- 1Key Laboratory of Ecohydrology of Inland River Basin, Northwest Institute of Eco-Environment and Resources, Chinese Academy of Sciences, Lanzhou, China
- 2University of Chinese Academy of Sciences, Beijing, China
- 3College of Geography and Environmental Science, Northwest Normal University, Lanzhou, China
- 4School of Environmental Sciences, University of Guelph, Guelph, ON, Canada
- 5Qilian Mountains Eco-Environment Research Center in Gansu Province, Lanzhou, China
- 6Key Laboratory of Land Surface Process and Climate Change in Cold and Arid Regions, Northwest Institute of Eco-Environment and Resources, Chinese Academy of Sciences, Lanzhou, China
Plants adapt to changes in elevation by regulating their leaf ecological stoichiometry. Potentilla anserina L. that grows rapidly under poor or even bare soil conditions has become an important ground cover plant for ecological restoration. However, its leaf ecological stoichiometry has been given little attention, resulting in an insufficient understanding of its environmental adaptability and growth strategies. The objective of this study was to compare the leaf stoichiometry of P. anserina at different elevations (2,400, 2,600, 2,800, 3,000, 3,200, 3,500, and 3,800 m) in the middle eastern part of Qilian Mountains. With an increase in elevation, leaf carbon concentration [(C)leaf] significantly decreased, with the maximum value of 446.04 g·kg−1 (2,400 m) and the minimum value of 396.78 g·kg−1 (3,500 m). Leaf nitrogen concentration [(N)leaf] also increased with an increase in elevation, and its maximum and minimum values were 37.57 g·kg−1 (3,500 m) and 23.71 g·kg−1 (2,800 m), respectively. Leaf phosphorus concentration [(P)leaf] was the highest (2.79 g·kg−1) at 2,400 m and the lowest (0.91 g·kg−1) at 2,800 m. The [C]leaf/[N]leaf decreased with an increase in elevation, while [N]leaf/[P]leaf showed an opposite trend. The mean annual temperature, mean annual precipitation, soil pH, organic carbon, nitrogen, and phosphorus at different elevations mainly affected [C]leaf, [N]leaf, and [P]leaf. The growth of P. anserina in the study area was mainly limited by P, and this limitation was stronger with increased elevation. Progressively reducing P loss at high elevation is of great significance to the survival of P. anserina in this specific region.
Introduction
Ecological stoichiometry focuses on the balance of multiple elements in ecological interactions and processes, such as energy flow and nutrient cycling, and has become an important topic of research in recent years in ecology and biology (Moe et al., 2005; Sistla and Schimel, 2012; Yang et al., 2019; Zhu et al., 2020). It is an important tool to reveal organisms' responses to external disturbances and nutrient supply balance mechanisms in ecosystems (Zeng et al., 2016), mainly by analyzing changes in carbon (C), nitrogen (N), and phosphorus (P) (Cao et al., 2020), which are well-known as basic elements constituting plants and are closely linked to plant photosynthesis, respiration, and various ecosystem functions (Elser et al., 2010; Marschner and Marschner, 2012; Wang et al., 2015; Yan et al., 2016; Croft et al., 2017).
Leaves are the most active and primary photosynthetic plant organ (Elser et al., 2010; Yang et al., 2019), their size and structure exhibit a tradeoff between the support cost and photosynthetic returns during plant adaptation to environmental changes (Shi et al., 2020, 2022; Guo et al., 2021; Li et al., 2022a,b), and leaf stoichiometry can reflect the tradeoff formed in this evolution from the angle of leaf chemical elements and its spatio-temporal variations (Baxter and Dilkes, 2012; Cao et al., 2020; Zhu et al., 2020). Leaf C/N and C/P were widely accepted as effective indicators of plants' N and P use efficiency and growth rate, and their lower values indicated lower nutrient utilization efficiency and higher plant growth (Weidner et al., 2015; Sun et al., 2017; Cao et al., 2020; Zhang et al., 2020). Leaf N/P can reflect plant nutrient states and limitations (Tao et al., 2016). However, there remain questions about the N/P thresholds assessing plants' nutrient limitation during growth due to multiple factors (Crowley et al., 2012). For example, according to the study of Koerselman and Meuleman (1996) on wetland plant communities, when leaf N/P < 14, plant growth was limited by N; 14 ≤ leaf N/P ≤ 16, plant growth has the common limit of N and P; and leaf N/P > 16, plant growth was limited by P, while the study of Güsewell (2004) on terrestrial plant communities found that when leaf N/P < 10, plant growth was limited by N; 10 ≤ leaf N/P ≤ 20, plant growth has the common limit of N and P; and leaf N/P > 20, plant growth was limited by P. Nevertheless, leaf N/P is still considered to be a valuable tool for assessing potential patterns in nutrient limitation across broad landscapes (Crowley et al., 2012).
Relationships between leaf stoichiometry and environmental factors, including soil nutrients and geographical and climatic factors, were widely explored at various scales including regional and global scales (e.g., McGroddy et al., 2004; Reich and Oleksyn, 2004; Sardans et al., 2011; Du et al., 2017; Tian et al., 2018; Qin et al., 2021). Elevation is a crucial factor and can affect plant leaf stoichiometry by altering the combination of heat and water, soil properties, and vegetation community composition (Bo et al., 2020; Liu et al., 2021). However, there is little consensus on leaf stoichiometric characteristics as they change along an elevation gradient. Some studies observed that leaf C concentration [(C)leaf] increased but leaf N [(N)leaf] and leaf P concentration [(P)leaf] decreased with an increase in elevation (e.g., Zhao et al., 2014, 2018; Bo et al., 2020), while other studies reported an opposite trend (e.g., Macek et al., 2009; Li et al., 2018). Therefore, the response of plant leaf ecological stoichiometry to elevation should be investigated at regional and species-specific levels. Situated in the transition zone between the Qinghai–Tibetan Plateau and the arid region of northwestern China, the Qilian Mountains preserve a wide variety of plant species (Gui et al., 2020; Wang et al., 2022) and serve as an ideal region to study the impact of elevation on leaf stoichiometry of different plant species (Cao et al., 2020; Qin et al., 2021). Potentilla anserina L., a typical forb, is widely distributed in the Qilian Mountains. Due to its wide ecological amplitude and vegetative reproduction ability, P. anserina is an important species of degraded grasslands or secondary succession lands and is considered a prime species for ecological restoration in alpine regions (Sheng et al., 2004). Previous studies mainly focused on P. anserina's chemical components (Zhao et al., 2020), pharmacological action (He et al., 2021), nutrient reabsorption (Li et al., 2020), and medicinal value (Cheng J. et al., 2021); information on its leaf stoichiometry remains unavailable. Exploring the leaf stoichiometry and growth nutrient limitation of P. anserina is of great significance for the ecological restoration and construction in the Qilian Mountains. It was found that the leaf stoichiometry of many dominant or common species in the Qilian Mountains was affected by elevation. For example, with increasing elevation, [C]leaf of Oxytropis ochrocephala first increased and then decreased, while [N]leaf and [P]leaf of the same species showed a trend of general increase in the identified soil organic carbon (SOC), the ratio of SOC to soil total phosphorus (STP), and the mean annual temperature (MAT) as dominant factors (Cao et al., 2020). [C]leaf and [P]leaf of Potentilla fruticosa first decreased and then increased with an increase in elevation, and SOC, STP, and soil pH were the main factors influencing the leaf stoichiometry (Qin et al., 2022). We hypothesized that elevation may also significantly influence leaf stoichiometry of P. anserina and leaf stoichiometry of P. anserina could be influenced by soil nutrients, MAT, and MAP. Previous studies have proposed that the soil P content of the Qilian Mountains was relatively low (Xu et al., 2018, 2019). Therefore, we hypothesized that P could be a limiting nutrient for P. anserina growth in this region.
This study attempted to: (i) explore how P. anserina changed leaf C/N/P stoichiometry to adapt to environmental changes along elevation (2,400–3,800 m) in the Qilian Mountains, (ii) identify the underlying mechanism of the effect of elevation on leaf C/N/P stoichiometry by building structural equation models, and (iii) determine the key nutrients limiting the growth of P. anserina in the Qilian Mountains.
Materials and methods
Study area
A field study was conducted at the Dayekou River Basin and the Haibei Alpine Meadow Ecosystem Research Station in the Middle East of the Qilian Mountains (93°30′-103°30′ E, 36°30′-39°30′ N). The Qilian Mountains ranging from 2,000 to 5,500 m elevation belong to a typical plateau continental climate, with MAT and MAP of −0.4°C and 405 mm, respectively, from 2000 to 2015. Grasslands with Carex tristachya, Stipa przewalskii, Leymus secalinus, and Polygonum viviparum dominate the southern slopes, while Qinghai spruce (Picea crassifolia Kom.) forests dominate the northern slopes. Soils were classified as Haplic Kastanozems and Haplic Phaeozems on the southern and northern slopes, respectively (IUSS Working Group World Reference Base for Soil Resources, 2014).
Field sampling
In August and September 2018, samples of healthy fresh leaves of P. anserina were collected from the following seven elevations: 2,400, 2,600, 2,800, 3,000, 3,200, 3,500, and 3,800 m (Figure 1). Sampling at 2,400, 2,600, 2,800, and 3,000 m was conducted at the Dayekou River Basin, while sampling at 3,200, 3,500, and 3,800 m was conducted at the Haibei Alpine Meadow Ecosystem Research Station due to their distribution as well as accessibility of the sites. At each elevation, leaves were collected from three random plots (10 × 10 m) and then packed in paper bags. All the sampling plots were on the southern slopes with slope gradients between 27° and 33°. In each plot, three quadrats (1 × 1 m) were positioned evenly along a diagonal line. Soil samples from 0 to 0.10, 0.10 to 0.20, and 0.20 to 0.40 m were collected by using a 100-mm-diameter soil auger (Cao et al., 2020). A total of 21 sampling plots (seven elevations × three plots) and 63 sample quadrats (21 plots × three quadrats in each plot) were investigated.
