-and-databases/harmonized-world-soil-database-v12/en/.
Fournier, C., Durand, J. L., Ljutovac, S., Schäufele, R., Gastal, F., Andrieu, B. (2005). A functional–structural model of elongation of the grass leaf and its relationships with the phyllochron. New Phytol. 166, 881–894. doi: 10.1111/j.1469-8137.2005.01371.x
Gao, X., Li, Zhang, S., Zhang, Y., Fang, F., Li, M., et al. (2021). Spread and resistance level of Aegilops tauschii to mesosulfuron- methyl in winter wheat field of Shandong province. Acta Pratac. Sin. 54, 969–979.
Gao, X., Li, J., Zhang, S., Zhang, Y., Fang, F., Li, M., et al. (2021a). Spread and resistance level of Aegilops tauschii to mesosulfuronmethyl in winter wheat field of Shandong province. Sci. Agr. Sin. 54, 969–979. doi: 10.3864/j.issn.0578-1752.2021.05.009
Gao, X., Zhang, Y., An, C., Li, M., Li, J., Fang, F., et al. (2021b). Investigation and analysis of weed community successuion in winter wheat field of Shandong province. Sci. Agr. Sin. 54, 5230–5239. doi: 10.3864/j.issn.0578-1752.2021.24.006
GBIF.org (2022a) GBIF occurrence download. Available at: doi: 10.15468/dl.6btcq9 (Accessed May 1, 2022).
GBIF.org (2022b) GBIF occurrence download. Available at: doi: 10.15468/dl.cgytgb (Accessed August 3, 2022).
Gong, P. (2022). Wheat seed propagation and control of Aegilops tauschii cosson. Agric. Technol. Equip. 388, 131–132+135.
Guevara, L., Gerstner, B. E., Kass, J. M., Anderson, R. P. (2018). Toward ecologically realistic predictions of species distributions: A cross-time example from tropical montane cloud forests. Global Change Biol. 24, 1511–1522. doi: 10.1111/gcb.13992
Hansen, W. D., Braziunas, K. H., Rammer, W., Seidl, R., Turner, M. G. (2018). It takes a few to tango: changing climate and fire regimes can cause regeneration failure of two subalpine conifers. Ecology 99, 966–977. doi: 10.1002/ecy.2181
IPCC (2021). Climate change 2021: The physical science basis. contribution of working group I to the sixth assessment report of the intergovernmental panel on climate change (Cambridge: Cambridge University Press).
Irl, S. D. H., Schweiger, A. H., Steinbauer, M. J., Ah-Peng, C., Arévalo, J. R., Beierkuhnlein, C., et al. (2021). Human impact, climate and dispersal strategies determine plant invasion on islands. J. Biogeogr. 48, 1889–1903. doi: 10.1111/jbi.14119
Jing, G., Cheng, J., Su, J., Wei, L., Shi, X., Jin, J. (2015). Response of dominant population niche breadths and niche overlaps to various disturbance factors in typical steppe fenced grassland of china's loess plateau region. Acta Pratac. Sin. 24, 43–52. doi: 10.11686/cyxb2014410
Kass, J., Muscarella, R., Galante, P., Bohl, C., Pinilla-Buitrago, G., Boria, R., et al. (2021). ENMeval 2.0: Redesigned for customizable and reproducible modeling of species’ niches and distributions. Methods Ecol. Evol. 12, 1602–1608. doi: 10.1111/2041-210x.13628
Keller, J. A., Shea, K. (2021). Warming and shifting phenology accelerate an invasive plant life cycle. Ecology 102, e03219. doi: 10.1002/ecy.3219
Kueffer, C. (2017). Plant invasions in the anthropocene. Science 358, 724–725. doi: 10.1126/science.aao6371
Le Maitre, D. C., Kotzee, I. M., O’Farrell, P. J. (2014). Impacts of land-cover change on the water flow regulation ecosystem service: invasive alien plants, fire and their policy implications. Land Use Pol. 36, 171–181. doi: 10.1016/j.landusepol.2013.07.007
Levins, R. (1968). Evolution in changing environments some theoretical explorations. (MPB-2) (Princeton: Princeton University Press).
Lin, J., Qiang, S., Wu, H., Duan, H., Guo, H. (2004). Lolium temulentum l. Weed Sci. 3, 55–57. doi: 10.19588/j.issn.1003-935x.2004.03.021
Liu, W., Kang, L., Gao, B., Chen, D. (2010). Research advances on the detection of lolium content in flour. Tianjin Agr. Sci. 16, 137–140.