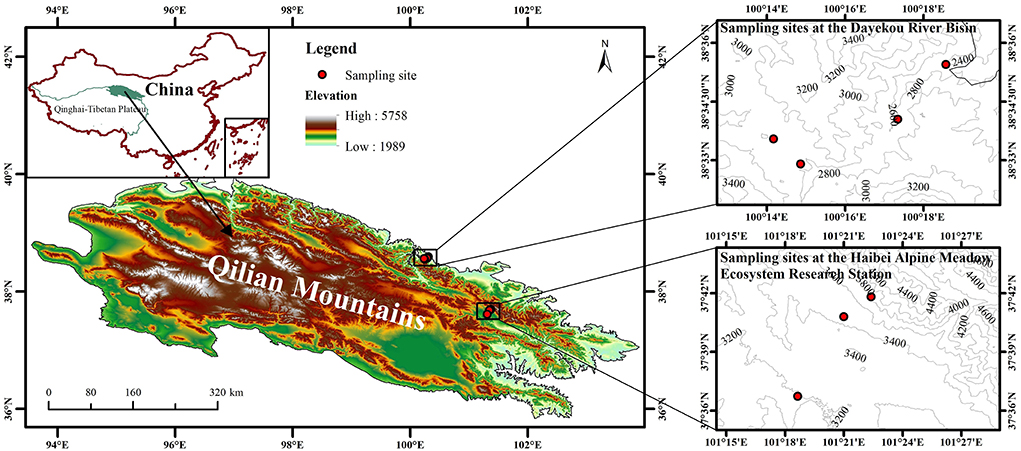
Figure 1. Sampling sites across elevations (2,400, 2,600, 2,800, 3,000, 3,200, 3,500, and 3,800 m) in the study area.
Leaf and soil analyses
The leaves were firstly oven-dried at 80°C for 24 h and then ground to determine [C]leaf, [N]leaf, and [P]leaf. Soil samples were air-dried and divided into two parts: One part was ground and sieved through a 100-mesh sieve for SOC, soil total nitrogen (STN), and STP determination, and the other part was ground and sieved through an 8-mesh sieve for soil pH determination. [C]leaf (g kg−1) and SOC (g kg−1), [N]leaf (g kg−1) and STN (g kg−1), and [P]leaf (g kg−1) and STP (g kg−1) were measured using the potassium bichromate titrimetric method (Nelson and Sommers, 1982), the Kjeldahl method (Bremmer and Mulvaney, 1982), and the molybdate blue method (Olsen and Sommers, 1982), respectively. Soil pH was measured by the potentiometric method with a soil–water ratio of 2:5.
Data analysis
MAT and MAP (Table 1) were calculated according to Zhao et al. (2005, 2006) as follows:
where ELEV is the elevation, LAT is the latitude, LONG is the longitude, and R2 is the regression coefficient.
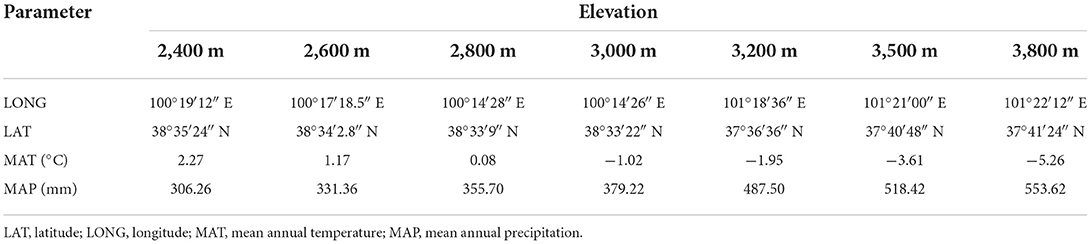
Table 1. Sampling site coordinates, mean annual temperature (MAT), and precipitation (MAP) in the study area.
All data were described by their average value and standard error (SE). Soil properties in the 0–0.40 m soil layer were expressed as an average of values in the 0–0.10, 0.10–0.20, and 0.20–0.40 m soil layers. The fixed effect (elevation) and random effect (experimental plots) on leaf stoichiometry of P. anserina and soil properties were tested by fitting linear mixed models (LMMs) in R3.3.1 (nlme vegan). The differences in soil properties among elevations were detected using the one-way ANOVA, followed by the least significant difference test to perform the significance analysis at P < 0.05 in SPSS 22.0 (SPSS Inc., Chicago, IL, USA). Pearson's correlation analysis was conducted to explore the correlations between the leaf stoichiometry of P. anserina and the topography-induced climatic factors and soil properties in SPSS 22.0. Path analysis was used in the structural equation modeling (SEM) to evaluate the direct and indirect effects of soil properties on leaf stoichiometry of P. anserina in Amos 22.0 (IBM SPSS Inc., Chicago, IL, USA).
Results
Variations in leaf stoichiometry of Potentilla anserina and soil properties with elevation
The results of LMMs showed that leaf stoichiometries of P. anserina and soil properties were significantly affected by elevation (Table 2). With increasing elevation, [C]leaf showed a generally decreasing trend (Figure 2A) and reached its maximum and minimum values at 2,400 m (446.04 ± 8.17 g kg−1) and 3,500 m (396.78 ± 7.38 g kg−1), respectively (Table 3). The [N]leaf showed a generally increasing trend (Figure 2B), with its maximum and minimum values at 3,500 m (37.57 ± 0.64 g kg−1) and 2,800 m (23.71 ± 0.40 g kg−1), respectively (Table 3). The [P]leaf decreased from 2,400 to 2,600 m elevation and reached the maximum and minimum values at 2,400 (2.79 ± 0.69 g kg−1) and 3,800 m (0.97 ± 0.72 g kg−1), respectively (Figure 2C, Table 3). However, there was no difference in [P]leaf at different elevations above 2,600 m (Table 3). In contrast to [N]leaf, the [C]leaf/[N]leaf showed a generally decreasing trend with an increase in elevation (Figure 2D), with a peak at 2,800 m (17.69 ± 0.46), and then reduced at 3,500 m (10.57 ± 0.27) (Table 3). The maximum and minimum values of [C]leaf/[P]leaf were 842.36 ± 1,031.11 at 3,800 m and 173.03 ± 62.38 at 2,400 m, respectively. The [N]leaf/[P]leaf showed a generally increasing trend with an increase in elevation (Figure 2F) and reached its maximum and minimum values at 3,800 m (69.40 ± 86.26) and at 2,400 m (10.05 ± 3.53), respectively (Table 3).
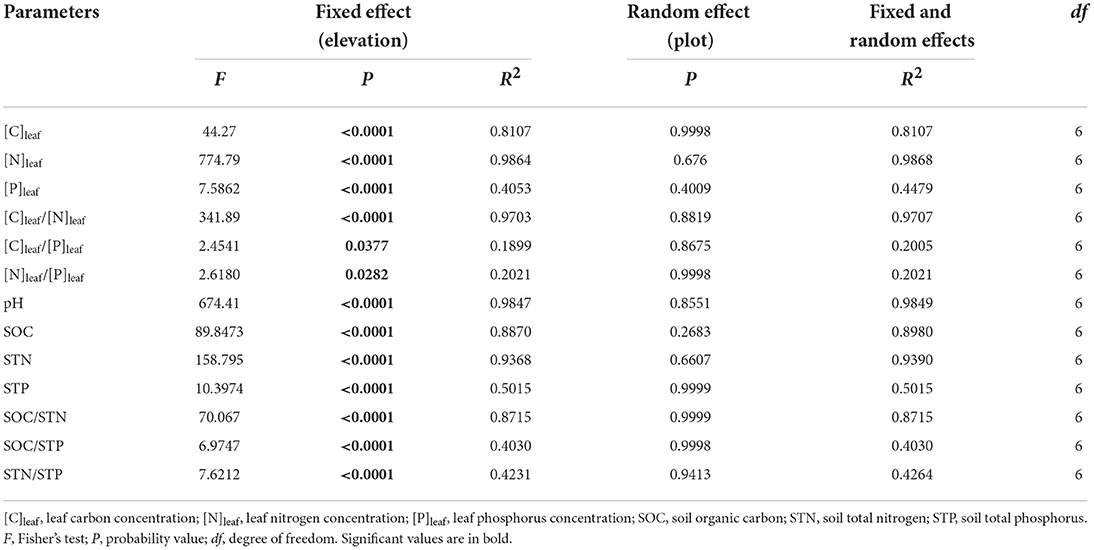
Table 2. Summary of the generalized linear mixed models for leaf stoichiometry and soil properties (0–0.40 m) (n = 9).
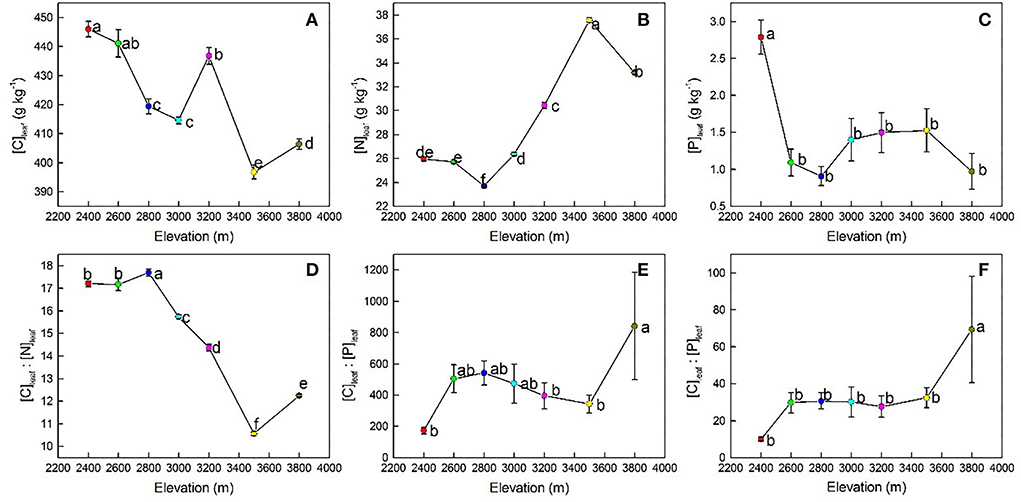
Figure 2. Changes in Potentilla anserina's [C]leaf (A), [N]leaf (B), [P]leaf (C), and their ratios (D–F) with an increase in elevation. [C]leaf, leaf carbon concentration; [N]leaf, leaf nitrogen concentration; [P]leaf, leaf phosphorus concentration. Different lowercase letters indicate a significant difference in leaf stoichiometry and soil properties among elevations (P < 0.05).
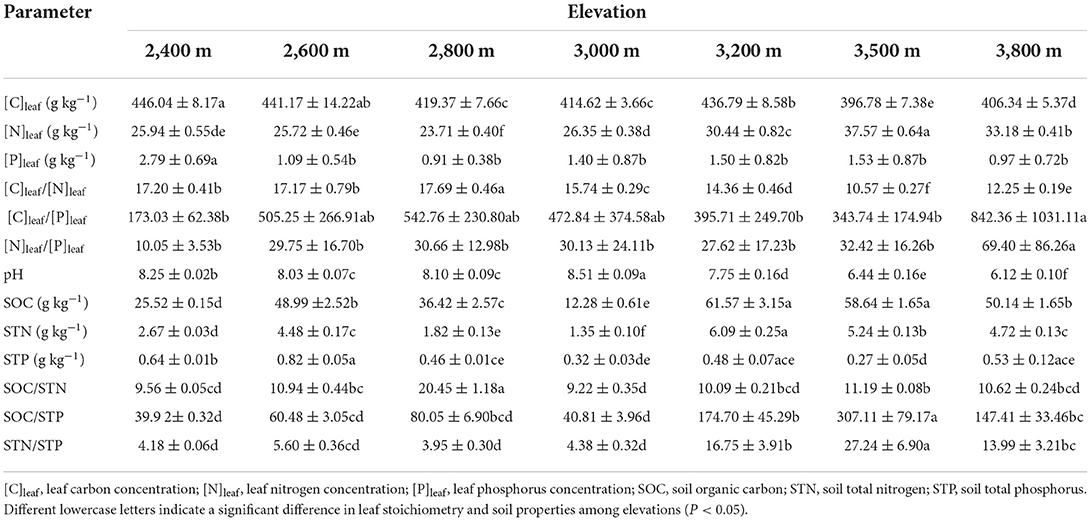
Table 3. Variation of leaf stoichiometry of Potentilla anserina and soil properties (0–0.40 m) across elevations (mean ± standard error, n = 9).
For soil properties, SOC and STN showed similar trends with their maximum values at 3,200 m (61.57 ± 3.15 g kg−1 and 6.09 ± 0.25 g kg−1, respectively), and minimum values at 3,000 m (12.28 ± 0.61 g kg−1 and 1.35 ± 0.10 g kg−1, respectively). The maximum and minimum values of STP were observed at 2,600 m (0.82 ± 0.05 g kg−1) and 3,500 m (0.27 ± 0.05 g kg−1), respectively. The maximum value of SOC/STN was observed at 2,800 m (20.45 ± 1.18), which was about two times that at other elevations. The values of SOC/STP and STN/STP at elevations ≥ 3,200 m were about two to seven times greater than those at elevations < 3,200 m. Soil pH at 3,000 m (8.51 ± 0.09) was the highest, while at 3,800 m (6.12 ± 0.10) it was the lowest.
Environmental factors influencing leaf ecological stoichiometry of Potentilla anserina
The SEM showed that the climatic and soil factors accounted for about 70, 91, 21, and 87% of the total variation in [C]leaf, [N]leaf, [P]leaf, and [C]leaf/[N]leaf, respectively (Figure 3). Specifically, MAT had direct effects on [C]leaf, [N]leaf, and [P]leaf and an indirect effect on [P]leaf which may be from the positive influence on soil pH and negative influence on SOC (Figures 3A–C). MAP exerted direct effects on [N]leaf and [C]leaf/[N]leaf and indirect effects on [C]leaf, [N]leaf, and [C]leaf/[N]leaf by influencing soil pH, STN, and STP (Figures 3A,B,D).
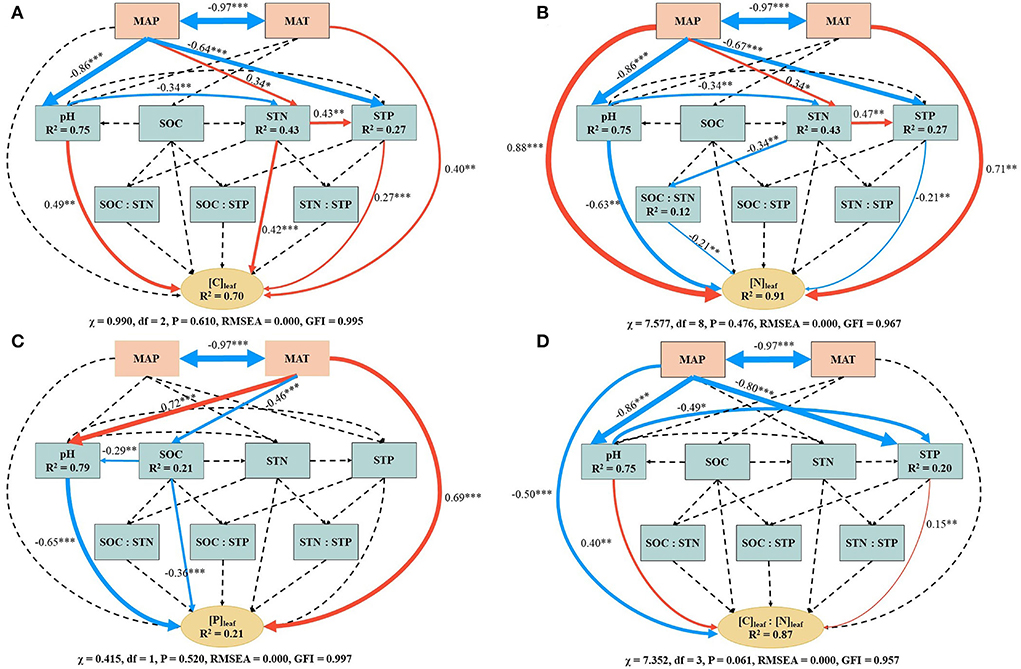
Figure 3. Structural equation modeling (SEM) examining the climatic and soil effects on leaf carbon concentration (A), leaf nitrogen concentration (B), leaf phosphorus concentration (C), and the ratio of leaf carbon concentration and leaf nitrogen concentration (D). [C]leaf, leaf carbon concentration; [N]leaf, leaf nitrogen concentration; [P]leaf, leaf phosphorus concentration; SOC, soil organic carbon; STN, soil total nitrogen; STP, soil total phosphorus; MAT, mean annual temperature; MAP, mean annual precipitation. Single- and double-headed arrows represent the hypothesized causal direction and covarying variables, respectively. Continuous and dashed arrows indicate the relationships with (*P < 0.05, **P < 0.01, ***P < 0.001) and without statistical significance (P > 0.05), respectively. Numbers next to continuous arrows are standardized path coefficients, which indicate the effect size of the relationship. Red and blue arrows indicate the positive and negative relationships, respectively, and arrow width is scaled proportionally to their effect size. R2 below variables indicates the proportion of variance explained.
Soil pH and STP, which were both affected by MAP, exerted a direct positive effect on [C]leaf and [C]leaf/[N]leaf and a negative effect on [N]leaf (Figures 3A,B,D). Soil pH also had a strong effect on [P]leaf (Figure 3C). The STN had not only a direct effect on [C]leaf, but also an indirect effect as it positively influenced STP (Figure 3A). STN also indirectly affected [N]leaf by negatively influencing SOC/STN and positively influencing STP (Figure 3B). The SOC had a direct negative effect on [P]leaf and an indirect effect on [P]leaf by negatively influencing soil pH (Figure 3C).
The SEM was not applicable for [C]leaf/[P]leaf and [N]leaf/[P]leaf because their models did not meet the requirement of P-values (P > 0.05). In this case, Pearson's correlation analysis was used to show their relationships with the environmental factors. Pearson's correlation analysis showed that the [C]leaf/[P]leaf was only negatively correlated with MAT (r = −0.250, P < 0.05) and [N]leaf/[P]leaf was positively correlated with MAP (r = 0.315, P < 0.05), but negatively correlated with MAT (r = −0.360, P < 0.01) and soil pH (r = −0.325, P < 0.01; Table 4).

Table 4. Pearson's correlations between environmental factors and leaf carbon-to-phosphorus ratio and the nitrogen-to-phosphorus ratio of Potentilla anserina.