Lush, W. M. (1976). Leaf structure and translocation of dry matter in a C3 and a C4 grass. Planta 130, 235–244. doi: 10.1007/BF00387827
Manel, S., Williams, H. C., Ormerod, S. J. (2001). Evaluating presence–absence models in ecology: the need to account for prevalence. J. Appl. Ecol. 38, 921–931. doi: 10.1046/j.1365-2664.2001.00647.x
McKinney, M. L. (2001). Effects of human population, area, and time on non-native plant and fish diversity in the united states. Biol. Conserv. 100, 243–252. doi: 10.1016/S0006-3207(01)00027-1
Merow, C., Smith, M. J., Edwards, T. C., Jr., Guisan, A., McMahon, S. M., Normand, S., et al. (2014). What do we gain from simplicity versus complexity in species distribution models? Ecography 37, 1267–1281. doi: 10.1111/ecog.00845
Muscarella, R., Galante, P. J., Soley-Guardia, M., Boria, R. A., Kass, J. M., Uriarte, M., et al. (2014). ENMeval: an r package for conducting spatially independent evaluations and estimating optimal model complexity for MaxEnt ecological niche models. Methods Ecol. Evol. 5, 1198–1205. doi: 10.1111/2041-210X.12261
Peacock, J. M. (1975). Temperature and leaf growth in Lolium perenne. II. the site of temperature perception. J. Appl. Ecol. 12, 115–123. doi: 10.2307/2401721
Pejchar, L., Mooney, H. A. (2009). Invasive species, ecosystem services and human well-being. Trends Ecol. Evol. 24, 497–504. doi: 10.1016/j.tree.2009.03.016
Peterson, A., Soberón, J., Pearson, R., Anderson, R., Martínez-Meyer, E., Nakamura, M., et al. (2011). Ecological niches and geographic distributions (Princeton: Princeton University Press).
Petitpierre, B., Kueffer, C., Broennimann, O., Randin, C., Daehler, C., Guisan, A. (2012). Climatic niche shifts are rare among terrestrial plant invaders. Science 335, 1344–1348. doi: 10.1126/science.1215933
Phillips, S. J. (2008). Transferability, sample selection bias and background data in presence-only modelling: a response to Peterson et al., (2007). Ecography 31, 272–278. doi: 10.1111/j.0906-7590.2008.5378.x
Phillips, S. J., Anderson, R. P., Schapire, R. E. (2006). Maximum entropy modeling of species geographic distributions. Ecol. Modell. 190, 231–259. doi: 10.1016/j.ecolmodel.2005.03.026
Phillips, S. J., Dudík, M. (2008). Modeling of species distributions with MaxEnt: new extensions and a comprehensive evaluation. Ecography 31, 161–175. doi: 10.1111/j.0906-7590.2008.5203.x
Pyšek, P., Hulme, P. E., Simberloff, D., Bacher, S., Blackburn, T. M., Carlton, J. T., et al. (2020). Scientists' warning on invasive alien species. Biol. Rev. 95, 1511–1534. doi: 10.1111/brv.12627
Pyšek, P., Jarošík, V., Hulme, P. E., Kühn, I., Wild, J., Arianoutsou, M., et al. (2010). Disentangling the role of environmental and human pressures on biological invasions across Europe. P. Natl. Acad. Sci. U.S.A. 107, 12157–12162. doi: 10.1073/pnas.1002314107
Ratera, E. L. (1983). Bibliographic guide to plants toxic to stock in the Argentine republic. (Second contribution: grasses) Vol. 4 (Argentina: Universidad Católica Argentina), 38–42.