Discussion
Effects of elevation on leaf ecological stoichiometry of Potentilla anserina and soil properties
The leaf stoichiometry of P. anserina changed due to heterogeneous habitat conditions with increased elevation. This agrees with the observations of van de Weg et al. (2009) and Hu et al. (2020) and supports our hypothesis that elevation would significantly influence leaf stoichiometry of P. anserina. However, Qin et al. (2022) found that elevation only significantly affected [C]leaf, [N]leaf, and [C]leaf/[N]leaf of Potentilla fruticosa, while Su et al. (2022) reported that elevation was the key factor regulating the leaf stoichiometries of Ligularia virgaurea except [P]leaf and [N]leaf/[P]leaf in the Qilian Mountains. This suggests diverse adaptation strategies of different species to environmental changes.
The [C]leaf of P. anserina showed a generally decreasing trend with an increase in elevation (Figure 2A), which is consistent with the study of Li et al. (2018) and Qin et al. (2022). This may be because photosynthesis was inhibited when the temperature dropped with an increase in elevation, which in turn weakened their carbon assimilation ability (Öquist, 1983). However, some studies (e.g., Zhao et al., 2018; Bo et al., 2020; Waigwa et al., 2020) reported that [C]leaf tended to be greater at higher elevations to balance cell osmotic pressure and improve the frost resistance of plants (Zhao et al., 2018). These conflicting results suggest that the response of [C]leaf to elevation still requires further studies based on species and at the regional level. The [N]leaf is an important indicator of plant adaptation to environmental changes (Li et al., 2014). It showed a generally increasing trend with an increase in elevation (Figure 2B), and this agrees with the observations of Cao et al. (2020). This may be because P. anserina could increase [N]leaf to offset the metabolic slowdown caused by a reduced enzymatic activity at low temperatures (McGroddy et al., 2004). The [P]leaf of P. anserina showed a significantly decreasing trend with an increase in elevation (Figure 2C), and this is in line with the observations of Waigwa et al. (2020). In the study area, the variation of P. anserina's [N]leaf was consistent with the temperature–plant physiological hypothesis, while that of P. anserina's [P]leaf was in contrast to this hypothesis. This may be because of the decoupling of N and P in plants under global changes (Yuan and Chen, 2015). Therefore, it is necessary to conduct targeted research on the mechanisms for their changes with elevation separately. The [C]leaf/[N]leaf and [C]leaf/[P]leaf reflected the nutrient utilization efficiency, and a lower value indicated a higher plant growth rate and a lower N utilization efficiency (Elser et al., 2003; Weidner et al., 2015; Sun et al., 2017). In the Qilian Mountains, [C]leaf/[N]leaf of P. anserina decreased with elevation, consistent with the study of Zhang Q. et al. (2019) and Zhang Y. et al. (2019), but contrary to the study of Hu et al. (2020), indicating that the growth rate of P. anserina increased but N use efficiency decreased with elevation (Guo et al., 2016; Zhang et al., 2020). This was consistent with the adaptive growth hypothesis that under N-limited environments, plants adopt a survival-first strategy and maintain higher C/N to increase N use efficiency and ensure survival, while under less N-limited environments, plants adopt a growth-first strategy and maintain lower C/N to keep higher growth rates (Zhang et al., 2020). In the study area, compared with lower elevations (i.e., 2,400–3,000 m), STN was relatively higher at higher elevations (i.e., 3,200–3,800 m) (Table 3), resulting in a less N-limited condition. Therefore, [C]leaf/[N]leaf of P. anserina decreased at higher elevations to benefit growth priority. The [N]leaf/[P]leaf of P. anserina increased with elevation, similar to the study of Cao et al. (2020) on Oxytropis ochrocephala and the study of Wang et al. (2019) on Sabina przewalskii in the Qilian Mountains, while Su et al. (2022) found no significant changes in Ligularia virgaurea's [N]leaf/[P]leaf across elevation in the Qilian Mountains. The increasing [N]leaf/[P]leaf of P. anserina with elevation indicates that the growth of P. anserina in the study area may be more susceptible to P-limitation at higher elevations.
In the study area, soil properties differ significantly with elevation (Tables 1, 2). Similar results were reported by Cao et al. (2020) and Niu et al. (2021), who found contrasting soil nutrient contents with elevation in the Qilian Mountains. The differences in soil properties may be from the variations in solar radiation, temperature, and precipitation at different elevations (Qin et al., 2022). Zonal climatic conditions could change soil nutrient flux and soil nutrient allocation by affecting vegetation composition, litter quality, and the exchange of matter and energy between the soil and the environment (Jiang et al., 2019). Likewise, under the combined influence of various factors, soil pH and SOC also changed with elevation (Yu et al., 2019; Zhu et al., 2019).
Mechanisms of elevation regulating leaf ecological stoichiometry of Potentilla anserina
As shown above, [C]leaf, [N]leaf, [P]leaf, and their ratios of P. anserina showed inconsistent responses to elevation (Table 3, Figure 2), similar to the study of Müller et al. (2017) and Tong et al. (2021), indicating that the mechanisms of elevation regulating leaf ecological stoichiometry of P. anserina were different. In order to figure out how elevation affects leaf ecological stoichiometry, the underlying mechanisms of the variation in each element under elevation need to be explored individually (Weiher and Keddy, 1999; Suding et al., 2008).
Mechanisms of elevation regulating [C]leaf of Potentilla anserina
Temperature plays a key role in plant functions by influencing enzyme activity and membrane system fluidity as well as changing the absorption of nutrients and water and thus, in turn, affects the leaf ecological stoichiometry (Reich and Oleksyn, 2004; Hall et al., 2010; Liu et al., 2019). In the present study, MAT had a direct positive effect on [C]leaf (Figure 3A), similar to the study of Fang et al. (2019). Previous researchers found that when MAT declined and photosynthesis weakened, plants increased leaf thickness and reduced leaf area in order to prevent freezing damage, finally leading to a decrease in [C]leaf (Park and Day, 2007). With increasing elevation, the increased MAP can increase [C]leaf by impacting soil pH, STN, and STP (Figure 3A).
The STN and STP exerted positive effects on [C]leaf because high STN and STP were more conducive to the synthesis of enzymes that play key roles in carbon assimilation and accumulation (Zhang Q. et al., 2019). Soil pH exerted a positive influence on [C]leaf, consistent with the study of Liu M. et al. (2021). However, most previous studies showed that higher soil pH had a significant negative impact on [C]leaf as it inhibits photosynthesis (e.g., He et al., 2016; Gong et al., 2018; Lin et al., 2022; Su et al., 2022). These results indicated that the impact of soil pH on [C]leaf was significant but varied with species.
Mechanisms of elevation regulating [N]leaf of Potentilla anserina
Elevation regulated [N]leaf by changing MAT, MAP, soil pH, SOC/STN, STN, and STP (Figure 3B). Persson et al. (2012) found that lower MAT could reduce [N]leaf by limiting the nutrient turnover rates. In the present study, MAT had a positive impact on [N]leaf (Figure 3B), consistent with the study of Li et al. (2021). However, with the decrease in MAT, [N]leaf showed a generally increasing trend (Table 3). This may be because the effect of MAT was weaker than the combined effects of MAP and soil physicochemical properties on [N]leaf. In general, MAP changed plant nutrient absorption and photosynthesis by affecting soil water content and soil nutrient availability (Han et al., 2011; Liu et al., 2019). In this study, MAP affected [N]leaf either by directly changing its concentration, or by indirectly changing soil properties, further proved by the research of Reich (2005). MAP had a direct positive effect on [N]leaf, similar to the research of Sardans et al. (2005) and Chen Y. H. et al. (2013). This may be because, with the increase in MAP, the increase in soil water content could improve the plant photosynthetic rate and sucrose synthase and nitrate reductase activities, thus resulting in increased [N]leaf (Patrick et al., 2009; Wang et al., 2011).
Decreased soil pH could promote the reabsorption of nitrogen to maintain plant growth, leading to increased [N]leaf (Su et al., 2022). Haynes (1986) found that when SOC/STN was ≤ 25, soil nitrogen was mineralized as available nitrogen for plant growth, while when it was > 25, microorganisms mineralized soil nitrogen for maintaining their own growth. In the present study, the SOC/STN was all < 25, suggesting that more soil available nitrogen was taken up by plants and increased [N]leaf. However, this needs further research based on soil available nitrogen analysis. In addition, STP exerted a negative effect on [N]leaf. This might be because the decreased STP reduced the availability of P and relatively weakened the limiting effect of available N on plants, thereby enhancing the competition of plants for available N and promoting the uptake and utilization of N by an individual plant (Li et al., 2017). However, some studies found that decreased STP could reduce [N]leaf (e.g., van Wijk et al., 2003; Mao et al., 2016). This was related to different responses of plant species to environmental changes due to their differentiation of niches based on functional attributes (Pontes et al., 2010; Adamidis et al., 2014; Li et al., 2017).
Mechanisms of elevation regulating [P]leaf of Potentilla anserina
MAT had a positive effect on [P]leaf (Figure 3C). This may be because the decrease in MAT restricted soil parent weathering, which led to a decrease in soil P absorption by plants (Tong et al., 2021). Besides, the decreased MAT can indirectly influence [P]leaf through decreasing soil pH and increasing SOC with an increase in elevation. Soil pH had a direct negative impact on [P]leaf. This may be because the decrease in soil pH could release phosphate radicals and increase soil available P, thereby improving the uptake of P and [P]leaf (Chen D. M. et al., 2013), while high soil pH may facilitate P adsorption to soil particles (Qiao et al., 2018).
The SOC had a negative effect on [P]leaf (Figure 3C), contrary to what was observed by Liu et al. (2016) and Cao et al. (2020), suggesting that plants have their unique nutrient absorption mechanism in the processes of growth. The utilization of SOC by plants was extremely complex and may be controlled by a variety of soil physical and chemical properties (Pan et al., 2015; Rong et al., 2015).
Mechanisms of elevation regulating [C]leaf/[N]leaf/[P]leaf of Potentilla anserina
According to the SEM, decreased [C]leaf/[N]leaf with an increase in elevation resulted from the positive impacts of soil pH and STP and the negative impact of MAP (Figure 3D). This suggests that the increase in soil pH and STP is beneficial to promote the growth of P. anserina within a certain range, while the increase in precipitation may inhibit the growth of P. anserina in the study area. The [C]leaf/[P]leaf at 3,800 m was significantly higher than that at 2,400, 3,200, and 3,500 m, similar to the study of Qin et al. (2022). However, there was no clear variation trend of [C]leaf/[P]leaf with elevation (Figure 2E). This may be because the similar variations of [C]leaf and [P]leaf with elevation weakened the relationship between [C]leaf/[P]leaf and elevation. MAT was negatively correlated with [N]leaf/[P]leaf (Table 4), which might be attributed to the positive effect of MAT on [P]leaf (Figure 3C), though MAT had a positive influence on [N]leaf. This indicated a stronger influence of MAT on [P]leaf than on [N]leaf. The positive relationship between MAP and [N]leaf/[P]leaf (Table 4) may be related to the positive effects of MAP on [N]leaf (Figure 3B). However, the significantly increasing [N]leaf/[P]leaf with elevation increase was mainly caused by significantly increasing [N]leaf rather than by decreasing [P]leaf in the study area.
Other factors impacting leaf ecological stoichiometry of Potentilla anserina
According to the SEM results, the environmental factors in the present study can only account for part of the total variation of leaf ecological stoichiometry of P. anserina (Figure 3). This indicates the possibility of effects of other factors, such as inheritance and genetic mutations of species (Luo et al., 2006), vegetation community structure and composition (Wang et al., 2014; Zhu et al., 2020), light (Zhu et al., 2020), soil microbial activity (Li et al., 2014), and intra- and inter-species competitions (Xu et al., 2015; Qin et al., 2016). As P. anserina is a pioneer species of degraded grassland, grassland management also needs to be taken into consideration in future research to fully understand the leaf ecological stoichiometry changes of P. anserina and provide basic information for ecological restoration in this region.
Nutrient limitation for Potentilla anserina growth across elevation
Due to the limited natural supply, N and P were the two most important elements to limit plant growth and functioning in a terrestrial ecosystem (Elser et al., 2000). Compared with the individual [N]leaf and [P]leaf, [N]leaf/[P]leaf was more reliable to identify plants' nutrient limitations (Li et al., 2018), although the thresholds were still controversial. In the present study area, the average [N]leaf/[P]leaf (32.86) was significantly higher than that of global and Chinese vegetations (11.8 and 16.3, respectively) (Reich and Oleksyn, 2004; Han et al., 2005). This suggests that P. anserina was more P-limited than the averages of global and Chinese plants. According to the study of Güsewell (2004) on terrestrial plant communities, when [N]leaf/[P]leaf < 10, plants growth was limited by N; 10 ≤ [N]leaf/[P]leaf ≤ 20, plant growth was co-limited by N and P; and [N]leaf/[P]leaf > 20, plant growth was limited by P. In the study area, the [N]leaf/[P]leaf of P. anserina from 2,600 to 3,800 m was all > 20 and at 2,400 m was 10.05 (Table 3), suggesting that the growth of P. anserina was limited by P from 2,600 to 3,800 m and by both N and P at 2,400 m.
With increasing elevation, [N]leaf/[P]leaf showed an increasing trend, indicating that the higher the elevation, the more severe the restriction of P element to the growth of P. anserina. This may be related to the decrease in MAT and soil pH and the increase in MAP (Tables 3, 4). Previous studies indicated that soil P deficiency was an important reason for P-limitation in plants in China (e.g., Han et al., 2005; Hu et al., 2017) and in the present study area (Cao et al., 2020; Qin et al., 2022). This also suggested that taking measures to increase soil available P and reduce P losses is of great importance for ecological restoration in the Qilian Mountains as well as in China.
Conclusion
According to the results of field sampling and experimental analysis, [C]leaf, [N]leaf, [P]leaf, and the stoichiometric ratios of P. anserina fluctuated with elevation in the middle eastern part of the Qilian Mountains: from 2,400 to 3,800 m, [C]leaf, [P]leaf, and [C]leaf/[N]leaf decreased, while [N]leaf and [N]leaf/[P]leaf increased. Elevation played a significant role in the leaf ecological stoichiometry of P. anserina through the effects of MAT, MAP, and soil pH. Based on [N]leaf/[P]leaf inference, P element was the restrictive resource that affected P. anserina growth in the study area, and it was particularly deficient with the increase in elevation.
As P. anserina is a useful species for restoring degraded grasslands, it is of great importance to increase soil available P and reduce P losses for the growth of P. anserina, especially at higher elevations. Due to the relatively small scale of the present study, further studies in the Qilian Mountains will ensure wider applicability of the observations in other areas.
Data availability statement
The original contributions presented in the study are included in the article/supplementary material, further inquiries can be directed to the corresponding author.
Author contributions
XZ, QF, and JC were involved in the conception and design of the research. XZ and HS were involved in the acquisition of data and drafted the manuscript. XZ and YQ analyzed and interpreted the data. XZ and WL performed the statistical analysis. AB, JC, and MZ were involved in the revision of the manuscript for important intellectual content. All authors have read and approved the final manuscript.
Funding
This work was supported by the National Natural Science Foundation of China (Grant No. 52179026), the Key R&D Program of Gansu Province, China (Grant No. 20YF8FA002), and the XPCC Science and Technique Foundation (Grant No. 2021AB021).
Conflict of interest
The authors declare that the research was conducted in the absence of any commercial or financial relationships that could be construed as a potential conflict of interest.
Publisher's note
All claims expressed in this article are solely those of the authors and do not necessarily represent those of their affiliated organizations, or those of the publisher, the editors and the reviewers. Any product that may be evaluated in this article, or claim that may be made by its manufacturer, is not guaranteed or endorsed by the publisher.
References
Adamidis, G. C., Kazakou, E., Fyllas, N. M., and Dimitrakopoulos, P. G. (2014). Species adaptive strategies and leaf economic relationships across serpentine and non-serpentine habitats on Lesbos, eastern Mediterranean. PLoS ONE 9, e96034. doi: 10.1371/journal.pone.0096034
Baxter, I., and Dilkes, B. P. (2012). Elemental profiles reflect plant adaptations to the environment. Science 336, 1661–1663. doi: 10.1126/science.1219992
Bo, F., Zhang, Y., Chen, H. Y. H., Wang, P., Ren, X., and Guo, J. (2020). The C:N:P stoichiometry of planted and natural larix principis-rupprechtii stands along altitudinal gradients on the Loess Plateau, China. Forests 11, 363. doi: 10.3390/f11040363
Bremmer, J. M., and Mulvaney, C. S. (1982). “Nitrogen-total,” in Methods of Soil Analysis in Part 2-Chemical and Biochemical Methods, eds H. R. Miller and D. R. Keeney (Madison, WI: American Society of Agronomy-Soil Science Society of America), 595–624.