Rew, L. J., Brummer, T. J., Pollnac, F. W., Larson, C. D., Taylor, K. T., Taper, M. L., et al. (2018). Hitching a ride: seed accrual rates on different types of vehicles. J. Environ. Manage. 206, 547–555. doi: 10.1016/j.jenvman.2017.10.060
Roca, M., James, C., Pružinská, A., Hörtensteiner, S., Thomas, H., Ougham, H. (2004). Analysis of the chlorophyll catabolism pathway in leaves of an introgression senescence mutant of Lolium temulentum. Phytochemistry 65, 1231–1238. doi: 10.1016/j.phytochem.2004.03.022
Rockwell-Postel, M., Laginhas, B. B., Bradley, B. A. (2020). Supporting proactive management in the context of climate change: Prioritizing range-shifting invasive plants based on impact. Biol. Invasions 22, 2371–2383. doi: 10.1007/s10530-020-02261-1
Sagar, G. R., Holm, L. G., Pancho, J. V., Herberger, J. P., Plucknett, D. L. (1979). A geographical atlas of world weeds. J. Appl. Ecol. 18, 334. doi: 10.2307/2806777
Sarno, R., D'Alessandro, F., Vinciguerra, P. (1986). First results from a trial on the chemical control of weeds in sunflowers in Sicily. Inf. Agrar. 42, 51–57.
Schoener, T. W. (1968). The Anolis lizards of bimini: Resource partitioning in a complex fauna. Ecology 49, 704–726. doi: 10.2307/1935534
Sexton, J. P., Montiel, J., Shay, J. E., Stephens, M. R., Slatyer, R. A. (2017). Evolution of ecological niche breadth. Annu. Rev. Ecol. Evol. S. 48, 183–206. doi: 10.1146/annurev-ecolsys-110316-023003
Siller-Clavel, P., Badano, E. I., Villarreal-Guerrero, F., Prieto-Amparán, J. A., Pinedo-Alvarez, A., Corrales-Lerma, R., et al. (2022). Distribution patterns of invasive buffelgrass (Cenchrus ciliaris) in Mexico estimated with climate niche models under the current and future climate. Plants 11, 1160. doi: 10.3390/plants11091160
Sillero, N. (2011). What does ecological modelling model? a proposed classification of ecological niche models based on their underlying methods. Ecol. Modell. 222, 1343–1346. doi: 10.1016/j.ecolmodel.2011.01.018
Singh, M., Arunachalam, R., Kumar, L. (2021). Modeling potential hotspots of invasive Prosopis juliflora (Swartz) DC in India. Ecol. Inform. 64, 101386. doi: 10.1016/j.ecoinf.2021.101386
Steiner, A. M., Ruckenbauer, P. (1995). Germination of 110-year-old cereal and weed seeds, the Vienna sample of 1877. verification of effective ultra-dry storage at ambient temperature. Seed Sci. Res. 5, 195–199. doi: 10.1017/S0960258500002853
Stevens, P. F. E., Meyes, P. (1976). “Experience with metoxuron in wheat and barley,” in Proceedings of the Sixth East African Weed Science Conference, 38–48.
Swallen, J. R. (1961). The grasses of Burma, Ceylon, India and Pakistan (excluding bambuseae). n. l. bor. pergamon, new York 1960. xviii + 767 pp. illus. $25. Science 133, 1125–1125. doi: 10.1126/science.133.3459.1125.a
Swets, J. A. (1988). Measuring the accuracy of diagnostic systems. Science 240, 1285–1293. doi: 10.1126/science.3287615
Terreix, E. E. (1968). “A taxonomic revision of the genus lolium,” in Technical bulletin 1392 (United States: United States Department of Agriculture).
Thomas, H., Archer, J. E., Turley, R. M. (2011). Evolution, physiology and phytochemistry of the psychotoxic arable mimic weed darnel (Lolium temulentum l.). Prog. Bot. 72, 73–104. doi: 10.1007/978-3-642-13145-5_3
Tu, W., Xiong, Q., Qiu, X., Zhang, Y. (2021). Dynamics of invasive alien plant species in China under climate change scenarios. Ecol. Indic. 129, 107919. doi: 10.1016/j.ecolind.2021.107919
Vilà, M., Ibáñez, I. (2011). Plant invasions in the landscape. Landsc. Ecol. 26, 461–472. doi: 10.1007/s10980-011-9585-3
Wang, N., Wang, L., Chen, H. (2021). Waterlogging tolerance of the invasive plant Aegilops tauschii translates to increased competitiveness compared to Triticum aestivum. Acta Physiol. Plant 43, 57. doi: 10.1007/s11738-021-03230-4
Wang, W., Gao, S., Wang, S. (2019). Predictive studies of potential invasive areas for four poisonous weeds in gansu grassland. Acta Ecol. Sin. 39, 5301–5307. doi: 10.5846/stxb201809111946
Warren, D. L., Glor, R. E., Turelli, M. (2008). Environmental nicheI equivalency versus conservatism: quantitative approaches to niche evolution. Evolution 62, 2868–2883. doi: 10.1111/j.1558-5646.2008.00482.x
Warren, D. L., Seifert, S. N. (2011). Ecological niche modeling in MaxEnt: the importance of model complexity and the performance of model selection criteria. Ecol. Appl. 21, 335–342. doi: 10.1890/10-1171.1
Warren, D. L., Glor, R. E., Turelli, M. (2010). ENMTools: A toolbox for comparative studies of environmental niche models. Ecography 33, 607–611.