Cao, J., Wang, X., Adamowski, J. F., Biswas, A., Liu, C., Chang, Z., et al. (2020). Response of leaf stoichiometry of Oxytropis ochrocephala to elevation and slope aspect. Catena 194, 104772. doi: 10.1016/j.catena.2020.104772
Chen, D. M., Lan, Z. C., Bai, X., Grace, J. B., and Bai, Y. F. (2013). Evidence that acidification-induced declines in plant diversity and productivity are mediated by changes in below-ground communities and soil properties in a semi-arid steppe. J. Ecol. 101, 1322–1334. doi: 10.1111/1365-2745.12119
Chen, Y. H., Han, W. X., Tang, L. Y., Tang, Z. Y., and Fang, J. Y. (2013). Leaf nitrogen and phosphorus concentrations of woody plants differ in responses to climate, soil and plant growth form. Ecography 36, 178–184. doi: 10.1111/j.1600-0587.2011.06833.x
Cheng, J., Liu, D., Zhao, L., Zhao, Q., Zhang, X., Wang, B., et al. (2021). Potentilla anserine L. polysaccharide inhibits cadmium-induced neurotoxicity by attenuating autophagy. Neurochem. Int. 147, 105045. doi: 10.1016/j.neuint.2021.105045
Croft, H., Chen, J., Luo, X., Bartlett, P., Chen, B., and Staebler, R. M. (2017). Leaf chlorophyll content as a proxy for leaf photosynthetic capacity. Glob. Chang. Biol. 23, 3513–3524. doi: 10.1111/gcb.13599
Crowley, K. F., Mcneil, B. E., Lovett, G. M., Canham, C. D., Driscoll, C. T., Rustad, L. E., et al. (2012). Do nutrient limitation patterns shift from nitrogen toward phosphorus with increasing nitrogen deposition across the northeastern united states?. Ecosystems 15, 940–957. doi: 10.1007/s10021-012-9550-2
Du, B. M., Ji, H. W., Peng, C., Liu, X. J., and Liu, C. J. (2017). Altitudinal patterns of leaf stoichiometry and nutrient resorption in quercus variabilis in the Baotianman mountains, China. Plant Soil 413, 193–202. doi: 10.1007/s11104-016-3093-9
Elser, J. J., Acharya, K., and Kyle, M. (2003). Growth rate-stoichiometry couplings in diverse biota. Eco. Lett. 6, 936–943. doi: 10.1046/j.1461-0248.2003.00518.x
Elser, J. J., Fagan, W. F., Denno, R. F., Dobberfuhl, D. R., Folarin, A., Huberty, A. F., et al. (2000). Nutritional constraints in terrestrial and freshwater food webs. Nature 408, 578–580. doi: 10.1038/35046058
Elser, J. J., Fagan, W. F., Kerkhoff, A. J., Swenson, N. G., and Enquist, B. J. (2010). Biological stoichiometry of plant production: metabolism, scaling and ecological response to global change. New Phytol. 186, 593–608. doi: 10.1111/j.1469-8137.2010.03214.x
Fang, Z., Li, D., Jiao, F., Yao, J., and Du, H. (2019). The latitudinal patterns of leaf and soil C:N:P stoichiometry in the Loess Plateau of China. Front. Plant Sci. 10, 85. doi: 10.3389/fpls.2019.00085
Gong, X., Xu, Z., Lu, W., Tian, Y., Liu, Y., Wang, Z., et al. (2018). Spatial patterns of leaf carbon, nitrogen, and phosphorus stoichiometry of aquatic macrophytes in the arid zone of northwestern China. Front. Plant Sci. 9, 1398. doi: 10.3389/fpls.2018.01398
Gui, J., Li, Z., Feng, Q., Yuan, R., Ning, T., Zhang, B., et al. (2020). Environmental effect and spatiotemporal pattern of stable isotopes in precipitation on the transition zone between the Tibetan Plateau and arid region. Sci. Total Environ. 749, 141559. doi: 10.1016/j.scitotenv.2020.141559
Guo, Q., Li, H., and Zhang, W. (2016). Variations in leaf functional traits and physiological characteristics of Abies georgei var. smithii along the altitude gradient in the Southeastern Tibetan Plateau. J. Mount. Sci. 13, 1818–1828. doi: 10.1007/s11629-015-3715-3
Guo, X., Shi, P., Niinemets, Ü., Hölscher, D., Wang, R., Liu, M., et al. (2021). “Diminishing returns” for leaves of five age-groups of Phyllostachys edulis culms. Am. J. Bot. 108, 1662–1672. doi: 10.1002/ajb2.1738
Güsewell, S. (2004). N: P ratios in terrestrial plants: variation and functional significance. New Phytol. 164, 243–266. doi: 10.1111/j.1469-8137.2004.01192.x
Hall, E. K., Singer, G. A., Kainz, M. J., and Lennon, J. T. (2010). Evidence for a temperature acclimation mechanism in bacteria: an empirical test of a membrane-mediated trade-off. Funct. Ecol. 24, 898–908. doi: 10.1111/j.1365-2435.2010.01707.x
Han, W. X., Fang, J. Y., Guo, D. L., and Zhang, Y. (2005). Leaf nitrogen and phosphorus stoichiometry across 753 terrestrial plant species in China. New Phytol. 168, 377–385. doi: 10.1111/j.1469-8137.2005.01530.x
Han, W. X., Fang, J. Y., Reich, P. B., Woodward, F. I., and Wang, Z. H. (2011). Biogeography and variability of eleven mineral elements in plant leaves across gradients of climate, soil and plant functional type in China. Ecol. Lett. 14, 788–796. doi: 10.1111/j.1461-0248.2011.01641.x
Haynes, R. J. (ed.)., (1986). “The decomposition process: mineralization, immobilization, humus formation, and degradation,” in Mineral Nitrogen in the Plant-Soil System (London: Academic Press), 52–126. doi: 10.1016/B978-0-12-334910-1.50006-6
He, L., Liu, N., Wang, K., Zhang, L., Li, D., Wang, Z., et al. (2021). Rosamultin from Potentilla anserine L. exhibits nephroprotection and antioxidant activity by regulating the reactive oxygen species/C/EBP homologous protein signaling pathway. Phytother. Res. 35, 6343–6358. doi: 10.1002/ptr.7285
He, Y., Chen, Y., Yu, C. L., Lu, K. X., Jiang, Q. S., Fu, J. L., et al. (2016). Photosynthesis and yield traits in different soybean lines in response to salt stress. Photosynthetica 54, 630–635. doi: 10.1007/s11099-016-0217-7
Hu, Q., Sheng, M., Bai, Y., Jie, Y., and Xiao, H. (2020). Response of C, N, and P stoichiometry characteristics of Broussonetia papyrifera to altitude gradients and soil nutrients in the karst rocky ecosystem, SW China. Plant Soil 475, 123–136. doi: 10.1007/s11104-020-04742-7
Hu, Y. K., Zhang, Y. L., Liu, G. F., Xu, P., and Ming, D. (2017). Intraspecific N and P stoichiometry of Phragmites australis: geographic patterns and variation among climatic regions. Sci Rep. 7, 43018. doi: 10.1038/srep43018
IUSS Working Group World Reference Base for Soil Resources (2014). 2014 International Soil Classification System for Naming Soils and Creating Legends for Soil Maps. Rome: FAO.
Jiang, L., He, Z., Liu, J., Xing, C., Gu, X., Wei, C., et al. (2019). Elevation gradient altered soil C, N, and P stoichiometry of pinus taiwanensis forest on Daiyun mountain. Forests 10, 1089. doi: 10.3390/f10121089
Koerselman, W., and Meuleman, A. M. F. (1996). The vegetation N:P ratio: a new tool to detect the nature of nutrient limitation. J. Appl. Ecol. 33, 1441–1450. doi: 10.2307/2404783
Li, F., Hu, J., Xie, Y., Yang, G., Hu, C., Chen, X., et al. (2018). Foliar stoichiometry of carbon, nitrogen, and phosphorus in wetland sedge Carex brevicuspis along a small-scale elevation gradient. Ecol. Indic. 92, 322–329. doi: 10.1016/j.ecolind.2017.04.059
Li, H., Ye, D. D., Wang, X. G., Settles, M. L., Wang, J., Hao, Z. Q., et al. (2014). Soil bacterial communities of different natural forest types in Northeast China. Plant Soil 1–2, 203–216. doi: 10.1007/s11104-014-2165-y
Li, L., Gao, X., Gui, D., Liu, B., Zhang, B., and Li, X. (2017). Stoichiometry in aboveground and fine roots of Seriphidium koroviniiin desert grassland in response to artificial nitrogen addition. J. Plant Res. 130, 689–697. doi: 10.1007/s10265-017-0930-8
Li, W., Huang, G., and Zhang, H. (2020). Enclosure increases nutrient resorption from senescing leaves in a subalpine pasture. Plant Soil 457, 269–278. doi: 10.1007/s11104-020-04733-8
Li, Y., He, W., Wu, J., Zhao, P., Chen, Y., Zhu, L., et al. (2021). Leaf stoichiometry is synergistically-driven by climate, site, soil characteristics and phylogeny in karst areas, Southwest China. Biogeochemistry 155, 283–301. doi: 10.1007/s10533-021-00826-3
Li, Y., Shi, P., Niinemets, Ü., Song, Y., Yu, K., Schrader, J., et al. (2022a). Diminishing returns among lamina fresh and dry mass, surface area, and petiole fresh mass among nine Lauraceae species. Am. J. Bot. 109, 377–392. doi: 10.1002/ajb2.1812
Li, Y., Zheng, Y., Ratkowsky, D. A., Wei, H., and Shi, P. (2022b). Application of an ovate leaf shape model to evaluate leaf bilateral asymmetry and calculate lamina centroid location. Front. Plant Sci. 2, 822907. doi: 10.3389/fpls.2021.822907
Lin, Y., Lai, Y., Tang, S., and Qin, Z. (2022). Climatic and edaphic variables determine leaf C, N, P stoichiometry of deciduous Quercus species. Plant Soil. 474, 383–394. doi: 10.21203/rs.3.rs-900936/v1
Liu, D., Zheng, D., Xu, Y., Chen, Y., Wang, H., Wang, K., et al. (2021). Changes in the stoichiometry of Castanopsis fargesii along an elevation gradient in a Chinese subtropical forest. PeerJ 9, e11553. doi: 10.7717/peerj.11553
Liu, J. T., Gu, Z. J., Shao, H. B., Zhou, F., and Peng, S. Y. (2016). N-P stoichiometry in soil and leaves of Pinus massoniana forest at different stand ages in the subtropical soil erosion area of China. Environ. Earth Sci. 75, 1901. doi: 10.1007/s12665-016-5888-7
Liu, J. X., Fang, X., Tang, X. L., Wang, W. T., Zhou, G. Y., and Xu, S. (2019). Patterns and controlling factors of plant nitrogen and phosphorus stoichiometry across China's forests. Biogeochemistry 143, 191–205. doi: 10.1007/s10533-019-00556-7
Liu, M., Shen, Y., Li, Q., Xiao, W., and Song, X. (2021). Arbuscular mycorrhizal fungal colonization and soil pH induced by nitrogen and phosphorus additions affects leaf C:N:P stoichiometry in Chinese fir (Cunninghamia lanceolata) forests. Plant Soil 461, 421–440. doi: 10.1007/s11104-021-04831-1
Luo, J. X., Zang, R. G., and Li, C. Y. (2006). Physiological and morphological variations of Picea asperata populations originating from different altitudes in the mountains of southwestern. For. Ecol. Manag. 221, 285–290. doi: 10.1016/j.foreco.2005.10.004
Macek, P., Macková, J., and de Bello, F. (2009). Morphological and ecophysiological traits shaping altitudinal distribution of three polylepis treeline species in the dry tropical Andes. Acta Oecol. 35, 778–785. doi: 10.1016/j.actao.2009.08.013
Mao, R., Chen, H. M., Zhang, X. H., Shi, F., and Song, C. (2016). Effects of P addition on plant C: N: P stoichiometry in an N-limited temperate wetland of Northeast China. Sci. Total Environ. 559, 1–6. doi: 10.1016/j.scitotenv.2016.03.158
Marschner, H., and Marschner, P. (2012). Marschner's Mineral Nutrition of Higher Plants. London: Academic Press.
McGroddy, M. E., Daufresne, T., and Hedin, L. O. (2004). Scaling of C: N: P stoichiometry in forests worldwide: implications of terrestrial redfield-type ratios. Ecology 85, 2390–2401. doi: 10.1890/03-0351
Moe, S. J., Stelzer, R. S., Forman, M. R., Harpole, S. W., Daufresne, T., and Yoshida, T. (2005). Recent advances in ecological stoichiometry: insights for population and community ecology. Oikos 109, 29–39. doi: 10.1111/j.0030-1299.2005.14056.x
Müller, M., Oelmann, Y., Schickhoff, U., Böhner, J., and Scholten, T. (2017). Himalayan treeline soil and foliar C:N:P stoichiometry indicate nutrient shortage with elevation. Geoderma 291, 21–32. doi: 10.1016/j.geoderma.2016.12.015
Nelson, D. W., and Sommers, L. E. (1982). “Total carbon, organic carbon, and organic matter,” in Methods of Soil Analysis: Part 3 Chemical Methods, eds D. L. Sparks, A. L. Page, P. A. Helmke, R. H. Loeppert, P. N. Soltanpour, M. A. Tabatabai, C. T. Johnston, and M. E. Sumner (Madison, WI: American Society of Agronomy), 539–579. doi: 10.2136/sssabookser5.3.c34
Niu, Y., Kang, J., Su, H., Adamowski, J. F., Biswas, A., Liu, C., et al. (2021). Elevation alone alters leaf N and leaf C to N ratio of Picea Crassifolia kom. in China's Qilian Mountains. Forests 12, 1325. doi: 10.3390/f12101325
Olsen, S. R., and Sommers, L. E. (1982). “Phosphorus, chemical and microbiological properties,” in Methods of Soil Analysis, eds R. H. Miller and D.R. Keeney (Madison, WI: American Society of Agronomy), 403–430.
Öquist, G. (1983). Effects of low temperature on photosynthesis. Plant Cell Environ. 6, 281–300. doi: 10.1111/1365-3040.ep11612087
Pan, F., Zhang, W., Liu, S., Li, D. J., and Wang, K. L. (2015). Leaf N:P stoichiometry across plant functional groups in the karst region of southwestern China. Trees 29, 883–892. doi: 10.1007/s00468-015-1170-y
Park, J. H., and Day, T. A. (2007). Temperature response of CO2 exchange and dissolved organic carbon release in a maritime Antarctic tundra ecosystem. Polar Biol. 30, 1535–1544. doi: 10.1007/s00300-007-0314-y
Patrick, L. D., Ogle, K., Bell, C. W., Zak, J., and Tissue, D. (2009). Physiological responses of two contrasting desert plant species to precipitation variability are differentially regulated by soil moisture and nitrogen dynamics. Glob. Change Biol. 15, 1214–1229. doi: 10.1111/j.1365-2486.2008.01750.x
Persson, I. L., Julkunen-Tiitto, R., Bergström, R., Wallgren, M., Suominen, O., and Danell, K. (2012). Simulated moose (Alces alces L.) browsing increases accumulation of secondary metabolites in bilberry (Vaccinium myrtillus L.) along gradients of habitat productivity and solar radiation. J. Chem. Ecol. 38, 1225–1234. doi: 10.1007/s10886-012-0209-4
Pontes, D. S. L., Louault, F., Carrère, P., Maire, V., Andueza, D., and Soussana, J. (2010). The Role of Plant Traits and Their Plasticity in the Response of Pasture Grasses to Nutrients and Cutting Frequency. London: Oxford University Press.
Qiao, Y., Yang, W., Zhao, Y., Jeelani, N., Xu, L., Zhao, H., et al. (2018). How Spartina alterniflora adapts to a new environment created by embankment reclamation through C-N-P stoichiometry in the coastal wetlands of eastern China. Mar. Freshw. Res. 69, 823–832. doi: 10.1071/MF17374
Qin, J., Xi, W., Rahmlow, A., Kong, H., Zhang, Z., and Shangguan, Z. (2016). Effects of forest plantation types on leaf traits of Ulmus pumila and Robinia pseudoacacia on the Loess Plateau, China. Ecol. Eng. 97, 416–425. doi: 10.1016/j.ecoleng.2016.10.038
Qin, Y., Feng, Q., Adamowski, J. F., Zhu, M., and Zhang, X. (2021). Community level response of leaf stoichiometry to slope aspect in a montane environment: a case study from the Central Qilian Mountains, China. Glob. Ecol. Conserv. 28, e01703. doi: 10.1016/j.gecco.2021.e01703
Qin, Y., Liu, W., Zhang, X., Adamowski, J. F., and Biswas, A. (2022). Leaf stoichiometry of Potentilla fruticosa across elevations in China's Qilian Mountains. Front. Plant Sci. 13, 814059. doi: 10.3389/fpls.2022.814059
Reich, P. B. (2005). Global biogeography of plant chemistry: filling in the blanks. New Phytol. 168, 263–266. doi: 10.1111/j.1469-8137.2005.01562.x
Reich, P. B., and Oleksyn, J. (2004). Global patterns of plant leaf N and P in relation to temperature and latitude. Proc. Natl. Acad. Sci. U.S.A. 101, 11001–11006. doi: 10.1073/pnas.0403588101
Rong, Q., Liu, J., Cai, Y., Lu, Z., Zhao, Z., Yue, W., et al. (2015). Leaf carbon, nitrogen and phosphorus stoichiometry of Tamarix chinensis Lour. in the Laizhou Bay coastal wetland, China. Ecol Eng. 76, 57–65. doi: 10.1016/j.ecoleng.2014.03.002
Sardans, J., Rivas-Ubach, A., and Penuelas, J. (2011). Factors affecting nutrient concentration and stoichiometry of forest trees in Catalonia (NE Spain). For. Ecol. Manag. 262, 2024–2034. doi: 10.1016/j.foreco.2011.08.019
Sardans, J., Rodà, F., and Peñuelas, J. (2005). Effects of water and a nutrient pulse supply on rosmarinus officinalis growth, nutrient content and flowering in the field. Environ. Exp. Bot. 53, 1–11. doi: 10.1016/j.envexpbot.2004.02.007
Sheng, H., Li, J., Yang, Y., and Li, H. (2004). Response of phenotypic plasticity of Potentilla anserine L. to soil moisture. Agric. Res. Arid Areas 3, 119–122.