Wildlife Conservation Society, Center for International Earth Science Information Network (2005). Data from: Last of the wild project, version 2, 2005 (LWP-2): Global human influence index (HII) dataset (geographic) (Palisades, New York: NASA Socioeconomic Data and Applications Center (SEDAC). Available at: https://sedac.ciesin.columbia.edu/.
Wu, R., Xu., H., Sun, L., Su, W., Wei, H., Xue, F., et al. (2022). Control effect of the mixture of mesosulfuron⁃methyl and pinoxaden on aegilops tauschii and Lolium multiflorum in wheat field. J. Henan Agr. Sci. 51, 103–110. doi: 10.15933/j.cnki.1004⁃3268.2022.06.011
Xian, X., Zhao, H., Wang, R., Zhang, H., Chen, B., Huang, H., et al. (2022). Predicting the potential geographical distribution of Ageratina adenophora in China using equilibrium occurrence data and ensemble model. Front. Ecol. Evol. 10. doi: 10.3389/fevo.2022.9733
Xu, H., Ding, H., Li, M., Qiang, S., Guo, J., Han, Z., et al. (2006). The distribution and economic losses of alien species invasion to China. Biol. Invasions 8, 1495–1500. doi: 10.1007/s10530-005-5841-2
Yan, X., Shou, H., Ma, J. (2012). The problem and status of the alien invasive plants in China. Plant Divers. 34, 287–313. doi: 10.3724/SP.J.1143.2012.12025
Yu, H.-Y., Li, X.-J. (2018). Distribution of Aegilops tauschii Coss. in China and its research progress. Weed Sci. 36, 1–7. doi: 10.19588/j.issn.1003-935X.2018.01.001
Zhang, A., Hu, X., Yao, S., Yu, M., Ying, Z. (2021a). Alien, naturalized and invasive plants in China. Plants 10, 2241. doi: 10.3390/plants10112241
Zhang, W. P. (1998). China's biodiversity: A country study (Beijing: China Environmental Science Press).
Zhang, X., Wang, Y., Peng, P., Wang, G., Zhao, G., Zhou, Y., et al. (2022). Mapping the distribution and dispersal risks of the alien invasive plant Ageratina adenophora in China. Diversity 14, 915. doi: 10.3390/d14110915
Zhang, Y., Tang, J., Ren, G., Zhao, K., Wang, X. (2021b). Global potential distribution prediction of Xanthium italicum based on maxent model. Sci. Rep. 11, 16545. doi: 10.1038/s41598-021-96041-z
Keywords: climate change, distribution patterns, Lolium temulentum, Aegilops tauschii, overlap area, maximum entropy
Citation: Yang M, Zhao H, Xian X, Wang R, Yang N, Chen L and Liu W-x (2023) Assessing risk from invasive alien plants in China: Reconstructing invasion history and estimating distribution patterns of Lolium temulentum and Aegilops tauschii. Front. Plant Sci. 14:1113567. doi: 10.3389/fpls.2023.1113567
Received: 01 December 2022; Accepted: 25 January 2023;
Published: 02 February 2023.
Edited by:
Shen Shicai, Yunnan Academy of Agricultural Sciences, ChinaReviewed by:
Lei Wang, Xinjiang Institute of Ecology and Geography, Chinese Academy of Sciences (CAS), ChinaWei Zhang, Southwest Forestry University, China
Copyright © 2023 Yang, Zhao, Xian, Wang, Yang, Chen and Liu. This is an open-access article distributed under the terms of the Creative Commons Attribution License (CC BY). The use, distribution or reproduction in other forums is permitted, provided the original author(s) and the copyright owner(s) are credited and that the original publication in this journal is cited, in accordance with accepted academic practice. No use, distribution or reproduction is permitted which does not comply with these terms.
*Correspondence: Wan-xue Liu, bGl1d2FueHVlQGNhYXMuY24=
†These authors have contributed equally to this work