Shi, P., Li, Y., Hui, C., Ratkowsky, D. A., Yu, X., and Niinemets, Ü. (2020). Does the law of diminishing returns in leaf scaling apply to vines?—Evidence from 12 species of climbing plants. Glob. Ecol. Conserv. 21, e00830. doi: 10.1016/j.gecco.2019.e00830
Shi, P., Miao, Q., Niinemets, Ü., Liu, M., Li, Y., Yu, K., et al. (2022). Scaling relationships of leaf vein and areole traits versus leaf size for nine Magnoliaceae species differing in venation density. Am. J. Bot. 109, 899–909. doi: 10.1002/ajb2.1856
Sistla, S. A., and Schimel, J. P. (2012). Stoichiometric flexibility as a regulator of carbon and nutrient cycling in terrestrial ecosystems under change. New Phytol. 196, 68–78. doi: 10.1111/j.1469-8137.2012.04234.x
Su, H., Cui, J., Adamowski, J. F., Zhang, X., Biswas, A., and Cao, J. (2022). Using leaf ecological stoichiometry to direct the management of Ligularia virgaurea on the Northeast Qinghai-Tibetan plateau. Front. Environ. Sci. 9, 805405. doi: 10.3389/fenvs.2021.805405
Suding, K. N., Lavorel, S., Chapin, F. S., Cornelissen, J. H. C., Díaz, S., Garnier, E., et al. (2008). Scaling environmental change through the community-level: a trait-based response-and-effect framework for plants. Glob. Change Biol. 14, 1125–1140. doi: 10.1111/j.1365-2486.2008.01557.x
Sun, L., Zhang, B., Wang, B., Zhang, G., Zhang, W., Zhang, B., et al. (2017). Leaf elemental stoichiometry of Tamarix Lour. species in relation to geographic, climatic, soil, and genetic components in China. Ecol. Eng. 106, 448–457. doi: 10.1016/j.ecoleng.2017.06.018
Tao, Y., Wu, G. L., Zhang, Y. M., and Zhou, X. B. (2016). Leaf N and P stoichiometry of 57 plant species in the Karamori Mountain Ungulate Nature Reserve, Xinjiang. China. J. Arid Land. 8, 935–947. doi: 10.1007/s40333-016-0019-6
Tian, D., Reich, P. B., Chen, H., Xiang, Y., Luo, Y., Shen, Y., et al. (2018). Global changes alter plant multi-element stoichiometric coupling. New Phytol. 221, 807–817. doi: 10.1111/nph.15428
Tong, R., Zhou, B., Jiang, L., Ge, X., and Cao, Y. (2021). A typical forbs in alpine plant communities. Catena 201, 105221. doi: 10.1016/j.catena.2021.105221
van de Weg, M. J., Meir, P., Grace, J., and Atkin, O. K. (2009). Altitudinal variation in leaf mass per unit area, leaf tissue density and foliar nitrogen and phosphorus content along an Amazon-Andes gradient in Peru. Plant Ecol. Divers. 2, 243–254. doi: 10.1080/17550870903518045
van Wijk, M. T., Williams, M., Gough, L., Hobbie, S. E., and Shaver, G. R. (2003). Luxury consumption of soil nutrients: a possible competitive strategy in above-ground and below-ground biomass allocation and root morphology for slow-growing arctic vegetation? J. Ecol. 91, 664–676. doi: 10.1046/j.1365-2745.2003.00788.x
Waigwa, A. N., Mwangi, B. N., Wahiti, G. R., Omengo, F., Zhou, Y., and Wang, Q. (2020). Variation of morphological and leaf stoichiometric traits of two endemic species along the elevation gradient of Mount Kenya, East Africa. J. Plant Ecol. 13, 785–792. doi: 10.1093/jpe/rtaa067
Wang, F., Zhang, F., Guo, X., Zhang, J., Wang, Y., and Niu, H. (2019). Spatial and temporal variation of leaf nitrogen and phosphorus stoichiometry of Qilian juniper (Sabina przewalskii) in the middle section of the Qilian Mountains, northwest China. J. Lanzhou Univer. 55, 525–532. doi: 10.13885/j.issn.0455-2059.2019.04.016
Wang, W., Cai, Y. X., Yang, J. C., and Zhu, Q. S. (2011). Effects of soil water deficit on physiological causes of rice grain-filling. Chin. J. Plant Ecol. 35, 195–202. doi: 10.3724/SP.J.1258.2011.00195
Wang, W., He, Z., Du, J., Ma, D., and Zhao, P. (2022). Altitudinal patterns of species richness and flowering phenology in herbaceous community in Qilian Mountains of China. Int. J. Biometeorol. 66, 741–751. doi: 10.1007/s00484-021-02233-7
Wang, W. Q., Sardans, J., Wang, C., Zeng, C., Tong, C., Asensio, D., et al. (2015). Ecological stoichiometry of C, N, and P of invasive phragmites australis and native Cyperus malaccensis species in the Minjiang river tidal estuarine wetlands of China. Plant Ecol. 216, 809–822. doi: 10.1007/s11258-015-0469-5
Wang, X. G., Lü, X. T., and Han, X. G. (2014). Responses of nutrient concentrations and stoichiometry of senesced leaves in dominant plants to nitrogen addition and prescribed burning in a temperate steppe. Ecol. Eng. 70, 154–161. doi: 10.1016/j.ecoleng.2014.05.015
Weidner, S., Koller, R., Latz, E., Kowalchuk, G., Bonkowski, M., Scheu, S., et al. (2015). Bacterial diversity amplifies nutrient-based plant-soil feedbacks. Funct. Ecol. 29, 1341–1349. doi: 10.1111/1365-2435.12445
Weiher, E., and Keddy, P. A. (1999). Ecological Assembly Rules: Perspectives, Advances, Retreats. Cambridge: Cambridge University Press. doi: 10.1017/CBO9780511542237
Xu, S., Fan, X., Wang, L., Zhang, X., and An, L. (2015). The patterns of nitrogen and phosphorus stoichiometry across communities along altitudinal gradients in Qilian Mountains, China. Biochem. Syst. Ecol. 62, 58–65. doi: 10.1016/j.bse.2015.07.037
Xu, X. Y., Qin, Y. Y., and Cao, J. J. (2019). Variation characteristics of Potentilla bifurca leaf stoichiometry along the elevation gradient on the northeastern Qinghai-Tibetan Plateau. Acta Ecol. Sin. 39, 9041–9055. doi: 10.5846/stxb201805231128
Xu, X. Y., Qin, Y. Y., Cao, J. J., Li, M. T., Gong, Y. F., and Zhang, X. F. (2018). Elevational variations of leaf stochiometry in Leontopodium leontopodioides on the Qinghai-Tibetan Plateau, China. Chin. J. Appl. Ecol. 29, 3934–3940. doi: 10.13287/j.1001-9332.201812.021
Yan, W., Zhong, Y., Zheng, S., and Shangguan, Z. (2016). Linking plant leaf nutrients/stoichiometry to water use efficiency on the Loess Plateau in China. Ecol. Eng. 87, 124–131. doi: 10.1016/j.ecoleng.2015.11.034
Yang, D., Song, L., and Jin, G. (2019). The soil C:N:P stoichiometry is more sensitive than the leaf C:N:P stoichiometry to nitrogen addition: a four-year nitrogen addition experiment in a Pinus koraiensis plantation. Plant Soil 442, 183–198. doi: 10.1007/s11104-019-04165-z
Yu, M., Wang, Y., Jiang, J., Wang, C., Zhou, G., and Yan, J. (2019). Soil organic carbon stabilization in the three subtropical forests: importance of clay and metal oxides. J. Geophys. Res. Biogeo. 124, 2976–2990. doi: 10.1029/2018JG004995
Yuan, Z., and Chen, H. (2015). Decoupling of nitrogen and phosphorus in terrestrial plants associated with global changes. Nat. Clim. Change 5, 465–469. doi: 10.1038/nclimate2549
Zeng, Q., Xin, L., Dong, Y., An, S., and Darboux, F. (2016). Soil and plant components ecological stoichiometry in four steppe communities in the Loess Plateau of China. Catena 147, 481–488. doi: 10.1016/j.catena.2016.07.047
Zhang, J., He, N., Liu, C., Xu, L., Chen, Z., Li, Y., et al. (2020). Variation and evolution of C:N ratio among different organs enable plants to adapt to N-limited environments. Glob. Change Biol. 26, 2534–2543. doi: 10.1111/gcb.14973
Zhang, Q., Liu, Q., Yin, H., Zhao, C., Zhang, L., Zhou, G., et al. (2019). C:N:P stoichiometry of Ericaceae species in shrubland biomes across Southern China: influences of climate, soil and species identity. J. Plant Ecol. 12, 346–357. doi: 10.1093/jpe/rty033
Zhang, Y., Li, C., and Wang, M. (2019). Linkages of C: N: P stoichiometry between soil and leaf and their response to climatic factors along altitudinal gradients. J. Soils Sediments 19, 1820–1829. doi: 10.1007/s11368-018-2173-2
Zhao, B., Tao, F., Wang, J., and Zhang, J. (2020). The sulfated modification and antioxidative activity of polysaccharides from Potentilla anserine L. New J. Chem. 44, 4726–4735. doi: 10.1039/D0NJ00356E
Zhao, C. Y., Nan, Z. R., and Cheng, G. D. (2005). Methods for modelling of temporal and spatial distribution of air temperature at landscape scale in the southern Qilian mountains. China. Ecol. Model 189, 209–220. doi: 10.1016/j.ecolmodel.2005.03.016
Zhao, C. Y., Nan, Z. R., Cheng, G. D., Zhang, J. H., and Feng, Z. D. (2006). GIS-assisted modelling of the spatial distribution of Qinghai spruce (Picea crassifolia) in the Qilian Mountains, northwestern China based on biophysical parameters. Ecol. Model 191, 487–550. doi: 10.1016/j.ecolmodel.2005.05.018
Zhao, N., He, N., Wang, Q., Zhang, X., Wang, R., and Xu, Z. (2014). The altitudinal patterns of leaf C: N: P stoichiometry are regulated by plant growth form, climate and soil on Changbai Mountain, China. PLoS ONE 9, e95196. doi: 10.1371/journal.pone.0095196
Zhao, W., Reich, P. B., Yu, Q., Zhao, N., Yin, C., Zhao, C., et al. (2018). Shrub type dominates the vertical distribution of leaf C : N : P stoichiometry across an extensive altitudinal gradient. Biogeosciences 15, 2033–2053. doi: 10.5194/bg-15-2033-2018
Zhu, D., Hui, D., Wang, M., Yang, Q., and Yu, S. (2020). Light and competition alter leaf stoichiometry of introduced species and native mangrove species. Sci. Total Environ. 738, 40301. doi: 10.1016/j.scitotenv.2020.140301
Keywords: plant growth strategies, plant environmental adaptability, leaf traits, mountainous regions, nutrient limitation
Citation: Zhang X, Feng Q, Cao J, Biswas A, Su H, Liu W, Qin Y and Zhu M (2022) Response of leaf stoichiometry of Potentilla anserina to elevation in China's Qilian Mountains. Front. Plant Sci. 13:941357. doi: 10.3389/fpls.2022.941357
Received: 11 May 2022; Accepted: 25 August 2022;
Published: 26 September 2022.
Edited by:
Peijian Shi, Nanjing Forestry University, ChinaReviewed by:
Xiangnan Li, Northeast Institute of Geography and Agroecology (CAS), ChinaFeng Li, Institute of Subtropical Agriculture (CAS), China
Jiahui Zhang, Institute of Geographic Sciences and Natural Resources Research (CAS), China
Copyright © 2022 Zhang, Feng, Cao, Biswas, Su, Liu, Qin and Zhu. This is an open-access article distributed under the terms of the Creative Commons Attribution License (CC BY). The use, distribution or reproduction in other forums is permitted, provided the original author(s) and the copyright owner(s) are credited and that the original publication in this journal is cited, in accordance with accepted academic practice. No use, distribution or reproduction is permitted which does not comply with these terms.
*Correspondence: Qi Feng, cWlmZW5nJiN4MDAwNDA7bHpiLmFjLmNu